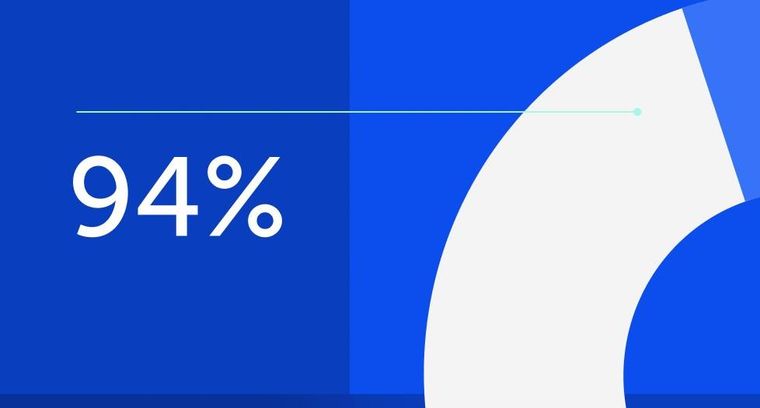
94% of researchers rate our articles as excellent or good
Learn more about the work of our research integrity team to safeguard the quality of each article we publish.
Find out more
ORIGINAL RESEARCH article
Front. Earth Sci., 11 October 2023
Sec. Geoscience and Society
Volume 11 - 2023 | https://doi.org/10.3389/feart.2023.1243975
This study was designed to determine the geotechnical parameters and stability of soils in the Abbottabad region of Khyber-Pakhtunkhwa, Pakistan. The seven major sites with high population density were selected, covering the entire city, which includes Kalapul, Mandian, Jhangi, Nawanshehr, Cantonment, Abbottabad Courts and Jinnahabad. A total of thirty-two (32) boreholes were drilled to a depth of 5 m for standard penetration assessment and thirty two (32) field densification tests were performed at the designated sites. The standard penetration tests were carried out at every meter depth of the bore hole for recording penetration resistance, bearing potential and sample collection. Laboratory tests consisting of Grain-size analysis, Atterberg limits, California Bearing Ratio (CBR), Unconfined Compressive Strength (UCS), Direct Shear Box, and Proctor Compaction were conducted according to the ASTM standards on the accrued samples. The values of the performed tests were utilized for soils characterization and inspecting the Liquidity Index, Consistency Index, and Foundation evaluation for quite a number of footings. The results showed that most of the soils of the area belong to the Clay category (CL and A6 class) with excessive values of Plasticity Index (16.9%–18.6%), Liquidity Index (−47.33% to −23.07%) and Lower CBR (3%–6%), Angle of Internal Friction (15°–20°), UCS (79 kPa–121 kPa), Dry Density (15.13 kN/m3–17.66 kN/m3), Consistency Index (123.07%–147.33%) and Bearing Capacities, except the parts of Kalapul area, which belonged to the GP (poorly graded gravels) category with significant geotechnical properties. Based on the obtained results, we found that the land in the vicinity needs significant improvement before construction. For this purpose, various concentrations of limestone (12.5% and 25%), which is heavily mined in the area, were used as an additive. The results showed that the addition of limestone powder produced a significant improvement in all investigated properties and made the soft soil suitable for construction, in addition to any extended stabilization measures. The main reason for this improvement is the presence of more dense and lower water-absorbing minerals in the limestone than in the ground, or mineralization reactions between them.
A geotechnical investigation is known as an investigation of the physical characteristics of the soil (Sushma, 2009). The majority of the soil’s characteristics are largely dependent on the type of soil, and it plays a significant role in foundation planning. The fine grained soils are usually very difficult to treat and require more care than coarse grained soils. Fine grained soils with a high clay content are commonly referred to as soft soils and create severe problems in the designing of geotechnical structures (Garala and Madabhush, 2018). These soils have a poor compressive strength and high consolidation, and they are usually stiff when they are moisture free and lose their stiffness when they interact with water. This results in a reduction in allowable bearing capacity and increased settlement, causing significant damage to buildings and foundations (McDowell, 1959; Bell, 1996; Venkaramuthyalu et al., 2012; Firozi et al., 2016; Shah et al., 2022a). In earthquake-prone areas, these soils become more challenging because they can increase seismic activity and can significantly enhance the amplitude, frequency, and duration of ground movement that hits the foundations and damages the superstructures (Garala and Madabhushi, 2018). Before starting any construction project on them, it is necessary to identify and stabilize them by conducting site investigations. Nowadays, the stabilization of soft soils through various admixtures including Polysaccharide, Xanthan Gum (XG), Guar Gum (GG), Granite Sand, Calcium Lignosulphonate, Lime, Cement, and Silica Fume, etc., is in practice (Alrubaye et al., 2016a; Alrubaye et al., 2016b; Fattah et al., 2016; Vydehi and Moghal, 2021; Amulya et al., 2022; Al-Gharbawi et al., 2023; Rasheed et al., 2023). This process can increase soil strength, durability and stiffness, and reduces soil plasticity and swelling/shrinkage potential (Hausmann, 1990; Sherwood, 1993; Prusinski and Bhattacharja, 1999; Naeini et al., 2012; Das, 2015).
