- 1Dipartimento di Fisica “E. R. Caianiello”, Università degli Studi di Salerno, Fisciano, Italy
- 2Risk Management Solutions, London, United Kingdom
Loss outcomes from geohazards are compounded by an array of human risk factors. The combination of geohazards and human risk factors can generate multi-risk cascades. In the historical record, disasters arising from such multi-risk cascades are comparatively rare. However, far more common are near-misses, where a disaster tipping point to massive destructive energy release and expanding losses was narrowly averted. What happened historically is only one realization of what might have happened. Due to psychological outcome bias, people pay far less attention to near-misses than to actual losses. A downward counterfactual is a psychological term for a thought about the past, where things turned for the worse. Exploration of downward counterfactuals enhances risk awareness and can contribute to risk preparedness. There are no databases of multi-risk cascade near-misses, but insights can be gained from downward counterfactual analysis. Geohazard examples of multi-risk downward counterfactuals are given, including cases of critical infrastructure damage. A downward counterfactual can drive a minor hazard event beyond the disaster tipping point boundary, and turn a disaster into a major catastrophe. To illustrate the latter, a downward counterfactual analysis is presented of the Fukushima nuclear accident of 11 March 2011, which might have crossed the tipping point boundary into a multi-risk cascade catastrophe.
1 Introduction
The interaction of severe geohazards with the human environment can lead to extreme societal losses, which are compounded by detrimental human risk factors, including misjudgement, misinformation, human error, complacency, negligence, cognitive dissonance and malice. Most geohazards have a human dimension; most notably, weather hazards are affected by climate change associated with greenhouse gas emissions. But the compounding of geohazards with human risk factors can generate loss outcomes so severe as to warrant being explicitly termed multi-risk events. Such events include wildfires, dam failures, chemical and nuclear accidents, associated with nonlinear tipping point phenomena. A multi-risk event is one where the severity of the event loss is driven by more than one causal factor. These multi-risk events involve the destructive release over a short time period of massive amounts of thermal, gravitational, chemical and explosive energy having a catastrophe potential well beyond human control, resulting in a cascade of severe human and property loss consequences, including mass casualties, business interruption, supply chain failure and criminal litigation.
As indicated in the schematic diagram Figure 1, above a moderate level of geohazard severity, the compounding impact of ever-increasing human factors severity is to create a multi-risk domain of cascade losses, which is demarcated by a tipping point boundary. This boundary is rather opaque, but it can be clarified by considering pathways by which a system state can transition across the boundary. Actual historical examples of such state transitions are comparatively rare. However, this boundary can be explored through the thought experiment of reimagining history (Woo et al., 2017). For a given historical event, a dangerous perturbation can drive the event across the tipping point boundary into the multi-risk domain of cascade losses. Such perturbations are termed downward counterfactuals, and are described in the next section.
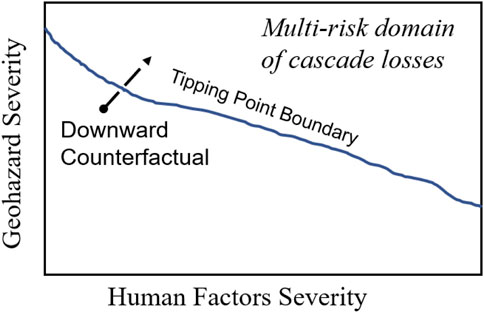
FIGURE 1. Multi-risk domain of cascade losses showing the tipping point boundary. A downward counterfactual perturbation can shift a system across the tipping point boundary into the multi-risk domain of cascade losses.
Beyond the existing literature on counterfactual analysis (e.g., Aspinall and Woo, 2019; Lin et al., 2020; Ciullo et al., 2021; Lin et al., 2021; Woo, 2021; Rabonza et al., 2022), the application to the compounding of geohazards with human factors is a notable development because of their combined practical importance. There are no databases of such compounds, which constitute a poorly investigated but nevertheless important region of the risk landscape. Investigation is limited by the comparatively weak interaction between social science and geoscience communities; few geoscientists read the social science literature, and vice versa. Downward counterfactual analysis is an innovative multi-risk tool for exploring this region. Anchored to past events, downward counterfactuals can reveal hitherto unforeseen but plausible cascade scenarios and so enhance risk awareness. Among these latent cascade scenarios are some involving human factors, which may not be readily recognizable or appreciated without special scrutiny.
Multi-risk cascades involving the compounding of geohazards with human factors are the prime focus of this contribution to the inter-disciplinary literature spanning geosciences and social sciences. As demonstrated starkly with the PG&E bankruptcy following the Camp Fire of 2018, which is reviewed in 2.1, consideration of downward counterfactuals following near-misses can expedite vital risk mitigation decisions, which might otherwise be deferred. Rather than reflect on a measure of fortune in avoiding disaster, decision-makers can reflect instead on downward counterfactuals.
2 Downward counterfactuals
A counterfactual is a thought about the past. A downward counterfactual is a thought about the past, where things turned for the worse. This downward counterfactual terminology was introduced to geoscientists by Woo (2019), and applies to all manner of extreme georisks. Appropriate for consideration of human risk factors, the downward counterfactual vocabulary originated in cognitive psychology (Roese, 1997), and has been used primarily in the psychological literature. This concise vocabulary constitutes a welcome additional bridge between the communities of psychologists and geoscientists. Whenever a geohazard event occurs, the following searching question should be asked: what are the downward counterfactuals?
Most counterfactual thoughts that people have are upward; how things might have been better. For an Olympic athlete, a silver medalist might ponder what might have been done to have won the gold medal. It is relatively uncommon for people to have downward counterfactual thoughts, but an Olympic bronze medalist might ponder what might have happened with no podium finish. A book on the social psychology of counterfactual thinking (Roese and Olsen, 1995) was entitled “What might have been,” indicative of personal feelings about the past. Daniel Kahneman, a leading cognitive psychologist, and economics Nobel laureate, has been a notable contributor to this literature (Kahneman and Miller, 1986). Recognizing the inevitable intrusion of cognitive bias into risk assessment, the downward counterfactual vocabulary is also relevant in a risk context. Most counterfactual thoughts are upward, how risk might have been mitigated. Few counterfactual thoughts are downward—how things might have been worse. But such thinking is necessary if risk analysts are to avoid surprise by rare events.
As a term drawn from the social sciences, the downward counterfactual is an enlightening concept, well suited for addressing human factors, and contributing to making society more disaster resilient. Limitations in the narrow way that people think about historical events can hinder progress towards achieving resilience against events on the distant risk horizon.
Exploration of how things might have turned for the worse is an uncommon endeavor, even for highly experienced and knowledgeable risk professionals. This is reflected in the recency of the use of the downward counterfactual vocabulary in the analysis of extreme risks (Woo, 2019). It takes time, resources and also imagination. Counterfactual risk analysis is an exercise in reimagining history (Woo et al., 2017). Such a deliberate historical exercise in disaster discovery is valuable as an exploratory tool for avoiding surprise by the unexpected or unanticipated.
The discovery of otherwise unexpected or unanticipated events fills a gap in understanding the applicability of power-law distributions in describing multi-risk cascades (Pisarenko and Rodkin, 2014). Counterfactual risk analysis shows explicitly how these multi-risk cascades can occur as alternative realizations of historical events, and so contribute to the heavy tails of the loss distributions.
2.1 Wildfire downward counterfactuals
Taking wildfire as an illustration, arson, hiker carelessness and inadequate maintenance of electric transmission lines can result in a forest region falling within a multi-risk domain of cascade loss, during a dry period with strong winds. However, with greater vigilance and strict fire control measures, it would require more extreme meteorological conditions for a region to fall within the domain of cascade losses. By contrast, in a forested region with very little fire control, even a moderate windstorm might drive a region into the domain of cascade losses.
