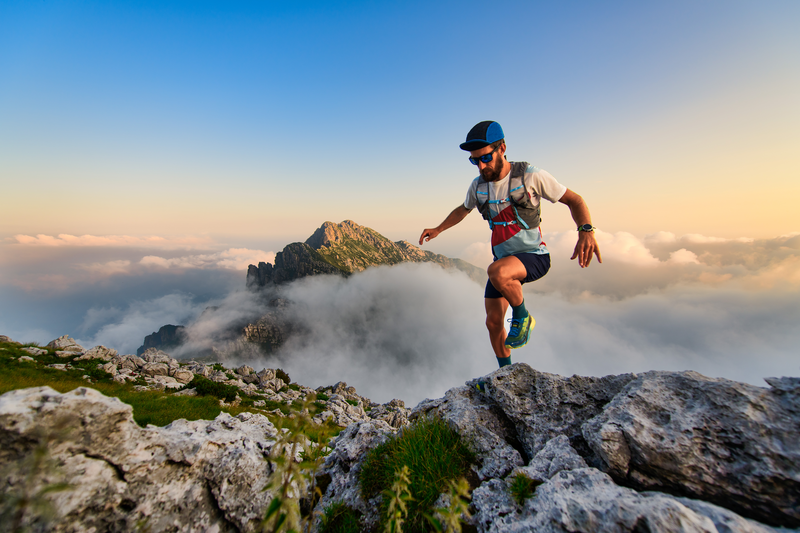
94% of researchers rate our articles as excellent or good
Learn more about the work of our research integrity team to safeguard the quality of each article we publish.
Find out more
ORIGINAL RESEARCH article
Front. Earth Sci. , 23 August 2023
Sec. Structural Geology and Tectonics
Volume 11 - 2023 | https://doi.org/10.3389/feart.2023.1247665
This article is part of the Research Topic Advances of New Technologies in Seismic Exploration View all 22 articles
To clarify the tectonic evolution of M15 block in the Andaman Sea, we perform a delicate study of fault geometry and dynamics using a 3D seismic data. The data reveal eight sequence interfaces from the Early Oligocene to the Quaternary, large scale and multi angle extensional strike-slip faults, and a series of normal faults. The two large scale faults F1 and F2 start in the Eocene and end in the Quaternary, controlling the regional structure. The NNE-SSW strike-slip F1 fault belongs to the South Sagaing fault and the NNE-SSW strike-slip F2 is the eastern Andaman fault, the strike-slip movement of which are controlled by the impact of the collision between the Indian plate and the Eurasian plate. Through the analysis of the fault development history by the method of the ancient drop and the growth index, we find that most of the large or secondary scale faults reach the maximum drop and growth index in the Miocene, indicating that the Miocene is a significant period of plate collision enhancing and faults generating. The regional stress field is dominated by E-W tension. The continental crust has expanded rapidly from the Oligocene to the Miocene which results in the rapid subsidence of the crust. This regional stress intensity becomes weak after the Miocene. The activities of the faults caused a large difference in terrain height between the west and the east in the study area, forming a pattern of the western depression and the eastern terrace. Many NNE-SSW, NE-SW or NEE-SWW trend strike-slip faults and minor faults develop in the Miocene. It echoes the event that the convergence and subduction of the Indian plate from SW to NE direction led to the right rotation and N-NNE strike-slip of the West Myanmar block in the Miocene, thus forming a regional large strike-slip fault. All of the faults affect the structure of the region.
The Andaman Sea has always been considered as a region of Cenozoic extensional and strike-slip activities and is related to the Himalayan compression event. Since the Cenozoic, due to the solid tectonic movement, the geomorphic pattern of the whole Southeast Asia and even the global geomorphology has undergone dramatic changes, especially the orogeny represented by the uplift of the Qinghai Tibet Plateau has a significant impact on the changes of the global environment and climate (Zachos et al., 2001; Hall, 2002; Yin, 2010). The Andaman Sea is located at the intersection of the Indian and Eurasian plates. Influenced by plate movement, it has complex structural characteristics, multiple fault patterns and wide distribution, forming a typical “trench arc basin” system (Diehl et al., 2013).
Controlled by the closure of the New Tethys Ocean Basin at 60–50 Ma, the collision between the Indian plate and the European plate (Garzanti and Gaetani, 2002; Najman, 2010; Wu et al., 2014), and the rifting and back-arc pull apart spreading of Andaman to the east of the Sunda subduction zone, multistage faults are widely distributed in the region (Khan and Chakraborty, 2005; Khan et al., 2017). The Sagaing fault extends southward from the Myanmar land to the Andaman Sea area. It is divided into several secondary faults at the sea inlet of the Ayeyarwaddy Delta and connected with the central expansion area. As a back-arc spreading center formed in a typical extensional environment in the Andaman Sea, the leading regional strike-slip faults are the West Andaman Fault, the Sagaing Fault and the Sumatra Fault from west to east. The characteristics of the faults in the sea area are related to the onshore area. The West Andaman fault separates the western accretion wedge zone, forearc depression zone and western highland uplift zone from the center back-arc basin. The Sumatra fault passes through the northern Sumatra basin and the southern back-arc basin from south to north, while the Sagaing fault runs through the entire eastern basin.
