- 1Istituto Nazionale di Geofisica and Vulcanologia, Rome, Italy
- 2Dipartimento di Scienze, Sezione di Scienze Geologiche, Università Roma TRE, Rome, Italy
The subduction of continental lithosphere is a complex process because the buoyancy of the crust is higher than the oceanic and should resist sinking into the mantle. Anyway, studies on the Alpine-Himalayan collision system indicate that a large portion of the continental crust is subducted, while some material is accreted in the orogens. The Apennine is a perfect case for studying how such processes evolve, thanks to high quality seismic images that illuminate a critical depth range not commonly resolved in many collisional settings. In this paper, we show the structure of the Apennines orogen, as jointly revealed by seismicity and deep structure from regional and teleseismic tomography and receiver function profiles. The westward subducting Adria lithosphere is well defined along the orogen showing a mid-crustal delamination. Seismicity within the underthrusting lower crust and velocity anomalies in the mantle wedge highlight how the subduction evolution is entangled with the liberation of fluids. The eclogitization of subducted material enhances the fluid release into the wedge, the delamination and retreat of the Adria plate. This delamination/subduction generates a coupled compression and extension system that migrates eastward following the retreat of the lithosphere, with broad sets of normal faults that invert or interfere with pre-existing compressional structures all over the roof plate. The sparseness and non-ubiquity of intermediate depth earthquakes along the subduction panel suggest that the brittle response of the subducting crust is governed by its different composition and fluid content. Therefore, the lower crust composition appears essential in conditioning the evolution of continental subduction.
1 Introduction
Continental collisions generally develop after an initial phase of oceanic subduction. The best example on Earth of such process is the collision of Eurasia with different plates that run from Gibraltar to India raising the Alpine-Himalayan belt for a length of thousands of kilometers (Molnar and Tapponier, 1975; Jackson and McKenzie, 1984; Royden, 2008; Faccenna and Becker, 2010). Some small relic subductions of oceans are spotted into this system, that is prevalently formed by the underthrusting of continental lithosphere following the closure of the puzzle of Tethysian realms (Dercourt et al., 1986; Stampfli et al., 2001). Data and models support the idea that the continental crust is partially subducted during the evolution of the orogen, as documented in the early stage of the Indian lithosphere collision in the Himalaya (Ingalls et al., 2016). A distinguishable feature is the level of coupling between the two colliding plates, which generates different shapes of belts and tectonics (Faccenna et al., 2014). The higher is the decoupling, the higher is the lithosphere loss (i.e., the removal of a small volume of lithosphere), rapid surface uplift, voluminous magmatism, and the change in crustal stress from compression to extension. These features have been used to infer a lithosphere removal in many mountain belts, such as in the Tibetan (England and Houseman, 1989) and Anatolian Plateaus (Sengor et al., 2003), the cause of which include either a localized gravity drip or a lithosphere delamination with a thermal evolution in the region of thinned lithosphere (Long et al., 2021). Following these broad scale processes, the Late Cenozoic evolution of the circum-Mediterranean orogens has been explained in terms of subduction and collision controlled by the buoyancy of the subducting lithosphere (Malinverno and Ryan, 1986; Royden and Faccenna, 2018). Decoupling of colliding plates varies along the central Mediterranean belts, being smaller in the Alps and higher in the Apennines. Underplating of crustal material and delamination-retreat (Chiarabba et al., 2014; D’Acquisto et al., 2020) have been proposed as distinct mechanisms active in the Apennines that favor the subduction of the continental lithosphere. In numerical delamination models, significant amounts of lower crustal material are subducted into the mantle, but the rheology of the lower crust and the existence of a weak layer play a critical role (Brun and Faccenna, 2008), along with viscosity and buoyancy of the lithosphere (Schott and Schmeling, 1998; Gogus and Pysklywec, 2008).
The Mediterranean area is an astonishing laboratory to investigate the continental subduction and its interaction with the fast retreat of oceanic slabs. Along the system, oceanic and continental subduction and collision of Gondwana-derived terranes with Eurasia took place since the Late Cretaceous (e.g., Dercourt et al., 1986; Stampfli and Borel, 2002; Garfunkel, 2004) and avenues of multidisciplinary studies concur to clarify this complex picture (Boschi et al., 2010; Faccenna and Becker, 2010). The today active tectonics (Figure 1) is controlled by the slow convergence between Africa and Eurasia (3–4 mm/yr), and the slab roll back of the Ionian oceanic lithosphere beneath the Calabrian and Hellenic subduction zones (Dewey et al., 1989; Jolivet and Brun, 2010; Ring et al., 2010). Relative velocities between the Aegean lithosphere and the subducting African plate are of about 4 cm/yr, while the process is slower underneath the Calabrian arc (Serpelloni et al., 2007; Reillinger and McClusky, 2011; Palano, 2015; Serpelloni et al., 2022). Seismicity of the region extends to broad areas on both sides of the Mediterranean basin, following the convergence of the two main plates (Figure 1). Opposed to the distribution of seismicity along plate boundaries, the deformation is spread over long portions of the two continents, all along the Alpine Himalayan system. Superimposed on the far field convergence, the subduction of the Hellenic and Calabrian systems dominates the deformation, coupled with backarc extension, volcanic arc migration, and plate extrusion along strike slip faults in the Anatolian block (Faccenna et al., 2003; Sengör et al., 2005). The long route of slab retreat in the two systems is well fossilized in mantle anisotropy (Lucente et al., 2006; Barruol et al., 2011; Faccenna et al., 2014). The Apennines post-collisional belt has undergone significant evolutionary changes since the Neogene, driven by an eastward migration of compressional units and foredeeps that have progressively incorporated terrains from the Adria continental margin (Bally et al., 1986; Doglioni et al., 2007; Hetenyi et al., 2007). One of the prominent characteristics of the Apennines is the spreading of uplift, extension, and heat thermal flow within its inner region following the eastward migration of compression (Barchi et al., 2001). During the Late Pliocene and Quaternary, this transition from compression to extension occurred, lead to the formation of various intermountain basins and crustal-scale normal faults (Mazzoli et al., 2000; Pucci et al., 2014). The northern Tyrrhenian back arc region has experienced stretching and is characterized by a notably high heat flow (Della Vedova et al., 2001). Furthermore, the crust in this area is relatively thin (Piana Agostinetti and Amato, 2009). The upper-plate extension (Malinverno and Ryan, 1986) and large-scale deformation have been related with discontinuous subduction and stepwise thrust-belt evolution (Royden et al., 1987). The resulting mountain range has a variable dimension and elevation, the cause of which are still debated. Given the high buoyancy of the Adria continental crust, its large-scale subduction into the mantle could be favored by a process of crustal eclogitization (i.e., the transformation of the lower crust to eclogite facies assemblages) during the underthrusting (Krystopowicz and Currie, 2013). As a response of this complex evolution, seismicity develops along the entire Apennines system, individuating a coupled pair of extension and compression along the belt and the eastern Adriatic front (Figure 2, see also Jackson and McKenzie, 1988; Chiarabba et al., 2005). In this paper, we propose an integrated picture of the Apennines structure from top to bottom, emphasizing the connection of upper mantle anomalies defined by regional and teleseismic models (Amato et al., 1993; Spakman et al., 1993; Faccenna et al., 2003; Piromallo and Morelli, 2003; Boschi et al., 2010; Giacomuzzi et al., 2012) with local ones that better resolve the crustal structure (Di Stefano et al., 2009; Di Stefano and Ciaccio, 2014; Magnoni et al., 2022; Menichelli et al., 2023). We document the relationship between deep processes and crustal deformation, revealing new keys for the seismic hazard.
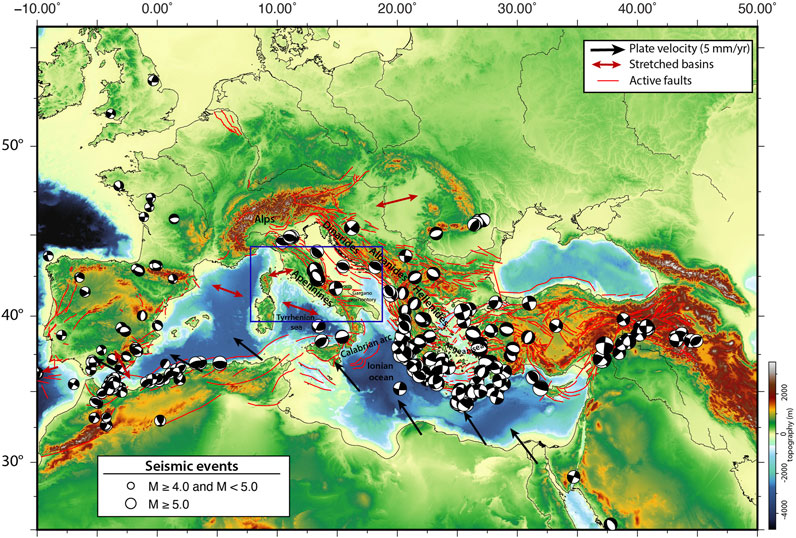
FIGURE 1. Sketch of the Mediterranean area with focal mechanisms of major earthquakes (1997–2023, extracted from the RCMT—Pondrelli et al., 2022) and main tectonic structures of the broad subduction and collisional system. Black arrows [from Serpelloni et al. (2007)] wwo indicate the velocity of plates relative to Europe and red arrows the stretching directions of backarc basins (Woudloper, https://commons.wikimedia.org/).