The driest period in Californian history was the California drought which persisted from December 2011 to March 2017. In October 2017, a combination of drought, strong winds and human failure in controlling ignition sources were multi-risk factors in fires which started when winds damaged electrical equipment, much of it owned by Pacific Gas and Electric (PG&E), and sparked flames that quickly grew out of control in several counties. In the worst of the blazes, the Tubbs Fire, 22 people died and about 5,600 homes and other buildings were incinerated over about 150 sq.km., largely in the Santa Rosa area. At the time, it was the most destructive wildfire in California history. The California Department of Forestry and Fire Protection (CAL FIRE) concluded that the Tubbs Fire was caused by a malfunction of a property owner’s privately maintained electrical system (Melo, 2020), not PG&E, which was deemed responsible for other lesser fires that started on the same night.
Even if PG&E was not responsible for the devastating 2017 Tubbs fire, counterfactually, it might have been. This near-miss should have triggered intensive action by PG&E to radically overhaul their electric transmission line maintenance program and schedule. This opportunity for urgent risk mitigation was missed. The following year, on 8 November 2018, a faulty PG&E electric transmission line ignited the Camp Fire which killed 85 people and covered an area of 620 sq.km., and destroyed more than 18,000 structures, with most of the destruction occurring within the first 4 hours. The towns of Paradise and Concow were almost completely destroyed, each losing about 95% of their structures. 50,000 people were displaced to local communities; a cascading impact that is often overlooked (Spearing and Faust, 2020). A grand jury report found that PG&E repeatedly ignored warnings about its aging power lines and faulty maintenance, and failed to follow state regulations (Butte County District Attorney, 2020). In early 2019, PG&E filed for Chapter 11 bankruptcy, in anticipation for being held liable for the 2018 Camp Fire (Sullivan et al., 2020).
The PG&E ignorance of risk warnings reflects a lack of corporate risk awareness of how moderate losses can cascade into catastrophic losses. To enhance such risk awareness, and the avoidance of future surprise, the construction of scenarios for multi-risk cascades is instructive. Plausible narratives for multi-risk cascades are not readily available. What has been lacking is a systematic and credible methodology for developing narratives, which is not unduly hypothetical or speculative. A formal procedure for constructing such scenarios is presented here, which avoids excessive speculation by being firmly rooted in the actual historical catalog. PG&E implementation of such a downward counterfactual procedure after some earlier damaging wildfires, including the Tubbs fire of 2017, might have prevented, or at least mitigated, the Camp Fire loss cascade of 2018.
With reference to Figure 1, the neglect of electric transmission lines is the human factors severity, and a more extensive wildfire is the geohazard severity. Based upon the Camp Fire evidence, the Butte County District Attorney (2020) concluded that: “PG&E reduced costs of inspection and patrol by reducing the amount of time budgeted for the inspections and patrols. As expected, the result of these reductions was less thorough and less complete inspections and patrols.”
For PG&E, the multi-risk domain of cascade losses was attained by a transition across the tipping point boundary from the Tubbs Fire of 2017. Rather than corporate relief at a narrow near-miss in not being liable for the Tubbs Fire, PG&E should have considered the class action negligence downward counterfactuals, and responded promptly by increasing the amount of time for inspections and patrols. This remedial action might have identified the electrical arc danger that led to the Camp Fire the following year. At least, this action would have lowered the danger.
Reflecting human outcome and optimism cognitive biases, good fortune may be mistaken for good decision-making. The outcome of the Tubbs Fire might have been perceived as vindication of PG&E’s tight inspection and patrol budgets. But decision-makers need to weigh savings in safety expenditure against potential future loss costs. To meet this objective, the explicit use of the downward counterfactual terminology in corporate and civic authority decision-making is advocated as a notable methodological advance in cascade loss avoidance. The stark realization that the Tubbs Fire might counterfactually have led to massive negligence claims should have concentrated PG&E attention on rapidly improving inspection and patrol programs. Lacking downward counterfactual analysis, the deficiencies in the inspection and patrol programs may not be readily apparent.
There are no geographical boundaries to the application of downward counterfactual analysis, which is a universal methodology. As another wildfire example at the urban-forest interface, consider the Israel wildfire of mid-August 2021. The human factor associated with this wildfire is arson, according to forensic evidence (Staff, 2021). Remote sensing and field data are the principal sources of Israel wildfire information (Guk et al., 2023). The first report of fire came from a resident of Beit Meir, a small village in the pine forests west of Jerusalem. Pine forest fires are notoriously hard to control because hot pine cones travel large distances in the heat of a fire. A massive fire broke out near Beit Meir, nine miles west of Jerusalem, just off the Jerusalem-Tel Aviv highway.
Ten communities were evacuated, with thousands of residents in the Judean Hills ordered to leave their homes as a precaution. A plume of black smoke covered Jerusalem, and residents were advised to limit outdoor activity because of high pollution levels. It took some 1,500 firefighters working for 3 days to put out the fires, which may have been intentionally lit in several places. The outcome of the fire was that around 3,200 acres of forest burned. But it could have been far worse; the Israel fire department initially feared that 5,000 acres might be consumed in the wildfire. This was fortunate, because the Israel National Fire and Rescue Services were stretched to the limit. Forest fires in hilly areas are very difficult to control, because a firestorm can generate dynamically its own local weather. To a large extent, the feasibility of human control depends crucially on nature, specifically the key meteorological variables of temperature and wind strength and direction.
The expansion of the conflagration to cover 5,000 acres is one counterfactual. As another downward counterfactual, instead of normal August temperatures at the time of the wildfire, there might have been a heatwave as occurred several weeks earlier. With sustained strong winds directed towards West Jerusalem as well as higher temperatures, firefighting would have been an even greater struggle and potentially posed an urban threat to West Jerusalem. As the fires spread rapidly in the Jerusalem Hills, a logistical bottleneck would form in both the supply of professional firefighters, and also associated firefighting airplane support. With further opportunist malicious ignitions, such as the launch of incendiary balloons, a multi-risk cascade might envisage the wildfire engulfing outer areas of West Jerusalem. Defensive measures to mitigate this multi-risk cascade include pine tree forest control and enhancing arrangements for international firefighting airplane support. Downward counterfactual analysis would highlight the need for the simulation of effective evacuation by car, as previously managed in a large wildfire in Haifa (Kuligowski, 2020).
2.2 Earthquake downward counterfactuals
The outbreak of fires following an earthquake is a well-recognized multi-risk cascade phenomenon associated with seismic activity. The most notorious example is the great 1906 San Francisco earthquake, where the fire outbreaks were exacerbated by the human factor of arson, attributed to some residents whose buildings suffered shaking damage, and had insurance coverage for fire but not earthquake.
But seismic ground motion alone is sufficient to cause a loss cascade. On 6 April 2009, a moderate moment magnitude Mw6.3 earthquake struck L’Aquila city and province in central Italy at 3.32 (local time) in the morning. There were 308 deaths and 1,500 injuries of which 202 were serious (Alexander and Magni, 2013). About 100,000 buildings were severely damaged, including the regional hospital, and 67,000 were left homeless. Before the Mw6.3 mainshock, there had been a prolonged sequence of foreshocks which caused alarm, and induced people to leave their homes, or sleep near an exit. Beginning in February 2009, there was media coverage of a series of misinformed amateur earthquake predictions issued by a technician (G. Giuliani) from the Institute of Interplanetary Space Physics seconded to the Gran Sasso National Laboratories near L’Aquila. These predictions, which were based on radon concentrations measured with gamma-ray detectors, had no official validity but were widely reported and taken seriously, causing some citizens to evacuate their towns (Jordan, 2013).
