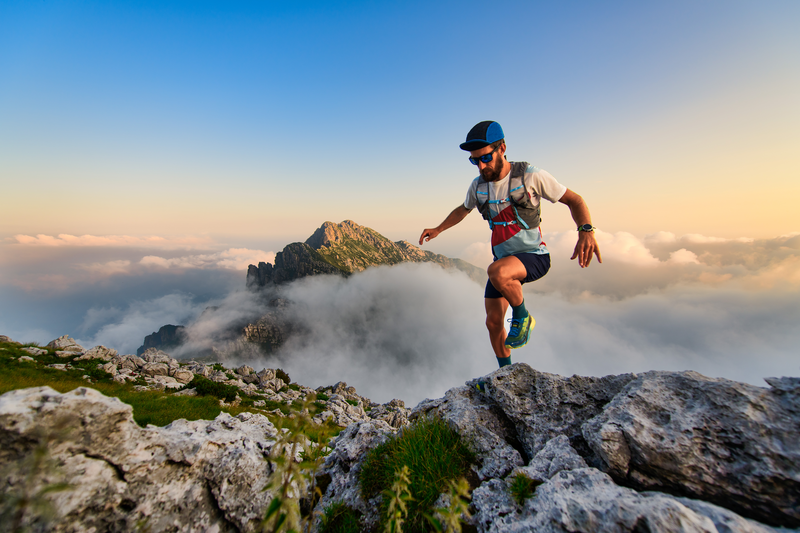
95% of researchers rate our articles as excellent or good
Learn more about the work of our research integrity team to safeguard the quality of each article we publish.
Find out more
ORIGINAL RESEARCH article
Front. Earth Sci. , 08 November 2023
Sec. Geomagnetism and Paleomagnetism
Volume 11 - 2023 | https://doi.org/10.3389/feart.2023.1225271
This article is part of the Research Topic Structural Inheritance at Tectonic Margins View all 4 articles
Geological evidence indicates that the deformation along the southwest Gondwana margin began during the Middle-to-Late Devonian (the Acadian-Cháñica orogenic phase in Argentina). It has been interpreted that this deformation occurred as a consequence of the collision of Chilenia from the west and Patagonia from the south-southwest with Gondwana. As both Chilenia and Patagonia collided at the same time, in this study, we proposed that these continental blocks conform to the same allochthonous drift terrain, named here as CHIPA. The geological evolution of this margin is still under debate. Field work, paleomagnetic studies, and anisotropy of magnetic susceptibility (AMS) studies were integrated from different localities along this paleomargin in Argentina. In Permian rocks, all the geological indicators show a clear regional NW-SE elongation signature and NE-SW shortening direction. The middle Devonian to Permian patterns are more complex as the result of stress interference and the overlapping of orogenic activities with different intensities and ages. The deformation that started as the product of the CHIPA collision with Gondwana during the Middle Devonian continued through the Permian (the Hercinian-San Rafael orogenic phase in Argentina) as post-collisional compressive deformation, consequence of the paleogeographic re-organization of Gondwana and Laurentia, which moved toward the Equator from the south and the north, respectively, to form the Pangea supercontinent during the Triassic.
Although Dalmayrac et al. (1980) proposed the existence of a “Southeast Pacific continent,” which collided with Gondwana during the Paleozoic, the controversy about the origin of Patagonia only began after (Ramos 1984; Ramos 2008) who proposed that Patagonia should be recognized as an allochthonous terrain. From that moment on, new models emerged that can be grouped into collisional or intracontinental deformation evolutional models (see references in Tomezzoli and Cristallini, 2004; Tomezzoli, 2012). There are still doubts about Patagonia possible, particularly regarding when the main deformation occurred along this part of the margin of Gondwana and whether it was associated with collisions. Although some researchers have proposed that the deformation occurred in a single phase during the late Permian-Triassic, others have suggested that it began in the Late Devonian-Early Carboniferous and continued up to the Permian.
The accepted terrains accreted on the western Gondwanan cratonic nucleus (Río de la Plata) before the Late Devonian-Early Carboniferous are as follows: Antofalla, Chilenia, Cuyania, and Pampia (Figure 1A). The collision between El Deseado Massif and the North Patagonian Massif would have occurred in the early Paleozoic (Ramos, 2008) or, according to Pankhurst et al. (2006), during the Middle Carboniferous. In recent years, abundant data has emerged demonstrating that during the Middle Devonian (390 Ma approx.) a peak of high-pressure metamorphism occurred at approximately 40°S (Martínez et al., 2012; García-Sansegundo et al., 2016; Hervé et al., 2018; Calderón et al., 2020; Dahlquist et al., 2020; Rapela et al., 2021; Serra-Varela et al., 2021; Oriolo et al., 2023). The evidence allowed Hervé et al. (2016, 2018) to propose a Late Devonian-Early Carboniferous accretion of an island arc complex, while Martínez et al. (2012) concluded that Chilenia would have subducted during the Middle Devonian (390 Ma approx.) below the North Patagonian Massif, extending the southern margin of Chilenia to the current 42° south latitude.
FIGURE 1. (A) Terrains accreted in the Argentinian territory during the Paleozoic. (B) Location map of the studied localities featured in this study along with the Gondwánides belt (Keidel, 1916; bluish stripe) or SamFrau belt (Du Toit, 1927), from Sierras Australes (East) to San Rafael Block (northwest).
The region analyzed in this work extends from the geological area of the Sierras Australes (Sierra de la Ventana or Ventania System), Chadileuvú Block, and San Rafael Block (Figure 1B), in a belt known as the “Cordon de los Gondwánides” (Keidel, 1916) or SamFrau belt (duToit, 1927).
Based on paleomagnetic studies (see the methodology in Valencio, 1980; Butler, 1992), anisotropy of magnetic susceptibility studies (the AMS ellipsoid is coaxial with the deformation ellipsoid; see the methodology in Tarling and Hrouda, 1993), and fieldwork in the localities mentioned above, an evolution model of the area is presented. It integrates a collisional process that began in the Middle Devonian (Acadian orogeny or Fase orogénica Cháñica—ChOP—in Argentina) related to the collision of Patagonia from the south and Chilenia from the west and a post-collisional process that continued until the Permian (Hercinian Phase-Fase orogénica San Rafael in Argentina, SROP-Gondwanan orogeny). The last manifested itself in a non-collisional way, generating intracontinental deformation as part of the adjustment of the previously coupled terrains. At the same time, Gondwana and Laurentia underwent latitudinal movements toward the Equator, resulting in the formation of the Triassic Pangea (Tomezzoli, 2001; Tomezzoli et al., 2018).
Perhaps one of the key localities to understand the paleogeographic evolution of the southwestern margin of Gondwana is the Sierras Australes area in the Buenos Aires province (Figure 1B). These ranges, with a general northwest-southeast strike and northeast vergence, are interpreted as a Paleozoic fold and thrust belt (Tomezzoli and Cristallini, 2004), deforming and exhuming part of the Claromecó Basin (Figures 1B, 2). Here outcrops the most complete stratigraphic column of the southwest Gondwana margin, with rocks ranging from Precambrian to Permian divided into three main orographic/geologic units: the Curamalal, Ventana, and Pillahuincó ranges/groups (Harrington, 1947; Suero, 1972). The oldest unit outcrops to the west and the youngest to the east (Figure 2A).
FIGURE 2. Sierras Australes study area located in the southwest of the Buenos Aires province (see Figure 1). (A) Stratigraphic profile. (B) Structural cross-section taken from Tomezzoli and Cristallini, (2004). Notice that the western sector presents (Sierras de Curamalal and Ventana) more deformed strata than the eastern sector (Sierra de las Tunas and Pillahuincó). The differences in the style of the deformation between the base and the top of the sequence are visible in the outcrops and are therefore indisputable. (C,D) To the west (base of the sequence; Curamalal and Ventana groups), up to eight folding orders were recognized (Harrington, 1947). (E,F) Toward the east (top of the sequence; Pillahuincó group), the folds tend to expand into a cylindrical type and smooth their wavelengths until they become horizontal.
Despite having good exposures, the geology of these ranges is complex, mainly because not all rocks are easily dated and/or distinguishable from each other. The Curamalal and Ventana groups are very si milar, and even the possibility that they are the same sequence repeated by inverse faults has been considered by some authors (Tomezzoli and Cristallini, 2004; Figure 2B). In the quartzites of the Curamalal and Ventana groups (Figures 2C, D), situated in the western sector (the base of the sequence), there is less greenschist metamorphism (Buggish, 1987; Von Gosen et al., 1991), while in the Pillahuincó group (Sierras de las Tunas, north and Sierras de Pillahuincó, south; Figures 2E, F), situated in the eastern sector (top of the sequence), there is a medium-to-high degree of diagenesis (Arzadún et al., 2016). Additionally, there are visible and indisputable differences in the style of the deformation between the base and the top of the sequence. To the west (Curamalal and Ventana groups), up to eight folding orders have been recognized (Figures 2C, D; Harrington, 1947). The folds tend to have shorter wavelengths and overturned defined flanks. Toward the east (Pillahuincó group), the folds tend to expand into a cylindrical type and smooth their wavelengths (Tomezzoli, 2001) until they become almost horizontal (Figure 2E, F) in the easternmost areas of the ranges. Furthermore, within the Pillahuincó group itself, there are differences in the intensity of the deformation, as the base is more deformed than the top (Figures 2E, F). It is not disputed that in the Sierras Australes area, the deformation attenuates in space (to the east–northeast) (Figure 2) and time (up to the Permian) (Tomezzoli, 2001; Arzadún et al., 2018). Moreover, the sequence is covered in unconformity (Cenozoic deposits).
The contact between the Middle Devonian Lolén Formation (Givetiano; Cingolani et al., 2002), which is the youngest unit of the Ventana group (Figure 2A), and the Carboniferous Sauce Grande Formation (Di Pasquo et al., 2008), which is the oldest unit of the Pillahuincó group, is controversial (Figure 3). There is a consensus that between both formations there is a regional unconformity and a hiatus that suppressed part of the Carboniferous (Harrington, 1947; Kilmurray, 1975; Varela, 1978; Andreis, 1979). Nevertheless, certain authors consider this as a rheological unconformity, exhibiting distinct deformation behavior above and below it, suggesting a singular deformation phase that took place during the late Permian (Japas, 1987; Ramos et al., 2017). For others, this unconformity is tectonic and a product of a deformation that started in the Devonian (Massabie and Rossello, 1984; López Gamundi et al., 1995; Tomezzoli, 1997; Tomezzoli and Cristallini, 1998) and continued until the Permian (Tomezzoli, 1997; Tomezzoli and Cristallini, 2004), even folding the Lolén-Sauce Grande unconformity. According to these authors, the Sauce Grande Formation originated from the erosion of the Lolén Formation, which was affected by the Devonian deformation.
FIGURE 3. (A) Structural sketch of the Lolén formation (top of the Ventana group; see Figure 2) at Las Acacias locality. Paleomagnetic sites are indicated. (B) The AMS fabric is oblate and the Kmax and Kint axes are contained in the cleavage planes, lying close to the vertical and aligned with the strike of the structure in a NW-SE direction. The Kmin axes are always grouped in a northeast direction close to the horizontal and perpendicular to those planes. From the disposition of the AMS axes on the cleavage planes, it can be deduced that this fabric is oblate and of tectonic origin (type C to D, following the conceptual model of Weil and Yonkee, 2009; Tomezzoli et al., 2018), indicating a SW-NE maximum compression direction. (C,D) Tight cleavage planes are common, cutting through previous structures and imparting fissility to the rocks. (E) Lolén PP position indicating a Permian remagnetization.
The Lolén Formation (Figures 3A–D) is composed of micaceous sandstones, phyllites, and shales with lenticular beds of fine conglomerate, with a strong northwest-southeast cleavage direction (Sellés Martínez and Quenardelle, 1992; Japas et al., 2001).
In slightly deformed clastic rocks, it is usual to find an oblate-type magnetic susceptibility fabric that responds to the depositional process, with the Kmin axes perpendicular to the bedding planes (Tarling and Hrouda, 1993; Weil and Yonkee, 2009; Hrouda and Chadima, 2020). In the Lolén Formation case (Figure 3B), the Kmax and Kint axes are contained in the cleavage planes that cut the previous folding structures, lying close to the vertical and aligned with the strike of the structure in a NW-SE direction. The Kmin axes are always grouped in a northeast direction close to the horizontal and perpendicular of those planes, defining dominantly oblate ellipsoids of tectonic origin (AMS fabric, transitional between types C and D, following the conceptual model of Weil and Yonkee, 2009). This AMS axes arrangement indicates a SW-NE maximum compression direction (Tomezzoli et al., 2018; Figure 3B). From paleomagnetic studies, it emerges that these rocks bear a postectonic remagnetization carried by hematite and/or magnetite, which is located in the apparent polar wander path (APWP) of South America (Tomezzoli, 2009; Gallo et al., 2017) in the Permian position close to that of the Tunas II paleomagnetic pole (PP; Tomezzoli, 2001; Figure 3E) aged 280.8 ± 1.9 Ma (López-Gamundi et al., 2013; Arzadún et al., 2018). The fact that these rocks are carriers of a postectonic remagnetization and a tectonic magnetic fabric indicates the overlap of different coaxial deformation events, as documented by other authors (Andreis, 1979; Japas, 1989; Sellés Martínez and Quenardelle, 1992; Japas et al., 2001).