The strike-slip fault is the main feature of fault in the study area. With the convergence and subduction of plates, the dip angle will change and the angle near the subduction center will be smaller (Fitch, 1972; McCaffrey, 1992; Philippon and Corti, 2016; Schütt and Whipp, 2020; Yang et al., 2021; Okuwaki and Fan, 2022). This will result in large strike-slip faults with a length of hundreds of kilometers or longer, which mostly on the overriding plate and close to volcanic arcs (Manaker et al., 2008), such as the Alpine fault in New Zealand (Norris and Cooper, 2001), the Great Sumatra Fault in Sunda (McCaffrey, 2009) and the Enriquillo Plaintain Garden Fault (EPGF) in Caribbean (Calais et al., 1992; Mann et al., 1995). Due to the strike-slip faults are prone to large earthquakes, many people have conducted research on this issue (Sylvester, 1988; Schmittbuhl et al., 2006; Melgar et al., 2015; Chu et al., 2021; Hu et al., 2021). The relationship between different fault attributes (such as length displacement) is very important for understanding and predicting its mechanical, hydraulic, topographic and seismological characteristics (Kim and Sanderson, 2005; Gutscher and Lallemand, 2010; Ul-Hadi et al., 2013; Fossen and Rotevatn, 2016; Zhang et al., 2020; Yuan et al., 2022; Cheng et al., 2023). The change of strike-slip fault geometry is easy to observe on the surface and is often used to study the geometry of dip-slip faults (Barka and Kadinsky-Cade, 1988; Zhang and Sagiya, 2018; Zuza and Carlson, 2018). The degree of fault deformation may vary with geometric complexity of the faults (Dolan and Haravitch, 2014; Milliner et al., 2016). Strike-slip faults include both small-scale millimeter scale faults and large-scale faults that run through the entire crust (Segall and Pollard, 1983; Martel and Pollard, 1989; Dickinson, 1996; Singh et al., 2013). Its sliding distribution are commonly determined by correlating offset stream channels and offset channels are formed by the interplay of tectonic and geomorphic processes (Papaleo et al., 2017; Reitman et al., 2019). To study the distribution and features of the strike-slip faults is significant to understanding the migration and accumulation of hydrocarbon resources (Qiu et al., 2019; Tian et al., 2022).
Predecessors have also done some work on the Andaman Sea. Polachan and Racey (1994) studied the geological situation and tectonic evolution of Mergui Basin, located in the south of the back-arc basin area. Good progress has also been made in the research on the tectonic evolution of the Alcock rise and Sewell rises in the middle of the back-arc basin and the central expansion zone (Morley and Alvey, 2015; Morley, 2017; Curray, 2005; Curray et al., 1979; Curray et al., 1982; Raju et al., 2004; Diehl et al., 2013; Morley, 2013; Mahattanachai et al., 2021). However, few studies have been conducted on the Mergui platform area centered on the M15 block and penetrated by the Sagaing fault. The tectonic evolution of the study area has not been reported yet. The development and distribution of faults play a controlling role in the regional tectonic evolution. Therefore, we study and analyze the fault structural characteristics of the M15 block in the Andaman Sea, so that make the tectonic evolution of the area clear and provide a guidance for understanding the migration and storage of petroleum.
The Andaman Sea is located in the eastern part of the Bay of Bengal, at the intersection of the Eurasian and Indian Ocean plates (Curray, 2005). The area is about 7.98×104 km2, 1200 km from north to south, and a width of 645 km from east to west. The average water depth is about 1000 m, but the water depth in the central area (about 5% of the sea area) exceeds 3000 m, and water depths very locally exceed 4000 m (Morley, 2017). As a marginal sea in Southeast Asia, it is a convergent continental margin (Luan et al., 2021) (Figure 1), stretching from the Malay Peninsula in the east to the Bay of Bengal in the west, from southern Myanmar in the north to northern Sumatra Island in the south (Curray, 2005; Srisuriyon and Morley, 2014). The study area, block M15 (Figure 1), is located in the eastern part of the Andaman Sea, with a latitude range of 11°40′00″-12°19′07″and a longitude range of 96°23′15″-97°01′48″. The temporal sampling rate of seismic data is 2 m, and the area of 3D survey data is about 4000 km2. The Andaman Sea is a Cenozoic Ocean basin, and the regional sedimentary strata are mainly Cenozoic strata (Table 1).
FIGURE 1. Map showing major geomorphologic features along the Andaman Sea. The study area is marked by the rectangle with the outline.
The survey area is about 200 km from the Mergui port in Myanmar, with a depth of more than 100 m. The maximum depth of the western boundary of the survey area is about 1500 m. The depth gradually deepens and varies significantly from eastern to western boundaries. The data acquisition was completed by the COSL seismic exploration vessel HAIYANGSHIYOU 721 (abbreviated as HYSY721). The seismic sampling rate is 2 m, and the 3D survey data area is about 4000 km2 consisting of 117 prime sail lines with an average sail line length of 75.725 km HYSY721 uses twelve 7050 m streamers and two alternately fired 4030 cubic inch air gun arrays in configuration-acquired data.
We use well-imaged high-resolution 3D seismic data to study the fault system in the region. Firstly, the sequence of the M15 block is divided by seismic interpretation profile and relevant geological data in the study area. Secondly, the six significant faults in the region are quantitatively analyzed by the ancient drop method and growth index method. Then, the dynamic origin of the faults is analyzed, and its influence on the regional tectonic evolution is analyzed.
