- 1Sichuan Communication Surveying and Design Institute Co., Ltd., Chengdu, China
- 2School of Civil Engineering, Sichuan Vocational and Technical College of Communications, Chengdu, China
The environment of the roadbed and pavement often has a significant impact on its dynamic performance. The stability of the strata in the Hilly Area is poor, and long-term complex environmental impacts will cause significant damage to the pavement. This article tests the dynamic response characteristics of semi rigid and inverted asphalt pavement through road load tests, and measures the humidity data of the roadbed during on-site rainfall. In addition, the variation of pore water pressure in the transition layer under the coupling effect of humidity and dynamic load was analyzed, revealing the influence of seasonal factors on the dynamic response of the pavement and roadbed. The test results indicate that the humidity inside the roadbed is greatly influenced by seasonal factors, and the humidity conditions of the roadbed and pavement vary significantly due to differences in measurement point depth, season, and rainfall. Graded crushed stone cushion is beneficial for improving the humidity conditions of the roadbed. The pore water pressure of the graded crushed stone transition layer did not show significant pore water reabsorption throughout the entire loading process. Meanwhile, the thickness of the surface layer and the magnitude of the load have a significant impact on the measurement of pore water pressure in the transition layer. The measured values of the dynamic response indicators of the pavement are greatly influenced by seasonal factors. The research in this article will provide theoretical and guiding significance for the dynamic response characteristics of pavement under the influence of multiple factors in the southwestern hilly area.
1 Introduction
The dynamic modulus, viscoelasticity and damping characteristics of asphalt mixtures are all-sensitive to temperature. The strength and permanent deformation characteristics of graded crushed stone and soil foundation are greatly affected by the dry and wet conditions, and the environment has a significant impact on the material properties and the response behavior of the pavement.
The research on the dynamic response of temperature to asphalt pavement has two main parts. 1) The dynamic modulus has temperature-dependent characteristics. When the dynamic modulus of the asphalt mixture is higher at low temperature than that is at high temperature, the influence of the research temperature on the bottom strain of the asphalt layer and the vertical earth pressure on the top surface of the subgrade is studied. 2) Temperature fluctuations in the asphalt mixture cause temperature shrinkage and expansion, and temperature strain is formed at the bottom of the asphalt layer (Garg and Hayhoe, 2001; Al-Qadi et al., 2004; Islam and Tarefder, 2013; Assogba et al., 2020; Gao, et al., 2023). However, it is not reasonable to use the temperature index to characterize the seasonal variation on the dynamic response of asphalt pavement. Meanwhile, there has been relatively little research on the dynamic response of asphalt pavement to seasonal temperature changes. This article studies the dynamic response characteristics of asphalt pavement under different seasonal temperature changes.
The humidity conditions inside the pavement structure change with the seasons, and there are significant differences in the internal humidity conditions of the pavement structure in different seasons. The inverted asphalt pavement is particularly obvious. The difference in dynamic response of pavement under different season conditions is the combined effect of temperature and humidity changes in the pavement structure. It is unreasonable to use the temperature index to characterize the seasonal variation in the literature (Ai et al., 2017; Chen et al., 2019; Zhang et al., 2023). The research on the dynamic response characteristics of the inverted asphalt pavement is mainly limited to the response characteristics of the asphalt layer and the soil foundation. There is little research on the response characteristics of the graded crushed stone transition layer based on the on-site vehicle loading test. In addition, the graded crushed stone belongs to the porous composite material. Under the load of the vehicle, the moisture in the transition layer of the graded crushed stone generates transient pulsating water pressure, changes the internal force condition, and forms a coupled stress field of load and pore water pressure, which forms the fatigue coupling load (Li, 2013; Liu et al., 2023a). The research on the influence factors and distribution law of the pore water pressure of the asphalt pavement is mainly based on numerical simulation (Dong et al., 2007), and its field test has mainly focused on the inside of the asphalt layer (Jiang et al., 2012). However, due to the differences in rainfall in different seasons, the humidity of the roadbed varies significantly, which has a significant impact on the dynamic response characteristics of asphalt pavement and roadbed.