The Pillahuincó group of Carboniferous-Permian is separated from base to top in the Sauce Grande, Piedra Azul, Bonete, and Tunas formations (Figure 4A). The Sauce Grande Formation consists of glaciomarine dating to the Late Carboniferous age (Pennsylvanian-Cisuralian; Di Pasquo et al., 2008). The Piedra Azul Formation is primarily comprised of mudrocks, whereas the Bonete Formation, which is of early Permian age, is characterized by fine sandstones with white spots, interbedded with gray mudrocks (Arzadún et al., 2016). The Tunas Formation, of Permian age, has fine-to-medium sandstones interbedded with green and red mudrocks. In this unit, the vegetal structure that corresponds to Glossopteris, Gangamopteris, Lycopsids, and woody tissues can be distinguished. Additionally, there are some tuff levels intercalated in the sequence, indicating the presence of pyroclastic activity in Gondwana during the early Permian, with U/Pb data of 291–280 Ma (López-Gamundi et al., 2013; Arzadún et al., 2018). The study of the Tunas Formation has accumulated knowledge spanning various scientific disciplines over the course of decades. Sedimentary (Andreis et al., 1979), structural (Cobbold et al., 1992; López Gamundi et al., 1995; Choque et al., 2022; between others), compaction (Arzadún et al., 2013; Arzadún et al., 2016; Febbo, 2023), paleomagnetic (Tomezzoli and Vilas, 1999; Tomezzoli, 2001), and AMS studies (Arzadún et al., 2016; Arzadún et al., 2021b; Febbo et al., 2021) indicate that during the Tunas sedimentation, the Claromecó Basin (Figure 1B) underwent significant paleogeographical changes. Differential shortening occurred between the base and top, as noted by Tomezzoli (1999), alongside distinct signs of continentalization, as observed by Zavala et al. (1993). The top of the Tunas Formation outcrops completely horizontally in the center of the basin close to the González Chávez locality (Figure 1B).
FIGURE 4. AMS results in the Pillahuincó group (Arzadún et al., 2021b). (A) Stratigraphic profile. (B) AMS ellipsoids in the Pillahuincó group. Kmax always remains in a NW-SE position, parallel to the axes of the folds. (C) The Sauce Grande Formation (base of the sequence) presents oblate ellipsoids, with Kmin grouped in the first quadrant, almost horizontally, suggesting a flattening of the fabric with tectonic control (type D, following the conceptual model of Weil and Yonkee, 2009). Moving stratigraphically upwards into Piedra Azul, Bonete and also the base of the Tunas Formation, the ellipsoids tend to change to prolate shapes with a persistent Kmax in a NW-SE position, while the Kmin axes grouped in the first and third quadrant tend to move toward the vertical (center of the stereographic network), showing a transition to a dominant sedimentary fabric (type C, following the conceptual model of Weil and Yonkee, 2009). (D) Different aspect between the Sauce Grande Formation (base; deformed) and the Tunas Formation (top; undeformed).
AMS results in the Pillahuincó group (Arzadún et al., 2021a) show that the Kmax axes trend always NW-SE, parallel to the fold axes (Figures 4B–D). The Kmin axis shows a bimodal distribution. At the base of the sedimentary column (Sauce Grande Formation, Figures 4B–D), on the westernmost and more deformed localities, the AMS study yields oblate ellipsoids (T > 0), with Kmin axes grouped in the first quadrant almost horizontally. These Kmin axes, trending SW-NE perpendicular or scattered away from the poles of the bedding planes, suggest a flattening of the fabric with tectonic control (AMS fabric type D, following the conceptual model of Weil and Yonkee, 2009), produced by a maximum compressive stress (σ1) parallel to that direction (Figure 4). The Piedra Azul and Bonete formations (Figure 4C) show ellipsoids with prolate shapes (T < 0) and Kmin axes in the first and third quadrant grouped toward the center of the stereographic net (AMS fabric type C, following the conceptual model of Weil and Yonkee, 2009). At the base of the Tunas Formation, the ellipsoids are oblates again, with the Kmin axes tending to be grouped horizontally (Arzadún et al., 2016; Arzadún et al., 2021b; Figure 5), indicating a reactivation of the deformation (AMS fabric type D, following the conceptual model of Weil and Yonkee, 2009) in a new cycle of greater orogenic activity in the basin during the early Permian, but always maintaining the SW-NE shortening direction (Figure 6). At the top of the sequence, the Kmin axes move again to a vertical position (AMS fabric type A, following the conceptual model of Weil and Yonkee, 2009), accompanied by a decrease in the degree of anisotropy (Arzadún et al., 2016; Figure 5), indicating an attenuation of the deformation to the younger strata located to the east in the center of the Claromecó Basin, in the González Chaves locality (see details of these outcrops in Arzadún et al., 2016; Febbo et al., 2021; Febbo, 2023; Figures 1, 5). The provided results offer additional proof of the migration of the orogenic front toward the foreland basin, where deformation occurred spasmodically in cycles of varying intensity (Figures 5, 6).
FIGURE 5. AMS results in the Tunas Formation (Arzadún et al., 2016), where the ellipsoids are oblates with Kmin axes tending to be horizontal at the base (type D, following the conceptual model of Weil and Yonkee, 2009); through the top they change to the vertical (type C, following the conceptual model of Weil and Yonkee, 2009). It is like the deformation acted spasmodically. The shortening direction indicates that the maximum stress along this part of Gondwana came from the southwest and remained stable during the Early Carboniferous up until the Permian. The deformation propagated in a diachronous fashion eastward to the foreland, displaying signs first of a decrease and a subsequent pick up of intensity to decay again, suggesting cycles of higher and lower deformation intensity; the first one between the Sauce Grande and Bonete formations (nearly 300 to 290 Ma) and the second one in the Tunas Formation (290–276 Ma; Arzadún et al., 2018).
FIGURE 6. (A) Pj vs. T (anisotropy degree and shape parameter, respectively; Jelinek, 1981; Tarling and Hrouda, 1993) showing that along the stratigraphic succession, in the Sierras Australes area, the ellipsoids changes from oblate to prolate, indicating different cycles of tectonic-sedimentary deformation (Arzadún et al., 2016; Arzadún et al., 2021a). (B) simplified curve that shows more clearly the evolution of the deformation. SD (in red): Spasmodic Deformation (moments in which the deformation was reactivated more strongly). The orange arrows indicate the increase of the shortening, from the top to the base, as the deformation decreased at the top of the sequence. R76, Ruta 76; LS, La Susana; SC, San Carlos; GA, Golpe de Agua; AP, Arroyo Paretas; LM, Las Mostazas; 2M, 2 de Mayo; GC, Gonzales Chaves (see location of the sample localities in Figures 1, 5, 6). (C) Comparison with conceptual models (Saint-Bezar et al., 2002; Parés and van der Pluijm, 2003; Weil and Yonkee, 2009).
Comparing our results with the conceptual models proposed by different authors (Saint-Bezar et al., 2002; Parés and van der Pluijm, 2003; Weil and Yonkee, 2009) about the parameters anisotropy degree (Pj) vs. the shape parameter (T), and looking at the evolution of the ellipsoids in the different localities from the base to the top of the sequence (Figure 6), it is clear that they change, showing a decreasing trend of the degree of anisotropy toward the younger formations, located toward the east (Figure 6). The shape parameter shows average values larger than zero (T > 0) in the Sauce Grande Formation, indicating oblate shapes of tectonic (AMS fabric type D, following the conceptual model of Weil and Yonkee, 2009) origin (Kmin in the horizontal) that changed to T average values minor than zero (T < 0) toward the Piedra Azul and Bonete formations, indicating prolate shapes, which suggests a transition to sedimentary fabric (type B to A, following the conceptual model of Weil and Yonkee, 2009), with Kmin moving to the vertical (Figures 4, 6). In the base of the Tunas Formation itself (Figures 5, 6), T values change again to oblate shapes (T > 0) of tectonic origin (Kmin in the horizontal) that move to the top of the sequence to prolate and oblate shapes of sedimentary origin (AMS fabrics are transitional between type D to B-A, following the conceptual model of Weil and Yonkee, 2009). Some changes in the AMS patterns were observed in the subsurface of the Claromecó well PANG 003 (Febbo et al., 2021), confirming that the degree of deformation was gradually attenuated upwards in the sequence to the younger strata and toward the foreland in the eastern Claromecó Basin. The AMS results in the Pillahuincó group are consistent with the outcrop structural observations (see previous references; Figure 2), compaction (Arzadún et al., 2013; Arzadún et al., 2016; Febbo, 2023), and paleomagnetic data (Tomezzoli, 1999; Tomezzoli and Vilas, 1999; Tomezzoli, 2001).
Paleomagnetic studies in the Tunas Formation (Tomezzoli and Vilas, 1999; Tomezzoli, 2001) allow the isolation of a characteristic remanent magnetization with reverse polarity (positive inclination for the Southern Hemisphere) carried by hematite. However, the type of magnetization is different in Sierra de las Tunas (see the San Carlos locality in Tomezzoli and Vilas, 1999) than in Sierra de Pillahuincó (see the Las Mostazas locality in Tomezzoli, 2001). In Sierra de las Tunas, at the base of the stratigraphic column, the magnetizations are syntectonic at 35% of unfolding (Tomezzoli and Vilas, 1999), whereas in Sierra de Pillahuincó, where the strata are younger, the magnetizations are syntectonic at 85% of unfolding (Tomezzoli, 2001). From the final mean directions corrected by structure, the Tunas I and Tunas II paleomagnetic poles (PPs; Tomezzoli and Vilas, 1999; Tomezzoli, 2001; respectively) were calculated. Both PPs have good consistency with coeval paleomagnetic poles from other regions of the southwest Gondwana margin (Tomezzoli, 2009; Gallo et al., 2017) with ages bound between the early Permian (Tunas I PP, with 295.5 ± 8.0 Ma; Arzadún et al., 2018) and early late Permian (Tunas II PP, with 280.8 ± 1.9 Ma; López Gamundi et al., 2013; Alessandretti et al., 2013; Arzadún et al., 2018).
The abrupt change in the trajectory (cusp) of the APWP of Gondwana during the late Paleozoic (see Figure 3E) is accompanied by alternating periods of strong and mild deformation (Figures 4–6), evidenced by geological changes in the outcrops (from Harrington, 1947, onwards), micro-tectonic characteristics (Arzadún et al., 2021a; Choque, 2022; Febbo, 2023), types of magnetizations (Tomezzoli and Vilas, 1999; Tomezzoli, 2001), and different AMS signatures (Arzadún et al., 2016; Arzadún et al., 2021b; Febbo, 2021). The shortening direction indicates that the maximum stress along this part of the Gondwana comes from the southwest and remained stable during the Early Carboniferous up to the Permian. The eastward propagation of deformation toward the foreland initially showed a decrease in intensity, followed by a subsequent increase, and then another decrease. This pattern suggests cycles of alternating higher and lower deformation intensity. The first one occurred between the Sauce Grande and Bonete formations (approximately 300 to 290 Ma), whereas the second took place within the Tunas Formation (290–276 Ma; Arzadún et al., 2016; Arzadún et al., 2018; Arzadún et al., 2021a).
In the same region, different types of igneous rocks outcrop (Figures 1, 7). From east to west, the first outcrop corresponds to the Cerro Colorado granite and the López Lecube syenite, and then the Cerro de los Viejos mylonitic-gneiss (Figures 1, 7). The Cerro Colorado granite is located 40 km west of Sierras Australes (Figures 1, 7A) and was interpreted to be part of the basement (Harrington, 1947; Varela et al., 1990). The body is elongated in a southwesterly direction, approximately 1 km long by 300 m wide, and is mainly composed of granites with a leucocratic tendency and grainy texture, with reddish and grayish tones (Grecco et al., 1984; Grecco and Gregori, 1993; Grecco et al., 1997). From a structural point of view, the Cerro Colorado granite presents a penetrative cleavage that gives rise to a genesis-mylonitic structure (Massabie et al., 1999). In fact, the cleavage is so strong that it is a ballast quarry. The ages calculated for the Cerro Colorado granite are as follows: Rb/Sr 427-392 Ma (Cingolani and Varela, 1973), Rb/Sr 487 ± 15 Ma (Varela et al., 1990), Rb/Sr 381 ± 9 Ma (Massabie et al., 1999), U/Pb 531 ± 4 Ma (Rapela et al., 2003), and U/Pb 523 ± 4 Ma (Tohver et al., 2012).
FIGURE 7. West of the Sierras Australes outcrop. (A) Cerro Colorado granite (this study) with a penetrative cleavage (ballas quarry) that gives rise to a gneiss-mylonitic texture and ages ranging from 531 Ma (Rapela et al., 2003) to 381 Ma (Massabie et al., 1999). (B) Lopez Lecube syenite (application rock quarry; Tomezzoli and Vilas, 1997) is considered post-orogenic because it appears as a very uniform and fresh rock, with no evidence of alteration or tectonic deformation (Cingolani and Varela, 1973, between others). Proposed ages for this rock are 258 ± 2 Ma (Pankhurst et al., 2006), 251.5 ± 3.0 Ma (Tohver et al., 2008), 227 ± 32 Ma, and 240 ± 12 Ma (Cingolani and Varela, 1973). (C) Cerro de los Viejos (structural data from Tickyj et al., 1997); mean magnetic fabric elements: Kmax and Kmin are shown in relation to principal mesoscopic fabric elements (main foliation = s1 and lineation = l1) (Tomezzoli et al., 2003).
The López Lecube syenite emerges 80 km west of Sierras Australes (Figures 1, 7B). This intrusive body is made up of a syenite with an alkaline and granite affinity (Gregori et al., 2003). It appears roughly as a very uniform and fresh rock, in which there is no evidence of alteration or apparent tectonic deformation (Cingolani and Varela, 1973; Tomezzoli and Vilas, 1997). These rocks were interpreted, based on the Rb/Sr age of 227 ± 32 Ma and K/Ar 240 ± 12 Ma, as an intrusive of postectonic origin related to the structural evolution of Sierras Australes (Cingolani and Varela, 1973) and correlated with the Gondwanic magmatic cycle that developed in the La Pampa and Mendoza provinces (located to the west; see Figure 1) due to their similarities in age and chemical composition (Linares et al., 1980; Varela et al., 1985; Rapela et al., 1996). More recently, U/Pb ages of 258 ± 2 Ma (Pankhurst et al., 2006) and 251.5 ± 3.0 Ma (Tohver et al., 2008) were obtained. Paleomagnetic behavior in the Lopez Lecube samples was stable in all the analyzed specimens and a reverse polarity characteristic remanent magnetization could be isolated for the Southern Hemisphere acquired during the Kiaman Supechron (late Permian; Tomezzoli and Vilas, 1997). No paleomagnetic results are available for the Cerro Colorado and Cerro de los Viejos localities.