In recent decades, Pakistan, a South Asian country, has seen so many earthquakes (Sajjad et al., 2014). The reason for this is that Pakistan is located in one of the world’s most seismically active tectonic zones, which includes collision boundaries between the Arabian, Indian, and Eurasian plates. The active and complex tectonic system has caused more than 12 earthquakes of magnitude greater than 6.0–7.6 in the last 30years (Qadri and Malik, 2021). The Kashmir earthquake of magnitude 7.6 in 2005, the Ziarat earthquake of magnitude 6.4 in 2008, the Awaran earthquake of magnitude 7.7 in 2013 and the 2015 Himalayan earthquake of magnitude 7.5, are only a few of the notable recent events (Qadri et al., 2022). These incidents resulted in many deaths and a burgeoning financial loss of billions of dollars, which is very worrying for any developing country (Khaliq et al., 2019; Qadri and Malik, 2021). Following the 2005 Kashmir earthquake, leading scientists and government departments updated the building codes model of Pakistan (BCP-SP 07, 2007).
Abbottabad, a city in the Khyber Pakhtunkhwa (KPK), Province of Pakistan, that is known as a hub of educational institutions and industries was also devastated by the 2005 Kashmir earthquake. The study area is in the red zone with high earthquake chances, according to the Pakistan’s Building Codes. It is also one of the KPK’s most densely populated areas, with rapidly increasing population (Shah et al., 2022b). Scientists have analyzed the seismicity and rocks of this area in detail. The area includes the Jurrasic (Samana suk formation), Cretaceous (Chichali, Lumshiwal, and Kawagarh formations), Palaeocene (Hangu and Lockhart formations), and Eocene sequences (the Nammal and Sakessar formations) (Umar et al., 2015; Akhtar et al., 2019). The area is a part of the fold-and-thrust belt, and has undergone significant deformation, leading to the construction of a large thrust faulting system. The Bhagnotar and Nathiagali faults are the main thrust faults in the area. The Bhagnotar thrust is the major one, where the Hazara formation of the Pre-cambrian age is pushed over the Samana suk formation of the Jurassic period (Akhtar et al., 2019). However, the detailed investigation regarding the soils of the study area is still missing, which is one of the major gaps in the literature. The classification of the soils and the identification of clays can be extremely helpful in understanding the geology of the area in more detail. A detailed soils classification information provides useful insight to the residents of the area to properly plan their building foundations. This is particularly significant in a high seismic zone due to the presence of many faults, which can severely affect the clay soils. The residents of the study area are mainly small-scale business persons, farmers, and self-employed workers, those cannot afford the hefty cost of the heavy foundations made up of huge amounts of steel bars, etc. Therefore it is imperative to identify the clay soil zones in the study area and provide a cost-effective way of stabilizing them to reduce the heavy foundation expenditures and also the extent of earthquake-related structural damage. Keeping this in view, the present study is designed to; (a) classify the soils of all major areas of Abbottabad city with proper foundation analysis and, (b) to explore the potential of limestone powder in stabilization of the weak soils of the area.
Recent studies show that limestone can enhance the geotechnical parameters of clay soils. Muhiddin et al., 2019; Ibrahim et al., 2020; Abdulrahman et al., 2022; Agrawal and Gupta, 2011; Pastor et al., 2019; Tanzadeh et al., 2021, utilized the limestone powder in their studies to stabilize the weak soils. Results showed that limestone powder effectively improves the Atterberg Limits, Compaction parameters, UCS, CBR, swelling potential, and Activity, etc., at significant levels. However, these studies have not analyzed the effect of limestone powder on some of the key segments in foundation design like Ultimate and Allowable bearing capacities of clay soils for various foundations, including strip footing, square footing and rectangular footing. The investigation of other parameters like cohesion, angle of internal friction, Liquidity Index, Consistency Index are also lacking in the literature. The deep bearing capacity and foundation analysis by considering all the factors, including 1) shear strength parameters, 2) compressive strength, 3) shape, depth and inclined load factors, were also limited in stabilization studies, in which other admixtures including lime, cement, fly ash, egg shells, rock powders, etc., were utilized for clay stabilization. Keeping in view these gaps, the limestone powder was chosen for stabilization, and we analyzed the suitability of limestone powder on least investigated parameters. Apart from that, various limestones were exposed in the Abbottabad region, including Samana Suk, Lockhart, Kawagarh, etc. (Umar et al., 2016), and are heavily used in the construction industry in the form of coarse aggregate and also as a decorative stone. During processing, a huge quantity of limestone waste powder is dumped into the Dor River, posing a serious environmental concern. In the present study we explored the application of limestone powder in soils stabilization as it is readily available and requires sustainable management. This work will be a great addition to the existing literature, and will help the engineers to understand the effect of limestone powder on clay’s behavior. The methodology adopted for this work will also be very beneficial for the researchers who are actively working on ground improvements through sustainable and cost-effective techniques.
To narrow down the areas of Abbottabad, covering the entire city, a detailed field visit was undertaken. The selected areas include; Kalapul (with two locations of Abbottabad Heights and Frontier Medical College), Mandian, Cantonment area, Jhangi, Nawanshehr, Jinnahabad, and Abbottabad Courts vicinity area (Figure 1). These areas form the heart of the Abbottabad city, with a dense population, multistory buildings, military installations, and educational institutes. Next, a total of thirty two (32) bore holes were dug in all the selected areas, each having 5 m depth. Consequently, one hundred and sixty (160) Standard Penetration Tests (SPT) were carried out to record the Penetration Resistance (known as “N” values) with the assistance of Hanan Tech Pakistan Private Limited (Geotechnical and Environmental Consultants at District Mansehra, Pakistan). The Meyerhof equations were utilized to estimate allowable bearing capacities by using the average “N” values of 1 and 2 m depth.
