- Research Institute of Petroleum Exploration and Development, Beijing, China
Introduction: The Muglad basin, located on the southern Central Africa Shear Zone, is an intracontinental passive rift basin. The Fula sag, situated in northeastern of the basin, is a hydrocarbon-rich depression. As exploration advanced, the Fula sag has entered the stage of fine exploration for complex fault blocks. Therefore, studying the characteristics and evolution of faults can aid in deepening the understanding of hydrocarbon accumulation rules in the area, and guide the next steps of oil and gas exploration. Previous studies have primarily focused on the division of fault level and their role in hydrocarbon accumulation, but have not delved into the geometric features and evolution of the fault system across the entire Fula sag.
Methods: In this paper, we systematically studied the fault system and its hydrocarbon control within the sag using petroleum seismic reflection profiles, as well as logging data.
Results and Discussion: And the conclusions are as followed: 1) tectonic features in diverse areas of the Fula sag vary significantly from a shovel-like half-graben to the asymmetric graben, and faults in the study area can be classified into five combination features. 2) Three complete rift-sag cycles have developed since the early Cretaceous, with continuously active faults controlling the formation of “inherited” sag. 3) The strong activity of faults in the first and second riftting period led to the development of mainly AG-Formation source rocks and middle reservoir-forming assemblages in the Fula sag. Additionally, the formation and distribution of oil and gas reservoirs in the sag were controlled by the faults in the central fault zone and the western steep slope zone. Furthermore, hydrocarbons migrated and formed reservoirs through the main faults in the central fault zone and the western main fault. Our research will provide a valuable insight for understanding the petroleum geological characteristics of basins with a similar genesis mechanism.
1 Introduction
Africa plays a pivotal role in global oil and gas exploration and development (Zhang et al., 2023). The basins that have evolved adjacent to the Central African Shear Zone (CASZ) within the African continent represent a significant onshore Frontier for oil and gas exploration in Africa (Dou et al., 2022). Since the 1990s, the entry of China National Petroleum Corporation (CNPC) into the oil and gas exploration and development market in the Muglad basin, central Africa has led to the discovery of numerous oil fields, including the Unity field and Fula field, which have significantly contributed to China’s overseas oil and gas strategy. As the exploration advanced, specific regions within the basin evolved to the phase of detailed exploration of complex fault blocks.
The fault system plays a pivotal role in controlling the formation of traps, as well as the migration and accumulation of hydrocarbons into reservoirs (Yang et al., 2007). Thus, a thorough investigation of fault system here can lead to a comprehensive understanding of reservoir formation in the region and guide further exploration efforts. A few researchers have classified the level and activity period of the fault system (Wang et al., 2000; Huang et al., 2017) and analyzed its hydrocarbon control in the Fula sag (Yu et al., 2007; Zhang et al., 2008). They categorized the faults within the Fula sag into four levels based on the size of their influence areas. Subsequently, they divided activity period of faults into five stages by considering the fault configurations on profiles, stratigraphic relationships, and their control over sedimentation. They also found that the role of faults in controlling hydrocarbons in the Fula sag have primarily focused on the faults’ control on the trap formation, prevention of continued hydrocarbon migration, and provision of channels for hydrocarbon migration and accumulation. However, the fault characteristics, evolution, and their controlling on the hydrocarbon remain poorly understood.
Hence, this study systematically examines fault attributes, evolutionary mechanisms, and the determinants influencing hydrocarbon occurrences within the Fula sag of the Muglad Basin, serving as a representative passive rift basin within an extensional tectonic setting. This endeavor aims to enhance comprehension of reservoir genesis and allow for a valuable insight into fault systems in intracontinental passive rift basins characterized by similar genetic processes.
2 Geological setting
Three major rift systems, namely, Western, Central and Eastern African Rift Syetem are developed within the African continent (Yasuhiro and Ken, 2004; Zhang et al., 2015). Among them, the Central African Rift System, also known as the CASZ, is strike-slip in nature, includes a series of sedimentary basins, like the Bongor, Doba-Doseo and Muglad basin (Browne and Fairhead, 1983; Browne et al., 1985; Fairhead and Green, 1989; Jorgensen and Bosworth, 1989). The Muglad basin is located in Sudan-South Sudan, the northwestern part of the African continent, and its tectonic position is in the southeast of the middle part of the CASZ (Figure 1A), which is a Middle and Cenozoic intra-land passive rift basin developed on Precambrian basement, with an overall triangular shape of wide-northwest and narrow-southeast (Zhang and Chen, 2002; Tong et al., 2004; Zhang and Qi, 2007; Shi et al., 2014). The Fula sag is located in the northeastern part of the Muglad basin, with an area of about 5,000 km2 and an overall sub-north-south elongated distribution (Figure 1B) (Zhang et al., 2008).
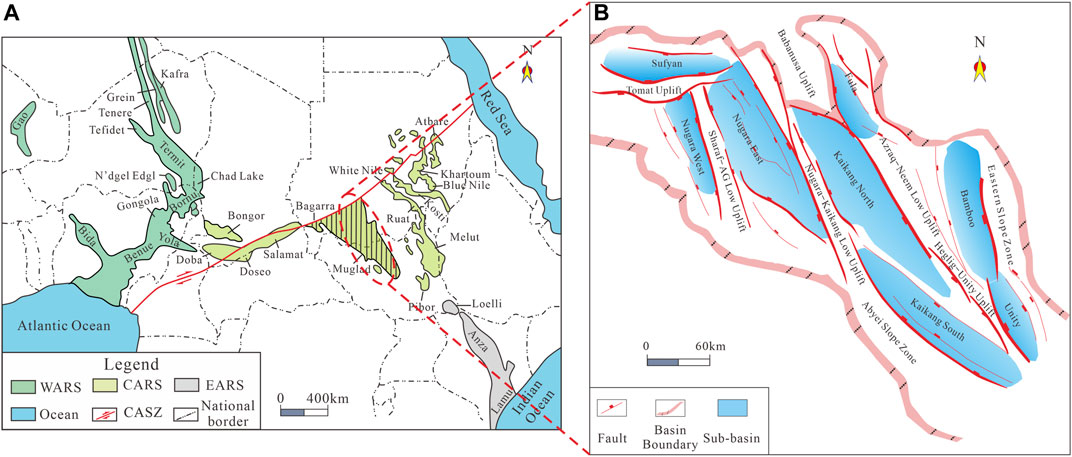
FIGURE 1. Location map (A) of Muglad basin and its tectonic unit division map (B) showing Fula sag at the northeast of the basin. Key: CASZ = Central African shear zone; WARS= West African rift system; CARS= Central African rift system; EARS= Eastern African rift system [modified after Dou et al. (2011)].