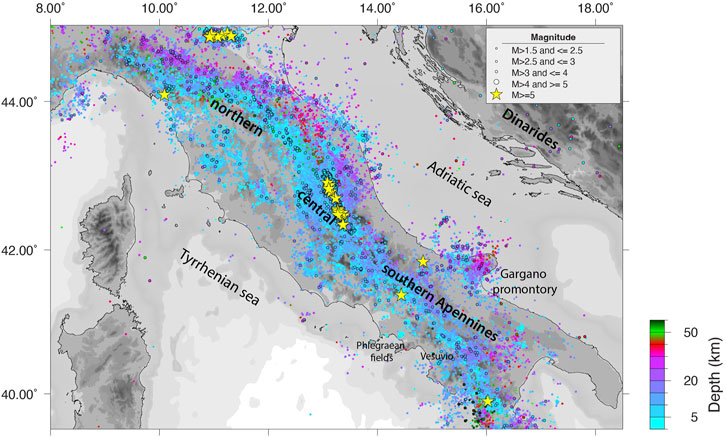
FIGURE 2. Seismicity of the Apennines as derived by relocation of seismic events (M>1.5) occurred in the past two decades (period 2005–2020). The main toponyms used in the paper are reported, NV is the Neapolitan volcanic area.
2 Materials and methods
We present here details of tomographic imaging obtained for the Apennines by local earthquakes and teleseismic data (Giacomuzzi et al., 2022; Menichelli et al., 2023). Vp, Vs, and Vp/Vs models at a regional scale were obtained by high quality hand-picked data, with a resolution of about 20–25 km in the crust and uppermost mantle. Models derive from linearized inversions of P and S arrival time data done with public teleseismic and local earthquake codes. Published models are used here jointly for the first time to get unified images of the upper 200 km of the Earth structure under the orogen. We combine a teleseismic model computed for the Apennines and resolved down to 200 km depth (Giacomuzzi et al., 2022, GM22) with a shallower model of the lithosphere recently published by Menichelli et al. (2023, MM23) bridging the gap between crustal and mantle processes.
We show a consistent image from RF (Receiver Function) profile across the belt obtained by Chiarabba et al. (2014). All these seismic images help reconstructing the sinking of the Adria lithosphere under the belt. Finally, we present seismicity maps derived from published seismic catalogs (Chiarabba et al., 2015; LaTorre et al., 2023).
3 Results
We first present and comment the structure of the Apennines system down to 200 km depth as defined by integrated tomographic models and then discuss the main pattern of seismicity and how the deformation spreads over the Adria lithosphere.
3.1 Velocity models
Vp and Vs models show high velocity anomalies between 60 and 100 km depth that highlight a first order continuity of the Adria lithosphere (Figure 3), despite the obvious different resolution of regional and teleseismic models. At shallow depth (60–65 km), the two models show low velocity anomalies in the Adriatic offshore, suggesting lateral heterogeneities in the uppermost lithospheric mantle. The difference at the same depth beneath the belt is probably related to the different resolution of the two models, with a partial vertical smearing of deeper high Vs anomalies in the teleseismic model. The integration of the two models agrees with a continuity in the continental subduction of the high velocity Adria lithosphere along the entire mountain range (Figure 3). In the peri-Tyrrhenian mantle, a broad volume of low velocity is present from 60 to 100 km depth, consistent with a hot mantle wedge in front of the Adria lithosphere.
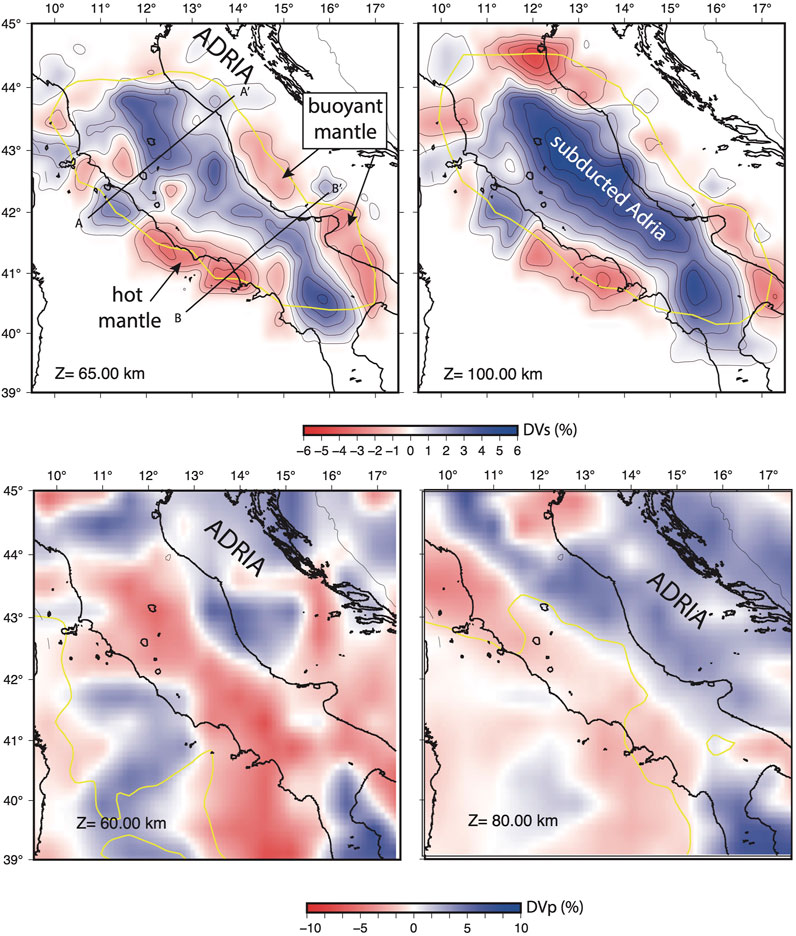
FIGURE 3. Tomographic maps of the Apennines at 65 and 100 km depth (teleseismic Vs model GM22 in top panels) and at 60–80 km depth (regional Vp model MM23 in lower panels). Yellow lines are the contour of the well resolved model volume. The low Vp anomalies mark the presence of a more buoyant and hotter mantle below the Adriatic and the Tyrrhenian coasts, respectively. Strong positive anomaly marks the subducted Adriatic plate. Traces for sections in Figure 4 are shown.
The process of continental subduction is well documented in the northern Apennines (Figure 4, section AA’), with the high velocity Adria lithosphere that deepens westward. The flexure of the lithosphere is well consistent with the decrease of velocity observed beneath the belt, indicating the subducting crustal material. The velocity model gives deep structural constraints on the role of sub-lithospheric load to flex the subducting lithosphere, proposed from analysis and modeling of the foredeep (Royden et al., 1987). The edge of delamination at 20 km depth is outlined by a spot of seismicity that indicates the point where the stripping of shallow material of the Adria lithosphere occurs. The west-dipping stripe of earthquakes is distributed within the flexed lower crust down to 70–80 km depth. The location of the positive anomaly of the Adria mantle lithosphere is fully consistent with the crustal features, indicating that the west-dipping continental subduction of Adria proceeds down to 160 km at least. A wide volume of high Vp/Vs resolved by teleseismic tomography is present beneath the belt and agrees with an intense hydration of the wedge that supports the topography of the belt and the active extension.