As can happen with responses to media misinformation, some statements were made by the authorities which ultimately contributed to the human factor dimension of the seismic crisis. This was a multi-risk disaster in as much as the associated protracted legal proceedings compounded the severe casualty and damage consequences of the earthquake through widespread misinformation and misunderstanding about the science of earthquake warning.
Counterfactually, a death toll several times greater might have resulted if the mainshock had occurred 8 hours later, when the city center, which was seriously damaged, would have been thronged with visitors (Alexander, 2010). This scenario would have been even worse if the earthquake had occurred not during early spring but during the busy summer tourist season.
A further downward counterfactual is that the magnitude of the mainshock might have been greater than Mw6.3. Paleoseismological investigations (Cinti et al., 2011) indicate the possibility of the occurrence of larger surface faulting earthquakes in the past (Mw>6.5) producing longer surface rupture and larger displacement. Such a greater event would have generated stronger and longer ground motion, resulting in more severely damaged buildings. The cost of the damage from the L’Aquila earthquake was estimated to be €16 billion. If a maximal earthquake event had struck, the damage would have been substantially greater. Allowing for inherent stochasticity in the casualty outcome, with 100,000 buildings severely damaged, it is plausible to envisage alternative realizations of the L’Aquila earthquake, which might have claimed as many as a thousand lives.
Such a severe loss of life, three or four times the actual death toll, would have triggered a cascade of judicial, societal and political consequences, far exceeding those which engulfed the seismological profession worldwide after the Mw6.3 earthquake. With many daytime casualties amongst visitors, the international civil engineering profession would also have become embroiled in the legal actions. The high death toll would have taken the event well past the tipping point boundary into the zone of a major societal catastrophe, with international ramifications. Preparedness for such a cascade of professional scientific and engineering acrimony, as a plausible scenario, is one of the purposes of downward counterfactual analysis.
The L’Aquila earthquake was a recognized seismic hazard scenario. Downward counterfactual analysis provides a methodology for identifying scenarios that otherwise would have been given little or no hazard attention. The 1833 Sumatran subduction zone earthquake (Zachariasen et al., 1999) was estimated to have a magnitude of 8.8–9.2. Downward counterfactual analysis would have posited a more northerly rupture of similar size, which would have posed a significant tsunami hazard to Sri Lanka and southeast India. Such a disastrous event occurred on 26 December 2004, less than 6 years after the 1999 publication by Zachariasen et al. The lack of warning, despite the several hour tsunami travel time to Sri Lanka and southeast India was a major human factor contribution to the disaster.
Consider the 6 February 2023 East Anatolian Fault earthquake disaster, which claimed the lives of tens of thousands of people in southeast Turkey. Five years earlier, a counterfactual analysis of runaway Turkish earthquakes had been undertaken by Woo and Mignan (2018). Extended combinations of fault segments of the North Anatolian Fault might have ruptured in the Kocaeli earthquake of 1999, potentially doubling the death toll to 40,000 in a great runaway earthquake impacting Istanbul. By a similar counterfactual argument, extended combinations of fault segments of the East Anatolian Fault also have the potential for rupturing together. Such a rare combination of ruptures, not adequately appreciated by seismic hazard analysts, occurred on 6 February 2023 in a runaway sequence.
This was an intrinsically multi-risk event, because human factors were substantially responsible for the degradation of building seismic resistance, so greatly exacerbating the casualty toll. Poor construction practices and building code non-compliance (Chadha, 2023) were compounded by a government amnesty for non-compliant buildings introduced for electoral popularity (Mart, 2023). Several hundred building contractors were arrested in Turkey for poor building construction after the 6 February 2023 earthquake. The economic trade-off of long-term security for short-term electoral gain is not unique to Turkey; it happens elsewhere when important risk mitigation projects are postponed so as not to burden taxpayers. However, it is antithetical to societal resilience against geohazards. As and when significant earthquakes occur, downward counterfactual analysis can warn of the potential danger in postponing risk management projects.
3 Critical infrastructure downward counterfactuals
In the previous section, the use of downward counterfactuals has been explained in the context of specific hazards. There are numerous practical applications relevant to human and property assets at risk. In this section, the focus is on critical infrastructure, which is of paramount importance in governing potential cascade losses.
Downward counterfactual analysis is a generic risk tool, universally applicable to all types of hazard combinations, and especially for tipping-point insights. Multi-risk cascades are often associated with critical infrastructure, crucial for the economic development and efficient functioning of a modern society. Damage to critical infrastructure can be a tipping point for catastrophic losses. Two especially crucial components of critical infrastructure in many parts of the world are dams and nuclear power plants, both of which are potentially exposed to geohazards such as severe earthquakes and windstorms, and which may be vulnerable to human factors such as misjudgement and human error. Chemical plants are similarly safety-critical and exposed to geohazards. For critical infrastructure managers, it is instructive and insightful to reflect that any major external hazard, e.g., a pandemic, can pose a potential threat to critical infrastructure. For example, the potential impact of earthquakes during the COVID-19 pandemic was highlighted by Silva and Paul (2021).
The South Korean multinational, LG Chemicals, had little experience in monitoring and maintaining full tanks of styrene that were idled for long periods of time without operation (Gargava, 2020). This was a significant human factor in the accident that followed. Their polymer plant in Venkatapuram, Andhra Pradesh, India, was shut down on 24 March 2020. This was due to COVID-19; counterfactually it might have been due to a flood or other geohazard. Lateral thinking beyond the geosciences is a positive attribute for all those professionally engaged in geohazards and georisks. Important lessons can be learned from the study of other risks.
Prime Minister David Cameron admitted (2023) the groupthink prevalent in United Kingdom pandemic preparedness, which planned for just one type of pandemic: influenza but not coronavirus. This oversight might have been redressed through downward counterfactual thinking. As with the Black Swan (Taleb, 2007), downward counterfactual analysis is universally applicable to all hazards. A 2017 report for Lloyd’s (Woo et al., 2017) included a pandemic coronavirus scenario, MERS+, which was a more contagious variant of the Middle East Respiratory Syndrome MERS.
Preparations for a restart at the LG Chemicals plant were made on 4 May 2020, with proposed resumption on 7 May. As a consequence of the shutdown, there was some polymerization at ambient temperature of styrene to polystyrene. This is an exothermic process, leading to an increase in temperature. This further increased the rate of reaction, increasing the pressure further. At around 3.15a.m., the entire facility was engulfed in dense styrene vapors. The temperature in one tank started to rise exponentially. The peak temperature was 153.7°C., which is above the 145°C boiling point of styrene. The consequent rise in pressure resulted in uncontrolled release from the vents. Tank insulation prevented the heat generated from being dissipated to the atmosphere, which would have slowed the rise in pressure.
Any uncontrolled release of toxic styrene gas poses a major environmental risk; but it might have been far worse. A plausible downward counterfactual is that the venting of the tank might have failed. This specific failure scenario was indeed considered in the official report on the styrene gas leak (Gargava, 2020), recognizing that the build-up of pressure might have caused a catastrophic rupture of the tank, escalating to a catastrophic Boiling Liquid Expanding Vapor Explosion (BLEVE). Even with a moderate release of the tank contents, the massive fireball and explosion would have completely wrecked the plant, and caused widespread regional casualties and damage over many kilometers radius. Furthermore, styrene metabolites can cause carcinogenic health effects. So the population living in a large area of many sq.km. Around the plant would need to be screened regularly for cancer risk. Accordingly, the multi-risk cascade of the BLEVE downward counterfactual extends far ahead into the provision of long-term healthcare.