The Fula sag is separated from the Babanusa uplift by an east-trending fault ① (Figure 2A). The eastern boundary is a west-trending fault ②, and the northeastern part transitions to the uplift by a slope. The southwestern part of the sag is separated from the Kaikang North sag by an SW-trending fault ③, and the southern part is adjacent to the Azraq-Shelungo-Unity uplift. However, due to the limitation of seismic data, the specific boundary between these areas is not known. Based on the sub-basial structure and fault characteristics, the Fula sag can be divided into seven sub-tectonic units from north to south, including the northeast fault terrace belt, the northern sub-sag, the southern sub-sag, and so on (Wu et al., 2015).
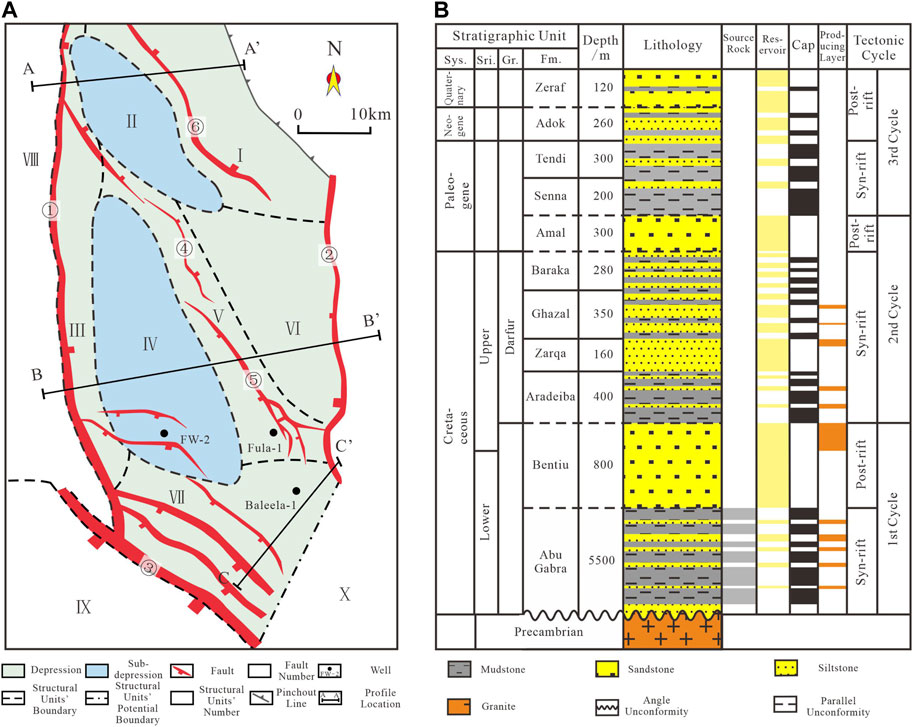
FIGURE 2. Tectonic unit division (A) and regional stratigraphy (B) of the Fula sag, Sudan and South Sudan [modified after Yang and Zhu (2008); Wu et al. (2015); Wang et al. (2019)] Tectonic unit: Ⅰ-Ⅶ northeast fault terrace belt, northern sub-sag, western steep slope zone, southern sub-sag, central fault zone, eastern slope zone and southwest fault terrace belt; Ⅷ is Babanusa uplift; Ⅸ is Kaikang North sag; Ⅹ is Azraq-Shelungo-Unity Uplift; ①∼⑥ are faults.
The Fula sag has undergone three cycles of rifting, driven by the expansion of the Atlantic Ocean, the northward movement of the Indian Plate, and the openness of the Red Sea (Wang et al., 2000; Tong et al., 2004; Dou, 2005; Zhang and Qi, 2007; Wang et al., 2019; 2021). The initial phase of rifting was predominantly driven by the opening of the Atlantic Ocean, inducing a right-lateral strike-slip stress regime along the CASZ (Fairhead and Binks, 1991; Fairhead, 1992; Guiraud and Maurin, 1992; Genik, 1993). As this shearing motion propagated northeastward from the Congo Craton, the CASZ evolved from a shear-dominated regime to one characterized by extensional stresses (Wei and Liu, 2003). Subsequently, the second rifting episode was primarily influenced by the rapid formation of the Indian Ocean, leading to a reduction in the near-NE-striking stress field across central Africa (Suo, 2014). Finally, the third rifting phase was associated with the separation of the Red Sea, resulting in a near-NE-striking extensional stresses within the African plate (Binks and Fairhead, 1992; Sun et al., 2010).
Each cycle comprises a syn-rift and a post-rift thermal subsidence (Figure 2B). The sedimentary period of Abu Gabra Formation (referred to as AG Formation) and Bentiu Formation are the syn-rift stage and the post-rift stage of the first rifting, respectively; the sedimentary period of Darfur Group and Amal Formation are the syn-rift stage and the post-rift stage of the second rifting, respectively; the sedimentary period of Senna-Tendi Formation and Adok-Zeraf Formation are the syn-rift stage and the post-rift stage of the third rifting, respectively. AG Formation can be divided into 5 members, AG1-AG5 Member, from top to bottom.
3 Datasets and methodologies
The seismic dataset utilized in this investigation encompasses three 2D seismic reflection profiles (Figure 2), which span the entirety of the Fula sag. Each of these profiles records a duration of 5.0 s two-way travel time (TWT), featuring a vertical sampling rate of 2 ms. The seismic data exhibits a positive polarity, with a predominant frequency range falling between 30 and 50 Hz. The acoustic velocities within the AG to Zaraf section vary from 2000 to 5,000 m/s, resulting in an estimated vertical seismic resolution ranging from 40 to 167 m. Stratigraphic correlation was conducted utilizing data from 10 wells, selected from a pool of more than 120 exploration and evaluation wells throughout the study area.
For the purpose of elucidating lateral and vertical variations in the structure of the extensional faults, we identified and interpreted eight key horizons. These horizons include the bottoms of the Senna Formation, Amal Formation, Darfur Group, Bentiu Formation, AG1 Member, AG2 Member, AG3 Member, AG4 Member, and AG5 Member, respectively. These horizons were consistently mapped across the research area, facilitating the delineation of the fault system under investigation, which we designated as Faults ① to ⑥ (as illustrated in Figure 2). This meticulous seismic interpretation has furnished us with an accurate portrayal of the structural geometry of the Fula sag.
To reconstruct the evolutionary history of the fault system within the study region, we conducted an in-depth analysis of the tectonic evolution in the central Fula sag, particularly focusing on the B-B′ section displayed in Figure 2. To achieve this, we employed the time-depth equation derived from data obtained from the FW-2 well (as indicated in Figure 2). Subsequently, we performed a time-depth conversion of section B-B’, followed by the recovery of its evolutionary history using the 2D Move 2018.1.0 software. Moreover, we selected the Fula-1 well and Baleela-1 well, both of which are depicted in Figure 2, for a comprehensive single-well subsidence history analysis.