For footing width, 4 feet or less (This equation was utilized for 1 m depth with width of 1.2 m).
For footing width, greater than 4 feet (This equation was utilized for 2 m depth with width of 2.4 m).
Where Qa is the Allowable soil bearing capacity in kips/ft2. N: Penetration Resistance. K = 1 + 0.33(D/B) ≤ 1.33. D: footing depth. B: footing width.
After SPT, a total of thirty two (32) Field Density Tests (FDT), five experiments on a single site, using the sand replacement technique, were performed to measure dry densities and moisture contents. Both SPT and FDT were performed according to ASTM D1586 and ASTM D1556, respectively. The various samples collected from these experiments were then sent to the laboratory for further analysis.
The following tests were performed on the soil samples collected from the field. Each test was conducted on forty samples (5 samples from every site) in the Laboratory and the average values were reported and utilized for further calculations.
1. For the purpose of knowing the exact percentages of gravel, sand, silt, and clay, sieve analysis and hydrometer analysis were conducted according to ASTM C136 and ASTM D7928 respectively.
2. For determining the liquid limit, plastic limit, and plasticity index, atterberg limits tests were conducted through ASTM D4318. The values of Atterberg limits were further used for calculating Liquidity Index and Consistency Index by following the equations used by Vardanega and Haigh’s, 2014; Oliveira et al., 2019 respectively in their studies.
Where LI and CI are Liquidity and Consistency Index.
W is the moisture content (Natural moisture content values were used for untreated samples, and OMC values were used for treated samples), LL and PL are Liquid Limit and Plastic Limit respectively, and PI is the Plasticity Index.
3. Strength parameters such as Soaked California Bearing Ratio (CBR), Unconfined Compressive Strength (UCS), cohesion, and angle of internal friction were determined by following the rules established by ASTM D1883, ASTM D2166, and ASTM D3080 respectively. Next, the values of compaction parameters, including Optimum Moisture Contents (OMC) and Maximum Dry Density (MDD), were obtained by proctor compaction test by following the steps provided by ASTM D 698. The standards of all the field and laboratory conducted tests are also presented in Table 1.
All studied regions were classified using AASHTO and the Unified Standards. Atterberg limits and particle size values were used in this classification. After this, the soils of the areas, which were classified as clayey soils were selected for stabilization with powdered limestone.
Limestone samples were collected from Murree Road, Abbottabad. The collected samples were limestone waste obtained as a by-product during the production of aggregates. Thin sections were prepared and analyzed under polarizing microscope for determining the mineralogical contents. Results showed that limestone is predominantly made up of calcite. Next, the samples were crushed to fine powder.
Various soil samples having high clay content were prepared with varying concentrations of powdered limestone (Table 2). Tests such as Atterberg limits, CBR, UCS, Proctor compaction and Direct shear box were performed on all prepared samples according to ASTM procedures. Liquidity and Consistency Index values were then determined using the methods described in Section 3.2.
Following the completion of the whole testing process, the allowable bearing capacity values of all treated and untreated samples were determined for strip, square, and rectangular footings of both vertical and inclined loads using Meyerhof equations (Meyerhof, 1951) provided below. For projects on flat sites, vertical load analysis is crucial, while inclined load analysis is for projects on sloped or non-flat sites.
For Vertical load Analysis:
For Inclined load Analysis:
Where:
Nc, Nq, Nγ are the bearing capacity factors, and determined by using following formulas provided by Meyerhof.
Sc, Sq, Sγ & Dc, Dq, Dγ are shape and Depth factors respectively and.
Ic, Iq, Iγ are incline load factors. These were determined by using the Meyerhof equations provided in Table 3.
C: Cohesion of soil
γ: unit weight of soil (Dry density values were used for untreated samples, and MDD values were used for treated samples),
D: depth of footing.
B, L: width and length of footing.
Kpr = tan2 (45+φ/2), passive pressure coefficient.
θ = angle of axial load to vertical axis.
Bearing capacities of three various footings including Strip, Square and Rectangular were determined through these equations by using various length, shape and depth dimensions that are currently in practice (Table 4). These dimensions were decided after detailed discussion with Structural Engineer.
For obtaining the Allowable Bearing Capacity values, the Ultimate Bearing Capacities were divided with Factor of Safety (FOS). The used value of FOS was 3.
The complete results of "N" values and bearing capacities are shown in Figures 2–10, respectively. The average "N" values of Abbottabad Heights and Frontier Medical College soils of Kalapul site ranged between 57.25–66.00 and 50.25–65.25 respectively, and the bearing capacities of 570 kPa and 471 kPa for 1 m depth, and 763 kPa and 691 kPa for 2 m depth respectively. For all other investigated areas, the “N” values and bearing capacities were lower than 16 and 200 kPa respectively (Figures 2–10). In Figure 11, the average values of dry density and natural moisture content are shown. Overall, thirty two (32) Field Density tests were conducted to record these parameters, and the average values were reported. For the Abbottabad Heights and Frontier Medical College area (Kalapul site), the dry density values were higher than 21 kN/m3 and lower than 18 kN/m3 for all other investigated sites (Figure 11).