In some specimens from Cerro Colorado, the AMS was measured, showing the Kmin axes distribution in intermediate positions between the horizontal and vertical in the NE quadrant of the stereographic net (Figure 7A), indicating an AMS fabric of transitional origin between magmatic and tectonic (AMS fabric type C, following the conceptual model of Weil and Yonkee, 2009). This geological body merits more detailed study. The AMS results from Lopez Lecube (Figure 7B) show a prolate pattern (AMS fabric type C, following the conceptual model of Weil and Yonkee, 2009), where the Kmax axis (located to the west) is parallel to the lineation given by the subparallel orientation of prismatic amphibole crystals (Tomezzoli and Vilas, 1997). This AMS fabric was related to the conditions during the magmatic emplacement as there is no evidence of deformation in the data or in the rocks. The AMS results in the López Lecube syenite differ from the regional AMS pattern measured in the other nearest localities (Cerro Colorado granite, this study; Tunas Formation, Arzadún et al., 2016; Arzadún et al., 2021b; Carapacha Basin; Tomezzoli et al., 2006; and Cerro de los Viejos; Tomezzoli et al., 2003), where there is clear tectonic signature.
The great diversity of lithology in the Paleozoic rocks that outcrop in the La Pampa province in the center of Argentina (Figures 1B, 7C) means this area is of special geological interest. Cerro de los Viejos (CLV), well studied by Tickyj et al. (1997), is located north of the Colorado River (Figure 1). This granite body forms an elliptical isolated outcrop, 1.5 km by 1 km, in an area of very low relief characterized by recent sedimentary deposits (Tickyj et al., 1997). U-Pb dating has yielded a concordia age of 468.0 ± 2.7 Ma (Chernicoff et al., 2022). The K/Ar ages in biotite are 304 ± 15 Ma and 330 ± 15 Ma (Linares et al., 1980). K/Ar ages obtained in muscovite are 260 ± 13 Ma, 265 ± 13 Ma, and 261 ± 13 Ma. Rb/Sr ages obtained in muscovite are 279.9, 358.3, and 406.9 Ma (two-point isochron; Ri assumed: 0.715). The 359 Ma age is considered as a mixture of the micas coming from the granite protolite and the micas recrystallized during the main deformation event (Tickyj et al., 1997). The discrepancies in the ages are the result of the superposition of different ductile deformation events. At least two events are the most prominent macroscopically with gradational contact, slightly foliated orthogneiss (or granitic gneiss) and mylonitic gneiss (Tickyj et al., 1997).
The principal and prominent foliation (s1) in the outcrops is defined by the parallelism of lenticular grains and aggregates of feldspar and quartz. S1 is penetrative throughout the mylonitic gneiss, with 146°/27° (average; convention used in this paper for planes: strike and dip RHR; Figure 7C; Tickyj et al., 1997), and is synchronous with a regional medium-grade metamorphism condition, with northeast vergence (Tickyj et al., 1997). In some areas, the granitic gneiss is more quartz-rich, and the contacts between fine and coarse-grained domains form narrow and well-foliated zones, with a well-defined mineral lineation (l1) plunging 25° toward 222°; this lineation is marked by aligned recrystallized quartz-feldspar-mica aggregates and tourmaline (Tomezzoli et al., 2003). The secondary foliation (s2) is observed only locally and is attributed to a NNE shear zone that tilts to the WNW (Figure 7C; Tickyj et al., 1997). Secondary structures in CLV are represented by orthogonal quartz veins (296°/68°; Figure 7C) truncated by undeformed granitic and pegmatitic dikes (036°/78°; Figure 7C). AMS studies have revealed that Kmax is commonly parallel to a major lineation but is sometimes parallel to the intersection line of two foliations (MacDonald and Ellwood, 1987). In the present case, the Kmax axes plunge more gently (06° towards 222°; Figure 7C) than the observed mineral lineation with opposite plunges to the NE and are far from the intersections of s1 and s2 (20° towards 276°; Figure 7C). Contrary to expectations, the AMS mean Kmin axis (67°–123°) does not align well with the mean petrofabric foliation pole (Figure 7C); instead, it corresponds most closely to the secondary foliation s2. The Kmin axes, which might be expected to lie near the foliation poles in the NE quadrant, lie mainly in the SE quadrant (Figure 7C). Therefore, we encounter a somewhat uncommon scenario in which an early strong mineral lineation controls the trend of Kmax, and a weaker secondary foliation determines the orientation of Kmin axes; the AMS Kmin axes for the CLV granitic gneisses clearly indicate the presence of a cryptic foliation that was not obvious in the field (probably an AMS fabric type C, following the conceptual model of Weil and Yonkee, 2009). This cryptic foliation is a secondary weaker foliation marked by oriented micas and opaque oxides, which contribute to the AMS signatures of the rock (Figure 7C; Tomezzoli et al., 2003). This complex pattern with the presence of secondary foliations is testifying that the area was subjected to more than one deformation event.
The Carapacha Basin is a continental half-graben of Permian age (Melchor, 1999) located in the south of La Pampa province, central Argentina, west of Sierras Australes (Figures 1, 8). Basement rocks include Late Cambrian to Early Devonian metamorphic rocks (the Las Piedras Metamorphic Complex), granitoids (the Pichi Mahuida group), and Paleozoic granite orthogneisses (the Cerro de los Viejos Complex, see above) that outcrop to the southeast (Tickyj et al., 1997). The basin filling is up to 630 m thick and entirely composed of clastic deposits of the Carapacha Formation type red and gray arkose or lithic sandstones, mudstones, and scarce conglomerates (Melchor, 1999). The base of the formation is not exposed. It has been divided into two members separated by an unconformity: the Urre-Lauquen member outcropping on the Río Curacó Member (38°11.6′S, 65°55.8′W) and the Calencó Member outcropping on Estancia San Roberto (38°13.5′S, 65°48′W) (Melchor, 1999). The formation has yielded a typical Permian Glossopteris macroflora dated as Early-to-Late Permian (Melchor and Césari, 1997). The succession of the basin is dominated by fluvial facies with subordinate lacustrine deposits. The rocks along the Río Curacó are gently folded, showing two scales of folds: mega-folds (wavelength of 1.1 km) and meso-folds (wavelength of 1–3 m). In addition, strike-slip, normal and reverse faults, and extensional veins are present. Outcrops of the Calencó member make up a homoclinal succession striking 230°/40°. Poor exposure precludes a more definite determination of the tectonic structure. The Carapacha Formation on the Río Curacó is intruded by an andesite, with a K/Ar age of 242 ± 10 Ma (Linares et al., 1980) and 40Ar/39Ar laser fusion age of 236.6 ± 1.0 Ma (Melchor and Llambias, 2000), and small rhyolitic dikes with an Rb/Sr age of 240 ± 2 Ma (Rapela et al., 1996). The volcanic activity in the area is associated with the Permian-Triassic Choiyoi group (big Permian volcanic province in Argentina; Llambias et al., 2003) coming from the neighboring Lihue Calel range. The paleomagnetic study yield two different postectonic paleomagnetic poles (Tomezzoli et al., 2006; Figure 8A). Samples of the Curacó Member have exclusively characteristic reverse polarity magnetization (Curacó PP; Tomezzoli et al., 2006), whereas normal and reverse characteristic magnetizations were isolated in the Calencó Member (Calencó PP; Tomezzoli et al., 2006). The paleopole positions that they occupy in the apparent polar wander path proposed by Tomezzoli (2009) and Gallo et al. (2017) are different (Figure 8A). The Curacó PP occupies an older position in the early Permian (typically reverse Kiaman magnetization; up to 266 Ma according to Gradstein et al., 2004), whereas the Calencó PP occupies a younger position in the late Permian (normal and reverse polarities, post Kiaman magnetizations [Figure 8A]; Gradstein et al., 2004). In the AMS study of the Curacó Member, the Kmax axes reflect the fold axes direction (stretching direction NW-SE; Figure 8B) and the Kmin axes (shortening direction SW-NE; Figure 8B) are bimodally distributed, parallel to the poles of the stratification planes. The relationship between the AMS axes and the structure shows a typically prolate ellipsoid that is tectonically controlled, with a maximal principal stress dominant from a SW-NE direction (AMS fabric type C, following the conceptual model of Weil and Yonkee, 2009). The Calencó Member (younger) shows less deformation than the Curacó Member in the AMS pattern, with typical triaxial ellipsoids (Figure 8B). The Kmax (stretching) is variable and does not reflect the direction of the fold axes. Therefore, it is possible that in the Calencó member the primary sedimentary fabric is more preserved than that in the Curacó Member (AMS fabric transitional between type B and A, following the conceptual model of Weil and Yonkee, 2009). The different paleopole positions calculated for both members of the Carapacha Formation, Curacó and Calencó, are consistent with their lithological, structural, biostratigraphy, and AMS pattern differences. The deformation in the area was linked to the San Rafaelic orogenic phase (SROP).
FIGURE 8. Paleomagnetic and AMS results in the Carapacha Basin (Tomezzoli et al., 2006). (A) Position of the Carapacha Basin PPs in the APWP of South America during the late Paleozoic. Even when both PPs are postectonic (Tomezzoli et al., 2006), the older Curacó member registered a tectonic AMS signature (type C, following the conceptual model of Weil and Yonkee, 2009) data, which was not the case in the Calencó member. (B) Different AMS signatures of both members in the Carapacha Basin (type B to A, following the conceptual model of Weil and Yonkee, 2009).
Close to the Carapacha Basin, less than 10 km away, emerge the volcanic rocks of Sierra Chica (37° 52′ S, 65° 27′ W; Figures 1, 9). This volcanism is considered as the pre-Choiyoi group. The sequence is made up of three units, each possessing distinct lithological, structural, radiometric, and petrofabric characteristics (Quenardelle and Llambías, 1997), in addition to the lack of physical continuity between them (Figure 9A). The oldest unit is composed of trachyandesite flows (outcrops on the route; strongly jointed; Figure 9A1), whereas the other two units are composed of pyroclastic flows of rhyolitic composition, well stratified in the lower part (Figure 9A2) and less in the upper layers (Figure 9A3). According to Quenardelle and Llambías (1997), lithological evidence suggests that these rocks are close to the emitting center and represent the remnant of a volcanic cone. In the tranquiandesitic lava at the base of the sequence, a crystallization age of 276.9 ± 7.9 Ma was obtained with the U-Pb (zircon) method, while in the rhyolitic breccia of the upper sector, the crystallization age is 263.4 ± 1.8 Ma (Tickyj et al., 2015).
FIGURE 9. Sierra Chica locality (Tomezzoli et al., 2013a) paleomagnetic and AMS results. (A) It is possible to appreciate the textural and structural differences between the different units; 1) trachyandesites outcropping on the route with intense jointing; 2) base of the sequence, with clear stratification planes that can reach up to a 50° dip (see hammer for reference) and internal lamination; 3) top units with less than 20° dip stratification. (B) Sierra Chica PP position (Tomezzoli et al., 2009). (C) AMS with different signatures and bedding planes between the base (tectonic; type D, following the conceptual model of Weil and Yonkee, 2009) and the top of the sequence (sedimentary; type C, following the conceptual model of Weil and Yonkee, 2009).
For its paleomagnetic study, 90 specimens distributed in 11 sites were measured (Tomezzoli et al., 2009). In almost all samples, it was possible to isolate a reverse (positive inclination for the Southern Hemisphere) characteristic remanent magnetization (ChRM) direction, located in the southeast quadrant. From the accepted final mean directions (see the discussion in Tomezzoli et al., 2009; Tomezzoli et al., 2013a), different virtual geomagnetic poles (VGPs) were calculated, conistent with the apparent polar wander path of South America (curve proposed by Tomezzoli, 2009; Gallo et al., 2017) with other syntectonic PPs of neighboring areas of early Permian ages (Figure 9B). It should be clarified that for this locality there are two published PPs (Tomezzoli et al., 2009; Domeier et al., 2011), which have the same sampling site, yield (as expected) the same ChRM direction in situ. However, they yield different positions with structural correction; this discrepancy arises because Domeier et al. (2011) calculated the paleopole position (PP) with a structural correction involving a bedding plane that is not present in the area (see explanations in Tomezzoli et al., 2013a). That is the reason why this has been not been taken into account in this study. However, in both studies (Tomezzoli et al., 2009; Domeier et al., 2011), it is clear that there are two different groups of magnetizations: one at the bottom and the other at the top of the sequence.
From the AMS study in the Sierra Chica area (Figure 9C), a significant contrast emerged in the AMS signature between the base and the top of the sequence (AMS fabric type C vs. type A, respectively, following the conceptual model of Weil and Yonkee, 2009) in concordance with the lithological, structural, and paleomagnetic differences mentioned above (Figure 9C). The unconformity within the Sierra Chica volcanic locality occurred after the deposition of the lower units (Figure 9A2) but before the deposition of the upper units (Figure 9A3). This unconformity is likely connected to the San Rafael orogenic phase, recorded in other lower Permian adjacent areas (Tomezzoli and Vilas, 1999; Tomezzoli, 2001; Tomezzoli et al., 2006; Tomezzoli et al., 2018).