Lastly, we conducted an extensive study encompassing source rock evaluation, sedimentary facies analysis, hydrocarbon distribution characteristics, and a comprehensive summary of the hydrocarbon migration-accumulation patterns to explore the influential role of faults in controlling hydrocarbon occurrences within the Fula sag. In the course of this research endeavor, geochemical profiles for 16 wells were meticulously established.
4 Fault characteristics
Under the influence of regional tectonic movements, the Fula sag mostly develops two groups of strike normal faults, whose strikes are respectively SN and NW (Zhang and Chen, 2006; Wang et al., 2007). They have flat fault surfaces and large dip angles, and no detachment is detected.
The tectonic features in different areas of the Fula sag vary hugely. In the northern part of the study area, the FW fault (fault ① in Figure 3) controls the deposition of the Northern Sub-sag, forming a shovel-like half-graben with a western fault and layers eastward overlapping; in the central part of the sag, under the joint control of the FW fault, the central fault zone and the FE fault (fault ①, fault groups ④ and ⑤ and fault ② in Figure 4), a graben-horst style consisting of two grabens and a horst is formed; in the southern part of the sag, the stratum is not overtopped in the adjacent Kaikang North sag, so this is a graben controlled by both the FE fault (fault ② in Figure 5) and the fault within the Kaikang North sag, rather than a half-graben.
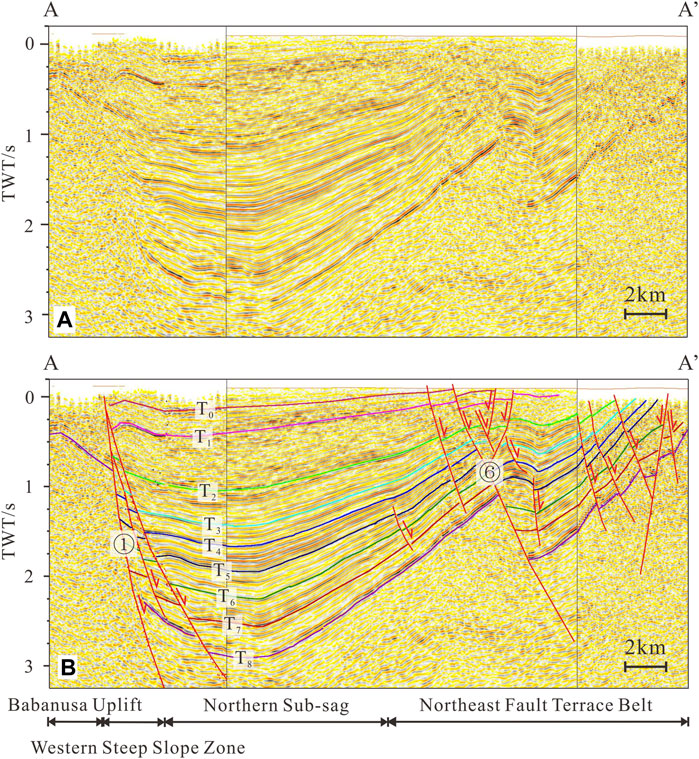
FIGURE 3. The A-A′ seismic profile (A) and its geological interpretation (B), northern Fula sag. The profile location is shown in Figure 2. To. Senna Fm. bottom; T1. Amal Fm. bottom; T2. Darfur Gr. bottom; T3. Bentiu Fm. bottom; T4. AG1 Mbr. bottom; T5. AG2 Mbr. bottom; T6. AG3 Mbr. bottom; T7. AG4 Mbr. bottom; T8. AG5 Mbr. bottom.
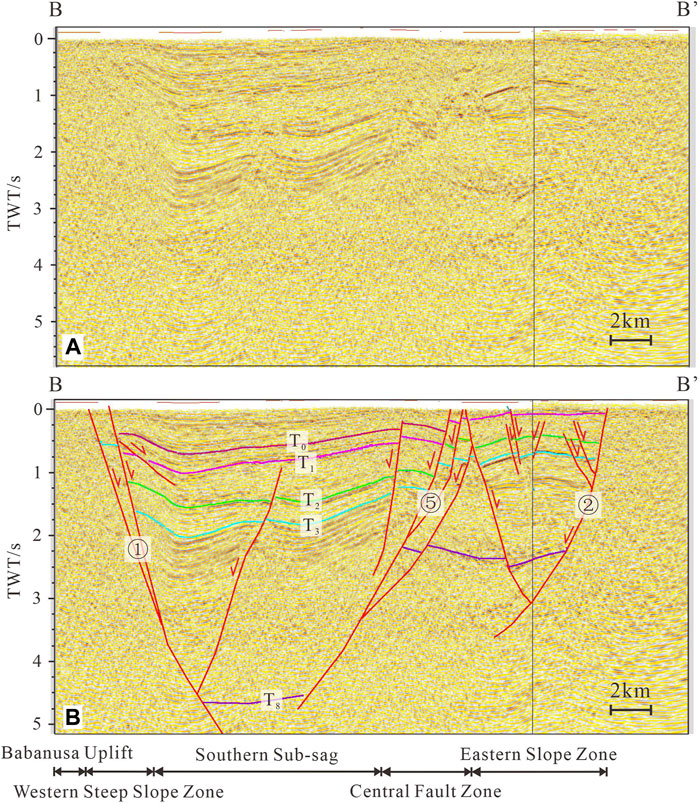
FIGURE 4. The B-B′ seismic profile (A) and its geological interpretation (B), central Fula sag. The profile location is shown in Figure 2; To- T3 and T8 are shown in Figure 3.