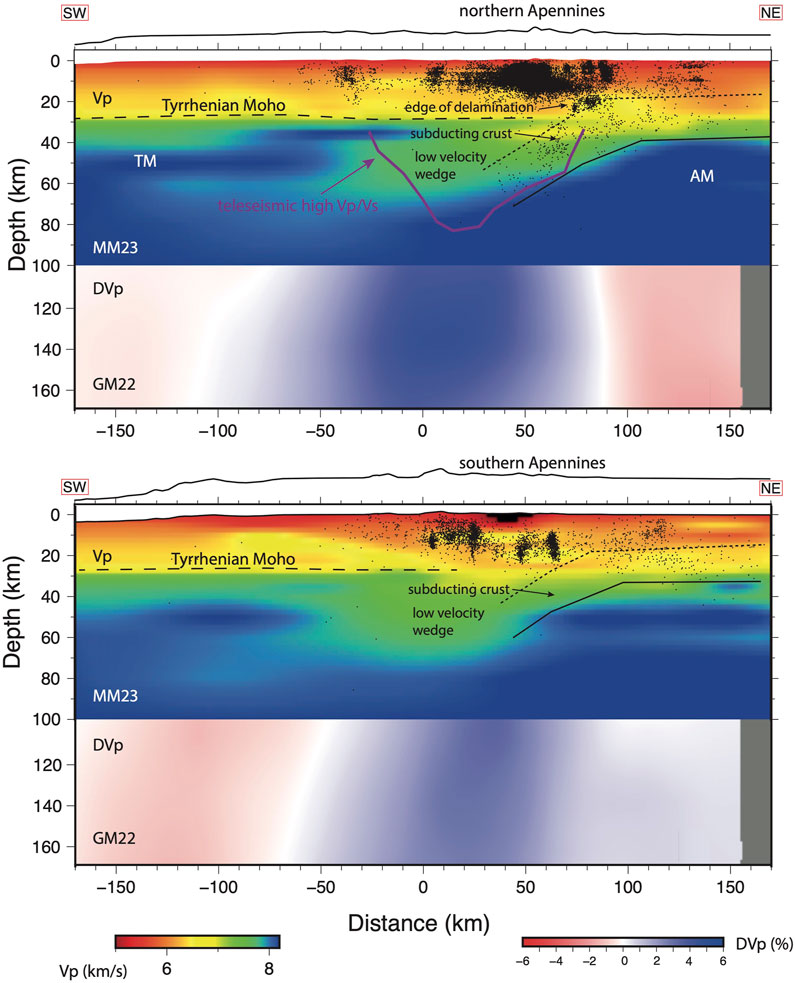
FIGURE 4. Vertical sections of Vp models from MM23 (depth 0–100 km) and a GM22 (depth 100–180 km) across northern and southern Apennines. TM and AM are the Tyrrhenian and Adria mantle, respectively. Seismicity from Chiarabba et al. (2015) was plotted to better constrain the active geodynamic processes. Dashed line is the Tyrrhenian Moho while small dashed and continuous lines indicate the middle-lower crust shear zone and the Moho of Adria. The purple line indicates the volume of high Vp/Vs observed in GM22.
The gross structure in southern Apennines is not that different. The Adria lithosphere is deflected and the connection with the deep high Vp anomaly in the mantle is consistent with that of the northern sector (Figure 4, section BB’). A broad low Vp anomaly is present beneath a wide portion of the belt, indicating that crustal rocks deepened up to the mantle during the evolution. Anyway, seismicity in the lower crust is poor or absent, as well as the high Vp/Vs in the wedge.
3.2 Seismicity
Although tectonic rates in central Mediterranean are minor than in the eastern sector, active tectonic processes generate abundant seismicity that concentrates along the Apennines. The across-belt extension of about 2–4 mm/yr spreads along the entire mountain chain (D’Agostino et al., 2014; Serpelloni et al., 2022), generating large normal faulting earthquakes, with a higher energy release in the southern portion. Compression is well defined by earthquakes with inverse fault mechanisms developing along the main arcs of northern Apennines (Chiarabba et al., 2015).
Moderate magnitude events of the past decades align on contiguous fault segments of the extensional belt (Figure 2). Seismicity is rather shallow beneath the mountain range, while it deepens to depths of 20–30 km to the east of the chain (La Torre et al., 2023). This outer belt is interrupted at about N43°, isolating a 100 km long section without seismicity, but it resumes further south in the Apulian foreland and continues up to the limit with the Calabrian arc (Figure 2).
In the northern Apennines, seismicity concentrates within the upper 12–14 km of the crust beneath the mountain range and spread within a SW dipping layer down to 70–80 km depth (Figure 4A). At the connection between the shallow, extensional and the deeper seismicity, a cluster identifies the edge of the delamination of the Adria lithosphere. In the southern Apennines, seismicity occurs prevalently within clusters around more silent blocks (Figure 2) and down to 15–20 km depth, but deeper earthquakes like those in the northern arc are poor or absent (Figure 4B), except for a small sector at the edge of the southern Apennines (Figure 2).
4 Discussion
The subduction of continental lithosphere is a process that interests wide portions of the Alpine-Himalayan belt (Ding et al., 2003; Leech et al., 2005; Nabelek et al., 2009). Anyway, the higher buoyancy of the continental lithosphere is a potential problem for an efficient evolution. The lithosphere delamination and the eclogitization of the lower crust have been proposed as potential conditions for the penetration of the continental lithosphere into the mantle and subduction (Le Pichon and Henry, 1997; Toussaint et al., 2004; Raimbourg and Jolivet., 2007; Krystopowicz and Currie, 2013). Eclogitization could also help in the support of the high topographic elevation of mountain belts and plateaus (Bousquet et al., 1997) and in regulating the long-term convergence rate in orogens (Doin and Henry, 2001). Finding the signatures of eclogitization is not simple. The occurrence of intermediate depth earthquakes in the India lower crust (Shi et al., 2018) and the concomitance with high shear wave velocities and low Vp/Vs (Wittlinger et al., 2009) has been proposed as possible evidence. Furthermore, a key role in the metamorphic reaction is the presence of free water in the system (Hetenyi et al., 2007), feature that could be argued by a decrease of Vs values in the uppermost mantle.
4.1 Crustal eclogitization and delamination of the Apennines
Numerical modeling shows that a couple of paired compression and extension is generated by delamination beneath the external front and the inner belt, respectively (Gogus and Pysklywec, 2008). Seismo-thermo-mechanical numerical modeling shows that negative slab buoyancy broadly reproduces the coupled extensional and compressional tectonic regimes of the Northern Apennines, in terms of both stress orientations and velocities (D’Acquisto et al., 2020).
Velocity structure and seismicity indicate that the two processes are active in the Apennines belt in agreement with the evolution of decoupled collisions (Faccenda et al., 2009). First, the paired system of extension and compression expected by delamination is observed in the Apennines, at least down to 43°N of latitude. The evolution of such processes over the past 17 Myr generated a thinned lithosphere in the northern Tyrrhenian back arc, intense stretching, elevated heat flow, and melting of the crust (Rossetti et al., 1999; Della Vedova et al., 2001; Peccerillo, 2005; Lustrino et al., 2011). All these features are coherent with predictions from numerical modeling of delamination. On the same side, the intermediate depth seismicity (35–70 km depth, Figure 4) is indirect evidence for the eclogitization of the Adria lower crust, and the branching of positive pulses in RF is coherent with a thick layer of delaminated lithosphere beneath the belt (Figure 5), whose active edge is prompted by seismicity at the kink of the lithosphere (Figure 4). Furthermore, the doubling of the Moho beneath the mountain range and the shallow Moho in the inner sector (Figure 5, see Bianchi et al., 2010) also agree with predictions from numerical models. Velocity images document that the eclogitization of lower crust material is active beneath the northern Apennines coupled with a strong hydration of the mantle wedge (broad high Vp/Vs anomaly in Figure 5) and a retreat of the delamination hinge. The progressive eclogitization of the underthrusting Adria led first to a weakening of the lower crust with decoupling and exhumation of the still buoyant crust (Labrousse et al., 2010) and then a densification of granulitic material that take place along seismic and ductile deformation and metamorphism (Austhreim, 2013). The process starts at about 35–40 km depth and is almost complete at 70–80 km depth, as traced by the seismicity in the lower crust and velocity changes at depth (Figure 4A). This depth interval is consistent with PT conditions for eclogitization of the lower crust (Labrousse et al., 2010). Conversely, deep seismicity is poor and eclogitization seems slowed in the southern Apennines, reasonably associated with a stalled retreat (Figure 4). High velocities down to 160 km indicate that no plate rupture has occurred.
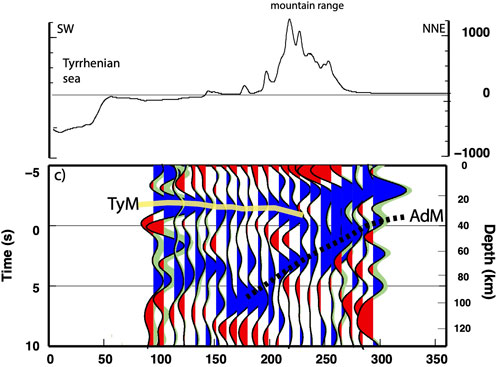
FIGURE 5. Receiver Function migration profile across Northern Apennines, modified from Chiarabba et al. (2014). TyM=Tyrrhenian Mantle, AdM=Adria mantle. Note the broadening of the high Vs (blue) pulse of the AdM at X=240–280 indicating the thickness of the crust that is undergoing eclogitization.
Evidence for a fossil process of eclogitization comes from the western Alps. Tomographic models show a distinct pattern of the subducting continental lithosphere (Menichelli et al., 2023). We observe a change from low to high Vp and low Vp/Vs at about 50–60 km depth within the European lower crust, and a broad high Vp/Vs anomaly in the Adria hydrated wedge on top of the subducting lithosphere (Figure 6). Intermediate depth earthquakes are poor or absent within the lower crust, and we hypothesize that the process is well mature and probably no more active. Anyway, the fossil example of the western Alps suggests that eclogitization, taking place during the late evolution of subductions, contributes to the generation of compositional anomalies within the continental mantle, documented here by the high Vp/Vs at the mantle top of Adria.