Near the Sierra Chica and Lihué Calel ranges, specifically in the town of Puelches, along the Rio Salado-Chadileuvú margins (Figures 1, 10), rock outcrops assigned to the La Horqueta Formation (Chernicoff et al., 2008 and references cited there) are unconformably covered by the easily distinguishable continental Permian sediments of the Carapacha Formation (see above). The La Horqueta Formation is part of the Curacó Basin of Late Ordovician-Devonian age. Aeromagnetic data demonstrated that the depocenter of the basin is aligned according to a narrow magnetic trough of NNW strike (Chernicoff et al., 2008), at the edge of the Cuyania terrain (Figure 1), also extending eastward over the Pampia terrain. The La Horqueta Formation is composed of sandstones and mudstones; U/Pb data in detrital zircons indicate a maximum deposition age of 466 Ma and the minimum age is provided by a granite intrusion of approximately 405 Ma (Early Devonian; Chernicoff et al., 2008). Geochemical data indicate that the Curacó Basin originated in an active continental margin, possibly from the Ordovician Famatinian arc (Chernicoff et al., 2008). The La Horqueta rocks have at least two clear patterns of joints and fractures (Figure 10A) and a metamorphic degree sufficient to completely obliterate the primary sedimentary structures. For that reason, the recognition of the top and base of the sequence, and therefore the structural interpretation of the area and its correlation with the AMS data, was difficult. From the in-situ arrangement of the AMS axes, it appears that most of the ellipsoids are triaxial to prolate type (Figure 10B). The Kmax axes are well grouped, always close to the horizontal in the SE-NW direction, subparallel or parallel to the foliation direction, whereas Kint and Kmin are distributed along a garland in a NE-SW direction (AMS fabric type C, following the conceptual model of Weil and Yonkee, 2009) with a compression component from a SW-NE direction. This Devonian style and degree of deformation is very different from that of the Carboniferous-Permian units (Carapacha Formation), which are gently folded to almost horizontal and lie unconformably over the Devonian (Chernicoff et al., 2008).
FIGURE 10. La Horqueta Formation (Late Ordovician—Devonian) in the Chadileuvú block. (A) outcrops on the Rio Salado, in the town of Puelches, La Pampa province (see also Figure 1; Ochoa, 2017 and this study). Views of the outcrops where it is possible to appreciate the high degree of joints in the rocks, which completely obliterate the primary stratification. (B) AMS results. Kmax axes are well grouped and always close to the horizontal in a SE-NW direction, subparallel or parallel to the direction of the foliation, whereas Kint and Kmin are distributed along a garland in a NE-SW direction. This general distribution of the AMS axes in space is interpreted as a transition to tectonic-type fabric (type C to D, following the conceptual model of Weil and Yonkee, 2009), with a compression component from the SW-NE. This Devonian style and degree of deformation is very different from that of the Carboniferous-Permian (Carapacha Formation), which is gently folded almost to the horizontal and lies in a regional unconformity over the Devonian (Chernicoff et al., 2008)
The volcanic rocks of the Cerro El Centinela (36º39′S-67º20′W; Figure 1) are part of the shoshonitic suite of the Choiyoi group (Permian-Triassic) that outcrops in the NW of La Pampa province (Figures 1, 11). They comprise a continuous volcanic sequence of lava flows that degrade to volcanic breccias, interbedded with pyroclastic rocks (Figure 11A). The set has a homoclinal attitude that changes from Az: 296° to 170°/15°–20° at the base to 152°/17º–15° to the top of the sequence (Tomezzoli et al., 2018). These variations were of primary origin and related to the paleoslope of the depositional environment. Therefore, the paleomagnetic directions were recorded in situ. The age of 276 ± 11 Ma has been obtained, allowing the placement of the top of the volcanic sequence of the Cerro El Centinela in the Kungurian stage of the early Permian (Tomezzoli et al., 2018). Samples were treated with thermal demagnetization. . The rocks carry a reversed ChRM, with positive (downwards) inclinations for the southern hemisphere (Tomezzoli et al., 2018). According to the age determinations, this magnetization was acquired during the Kiaman Superchron.
FIGURE 11. Cerro El Centinela exposed in the northwest of La Pampa province (Figure 1; Tomezzoli et al., 2018), Argentina, as part of the Gondwanides belt; the profile was sampled from the base to the top. (A) Cerro El Centinela consists of a continuous volcanic sequence of lava flows that degrade to volcanic breccias, interbedded with pyroclastic rocks. Stratigraphic boundary between the different ChRM is in the upper part of the sequence (Tomezzoli et al., 2018). (B) Two high quality PPs have been calculated (Tomezzoli et al., 2018). Both PPs have a good consistency with coeval paleomagnetic poles from other regions of the southwest Gondwana margin with ages bound between the early Permian and the early late Permian.
In this area, the ChRM directions were subdivided in two populations, with the stratigraphic boundary located in the upper part of the sequence where the first tuff layer appears at approximately 100 m above the base (Figure 11A; Tomezzoli et al., 2018). By averaging the virtual geomagnetic poles (VGPs), two high quality paleomagnetic poles (PPs) have been calculated (Tomezzoli et al., 2018); they have good consistency with other PPs from neighboring areas along the southwest Gondwana margin (Figure 11B), with ages ranging between the early Permian and the early late Permian. The presence of these two paleopolar positions in the same continuous and undeformed volcanic stratigraphic sequence makes this location an excellent site for studying Gondwana´s paleogeography during the late Paleozoic (Tomezzoli et al., 2018). With these poles, it was possible to precisely track the apparent polar wander path (APWP) for South America during the late Paleozoic and Triassic (Tomezzoli, 2009; Gallo et al., 2017). The plate´s movements and the associated deformation are reflected in the inflection that has the APWP of Gondwana in the late Paleozoic (Tomezzoli, 2009; Gallo et al., 2017).
In the Agua Escondida area (Figures 1, 12), a basement granite assigned to the Piedras de Afilar Formation crops out, where two igneous bodies were identified: La Menta Granite (36º03′S—68º26′W) and Borboran Granite (36º01′S–68º24′W), with concord ages of 388.4 ± 3.0 Ma (Middle Devonian) and 376.6 ± 1.1 Ma (Late Devonian; Tickyj et al., 2015), respectively. Over these granites separated through an angular unconformity lies the marine sediments of the Late Carboniferous (Agua Escondida Formation), which in turn are intruded and covered by igneous rocks that belong to the Choiyoi magmatism, with two different members of concordia ages of 283.8 ± 0,98 Ma (early Permian) and 266.7 ± 1.0 Ma (middle Permian; Tickyj et al., 2014) (Figure 12A). On this set, there are Plio-Pleistocene basalts and recent sediments. AMS measurements were obtained for 132 specimens collected from 13 sites across varied lithologies (Figure 12A). The AMS patterns (Figure 12B) corresponded to the differences in lithologies, texture, and chronology, revealing shifts in primary stress orientations over time, including those of the Plio-Pleistocene basalt.
FIGURE 12. Different lithostratigraphic units were analyzed in the Agua Escondida area, San Rafael Block (Battler, 2015; this work; see Figure 1). (A) The Agua Escondida Formation, including pre-Carboniferous granite (Piedras de Afilar Formation), sedimentary rocks of Late Carboniferous age (Agua Escondida Formation), porphyritic granite (Granito Cavado), rhyolite dikes belonging to the Choiyoi group, and quaternary basalts; in the northwest of the Gondwanides belt, it is observed the unconformity between La Horqueta Formation (Silurian—Devonian; down right) and El Imperial Formation (Carboniferous), where the Cháñica Orogenic Phase (ChOP) was defined in Argentina. (B) the AMS pattern is complex, indicating different maximum compression directions. This could be the result of non-coaxial events, possibly diachronic, that acted regionally and locally obliterating the primary fabrics. (C) The Cochicó PP (Tomezzoli et al., 2005a y b), consistent with other syntectonic PPs from the early Permian in South America.
The San Rafael Block occupies the northwestern end of the Gondwanides belt (Figure 1B). The Devonian deformation (ChOP; Figure 12A) is manifested in the angular unconformity that mediates between La Horqueta (Devonian) and El Imperial formations (Carboniferous; Criado Roque, 1972); the Cochicó group lies unconformably, belonging to the lower section of the Choiyoi volcanism (early Permian), located in a transpressional regime attributed to the SROP (Japas and Kleiman, 2004; Kleiman and Japas, 2009). The Cochicó group is composed of conglomerates, andesitic and ignimbrite breccias, and redeposited aeolian sandstones. U/Pb ages were recently obtained in zircons of 281.4 ± 2.5 Ma (Rocha Campos et al., 2011). Paleomagnetic studies in Sierra Pintada allowed the isolation of a reverse polarity ChRM carried by titanohematites and hematites (Tomezzoli et al., 2002; Tomezzoli et al., 2005a; Tomezzoli et al., 2005b). The Cochicó PP is consistent with other syntectonic PPs from the early Permian of South America (Figure 12C).
North of the San Rafael block, in the southern sector of the Precordillera, the structure was studied in detail by Giambiagi et al. (2010) and other authors cited there and has been proven to be the result of a complex series of overlapping deformation events (Eopaleozoic, Neopaleozoic, Permo-Triassic, and Cenozoic), each of them characterized by structures exhibiting distinct orientations, vergences, and styles. The kinematic analysis of the Eopaleozoic structures allowed these authors to distinguish two deformation events with different kinematic characteristics. The first event, D1, according to Giambiagi et al. (2010), has an E-W maximum shortening direction and western vergence, while the second event, D2, has a NW to WNW maximum shortening direction and double vergence. In one of the models proposed by these authors to explain these kinematic variations, the Permian deformation is characterized by the generation of a thin-skinned fold and thrust belt in the eastern sector and a thick-skinned one in the western area, as the result of the reactivation of the Eopaleozoic structures.
Late Paleozoic granitoids outcrop in the northeast of the North Patagonian Massif; an AMS study was carried out with these rocks (Figure 1B; López de Luchi et al., 2010). The units involve the Late Carboniferous Yaminué Complex and the early Permian Navarrete Plutonic Complex (López de Luchi et al., 2010). These granitoids of ca. 300 Ma were placed under a complex compressive regime with a SSW-NNE shortening direction. Approximately 280 million years ago, this regime shifted to a WNW-ESE direction (López de Luchi et al., 2010). In the same area, the absence of solid-state deformation features and low anisotropy degrees in La Esperanza pluton-volcanic complex, bracketed between 270 Ma and 250 Ma, indicate that its fabric is of magmatic origin, evidencing the attenuation of the compressive regime toward the end of the Paleozoic (Martinez Dopico et al., 2017). Within the Ordovician Punta Sierra granite, located in the same area, a syntectonic reverse remagnetization acquired during the early Permian has been isolated (Rapalini and Vilas, 1991; Rapalini, 1998; Tomezzoli et al., 2013b). The congruence of the Punta Sierra PP (Tomezzoli et al., 2013b) with other coeval PPs in the Gondwana path provides substantial evidence that Patagonia was indeed an integral component of Gondwana during the early Permian.
The deformation along the southwest Gondwana margin that started in the Early-to-Middle Devonian in Argentina is called the Cháñica orogenic phase (ChOP; Turner and Mendez, 1975) . This orogenic event was related to the collision of Chilenia with Gondwana (Ramos et al., 1984) and is thought to have occurred as a result of an eastward-dipping subduction zone by some authors (Haller and Ramos, 1984; Ramos et al., 1984) or by a westward-dipping subduction zone by others (Astini et al., 1995; Davis et al., 1999; Gerbi et al., 2002). For some researchers, this deformation was also justified by the collision of Precordillera (von Gosen and Prozzi, 1998; Keller et al., 1998; Rapela et al., 1998; Pankhurst and Rapela, 1998; Keller, 1999). According to Rapela et al. (1998), Chilenia was part of Cuyania and it was not an independent terrain. The position and polarity of the subduction zone related to the Chilenia accretion process remain controversial, as no evidence of a Late Ordovician to Early Devonian magmatic arc related to this subduction has been found (Alvarez et al., 2011, and references cited therein). In the late Paleozoic, specifically in the early Permian, a phase of deformation known in Argentina as the San Rafael orogenic phase (SROP; Azcuy and Caminos, 1987; Hercinian Phase in the rest of the world) began to play a leading role along this part of Gondwana (Tomezzoli and Vilas, 1999; Tomezzoli, 2001). This Permian deformation is also currently controversial and different geodynamic scenarios have been delineated to explain it (Martínez, 1980; Dalmayrac et al., 1980; Lock, 1980; Ramos, 1984; Cobbold et al., 1992; Dalziel et al., 2000; von Gosen, 2003; Japas and Kleiman, 2004; Chernicoff and Zappettini, 2004; Pankhurst et al., 2006; Rapalini, 2005; Kleiman and Japas, 2009; Gregori et al., 2015; among others).
In this study, the SROP is proposed to be the result of the final rearrangement of microplates that had previously collided within Gondwana and Laurentia and continued moving during the Permian toward the Equator from the South and the North, respectively, to form the Pangea supercontinent during the Triassic.