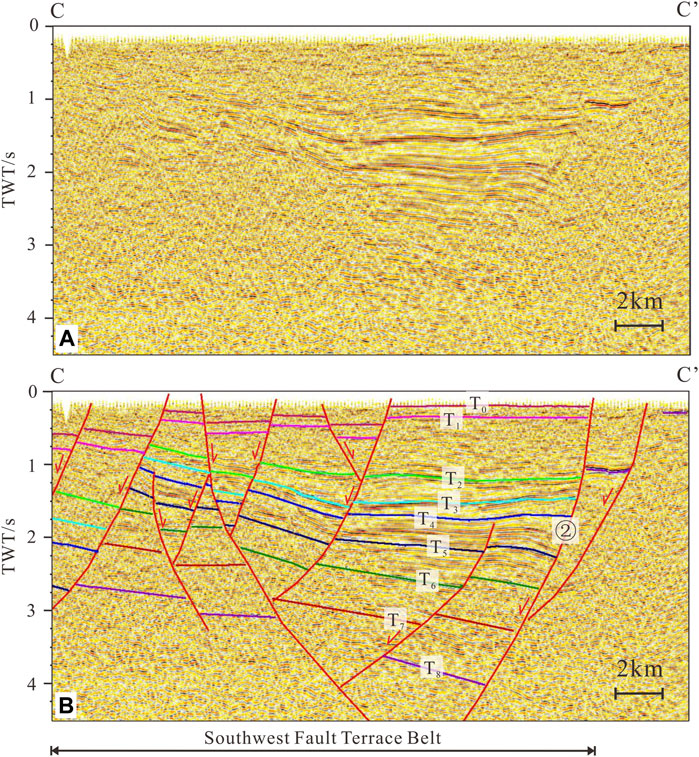
FIGURE 5. The C-C′ seismic profile (A) and its geological interpretation (B), southern Fula sag. The profile location is shown in Figure 2; To- T3 and T8 are shown in Figure 3.
The stratum is complete in the northern part of the Fula sag (Figure 3), and the maximum thickness is located in the northern sub-sag, which can reach 4,900 m. The whole area is a half-graben with a western fault and layers eastward overlapping, in which the FW normal fault is a shovel-shaped normal fault, and the stratum is eastward overlapping. The domino normal faults dipping NE and Y-shaped normal fault are also developed in the northeastern northeast fault terrace belt.
The stratum of the central Fula sag (Figure 4) is well developed, with the largest thickness in the Southern sub-sag up to 9,500 m. The FW fault (① in Figure 4) is a shovel-fan normal fault, which is highly active, and the fault group ⑤ is a west-dipping shovel-fan normal fault in the southern part of the central fault zone. The eastern slope zone is developed with conjugate and Y-shaped normal faults.
The southern part of the Fula sag predominantly passes through the Southwest Fault Terrace Belt, a sub-structural unit (Figure 5). The stratum in this region is complete, and the maximum thickness is 7,300 m. The stratigraphic layer is not overtopped in the adjacent Kaikang North Depression, so this is a graben controlled by both the FE fault (fault ② as a west-dipping shovel-fan in Figure 5) and the fault within the Kaikang North sag, rather than a half-graben where the east is broken and the west is overtopped. In addition to the domino-type normal faults dipping SW, there are also Y-shape normal faults in this area.
In a word, five structural styles are identified in the Fula sag, including shovel-shaped, Y-shaped, domino-type, shovel-fan, and conjugate (Table 1).
5 Evolution of fault system
5.1 Fault activity period
Fault evolution history recovery is a vital tool to reflect the evolutionary history of fault systems (Shen et al., 2005; Kumar et al., 2023). Therefore, based on previous research (Lu et al., 2001), the evolutionary history of the fault system in the study area was recovered by studying the tectonic evolutionary history in the central Fula sag (B-B′ section in Figure 4). The time-depth equation derived from the FW-2 well (the well location is shown in Figure 2 and the time-depth equation is shown in Figure 6) was used to perform a time-depth conversion of the section B-B′, then its evolutionary history was recovered. The minimum depth of the FW-2 well is 23m, and the maximum depth is 3,513 m. The deepest depth is drilled to the AG2 Member. The well did not cross the fault and there was no interruption of stratigraphy; the correlation coefficient of the well’s time-depth equation is R2=0.9985, which is a good fit. According to the above reasons, the time-depth equation of the FW-2 well is selected for time-depth conversion.
In the vertical direction (Figure 7), the thickness of depression deposition is the largest in the first rifting period (i.e., AG-Bentiu period). The activity of the depression gradually decreases and the thickness of deposition also gradually thins. Its specific performance is that the second rifting period (i.e., Darfur-Amal period) is thinner than the first rifting period, and the third phase of rifting is thinner than the second rifting period. The whole depression shows the “inherited” development characteristic with gradually lighting activity. On the map view, among the sedimentary strata in the same period, the western strata are thicker than the eastern one, which is mainly due to the stronger activity of fault ① and ⑤ than fault ②.
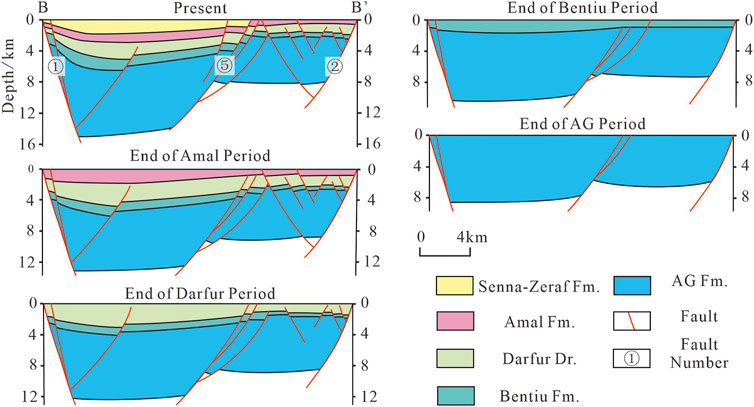
FIGURE 7. The B-B′ profile tectonic evolution, central Fula sag. The profile location and order number of faults are shown in Figure 2; the seismic section is shown in Figure 4.
The main faults controlling the depressions and tectonic zones, such as fault ① (Figure 7), have been continuously active since the Early Cretaceous and control the sedimentation in the area, with the strongest activity in the first syn-rift period (i.e., AG period); fault ⑤ (Figure 7) has been continuously active since the Early Cretaceous, with the stronger activity in the first two rifting periods (i.e., AG-Amal period).
5.2 Fault activity intensity
Fula-1 well and Baleela-1 well were selected for single-well subsidence history analysis (see Figure 2 for well locations) for the following reasons. First of all, both wells were deep enough and drilled to the middle layer of the AG Formation, with the maximum depth of 2,404 m for the Fula-1 well, which was drilled to the AG2 Member, and the maximum depth of 3,210 m for the Baleela-1 well, which was drilled to the AG3 Member. Then, both wells were not encountering faults and the whole stratigraphy was intact. Lastly, these two wells were located in different tectonic positions in the Fula sag, especially the Fula-1 well is located in the central fault zone and the Baleela-1 well is located in the southwest fault terrace belt.
From the subsidence history of the above wells (Figures 8, 9), it is known that the Fula sag is a typical “inherited” depression. The first rifting period is most active, with subsidence values of 4,300 m and 3,800 m, and subsidence rates of 84 m/Ma and 74 m/Ma, respectively. The second rifting period is relatively active, with subsidence values of 750 m and 1,000 m, and subsidence rates of 24 m/Ma and 32 m/Ma, separately. Fula-1 well is located in the southern sub-sag, so the subsidence value is larger.