Referring to the relevant stratigraphic sequence division (Ye et al., 2022; Chen et al., 2023), we divided the stratigraphic sequence in more detail and identified seven stratigraphic sequences (from old to new) in the seismic data profiles: the Early Oligocene, the Late Oligocene, the Early Miocene, the Middle Miocene, the Late Miocene, the Pliocene and the Quaternary, which are defined by eight sequence interfaces named Tg, T6, T5, T4, T3, T2, T1 and SF, respectively. We also identified six large strike-slip faults and secondary faults, named F1, F2, F3, F4, F5 and F6, respectively. The distribution of them is shown in Figure 2. The color of Figure 2 represents the time of T6 horizon. In order to clearly show the faults development history, we select three seismic profiles (Figure 3A; Figure 3B; Figure 3C), the position of which are shown in Figure 2, the yellow lines named line1, line2 and line3. From Figure 3A, we can see that there is a very bit fault named F1 passing through the basement Tg until SF. It is easy to see that the F1 fault was active before the Oligocene. Through analysis, we know that the F1 fault is the known South Sagaing fault. It is the Sagaing fault that controls the tectonic movement in the area, and the Sagaing fault was formed by plate movement before the Eocene. After the Eocene, the Andaman Sea suffered from land and land subduction, and the Myanmar microplate collided with the Indian plate, resulting in the counterclockwise rotation of the Indian plate, while the Myanmar microplate further moved northward, eventually coupling with the Indian plate boundary. Although there is no sufficient evidence to prove that the F1 fault belongs to the same fault as the Sagaing fault, we still call it the South Sagaing fault because it has the same strike as the dextral strike slip Sagaing fault. Besides, in the process of fault analysis for the seismic profiles, it is found that the F1 fault is obviously connected with the South Sagaing fault in the north and south of the study area, which further proves that the F1 fault belongs to the South Sagaing fault. The activity of the fault caused a large difference in terrain height between the east and the west in the study area, forming a pattern of the separation of the western depression, the eastern slope zone and the eastern terrace.
FIGURE 2. The distribution of Fault F1-F6 on T6 horizon. The three yellow lines on the map mark the location of three seismic profiles, respectively.
FIGURE 3. Stratigraphic sequences and faults in the study area. (A) Seismic profile of line1 (the up map) and the interpreted stratigraphic sequences (the down map). Fault F1 and F6 are shown on the map. (B) Seismic profile of line2 (the up map) and the interpreted stratigraphic sequences (the down map) and Fault F2, F4 and F5 are shown on the map. (C) Seismic profile of line3 (the up map) and the interpreted stratigraphic sequences (the down map) and Fault F2, F3 and F5 are shown on the map.
The F2 fault (Figure 3B; Figure 3C) we call it the eastern Andaman fault separates the Mergui Ridge from the eastern platform. Affected by the F2 fault, the Oligocene strata on the right side of F2 were uplifted and received some denudation, leading to the thickness decreased significantly. Moreover, the strike-slip and rifting in the north of Mergui Basin further increased the thickness difference between the east and west sides of the fault (Luan et al., 2023). In the Early Miocene and middle Miocene, rifting and strike-slip occurred in the northern part of the Mergui Basin and the degree of back-arc expansion increased. At the same time, there was a large-scale eruption of volcanic magma, coupled with the uplift of the volcanic island arc, and thus the regional terrain difference also increased. From Figure 3B and Figure 3C, we can also see that the changes of the strata (T3-SF) were not so obvious after Miocene. It is due to the activities of the faults attenuated.
NNE-SSW strike-slip faults and several NEE-SWW secondary faults (such as F3-F6 faults in Figures 3A–C) control the structural development in the region. A series of normal faults are also developed in M15 block of Andaman Sea. In order to clearly show the trend of the faults, we extract the coherence attributes of T6 horizon in the red rectangle area (Figure 4A) and the blue rectangle area (Figure 4B) of Figure 2. From Figure 4, we can clearly see the F1 fault, the F2 fault and other secondary or smaller faults. The faults mostly are distributed in NNE-SSW, NE-SW or NEE-SWW directions. The secondary faults, mainly showing extensional displacement, are very closely distributed in the basin and tend to form arrays with dominant dip or conjugate fault groups. The NNE-SSW trending Sagaing fault in the study area is consistent with the further northward trending Sagaing fault. These faults interact with each other to form the current tectonic structure.
FIGURE 4. Faults distribution in the study area. (A) The coherence attribute slice of T6 horizon in the red rectangle area of Figure 2. (B) The coherence attribute slice of T6 horizon in the blue rectangle area of Figure 2.
Faults developed in the study area comprise four fault families based on their geometry, orientation, distribution and relationship with main structures (Figure 5).
FIGURE 5. Geological sections and faults across the M15 block of Andaman Sea ((A–C) is the profile of the study area from south to north).
The study area has produced large-scale and multi-angle extensional strike-slip faults. Through refined interpretation of seismic profiles and identification of faults in the study area, it is believed that the area mainly includes negative flower shaped faults, “Y" type composite faults, domino-type fault zones and graben horst structural combination styles, which are widely distributed in the whole study area.
1) The negative flower structure is associated with the strike-slip fault under the tensile and torsional stress field conditions (Figure 5A; Figure 5C). The fault forms a syncline structure above the strike-slip fault. The flower structure is dominated by positive slip distance, and the cross-section is steep and gentle. The stratum between the faults is a graben structure. The folds and faults derived simultaneously on the flank are arranged in the echelon.
2) Domino fault zone: a series of faults with a consistent or nearly consistent dip in the profile and parallel or nearly parallel strike in the plane from the domino structural style (Figure 5B).
3) “Y" type fault is often developed in an extensional fault depression basin (Figure 5A). The intersection of the main fault and the reverse dipping secondary fault presents a “Y" shape. The main fault is significant in scale and has been developed for a long time. The fault usually passes through the lower sedimentary layer, which is generally a basin-controlling or belt-controlling fault, and controls the depression and boundary of the region. The secondary fault is generally located in the shallow section of the stratigraphic depression side, opposite the tilt direction of the main fault.