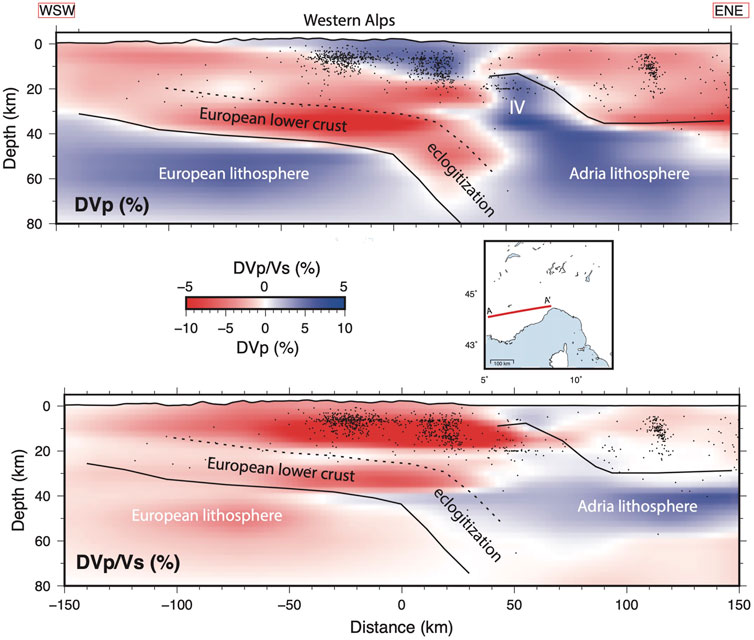
FIGURE 6. Cross-section and seismicity along the western Alps of the Vp (top panel) and Vp/Vs (bottom panel) models from MM23 highlighting the southward subduction of the European plate beneath Adria. IV= Ivrea Body. Black dots are the seismicity from the INGV catalog. Note the switch from low Vp to high Vp at 60 km depth within the subducted lithosphere and the intense high Vp/Vs anomaly in the Adria top mantle generated by the protracted eclogitization of the European lower crust.
4.2 Evolution of the Apennines subduction
The Apennines has been a repeated target of geophysical investigations for many decades (Amato et al., 1993; Spakman et al., 1993; Piromallo and Morelli, 2003; Di Stefano et al., 2009; Benoit et al., 2011; Giacomuzzi et al., 2011; Giacomuzzi et al., 2012; Giacomuzzi et al., 2022). A solid consensus exists for some gross and main features of the mantle structure, but there are still some differences that fuel the debate on contrasting models of the belt evolution. Many models reported the existence of high velocity anomalies in the uppermost mantle compatible with subducted lithosphere (Lucente et al., 1999; Piromallo and Morelli, 2003; Benoit et al., 2011; Giacomuzzi et al., 2011; Giacomuzzi et al., 2012). Models with west-dipping slab largely prevail, although the almost vertical geometry of the high velocity slab. In addition, the lack of lateral and vertical continuity of the high velocity anomalies is interpreted as possible evidence for a slab break off (Wortel and Spakman, 2000; Lucente and Margheriti, 2008) or slab window (Amato et al., 1993; Lucente et al., 1999), although the meaning of the latter remains rather ambiguous in terms of origin and evolution. More recently, Giacomuzzi et al. (2022) proposed that pre-existing compositional anomalies of the Adria continental lithosphere might explain the reduced Vp and low Vp/Vs alternatively to the slab window or slab interruptions.
Receiver function modeling yields the definition of the Adria lithosphere, which is westward plunging beneath the Apennines (Bianchi et al., 2010). Several studies focused on the modeling of the Moho geometry (Agostinetti et al., 2011; Di Stefano et al., 2011; Spada et al., 2013), and on the Lithosphere-Asthenosphere boundary depth (Miller and Agostinetti, 2012). The correlation between intermediate-depth seismicity and positive pulses in RF profiles across the chain suggests that part of the crust underthrusts the belt with the Adria lithosphere, with a flow at the nose of the wedge facilitated by slab dehydration (Agostinetti et al., 2011). The difference between the thickness of the seismic layer (∼30–35 km, LaTorre et al., 2023) and the depth of the moho (∼70 km) beneath the mountain belt is consistent with the hypothesis that seismicity and deformation of the roof plate are decoupled from the westward plunging delaminated lithosphere. Anyway, the reconstruction to greater depths and the break in the west-dipping Adria lithosphere seem to conflict with a westward-subduction scenario continuous from the Oligocene (Chiarabba et al., 2014).
The SKS anisotropy pattern suggests a differential evolution of the trench-retreat process along the Northern Apennines (Salimbeni et al., 2013; Pondrelli et al., 2022). The pattern is typical of retreat in the southern part of the Northern Apennines, with the no orogen-normal measurements found in the inner sector of the northern part in contrast to a significant pure retreat that has been interpreted as an oblique motion since around 5 Ma (Salimbeni et al., 2008). The different behavior is also evidenced by the lithosphere reconstruction that supports an irregular delamination/retreat process along the belt, with a maximum in the southern portion of northern Apennines (Chiarabba et al., 2014).
Our results show that the subduction of Adria followed the delamination of the lower crust and the underthrusting of the lithosphere beneath the orogen (Figure 4). The first order similarity in velocity structure across northern and southern Apennines indicates that the process is broadly similar for the entire Apennines belt. Anyway, difference in structural details, the discontinuous presence of deep seismicity and fluids within the wedge, and, finally, anomalies in the Adria mantle (Figures 3, 4) suggest that continental delamination is not uniform and is influenced by heterogeneities of the Adria lithosphere.
The lateral space/time heteropy of the belt might be partially controlled by difference in buoyancy of the Adria continental mantle. Such differences can be even more pronounced in continental margins, like the Adria microplate at the margin of the Neo-Tethys Ocean (Stampfli and Borel, 2002; Handy et al., 2010), where intense underplating of material occurs during the ridge formation.
The lateral varying buoyancy of the lithosphere played a key role in conditioning the subduction of the Adria lithosphere. This lateral variation in buoyancy resulted in changes in lithospheric stiffness, which in turn influenced the overall configuration of the Apennines belt. These observations match the predictions by numerical models (Lo Bue et al., 2021). Delamination is the primary process responsible for shaping the northern Apennines over the last 10 million years. However, in the southern Apennines, this process interacts with the retreat of the Ionian lithosphere beneath the southern Tyrrhenian subduction zone, and it is also influenced by the thermal and compositional characteristics of the underlying mantle. Tomographic images reveal the presence of a pronounced low-velocity (Vp) anomaly beneath southern Italy. This anomaly may arise from either an upwelling of the asthenosphere or the presence of a thermally and compositionally distinct mantle region. A compelling hypothesis to explain these observations is the varying distribution of fluids associated with the eclogitization process, which may account for the differences in the retreat rates of the two subduction arcs. The deep geometry that we have defined documents new evidence for modeling the prediction that buoyancy variations in the subducting lithosphere exert a first-order control on subduction rate, with more buoyant slab segments correlated with slower subduction rates and steeper slab dip (Royden and Husson, 2009).
4.3 Seismicity and seismic hazard
In the central Mediterranean area, seismicity distribution and strain rate from geodetic data clearly show that the deformation, confined at plate boundaries along the Alpine and Dinarides collision zones, spreads for hundreds of kilometers within the continental lithosphere of Adria (Serpelloni et al., 2022). Focusing on the Apennines (Figure 7), deformation pervaded the roof plate that consists of a mixture of continental terranes accreted during the belt formation (Bally et al., 1986). The process is not that of a coupled collision like the Alps, but the continental underthrusting of Adria concurs to edify the Apennines Mountain belt with crustal material that is peeled off and accreted in east-verging stacks. The evolution of this decoupled collision system is characterized by the disruption of the accretionary wedge and the formation of an extensional basin in the inner part of the orogen (Faccenda et al., 2009). This thinned lithosphere block (i.e., the Tyrrhenian block) has an independent motion from that of Europe and Adria (Serpelloni et al., 2022). A 2–4 mm/yr of across-belt extension characterizes the mountain range (Figure 7), paired by compression along the external front in the eastern Adriatic area. The low deformation rate is responsible for devastating earthquakes that struck the area in recent and in historical times (Rovida et al., 2022). A close look at recent large earthquakes of the Apennines (Figure 7) reveals that they prevalently clustered in the extensional and compressional deformation spines along the mountain range and the eastern Adria front, while some strike the Apulian foreland area, where strain rate is rather low. While extension is defined for almost the entire belt, compression is well defined only down to 43°N, becoming smaller or absent southward, or transferred Eastward (i.e., along the southern Croatian and Montenegro coasts (Figure 1). This observation opens a debate on whether crustal delamination is still active or if it is in an immature stage in southern Apennines.