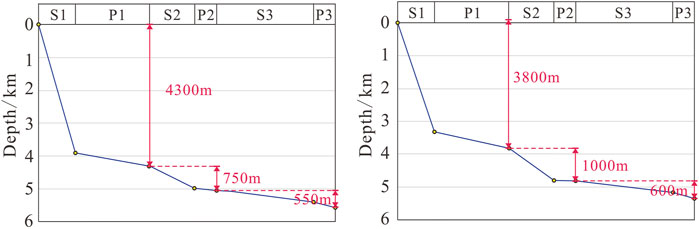
FIGURE 8. Typical-well subsidence history in Fula sag. well locations are shown in Figure 2. R1-R3 represent 1st-to-3rd syn-rift periods; P1-P3 represent 1st-to-3rd post-rift periods.
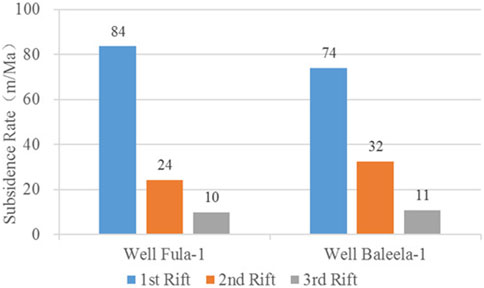
FIGURE 9. Subsidence rate comparison of typical wells in Fula sag. Well locations are shown in Figure 2.
Combined with the previous studies on the fault activity period and activity intensity, it is clear that fault activity intensity in the Fula sag is the strongest and the subsidence is the largest in the first rifting period; fault activity in the Fula sag is stronger and the depressional subsidence is larger in the second rifting period; the fault activity is the weakest and the subsidence is also the smallest in the third rifting period. Main faults in the Fula sag have been continuously active since the Early Cretaceous, controlling the formation of “inherited” depression.
6 Controlling of faults on the hydrocarbon
Based on the fault characteristics, evolutionary history analysis of Fula sag, and combined with geological and geochemical data, we carried out research on source rock evaluation, sedimentary face analysis, hydrocarbon distribution characteristics and hydrocarbon migration-accumulation pattern summary, and discussed the faults’ control role on hydrocarbon in Fula sag.
6.1 Control on source rocks
The AG Formation lacustrine dark mudstone is the only mature source rock in the Fula sag, and all the hydrocarbons found in the depression originate from this set of source rocks (Dou et al., 2013). Among them, the mudstone is more developed during the lake level rising in the AG2 period. In this paper, we applied the results of extensive geochemical analysis and logging source rock evaluation, explored the control role of fault evolution on source rocks, predicted and evaluated the distribution of AG2 source rocks.
In this research, geochemical profiles of 16 wells were established for the source rocks in the AG2 Member, among which the representative geochemical profile is the Baleela-1 well (Figure 10). From these geochemical profiles, it is well known that the TOC, S1+S2 are all high in the AG2 Member of this depression, which represents excellent source rocks; HI is mostly greater than 350 mg/g. TOC and even greater than 700 mg/g. TOC, which indicates that its organic matter types are dominantly II1 and I; Tmax is all greater than 435°C, indicating that source rocks in the AG2 Member are mature.
According to the experimental analysis data, single-well geochemical profiles were established. And TOC, Ro, and thickness (TOC>1%) contours of hydrocarbon source rocks in the AG2 Member of the Fula sag are plotted separately by integrating above single-well geochemical profiles and fault spreading (Figure 11). The TOC content of the AG2 Member of the Fula sub-sag is greater than 1%, and the hydrocarbon source rocks are good and excellent. The thickness is greater than 100m, and has high hydrocarbon generation potential. According to the current study, the lower limit of hydrocarbon discharge of the AG Formation in the Fula sag should be Ro=0.6% (Dou et al., 2011). According to this criterion, the northern sub-sag of the Fula sag has just entered the threshold of hydrocarbon discharge, while the southern sub-sag has entered the peak of hydrocarbon generation, so the hydrocarbon generation center should be the southern sub-sag. The source rocks of the AG2 Member in the southern sub-sag started to produce and discharge hydrocarbons in the second syn-rift period (i.e., Darfur period) and reached the peak since the Paleoproterozoic (Li et al., 2018).
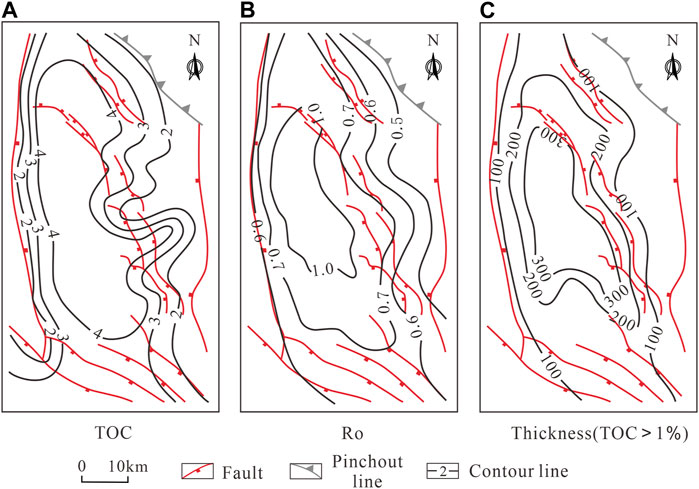
FIGURE 11. Source rock evaluation map of AG2 Member in Fula sag. (A) represents the total organic carbon content (TOC) of the AG2 Member source rocks, while in (B), the maturity of AG2 Member source rocks is illustrated. In (C), the thickness of stratigraphic units with TOC > 1% in the AG2 Member is depicted.
From the analysis of the previous fault evolution history, we can learn that: during the first syn-rift period (i.e., AG period), the Fula sag had the largest subsidence rate and the strongest fault activity, and the lacustrine mudstone is mainly developed in the depression, which is favorable to the development of hydrocarbon source rocks of the AG Formation; since the Early Cretaceous, the fault ① was more active, and the depression subsidence center was located in the southern sub-depression near that fault, so that the hydrocarbon source rocks here were buried rapidly and reached the hydrocarbon generation threshold earliest.