4) Graben and horst: the two sides of the graben are surrounded by faults, and the middle is a falling fault block common in fault basins. The horst is opposite to the graben; the middle part is a rising fault block, and the fault blocks on both sides are relatively falling. Graben is more developed in the areas where the crust is stretched, and graben horsts are often associated with development, which occurs alternately under the control of faults. A representative horst combination can be found in Block M15 (Figure 5C).
In this section, we select six representative faults from the four fault families to compile. These data are used to evaluate the growth history of faults.
The fault system is divided into two levels. The first level fault mainly controls the slope break zone boundary between the eastern Andaman Sea platform and the western depression or eastern slope zone. It controls the formation and evolution of oil and gas in the region. The secondary fault mainly controls the characteristics of the secondary depression and graben horst in the area, leading to the uplift and subsidence of the stratum. The secondary fault significantly restricts oil and gas accumulation and storage. In the study area, faults have an important influence on the sedimentation and distribution of provenance.
The F1 and F2 faults are the primary boundary significant faults controlling the eastern part of the Andaman Sea. They extend long and have a large scale. The profile shows that they penetrate the T6 reflector downward. The quantitative analysis of the F1-F6 faults in the study area is carried out by using the method of ancient fall and growth index to study the characteristics of fault activity in the area (Figure 6).
FIGURE 6. Analysis of the ancient drop and the growth index of F1–F6 faults ((A–F) correspond to F1–F6 respectively).
F1 is the Sagaing fault. It controls the regional structure. We can see from Figure 6A that the fault began in the Eocene and then started to grow. In the Oligocene, it reached the maximum ancient drop, 1456.17 m with the maximum growth index,5.87. After the Oligocene, the ancient drop and growth index showed a decreasing trend as a whole. This is because the strata in the west of the fault had been in subsidence and the degree of subsidence was severe in the Oligocene, leading to the maximum growth index and ancient drop. After that, the subsidence became slowly.
F2 is the East Andaman Fault. The fault trend in the region is NNE-SSW; The west of F2 is the eastern platform, where the traps are relatively well-defined. The east side of F2 is Mergui Ridge. Figure 6B shows the ancient drop and growth index change dramatically before the Miocene, illustrating that a significant difference in strata drop and thickness before and after the Miocene. The reason is that the F2 fault was more active before the Miocene than after it, characterized by higher localized basement and thinner strata due to denudation.
It can be seen from the figures (Figures 6C–F) that the faults ancient drop and growth index are generally represented as one activity peak in the Middle Miocene. Since the Late Oligocene, the faults ancient drop and growth index in the study area have gradually increased, indicating that the faults activities have gradually increased; In the Early Miocene, the faults ancient drop and growth index reached the maximum, indicating that the faults activities reached the peak, which was the most active period of tectonic activity; After the Early Miocene, the faults ancient drop and growth index decreased, indicating that the activity rate of faults and tectonic decreased gradually.
Since the Oligocene, the fault ancient drop and growth index trend tend to be consistent. The fault ancient drop and growth index reached the maximum in the Early Miocene, indicating that the fault activity reached the maximum in this period. At this time, due to the collision between the Indian Plate and the Myanmar microplate, the Sagaing fault started to dextral strike-slip, the Myanmar Basin experienced tension and fracture, the Andaman Sea expanded, and the Mergui basin was formed. At the same time, large-scale marine transgression began to occur, characterized by progressive sedimentation of sandstone and mudstone from river facies and delta facies to shallow sea facies, leading to an increase in the thickness difference between the hanging wall and footwall of the fault. The reason for the thinning of the hanging wall of the fault may be due to the effects of ocean current scouring, seawater erosion, etc. Therefore, the fault growth index increase may be caused by the combined effects of sedimentation and seabed erosion. After that, the ancient drop and growth index decreased. In the Late Miocene, the ancient drop and growth index reached the lowest, and the fault activity gradually weakened. This indicates that a large-scale strike-slip pull-apart movement along the Sagaing fault was from the Early Miocene to the Early Pliocene. The East Andaman Sea Basin gradually developed, and the Mergui Basin then turned into a depression stage.
From the Early Pliocene to the present, the Myanmar microplate continued to move northward and collided with the Himalayan fault of the Eurasian plate. Due to the blocking effect of the Himalayan fault, the region underwent structural inversion. A series of negative flower structures, “Y" type faults and other structural styles were produced by torsional compression and extrusion and the region is in an east-west torsional compression tectonic stress environment. During this period, the Andaman Sea was in the stage of regression.
It can be seen from the above analysis that a large number of NNE-SSW, NE-SW and NEE-SWW trending faults were developed during the Late Oligocene and the Early Miocene. During the Oligocene, the growth index and ancient fall of the study area were both at low values, indicating that the collision between the Indian plate and the Eurasian plate was a soft collision at this time, and the fault gradually began to form. The characteristics of the primary and secondary faults have yet to appear. The Sagaing fault started to dextral strike-slip and the Mergui basin was formed. At this time, the study area (red rectangle in Figure 7) belongs to a continental crust environment (Figure 7D). The continental crust began to expand, resulting in rapid subsidence of the stratum, which lasted until the Early Miocene, and then the subsidence slowed down, and Graben and half-graben almost filled the sea level (Curray, 2005). The Miocene is an important period of fault development in the study area, and the plate movement at that time is relatively active. During this period, the plate collision gradually strengthened, and the volcanic arc was uplifted, and the fore-arc and back-arc basins in Andaman Sea areas began to take initial shape, and the strike-slip fault extended southward to the north of the Mergui basin (Figure 7C). The back-arc basin affected the formation of the study area. With the change in the extension direction of the fault, the strike-slip fault developed to the Mergui basin. This change promoted regional tension deformation, and the growth index and ancient fall gradually increased during this period, reaching the peak in the Middle Miocene (Figure 6). The coupling effect between Indian and Australian plates has a significant influence on the fault activities in the study area, which is the main reason for the development of faults; The accelerated uplift of the volcanic island arc leads to the gradual increase of the thickness difference of the stratum in the Andaman Sea area (Figure 7B). In the Late Miocene, with the coupling of the Indian plate and the West Myanmar block, the Sagaing fault began to move, and large-scale strike-slip pull apart movement occurred along the Sagaing fault, and the “trench-arc-basin” system of the Andaman Sea gradually developed. Now, the study area is in marine environment (Figure 7A).