Grain size analysis results are shown in Figure 12. More than 60% of the silt and clay sized particles were found in the soils of Mandian, Jhangi, Nawanshehr, Abbottabad Courts, Cantonment, and Jinnahabad, while Frontier Medical College and Abbottabad Heights soils of Kalapul area were found to be mainly sands and gravels as compared to clay. Figure 13 show the results of Atterberg limits. The soils with a high clay content had higher percentages of the liquid limit, the plastic limit, and the plasticity index, while the poor clay soils had non-plastic behavior. For the determination of consistency and liquidity index, the atterberg limits of clay rich areas were used and the results are shown in Figure 13.
FIGURE 12. Grain size analysis of studied soils (Mandian area (A), Cantonment area (B), Jhangi area (C), Nawanshehr area (D), Abbottabad Courts area (E), Jinnahabad area (F), Abbottabad Heights area (G), and Frontier Medical College area (H).
FIGURE 13. Average values of Plasticity Index, Consistency Index, Liquidity Index, Plastic Limit and Liquid Limit of Abbottabad area clays.
The soils of Mandian, Jhangi, Nawanshehr, Abbottabad Courts, Cantonment, and Jinnahabad are a part of fine grained soils, and classified as “CL” soils through Unified classification system (Figure 14), whilst the soils of Abbottabad Heights and Frontier Medical College of Kalapul area are in the coarse grained group, and are categorised as “GP” soils. CL soils are described as “inorganic clays of low to medium plasticity, gravely clays, silty clays, sandy clays, lean clays” and GP soils are referred to as poorly graded gravels, gravels-sandmixtures, little or no fines in accordance to the unified classification criteria.
According to AASHTO standards, the soils of all the above referred areas, which belong to the “CL” group, are classified as “A6” soils (Figure 15), which are known as clay soils of poor strength. The soils of Abbottabad Heights and the Frontier Medical College zone are part of the A1 group of AASHTO classification (Figure 15), which are referred to as stone fragments, gravels and sand of excellent to good strength (Figure 15).
The results of strength parameters such as CBR, UCS, and shear strength (cohesion and angle of internal friction) are shown in Figure 16. The values of CBR, UCS, and angle of internal friction were higher in the Kalapul region, except for cohesion, while these values were much lower in all other investigated areas (Figure 16).
FIGURE 16. Average values of UCS, CBR, angle of internal friction and cohesion of Abbottabad area soils.
The limestone was added to the soils of areas such as Mandian, Jhangi, Nawanshehr, Cantonment, Abbottabad Courts, and Jinnahabad, where the percentage of silt and clay was higher with very poor Geotechnical properties, and they required significant improvement. In Table 5, it is clearly seen that the limestone addition, improved all the studied parameters significantly. It increased the values of maximum dry density, CBR, UCS, angle of internal friction and consistency index, and decreased the liquid limit, plasticity index, cohesion, liquidity index and OMC (Table 5). The effect of limestone addition to plastic limit of the soils was found to be very minor.
TABLE 5. Geotechnical properties of Abbottabad area clays before and after treatment with limestone powder.
All stabilization studies have the primary aim of improving the soil conditions for a safe foundation structure. In Tables 6–8, the complete foundation analysis of the soils with/without limestone treatment for the Strip, Square, and Rectangular footings is provided. The information regarding the bearing capacity factors is given in Table 6, and the values of the shape, depth, and inclined load parameters for all the footings are shown in Table 7. All these factors have pronounced influence on the values of bearing capacity and it cannot be ignored in determining the values of allowable strength, as the distribution of superstructure forces is completely dependent on them. In Table 8, the complete allowable bearing capacity values of all the studied soils are provided. Based on Table 8 results, the allowable bearing capacity of Kalapul area was higher, which required no modification, while the values for all other areas were much lower. However, the addition of limestone up to 25 percent increased these low bearing capacities for all the footings very well (Table 8).
TABLE 6. Bearing Capacity factors for Abbottabad area soils before and after treatment with limestone powder.
TABLE 7. Shape, Depth and Inclined load factors of various footings for Abbottabad area soils before and after treatment with limestone powder.
TABLE 8. Allowable Bearing Capacities (Qa) in kPa of various footings for Abbottabad area soils before and after treatment with limestone powder.