To explain the evolution of the southwestern margin of Gondwana, paleomagnetic, AMS studies, field observations, and data obtained by other authors were integrated by Tomezzoli (1997), up until today, indicating that there was deformation during the Permian (Figures 5–7). As the Tunas Formation is of Permian age, the paleomagnetic results obtained there (Tomezzoli and Vilas, 1999; Tomezzoli, 2001) do not account for the pre-Permian geological history of the region. Therefore, it is necessary to resort to other arguments to understand the geological evolution and onset of deformation in the region. However, the Devonian deformation is the most discussed in the area. This is probably because, although in the western margin (Chilenia) the suture zones are covered by younger sediments and volcanic rocks (Álvarez et al., 2011), the Andean deformation exposed some key outcrops that aid in their comprehension. On the other hand, the southern margin, less influenced by recent tectonic events, does not show such clear evidence of the Devonian deformation. Although, in the San Rafael Block, west of the Gondwana margin, the geological manifestations regarding the existence of the ChOP and SROP are clear and are not the subject of discussion, the existence of the ChOP along the South margin of Gondwana is still under debate. One of the key locations for understanding the model proposed here is the contact between the Lolén and Sauce Grande formations, in the Sierras Australes area (Figures 2, 3), for which there are different interpretations (see Tomezzoli, 2012). Nonetheless, what remains unaddressed is the substantial hiatus between these formations, spanning a significant portion of the Carboniferous period (Figure 2), and the structural, topographic, and lithological differences between the early Paleozoic Curamalal and Ventana groups and the late Paleozoic Pillahuincó group (Harrington, 1947; Suero, 1972). We propose here that these differences are the consequence of a first-order paleogeographic event that could be the collision of a terrain here called CHIPA (Chilenia plus Patagonia).
The Cerro Colorado granite and Cerro de los Viejos gneiss (Figure 7) are also key locations for understanding the evolution of this sector of Gondwana as there is a record of at least two deformation events: one high temperature event that was ductile and penetrative, and another low temperature event that was brittle and spaced. In the same region, during the late Paleozoic, post-orogenic magmatism was recorded in López Lecube (Figure 7; Tomezzoli and Vilas, 1997). Geological data suggest that within the same region, magmatic manifestations exhibit distinct lithological and structural characteristics. These differences indicate that the older intrusive bodies have undergone deformation, whereas younger ones appear either undeformed or exhibit less prominent deformation. Consequently, we can infer that the regional deformation was attenuated or concluded during the geological period of the López Lecube intrusion, aligning with the observations made in the Tunas Formation.
In the Carapacha basin locality (Figures 1, 10; as well as in the Sierras Australes area, remembering the unconformity between the Lolén and Sauce Grande formations), there is also a hiatus that exceeds 50 Ma between the Devonian and the Carboniferous periods. Notable lithological and structural distinctions can be observed between the rocks of La Horqueta Formation (Devonian), which exhibit a higher degree of deformation and superimposed styles (Figure 10), and the overlying rocks of the Carapacha Formation (Figure 8; Permian). In the same area, the SROP was recorded in the magmatism of Sierra Chica between the base and the top of the sequence (Tomezzoli et al., 2009; Tomezzoli et al., 2013a; Figure 9).
Available paleomagnetic results for Patagonia (Rapalini and Vilas, 1991; Rapalini et al., 1994; Rapalini, 1998; Tomezzoli et al., 2013b; Martinez Dopico et al., 2020), which were interpreted as Permian remagnetizations, are consistent with the expected paleolatitudes for Gondwana on the APWP of South America (Tomezzoli, 2009; Gallo et al., 2017). This indicates that during the Permian there were no significant and independent latitudinal displacements between Gondwana and Patagonia, inferring that in the Permian, both blocks were already amalgamated. Syntectonic magnetizations and AMS studies, as well as the continentalization evidence (Andreis and Cladera, 1992; Zavala et al., 1993; López Gamundi et al., 1995), throughout the southwest margin of Gondwana (Figure 1), are consistent with a model of a fold and thrust belt advancing over a northern foreland basin since the Middle Devonian, with a gradual attenuation of deformation in space and time: toward the east-northeast and toward the late Permian. The intensity of the Permian deformation was not uniform (Figure 6); on the contrary, there were two peaks of deformation: the first one occurred between the Sauce Grande and Bonete formations (approximately between 300 and 290 Ma) and the second took place within the Tunas Formation (approximately between 290 and 276 Ma; Arzadún et al., 2016; Arzadún et al., 2018; Arzadún et al., 2021a), followed by a gradual decrease toward the end of the Permian (Tomezzoli, 1999; Tomezzoli and Vilas, 1999; Tomezzoli, 2001; Arzadún et al., 2016; Arzadún et al., 2021b; Tomezzoli et al., 2022).
It was determined that there was a distinct distribution pattern of paleopoles during the Permian (Tomezzoli, 2009; Gallo et al., 2017), one in the early Permian, where magnetizations and/or remagnetizations were clearly syntectonic: Tunas I, Curacó, Cochicó, Sierra Chica, Hoyada Verde, Alcaparrosa, Ponón Trehue (Font et al., 2012, and references cited therein), and Centinela I (Tomezzoli et al., 2018). The other one was in the early late Permian, where magnetizations and/or remagnetizations were less affected by the deformation: Tunas II, San Roberto, Independencia (Font et al., 2012, and references cited therein), Centinela II (Tomezzoli et al., 2018). The presence of different paleopolar positions for Gondwana [early Permian, late Permian, and Triassic (Gallo et al., 2017)] highlights that in this short period of time (millions of years in geological terms) there was a strong latitudinal movement of at least 28° (Figure 13) that would correspond to a displacement of approximately 3,200 km in 40 million years with a rate of approximately 8 cm/year (Tomezzoli, 2009). It should be noted that this movement only considers the latitudinal component recorded paleomagnetically, and the velocities may be even higher.
FIGURE 13. Different stages of the paleogeographic reconstruction of Gondwana from the Late Devonian-Early Carboniferous to the late Permian-Early Triassic (modified from Gallo et al., 2017). Gondwana first moved to the north and then there was a bounce to the south. This rebound could be reflected in the change of the shortening direction from SW-NE to SE-NW toward the end of the Permian.
This continental margin is complex, with deformation that persists for many millions of years, with stress directions that can come from the west, south, or southwest, along a curve margin, resulting in deformation partitioning and variations in stress magnitudes (Tomezzoli et al., 2022). This is why, as previously discussed, various regions exhibit distinct deformational patterns that can be either coaxial or not, depending on the geological timeframe and location along the margin. Certain areas exhibit dextral transpressive regimes, some display sinistral patterns, and others manifest transtensive characteristics (Tapia et al., 2023). Numerous studies based on different disciplines have given rise to different models to explain the evolution of the western and southern margins of Gondwana. Among many of the models, there are common points with the present study. For example, Martínez et al. (2012), with detailed studies, demonstrated that it is possible to extend the southern margin of Chilenia to a 42° south latitude, concluding that Chilenia subducted during the Middle Devonian beneath the North Patagonian Massif. The position of high-pressure metamorphism studied by Martínez et al. (2012) is consistent with the geological evolution model proposed here.
Another interesting observation that can be drawn is that the examined sites within the northeastern sector of the deformation of the southwestern Gondwana margin (Lolén, Sauce Grande to Tunas, Cerro Colorado, and Cerro de los Viejos) display a magnetic fabric with their Kmin axis inclined toward the northeast (Quadrant I; Figure 14A). On the contrary, the sites situated to the southwest of the deformation (Carapacha, Puelches, Sierra Chica, Yaminué, and Agua Escondida) exhibit a Kmin axis inclined toward the southwest (Quadrant III; Figure 14A). Assuming that the Kmin axis of AMS is subparallel to the maximum principal stress (Sigma 1), it could be interpreted that this arrangement of the AMS axes may be related to the stress distribution in a fold and thrust belt formed during the late Paleozoic. In a theorical scheme of a collisional zone, two folded and thrust belts are developed: the synthetic and antithetic belts (McClay et al., 2004; Figure 14B). The arrangement of the maximum principal stress (Sigma 1) will be symmetrical with respect to the suture zone, inclining in each belt in the direction of tectonic vergence (Figure 14B). Therefore, assuming a NW-SE-oriented suture zone for the Chilenia-Patagonia (referred here as CHIPA) collision with Gondwana (Figure 14B), the rocks within the antithetic (retrowedge) fold and thrust belt, characterized by NE vergence, should display an AMS influenced by a maximum principal stress (Sigma 1) inclined toward the NE (Figure 14). Conversely, rocks situated on the synthetic (prowedge) folded and thrust belt should develop an AMS conditioned by a Sigma 1 inclined toward the SW (Figure 14).
FIGURE 14. (A) The analyzed localities in the northeastern sector (Lolén, Sauce Grande to Tunas, Cerro Colorado, and Cerro de los Viejos) exhibit a magnetic fabric, with their Kmin axis dipping to the NE (I quadrant). Conversely, the localities located toward the southwest (Carapacha, Puelches, Sierra Chica, Yaminué, and Agua Escondida) show their Kmin axis tilting to the SW (III quadrant). Assuming that the Kmin axis of AMS is subparallel to the maximum principal stress (Sigma1), it could be interpreted that this arrangement of the AMS axes may be related to the stress distribution in a fold and thrust belt formed during the late Paleozoic (this study). (B) In a theorical scheme of a collisional zone (McClay et al., 2004), two folded and thrust belts develop: the synthetic and antithetic; the arrangement of the maximum principal stress will be symmetrical with respect to the suture zone, inclining in each belt in the direction of the tectonic vergence. Assuming a NW-SE trending suture zone for the CHIPACHIPA to Gondwana collision, the rocks located in the antithetic (retrowedge) folded and thrust belt with NE vergence should exhibit an AMS conditioned by a maximum principal stress inclined toward the NE. Conversely, rocks situated on the synthetic (prowedge) folded and thrust belt should develop an AMS conditioned by a Sigma 1 inclined toward the SW.
All the late Paleozoic deformation in this region is a product of the collisions that occurred during the Middle Devonian (CHIPA against Gondwana) and Middle Carboniferous (Deseado Massif against Gondwana plus CHIPA, which collided before). As a result of the final adjustment of the microplates engaged in the late Paleozoic collisional events, intracontinental deformation processes persisted until the Permian period (Figure 15). However, the Permian deformation cannot be attributed solely to a local process (Figure 13). It is known that one of the most important life extinctions in Earth’s history occurred at the Permian-Triassic boundary. This phenomenon must have necessarily responded to a global process, related to the abrupt paleogeographic changes that occurred during those times: the movements of Gondwana and Laurentia to the Equator (Figure 13). The Permian deformation all around the planet, including Argentinian territory, was nothing more and nothing less than the birth of Pangea (Wegener, 1924). The presence of different paleopole positions, evidenced in the abrupt cusp of the APWP of Gondwana during the Permian-Triassic, shows that there were significant latitudinal movements during a short period of time that must necessarily involve significant deformation (Figure 13). This deformation was attenuating in space and time toward the end of the Permian with a change in the direction of maximum compression from SW-NE to SE-NW that could be a final rebound of the Pangea supercontinent.
FIGURE 15. (A) Terrains accreted in the Argentinian territory during the Paleozoic; regional integration of paleomagnetic data, AMS data, field observations, our own data, and data obtained by other authors provide evidence to propose that the main compressive deformation along the south-southwestern margin of Gondwana, from the Sierras Australes to the San Rafael block and North Patagonian massif, have a SW-NE direction and started at least in the Middle Devonian. This deformation is related to the collision of CHIPA against Gondwana (this study). (B) The proposal of evolution along the southwest Gondwana margin (this study) involves the sum of the stresses generated as a product of successive collisions that occurred from the Cambrian to the Carboniferous. During the Middle Devonian, CHIPA collided with Gondwana (ChOP); subsequently, in the Middle Carboniferous, the Deseado Massif collided with Gondwana (which had already incorporated CHIPA, which had collided previously). Post-collisional effects persisted until the Permian (SROP), resulting in an intracontinental stress-deformation product of the adjustment and a coupling of the microplates involved. However, it is necessary to remember that the Permian deformation occurred all around the planet, including in Argentinian territory, and is nothing more and nothing less than the birth of Pangea (Wegener, 1924). The presence of different paleopole positions (see Figure 13) evidenced in the abrupt cusp of the APWP of Gondwana during the Permian-Triassic (see figures above in this study) show that there were significant latitudinal movements during a short period of time that must necessarily involve deformation.
Geological evidence indicates that the deformation along the southwest Gondwana margin began during the Middle-to-Late Devonian (the Acadian-Cháñica orogenic phase in Argentina) and continued up until the Permian. Here, it is interpreted that the beginning of this deformation was related to the collision of Chilenia from the west and Patagonia from the south-southwest. As Chilenia and Patagonia collided against Gondwana at the same time, in this study we proposed that conform the same allochthonous drift terrain which We have named “CHIPA”. The regional data integration, from the Sierras Australes to the San Rafael block and North Patagonian massif, provide evidence of that the deformation started in the Devonian. Later, during the Middle Carboniferous, the Deseado Massif from the south was incorporated (Figure 15). Post-collisional deformation continued up until the Permian with the San Rafaelic orogenic phase, which would then have been the consequence of the last assembly and coupling stages of the different microplates accreted along the entire Gondwana margin, while they were subjected to latitudinal displacement toward the Equator (Figure 15). That translation phenomenon to the Equator was not exclusive from the south-southwest of Gondwana. The same processes occurred at the same time with the continents in the Northern Hemisphere when Laurentia was assembling with Laurasia while moving latitudinally toward the Equator. The sum of all these geological processes were part of the gestation of Pangea (Wegener, 1924). It is possible to visualize the Gondwana (Africa) supercontinent as a central cratonic accretionary nucleus onto which various smaller plates fused, subsequently interacting to ultimately achieve their complete amalgamation during the Triassic assembly. Future research will surely continue to yield fresh data and evidence that will aid us in determining whether Chilenia and Patagonia comprised the same drifting continent, CHIPA, and gaining a deeper understanding of the Earth’s evolution across its geological timeline.
The raw data supporting the conclusion of this article will be made available by the authors, without undue reservation.
RNT designed and conducted this work: analysis, investigation, measurement, conceptualization, writing–review and editing; EOC participate in the field trips and edition; HT collaborated in the geology revision, field trip and was responsible of the petrology and geochronology; GA participate in the field trips, measurements, interpretation and edition; JMC, GC, LBF, and ES collaborated in the field trips and measurements of the samples. All authors contributed to the article and approved the submitted version.