Seasonal changes lead to significant changes in temperature and humidity inside asphalt pavement. More and more attention has been paid to the impact of temperature on road bearing capacity, with less attention paid to the changes in humidity. However, the impact of humidity on the dynamic response of the road surface is significant, and previous research has not focused on testing under coupling of hydrodynamic pressure and axle load. At the same time, there are relatively few studies on changes in hydraulic stress response indicators of the graded crushed stone transition layer under different speed and axle weight. In view of this, based on the design of the asphalt load response test and the monitoring system, the internal humidity of the subgrade and pavement in the rainfall process is tested. In this paper, the variation of pore water pressure in the transition layer under the coupling of humidity and dynamic load is studied, and the influence of seasonal variation on the dynamic response of the pavement structure is discussed. The analysis improves the adaptability of modified semi-rigid base asphalt pavement in the hilly area of eastern Sichuan.
2 Test plan layout of test section
2.1 Test section pavement structure
There are three pavement structures in the dynamic response test section of the asphalt pavement of the Sui-guang Expressway, including a traditional semi-rigid asphalt pavement (S1) and two inverted asphalt pavements (S2 and S3), which are mainly distributed in the excavated section. The composition is shown in Figure 1. The inverted asphalt pavements are that flexible graded crushed stone transition layer is arranged between asphalt layer and cement stabilized macadam base layer. The S1 is the original design document for the pavement structure, while S2 and S3 are designed with inverted asphalt pavement structures that are more suitable for the characteristics of the southwest hilly area based on the characteristics of the roadbed and rainfall. The inverted asphalt pavement adds a graded crushed stone transition layer between the base layer and the asphalt surface layer, which significantly reduces the reflection cracks of the asphalt pavement and eliminates internal moisture.
2.2 Sensor component layout
6 types of sensing elements are installed in the dynamic response test section of the asphalt road of Sui-guang Expressway, including horizontal asphalt strain gauges (transversal and longitudinal), vertical large deformation strain gauges, earth pressure gauges, temperature sensors, pore water pressure gauges and soil moisture sensors. The specific layout scheme is shown in Figures 2A–C (taking structure S3 as an example (Cao et al., 2022). Among them, the horizontal asphalt strain gauges are arranged in a 3×4 matrix form, 12 horizontal asphalt strain gauges are embedded in each pavement structure, and the strain amplitude is the sum of the absolute values of tensile and compressive strains (Dong et al., 2008; Dong et al., 2013; Liu et al., 2023b). The structural S2 and S3 transition layer two vertical large deformation strain gauges are arranged with wheel tracks positions, and one vertical large deformation strain gauge is arranged at the central position of the traffic lane. Two dynamic earth pressure gauges are arranged along the wheel track on the top surface of each structural soil foundation. There are 4 dynamic earth pressure gauges arranged on both sides of the bottom wheel of the graded crushed stone transition layer of structures S2 and S3. The soil moisture sensor is set at the 30 cm below the soil base of the structure S1, the middle part of the structural S2 transition layer, the middle part of the cushion layer, at the 30cm and 50 cm below the soil foundation. At the same, the soil moisture sensor is arranged at 30 cm below the structural S3 soil foundation and in the middle of the transition layer. Seven soil moisture sensors were buried in the test section. Two pore water pressure gauges are arranged along the wheel track in the middle of the structural S2 and S3 transition layers. The temperature sensors are placed in the middle of upper surface layer, middle surface layer and lower surface layer. It should be noted that the strain or stress values used in the analysis below refer to the peak value of the corresponding axial position on the time history curve unless otherwise specified.
2.3 Testing vehicles
Different types of vehicles are driving on the road. Different models have different natural frequencies. Large-duty trucks have more damage to road surface than cars. The load response characteristics of pavement structures under different vehicle loads are different. The two models are loaded and tested on different pavement structures. The vehicle parameters are shown in Table 1; Figures 3A, B.
3 Coupling response analysis of humidity to dynamic load of inverted asphalt pavement
3.1 Overview of the field test process
The rebound modulus of the subgrade soil and transition layer graded crushed stone is very sensitive to the internal humidity of the corresponding structural layer (Li and Selig, 1994; Cao, 2007; Zhou, 2014). Therefore, while the road surface dynamic response tested, the typical layer moisture conditions inside the pavement structure were observed to investigate the effect of rainfall on the change of the humidity status of the new pavement graded crushed stone transition layer, cushion, and subgrade soil. The stress and strain state of pavement structure provides basis and reference.