6.2 Control on reservoir-forming combinations
The Fula sag developed three reservoir-forming combinations: upper, middle, and lower. The main reservoir combination is the middle reservoir-forming combination, whose reservoir is the Bentiu-Aradeiba Formation sandstone and caprock is the Aradeiba Formation mudstone deposited by the second syn-rift period (i.e., Darfur period). Petroleum discovery in this reservoir-forming combination accounts for 76% of the total discovery. The secondary reservoir-forming combinations include the lower and upper one. The lower reservoir-forming combination is an autogenic self-storage combination within the AG Formation during the first syn-rift period, whose reservoir is the deltaic submerged channel and beach-dam-face sandstones within the AG Formation, and whose cap rock is the widely developed lacustrine mudstones within the AG Formation. Lower reservoir-forming combination accounts for about 16% of the total discovery. The upper formation consists of the Zarqa, Ghazal, and Baraka Formation sandstones, capped by the Ghazal and Baraka Formation mudstones, which account for about 8% of the total.
From the analysis of the previous fault evolution history, it is clear that in the Fula sag, fault activity was the strongest in the first syn-rift period (i.e., AG period), which was favorable to the development of hydrocarbon source rocks of AG Formation, and the fault activity was weaker in the first post-syn period (i.e., Bentiu period), when the thick-layered sandstone of Bentiu Formation was developed. In the second rifting period (i.e., Darfur-Amal period), faults in the Fula sag were relatively active and the depressional subsidence was relatively large, depositing the regional cap rock, Aradeiba Formation mudstone. In the third rifting period (i.e., Sennal-Zeraf period), the faults’ activity was weakest, and most of the hydrocarbon was stored under this set of mudstones, making the Bentiu group sandstone the main reservoir in the whole area. The key factors controlling the reservoir resources and distribution of the Bentiu Formation are the throw of the controlling-trap faults and the lateral blocking of the Aradeiba Formation mudstones, and the oil column height of the reservoir depends on the throw of the controlling-trap faults and the thickness of the Aradeiba Formation mudstones.
6.3 Control on reservoir types
The Fula sag is dominated by tectonic and tectonic-lithologic reservoirs such as reverse fault blocks and faulted horsts (Figure 12). Among them, the reverse block reservoirs are developed in the central fault zone, whose reservoirs are Bentiu Formation sandstones and whose cap rocks are Aradeiba Formation mudstones. The faulted-horst reservoirs are concentrated in the southern part of the central fault zone, and their reservoirs and caps are also Bentiu group sandstones and Aradeiba group mudstones. The consequent-fault-block reservoirs are mainly concentrated in the western steep slope zone, whose reservoirs are the layered sandstone within AG Formation, and whose cap rock is the mudstone of AG Formation around these reservoirs, and where the hydrocarbon is sealed by the fault ① and the mudstone cap rocks. Faulted-anticline reservoirs are mainly concentrated in the southern sub-sag and the central region of the central fault zone, among which the reservoirs in the southern sub-sag are also sandstones within the AG Formation, and the reservoirs in the central part of the central fault zone are mainly concentrated in the middle reservoir-forming combination. The rollover-anticline reservoirs are mainly concentrated in the upper combination in the northern region of the western steep slope zone, where the reservoir is the layered sandstone within the Darfur group and the caprock is the mudstone within the Darfur group around the reservoirs.
Many secondary faults are formed at the transition position between fault ④ and fault ⑤ in the central fault zone, and the sandstone reservoirs of Bentiu Formation are cut by the above secondary faults to produce reverse-fault-block, faulted-anticline, and other reservoirs; rollover-anticline reservoirs are developed in the southern sub-sag under the control of fault ⑤, and the sandstones of Bentiu-Aradeiba Formation above are cut by the secondary faults to form reverse fault blocks and faulted horsts; the fault ① makes the AG Formation and the Darfur group in the western steep slope zone develop consequent-fault-block and rollover-anticline reservoirs respectively.
In conclusion, the faults within the central fault zone and the western steep slope zone control the Formation of tectonic and tectonic-lithologic reservoirs within the depression.
6.4 Control on migration and accumulation
The hydrocarbons discovered in the Fula sag are distributed around the fault zone near the southern sub-sag and are concentrated along the central fault zone and the western steep slope zone in the planar. They are controlled by the development degree of the three rifting periods in the vertical, and are concentrated in the middle and lower reservoir-forming combinations in the central fault zone, and are distributed in the three combinations in the western steep slope zone.
In the first syn-rift period of the Early Cretaceous (i.e., AG Formation period), the rifting is the strongest, and undercompensating is significant when the depressional deposition is dominated by lake-face organic-rich dark mudstone. In the second syn-rift stage of the Late Cretaceous (i.e., Darfur group deposition period), the rifting weakened. The burial depth of AG-group hydrocarbon source rocks increased, and AG-group hydrocarbon source rocks entered the hydrocarbon generation and discharge threshold then. Finally, in the third syn-rift stage (i.e., Senna-Tendi Formation period), the boundary faults are less active, the sedimentary stratigraphy is thinner, and the AG- Formation hydrocarbon source rocks reach the peak of hydrocarbon production and discharge.
The AG-Formation source rocks in the southern sub-sag began to discharge hydrocarbons during the second syn-rift period and reached the peak since the Paleocene, and hydrocarbons were migrated into the trap through the main faults on both sides (Figure 13). It is believed that the central fault zone is more active in the first and second syn-rift periods (i.e., AG and Darfur period), and hydrocarbons gathered here to form reservoirs then, while the third syn-rift period (i.e., Senna-Tendi period) is less active or inactive, and most of the hydrocarbons stay under this set of mudstone, making the Bentiu Formation sandstones the primary reservoirs in the central fault zone; while the fault ① is strong and persistently active, resulting in all three reservoir-forming combinations have hydrocarbon distribution.
Therefore, the main faults of the central fault zone and fault ① are the oil-source faults connecting the hydrocarbon source rocks of the AG Group and reservoirs in the middle and upper reservoir-forming combinations, and the hydrocarbon discharged in the Late Cretaceous and Neoproterozoic were migrated upward to the reservoir through these faults.
7 Conclusion
Using drilling, logging, and seismic data from the Fula sag, we conducted a comprehensive analysis of fault characteristics, formation, and evolution, and their impact on hydrocarbons. Our research involved calibrating seismic synthetic records, interpreting seismic data, and creating tectonic maps. The final findings can be summarized as follows:
(1) Tectonic features in the Fula sag vary greatly across different regions. The northern part exhibits a shovel-shaped half-graben with a western fault and overlapping layers that extend eastward. The central region features a graben-horst structure, while the southern part displays a graben. We identified five types of fault structural styles in the Fula sag, including shovel-shaped, Y-shaped, domino, shovel-fan, and conjugate.
(2) The Fula sag has undergone three complete rifting cycles. Fault activity was strongest during the first rifting, relatively strong during the second rifting, and weakest during the third rifting. The continuously active faults in the first and second rifting were responsible for forming the Fula sag as an “inherited” depression.