3.1 Dam downward counterfactuals
California is known around the world as earthquake country. Consider earthquake fatality risk in California. From 1950 to 2022, there were around two hundred earthquake-related deaths in California. This is a testament to strict state-of-the-art seismic building codes and high code compliance. However, this number might have been higher by two orders of magnitude if the Lower San Fernando Dam had been overtopped on 6 February 1971. The dam was badly damaged by the M6.7 San Fernando earthquake (Seed et al., 1989), and if the water had been at its maximum height, as it had been the previous year, the valley would have been flooded, with massive downstream consequences. A combination of heavy rain and strong seismic ground motion might have triggered an extreme engineering disaster, and cascade of losses. Aftershocks might also have caused further significant damage.
Officials and investigators agreed that a major catastrophe was narrowly averted (Sykora, 2019). This is one of a range of historical examples from the accumulating library of multi-hazard near-miss disasters, spanning the world. The Lower San Fernando Dam was not operated by PG&E, but there are some PG&E dams in California causing concern over seismic safety. One such dam is Scott Dam in northern California. A study by US Geological Survey (Lozos et al., 2015) shows that the 170 km long Bartlett Spring Fault, which runs right through the reservoir, is capable of producing earthquakes up to a Mw7.2, which is larger than the Mw5.9 PG&E used in their previous seismic safety modeling for Scott Dam. PG&E is considering the expedited partial or full removal of Scott Dam as a long-term mitigation measure, and plans to lower the water level in the reservoir. Counterfactually, the human factor underestimation of seismic hazard might have been revealed not by a geological study, but by a severe earthquake.
Another California dam near-miss occurred at the Oroville dam in northern California. With a height of 770 feet, Oroville Dam is the tallest dam in the United States. Completed in 1968 and located north of Sacramento in the foothills of the Sierra Nevada mountains, this large earthfill embankment dam is owned by the California Department of Water Resources. On 7 February 2017, water from powerful winter storms rushed under the spillway, which forced up giant slabs and ripped a huge hole in the structure. Due to the very large size of the dam and spillways, and the number of people at risk, this was one of the most serious dam safety incidents in United States history, and the estimated cost for the repairs and recovery was about $1.1 billion.
Creditably, this was the first major investigation of a dam incident in which human factors were given the same level of attention as physical factors, and is an exemplary case study (IFTP, 2018). Investigation of human factors enabled identification of underlying reasons for why judgements and decisions were made which resulted in the physical sequence of events which culminated in the spillway failures. This was an intrinsically multi-risk event that might have cascaded into a catastrophe; the risk of dam failure is a compound function of spillway design as well as external loading. Avoiding outcome bias, Koskinas et al. (2019) have recommended that inspections of all related facilities must be conducted by dam operators on a weekly or bi-weekly basis, in accordance with existing guidelines so as to detect signs of imminent failure before the potentially worst outcome becomes a reality.
Heavy precipitation events are common in coastal regions bordering the Mediterranean, and regularly cause flash floods with tragic consequences, as the catchment areas are small and therefore react very quickly to very heavy rainfall (Caumont et al., 2020). Rapid changes in weather dynamics with very heavy rainfall can impact the safety of vulnerable dams.
The most extreme events may be due to the stationary nature of the precipitation or its long duration. In mid-October 2018, the Aude river in Southwest France rose to a height of 7 m, the highest since 1891. The town of Carcassone received 160mm–180 mm of rainfall within 5 hours, and the water level in the city rose 8 m during that period. At least 14 people died because of the flash flood, mainly in the town of Villegailhenc, Aude, where a small bridge collapsed and was under water.
The storm was triggered by the collision of warm and humid air from the Mediterranean with colder air around the Massif Central. This weather pattern occurs just a few times per year, but there are indications that the frequency and severity are increasing. This suggests the downward counterfactual that the rainfall might have been even more intense and prolonged, with the influence of a stronger Hurricane Leslie transitioning into an extra-tropical cyclone.
An increase in extreme precipitation events is expected for northwestern Mediterranean watersheds under climate change (Colmet-Daage et al., 2018). In the area of Pezens, the population of around 1,800 were evacuated due to fears that a nearby dam could burst. Upstream of Pezens are several dams, such as Cenne-Monestiés, in the commune of Saissac. By luck, the sluice gates of one of the local dams had been opened the previous day for maintenance work (Perez, 2018). As a consequence, Le Fresquel river catchment was almost dry. Counterfactually, more intense and prolonged rainfall might have triggered a dam failure. Without the good fortune associated with the timely scheduling of maintenance work, the regional flooding might have been much worse than it turned out. Flood risk is one of the most challenging georisks to model. Merz et al. (20121) have pointed out that when exploring the possibility space of future floods, biases of wishful thinking should be avoided by purposefully constructing downward counterfactuals.
Another European example of heavy rainfall creating a dam failure crisis occurred the following year in central England. Toddbrook Reservoir is located just upstream of the town of Whaley Bridge in the High Peak area of Derbyshire, England. The almost 80-foot tall embankment was constructed between 1837 and 1840 for water supply. At the time of construction, Toddbrook Reservoir Dam was the tallest dam in the United Kingdom. Toddbrook Dam was originally constructed with a single spillway at the left abutment of the dam.
A concrete auxiliary spillway was completed in 1970 on the central portion of the downstream face of the embankment. This auxiliary spillway suffered a serious incident on 1 August 2019 after almost a week of intense rainfall occurring during two separate storm events. On the river Goyt, the peak flow on July 31 had a return period estimate of 20–45 years (Sefton et al., 2021). Counterfactually, a longer return period peak flow, such as happened in 1819, would have been even worse.
The reservoir peaked during the evening of July 31, and distress in the auxiliary spillway chute panels was observed starting the next morning. According to the local Derbyshire police chief, the structural integrity of the dam wall was at a critical level and there was a substantial risk to life should the dam wall fail. Counterfactually, the dam might have failed if the heavy rain had persisted, and emergency remedial measures not been taken, such as the use of military helicopters to drop sand bags.
A year before the spillway failure, an inspector identified the risk posed by the inadequate spillway design and recommended measures to address the deficiencies. However, he did not convey any sense of urgency or propose any interim risk reduction measures, such as a drawdown of the reservoir, so no actions to address these known deficiencies had been completed.
In an independent review of the failure, Balmforth (2020) determined that the most likely cause of the failure of the auxiliary spillway at Toddbrook Reservoir was its poor design, exacerbated by intermittent maintenance over the years which would have caused the spillway to deteriorate. A downward counterfactual perspective on near-misses would have heightened the sense of urgency in tackling the risk posed by inadequate spillway design.
There is a natural tendency for systems to move towards failure: identification of near-misses is an observational tool that can track this progression. Continual human effort is needed to maintain order and prevent failure, especially throughout the life of a structure like Toddbrook Dam, which is more than 180 years old. Downward counterfactual analysis is a conceptual aid to this much needed continual human effort.
3.2 Nuclear power plant downward counterfactuals
The International Atomic Energy Agency (IAEA) establishes very high standards of nuclear safety around the world, and nuclear accidents are accordingly very rare. As and when near-misses occur, lessons need to be learned for the benefit of the global nuclear power community. As one US example, on 27 January 2015, Winter Storm Nor’Easter Juno knocked out both of the 345,000 V transmission lines connecting the Pilgrim nuclear plant in Plymouth, Mass. The reactor automatically shut down when the second offsite power line was lost. Equipment problems and operator errors complicated the intended response. In March 2012, federal regulators had required nuclear station operators to evaluate existing risk to floods, including those linked to climate change. A tightening of safety requirements often follows a major accident, such as happened the previous year in Japan.