The humidity test data of subgrade and graded crushed stone layer are shown in Table 2. The volumetric water content of the subgrade with the depth of 20 cm in the asphalt pavement structure tested in May 2016 is about 30%± 5%, which is higher than when the sensor is buried (On the 29th of December 2015), the initial volumetric water content of the test (5–10%). The volumetric water content of the soil foundation at 40 cm depth of the subgrade is 1.9–2.0 times of the depth of the subgrade at 20cm, and the volumetric water content of the graded crushed stone cushion tested before the rain is 7.0–7.5%.
On May 18th of 2016, the day was cloudy, and on the 18th, there was 20 h of rain from 20:00 to 19:00, and the peak of rainfall was concentrated within 12 h from the early morning to noon on the 19th, with a rainfall of 10 mm. In the meantime, the humidity variation data of the graded crushed stone transition layer was recorded every half hour by i500-ES. It was found that the volumetric water content in the middle of the structural S2 graded crushed stone transition layer was stable at around 5.9%, and the water content of the structural S3 was stable at about 4.7%. There is no obvious fluctuation, because the test section is a new highway, the asphalt surface layer is basically not water seepage, the road surface water cannot penetrate into the inner pavement. The cement stabilized macadam layer blocks the groundwater ascending channel. Therefore, the transition layer is a self-contained relatively closed system, and the water content has relatively less affected by environmental factors.
As for the subgrade, before the rainy season, the rainfall has no significant effect on the internal humidity of the subgrade. The volumetric water content at 20 cm below the subgrade is 26.6–27%, and the volumetric water content at 40 cm below the subgrade is 52.1%. Obviously, before the rainy season, due to no heavy rainfall or long-term continuous rainfall, the water content of the subgrade soil is stable for a long period, and the humidity of the subgrade soil will not fluctuate significantly due to short-term rainfall or a small amount. At this time, the impact of rainfall on the moisture content of the subgrade soil is relatively small. Conversely, in the rainy season, the fluctuation of the water content of the subgrade soil is greatly affected by rainfall (Jiang, 2014).
In contrast, the effect of rainfall on the humidity of the graded crushed stone cushion is more obvious. The volumetric water content of the graded crushed stone cushion tested before rain, during rain (close the stop of the rain) and after rain (within 24 h) respectively at 7.1%, 16.2%, and 18.9%, the volumetric water content of the graded crushed stone cushion during and after the rain was about 2.3 times and 2.7 times that before the rain, respectively. The volumetric water content of the graded crushed stone cushion is still rising within 24 h after the rain stop, but the increase is less than that during the rainfall process. The graded crushed stone cushion effectively improves the internal humidity of the subgrade and has a good drainage effect. The literature (Liu et al., 2012) draws the same conclusions through an indoor model test.
3.2 Basic characteristics of dynamic response of pore water pressure in transition layer
For the structure S2, under the condition that the volumetric water content of the graded crushed stone transition layer is 5.9%, when the 10t single rear axle and the 23t double rear axle load pass the test section at a speed of 20 km/h, the pore water pressure stress in the transition layer is measured. The time history curve is shown in Figure 4.
Analysis test data is visible: the pore water pressure field has the same wave propagation characteristics as the displacement field under the action of dynamic load, consistent with literature results (Dong et al., 2009). As the wheel-loaded adjacent pore water pressure timing, the pore water pressure stress inside the graded crushed stone increases sharply, and it quickly reached the peak and then quickly dissipated. Due to the test error, the pore water pressure measured fluctuated near 0 o’clock, but overall, it was not found that the pore water re-absorbed due to vacuum negative pressure suction, resulting in negative pores water pressure, which proves the good drainage performance of the inverted asphalt pavement graded crushed stone transition layer and realizes the basic intention of the design. In addition, the weight difference between the front and rear axles of the single-rear axle truck is large, and the difference of the mechanical response index is obvious, but the load assigned to each front axle tire by the double rear axle truck is slightly larger than the load on each tire of the rear axle. Therefore, the amplitude difference among the shafts before and after the pore water pressure of the rear axle truck graded crushed stone transition layer is small, and the rear axle amplitude may be smaller than the front axle.