Geotechnical investigations are really necessary to analyze the underlying ground soil before starting any mission to construct on it. Knowledge about the index and engineering properties of soil plays a vital role in understanding the behavior of soil and its use as a building, road, or different construction material (Sushma, 2009; Garala and Madabhushi, 2019; Rehman et al., 2021; Shah et al., 2021; Zaheer et al., 2021; Shah et al., 2022a; Shah et al., 2022c). Most of the soils of the Abbottabad city besides the Kalapul location belong to group A 6 (clay soils of poor strength), whilst the Kalapul soils fall into class A-1, which is known as stone fragments, gravels and sand of excellent to good strength by the AASHTO classification system (Figure 15). On the basis of the Unified classification (Figure 14), Abbottabad clay is categorized as CL soil, which is known as low to medium plastic clay, which means that the chances of swelling of this soil are less. However, it includes smaller grain dimensional particles, i.e., more than 60 percentage of the material is smaller than seventy-five microns. This size can lead to extreme settlement/consolidation problems due to heavy construction loads. The values of liquid limit, plasticity index, and optimum moisture contents were notably excessive in Abbottabad clay (Figure 13), which in turn increase the Liquidity Index, and diminished the Consistency Index. Both of these parameters are very important to understand the clay behavior. The LI is a measure of the extent of closeness of the soil moisture content to its LL. Where the soil is at its LL, the value of its LI is 100% and the soil acts as a liquid. In contrast, zero LI means that the soil is at its PL, while negative values of LI indicate that the water content of soil is lower than its PL and so the soil is hard. The soil with zero CI means that it is in the liquid state and thus very soft and weak, whereas soil possessing high (e.g., 100) CI indicates that it is in the plastic state, that is, it is firmer/stronger. The soil becomes strong when its CI exceeds 100; increases in CI beyond 100 increases the strength of the soil significantly (Arora, 2000; Budhi, 2010). The obtained results of LI and CI indicated that the Abbottabad clays are currently in the hard state, but these values are very nearer to the plastic stage, which means that any small increase in moisture content may cause the excessive settlements of the structures build on it. On the other hand, the CBR, UCS, density, angle of internal friction, bearing capacity, and penetration resistance are lower in these clays due to the presence of smaller grain-sized particles in large proportions. It is regarded that soil with such grain size absorbs a giant quantity of water, which increased plasticity parameters, diminished CBR, UCS, shear strength, dry density, angle of internal friction, and overall bearing ability of various vertical and inclined loads. On the basis of the outcomes obtained, Abbottabad soils require substantial enhancements before they can be used for building, development, and sub-base materials. The area is also a part of an excessive seismic zone (Umar et al., 2015; Akhtar et al., 2019), which is another challenging task to construct on the soils of this region, because clays can enhance earthquake activity due to its higher potential to increase amplitude, frequency, and duration of ground movement that badly effects the foundations and buildings (Garala and Madabhushi, 2018). In addition, water drainage issues are also very frequent in the city. In the rainy season, water in the location starts to flow onto the roads and grounds, which may also cause massive losses to the buildings erected on them, as the soil has higher plasticity and lower strength parameters. As exists in Pakistan, the Geotechnical engineers normally recommend raft footing for masonry in areas like Abbottabad, which has many active faults and higher clay contents to prevent differential settlement of individual feet. These risks have made the study area unsuitable for other shallow foundations, including strip, square and rectangular, etc. The raft base is designed as a single mat (or common base) for all the load-bearing elements of the structure. It includes a huge amount of steel bars, which makes it very expensive and unaffordable for the people of the area due to their low economic conditions.
The addition of up to 25% limestone powder changed the Abbottabad clays category to A4-type and MH type from A6 and CL type respectively. The strength of A4 and MH soils is considered to be fair according to AASHTO and Unified standards. This addition results in a reduction in the Plasticity Index (from 16.9%–18.6% to 3.6%–8.3%), Liquid Limit (from 30.21%–34.9% to 21.0%–26.6%), Liquidity Index (from −47.33%–−23.07% to −202.7%–−63.8%), and enhancement in soil UCS (from 79 kPa–121 kPa to 101 kPa–145 kPa), soaked CBR (from 3%–6% to 9%–13%), Consistency Index (from 123.07%–147.33% to 163.8%–308.3%), angle of internal friction (from 15°–20° to 19°–25°), density (from 15.13 kN/m3–17.66 kN/m3 to 16.85 kN/m3–19.40 kN/m3) and bearing capacities for various footings and loads including strip, square and rectangular. Similar findings were also achieved by Pastor et al., 2019, Ibrahim et al., 2020; Muhiddin et al., 2019 etc. According to Pastor et al., 2019, the addition of limestone up to 25%, significantly reduces the Liquid Limit, Plastic Limit, Swelling potential and Compressibility, and it can enhance the unconfined compressive strength very effectively. The study conducted by Ibrahim et al., 2020, indicated that the 18% limestone percentage is the most effective one to reduce the Atterberg Limits, Optimum moisture contents, void ratio and swelling potential of clay soil, and increases the UCS and Maximum Dry Density (MDD). The results of study conducted by Muhiddin et al., 2019, shows that the 30% limestone addition enhances the UCS, CBR and MDD and diminishes the OMC, and Atterberg limits. All of these scientists have not determined the effect of limestone addition on the clays liquidity index, consistency index, shear strength, and bearing capacities for various foundations.
This overall improvement and conversion of clay soil class to silt class due to the addition of limestone (Figures 14, 15) will help to reduce the strength and speed of the seismic waves to reach the surface to impact the foundations in earthquakes, which in turn will allow people of the area to build square/rectangular foundations instead of mat/raft foundation. Each of these supports is based on columns reinforced with steel bars and concrete. The quantity of steel billets required in these is much less compared to a raft, which makes them inexpensive, because steel billets per ton are currently priced in Pakistan at around 1071 USD. This will make the construction process very cost-effective in the city.
The main reasons for the reduction of clay plasticity parameters with the limestone addition is its low water adoring minerals, including calcite, etc. The activity value of calcite is 0.33 (Moos, 1938; Shah et al., 2022b), which shows their reduced water absorption capacity. The increase in soil strength parameters (Compressive and Shear), CBR, consistency index, etc., can be attributed to the higher density of limestone than clay or the reaction between clay and limestone minerals or grain size variations between admixture and the clay soil.