3.2.1 Downward counterfactual analysis of the Fukushima Dai’ichi accident
At 2.46p.m. on Friday, 11 March 2011, a great Mw9.0 earthquake occurred offshore northern Japan, with an epicenter 130 km east of Sendai on the east coast of Honshu. The ground motion automatically triggered shutdowns of several nuclear power facilities along the northeast coast of Honshu. Although each of these nuclear facilities experienced some damage, shutdown procedures were able to achieve and maintain a safe, cold shutdown condition in all plants, except for those at Fukushima Dai’ichi.
Fukushima Dai’ichi units 1, 2, and 3 were operating at the time of the earthquake and consequent tsunami. Units 4, 5, and 6 had previously been taken offline for inspection and planned maintenance. The plants had been modified in 2002 with a seawall designed to withstand a tsunami with a height of 5.7 m. But this was less than half the unprecedented wave height of 14–15 m of the tsunami which arrived within an hour of the earthquake. The prime reason for this disparity was that the maximum magnitude had been significantly underestimated (Uchida and Bürgmann, 2021) on the basis of the age of the subduction zone, to be 8.2, which is far less than 9.0 (Ruff and Kanamori, 1980). Such an underestimation poses a significant human factor in seismic risk assessment. The subjective element in seismic hazard analysis is reflected in the dominance of Kanamori’s expert opinion over statistical evidence that there was a high probability of Mw9.0+ earthquakes in Japan (Pisarenko and Rodkin, 2010). The massive flooding that ensued disabled critical equipment, including all six external power supply sources and on-site backup power emergency diesel generators. The power loss resulted in loss of coolant to each of the reactors 1, 2, and 3, and associated spent-fuel pools. As the facility experienced a significant loss of cooling capabilities, the pressures within the primary containment vessels rose beyond design limits, and plant operators started primary containment venting to prevent extensive damage to the reactor, and a possible large-scale environmental release of radioactive material.
In the afternoon of March 12, a hydrogen explosion occurred at the top of the unit 1 building, severely damaging the roof and walls of the top floor. On March 14, unit 3 also experienced a large hydrogen explosion, damaging the upper portion of the building. On March 15, unit 2 experienced an explosion. These three hydrogen explosions not only released a significant amount of radioactivity into the environment, but also impeded emergency work and attempts to provide active cooling. Counterfactually, there might have been additional hydrogen explosions at the other reactors 4, 5, and 6, had they not been offline. As it was, on March 17, one electrical generator at unit 6 was restored to operation, and external power was returned to units 5 and 6. The facility was unable to maintain adequate cooling of the spent-fuel pools in units 1 to 4. Overheating of the unit 4 pool led to a hydrogen explosion which caused significant damage to the upper floors of the reactor building, and the release of additional radioactivity.
The prevailing wind in Japan blows from the west, and would have carried most of the radioactivity east over the Pacific Ocean. Counterfactually, had the accident occurred one or 2 months earlier, the transport of nuclear radiation in the atmosphere across the Pacific would have been faster, so increasing the international impact (Qiao et al., 2011). But other counterfactuals might have had a greater societal impact within Japan.
As shown in Figure 2, there was significant onland deposition to the northwest, and an additional pathway to the southwest. To control public exposure, an evacuation zone of 20 km was established, with a shelter-in-place zone of 30 km. Early protective actions taken by Japanese authorities mitigated the public risk from radioactive iodine and caesium.
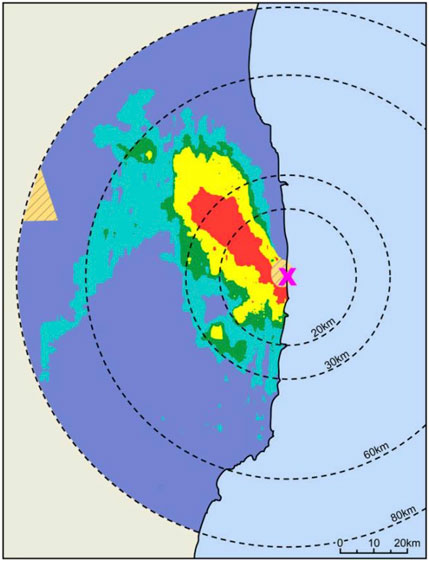
FIGURE 2. Geographic distribution of radioactive caesium fission products after the Fukushima Daiichi nuclear accident, based on aerial monitoring. In MBq/m2: Zone red 3-30; yellow 1-3; green 0.6-1; light blue-green 0.3-0.6; darker blue <0.3 (From Dauer et al. (2011)).
Despite their huge impact on coastal areas, the Mw9.0 earthquake and tsunami impacts were largely regional. Industrial production for the whole of Japan declined by 15% in April 2011, but was back to its pre-earthquake growth rate a year later (Carvalho et al., 2021). Even though infrastructure across northeast Japan was severely affected by the Mw9.0 earthquake and tsunami, pre-earthquake levels of activity were largely restored in a few weeks, with the obvious exception of electricity supply because of nuclear plant outages.
With all industrial facilities, there are occasional periods of maintenance, which are generally kept as short as possible to minimize loss of production. It was fortunate that units 4, 5, and 6 were offline at the time of the earthquake and tsunami. Counterfactually, had they been operational, there would have been pressure build-up and hydrogen explosions, (as with units 1, 2, and 3), which potentially might have doubled the release of radioactivity. Within the nuclear industry, the annual proportion of offline time is typically about 10%.
The prevailing meteorological conditions of wind direction and rainfall are further salient downward counterfactuals. As shown in Figure 2, most of the radiation caused environmental pollution in rural mountainous areas of low population. Counterfactually, the radiation may have been driven more southwards towards Ibaraki prefecture, and the rainfall might have dumped radiation over Ibaraki and Chiba prefectures. A dangerous consequence would have been radiological pollution of the water supply to Tokyo. 38% of Tokyo’s water supply comes from the Edo River, which had a significant concentration of 3.51 Bq/g of Iodine-131, following the Fukushima Dai’ichi nuclear accident. The Edo river is a boundary between Chiba and Tokyo prefectures. Because of the heightened risk to those of low body mass, bottled water was provided to infants by the Tokyo Metropolitan Authority.
As indicated after the 1986 Chernobyl disaster (Vakulovsky et al., 1994), after the period of atmospheric fallout originating from the accident and following radioactive decay of the short-lived radionuclides such as iodine-131, the major contribution to radioactive contamination of natural waters is made by Cesium-137, which has a long half-life of 30 years. Cesium-137 accumulates in the body’s soft tissues, where it increases cancer risk. A detailed study of Cesium-137 deposition and contamination of soils over all of Japan was made by Ysunari et al. (2011). Counterfactually, with the doubling of the amount of radioactive release, and the directivity southwards of the radioactive plume, the Cesium-137 contamination in the Edo River might have been as much as four or five times what it actually was in March 2011. The conditional probability of this downward counterfactual can be estimated as 22.5%, being the product of the probability that all the Fukushima Dai’ichi Units were operational and the probability of the wind blowing towards Ibaraki and Chiba prefectures.
Even if the radiological fallout in the Tokyo metropolitan area were well below the threshold dosage for personal injury, there would be widespread concern over the safety of the water supply. This would be compounded by Cesium-137 contamination of agricultural produce, and the impact on farming and fisheries. Widespread fear might take hold and cause panic among Tokyo residents, leading to the potential of recommended evacuation for visitors and foreign residents of Tokyo.