3.3 Analysis of driving speed and load sensitivity of pore water pressure in transition layer
Under the driving load, the measured pore water pressure in the transition layer of the inverted structure S2 and S3 is shown in Figures 5A, B. It can be concluded that the pore water pressure decreases with the growth of the traveling speed regardless of the structure S2 or the structure S3. Taking the test of structure S2 with 10t and 16t single rear axle trucks as an example, when the driving speed is increased from 5 km/h to 40 km/h, the pore water pressure caused by the rear axle and decreased by about 21–26%. Further, when the comparison vehicle is traveling at a speed of 40 km/h, the structure S2 is at 22.6 t (rear axis, the same below) and the structure S3 is under the action of 21.6 t axle load, and the structure S2 is at 9.66 t and the structure S3 is at 11.48 t axle load. Under the difference of the pore water pressure in the transition layer of the two pavement structures, it is found that the difference is mainly due to the difference in the applied load. It is noted that the structure S2 and the structure S3 adopt different ways (surface layer reinforcement or thickening) to compensate for the influence of the thickness of the cement stabilized macadam layer on the bearing capacity of the pavement structural. Therefore, it is reasonable to speculate that the performance of the diffusion load is enhanced, resulting that the deformation of the gradation gravel transition layer is smaller; the more effectively reduce the pore water pressure in the transition layer and reduce the erosion or seepage erosion of the graded crushed stone by the pore water.
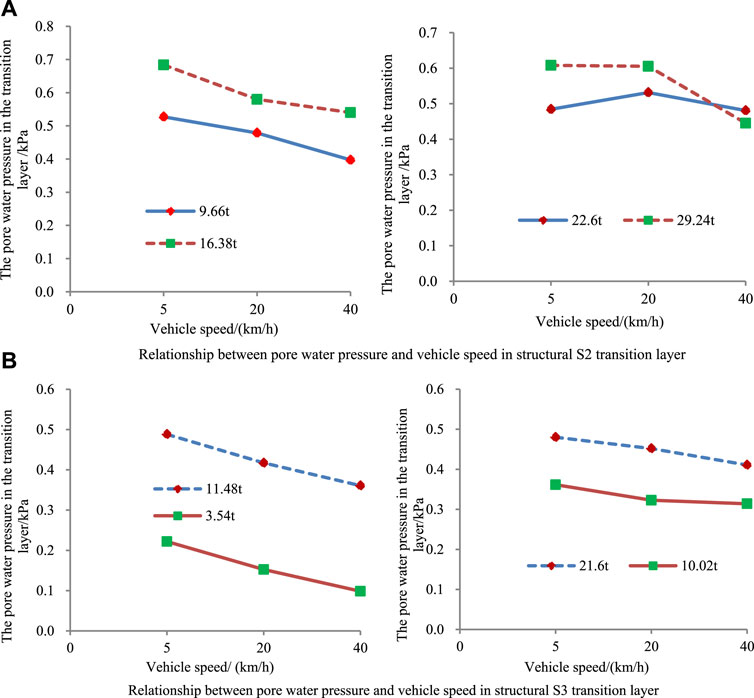
FIGURE 5. Relationship between pore Water pressure and Speed in transition layer (left: single rear axle truck, right: double rear axle truck).
It is worth noting that in this test, the graded crushed stone of the transition layer is far from saturated, resulting in a very small amount of pore water pressure, which is less than 1% of the vertical pressure (see Figure 6). The transition layer can effectively reduce the damage degree of water seepage to the pavement structure.
4 Analysis of seasonal influence factors in field test
The field test eliminates the distortion of the test results caused by the material scale effect, which reproduces the pavement structural response to the driving load. However, in the test under the changing conditions of the natural environment, the detection data is bound to produce large dispersion, especially the extremely sensitive temperature of the pavement material. The significant variability of the acquisition index is inevitable, and the reasonable definition of the experimental environment parameters is therefore more important (Ovik et al., 2000; Drumm and Meier, 2003). Most studies have adopted the temperature in the middle of the asphalt layer to establish the relationship between temperature and strain (Priest and Timm, 2006; Willis, 2009). The literature (Mateos and Snyder, 2002) considers that the average temperature of the asphalt layer is more representative. Considering the nonlinear distribution of the pavement temperature along the depth, the equivalent temperature of asphalt layer calculated in the pavement surface, the middle of the asphalt middle surface layer and of the asphalt lower surface layer of this paper. A field comparison test was conducted in two seasons, and in the deep winter (January 2016, equivalent temperature about 11 °C) and early summer (May 2016, the equivalent temperature is about 46 °C). The test uses a single rear axle truck (rear axle weight 6t), and is carried out at a travel speed of 30–35 km/h, and the test data shown in Figures 7A–E, 8A, B. The results showed that.