This article discusses the Geotechnical parameters and stability of soils in Abbottabad, Pakistan. Limestone powder is used as a strengthening additive. The conclusions are drawn as follows.
1. Most of the soils of Abbottabad belong to the A6 and CL group except for the Kalapul area, which is in the A1 and GP category.
2. Abbottabad clay has high values of plasticity index, liquid limit, optimum moisture content, and liquidity index, while Kalapul soil was labelled as non-plastic soil with low optimum moisture content and liquidity index.
3. Clays of Abbottabad area has lower values of CBR, UCS, consistency index, maximum dry density, internal friction angle, and bearing capacities values of strip, square and rectangular footings for various vertical and inclined loads, and penetration resistance, whilst the soils of Kalapul region has very high values for all these parameters except soil cohesion.
4. The addition of limestone waste powder to Abbottabad clay effectively improved clay properties by:
a) Escalating CBR, UCS, CI, internal friction angle and all bearing capacities of different foundations.
b) Significantly reducing the liquid limit, plasticity index, optimum moisture contents, and liquidity index.
In general, this research will be useful for the local community to format the foundations of buildings, and to explore other inexpensive foundations instead of Raft after significant limestone treatment. Likewise, the use of limestone waste will also decrease the negative environmental impacts and gain monetary and environmental advantages from its reuse. Moreover, this work will benefit engineers, people and the scientific neighborhood around the world to gain in depth understanding of the impacts of limestone powder application on the clay parameters.
The original contributions presented in the study are included in the article/Supplementary material, further inquiries can be directed to the corresponding author.
This study was designed and completed by SS (Lecturer, Department of Earth and Environmental Sciences, Hazara University, Mansehra, Pakistan) as the principal investigator. He prepared the initial and final draft of the paper. RS, AJ, and FA played a significant role in the field and laboratory work along with SS. FA also helped with bearing capacity analysis and foundation recommendations. UH and AM helped with manuscript writing, proof reading, improvement in figures and foundation analysis. All authors contributed to the article and approved the submitted version.
The author(s) declare that no financial support was received for the research, authorship, and/or publication of this article.
The study is partially funded by Future University in Egypt.
The authors declare that the research was conducted in the absence of any commercial or financial relationships that could be construed as a potential conflict of interest.
All claims expressed in this article are solely those of the authors and do not necessarily represent those of their affiliated organizations, or those of the publisher, the editors and the reviewers. Any product that may be evaluated in this article, or claim that may be made by its manufacturer, is not guaranteed or endorsed by the publisher.
Abdulrahman, S. M., Al-Gharbawi, A. S. A., Ammar, A., and Al-Sultan, A. A. (2022). Effect of engineering properties of soft clay soil stabilized with limestone, eggshells powder and eggshells ash. Water Environ. Sustain. (WES), 68–75. doi:10.52293/WES.3.2.6875
Agrawal, V., and Gupta, M. (2011). Expansive soil stabilization using marble dust. Int. J. Earth Sci. Eng. 4, 974–5904.
Akhtar, S., Rahim, Y., Hu, B., Tsang, H., Ibrar, K. M., Ullah, M. F., et al. (2019). Stratigraphy and structure of dhamtaur area, District Abbottabad, eastern Hazara, Pakistan. Open J. Geol. 9, 57–66. doi:10.4236/ojg.2019.91005
Al-Gharbawi, , Ahmed, S. A., Najemalden, Ahmed M., and Fattah, Mohammed Y. (2023). Expansive soil stabilization with lime, cement, and Silica Fume. Appl. Sci. 13 (1), 436. doi:10.3390/app13010436
Alrubaye, A. J., Hasan, M., and Fattah, M. Y. (2018). Effects of using Silica Fume and lime in the treatment of kaolin soft clay. Geomechanics Eng. 14 (3), 247–255. doi:10.12989/gae.2018.14.3.247
Alrubaye, A. J., Hasan, M., and Fattah, M. Y. (2016b). Engineering properties of clayey soil stabilized with lime. ARPN J. Eng. Appl. Sci. 11 (4), 2434–2441.
Alrubaye, A. J., Hasan, M., and Fattah, M. Y. (2016a). Improving geotechnical characteristics of kaolin soil using Silica Fume and lime. Special Top. Rev. Porous Media Int. J. 7 (1), 77–85. doi:10.1615/SpecialTopicsRevPorousMedia.v7.i1.70
Amulya, G., Moghal, A. A. B., Basha, B. M., and Almajed, A. (2022). Coupled effect of granite sand and Calcium lignosulphonate on the strength behavior of cohesive soil. Buildings 12 (10), 1687. doi:10.3390/buildings12101687
Arora, K. R. (2000). “Soil mechanics and foundation engineering,” in SI units (New Delhi, India: Standard Publishers Distributions).
ASTM C136/C136M (2019). Standard test method for sieve analysis of fine and coarse aggregates. West Conshohocken, PA, USA: ASTM International.
ASTM D1556 (2007). Standard test method for density and unit weight of soil in place by the sand-cone method. West Conshohocken, PA, USA: ASTM International.
ASTM D1586 (2008). Standard test method for standard penetration test (SPT) and split-barrel sampling of soils. West Conshohocken, PA, USA: ASTM International.