FIGURE 7. Structural evolution model of Andaman Sea (According to the revision of literature (The age of (A–D) is from new to old) (Srisuriyon and Morley, 2014)).
The development of faults controls the discharge of fluids and the migration of oil and gas. The strike-slip form of the Sagaing fault in the back-arc depression of the study area determines the direction of E-W and NNE-SSW tensile stress (Singha et al., 2019). The tension in the E-W direction has led to the occurrence of strike-slip movements and N-S trending Sagaing fault, resulting in the formation of central extension zones and near N-S trending regional faults. When the local formation pressure gradually increases and finally reaches the formation fracture pressure, the formation will break. At this time, due to the occurrence of the fracture, the fluid will start to release upward through the fracture (Mohan et al., 2006).
From the detailed analysis of seismic data, we reach the following conclusions:
1) It is found that a series of NNE-SSW strike-slip faults and NE-SW or NEE-SWW faults are mainly developed in the study area. Strike-slip faults control the depression and uplift in this area, forming a geomorphic morphology of high east and low west, which echoes the collision between the Indian plate and the Eurasian plate.
2) The fault mainly developed between the Late Oligocene and the Early Miocene. Since the Late Oligocene, the collision between the Indian and Eurasian plates has become more robust. At the same time, the coupling between the Indian plate and the Myanmar microplate has led to a significant dextral strike-slip movement of the Sagaing fault. F1 Sagaing fault and F2 East Andaman fault developed before the Oligocene. The continuous activities of the primary and secondary faults until the Early Miocene promoted the formation of the tectonic framework in the study area.
3) The regional stress field affects the fault’s development. The stress field in the region is dominated by E-W tension. The continental crust has expanded rapidly since the Oligocene, resulting in rapid subsidence, which lasted until the Early Miocene and then slowed down. This regional stress intensity was significantly greater from the Late Oligocene to the Early Miocene than that after the Early Miocene, so many near NNE-SSW growth normal faults were developed in the study area before the Early Miocene.
The original contributions presented in the study are included in the article/supplementary material, further inquiries can be directed to the corresponding author.
MG and XL contributed to conception and design of the study. MG wrote the first draft of the manuscript. HZ and BH reviewed and modified the manuscript. All authors contributed to the article and approved the submitted version.
This study is supported by National Natural Science Foundation of China (Nos. 92055211 and 42249801), China-ASEAN Maritime Cooperation Fund Project (No. 12120100500017001).
The authors declare that the research was conducted in the absence of any commercial or financial relationships that could be construed as a potential conflict of interest.
All claims expressed in this article are solely those of the authors and do not necessarily represent those of their affiliated organizations, or those of the publisher, the editors and the reviewers. Any product that may be evaluated in this article, or claim that may be made by its manufacturer, is not guaranteed or endorsed by the publisher.
Barka, A. A., and Kadinsky-Cade, K. (1988). Strike-slip fault geometry in Turkey and its influence on earthquake activity. Tectonics 7 (3), 663–684. doi:10.1029/TC007i003p00663
Calais, E., Béthoux, N., and de Lépinay, B. M. (1992). From transcurrent faulting to frontal subduction: a seismotectonic study of the north-ern caribbean plate boundary from Cuba to Puerto Rico. Tectonics 11 (1), 114–123. doi:10.1029/91tc02364
Chaipornkaew, L., Elston, H., Cooke, M., Mukerji, T., and Graham, S. A. (2022). Predicting off-fault deformation from experimental strike-slip fault images using convolutional neural networks. Geophys. Res. Lett. 49, e2021GL096854. doi:10.1029/2021GL096854
Chen, J. R., Luan, X. W., and Wei, X. Y. (2023). Sedimentary evolution process and genesis of Danlao continental slope canyon group in the eastern Andaman Sea. Acta Sedimentol. Sin., 1–20. (in Chinese). doi:10.14027/j.issn.1000-0550.2022.052
Cheng, C., Wang, D., Yao, Q., Fang, L., Xu, S., Huang, Z., et al. (2023). The 2021 Mw 7.3 madoi, China earthquake: transient supershear ruptures on a presumed immature strike-slip fault. J. Geophys. Res. Solid Earth 128, e2022JB024641. doi:10.1029/2022JB024641
Chu, S. X., Tsai, V. C., Trugman, D. T., and Hirth, G. (2021). Fault interactions enhance high-frequency earthquake radiation. Geophys. Res. Lett. 48 (20), e2021GL095271. doi:10.1029/2021gl095271
Curray, J. R., Emmel, F. J., Moore, D. G., and Raitt, R. W. (1982). “Structure, tectonics and geological history of the northeastern Indian Ocean,” in The Ocean basins and margins. The Indian ocean. Editors A. E. M. Nairn, and F. G. Stehli (New York: Plenum Press), 6, 399–450.
Curray, J. R., Moore, D. G., Lawver, L. A., Emmel, F. J., and Kieckheffer, R. (1979). Tectonics of the andaman sea and Burma. Am. Assoc. Pet. Geol. Mem. 29, 189–198.