This work is dedicated with great affection to Maria Elena Woroszylo and Roberto Molina Garza, both colleagues and friends. Almost 30 years ago, Roberto rejected one of my first papers. Despite that, over the years, we forged a beautiful friendship. For many of us, Roberto will be a very present absence in our LatinMag meetings. Each study area presented here is the result of joint work with many colleagues whom we sincerely thank, including S. Japas, L. Kleiman, W. D. Mac Donald, A. E. Rapalini, H. Vizán, J. Chernicoff, E. Llambías, C. Cingolani, L. Gallo, P. Franceschinis, J. Salvarredi, R. Melchor, among others. Lics. Ochoa and Battler also contributed to this study. We thank Drs Laura Giambiagi and Baochun Huang and another reviewer who helped improve the original manuscript. This research was funded in recent years by the following grants: PIP-CONICET-2828, UBACYT X220, UBACyT—894, and ANPCyT- PICT-2272—3505.
The authors declare that the research was conducted in the absence of any commercial or financial relationships that could be construed as a potential conflict of interest.
All claims expressed in this article are solely those of the authors and do not necessarily represent those of their affiliated organizations, or those of the publisher, the editors and the reviewers. Any product that may be evaluated in this article, or claim that may be made by its manufacturer, is not guaranteed or endorsed by the publisher.
Alessandretti, L., Philipp, R. P., Chemale, F., Brückmann, M. P., Zvirtes, G., Mette, V., et al. (2013). Provenance, volcanic record, and tectonic setting of the Paleozoic Ventania Fold Belt and the Claromecó Foreland Basin: implications on sedimentation and volcanism along the southwestern gondwana margin. J. S. Am. Earth Sci. 71, 12–31. doi:10.1016/j.jsames.2013.05.006
Alvarez, J., Mpodozis, C., Arriagada, C., Astini, R., Morata, D., Salazar, E., et al. (2011). Detrital zircons from late Paleozoic accretionary complexes in north-central Chile (28º-32ºS): possible fingerprints of the chilenia terrane. J. S. Am. Earth Sci. 32, 460–476. doi:10.1016/j.jsames.2011.06.002
Andreis, R. R., and Cladera, G. (1992). “Las epiclastitas pérmicas de la Cuenca Sauce Grande (sierras australes, Buenos Aires, Argentina),” in Parte 2: emplazamiento geotectónico de las áreas de aporte (La Plata, Actas: Cuarta Reunión de Sedimentología), 135–142.
Andreis, R. R., Lluch, J. J., and Iñíguez Rodríguez, A. M. (1979).Paleocorrientes y Paleoambientes de las Formaciones Bonete y Tunas, Sierras Australes de la provincia de Buenos Aires, Argentina, Bahía Blanca, Actas: Congreso Geológico Argentino, 207–224.
Arzadún, G., Lovecchio, J. P., Becchio, R., Uriz, N. J., Cingolani, C., Febbo, M. B., et al. (2021b). Thermochronology of the Ventana Ranges and Claromecó Basin, Argentina: record of gondwana breakup and south atlantic passive margin dynamics. J. S. Am. Earth Sci. 105, 102965. doi:10.1016/j.jsames.2020.102965
Arzadún, G., Tomezzoli, R. N., Trindade, R., Gallo, L. C., Cesaretti, N. N., and Calvagno, J. M. (2018). Shrimp zircon geochronology constrains on permian pyroclastic levels, Claromecó Basin, south west margin of gondwana, Argentina. J. S. Am. Earth Sci. 85, 191–208. ISSN 0895-9811. doi:10.1016/j.jsames.2018.05.001
Arzadún, G., Tomezzoli, R. N., and Cesaretti, N. (2013). “Análisis de la anisotropía de susceptibilidad magnética (ASM) y compactación en la Formación Tunas, Sierras Australes de la Provincia de Buenos Aires, Argentina,” in Proceedings of third biennial meeting of latinmag letters, 3. Special Issue Montevideo Available at: www.geofisica.unam.mx/LatinmagLetters/LL13-03-SP/LLv3.
Arzadún, G., Tomezzoli, R. N., and Cesaretti, N. N. (2016). Tectonic insight based on anisotropy of magnetic susceptibility and compaction studies in the Sierras Australes thrust and fold belt (Southwest Gondwana boundary, Argentina). Tectonics 35, 1015–1031. doi:10.1002/2015TC003976
Arzadún, G., Tomezzoli, R. N., Fortunatti, N., Cesaretti, N. N., Febbo, B., and Calvagno, J. M. (2021a). Deformation understanding in the upper paleozoic of Ventana ranges at southwest Gondwana boundary. Nature-Scientific Rep. 11, 20804. doi:10.1038/s41598-021-99087-1
Astini, R. A., Benedetto, J. L., and Vaccari, N. E. (1995). The early Paleozoic evolution of the Argentine Precordillera as a Laurentian rifted, drifted, and collided terrane: a geodynamic model. Geol. Soc. Am. Bull. 107, 253–0273. doi:10.1130/0016-7606(1995)107<0253:tepeot>2.3.co;2
Azcuy, C. L., and Caminos, R. (1987). “Diastrofismo,” in El Sistema Carbonífero en la República Argentina. Editor S. En Archangelsky (Córdoba: Academia Nacional de Ciencias), 239–251.
Battler, J. M. (2015). Paleomagnetismo y fábrica magnética del Grupo Choiyoi en área de Agua Escondida de San Rafael, Mendoza. UNLPam: Trabajo Final de Licenciatura, 111. unpublished.
Buggisch, W. (1987). Stratigraphy and very low grade metamorphism of the Sierras Australes de la provincia de Buenos Aires (Argentina) and implications in Gondwana correlation. Zentralbatt für Geol. Paläontologie 5, 819–837.
Butler, R. F. (1992). Paleomagnetism: magnetic domains to geologic terranes. Boston: Blackwell Scientific Publications, 319.
Calderón, M., Hervé, F., Munizaga, F., Pankhurst, R. J., Fanning, C. M., and Rapela, C. W. (2020). “Geochronological record of plutonic activity on a long-lived active continental margin, with 930 emphases on the pre-Andean rocks of Chile,” in Geocronologia e Evolução Tectônica do Continente Sul-Americano: a contribuição de Umberto Giuseppe Cordani, Solaris Edições Culturais. Editors A. Bartorelli, W. Teixeira, and B. B. Brito Neves, 392–407. São Paulo.
Chernicoff, C. J., and Zappettini, E. O. (2004). Geophysical evidence for terrane boundaries in south-central Argentina. Gondwana Res. 7, 1105–1116. doi:10.1016/s1342-937x(05)71087-x
Chernicoff, C. J., Zappettini, E. O., Santos, J. O. S., Beyer, E., and McNaughton, N. J. (2022). Combined isotope study of the Ordovician Cerro de los Viejos Granite, La Pampa province, Argentina. Its derivation from the autochthonous, early Mesoproterozoic southwesternmost border of the Río de la Plata craton. J. S. Am. Earth Sci. 120, 104088. doi:10.1016/j.jsames.2022.104088
Chernicoff, C. J., Zappettini, E. O., Santos, J. O. S., Beyer, E., and McNaughton, N. J. (2008). Foreland basin deposits associated with Cuyania accretion in La Pampa Province, Argentina. Gondwana Res. 13, 189–203. doi:10.1016/j.gr.2007.04.006
Choque, G., Fortunatti, N. B., Febbo, B., and Tomezzoli, R. N. (2022). Fracture frequency and anisotropy of magnetic susceptibility: a case of study in the Claromecó ´Basin (PANG 0003 well), southwestern Gondwana boundary. J. S. Am. Earth Sci. 120, 104094. doi:10.1016/j.jsames.2022.104094
Cingolani, C. A., Berry, C. M., Morel, E., and Tomezzoli, R. N. (2002). Middle devonian Lycopsids from high southern palaeolatitudes of gondwana (Argentina). Geol. Mag. 139, 641–649. doi:10.1017/s0016756802006957
Cingolani, C. A., and Varela, R. (1973). Examen geocronológico por el método Rubidio – estroncio de las rocas ígneas de las Sierras Australes Bonaerenses, 1. Villa Carlos Paz, Actas: 5º Congreso Geológico Argentino, 349–371.
Cobbold, P. R., Gapais, D., Rossello, E. A., Milani, E., and Szatmari, P. (1992) “Permo-Triassic intracontinental deformation in SW Gondwana,” in Inversion tectonics of the cape fold belt, karoo and cretaceous basins of southern africa, balkema 23-26 Editors J. y. En Wit, and N. Ransome Rotterdam.
Criado Roque, P. (1972). “Bloque de San rafael,” in Geología regional Argentina. Editor A. F. En Leanza (Córdoba: Academia Nacional de Ciencias), 283–295.
Dahlquist, J. A., Morales Cámera, M. M., Alasino, P. H., Tickyj, H., Basei, M. A. S., Galindo, C., et al. (2020). Geochronology and geochemistry of Devonian magmatism in the Frontal Cordillera (Argentina): geodynamic implications for the pre-Andean SW Gondwana margin. Int. Geol. Rev. 64, 233–253. doi:10.1080/00206814.2020.1845994
Dalmayrac, B., Laubacher, G., Marocco, R., Martinez, C., and Tomasi, P. (1980). La chaine hercynienne d’Amerique du Sud. Structure et evolution d’un orogene intracratonique. Sonderdr. A.d. Geol. Rundsch. 69, 1–21. doi:10.1007/bf01869020
Dalziel, I. W. D., Lawver, L. A., and Murphy, J. B. (2000). Plumes, orogenesis, and supercontinental fragmentation. Earth Planet Sci. Lett. 178, 1–11. doi:10.1016/s0012-821x(00)00061-3
Davis, J. S., Roeske, S. M., McClelland, W. C., and y Snee, L. W. (1999). “Closing the ocean between the Precordillera terrane and Chilenia: early devonian ophiolite emplacement and deformation in the sw precordillera,” in Laurentia-gondwana connections before Pangea. Editors V. A. y. En Ramos, and J. D. Keppie (Geological Society of America, Special Paper), 336, 115–138.
Di Pasquo, M., Martínez, M. A., and Freije, H. (2008). Primer registro palinológico de la Formación Sauce Grande (Pennsylvaniano-Cisuraliano) en las Sierras Australes, provincia de Buenos Aires, Argentina. Ameghiniana 45, 69–81.
Domeier, M., Van der Voo, R., Tohver, E., Tomezzoli, R. N., Vizan, H., Torsvik, T. H., et al. (2011). A new late permian constraint on the apparent polar wander path of gondwana. Geochem. Geophys. Geosystems 12, Q07002. doi:10.1029/2011GC003616
du Toit, A. L. (1927). “A geological comparison of South America with South Africa,” in With a paleontological contribution by F.R. Cowper Red (Washington, pps: Carnegie Institute), 158.
Febbo, B., Tomezzoli, R. N., Calvagno, J. M., Gallo, L. C., Cessaretti, N. N., and Cesaretti, N. N. (2021). Anisotropy of magnetic susceptibility analysis in Tunas Formation cores (Permian), Claromecó Basin, Buenos Aires, Argentina: its relation to depositional and post-depositional conditions. J. S. Am. Earth Sci. 107, 103144. doi:10.1016/j.jsames.2020.103144
Febbo, M. B. (2023). Análisis diagenético y de anisotropía de susceptibilidad magnética en registros de subsuelo pérmicos de la Formación Tunas: pozos PANG 0001 y PANG 0003, Cuenca de Claromecó, Buenos Aires, Argentina. Argentina: PhD Tesis, Universidad Nacional del Sur, 353.
Font, E., Rapalini, A. E., Tomezzoli, R. N., Trindade, R. I. F., and Tohver, E. (2012). “Episodic remagnetizations related to tectonic events and their consequences for the South America Polar Wander Path,” in Remagnetization and chemical alteration of sedimentary rocks. Editors R. D. En Elmore, A. R. Muxworthy, M. M. Aldana, and M. y Mena (London: Geological Society, Special Publication), 371. doi:10.1144/SP371.7
Gallo, L. C., Tomezzoli, R. N., and Cristallini, E. O. (2017). A pure dipole analysis of the Gondwana apparent polar wander path: paleogeographic implications in the evolution of Pangea. Geochem. Geophys. Geosystems 18, 1499–1519. doi:10.1002/2016GC006692
García-Sansegundo, J., Gallastegui, G., Farías, P., Rubio-Ordóñez, A., Cuesta, A., Heredia, N., et al. (2016). Evolución tectono-metamórfica Cháñica del Complejo Guarguaraz, Cordillera Frontal de los Andes (Mendoza, Argentina). Geo-Temas 16 (2), 1576–5172. ISSN.
Gerbi, C., Roeske, S. M., and y Davis, J. S. (2002). Geology and structural history of the southwest Precordillera margin, northern Mendoza Province, Argentina. J. S. Am. Earth Sci. 14, 821–835. doi:10.1016/s0895-9811(01)00080-3
Giambiagi, L., Mescua, J., Folguera, A., and Martínez, A. (2010). Estructuras y cinemática de las deformaciones pre-andinas del sector sur de la Precordillera, Mendoza. Rev. Asoc. Geol. Argent. 66, 5–20.
Gradstein, F. M., Ogg, J. G., and Smith, A. G. (2004). A geologic time scale 2004. Cambridge, UK: Cambridge Univ. Press, 610.
Grecco, L. E., and Gregori, D. A. (1993). “Estudios geoquímicos de los intrusivos graníticos Cerros Colorados y Aguas Blancas, Sierras Australes, provincia de Buenos Aires, Argentina,” in 12º congreso Geológico argentino y 2º congreso de Exploración de Hidrocarburos 4, 81–89. Mendoza.
Grecco, L. E., Gregori, D. A., and Maiza, P. J. (1984). Relación del contenido de flúor y de (OH) en las biotitas de las rocas graníticas de la cantera Cerros Colorados, provincia de Buenos Aires, Argentina. 9º Congr. Geol. Argent. Actas 3, 368–375. San Carlos de Bariloche.