1) At the same measuring point, the detection values of the structural response indicators of the summer pavement are higher than the winter detection values, and the difference between them can be as high as several times. Taking the strain amplitude at the bottom of the asphalt layer as an example, the measured value in summer is about 1.4–3.9 times of the measured value in winter. The trend of strain response is further analyzed. It is found that the variation of longitudinal and transverse strain along the road is different after the temperature rises. Among them, along the longitudinal direction, the tensile strain and compressive strain at the bottom of the asphalt layer increase greatly. While in the transversal direction, the tensile strain at the bottom of the asphalt layer increases with temperature, and the compressive strain hardly increase in magnitude, which stay near zero.
2) Due to the difference in water content between the graded crushed stone cushion and the subgrade in January and May, especially the water content of the subgrade soil is quite different. In January, the atmosphere is dry and the water content is low. The cushion and the subgrade strength are both relatively high. While the rainfall in May increased significantly, the water content of the subgrade and the cushion was relatively large, and its strength was relatively low. The mechanical response of the pavement under load was relatively severe, so the vertical compressive stress on the top surface of the subgrade was affected by seasonal factors. The measured value in May is larger about 1.5–7.5 times than that is in January. The seasonal variation performance of the cushion and subgrade will inevitably affect the load response of the superstructure, hence, a vertical compressive stress at the bottom of the graded crushed stone transition layer increased by 3.5–5.5 times in May compared with January, and the vertical displacement increase by 2–3 times.
3) The sensitivity of the three pavement structures to seasonal factors is different. Overall, the sensitivity of the structure S1 to the season is lower than that of the structure S2 and the structure S3, and the structure S3 is most sensitive to the season. The structure S1 is a semi-rigid asphalt pavement, and the main bearing structure layer is a cement stabilized gravel layer. The temperature is higher, the inter-layer strain transmission performance is worse between the asphalt layer and the cement stabilized macadam, and the load transmitted to the top surface of the subgrade is small. The thickness of the asphalt layer is thinner. The influence of temperature on the mechanical response of the pavement structure is also lower than that of the structure S3. However, the upper 30 or 34 cm of the inverted asphalt pavement is a flexible structural layer. The mechanical properties of the asphalt mixture are greatly affected by the temperature, and the mechanical properties of the graded crushed stone are greatly affected by the water content, which leads to the seasonal sensitivity of the inverted structure more than the semi-rigid structure. The thickness of the structural S3 asphalt surface layer is larger than the structure S1 and the structure S2, and the mechanical response characteristics are relatively more affected by the season and temperature.
4) The summer temperatures in the eastern Sichuan region are relatively high (with the highest temperature reaching 41.6°C in recent years), the climate is hot, and the rainfall is large (with an average precipitation of 1,014–1,282 mm in Guang’an for many years, and the maximum annual rainfall is 1,485 mm). The continuous rain time is long, and the roadbed filler is mainly shale, which will sharply reduce its strength under rainwater immersion. In addition, some roads have high traffic volume, which requires high performance requirements for asphalt pavement resistance to water damage and high-temperature deformation. In view of this, it is recommended to install a graded crushed stone cushion under the pavement structure in the eastern Sichuan region to regulate the humidity of the roadbed and extend the service life of the asphalt pavement. Meanwhile, the inverted asphalt pavement structure has good adaptability in the hilly areas of eastern Sichuan.
4 Conclusion
1) The rainwater is blocked from entering new pavement transition layer by the upper and lower structural layers, so the volumetric water content in the middle part of the transition layer of the asphalt pavement has no obvious fluctuation during the rainfall process. At the same time, the rainfall has a great influence on the water content of the subgrade soil (excavated section) during the rainy season. The fluctuation range is different due to the different depth of the subgrade layer. The water content at the middle of cushion fluctuated significantly during the rainfall process, and the fluctuation peak lags behind the rainfall peak. The addition of the cushion layer helps to improve the humidity inside the subgrade.
2) There is a wave propagation characteristic of the pore water pressure in the transition layer, and the measured value increases with the growth of the axle weight, and decreases with the growth of the driving speed. However, the good drainage performance makes the pore water pressure in the transition layer of graded crushed stone small in magnitude. It eliminates the time-dependent effect of the pore water pressure caused by the axle load, and the pore water-sucking phenomenon caused by the vacuum negative pressure suction. Especially for new pavements, the water content of the transition layer is relatively weakly affected by rainfall with the participation of the surface layer and the underlying cement stabilized macadam base. Largely, the erosion or seepage erosion of the graded crushed stone by the pressurized water is alleviated.