ASTM D1883 (1999). Standard test method for CBR (California bearing ratio) of laboratory compacted soils. West Conshohocken, PA, USA: ASTM International.
ASTM D2166-06 (2006). Standard test method for unconfined compressive strength of cohesive soil. West Conshohocken, PA, USA: ASTM International.
ASTM D3080/D3080M (2011). Standard test method for direct shear test of soils under consolidated drained conditions. West Conshohocken, PA, USA: ASTM International.
ASTM D4318 (2000). Standard test methods for liquid limit, plastic limit and plasticity index of soils. West Conshohocken, PA, USA: ASTM International.
ASTM D698 (2000). Standard test method for laboratory compaction characteristics of soil using standard effort. West Conshohocken, PA, USA: ASTM International.
Astm D7928, (2017). Standard test method for particle size distribution (gradation) of fine-grained soils using the sedimentation (hydrometer) analysis. West Conshohocken, PA, USA: ASTM International.
Bcp-Sp 07, (2007). Building code of Pakistan. Islamabad, Pakistan: Ministry of Housing. Islamic Republic of Pakistan.
Bell, F. (1996). Lime stabilization of clay minerals and soils. Eng. Geol. 42 (4), 223–237. doi:10.1016/0013-7952(96)00028-2
Budhi, M. (2010). Soil mechanics and foundations. 3rd edn. Hoboken, New Jersey, United States: Wiley & Sons, INC.
Das, B. M. (2015). Principles of foundation engineering. Boston, Massachusetts, United States: Cengage learning.
Fattah, M. Y., Al-Saidi, A. A., and Jaber, M. M. (2015). Characteristics of clays stabilized with lime-silica Fume mix. Italian J. Geosciences 134 (1), 104–113. doi:10.3301/IJG.2014.36
Firoozi, A. A., Olgun, G., Firoozi, A. A., and Baghini, M. (2017). Fundamentals of soil stabilization. Int. J. Geo-Engineering 8, 26. doi:10.1186/s40703-017-0064-9
Garala, T., and Madabhushi, G. (2019). Seismic behaviour of soft clay and its influence on the response of friction pile foundations. Bull. Earthq. Eng. 17, 1919–1939. doi:10.1007/s10518-018-0508-4
Hausmann, M. R. (1990). Engineering principles of ground modifcation. New York, New York, United States: McGraw-Hill.
Ibrahim, H. H., Alshkane, Y. M., Mawlood, Y. I., Noori, K. M. G., and Hassan, A. M. (2020). Improving the geotechnical properties of high expansive clay using limestone powder. Innov. Infrastruct. Solut. 5, 112. doi:10.1007/s41062-020-00366-z
Islam, M. (2023). Terzaghi bearing capacity equations. https://www.academia.edu/11175107/Terzaghis_Bearing_Capacity_Equations.
Khaliq, A. H., Waseem, M., Khan, S., Ahmed, W., and Khan, M. A. (2019). Probabilistic seismic hazard assessment of Peshawar District, Pakistan. J. Earth Syst. Sci. 128, 6. doi:10.1007/s12040-018-1028-y
McDowell, C. (1959). Stabilization of soils with lime, lime-fly ash, and other lime reactive materials. Highw. Res. Board Bull. 231.
Meyerhof, G. G. (1951). The ultimate bearing capacity of foudations. Geotechnique 2, 301–332. doi:10.1680/geot.1951.2.4.301
Moos, A. V. (1938) “Geotechnical properties and investigation methods of loose rocks; earthwork course of E T H” in No 4 (zurich); faculty of science, London, UK: University of London
Muhiddin, A., Harianto, T., Arsyad, A., and Indrayanti, (2019). Experimental study on clay stabilization with waste limestone from marble industri. Lowl. Technol. Int. 21, 172–186.
Naeini, S. A., Naderinia, B., and Izadi, E. (2012). Unconfined compressive strength of clayey soils stabilized with waterborne polymer. KSCE J. Civ. Eng. 16 (6), 943–949. doi:10.1007/s12205-012-1388-9
Oliveira, D. G. G., Thewes, M., Diederichs, M. S., and Langmaack, L. (2019). Consistency index and its correlation with EPB excavation of mixed clay–sand soils. Geotech. Geol. Eng. 37 (1), 327–345. doi:10.1007/s10706-018-0612-x
Pastor, J. L., Tomás, R., Cano, M., Riquelme, A., and Gutiérrez, E. (2019). Evaluation of the improvement effect of limestone powder waste in the stabilization of swelling clayey soil. Sustainability 11, 679. doi:10.3390/su11030679
Prusinski, J., and Bhattacharja, S. (1999). Effectiveness of portland cement and lime in stabilizing clay soils. Transp. Res. Rec. J. Transp. Res. Board 1652, 215–227. doi:10.3141/1652-28
Qadri, S. M. T., and Malik, O. A. (2021). Establishing site response based microzonation by applying machine learning techniques on ambient noise data: A case study from northern potwar region, Pakistan. Environ. Earth Sci. 80, 53. doi:10.1007/s12665-020-09322-7
Qadri, S. M. T., Mirza, M. Q., Raja, A., Yaghmaei-Sabegh, S., Hakimi, M. H., Ali, S. H., et al. (2023). Application of probabilistic seismic hazard assessment to understand the earthquake hazard in attock city, Pakistan: A step towards linking hazards and sustainability. Sustainability 15, 1023. doi:10.3390/su15021023
Rasheed, R. M., Moghal, A. A. B., Rambabu, S., and Almajed, A. (2023). Sustainable assessment and carbon footprint analysis of Polysaccharide biopolymer-amended soft soil as an alternate material to canal lining. Front. Environ. Sci. 11, 1214988. doi:10.3389/fenvs.2023.1214988
Rehman, Q., Ahmed, W., Waseem, M., Khan, S., Farid, A., and Shah, S. H. A. (2021). Geophysical investigations of a potential landslide area in mayoon, hunza District, gilgit-baltistan, Pakistan. Rud. Geolosko Naft. Zb. 36 (3), 127–141. doi:10.17794/rgn.2021.3.9
Sajjad, S. H., Waheed, S. A., Khan, T., Qadri, S. M. T., and Gilani, N. (2014). Natural Hazards and related contents in curriculum of Geography in Pakistan. Asian J. Nat. Appl. Sci. 3, 40–48.