At the time of the March 2011 Fukushima accident, the United Kingdom chief scientific adviser, John Beddington, was requested by the United Kingdom government to consider the desirability of such an evacuation of United Kingdom citizens from the Tokyo region. Given that most of the radiation release had been directed elsewhere, no such evacuation was recommended nor took place (Grimes et al., 2014). However, this was by no means a straightforward decision. Under the downward counterfactual scenario, such an evacuation might well have been recommended.
As noted by Cialdini (2007) in his influential book on the psychology of persuasion, such an evacuation of United Kingdom citizens might have been taken as social proof of the desirability of other foreign residents of Tokyo to evacuate. This in turn might have triggered a cascade of self-evacuation among Tokyo residents. The bitter memory of the fallout from Hiroshima in 1945 is deeply imprinted in the Japanese psyche, and the evacuation of foreigners might have served as a tipping point for the evacuation of Japanese citizens.
In the event of a radiological accident threatening Tokyo, the decision-making process of civil authorities, as well as households and corporations, would involve a complex cascade of mitigating defensive actions, which would impact the entire Japanese national economy, and disrupt the more fragile supply chains. As one corporate example, Hitachi, a global electronics company with 360,000 employees worldwide, had office and manufacturing sites spread around Ibaraki and Chiba prefectures (see Figure 3). Concern over Iodine-131 exposure, which carries a thyroid cancer risk, might have persuaded the Hitachi management to close sites for a number of weeks to allow for the decay of Iodine-131, which has a short half-life of 8 days.
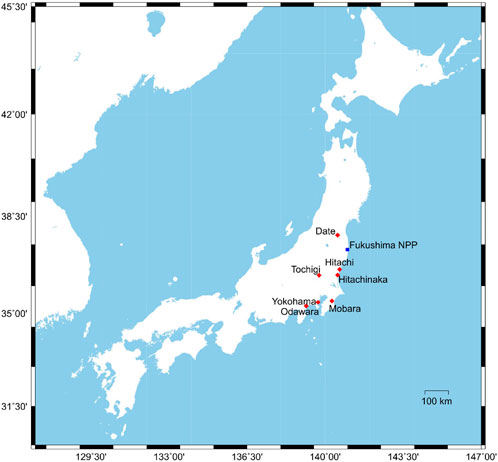
FIGURE 3. Map of Japan, showing Hitachi and other manufacturing sites in China and Ibaraki prefectures.
After the Fukushima accident, Hitachi had been able to maintain shipment of automotive parts. This may not have been possible under the downward counterfactual scenario, where there would have been even greater supply bottlenecks of automotive parts than those which already paralyzed the automobile industry after the Fukushima accident. The production complex for xirallic metallic paint at the Merck plant at Onahama, Fukushima prefecture, was shut down when the earthquake struck. This was the only plant in the world manufacturing xirallic; the consequent bottleneck highlighted the need for just-in-case strategies as alternatives to just-in-time management, if resilience is to be maintained.
Beyond the impact within Japan, this downward counterfactual cascade would have increased safety pressure on the civil nuclear industry worldwide, and might have encouraged more countries to follow Germany and abandon nuclear power, with negative consequences on the global control of greenhouse gas emissions, and on the political influence of major gas producers, such as Russia.
A Bayesian network provides a basic graphic representation of the downward counterfactual pathways towards cascading losses. The tree structure defines the causal structure of a cascade. For economic analysis of the cost-effectiveness of alternative choices of risk mitigation, probabilities may be assigned to the individual branches. The purpose here is to identify a quantitative framework for cascade analysis, which could be adopted for important government applications, such as a large scale evacuation, which was initially contemplated for Tokyo (Japan Atomic Energy Commission, 2011; Fackler, 2012).
A Bayesian network capturing the key features of the downward counterfactual multi-risk cascade is shown in Figure 4. There are nodes for the operation of units 4, 5, and 6; the wind blowing radiation towards Ibaraki and Chiba; rain dumping radiation further south; contamination of tap water, and agriculture products; public anxiety and panic leading to the recommended evacuation of foreign visitors and tourists and some Japanese self-evacuation; impact on Hitachi and other important international businesses; and substantial Tokyo business disruption and supply chain failure.
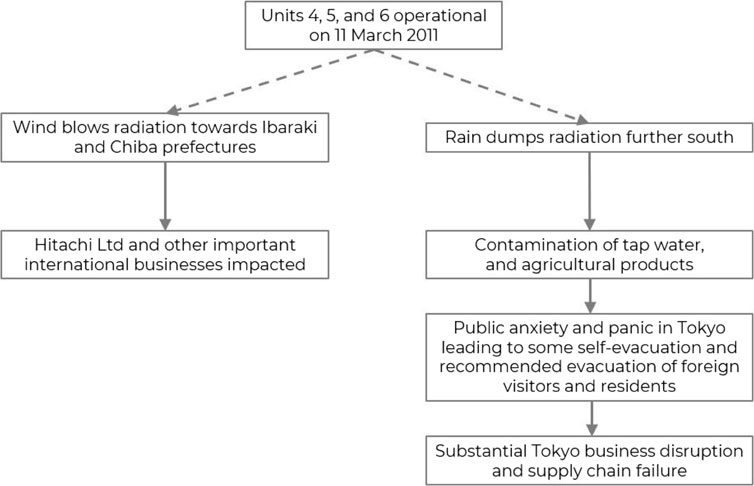
FIGURE 4. Bayesian network showing the key features of the downward counterfactual analysis. Dashed arrows link independent events, while dependent events are linked by a solid arrow.
The radiation released from the Fukushima accident was 10% of that from the 1986 Chernobyl disaster in Ukraine, which stands as the world’s worst nuclear accident. Lessons from Fukushima should be learned not only from what actually happened, but also what might have happened. The radiation release might well have been significantly larger, and the socio-economic consequences far worse. There is a downward counterfactual route crossing the tipping-point boundary, with a substantially higher cascade socio-economic loss than actually materialized on 11 March 2011.
4 Conclusion
The compounding of an external geohazard event with human factors can generate a multi-risk loss cascade. Any near-miss presents an opportunity to learn and take action to prevent the crossing of the tipping point boundary into the domain of a multi-risk disaster cascade. Downward counterfactual analysis of multi-risk cascades is an insightful tool for promoting risk awareness and enhancing resilience. General motivation for this tool comes from the applicability of power-law distributions in describing multi-risk cascades (Pisarenko and Rodkin, 2014).
After the Tubbs wildfire in California, for which PG&E was absolved from responsibility, a downward counterfactual analysis would have alerted PG&E to the urgency of a substantial increase in inspections and patrols. Downward counterfactual analysis is an antidote to cognitive outcome bias, which presumes that urgent action is not needed over near-misses, or at least is not a priority. For PG&E, such outcome bias was dispelled as early as the following year with the even more disastrous Camp fire. Decision-making on corporate safety expenditure should include consideration of downward counterfactuals.
Even the largest safety-conscious organizations are prone to outcome bias: NASA missed a number of opportunities to learn prior to the Challenger disaster of 2003 (Dillon and Tinsley, 2008). During the Challenger launch, a piece of the insulating foam broke off from the Space Shuttle external tank, and struck the thermal protection system tiles on the orbiter’s left wing. This had happened before: similar foam shedding had occurred during previous Space Shuttle launches, causing damage from minor to near-catastrophic.
Downward counterfactual analysis would have prepared the Turkish population better for runaway multiple fault segment ruptures, such as might have occurred on the North Anatolian Fault in 1999, and eventually did occur on the East Anatolian earthquake of 6 February 2023. The loss cascading impact of systemic building code non-compliance and government building amnesties could also have been anticipated.