(3) Due to stronger fault activity during the first and second rifting periods, the Fula sag primarily features AG-formation hydrocarbon source rocks and middle reservoir-forming combination. Faults in the central fault zone and western steep slope zone control the formation and distribution of tectonic reservoirs in fault blocks and horsts. The main faults of the central fault zone and fault ① served as oil-source faults, connecting AG-Formation source rocks with middle and upper reservoir-forming combinations. Hydrocarbons discharged during the Late Cretaceous and Neoproterozoic were able to migrate upward to the reservoir through these faults.
Data availability statement
The original contributions presented in the study are included in the article/Supplementary Material, further inquiries can be directed to the corresponding authors.
Author contributions
YW conceived the idea; YZ conducted the analyses; RR provided the data; LW analysed the data; ZC, GW and YW interpreted the results; All authors contributed to the article and approved the submitted version.
Funding
The authors declare that this study received funding from China National Petroleum Corporation Limited (CNPC) Science and Technology Project (2021DJ0301). The funder was not involved in the study design, collection, analysis, interpretation of data, the writing of this article, or the decision to submit it for publication.
Acknowledgments
Jiguo Liu, Weili Ke, Quan Zou and other experts are gratefully acknowledged for assisting in data collection. We also acknowledge the reviewers and editor for their useful comments and corrections and their invaluable help.
Conflict of interest
The authors YW, GZ, GW, ZC, RR, LW, and YZ are employed by PetroChina Research Institute of Petroleum Exploration and Development.
Publisher’s note
All claims expressed in this article are solely those of the authors and do not necessarily represent those of their affiliated organizations, or those of the publisher, the editors and the reviewers. Any product that may be evaluated in this article, or claim that may be made by its manufacturer, is not guaranteed or endorsed by the publisher.
References
Binks, R. M., and Fairhead, J. D. (1992). A plate tectonic setting for Mesozoic rifts of West and Central Africa. Tectonophysics 213 (1-2), 141–151. doi:10.1016/0040-1951(92)90255-5
Browne, S. E., and Fairhead, J. D. (1983). “Gravity study of the central African rift system: a model of continental disruption: 1. The ngaoundere and Abu Gabra rifts,” in Developments in geotectonics. Editors P. Morgan, and B. H. Baker (Elsevier), Amsterdam, Netherlands, 187–203.
Browne, S. E., Fairhead, J. D., and Mohamed, I. I. (1985). Gravity study of the White Nile Rift, Sudan, and its regional tectonic setting. Tectonophysics 113 (1), 123–137. doi:10.1016/0040-1951(85)90113-1
Dou, L. R., Cheng, D. S., Li, Z., Zhang, Z. W., and Wang, J. C. (2013). Petroleum geology of the Fula sub-basin, Muglad Basin, Sudan. J. Pet. Geol. 36 (1), 43–59. doi:10.1111/jpg.12541
Dou, L. R. (2005). Formation mechanism and model of oil and gas accumulations in the Melut basin, Sudan. Bull. mineralogy, petrology Geochem. 24 (1), 50–57. doi:10.3969/j.issn.1007-2802.2005.01.007
Dou, L. R., Xiao, K. Y., Hu, Y., Song, H. R., Cheng, D. S., and Du, Y. B. (2011). Petroleum geology and a model of hydrocarbon accumulations in the Bongor basin, the Republic of Chad. Acta Pet. Sin. 32 (3), 379–386. doi:10.7623/syxb201103002
Dou, L., Xiao, K., Du, Y., Wang, L., Zhang, X., Cheng, D., et al. (2022). Exploration discovery and hydrocarbon accumulation characteristics of the Doseo strike-slip and inverted basin, Chad. Pet. Explor. Dev. 49 (2), 247–256. doi:10.1016/S1876-3804(22)60021-1
Fairhead, J. D., and Binks, R. M. (1991). Differential opening of the central and South atlantic oceans and the opening of the west African rift system. Tectonophysics 187, 191–203. doi:10.1016/0040-1951(91)90419-S
Fairhead, J. D., and Green, C. M. (1989). Controls on rifting in Africa and the regional tectonic model for the Nigeria and East Niger rift basins. J. Afr. Earth. Sci. 8 (2), 231–249. doi:10.1016/S0899-5362(89)80027-2
Fairhead, J. D. (1992). The west and central african rift systems: foreword. Tectonophysics 213, 139–140. doi:10.1016/B978-0-444-89912-5.50033-8
Genik, G. J. (1993). Petroleum geology of Cretaceous-Tertiary rift basins in Niger, Chad, and Central African Republic. AAPG Bull. 77 (8), 1405–1434. doi:10.1306/bdff8eac-1718-11d7-8645000102c1865d
Guiraud, R., and Maurin, J.-C. (1992). Early cretaceous rifts of western and central africa: an overview. Tectonophysics 213 (1-2), 153–168. doi:10.1016/0040-1951(92)90256-6
Huang, T. F., Zhang, G. Y., Liu, A. X., Shi, Y. L., Huang, S. P., Ke, W. L., et al. (2017). Structural features and evolution of the sufyan depression, Muglad Basin in Sudan-south Sudan. Sci. Geol. Sin. 52 (1), 34–45. doi:10.12017/dzkx.2017.002
Jorgensen, G. J., and Bosworth, W. (1989). Gravity modeling in the Central African Rift System, Sudan: rift geometries and tectonic significance. J. Afr. Earth. Sci. 8 (2), 283–306. doi:10.1016/S0899-5362(89)80029-6
Kumar, P. C., Sain, K., and Omosanya, K. O. L. (2023). Geometry and Kinematics of strike-slip faults in the Dibrugarh field of the Upper Assam foreland basin, NE India. Mar. Pet. Geol. 153, 106291. doi:10.1016/j.marpetgeo.2023.106291
Li, W., Dou, L. R., Zhang, G. Y., Cheng, D. S., Wen, Z. G., Liu, A. X., et al. (2018). Efficacy and safety of tenofovir in preventing mother-to-infant transmission of hepatitis B virus: a meta-analysis based on 6 studies from China and 3 studies from other countries. Earth Sci. Front. 25 (02), 121–129. doi:10.1186/s12876-018-0847-2
Lu, Y. C., He, B. Z., Wang, X. L., and Wang, W. Q. (2001). Petroleum geologic characteristics and exploration prospect of Fula sub-basin in Muglad Basin. Pet. Explor. Dev. 28 (03), 95–98. doi:10.3321/j.issn:1000-0747.2001.03.029
Shen, H., Li, C. B., Chen, F. J., Yin, W., Zhang, Y., Ligaba, A., et al. (2005). Citrate secretion coupled with the modulation of soybean root tip under aluminum stress. Up-regulation of transcription, translation, and threonine-oriented phosphorylation of plasma membrane H+-ATPase. Geoscience 19 (02), 287–296. doi:10.1104/pp.104.058065
Shi, Z. S., Fang, L. H., Wang, T. Q., Li, B. N., Su, Y. P., Ma, F. L., et al. (2014). Controlling effect of basin structure on hydrocarbon accumulation in Muglad basin, Sudan-South Sudan. Geol. Rev. 60 (02), 389–396. doi:10.16509/j.georeview.2014.02.014
Sun, H. T., Zhong, D. K., and Zhang, S. M. (2010). Difference in hydrocarbon distribution in passive margin basins of east and west Africa. Pet. Explor. Dev. 37 (05), 561–567.