Curray, J. R. (2005). Tectonics and history of the Andaman Sea region. J. Asian Earth Sci. 25 (1), 187–232. doi:10.1016/j.jseaes.2004.09.001
Dickinson, W. R. (1996). Kinematics of transrotational tectonism in the California Transverse Ranges and its contribution to cumulative slip along the San Andreas transform fault system. Denver, Colorado: Geological Society of America Special Paper 305, 1–46.
Diehl, T., Waldhauser, F., Cochran, J. R., Kamesh Raju, K. A., Seeber, L., Schaff, D., et al. (2013). Back-Arc extension in the Andaman Sea: Magmatic and tectonic processes imaged by high-precision teleseismic double-difference relocation of earthquake swarms. AGU Fall Meeting Abstracts. doi:10.1002/jgrb.50192
Diehl, T., Waldhauser, F., Cochran, J. R., Kattoju, K. R., Seeber, L., Schaff, D. P., et al. (2010). Back-Arc extension in the Andaman Sea: magmatic and tectonic processes imaged by high-precision teleseismic double-difference relocation of earthquake swarms. AGU Fall Meet Abstr.
Dolan, J. F., and Haravitch, B. D. (2014). How well do surface slip measurements track slip at depth in large strike-slip earthquakes? The importance of fault structural maturity in controlling on-fault slip versus off-fault surface deformation. Earth Planet. Sci. Lett. 388, 38–47. doi:10.1016/j.epsl.2013.11.043
Fitch, T. J. (1972). Plate convergence, transcurrent faults, and internal deformation adjacent to southeast Asia and the western Pacific. J. Geophys. Res. 77 (23), 4432–4460. doi:10.1029/jb077i023p04432
Fossen, H., and Rotevatn, A. (2016). Fault linkage and relay structures in extensional settings - a review. Earth-Science Rev. 154, 14–28. doi:10.1016/j.earscirev.2015.11.014
Garzanti, E., and Gaetani, M. (2002). Unroofing history of late paleozoic magmatic arcs within the "Turan Plate" (Tuarkyr, Turkmenistan). Sediment. Geol. 151 (1-2), 67–87. doi:10.1016/S0037-0738(01)00231-7
Gutscher, M., and Lallemand, S. (2010). Birth of a major strike-slip fault in SW Japan. Terra nova. 11 (5), 203–209. doi:10.1046/j.1365-3121.1999.00247.x
Hall, R. (2002). Cenozoic geological and plate tectonic evolution of se asia and the SW pacific: computer-based reconstructions, model and animations. J. Asian Earth Sci. 20 (4), 353–431. doi:10.1016/S1367-9120(01)00069-4
Han, X. Y., Tang, L. J., Deng, S., and Cao, Z. C. (2020). Spatial characteristics and controlling factors of the strike-slip fault zones in the northern slope of tazhong uplift, tarim basin: insight from 3D seismic data. Acta Geol. Sin. Engl. Ed. 94 (2), 516–529. doi:10.1111/1755-6724.14333
Hu, Z., Yang, X., Yang, H., Huang, W., Wu, G., Miao, S., et al. (2021). Slip rate and paleoseismology of the Bolokenu-Aqikekuduk (Dzhungarian) right-lateral strike-slip fault in the northern Tian Shan, NW China. Tectonics 40, e2020TC006604. doi:10.1029/2020TC006604
Khan, P. K., and Chakraborty, P. P. (2005). Two-phase opening of Andaman Sea: a new seismotectonic insight. Earth Planet. Sci. Lett. 229 (3-4), 259–271. doi:10.1016/j.epsl.2004.11.010
Khan, P. K., Shamim, S., Mohanty, M., Kumar, P., and Banerjee, J. (2017). Myanmar-Andaman-Sumatra subduction margin revisited: insights of arc-specific deformations. J. Earth Sci. 28 (4), 683–694. doi:10.1007/s12583-017-0752-6
Kim, Y. S., and Sanderson, D. J. (2005). The relationship between displacement and length of faults: a review. Earth Sci. Rev. 68, 317–334. doi:10.1016/j.earscirev.2004.06.003
Klinger, Y. (2022). Imprint of the continental strike-slip fault geometrical structure in geophysical data. Geophys. Res. Lett. 49, e2022GL098146. doi:10.1029/2022GL098146
Luan, X. W., Li, L., Lu, Y. T., Fan, G. Z., Wei, X. Y., and Lunt, P. (2023). Hydrocarbon accumulation controlled by early stage rifting and late stage orthogonal subduction, the Madura Sub-Basin, Indonesia as example. Journal of Asian Earth Sciences 253, 105704. doi:10.1016/j.jseaes.2023.105704
Luan, X. W., Mohammad, S. I., Wei, X. Y., Lu, Y. T., Fan, G. Z., Suang, K. P., et al. (2021). Hydrocarbon accumulation in an active accretionary prism, a case study in the deepwater Rakhine Basin, Myanmar offshore. Journal of Asian Earth Sciences 221, 1367–9120. doi:10.1016/j.jseaes.2021.104941
Mahattanachai, T., Morley, C. K., Charusiri, P., and Kanjanapayont, P. (2021). The andaman basin central fault zone, Andaman Sea: characteristics of a major deepwater strike-slip fault system in a polyphase rift. Mar. Petroleum Geol. 128, 104997. doi:10.1016/j.marpetgeo.2021.104997
Manaker, D. M., Calais, E., Freed, A. M., Ali, S. T., Przybylski, P., Mattioli, G., et al. (2008). Interseismic plate coupling and strain partition-ing in the northeastern Caribbean. Geophys. J. Int. 174 (3), 889–903. doi:10.1111/j.1365-246x.2008.03819.x