Most types of disaster might have happened before in a long historical record. Downward counterfactual analysis of the 869 Sanriku earthquake, which left a large tsunami footprint covering three prefectures, would have warned of the possibility of earthquakes off Northeast Japan far larger than the 8.2 maximum magnitude estimated before 2011, with correspondingly massive tsunamis.
It is insightful from a risk management perspective to consider how the Fukushima Dai’ichi accident might have been worse. Counterfactually, the amount of radioactive release might have been higher with the operation of units 4, 5, and 6; and the meteorological conditions might have driven the radioactive plume southwards towards Ibaraki and Chiba prefectures. Elevated levels of radioactive contamination of the rivers supplying tap water to Tokyo might have been sufficient to discourage visitors and foreign residents from staying in Tokyo. Through social proof, such foreign evacuation might have encouraged some Japanese residents to self-evacuate at least until the concentration had dissipated of the thyroid cancer threat Iodine-131. This would have resulted in massive business interruption, commercial chaos and supply chain disruption.
Until 2011, Japan was generating some 30% of electricity from its nuclear reactors and this was expected to increase to at least 40% by 2017. The plan is now for at least 20% by 2030, from a depleted number of nuclear reactors. The downward counterfactual of the multi-risk cascade following the 11 March 2011 accident would have scaled back this 2030 plan, to the detriment of the goal of eliminating fossil fuel consumption as soon as possible. Increased reliance on hydroelectric and nuclear power in a decarbonized world requires enhanced scrutiny of the safety of dams and nuclear power plants, including downward counterfactual analysis of disaster near-misses, accounting for the compounding of geohazard and human factors.
Data availability statement
Publicly available datasets were analyzed in this study. This data can be found here: https://www.meteoblue.com/it/tempo/archive/windrose.
Author contributions
MG developed the multi-risk approach within the CORE project, linking science and human factors; GW created the downward counterfactual methodology; EB designed the Bayesian network analysis with OA and FN; RR was the manuscript reviewer and ethics coordinator; PC was the overall project manager. All authors contributed to the article and approved the submitted version.
Funding
This work was undertaken as part of the EU Horizon 2020 CORE project: Science and Human Factor for Resilient Society [SU-DRS01-2018-2019-2020], under grant agreement No. 101021746.
Conflict of interest
The authors declare that the research was conducted in the absence of any commercial or financial relationships that could be construed as a potential conflict of interest.
Publisher’s note
All claims expressed in this article are solely those of the authors and do not necessarily represent those of their affiliated organizations, or those of the publisher, the editors and the reviewers. Any product that may be evaluated in this article, or claim that may be made by its manufacturer, is not guaranteed or endorsed by the publisher.
References
Alexander, D., and Magni, M. (2013). Mortality in the L’aquila (central Italy) earthquake of 6 April 2009. PLOS Curr. 5, e50585b8e6efd1. doi:10.1371/50585b8e6efd1
Alexander, D. (2010). The L'aquila earthquake of 6 April 2009 and Italian government policy on disaster response. J. Nat. Resour. Policy Res. 2, 325–342. doi:10.1080/19390459.2010.511450
Aspinall, W. P., and Woo, G. (2019). Counterfactual analysis of runaway volcanic explosions. Front. Earth Sci. 7. doi:10.3389/feart.2019.00222
Balmforth, D. (2020). Toddbrook reservoir independent review report. Available at: www.gov.uk/government/publications.
Butte County District Attorney (2020). The Camp fire public report. Available at: www.buttecountynet/da.
Cameron, D. (2023). Emerging diseases and learnings from COVID-19 – oral evidence to UK parliamentary committee from former Prime Minister.
Carvalho, V. M., Nirei, M., Saito, Y. U., and Tahbez-Salehi, A. (2021). Supply chain disruptions: Evidence from the great east Japan earthquake. Q. J. Econ. 136, 1255–1321. doi:10.1093/qje/qjaa044
Caumont, O., Mandement, M., Bouttier, F., Eeckman, J., Brossier, C. L., Lovat, A., et al. (2020). The heavy precipitation event of 14-15 october 2018 in the Aude catchment: A meteorological study based on operational numerical weather prediction systems, and standard and personal observations. Nat. Hazards Earth Syst. Sci. 21, 1135–1157. doi:10.5194/nhess-21-1135-2021
Chadha, R. K. (2023). An Mw7.8 earthquake on 6 february 2023 on the East Anatolian Fault, Turkey. J. Geol. Soc. India. 99, 449–453. doi:10.1007/s12594-023-2331-z
Cinti, F. R., Pantosti, D., DeMartini, P. M., Pucci, S., Civico, R., Pierdominici, S., et al. (2011). Evidence for surface faulting events along the Paganica fault prior to the 6 April 2009 L’Aquila earthquake (Central Italy). J. Geophys. Res. 116, B07308. doi:10.1029/2010jb007988
Ciullo, A., Martius, O., Strobl, E., and Bresch, D. N. (2021). A framework for building climate storylines based on downward counterfactuals: The case of the European Union Solidarity fund. Clim. Risk Manag. 33, 100349. doi:10.1016/j.crm.2021.100349
Colmet-Daage, A., Sanchez-Gomez, E., Ricci, S., Llovel, C., Borrell Estupina, V., Quintana-Seguí, P., et al. (2018). Evaluation of uncertainties in mean and extreme precipitation under climate change for northwestern Mediterranean watersheds from high-resolution Med and Euro-CORDEX ensembles. Hydrol. Earth. Syst. Sci. 22, 673–687. doi:10.5194/hess-22-673-2018
Dauer, L. T., Zanzonico, P., Tuttle, R. M., Quinn, D. M., and Strauss, H. W. (2011). The Japanese tsunami and resulting nuclear emergency at the Fukushima Daiichi power facility: Technical, radiologic and response perspectives. J. Nucl. Med. 52, 1423–1432. doi:10.2967/jnumed.111.091413
Dillon, R. L., and Tinsley, C. H. (2008). How near-misses influence decision making under risk: A missed opportunity for learning. Manag. Sci. 54 (8), 1425–1440. doi:10.1287/mnsc.1080.0869
Fackler, M. (2012). Japan weighed evacuating Tokyo in nuclear crisis. Tokyo: New York Times. February 27.
Gargava, P. (2020). Report of the joint monitoring committee on the gas leak at LG Polymers chemical plant in RR Venkatapuram Village, Visakhapatnam, Andhra Pradesh. Delhi: Central Pollution Control Board Report. Available at: https://pcb.nic.in.
Grimes, R. W., Chamberlain, Y., and Oku, A. (2014). The UK Response to Fukushima and Anglo-Japanese Relations. Sci. Dipl. 3 (2). http://www.sciencediplomacy.org/perspective/2014/uk-response-fukushima-and-anglo-japanese-relations.
Guk, E., Bar-Massada, A., and Levin, N. (2023). Constructing a comprehensive national wildfire database from incomplete sources: Israel as a case study. Fire 6, 131. doi:10.3390/fire6040131
IFTP (2018). Oroville dam spillway incident. Lexington, KY: Independent Forensic Team Report. Association of Dam Safety Officials. Available at: https://damsafety.org.