3) Seasonal changes have significant influence on the dynamic response of asphalt pavement, which is affected by the difference of temperature and water content in different seasons. The bottom strain amplitude of asphalt layer, the vertical compressive stress of top surface of subgrade, the vertical compressive stress at the bottom layer of graded crushed stone transition layer and the vertical displacement of the graded crushed stone transition layer of the summer are 1.4–3.9 times, 1.5–7.5 times, 4.5–6.5 times and 3–4 times of the winter, respectively, and the sensitivity of the inverted asphalt pavement to the season is greater than semi-rigid asphalt pavement.
Data availability statement
The original contributions presented in the study are included in the article/Supplementary material, further inquiries can be directed to the corresponding author.
Author contributions
MC: methodology, formal analysis and Writing—Original Draft; WH: data Curation, Writing—Review and Editing; GL: investigation, visualization; ZW: Writing—Review and Editing, visualization. All authors contributed to the article and approved the submitted version.
Funding
This study was supported by the Sichuan transportation science and technology project (grant number 2015 from 4-1, 2019-ZL-13), the Sichuan Province Science and Technology Planning Project (Application Fundamental Research) (grant number 2020YFS0362), and supported by Sichuan Science and Technology Program (grant number 2022YFG0048).
Conflict of interest
Authors MC, WH, and ZW were employed by the Sichuan Communication Surveying and Design Institute Co., Ltd.
The remaining author declares that the research was conducted in the absence of any commercial or financial relationships that could be construed as a potential conflict of interest.
Publisher’s note
All claims expressed in this article are solely those of the authors and do not necessarily represent those of their affiliated organizations, or those of the publisher, the editors and the reviewers. Any product that may be evaluated in this article, or claim that may be made by its manufacturer, is not guaranteed or endorsed by the publisher.
References
Ai, C., Rahman, A., Xiao, C., Yang, E., and Qiu, Y. (2017). Analysis of measured strain response of asphalt pavements and relevant prediction models. Int. J. Pavement Eng. 18 (12), 1089–1097. doi:10.1080/10298436.2016.1149836
Al-Qadi, I. L., Loulizi, A., Elseifi, M., and Lahouar, S. (2004). The Virginia Smart Road: The impact of pavement instrumentation on understanding pavement performance. J. Assoc. Asphalt Paving Technol. 73 (3), 427–465.
Assogba, O. C., Tan, Y., Zhou, X., Zhang, C., and Anato, J. N. (2020). Numerical investigation of the mechanical response of semi-rigid base asphalt pavement under traffic load and nonlinear temperature gradient effect. Constr. Build. Mater. 235, 117406. doi:10.1016/j.conbuildmat.2019.117406
Cao, C. (2007). “Study on the humidity condition and moduli adjustment coefficient of Subgrade,”. Doctoral dissertations (Shanghai: Tongji University).
Cao, M., Huang, W., and Wu, Z. (2022). Influence of axle load and asphalt layer thickness on dynamic response of asphalt pavement. Geofluids 2022, 1–16. doi:10.1155/2022/9592960
Chen, J., Wang, H., and Xie, P. (2019). Pavement temperature prediction: Theoretical models and critical affecting factors. Appl. Therm. Eng. 158, 113755. doi:10.1016/j.applthermaleng.2019.113755
Dong, Z., Cao, L., and Tan, Y. (2009). Time history analysis of dynamic response for saturated asphalt pavement. J. Wuhan Univ. Technol. Transp. Sci. Enginee ring) 33 (6), 1033–1036. doi:10.3963/j.issn.1006-2823.2009.06.003
Dong, Z., Zhang, Y., and Peng-Min, L. V. (2013). Fatigue performance of asphalt mixture under tensile-compressive alternating loading. China J. Highw. Transp. 26 (2), 15–19.
Dong, Z., Zhang, Z., and Peng-Min, L. V. (2008). Experiment study on the influence of the vehicle velocity on the asphalt pavement dynamic response. J. Zhengzhou Univ. Eng. Sci. 29 (2), 123–126.
Dong, Z. J., Tan, Y. Q., Cao, L., et al. (2007). Research on pore pressure within asphalt pavement under the coupled moisture-loading action. J. Harbin Inst. Technol. 10, 1614–1617. doi:10.3321/j.issn:0367-6234.2007.10.024
Drumm, E. C., and Meier, R. (2003). LTPP data analysis: Daily and seasonal variations in insitu material properties. Washington, DC: Transportation Research Board, National Research Council.