Grecco, L. E., Gregori, D. A., and Ruvimos, M. A. (1997). Characteristics of neoproterozoic magmatism in Sierras Australes, southeast Argentina. Zentralblatt Geol. Paläontologie Teil 1, 609–619.
Gregori, D. A., Grecco, L. E., and Llambías, E. J. (2003). El intrusivo López Lecube: evidencias de magmatismo alcalino gondwánico en el sector sudoeste de la provincia de buenos aires, Argentina. Rev. Asoc. Geol. Argent. 58, 167–175.
Gregori, D. A., Saini-Eidukat, B., Benedini, L., Strazzere, L., Barros, M., Kostadinoff, J., et al. (2015). The Gondwana Orogeny in northern North Patagonian Massif: evidences from the caita có granite, la seña and pangaré mylonites, Argentina. Geosci. Front. 7, 621–638. doi:10.1016/j.gsf.2015.06.002
Haller, M. J., and Ramos, V. A. (1984). Las ofiolitas famatinianas (Eopaleozoico) de las provincias de San Juan y Mendoza. 9° Congr. Geol. Argent. Actas 2, 66–83. San Carlos de Bariloche.
Harrington, H. J. (1947). Explicación de las Hojas Geológicas 33m y 34m, Sierras de Curamalal y de la Ventana, provincia de Buenos Aires, 61. Buenos Aires, Boletín: Servicio Nacional de Minería y Geología, 43.
Hervé, F., Calderon, M., Fanning, C. M., Pankhurst, R. J., Fuentes, F., Rapela, C. W., et al. (2016). Devonian magmatism in the accretionary complex of southern Chile. J. Geol. Soc. 173 (4), 587–602. doi:10.1144/jgs2015-163
Hervé, F., Calderón, M., Fanning, C. M., Pankhurst, R. J., Rapela, C. W., and Quezada, P. (2018). The country rocks of devonian magmatism in the North patagonian massif and chaitenia. Andean Geol. 45, 301–317. doi:10.5027/andgeov45n3-3117
Hrouda, F., and Chadima, M. (2020). Examples of tectonic overprints of magnetic fabrics in rocks of the Bohemian Massif and Western Carpathians. Int. J. Earth Sci. 109, 1321–1336. doi:10.1007/s00531-019-01786-8
Japas, M. S. (1987). Caracterización geométrico-estructural del Grupo Pillahuincó. II. Formación Sauce Grande. Perfil del Cordón Mambacher y Sierra de las Tunas Occidental, Sierras Australes de Buenos Aires, 39. Buenos Aires: Academia Nacional de Ciencias Exactas, Físicas y Naturales, Anales, 125–144.
Japas, M. S., and Kleiman, L. E. (2004). El ciclo Choiyoi en el bloque de San Rafael (Mendoza): de la orogénesis tardía a la relajación mecánica. Asoc. Geol. Argent. Ser. D. Publicación Espec. 7, 89–100.
Japas, M. S. (1989). La deformación de la cadena plegada de las Sierras Australes de la provincia de Buenos Aires, 40. Buenos Aires, Anales: Academia Nacional de Ciencias Exactas Físicas y Naturales, 193–215.
Japas, M. S., Quenardelle, S. M., and Sellés Martínez, J. (2001). Inversión del sentido de cizallamiento no-coaxial debido a deformación progresiva. Formación Lolén, Sierras Australes de Buenos Aires. Asociación Geológica Argentina. Ser. D. Publicación Espec. 5, 97–102.
Keidel, J. (1916). La geología de las sierras de la provincia de Buenos Aires y sus relaciones con las montañas del Cabo y los Andes, 9. Mineralogía y Minería, Anales, Buenos Aires: Ministerio de Agricultura de la Nación, Dirección de Geología, 5–77.
Keller, M. (1999). Argentine Precordillera: sedimentary and plate tectonic history of a Laurentian crustal fragment in South America, 341. Boulder: Geological Society of America, Special Paper, 131.
Keller, M., Buggish, W., and Lehnert, O. (1998). The stratigraphic record of the Argentine Precordillera and its plate tectonic background, 142. London: Geological Society, Special Publication, 35–56.
Kilmurray, J. O. (1975). Las Sierras Australes de la provincia de Buenos Aires, las facies de deformación y nueva interpretación estratigráfica. Rev. Asoc. Geol. Argent. 30, 331–348.
Kleiman, L. E., and Japas, M. S. (2009). The Choiyoi volcanic province at 34°S-36° S (San Rafael, Mendoza, Argentina): implications for the late Palaeozoic evolution of the southwestern margin of Gondwana. Tectonophysics 473, 283–299. doi:10.1016/j.tecto.2009.02.046
Linares, E., Llambías, E. J., and La Torre, C. A. (1980). Geología de la provincia de La Pampa, República Argentina y geocronología de sus rocas metamórficas y eruptivas. Rev. Asoc. Geol. Argent. 35, 87–146.
Llambías, E. J., Quenardelle, S., and Montenegro, T. (2003). The Choiyoi Group from central Argentina: a subalkaline transitional to alkaline association in the craton adjacent to the active margin of the Gondwana continent. J. South Am. Earth Sci. 16, 243–257. doi:10.1016/S0895-9811(03)00070-1
Lock, B. E. (1980). Flat-plate subduction and the cape fold belt of South Africa. Geology 8, 35–39. doi:10.1130/0091-7613(1980)8<35:fsatcf>2.0.co;2
López de Luchi, M. G., Rapalini, A. E., and Tomezzoli, R. N. (2010). Magnetic fabric and microstructures of late paleozoic granitoids from the North patagonian massif: evidence of a collision between patagonia and gondwana? Tectonophysics 494, 118–137. doi:10.1016/j.tecto.2010.09.003
López Gamundi, O. R., Conaghan, P. J., Rossello, E. A., and Cobbold, P. R. (1995). The Tunas Formation (Permian) in the Sierras Australes foldbelt, east central Argentina: evidence for syntectonic sedimentation in a foreland basin. J. S. Am. Earth Sci. 8, 129–142. doi:10.1016/0895-9811(95)00001-v
López Gamundi, O. R., Fildani, A., Weislogel, A., and Rossello, E. (2013) The age of the Tunas Formation in the Sauce Grande basin-ventana foldbelt (Argentina): implications for the permian evolution of the southwestern margin of gondwana. J. South Am. Earth Sci. 45, 250–258. doi:10.1016/j.jsames.2013.03.011
MacDonald, W. D., and Ellwood, B. B. (1987). Anisotropy of magnetic susceptibility: sedimentological, igneous, and structural-tectonic applications. Rev. Geophys. 25, 905–909. doi:10.1029/rg025i005p00905
Martínez Dopico, C., Antonio, J., Rapalini, A., López de Luchi, M., and Grillo Vidal, C. (2020). Reconciling patagonia with gondwana in early paleozoic? Paleomagnetism of the valcheta granites, NE North Patagonian massif. J. S. Am. Earth Sci. 106, 102970. doi:10.1016/j.jsames.2020.102970
Martínez Dopico, C., López de Luchi, M. G., Rapalini, A. E., Wemmer, K., Mark Fanning, C., and Basei, A. S. (2017). Emplacement and temporal constraints of the Gondwanan intrusive complexes of northern Patagonia: la esperanza plutono-volcanic case. Tectonophysics 712-713, 249–269. doi:10.1016/j.tecto.2017.05.015
Martínez, J. C., Dristas, J. A., and Massonne, H. J. (2012). Palaeozoic accretion of the microcontinent Chilenia, North Patagonian Andes: high-pressure metamorphism and subsequent thermal relaxation. Int. Geol. Rev. 54, 472–490. doi:10.1080/00206814.2011.569411
Massabie, A., Rossello, E. A., Linares, E., Párica, C., and Powell, C. A. (1999). Granito Los Chilenos: una nueva unidad granítica jurásica en Cerro Colorado, Sierras Australes de Buenos Aires. Implicancias tectónicas. Rev. Asoc. Geol. Argent. 54, 281–289.
Massabie, A., and Rossello, E. (1984). La discordancia pre-Formación Sauce Grande y su entorno estratigráfico Sierras Australes de Buenos Aires, Argentina. 9º Congr. Geol. Argent. Actas 1, 337–352. San Carlos de Bariloche.
McClay, K. R., Whitehouse, P. S., Dooley, T., and Richards, M. (2004). 3D evolution of fold and thrust belts formed by oblique convergence. Mar. Petroleum Geol. 21 (7), 857–877. doi:10.1016/j.marpetgeo.2004.03.009
Melchor, R. N., and Césari, S. N. (1997). Permian floras fromCarapacha Basin, central Argentina. Description and importance. Geobios 30, 607–633. doi:10.1016/s0016-6995(97)80152-0
Melchor, R. N., and Llambías, E. J. (2000). Descripción de la hoja geológica 3766-I “Santa Isabel” (1:250.000), provincia de La Pampa. Buenos Aires: Instituto de Geología y Recursos Minerales, Servicio Geológico Minero Argentino, 43.
Melchor, R. N. (1999). Redefinición estratigráfica de la Formación Carapacha (pérmico), provincia de La Pampa, Argentina. Rev. Asoc. Geol. Argent. 54, 99–108.
Ochoa, M. J. (2017). Anisotropía de susceptibilidad magnética en la Formación La Horqueta, localidad de Puelches, provincia de La Pampa, Trabajo Final de Licenciatura. UNLPam: Universidad Nacional de la pampa, 93.
Oriolo, S., González, P. D., Alegre, P., Wemmer, K., Varela, R., and Basei, S. (2023). The cuesta de Rahue basement inlier (southern neuquén Precordillera, Argentina): a devonian to triassic polyphase orogenic record in northern Patagonia. doi:10.1144/jgs2022-143
Pankhurst, R. J., and Rapela, C. W. (1998). “The proto-Andean margin of Gondwana: an introduction,” in The proto-andean margin of Gondwana. Editors R. J. En Pankhurst, and C. W. y Rapela (London: Geological Society, Special Publication), 142, 1–9.
Pankhurst, R. J., Rapela, C. W., Fanning, C. M., and Márquez, M. (2006). Gondwanide continental collision and the origin of Patagonia. Earth-Science Rev. 76, 235–257. doi:10.1016/j.earscirev.2006.02.001
Parés, J. M., and van der Pluijm, B. A. (2003). Magnetic fabrics and strain in pencil structures of the knobs formation, valley and ridge province, US appalachians. J. Struct. Geol. 25, 1349–1358. doi:10.1016/s0191-8141(02)00197-9
Quenardelle, S. M., and Llambías, E. J. (1997). Las riolitas de Sierra Chica (37◦S, 65◦O): un centro eruptivo gondwánico en el bloque del Chadileuvú, provincia de La Pampa, Argentina. Rev. Asoc. Geol. Argent. 52, 549–558.
Ramos, V. A. (2008). Patagonia: a Paleozoic continent adrift? J. S. Am. Earth Sci. 26, 235–251. doi:10.1016/j.jsames.2008.06.002
Ramos, V. A., Cingolani, C., Chemale, F., Naipauer, M., and Rapalini, A. (2017). The Malvinas (Falkland) islands revisited: the tectonic evolution of southern Gondwana based on U-Pb and Lu-Hf detrital zircon isotopes in the Paleozoic cover. J. South Am. Earth Sci. 76, 320–345. doi:10.1016/j.jsames.2016.12.013
Ramos, V. A. (1984). Patagonia: ¿Un continente paleozoico a la deriva? 9º Congreso Geológico Argentino. Actas 2, 311–325. San Carlos de Bariloche.
Ramos, V., Jordan, T., Allmendinger, R., Kay, S., Cortés, J., and Palma, M. (1984). Chilenia: un terreno alóctono en la evolución paleozoica de los Andes Centrales. 9° Congr. Geol. Argent. Actas 1, 84–106. San Carlos de Bariloche.
Rapalini, A. E. (1998). Syntectonic magnetization of the mid-Palaeozoic Sierra Grande Formation: further constraints on the tectonic evolution of Patagonia. J. Geol. Soc. 155, 105–114. London. doi:10.1144/gsjgs.155.1.0105
Rapalini, A. E., Tarling, D. H., Turner, P., Flint, S., and Vilas, J. F. (1994). Paleomagnetism of the carboniferous tepuel group, central patagonia, Argentina. Tectonics 13, 1277–1294. doi:10.1029/94tc00799
Rapalini, A. E. (2005). “The accretionary history of southern South America from the latest Proterozoic to the Late Palaeozoic: some palaeomagnetic constraints,” in Terrane processes at the margins of Gondwana. Editors A. E. M. En Vaughan, P. T. Leat, and R. J. y Pankhurst (London: Geological Society, Special Publications), 246, 305–328.
Rapalini, A. E., and Vilas, J. F. (1991). Preliminary paleomagnetic data from the Sierra Grande Formation: tectonic consequences of the first mid-paleozoic paleopoles from patagonia. J. S. Am. Earth Sci. 4, 25–41. doi:10.1016/0895-9811(91)90016-e
Rapela, C. W., Pankhurst, R. J., Llambías, E. J., Labudía, C., and Artabe, A. (1996). “Gondwana magmatism of Patagonia: inner Cordilleran calc-alkaline batholiths and bimodal volcanic provinces,” in 3º international symposium on andean geodynamics (Saint-Malo), 791–794.