Jordan, T. (2013). Lessons of L’Aquila for operational earthquake forecasting. Seismol. Res. Lett. 84, 4–7. doi:10.1785/0220120167
Kahneman, D., and Miller, D. T. (1986). Norm theory: Comparing reality to its alternatives. Psych. Rev. 93 (2), 136–153. doi:10.1037/0033-295x.93.2.136
Koskinas, A., Tegos, A., Tsira, P., Dimitriadis, P., Iliopoulou, T., Papanicolaou, P., et al. (2019). Insights into the Oroville dam 2017 spillway incident. Geosciences 9, 37. doi:10.3390/geosciences9010037
Kuligowski, E. (2020). Evacuation decision-making and behavior in wildfires: Past research, current challenges and a future research agenda. Fire Saf. J. 120, 103129. doi:10.1016/j.firesaf.2020.103129
Lin, C. L., Jenkins, S. F., Chow, J. R., Biass, S., Woo, G., and Lallemant, D. (2020). Modeling downward counterfactual events: Unrealized disasters and why they matter. Front. Earth Sci. 8. doi:10.3389/feart.2020.575048
Lin, Y. C., Mestav Sarica, G., Chua, T. J., Jenkins, S. F., Switzer, A. D., Woo, G., et al. (2021). Asia’s looming Black Elephant events. Commun. Earth Environ. 2, 214. doi:10.1038/s43247-021-00283-8
Lozos, J., Harris, R. A., Murray, J. R., and Lienkaemper, J. J. (2015). Dynamic rupture models of earthquakes on the Bartlett springs fault, northern California. Geophys. Res. Lett. 42, 4343–4349. doi:10.1002/2015GL063802
Mart, S. G. (2023). Turkey’s government uses disaster for profit. 28 March. Available at: www,foreignpolicy.com.
Melo, R. T. (2020). We didn’t start the fire…Did we? analyzing why california cannot seem to extinguish its worsening wildfire problem. Vill. Envtl. L.J. 31. Available at: https://digitalcommons.law.villanova.edu/elj/vol31/iss1/5.
Perez, G. R. (2018). Inondations: Dans Pezens, village évacuée en moins de deux heures. Paris: Le Parisien. 15 October.
Pisarenko, V., and Rodkin, M. (2010). “Heavy-tailed distributions in disaster analysis,” in Advances in natural and technological hazards research, volume 30 (Dordrecht-Heidelberg-London-New York: Springer). doi:10.1007/978-90-481-9171-0
Pisarenko, V., and Rodkin, M. (2014). “Statistical analysis of natural disasters and related losses,” in Springer briefs in Earth sciences (Dordrecht-Heidelberg-London-New York: Springer), 82. ISBN: 978-3-319-01453-1 (Print) 978-3-319-01454-8 (Online).
Qiao, F. L., Wang, G. S., Zhai, W., Zhao, J. C., Dai, D. J., Song, Y. J., et al. (2011). Predicting the spread of nuclear radiation from the damaged Fukushima nuclear power plant. Chin. Sci. Bull. 56, 1890–1896. doi:10.1007/s11434-011-4513-0
Rabonza, M. L., Lin, Y. C., and Lallemant, D. (2022). Learning from success, not catastrophe: Using counterfactual analysis to highlight successful disaster risk reduction interventions. Front. Earth Sci. 10. doi:10.3389/feart.2022.847196
Roese, N. J., and Olsen, J. M. (1995). What might have been: The social psychology of counterfactual thinking. London: Psychology Press.
Roese, N. J. (1997). Counterfactual thinking. Psychol. Bull. 121, 133–148. doi:10.1037/0033-2909.121.1.133
Ruff, L., and Kanamori, H. (1980). Seismicity and the subduction process. Phys. Earth Planet. Int. 23 (3), 240–252. doi:10.1016/0031-9201(80)90117-X
Seed, H. B., Seed, R. B., Harder, L. F., and Jong, H-L. (1989). Re-evaluation of the lower san Fernando dam, report 2: Examination of the post-earthquake slide of february 9, 1971. WES contract report GL-89-2. Vicksburg, Mississippi: United States Army Corps of Engineers.
Sefton, C., Muchan, K., Parry, S., Matthews, B., Barker, L. J., Turner, S., et al. (2021). The 2019/2020 floods in the UK: A hydrological appraisal. Weather 76 (12), 378–384. doi:10.1002/wea.3993
Silva, V., and Paul, N. (2021). Potential impact of earthquakes during the 2020 COVID-19 pandemic. Earthq. Spectra 37 (1), 73–94. doi:10.1177/8755293020950328
Spearing, L. A., and Faust, K. M. (2020). Cascading system impacts of the 2018 Camp fire in California: The interdependent provision of infrastructure services to displaced populations. Int.J. Disaster Risk Reduct. 50, 101822. doi:10.1016/j.ijdrr.2020.101822
Staff, T. (2021). Report: Investigators increasingly believe Jerusalem area blaze caused by arson. Jerusalem: The Times of Israel. August 20.
Sullivan, E., Jackson, C., Broberg, D., O’Dair, M., and Velan, V. (2020). California lawmakers should take action to mitigate the effects of the 2019 PG&E bankruptcy. UC Berkeley: Science Policy Group. Available at: https://escholarship.org/uc/item/3vm5d69x.
Sykora, D. (2019). Case study: Lower san Fernando dam (California, 1971). Available at: www.damfailures.org.
Uchida, N., and Bürgmann, R. (2021). A decade of lessons learned from the 2011 tohoku-oki earthquake. Rev. Geophys. 59. doi:10.1029/2020RG000713
Vakulovsky, S. M., Nikitin, A. I., Chumichev, V. B., Katrich, I. U., Voitsekhovich, O. A., Medinets, V. I., et al. (1994). Cesium-137 and strontium-90 contamination of water bodies in the areas affected by releases from the Chernobyl nuclear power plant accident: An overview. J. Environ. Radioact. 23, 103–122. doi:10.1016/0265-931x(94)90055-8
Woo, G., and Mignan, A. (2018). Counterfactual analysis of runaway earthquakes. Seismol. Res. Lett. 89 (6), 2266–2273. doi:10.1785/0220180138
Woo, G., Maynard, T., and Seria, J. (2017). Reimagining history: Counterfactual risk analysis. Available at: www.lloyds.com.
Woo, G. (2019). Downward counterfactual search for extreme events. Front. Earth Sci. 7. doi:10.3389/feart.2019.00340
Woo, G. (2021). A counterfactual perspective on compound weather risk. Weather Clim. Extrem. 12, 100314. doi:10.1016/j.wace.2021.100314
Ysunari, T. J., Stohl, A., Hayano, R. S., Burkhart, J. F., Eckhardt, S., and Yasunari, T. (2011). Cesium-137 deposition and contamination of Japanese soils due to the Fukushima nuclear accident. PNAS 108 (49), 19530–19534. doi:10.1073/pnas.1112058108
Keywords: downward counterfactual, multi-risk, CASCADE, geohazard, human factors
Citation: Gargiulo MV, Woo G, Battimelli E, Amoroso O, Napolitano F, Russo R and Capuano P (2023) Downward counterfactual analysis of multi-risk cascades. Front. Earth Sci. 11:1236321. doi: 10.3389/feart.2023.1236321
Received: 07 June 2023; Accepted: 17 July 2023;
Published: 25 July 2023.
Edited by:
Carmine Galasso, University College London, United KingdomReviewed by:
Gemma Cremen, University College London, United KingdomMikhail Rodkin, Institute of Earthquake Prediction Theory and Mathematical Geophysics (RAS), Russia
Copyright © 2023 Gargiulo, Woo, Battimelli, Amoroso, Napolitano, Russo and Capuano. This is an open-access article distributed under the terms of the Creative Commons Attribution License (CC BY). The use, distribution or reproduction in other forums is permitted, provided the original author(s) and the copyright owner(s) are credited and that the original publication in this journal is cited, in accordance with accepted academic practice. No use, distribution or reproduction is permitted which does not comply with these terms.
*Correspondence: M. V. Gargiulo, bWdhcmdpdWxvQHVuaXNhLml0