Suo, Y. H. (2014). Tectonic-magmatic processes of the Indian Ocean: evidence on the residual mantle Bouguer anomaly. P.D. thesis (Ocean University of China) Qingdao, China.
Tong, X. G., Dou, L. R., Tian, Z. J., Pan, X. H., and Zhu, X. D. (2004). Geological mode and hydrocarbon accumulation mode in Muglad passive rift basin of Sudan. Acta Pet. Sin. 25 (01), 19–24. doi:10.3321/j.issn:0253-2697.2004.01.004
Wang, W. Q., Dou, L. R., Fan, T. L., Li, Z., and Zhang, Z. M. (2007). Structures and hydrocarbon accumulation in Fula sag of Muglad basin in Central Africa. Xinjiang Pet. Geol. 28 (03), 387–390. doi:10.3969/j.issn.1001-3873.2007.03.036
Wang, X. L., Wang, W. Q., Li, S. Z., Zhang, Y. M., and Fang, J. M. (2000). Structural feature of M Basin and its relation to oil and gas. Oil Gas. Geol. 21 (01), 76–79. doi:10.3321/j.issn:0253-9985.2000.01.019
Wang, Y. Q., Zhang, G. Y., Liu, A. X., Huang, T. F., and Ke, W. L. (2019). Fault characteristics of Fula sag, Muglad Basin in central africa. Chin. J. Geol. 54 (1), 145–158. doi:10.12017/dzkx.2019.009
Wang, Y. Q., Huang, T. F., Zhang, G. Y., Liu, A. X., and Ke, W. L. (2021). Fault characteristics of Fula sag, Muglad basin in central Africa. Chin. J. Geol. 56 (01), 340–353. doi:10.12017/dzkx.2021.020
Wei, Y. P., and Liu, C. Y. (2003). Geological model of the Mugland Basin -an identical example of basin evolution at the end of giant strike-slip faults. Pet. Geol. Exp. 25 (02), 129–136. doi:10.3969/j.issn.1001-6112.2003.02.005
Wu, D., Zhu, X. M., Li, Z., Su, Y. D., Liu, Y. H., Zhang, M. Y., et al. (2015). Depositional models in Cretaceous rift stage of Fula sag, Muglad Basin, Sudan. Pet. Explor. Dev. 42 (03), 348–357. doi:10.1016/s1876-3804(15)30025-2
Yang, H. J., Li, Y. J., Feng, X. J., Zheng, M., Zhang, C. L., and Zhao, Y. (2007). Analysison on thrustings of the Mazhatage structural belt in the Tarim basin. Sci. Geol. Sin. (03), 506–517. doi:10.3321/j.issn:0563-5020.2007.03.008
Yang, J. S., and Zhu, X. M. (2008). Lower cretaceous sequence stratigraphy of Fula depression, Muglad Basin, Sudan. Acta Sedimentol. Sin. 26 (06), 994–1004. doi:10.14027/j.cnki.cjxb.2008.06.020
Yasuhiro, Y., and Ken, M. (2004). 3-D analog modeling of inversion thrust structures. Washington, DC, USA: AAPG Special Volumes.
Yu, C., Bai, Y., Yu, Z. Y., and Yu, Z. L. (2007). Reservoir formation and oil and gas distribution of Fula Oilfiled, Sudan. Pet. Explor. Dev. 05, 633–639. doi:10.3321/j.issn:1000-0747.2007.05.021
Zhang, X., Dou, L., Nie, Z., Xiao, K., Wang, L., Du, Y., et al. (2023). Role of two-stage strike slip faulting in the tectonic evolution of the Doseo depression in the central Africa rift system. Front. Earth Sci. 11, 1–16. doi:10.3389/feart.2023.1087217
Zhang, Y. M., and Chen, F. J. (2006). Structure accommodation zone and exploration prospect in Muglad Basin. China Pet. explor. (03), 79–83. doi:10.3969/j.issn.1672-7703.2006.03.015
Zhang, Y. M., and Chen, F. J. (2002). The development feature and hydrocarbon exploration potential in Muglud Basin. Oil Gas. Geol. 23 (03), 236–240. doi:10.3321/j.issn:0253-9985.2002.03.007
Zhang, Y. M., and Qi, J. F. (2007). Structural geological characteristics and hydrocarbon enrichment of the Muglad Basin. Oil Gas. Geol. 28 (05), 669–674. doi:10.3321/j.issn:0253-9985.2007.05.019
Zhang, Y. M., Zhao, W. Y., Cheng, X. J., Chen, D. B., Xu, H., and Ding, L. (2008). Hydrocarbon enrichment patterns of Bonanza pool in Fula depression of Muglad Basin, Sudan. Geosci (04), 633–639. doi:10.3969/j.issn.1000-8527.2008.04.021
Keywords: Muglad basin, Fula sag, fault system, fault characteristics, hydrocarbon control
Citation: Wang Y, Zhang G, Wei G, Chen Z, Ren R, Wang L and Zhang Y (2023) The analysis of the fault system in the passive rift basin—an example from the Muglad Basin, central Africa. Front. Earth Sci. 11:1199070. doi: 10.3389/feart.2023.1199070
Received: 06 April 2023; Accepted: 13 October 2023;
Published: 24 October 2023.
Edited by:
Stanislaw Mazur, Polish Academy of Sciences, PolandReviewed by:
Yuejun Li, Chinese Academy of Sciences (CAS), ChinaKalachand Sain, Wadia Institute of Himalayan Geology, India
Copyright © 2023 Wang, Zhang, Wei, Chen, Ren, Wang and Zhang. This is an open-access article distributed under the terms of the Creative Commons Attribution License (CC BY). The use, distribution or reproduction in other forums is permitted, provided the original author(s) and the copyright owner(s) are credited and that the original publication in this journal is cited, in accordance with accepted academic practice. No use, distribution or reproduction is permitted which does not comply with these terms.
*Correspondence: Guangya Zhang, emd5QHBldHJvY2hpbmEuY29tLmNu; Guoqi Wei, d2VpZ3FAcGV0cm9jaGluYS5jb20uY24=