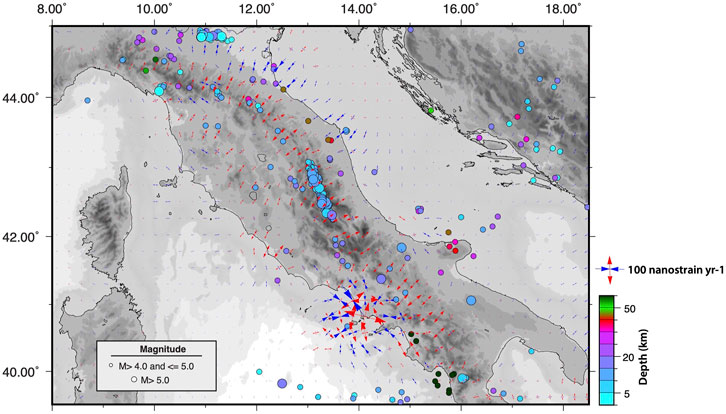
FIGURE 7. Strain rate pattern [extracted from Serpelloni et al. (2022)] and past decades seismicity (ML > 4.0) of the Apennines. Blue arrows mark the compressional field whereas the red ones the extensional one. The dots are colored according to the depth value (0–50 km). An extension deformation field is recorded along the entire mountain chain while compression occurs along the Adriatic coast of northern Apennines. Larger arrows show the deformation of the Neapolitan volcanic area.
Although the low strain rate, the completeness of the historical catalog for M>6 in the past millennium (Rovida et al. 2011) ensures a proper imaging of the long-term seismic energy release of the belt. The extension rate is almost similar between the northern and southern Apennines (Figure 7), but the energy released by historical earthquakes is higher in the south (Figure 8). Higher magnitude could be partially explainable by the greater thickness of the seismogenic layer (see Chiarabba and De Gori, 2016; LaTorre et al., 2023) and the dimension of fault segments. This feature could be consistent with the hypothesis that the energy release is controlled by the thermal state of the Apennines crust. Alternatively, we are inclined to attribute this difference to a different state of delamination, progressing from a more mature state in the north to a less advanced state in the south. The ductile deformation of the intermediate crust is notably favored by the greater maturity of the delamination process in the northern region.
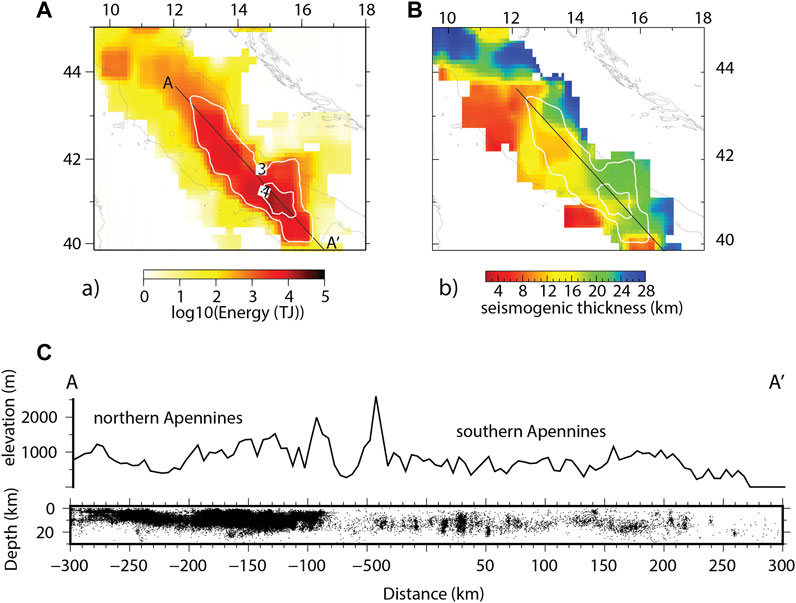
FIGURE 8. Seismic energy released by earthquakes that have occurred in the past 1 ka (A) and seismogenic thickness of the Apennines and (B), modified from Chiarabba and De Gori (2016). The white lines are the contour of high energy release. (C) Vertical section of seismicity along the Apennines belt (trace in panel a). Note the differences in thickness of the seismogenic layer and in background seismicity between the northern and southern Apennines with the presence of several locked patches in the high energy release zone of southern Apennines.
Oppositely to the higher seismic release to the south in historical times, l arge magnitude earthquakes of the past decades (Figure 6) are more clustered in the northern arc, with the impressive decade-long series of events that struck central Italy. Seismicity is sparser to the south, with M>3 earthquakes occurring within clusters that isolate blocks with low seismicity, suggesting that fault segments in this portion of the belt are locked during the interseismic period. Two regions of large strain rate and poor seismicity are present at the northern and southern tips of the segment repeatedly struck in central Italy. These two zones of the normal faulting system deserve effort and ad hoc monitoring. An ambiguous region is the Apulian foreland, where the energy released by past earthquakes is high, but the strain rate is low. This continental margin represents a transition zone between the ea stward-verging Apennines and west-verging Albanides-Hellenides. The flexural bending of the Apulia foreland is linked with E-W strike slip kinematics and extension (Doglioni et al., 1994; Argnani et al., 2001; Maesano et al., 2020). We hypothesize that the transpressive tectonics in the region can be attributed to one of two factors: either an interaction between the subduction of the Adria plate beneath the two mountain belts or an incipient delamination in the Gargano area. In the latter scenario, the stress reorientation induced by the delamination process in the Gargano area has not reached the level observed in the northern arc, where the delamination process is mature.
Several portions of the compressional belt lack consistent seismicity over the past decades, suggesting that the thrust system is either locked or only partially coupled. Earthquakes typical of the belt develop on shallow S- and SW- dipping segments forming broad arcs like for the 2012 Emilia seismic sequence (Govoni et al., 2014; Chiarabba et al., 2014). The Mw 5.7 earthquake of November 2022 ruptured a small segment of the system with the rupture of a deep portion of the thrust plane (Pezzo et al., 2023). Earthquakes like these suggest that the compressional system is highly segmented with a limited vertical and lateral continuity of faults at depth.
5 Conclusion
We propose new evidence and constraints for the evolution of the continental subduction through the delamination and eclogitization of the crust. Geophysical data give us exemplary evidence of the Adria subduction beneath the Apennines. The first order continuity of shallow and deep positive velocity anomalies does not require vertical or lateral tears within the subducted continental lithosphere and the absence of intermediate depth seismicity could only reflect a vigorous process of eclogitization and therefore of delamination. Anyway, differences in Vp and Vp/Vs anomalies observed in the uppermost mantle of Adria suggest that possible local changes in the composition are present. The delamination of the crust constitutes an almost unique scenario for which a distributed seismic hazard originates from the evolution of a continental plate.
Data availability statement
Italian seismicity data have been provided by Istituto Nazionale di Geofisica e Vulcanologia (INGV) and can retrieved in the European Integrated Data Archive (EIDA) database (http://eida.rm.ingv.it). The information about Italian Seismological Instrumental and Parametric Data-Base (ISIDe) is available at http://terremoti.ingv.it/iside. (All websites were last accessed in August 2023).
Author contributions
CC: Conceptualization, Supervision, Writing–original draft, Writing–review and editing. IM: Data curation, Formal Analysis, Investigation, Writing–original draft, Writing–review and editing. IB: Data curation, Investigation, Methodology, Software, Validation, Writing–review and editing. GG: Conceptualization, Data curation, Investigation, Methodology, Software, Supervision, Writing–review and editing. PG: Data curation, Investigation, Methodology, Software, Supervision, Writing–review and editing.
Acknowledgments
The views expressed herein are those of the author(s) and do not necessarily reflect the views of the CTBTO Preparatory Commission. Figures are generated by The Generic Mapping Tools (GMT) by Wessel et al. (2019). We thank all the INGV analysts involved in developing the BSI (Bollettino Sismico Italiano). We also thank reviewers Gianluca Vignaroli and Stephan Dominguez for comments that improved the quality of the paper.
Conflict of interest
The authors declare that the research was conducted in the absence of any commercial or financial relationships that could be construed as a potential conflict of interest.
Publisher’s note
All claims expressed in this article are solely those of the authors and do not necessarily represent those of their affiliated organizations, or those of the publisher, the editors and the reviewers. Any product that may be evaluated in this article, or claim that may be made by its manufacturer, is not guaranteed or endorsed by the publisher.
References
Agostinetti, N. P., Bianchi, I., Amato, A., and Chiarabba, C. (2011). Fluid migration in continental subduction: the Northern Apennines case study. Earth Planet. Sci. Lett. 302 (3–4), 267–278. doi:10.1016/j.epsl.2010.10.039
Amato, A., Alessandrini, B., and Cimini, G. B. (1993). in Teleseismic wave tomography of Italy, in seismic tomography: theory and practice. Editors H. M. Iyer, and K. Hirahara (London: Chapman & Hall), 361–397.