Mann, P., Taylor, F., Edwards, R. L., and Ku, T.-L. (1995). Actively evolving microplate formation by oblique collision and sideways motion along strike-slip faults: an example from the northeastern caribbean plate margin. Tectonophysics 246 (1–3), 1–69. doi:10.1016/0040-1951(94)00268-e
Martel, S. J., and Pollard, D. D. (1989). Mechanics of slip and fracture along small faults and simple strike-slip fault zones in granitic rock. J. Geophys. Res. 94, 9417–9428. doi:10.1029/JB094iB07p09417
McCaffrey, R. (1992). Oblique plate convergence, slip vectors, and forearc deformation. J. Geophys. Res. 97 (B6), 8905–8915. doi:10.1029/92jb00483
McCaffrey, R. (2009). The tectonic framework of the Sumatran subduction zone. Annu. Rev. Earth Planet. Sci. 37, 345–366. doi:10.1146/annurev.earth.031208.100212
Melgar, D., Geng, J., Crowell, B. W., Haase, J. S., Bock, Y., Hammond, W. C., et al. (2015). Seismogeodesy of the 2014Mw6.1 napa earthquake, California: rapid response and modeling of fast rupture on a dipping strike-slip fault: seismogeodesy of the napa earthquake. J. Geophys. Res. Solid Earth. 120, 5013–5033. doi:10.1002/2015JB011921
Milliner, C., Sammis, C., Allam, A., Dolan, J., Hollingsworth, J., Leprince, S., et al. (2016). Resolving fine-scale heterogeneity of co-seismic slip and the relation to fault structure. Sci. Rep. 6 (1), 27201–27209. doi:10.1038/srep27201
Mohan, K., Damgwal, S. G. V., Sengupta, S., and Desai, A. G. (2006). Andaman basin—A future exploration target. Lead. Edge 25 (8), 964–967. doi:10.1190/1.2335164
Morley, C. K., and Alvey, A. (2015). Is spreading prolonged, episodic or incipient in the Andaman Sea? Evidence from deep-water sedimentation. J. Asian Earth Sci. 98, 446–456. doi:10.1016/j.jseaes.2014.11.033
Morley, C. K. (2017). Chapter 4 cenozoic rifting, passive margin development and strike-slip faulting in the Andaman Sea: a discussion of established v. new tectonic models. Geol. Soc. Lond. Memoirs 47 (1), 27–50. doi:10.1144/M47.4
Morley, C. K. (2013). Discussion of tectonic models for Cenozoic strike-slip fault-affected continental margins of mainland se Asia. J. Asian Earth Sci. 76 (oct.25), 137–151. doi:10.1016/j.jseaes.2012.10.019
Najman, Y., Appel, E., Boudagher-Fadel, M., Bown, P., Carter, A., Garzanti, E., et al. (2010). Timing of India-asia collision: geological, biostratigraphic, and palaeomagnetic constraints. J. Geophys. Res. Solid Earth 115, B12416. doi:10.1029/2010JB007673
Norris, R. J., and Cooper, A. F. (2001). Late Quaternary slip rates and slip partitioning on the Alpine Fault, New Zealand. J. Struct. Geol. 23 (2–3), 507–520. doi:10.1016/s0191-8141(00)00122-x
Okuwaki, R., and Fan, W. (2022). Oblique convergence causes both thrust and strike-slip ruptures during the 2021 M 7.2 Haiti earthquake. Geophys. Res. Lett. 49, e2021GL096373. doi:10.1029/2021GL096373
Papaleo, E., Cornwell, D. G., and Rawlinson, N. (2017). Seismic tomography of the north anatolian fault: new insights into structural heterogeneity along a continental strike-slip fault. Geophys. Res. Lett. 5, 2186–2193. doi:10.1002/2017GL072726
Philippon, M., and Corti, G. (2016). Obliquity along plate boundaries. Tectonophysics 693, 171–182. doi:10.1016/j.tecto.2016.05.033
Polachan, S., and Racey, A. (1994). Stratigraphy of the Mergui basin, Andaman Sea: implications for petroleum exploration. J. Petroleum Geol. 17 (4), 373–406. doi:10.1111/j.1747-5457.1994.tb00147.x
Qiu, H., Deng, S., Cao, Z., Yin, T., and Zhang, Z. (2019). The evolution of the complex anticlinal belt with crosscutting strike- slip faults in the central Tarim Basin, NW China. Tectonics 38, 2087–2113. doi:10.1029/2018TC005229
Raju, K., Ramprasad, T., Rao, P. S., Rao, B. R., and Varghese, J. (2004). New insights into the tectonic evolution of the andaman basin, northeast indian ocean. Earth Planet. Sci. Lett. 221 (1-4), 145–162. doi:10.1016/S0012-821X(04)00075-5
Reitman, N. G., Mueller, K. J., Tucker, G. E., Gold, R. D., Briggs, R. W., and Barnhart, K. R. (2019). Offset channels may not accurately record strike slip fault displacement: evidence from landscape evolution models. J. Geophys. Res. Solid Earth 124, 13427–13451. doi:10.1029/2019JB018596
Schmittbuhl, J., Chambon, G., Hansen, A., and Bouchon, M. (2006). Are stress distributions along faults the signature of asperity squeeze? Geophys. Res. Lett. 33 (13), L13307. doi:10.1029/2006gl025952
Schütt, J. M., and Whipp, D. M. (2020). Controls on continental strain partitioning above an oblique subduction zone, Northern Andes. Tectonics 39 (4), e2019TC005886. doi:10.1029/2019tc005886
Segall, P., and Pollard, D. D. (1983). Nucleation and growth of strike slip faults in granite. J. Geophys. Res. Solid Earth 88, 555–568. doi:10.1029/JB088iB01p00555
Singh, S. C., Moeremans, R., Mcardle, J., and Johansen, K. (2013). Seismic images of the sliver strike-slip fault and back thrust in the andaman-nicobar region. J. Geophys. Res. Solid earth JGR 118, 5208–5224. doi:10.1002/jgrb.50378