Gao, Y., Yu, Z., Chen, W., Yin, Q., Wu, J., and Wang, W. (2023). Recognition of rock materials after high-temperature deterioration based on SEM images via deep learning. J. Mater. Res. Technol. 25, 273–284. doi:10.1016/j.jmrt.2023.05.271
Garg, N., and Hayhoe, G. F. (2001). “Asphalt concrete strain responses at high loads and low speeds at the national airport pavement test facility (NAPTF),” in Advancing airfield pavements (Chicago, Illinois: Springer), 1–14.
Islam, M. R., and Tarefder, R. A. (2013). Measuring thermal effect in the structural response of flexible pavement based on field instrumentation. Int. J. Pavement Res. Technol. 6 (4), 274–279. doi:10.6135/ijprt.org.tw/2013.6(4).274
Jiang, Z., Gao, J., Ji, T., et al. (2012). Research on piezo-electric sensor measuring pavement hydrodynamic pressure. Transducer Microsyst. Technol. 31 (4), 17–19.
Jiang, Z. (2014). “Research on existent subgrade moisture condition and stability evaluation for expressway,”. Master’s degree thesis (Xi’an: Xi’an of Chang'an University).
Li, D., and Selig, E. T. (1994). Resilient modulus for fine-grained subgrade soils. J. geotechnical Eng. 120 (6), 939–957. doi:10.1061/(asce)0733-9410(1994)120:6(939)
Li, R. (2013). “Study on unsaturated hydraulic asphalt concrete pavement internal hydrodynamic pressure distribution,”. Master’s degree thesis (Chongqing: Chongqing Jiaotong University).
Liu, J., Yao, H., Hu, M., et al. (2012). Study of moisture dynamic response and underground drainage test of subgrade model under water level fluctuation. Rock Soil Mech. 33 (10), 2917–2922.
Liu, S. M., Sun, H. T., Zhang, D. M., Yang, K., Li, X., Wang, D., et al. (2023a). Experimental study of effect of liquid nitrogen cold soaking on coal pore structure and fractal characteristics. Energy 275 (7), 127470. doi:10.1016/j.energy.2023.127470
Liu, S. M., Sun, H. T., Zhang, D. M., Yang, K., Wang, D., Li, X., et al. (2023b). Nuclear magnetic resonance study on the influence of liquid nitrogen cold soaking on the pore structure of different coals. Phys. Fluids 35 (1), 012009. doi:10.1063/5.0135290
Mateos, A., and Snyder, M. (2002). Validation of flexible pavement structural response models with data from the Minnesota road research project. Transp. Res. Rec. J. Transp. Res. Board 1806, 19–29. doi:10.3141/1806-03
Ovik, J. M., Birgisson, B., and Newcomb, D. (2000). Characterizing seasonal variations in pavement material properties for use in a mechanistic-empirical design procedure. United States: University of Minnesota.
Priest, A. L., and Timm, D. H. (2006). Methodology and calibration of fatigue transfer functions for mechanistic empirical flexible pavement design (No NCAT Report 06-03). United States: Auburn University.
Willis, J. R. (2009). Field-based strain thresholds for flexible perpetual pavement design. United States: Auburn University.
Keywords: pore water pressure, dynamic response, seasonal factor, the hilly area, graded crushed stone
Citation: Cao M, Huang W, Liu G and Wu Z (2023) Study on the dynamic response characteristics of roadbed and pavement under the humidity and season factors in the hilly area of Southwest China. Front. Earth Sci. 11:1239495. doi: 10.3389/feart.2023.1239495
Received: 13 June 2023; Accepted: 05 July 2023;
Published: 13 July 2023.
Edited by:
Xuelong Li, Shandong University of Science and Technology, ChinaReviewed by:
Lei Shi, China University of Mining and Technology, ChinaYu Xuguang, Tangshan Vocational and Technical College, China
Copyright © 2023 Cao, Huang, Liu and Wu. This is an open-access article distributed under the terms of the Creative Commons Attribution License (CC BY). The use, distribution or reproduction in other forums is permitted, provided the original author(s) and the copyright owner(s) are credited and that the original publication in this journal is cited, in accordance with accepted academic practice. No use, distribution or reproduction is permitted which does not comply with these terms.
*Correspondence: Mingming Cao, 707360021@qq.com