Argnani, A., Frugoni, F., Cosi, R., Ligi, M., and Favali, P. (2001). Tectonics and seismicity of the apulian ridge south of salento peninsula (southern Italy).
Austrheim, H. (2013). Fluid and deformation induced metamorphic processes around Moho beneath continent collision zones: examples from the exposed root zone of the Caledonian mountain belt, W-Norway. (2013) Tectonophys. 609, 620–635. doi:10.1016/j.tecto.2013.08.030
Bally, A. W., Burbi, L., Cooper, L., and Ghelardoni, L. (1986). Balanced sections and seismic reflection profiles across the Central Apennines. Mem. Soc. Geol. Ital. 35, 257–310.
Barchi, M., Landuzzi, A., Minelli, G., and Pialli, G. (2001). “Outer northern Apennines,” in Anatomy of an orogen: the Apennines and adjacent mediterranean basins. Editors G. B. Vai, and I. P. Martini (Dordrecht: Springer). doi:10.1007/978-94-015-9829-3
Barruol, G., Bonnin, M., Pedersen, H., Bokelmann, G. H. R., and Tiberi, C. (2011). Belt-parallel mantle-flow beneath a halted continental collision: the Western Alps,. Earth Planet. Sci. Lett. 302, 429–438. doi:10.1016/j.epsl.2010.12.040
Benoit, M. H., Torpey, M., Liszewski, K., Levin, V., and Park, J. (2011). P and S wave upper mantle seismic velocity structure beneath the northern Apennines: new evidence for the end of subduction. Geochem. Geophys. Geosyst. 12, Q06004. doi:10.1029/2010GC003428
Bianchi, I., Park, J., Agostinetti, N. P., and Levin, V. (2010). Mapping seismic anisotropy using harmonic decomposition of receiver functions: an application to Northern Apennines, Italy. Italy. J. geophys. Res. 115 (B12), B12317. doi:10.1029/2009JB007061
Boschi, L., Faccenna, C., and Becker, T. W. (2010). Mantle structure and dynamic topography in the Mediterranean Basin: MEDITERRANEAN MANTLE AND TOPOGRAPHY. Res. Lett. 37, L20303. doi:10.1029/2010GL045001
Bousquet, R., Goffé, B., Henry, P., Le Pichon, X., and Chopin, C. (1997). Kinematic, thermal and petrological model of the Central Alps: lepontine metamorphism in the upper crust and eclogitisation of the lower crust. Tectonophysics 273, 105–127. doi:10.1016/s0040-1951(96)00290-9
Brun, J.-P., and Faccenna, C. (2008). Exhumation of high-pressure rocks driven by slab rollback. Sci. Lett. 272, 1–7. doi:10.1016/j.epsl.2008.02.038
Chiarabba, C., and De Gori, P. (2016). The seismogenic thickness in Italy: constraints on potential magnitude and seismic hazard. Terra nova. 28 (6), 402–408. doi:10.1111/ter.12233
Chiarabba, C., De Gori, P., and Mele, F. M. (2015). Recent seismicity of Italy: active tectonics of the central Mediterranean region and seismicity rate changes after the Mw 6.3 L'Aquila earthquake. Tectonophysics 638, 82–93. doi:10.1016/j.tecto.2014.10.016
Chiarabba, C., Giacomuzzi, G., Bianchi, I., Piana Agostinetti, N., and Park, J. (2014). From underplating to delamination-retreat in the Northern Apennines. Earth Planet. Sci. Lett. 403, 108–116. doi:10.1016/j.epsl.2014.06.041
Chiarabba, C., Jovane, L., and DiStefano, R. (2005). A new view of Italian seismicity using 20 years of instrumental recordings. Tectonophysics 395 (3-4), 251–268. doi:10.1016/j.tecto.2004.09.013
D'Acquisto, M., Dal Zilio, L., Molinari, I., Kissling, E., Gerya, T., and van Dinther, Y. (2020). Tectonics and seismicity in the Northern Apennines driven by slab retreat and lithospheric delamination. Tectonophysics Vol. 789, 228481. doi:10.1016/j.tecto.2020.228481
D'Agostino, N., England, P., Hunstad, I., and Selvaggi, G. (2014). Gravitational potential energy and active deformation in the Apennines. Earth Planet. Sci. Lett. Vol. 397, 121–132. doi:10.1016/j.epsl.2014.04.013
Della Vedova, B., Bellani, S., Pellis, G., and Squarci, P. (2001). “Deep temperatures and surface heat flow distribution,” in Anatomy of an orogeny: the Apennines and adjacent mediterranean basins. Editors G. B. Vai, and I. P. Martini (Dordrecht: Kluwer Academic Publish- ers), 65–76.
Dercourt, J., Zonenshain, L. P., Ricou, L. E., Kazmin, V. G., Le Pichon, X., Knipper, A. L., et al. (1986). Geological evolution of the tethys belt from the atlantic to the pamirs since the lias. Tectonophysics 123, 241–315. doi:10.1016/0040-1951(86)90199-x
Dewey, J. F., Helman, M. L., Torco, E., Hutton, D. H. W., and Knott, S. D. (1989). Kinematics of the western mediterranean. Mediterr. Geol. Soc. Lond. Spec. Publ. 45, 265–283. doi:10.1144/gsl.sp.1989.045.01.15
Di Stefano, R., Bianchi, I., Ciaccio, M. G., Carrara, G., and Kissling, E. (2011). Three-dimensional Moho topography in Italy: new constraints from receiver functions and controlled source seismology. Geochem. Geophys. Geosystems 12. doi:10.1029/2011gc003649
Di Stefano, R., and Ciaccio, M. G. (2014). The lithosphere and asthenosphere system in Italy as inferred from the Vp and vs 3D velocity model and Moho map. J. Geodyn. 82, 16–25. doi:10.1016/j.jog.2014.09.006
Di Stefano, R., Kissling, E., Chiarabba, C., Amato, A., and Giardini, D. (2009). Shallow subduction beneath Italy: three-dimensional images of the Adriatic-European-Tyrrhenian lithosphere system based on high-quality P wave arrival times. J. Geophys. Res. Solid Earth 114 (5), B05305–B05317. doi:10.1029/2008JB005641
Ding, L., Kapp, P., Zhong, D., and Deng, W. (2003). Cenozoic volcanism in Tibet: evidence for a transition from oceanic to continental subduction. J. Petrol 44, 1833–1865. doi:10.1093/petrology/egg061
Doglioni, C., Carminati, E., Cuffaro, M., and Scrocca, D. (2007). Subduction kinematics and dynamic constraints. Earth Sci. Rev. 83, 125–175. doi:10.1016/j.earscirev.2007.04.001
Doglioni, C., Mongelli, F., and Pieri, P. (1994). The Puglia uplift (SE Italy): an anomaly in the foreland of the Apenninic subduction due to buckling of a thick continental lithosphere. Tectonics 13 (5), 1309–1321. doi:10.1029/94TC01501
DoinHenry, M. P. P. (2001). Subduction initiation and continental crust recycling: the roles of rheology and eclogitization. Tectonophysics 342, 163–191. doi:10.1016/S0040-1951(01)00161-5
England, P., and Houseman, G. (1989). Extension during continental convergence, with application to the Tibetan Plateau. J. Geophys. Res. 94, 17561–17579. doi:10.1029/jb094ib12p17561
Faccenda, M. (2014). Mid mantle seismic anisotropy around subduction zones. Phys. Earth Planet. Interiors 227, 1–19. doi:10.1016/j.pepi.2013.11.015
Faccenda, M., Minelli, G., and Gerya, T. (2009). Coupled and decoupled regimes of continental collision: numerical modeling. Earth Planet. Sci. Lett. 278 (3–4), 337–349. doi:10.1016/j.epsl.2008.12.021
Faccenna, C., Jolivet, L., Piromallo, C., and Morelli, A. (2003). Subduction and the depth of convection in the Mediterranean mantle. J. Geophys. Res. 108 (B2), 2099. doi:10.1029/2001jb001690
Faccenna, C., and Becker, T. W. (2010). Shaping mobile belts by small-scale convection. Nature 465, 602–605. doi:10.1038/nature09064
Faccenna, C., Becker, T. W., Auer, L., Billi, A., Boschi, L., Brun, J. P., et al. (2014). Mantle dynamics in the mediterranean. Rev. Geophys. 52, 283–332. doi:10.1002/2013RG000444
Garfunkel, Z. (2004). Origin of the eastern Mediterranean basin: a reevaluation. Tectonophysics 391 (1-4), 11–34. doi:10.1016/j.tecto.2004.07.006
Giacomuzzi, G., Chiarabba, C., and De Gori, P. (2011). Linking the Alps and Apennines subduction systems: new constraints revealed by high-resolution teleseismic tomography. Earth Planet. Sci. Lett. 301 (3–4), 531–543. doi:10.1016/j.epsl.2010.11.033