Singha, P., Dewangan, P., Raju, K. K., Aswini, K. K., and Reddy, T. R. (2019). Geometry of the subducting Indian plate and local seismicity in the Andaman region from the passive obs experiment. Bull. Seismol. Soc. Am. 109 (2), 797–811. doi:10.1785/0120180178
Srisuriyon, K., and Morley, C. K. (2014). Pull-apart development at overlapping fault tips: oblique rifting of a cenozoic continental margin, northern Mergui basin, Andaman Sea. Geosphere 10 (1), 80–106. doi:10.1130/GES00926.1
Sylvester, A. (1988). Strike-slip faults. Geol. Soc. Am. Bull. 100 (11), 1666–1703. doi:10.1130/0016-7606(1988)100<1666:ssf>2.3.co;2
Tian, F. L., He, D. F., Chen, J. J., and Mao, D. F. (2022). Vertical differential structural deformation of the main strike-slip fault zones in the shunbei area, central tarim basin: structural characteristics, deformation mechanisms, and hydrocarbon accumulation significance. Acta Geol. Sin. Engl. Ed. 96 (4), 1415–1431. doi:10.1111/1755-6724.14973
Ul-Hadi, S., Khan, S. D., Owen, L. A., and Khan, A. S. (2013). Geomorphic response to an active transpressive regime: a case study along the chaman strike-slip fault, western Pakistan. Earth Surf. Process. Landforms J. Br. Geomorphol. Res. Group 38 (3), 250–264. doi:10.1002/esp.3272
Wu, F. Y., Ji, W. Q., Wang, J. G., Liu, C. Z., Chung, S. L., and Clift, P. D. (2014). Zircon u-pb and hf isotopic constraints on the onset time of India-asia collision. Am. J. Sci. 314 (2), 548–579. doi:10.2475/02.2014.04
Yang, X., Singh, S. C., and Deighton, I. (2021). The margin-oblique kumawa strike-slip fault in the banda forearc, east Indonesia: structural deformation, tectonic origin and geohazard implication. Tectonics 40, e2020TC006567. doi:10.1029/2020TC006567
Ye, C. H., Luan, X. W., Fan, A. P., Ran, W. M., Mu, J. X., He, M. Y., et al. (2022). Miocene reef evolution and palaeo-environmental response in platform margins of the eastern Andaman Sea. Adv. Earth Sci. (in Chinese) 37 (03), 316–330. doi:10.11867/j.issn.1001-8166.2021.037
Yin, A. (2010). Cenozoic tectonic evolution of asia: a preliminary synthesis:a preliminary syn-thesis. Tectonophysics 488 (1/2/3/4), 293–325. doi:10.1016/j.tecto.2009.06.002
Yuan, Z., Li, T., Su, P., Sun, H., Ha, G., Guo, P., et al. (2022). Large surface rupture gaps and low surface fault slip of the 2021 M w 7.4 maduo earthquake along a low activity strike slip fault, Tibetan plateau. Tibetan Plateau. Geophysical Research Letters 49, e2021GL096874. doi:10.1029/2021GL096874
Zachos, J., Pagani, M., Sloan, L., Thomas, E., and Billups, K. (2001). Trends, rhythms, and aberrations in global climate 65 Ma to present. Science 292 (5517), 686–693. doi:10.1126/science.1059412
Zhang, X., and Sagiya, T. (2018). Intraplate strike-slip faulting, stressaccumulation, and shear localization of a crust-upper mantle system with nonlinear viscoelastic material. Journal of Geophysical Research Solid Earth 123, 9269–9285. doi:10.1029/2018JB016421
Zhang, Y. W., Guang, H., Wang, J. F., Wan, X., and Yang, T. (2020). A new growth model of Fault attributes in a strike-slip fault system in the tarim basin. Acta Geologica Sinica (English Edition) 94 (5), 1373–1380. doi:10.1111/1755-6724.14581
Keywords: Andaman sea, strike-slip, Sagaing fault, Mergui Basin, structure
Citation: Guo M, Luan X, Zhang H and He B (2023) Geometric and kinematic analysis of faults bordering the Andaman sea continental shelves: a 3D seismic case study. Front. Earth Sci. 11:1247665. doi: 10.3389/feart.2023.1247665
Received: 26 June 2023; Accepted: 08 August 2023;
Published: 23 August 2023.
Edited by:
Peng Guo, Commonwealth Scientific and Industrial Research Organisation (CSIRO), AustraliaReviewed by:
Jia-jia Yang, Qingdao Institute of Marine Geology (QIMG), ChinaCopyright © 2023 Guo, Luan, Zhang and He. This is an open-access article distributed under the terms of the Creative Commons Attribution License (CC BY). The use, distribution or reproduction in other forums is permitted, provided the original author(s) and the copyright owner(s) are credited and that the original publication in this journal is cited, in accordance with accepted academic practice. No use, distribution or reproduction is permitted which does not comply with these terms.
*Correspondence: Xiwu Luan, eGx1YW5Ac2R1c3QuZWR1LmNu
Disclaimer: All claims expressed in this article are solely those of the authors and do not necessarily represent those of their affiliated organizations, or those of the publisher, the editors and the reviewers. Any product that may be evaluated in this article or claim that may be made by its manufacturer is not guaranteed or endorsed by the publisher.
Research integrity at Frontiers
Learn more about the work of our research integrity team to safeguard the quality of each article we publish.