Rapela, C. W., Hervé, F., Pankhurst, R. J., Calderón, M., Fanning, C. M., Quezada, P., et al. (2021). The Devonian accretionary orogen of the North Patagonian cordillera. Gondwana Res. 96, 1–21. doi:10.1016/j.gr.2021.04.004
Rapela, C. W., Pankhurst, R. J., Casquet, C., Baldo, E., Saavedra, J., and Galindo, C. (1998). Early evolution of the proto-Andean margin of South America. Geology 26, 707–710. doi:10.1130/0091-7613(1998)026<0707:eeotpa>2.3.co;2
Rapela, C. W., Pankhurst, R. J., Fanning, C. M., and y Grecco, L. E. (2003). Basement evolution of the Sierra de la Ventana Fold Belt: new evidence for Cambrian continental rifting along the southern margin of Gondwana. J. Geol. Soc. 160, 613–628. London. doi:10.1144/0016-764902-112
Rocha-Campos, A. C., Basei, M. A., Nutran, A. P., Kleiman, L. E., Varela, R., Llambías, E., et al. (2011). 30 million years of Permian volcanism recorded in the Choiyoi igneous province (W Argentina) and their source for younger ash fall deposits in the Paraná Basin: SHRIMP U–Pb zircon geochronology evidence. Gondwana Res. 19, 509–523. doi:10.1016/j.gr.2010.07.003
Saint-Bezar, B., Herbert, R. L., Aubourg, C., Robion, P., Swennen, R., and Frizon de Lamotte, D. (2002). Magnetic fabric and petrographic investigation of hematite bearing sandstones within Ramprelated folds: examples from the south atlas front (Morocco). J. Struct. Geol. 24 (9), 1507–1520. doi:10.1016/s0191-8141(01)00140-7
Sellés Martinez, J., and Quenardelle, S. M. (1992). “Evidencias microestructurales de deformación progresiva en la Formación Lolén (Devónico de Sierra de la Ventana. Provincia de Buenos Aires,” in VI Reunión sobre microtectónica (Buenos Aires: Monografías de la Academia Nacional de Ciencias Exactas, Físicas y Naturales), 127–131.
Serra-Varela, S., Heredia, N., Otamendi, J., and Giacosa, R. (2021). Petrology and geochronology of the San Martín de los Andes batholith: insights into the devonian magmatism of the north patagonian andes. J. S. Am. Earth Sci. 109, 103283. doi:10.1016/j.jsames.2021.103283
Suero, T. (1972). Compilación geológica de las Sierras Australes de la provincia de Buenos Aires. Minist. Obras Públicas, LEMIT, Div. Geol. An. 3, 135–147. La Plata.
Tapia, F., Ramos, M., and Insaurralde, F. (2023). Late Mississippian-Pennsylvanian extensional deformation along the SW Gondwana margin: insight from the san rafael basin, western Argentina. J. S. Am. Earth Sci. 127, 104400. doi:10.1016/j.jsames.2023.104400
Tarling, D. H., and Hrouda, F. (1993). The magnetic anisotropy of rocks. London: Chapman & Hall, 217.
Tickyj, H., Tomezzoli, R. N., and Basei, M. A. (2014). Primeras edades U-Pb en intrusivos pérmicos del Distrito Minero Agua Escondida, Mendoza. Córdoba: XIX Congreso Geológico Argentino.
Tickyj, H., Dimieri, L. V., Llambías, E. J., and Sato, A. M. (1997). Cerro de los Viejos (38° 28’ S - 64° 26’O): cizallamiento dúctil en el sudeste de La Pampa. Rev. Asoc. Geol. Argent. 52, 311–321.
Tickyj, H., Tomezzoli, R. N., Basei, M. A., Fernández, M. A., Blatter, J. M., Rodriguez, N., et al. (2015). Geología de la Formación Piedras de Afilar, basamento granítico del Distrito Minero Agua Escondida, Mendoza. General Roca, Argentina: Actas del 3º PIMA.
Tohver, E., Cawood, P. A., Rossello, E. A., and Jourdan, F. (2012). Closure of the Clymene Ocean and formation of West Gondwana in the Cambrian: evidence from the sierras australes of the southernmost rio de la plata craton, Argentina. Gondwana Res. 21, 394–405. doi:10.1016/j.gr.2011.04.001
Tohver, E., Cawood, P. A., Rossello, E., López de Luchi, M. G., Rapalini, A., and Jourdan, F. (2008). New SHRIMP U-Pb and 40Ar/39Ar constraints on the crustal stabilization of southern South America, from the margin of the Rio de Plata (Sierra de Ventana) craton to northern Patagonia. San Francisco: American Geophysical Union, Fall Meeting. Abstract T23C-2052.
Tomezzoli, R. N., Kleiman, L. E., Salvarredi, J. A., Terrizzano, C., and Cristallini, E. O. (2002). “Paleogeographic evolution of the southwest Gondwana boundary during the late paleozoic. News studies in the pre-andean volcanism of the san rafael block, Mendoza province, Argentina,” in 5º International Symposium on Andean Geodynamics, Toulouse, 16-18 Septembre 2002, 645–648.
Tomezzoli, R. N., Arzadún, G., and Cristallini, E. O. (2017). Anisotropía de susceptibilidad magnética y paleomagnetismo en la Formación Lolén de edad Devónica. Sierras Australes de la provincia de Buenos Aires. Rev. Asoc. Geol. Argent. 74 (3), 326–337.
Tomezzoli, R. N., Arzadún, G., Fortunatti, N., Cesaretti, N. N., Febbo, M. B., Calvagno, J. M., et al. (2022). “Spasmodic deformation in the southwest of the gondwana boundary, upper paleozoic of Ventana ranges in Argentina,” in EGU General Assembly 2022, Vienna, Austria, 23–27 May 2022. EGU22-8926.
Tomezzoli, R. N. (2012). Chilenia y Patagonia: ¿un mismo continente a la deriva? Rev. Asoc. Geol. Argent. 69 (2), 222–239.
Tomezzoli, R. N., and Cristallini, E. O. (1998). Nuevas evidencias sobre la importancia del fallamiento en la estructura de las Sierras Australes de la provincia de Buenos Aires. Rev. Asoc. Geol. Argent. 53, 117–129.
Tomezzoli, R. N., and Cristallini, E. O. (2004). Secciones estructurales de las Sierras Australes de la provincia de Buenos Aires: ¿repetición de la secuencia estratigráfica a partir de fallas inversas? Rev. Asoc. Geol. Argent. 59, 330–340.
Tomezzoli, R. N. (1999). Edad de la sedimentación y deformación de la Formación Tunas en las Sierras Australes de la provincia de Buenos Aires (37°-39°S-61°-63°W). Rev. Asoc. Geol. Argent. 54, 220–228.
Tomezzoli, R. N. (2001). Further palaeomagnetic results from the Sierras Australes fold and thrust belt, Argentina. Geophys. J. Int. 147, 356–366. doi:10.1046/j.0956-540x.2001.01536.x
Tomezzoli, R. N. (1997). Geología y Paleomagnetismo en el ámbito de las Sierras Australes de la provincia de Buenos Aires. Buenos Aires: Tesis Doctoral, Universidad de Buenos Aires 327 pps.
Tomezzoli, R. N., Kleiman, L. E., Salvarredi, J. A., Terrizzano, C., and Cristallini, E. O. (2005a). Relaciones estratigráficas de volcanitas del Choiyoi Inferior sobre la base de estudios paleomagnéticos, Bloque de San Rafael, Mendoza, Argentina, 1. Actas, La Plata: 16º Congreso Geológico Argentino, 227–232.
Tomezzoli, R. N., Kleiman, L. E., Salvarredi, J. A., Terrizzano, C., and Cristallini, E. O. (2005b). “Paleogeographic evolution of the southwest Gondwana boundary during the late paleozoic. Paleomagnetism of the lower Choiyoi volcanics in the san rafael block, Mendoza, Argentina,” in 6º International Symposium on Andean Geodynamics, Barcelona, September 12–14, 2005, 730–733.
Tomezzoli, R. N., Mac Donald, W. D., and Tickyj, H. (2003). Composite magnetic fabrics and S–C structure in granitic gneiss of Cerro de los Viejos, La Pampa province, Argentina. J. Struct. Geol. 25, 159–169. doi:10.1016/s0191-8141(02)00030-5
Tomezzoli, R. N., Melchor, R., and MacDonald, W. D. (2006). Tectonic implications of post-folding permian magnetizations in the Carapacha Basin, La Pampa province, Argentina. Earth Planets Space 58, 1235–1246. doi:10.1186/bf03352619
Tomezzoli, R. N., Rapalini, A., López de Luchi, M., and Dopico, C. (2013b). Further evidence of widespread Permian remagnetization in the North Patagonian massif, Argentina. Gondwana Res. 24, 192–202. doi:10.1016/j.gr.2012.08.019
Tomezzoli, R. N., Saint Pierre, T., and Valenzuela, C. (2009). New Palaeomagnetic results from late Paleozoic volcanic units along the western Gondwana in La Pampa, Argentina. Earth Planets Space 60, 1–7. doi:10.1186/BF03352898
Tomezzoli, R. N. (2009). The apparent polar wander path for South America during the permian–triassic. Gondwana Res. 15, 209–215. doi:10.1016/j.gr.2008.10.005
Tomezzoli, R. N., Tickyj, H., Rapalini, A. E., Gallo, L. C., Cristallini, E. O., Arzadún, G., et al. (2018). Gondwana’s apparent polar wander path during the permian-new insights from south America. Nature-Scientific Rep. 8, 8436. doi:10.1038/s41598-018-25873-z
Tomezzoli, R. N., and Vilas, J. F. (1999). Palaeomagnetic constraints on the age of deformation of the Sierras Australes thrust and fold belt, Argentina. Geophys. J. Int. 138, 857–870. doi:10.1046/j.1365-246x.1999.00914.x
Tomezzoli, R. N., and Vilas, J. F. (1997). Paleomagnetismo y fábrica magnética en afloramientos cercanos a las Sierras Australes de la provincia de Buenos Aires (López Lecube y González Chaves). Rev. Asoc. Geol. Argent. 52, 419–432.
Tomezzoli, R. N., Vizán, H., Tickyj, H., and Woroszylo, M. E. (2013a). Revisión de la posición del polo paleomagnético de Sierra Chica en la curva de desplazamiento polar aparente del Gondwana. Proc. Third Bienn. Meet. Latinmag 3. Special Issue Montevideo.
Turner, J. C., and Méndez, V. (1975). Geología del sector oriental de los departamentos de Santa Victoria e Iruyá, provincia de Salta, Argentina, 51. Córdoba: Academia Nacional de Ciencias de Córdoba, Boletín, 11–24.
Varela, R., Cingolani, C. A., and Dalla Salda, L. H. (1990). “Edad del granito del Cerro Colorado y su implicancia geotectónica,” in Sierras Australes de Buenos Aires (Actas, San Juan: 11° Congreso Geológico Argentino), 2, 279–282.
Varela, R., Dalla Salda, L., and Cingolani, C. A. (1985). Estructura y composición geológica de las sierras Colorada, Chasicó y Cortapié, Sierras Australes de Buenos Aires. Rev. Asoc. Geol. Argent. 40, 254–261.
Varela, R. (1978). Sierras Australes de la provincia de Buenos Aires: hipótesis de trabajo sobre su composición geológica y rasgos geotectónicos salientes. Rev. Asoc. Geol. Argent. 33, 52–62.
von Gosen, W., Buggisch, W., and Krumm, S. (1991). Metamorphism and deformation mechanisms in the sierras australes fold and thrust belt (Buenos Aires province, Argentina). Tectonophysics 185, 335–356. doi:10.1016/0040-1951(91)90453-y
von Gosen, W., and Prozzi, C. (1998). “Structural evolution of the Sierra de San luis (eastern sierras pampeanas, Argentina): implications for the proto-andean margin of gondwana,” in The proto-andean margin of Gondwana. Editors R. J. En Pankhurst, and C. W. y Rapela (London: Geological Society), 142, 235–258.
von Gosen, W. (2003). Thrust tectonics in the North Patagonian massif (Argentina): implications for a Patagonian plate. Tectonics 22, 5–33.
Weil, A. B., and Yonkee, A. (2009). Anisotropy of magnetic susceptibility in weakly deformed red beds from the Wyoming salient, Sevier thrust belt: relations to layer-parallel shortening and orogenic curvature. Lithosphere 1 (4), 235–256. doi:10.1130/l42.1
Keywords: CHIPA, Chilenia, Patagonia, southwest Gondwana, paleomagnetism, anisotropy of magnetic susceptibility, Paleozoic, paleogeography
Citation: Tomezzoli RN, Cristallini EO, Tickyj H, Arzadún G, Calvagno JM, Choque G, Febbo B and Saguas E (2023) Following the steps of CHIPA: Chilenia and Patagonia formed the same drift terrain that collided with the southwest Gondwana margin during the middle Paleozoic. Front. Earth Sci. 11:1225271. doi: 10.3389/feart.2023.1225271
Received: 19 May 2023; Accepted: 02 October 2023;
Published: 08 November 2023.
Edited by:
Laura Giambiagi, CONICET Mendoza, ArgentinaCopyright © 2023 Tomezzoli, Cristallini, Tickyj, Arzadún, Calvagno, Choque, Febbo and Saguas. This is an open-access article distributed under the terms of the Creative Commons Attribution License (CC BY). The use, distribution or reproduction in other forums is permitted, provided the original author(s) and the copyright owner(s) are credited and that the original publication in this journal is cited, in accordance with accepted academic practice. No use, distribution or reproduction is permitted which does not comply with these terms.
*Correspondence: Renata Nela Tomezzoli, cmVuYXRhQGdsLmZjZW4udWJhLmFy, cnRvbWV6em9saUBnbWFpbC5jb20=
Disclaimer: All claims expressed in this article are solely those of the authors and do not necessarily represent those of their affiliated organizations, or those of the publisher, the editors and the reviewers. Any product that may be evaluated in this article or claim that may be made by its manufacturer is not guaranteed or endorsed by the publisher.
Research integrity at Frontiers
Learn more about the work of our research integrity team to safeguard the quality of each article we publish.