Giacomuzzi, G., Civalleri, M., De Gori, P., and Chiarabba, C. (2012). A 3D vs model of the upper mantle beneath Italy: insight on the geodynamics of central Mediterranean. Earth Planet. Sci. Lett. 335–336, 105–120. doi:10.1016/j.epsl.2012.05.004
Giacomuzzi, G., De Gori, P., and Chiarabba, C. (2022). How mantle heterogeneities drive continental subduction and magmatism in the Apennines. Sci. Rep. 12 (1), 13631. doi:10.1038/s41598-022-17715-w
Gogus, O. H., and Pysklywec, R. N. (2008). Near-surface diagnostics of dripping or delaminating lithosphere. J. Geophys. Res. 113, B11404. doi:10.1029/2007JB005123
Handy, M. R., Schmid, S. M., Bousquet, R., Kissling, E., and Bernoulli, D. (2010). Reconciling plate-tectonic reconstructions of Alpine Tethys with the geological–geophysical record of spreading and subduction in the Alps. Earth-Sci. Rev. 102 (3–4), 121–158. doi:10.1016/j.earscirev.2010.06.002
Hetenyi, G., Cattin, R., Brunet, F., Bollinger, L., Vergne, J., Nábělek, J. L., et al. (2007). Density distribution of the India plate beneath the Tibetan Plateau: geophysical and petrological constraints on the kinetics of lower-crustal eclogitization. Earth Planet. Sci. Lett. 264, 226–244. doi:10.1016/j.epsl.2007.09.036
Ingalls, M., Rowley, D., Currie, B., and Colman, A. S. (2016). Large-scale subduction of continental crust implied by India–Asia mass-balance calculation. Nat. Geosci. 9, 848–853. doi:10.1038/ngeo2806
Jackson, J., and McKenzie, D. (1984). Active tectonics of the alpine–himalayan belt between western Turkey and Pakistan. Geophys. J. R. Astronomical Soc. 77, 185–264. doi:10.1111/j.1365-246X.1984.tb01931.x
Jackson, J., and McKenzie, D. (1988). The relationship between plate motions and seismic moment tensors, and the rates of active deformation in the Mediterranean and Middle East. Geophys. J. 93, 45–73. doi:10.1111/j.1365-246x.1988.tb01387.x
Jolivet, L., and Brun, J.-P. (2010). Cenozoic geodynamic evolution of the Aegean. Int. J. Earth Sci. 99, 109–138. doi:10.1007/s00531-008-0366-4
Jolivet, L., Faccenna, C., Goffé, B., Mattei, M., Rossetti, F., Brunet, C., et al. (1998). Midcrustal shear zones in postorogenic extension: example from the northern Tyrrhenian Sea. J. Geophys. Res. 103 (6), 12123–12160. doi:10.1029/97JB03616
Krystopowicz, N. J., and Currie, C. A. (2013). Crustal eclogitization and lithosphere delamination in orogens. Earth Planet. Sci. Lett. 361, 195–207. doi:10.1016/j.epsl.2012.09.056
Labrousse, L., Hetényi, G., Raimbourg, H., Jolivet, L., and Andersen, T. B. (2010). Initiation of crustal-scale thrusts triggered by metamorphic reactions at depth: insights from a comparison between the Himalayas and Scandinavian Caledonides: eCLOGITES AND THRUSTS, Initiation crustal-scale thrusts triggered by Metamorph. A. T. depth Insights a Comp. between theHimalayas Scand. Tect.,29, TC5002doi:10.1029/2009TC002602
Latorre, D., Di Stefano, R., Castello, B., Michele, M., and Chiaraluce, L. (2023). An updated view of the Italian seismicity from probabilistic location in 3D velocity models: the 1981–2018 Italian catalog of absolute earthquake locations (CLASS). Tectonophysics 846, 229664. doi:10.1016/j.tecto.2022.229664
Le Pichon, X., Henry, P., and Goffé, B. (1997). Uplift of tibet: from eclogites to granulites; implications for the andean plateau and the variscan belt. Tectonophisics 273 (1–2), 57–76. doi:10.1016/s0040-1951(96)00288-0
Leech, M. L., Singh, S., Jain, A. K., Klemperer, S. L., and Manickavasagam, R. M. (2005). The onset of India–Asia continental collision: early, steep subduction required by the timing of UHP metamorphism in the western Himalaya. Earth Planet. Sci. Lett. 234, 83–97. doi:10.1016/j.epsl.2005.02.038
Lo Bue, R., Faccenda, M., and Yang, J. (2021). The role of Adria plate lithospheric structures on the recent dynamics of the Central Mediterranean region. Journal of Geophysical Research:. Solid earth. 126, e2021JB022377. doi:10.1029/2021JB022377
Long, M. D., Wagner, L. S., King, S. D., Evans, R. L., Mazza, S. E., Byrnes, J. S., et al. (2021). Evaluating models for lithospheric loss and intraplate volcanism beneath the Central Appalachian Mountains. J. Geophys. Res. Solid Earth 126, e2021JB022571. doi:10.1029/2021JB022571
Lucente, F. P., Chiarabba, C., Cimini, G. B., and Giardini, D. (1999). Tomographic constraints on the geodynamic evolution of the Italian region. J. Geophys. Res. 104, 20307–20327. doi:10.1029/1999JB900147
Lucente, F. P., Margheriti, L., Piromallo, C., and Barruol, G. (2006). Seismic anisotropy reveals the long route of the slab through the western-central Mediterranean mantle. Earth Planet. Sci. Lett. 241, 517–529. doi:10.1016/j.epsl.2005.10.041
Lucente, F. P., and Margheriti, L. (2008). Subduction rollback, slab breakoff, and induced strain in the uppermost mantle beneath Italy. Geology 36 (5), 375–378. doi:10.1130/G24529A.1
Lustrino, M., Duggen, S., and Rosenberg, C. L. (2011). The Central-Western Mediterranean: anomalous igneous activity in an anomalous collisional tectonic setting. EarthScience Rev. 104 (1-3), 1–40. doi:10.1016/j.earscirev.2010.08.002
Maesano, F. E., Volpi, V., Civile, D., Basili, R., Conti, A., Tiberti, M. M., et al. (2020). Active extension in a foreland trapped between two contractional chains: the south Apulia fault system (SAFS). Tectonics 39 (7), e2020TC006116. doi:10.1029/2020tc006116
Magnoni, F., Casarotti, E., Komatitsch, D., Di Stefano, R., Ciaccio, M. G., Tape, C., et al. (2022). Adjoint tomography of the Italian lithosphere. Commun. Earth Environ. 3 (1), 69. doi:10.1038/s43247-022-00397-7
Malinverno, A., and Ryan, W. B. F. (1986). Extension in the Tyrrhenian Sea and shortening in the Apennines as result of arc migration driven by sinking of the lithosphere. Tectonics 5, 227–245. doi:10.1029/TC005i002p00227
Mazzoli, S., Cello, G., Deiana, G., Galdenzi, S., Gambini, R., Mancinelli, A., et al. (2000). Modes of foreland deformation ahead of the Apennine thrust front. J. Czech Geol. Soc. 45, 246.
Menichelli, I., De Gori, P., Lucente, F. P., Improta, L., and Chiarabba, C. (2023). Lithosphere structure, processes, and physical state of the alpine-apennine system. J. Geophys. Res. Solid Earth 128 (4), e2023JB026411. doi:10.1029/2023jb026411
Miller, M. S., and Agostinetti, N. P. (2012). Insights into the evolution of the Italian lithospheric structure from S receiver function analysis. Earth Planet. Sci. Lett. 345–348, 49–59. doi:10.1016/j.epsl.2012.06.028
Molnar, P., and Tapponnier, P. (1975). Cenozoic Tectonics of Asia: effects of a Continental Collision: features of recent continental tectonics in Asia can be interpreted as results of the India-Eurasia collision. Science 189, 419–426. doi:10.1126/science.189.4201.419
Nabelek, J., Hetenyi, G., Vergne, J. R. M., Sapkota, S., Kafle, B., Jiang, M., et al. (2009). Underplating in the Himalaya–Tibet collision zone revealed by the Hi-CLIMB experiment. Science 325 (5946), 1371–1374. doi:10.1126/science.1167719
Palano, M. (2015). On the present-day crustal stress, strain-rate fields and mantle anisotropy pattern of Italy. Geophys. J. Int. 200 (2), 969–985. doi:10.1093/gji/ggu451
Peccerillo, A. (2005). Plio-quaternary volcanism in Italy. Petrology, geochemistry. Heidelberg: Geodynamics. Springer.