Shah, S. H. A., Arif, M., Sabir, M. A., Tanoli, J. I., Saleem, A., and Lodhi, H. T. (2022c). The effect of weathering on the appropriateness of granite for clay stabilization. Acta Geotech. Slov. 1, 48–55.
Shah, S. H. A., Arif, M., ur Rehman, Q., and Manzoor, F. (2021). Utilization of dolerite waste powder for improving geotechnical parameters of compacted clay soil. Open Geosci. 13 (1), 1523–1535. doi:10.1515/geo-2020-0323
Shah, S. H. A., Habib, U., Mohamed, A., Aziz, M., Rehman, Q. u., and Saleem, A. (2022a). Laboratory and in situ stabilization of compacted clay through granite waste powder. Sustainability 14, 14459. doi:10.3390/su142114459
Shah, S. H. A., Tanoli, J. I., Habib, U., Rehman, Q. u., Ali, E., and Mohamed, A. (2022b). Assessing potential of Dor River as small hydro project for lessening energy crisis and enhancing tarbela reservoir life in khyber Pakhtunkhwa, Pakistan. Water 14, 2683. doi:10.3390/w14172683
Sherwood, P. (1993). Soil stabilization with cement and lime. Crowthorne, Wokingham, UK: Transport Research Laboratory.
Sushma, B. V. (2009). Importance of reliable geotechnical investigation for safe and economical foundation design of civil structures. Guntur, India: IGC.
Tanzadeh, R., Vafaeian, M., and Fard, M. Y. (2021). The influence of lime powder on the behavior of clay soil. Gradevinar 73 (9), 907–915.
Umar, M., Sabir, M. A., Farooq, M., Khan, M. M. S. S., Faridullah, F., Jadoon, U. K., et al. (2015). Stratigraphic and sedimentological attributes in Hazara basin lesser himalaya, north Pakistan: Their role in deciphering minerals potential. Arab. J. Geosci. 8, 1653–1667. doi:10.1007/s12517-014-1322-1
Vardanega, P. J., and Haigh, S. K. (2014). The undrained strength – liquidity index relationship. Can. Geotech. J. 51, 1073–1086. doi:10.1139/cgj-2013-0169
Venkaramuthyalu, P. K., Ramu, , and Raju, G. P. (2012). Study on performance of chemically stabilized expansive soil. Int. J. Adv. Eng. Technol. 2, 139–148.
Vydehi, K. V. V., and Moghal, A. A. B. (2021). Effect of biopolymeric stabilization on the strength and compressibility characteristics of cohesive soil. J. Mater. Civ. Eng. 34 (2). doi:10.1061/(asce)mt.1943-5533.0004068
Keywords: foundations, liquidity index, consistency index, stabilization, mineralogical reactions
Citation: Shah SHA, Sajjad RU, Javed A, Habib U, Ahmad F and Mohamed A (2023) Geotechnical investigation and stabilization of soils through limestone powder at Abbottabad, Khyber-Pakhtunkhwa, Pakistan: a cost effective and sustainable approach. Front. Earth Sci. 11:1243975. doi: 10.3389/feart.2023.1243975
Received: 21 June 2023; Accepted: 31 July 2023;
Published: 11 October 2023.
Edited by:
Marcelo Cohen, Federal University of Pará, BrazilReviewed by:
Arif Ali Baig Moghal, National Institute of Technology Warangal, IndiaCopyright © 2023 Shah, Sajjad, Javed, Habib, Ahmad and Mohamed. This is an open-access article distributed under the terms of the Creative Commons Attribution License (CC BY). The use, distribution or reproduction in other forums is permitted, provided the original author(s) and the copyright owner(s) are credited and that the original publication in this journal is cited, in accordance with accepted academic practice. No use, distribution or reproduction is permitted which does not comply with these terms.
*Correspondence: Syed Husnain Ali Shah, c2hhcy5odXNuYWluQGdtYWlsLmNvbQ==, c3llZGh1c25haW5AaHUuZWR1LnBr
Disclaimer: All claims expressed in this article are solely those of the authors and do not necessarily represent those of their affiliated organizations, or those of the publisher, the editors and the reviewers. Any product that may be evaluated in this article or claim that may be made by its manufacturer is not guaranteed or endorsed by the publisher.
Research integrity at Frontiers
Learn more about the work of our research integrity team to safeguard the quality of each article we publish.