Pezzo, G., Billi, A., Carminati, E., Conti, A., De Gori, P., Devoti, R., et al. (2023). Seismic source identification of the 9 November 2022 Mw 5.5 offshore Adriatic sea (Italy) earthquake from GNSS data and aftershock relocation. Sci. Rep. 13 (1), 11474. doi:10.1038/s41598-023-38150-5
Piana Agostinetti, N., and Amato, A. (2009). Moho depth andVp/Vsratio in peninsular Italy from teleseismic receiver functions. J. Geophys. Res. 114, B06303. doi:10.1029/2008JB005899
Piromallo, C., and Morelli, C. (2003). P wave tomography of the mantle under the Alpine–Mediterranean area. J. Geophys. Res. 108 (2065), B2. doi:10.1029/2002JB001757
Pondrelli, S., Confal, J. M., and Baccheschi, P. (2022). New SKS splitting anisotropy measurements point to tearing beneath Central Italy. Tectonophysics Vol. 840, 229549–231951. doi:10.1016/j.tecto.2022.229549
Pucci, S., Mirabella, F., Pazzaglia, F., Barchi, M. R., Melelli, L., Tuccimei, P., et al. (2014). Interaction between regional and local tectonic forcing along a complex Quaternary extensional basin: upper Tiber Valley, Northern Apennines, Italy. Quat. Sci. Rev. 102, 111–132. doi:10.1016/j.quascirev.2014.08.009
Raimbourg, H., Jolivet, L., and Leroy, Y. (2007). Consequences of progressive eclogitization on crustal exhumation, a mechanical study. Geophys. J. Int. 168, 379–401. doi:10.1111/j.1365-246X.2006.03130.x
Reilinger, R., and McClusky, S. (2011). Nubia-Arabia-Eurasia plate motions and the dynamics of Mediterranean and Middle East tectonics. Geophys. J. Int. 186, 971–979. doi:10.1111/j.1365-246X.2011.05133.x
Ring, U., Glodny, J., Will, T., and Thomson, S. (2010). The Hellenic subduction system: high-pressure metamorphism, exhumation, normal faulting, and large-scale extension. Annu. Rev. Earth Planet. Sci. 38, 45–76. doi:10.1146/annurev.earth.050708.170910
Rossetti, F., Faccenna, C., Jolivet, L., Funiciello, R., Tecce, F., and Brunet, C. (1999). Syn-versus post-orogenic extension: the case study of giglio island (northern tyrrhenian sea, Italy). Tecto- nophysics 304, 71–93. doi:10.1016/s0040-1951(98)00304-7
Rovida, A., Camassi, R., Gasperini, P., and Stucchi, M. (2011). Catalogo parametrico dei terremoti italiani, versione CPTI11.
Rovida, A., Locati, M., Camassi, R., Lolli, B., Gasperini, P., and Antonucci, A. (2022). Catalogo Parametrico dei Terremoti Italiani (CPTI15), versione 2.0, INGV.
Royden, L., and Faccenna, C. (2018). Subduction orogeny and the late cenozoic evolution of the mediterranean arcs. Annu. Rev. Earth Planet. Sci. 46, 261–289. doi:10.1146/annurev-earth-060115-012419
Royden, L. H., Burchfiel, B. C., and van der Hilst, R. D. (2008). The geological evolution of the Tibetan Plateau. Science 321, 1054–1058. doi:10.1126/science.1155371
Royden, L., and Husson, L. (2009). “Subduction with variations in slab buoyancy: models and application to the Banda and Apennine systems,” in Frontiers in Earth sciences. Editors S. Zone Geodynamics, F. Funiciello, and S. Lallemand (Berlin Heidelberg: Springer-Verlag). doi:10.1007/978-3-540-87974-9
Royden, L., Patacca, E., and Scandone, P. (1987). Segmentation and configuration of subducted lithosphere in Italy - an important control on thrust-belt and foredeep-basin Evolution. Geology 15, 714–717. doi:10.1130/0091-7613(1987)15<714:sacosl>2.0.co;2
Salimbeni, S., Pondrelli, S., and Margheriti, L. (2013). Hints on the deformation penetration induced by subductions and collision processes: seismic anisotropy beneath the Adria region (Central Mediterranean). J. Geophys. Res. Solid Earth 118 (11), 5814–5826. doi:10.1002/2013jb010253
Salimbeni, S., Pondrelli, S., Margheriti, L., Park, J., and Levin, V. (2008). SKS splitting measurements beneath Northern Apennines region: a case of oblique trench-retreat. Tectonophysics 462 (1-4), 68–82. doi:10.1016/j.tecto.2007.11.075
Schott, B., and Schmeling, H. (1998). Delamination and detachment of a lithospheric root. Tectonophysics 296 (1998), 225–247. doi:10.1016/s0040-1951(98)00154-1
Sengör, A. M. C., Özeren, S., Genc, T., and Zor, E. (2003). East Anatolian high plateau as a mantle-supported, north–south shortened domal structure. Geophys. Res. Lett. 30 (2003), 8045. doi:10.1029/2003gl017858
Sengör, A. M. C., Tüysüz, O., Imren, C., Sakınç, M., Eyidoğan, H., Görür, N., et al. (2005). The North anatolian fault. A new look:Annual Rev. Earth Planet. Sci. 33, 37–112. doi:10.1146/annurev.earth.32.101802.120415
Serpelloni, E., Cavaliere, A., Martelli, L., Pintori, F., Anderlini, L., Borghi, A., et al. (2022). Surface velocities and strain-rates in the Euro-Mediterranean region from massive GPS data processing. Front. Earth Sci. 10. doi:10.3389/feart.2022.907897
Serpelloni, E., Vannucci, G., Pondrelli, S., Argnani, A., Casula, G., Anzidei, M., et al. (2007). Kinematics of the Western Africa-Eurasia plate boundary from focal mechanisms and GPS data. Geophys. J. Int. 169, 1180–1200. doi:10.1111/j.1365-246x.2007.03367.x
Shi, F., Wang, Y., Yu, T., Zhu, L., Zhang, J., Wen, J., et al. (2018). Lower-crustal earthquakes in southern Tibet are linked to eclogitization of dry metastable granulite. Nat. Commun. 9, 3483. doi:10.1038/s41467-018-05964-1
Spada, M., Bianchi, I., Kissling, E., Agostinetti, N. P., and Wiemer, S. (2013). Combining controlled-source seismology and receiver function information to derive 3-D Moho topography for Italy. Geophys. J. Int. 194 (2), 1050–1068. doi:10.1093/gji/ggt148
Spakman, W., Van Der Lee, S., and Van Der Hilst, R. (1993). Travel-time tomography of the European-Mediterranean mantle down to 1400 km. Interiors 79, 3–74. doi:10.1016/0031-9201(93)90142-v
Stampfli, G. M., Borel, G., Cavazza, W., Mosar, J., and Ziegler, P. A. (2001). The paleotectonic atlas of the PeriTethyan domain. Eur. Geophys. Soc.
Stampfli, G. M., and Borel, G. D. (2002). A plate tectonic model for the Paleozoic and Mesozoic constrained by dynamic plate boundaries and restored synthetic oceanic isochrons. Earth Planet. Sci. Lett. 196, 17–33. doi:10.1016/S0012-821X(01)00588-X
Toussaint, G., Burov, E. E. B., and Avouac, J.-P. (2004). Tectonic evolution of a continental collision zone: a thermomechanical numerical model: NUMERICAL MODEL OF CONTINENTAL COLLISION. Tectonics 23, 1–24. doi:10.1029/2003TC001604
Wessel, P., Luis, J. F., Uieda, L., Scharroo, R., Wobbe, F., Smith, W. H., and Tian, D. (2019). The generic mapping tools version 6. Geochem. 20 (11), 5556–5564
Wittlinger, G., Farra, V., Hetenyi, G., Vergne, J., and Nabelek, J. (2009). Seismic velocities in southern Tibet lower crust: a receiver function approach for eclogite detection. Geophys. J. Int. 177, 1037–1049. doi:10.1111/j.1365-246x.2008.04084.x
Keywords: continental subduction and collision belt, lithosphere delamination, tomographic models, seismicity and crustal structure, seismicity and deformation
Citation: Chiarabba C, Menichelli I, Bianchi I, Giacomuzzi G and De Gori P (2023) Continental subduction of Adria in the Apennines and relation with seismicity and hazard. Front. Earth Sci. 11:1253443. doi: 10.3389/feart.2023.1253443
Received: 05 July 2023; Accepted: 28 September 2023;
Published: 17 October 2023.
Edited by:
Marc-André Gutscher, UMR6538 Geo-Ocean (Univ Brest, CNRS, Ifremer, UBS), FranceReviewed by:
Stephane Dominguez, UMR5243 Géosciences Montpellier, FranceGianluca Vignaroli, University of Bologna, Italy
Copyright © 2023 Chiarabba, Menichelli, Bianchi, Giacomuzzi and De Gori. This is an open-access article distributed under the terms of the Creative Commons Attribution License (CC BY). The use, distribution or reproduction in other forums is permitted, provided the original author(s) and the copyright owner(s) are credited and that the original publication in this journal is cited, in accordance with accepted academic practice. No use, distribution or reproduction is permitted which does not comply with these terms.
*Correspondence: C. Chiarabba, claudio.chiarabba@ingv.it