Glacial history and depositional environments in little Storfjorden and Hambergbukta of Arctic Svalbard since the younger dryas
- 1Division of Glacial Environment Research, Korea Polar Research Institute, Incheon, Republic of Korea
- 2Department of Geosciences, UiT The Arctic University of Norway, Tromsø, Norway
A Commentary on
Commentary: Glacial history and depositional environments in little Storfjorden and Hambergbukta of Arctic Svalbard since the younger dryas
by Noormets R and Kirchner N (2023). Front. Earth Sci. 11:1186972. doi: 10.3389/feart.2023.1186972
The main concern of the comment raised by Noormets and Kirchner is our interpretation of the Terminal Moraine Complex (TMC) formation at the Hambergbukta fjord mouth proposed by Joe et al. (2022). Noormets and Kirchner argue that the complex morphological features of the TMC likely resulted from multiple glacier advances and retreats after the Younger Dryas. They also point out that insufficient geophysical data sets and age uncertainty of core 905 (HH19-905-GC) obtained from the distal side of TMC led to an incomplete assessment of the TMC formation in Joe et al. (2022).
Noormets and Kirchner additionally defined three debris flow lobes (DFL-1, DFL-2, and DFL-3) on the distal flank of TMC. Based on these stacked debris flow lobes, they argue that the Hambergbreen may have reached the mouth of Hambergbukta fjord multiple times since the initial formation of TMC. We agree that multiple glacial advances can form multiple debris flow lobes. However, it is also possible for a single event of glacial advance or surge to generate multiple debris flows since the depositions of debris flow lobes can occur either concurrently with the terminal moraine formation or after its formation (Plassen et al., 2004). Here, we present additional subbottom profiling data (line 051 in Figure 1) covering two debris lobes (DFL-2 and DFL-3). Both DFL-2 and DFL-3 consist of acoustically transparent echo characters and are bounded by an erosive lower boundary, which traces into acoustic unit F-4C or lies above F-2C (Figure 1). If these lobe sets were deposited by different glacial advances, we would expect to identify some reflectors separating DFL-2 and DFL-3 (cf. Figures 3 and 4 in Kempf et al., 2013). However, a lack of prominent reflectors between the two debris flow lobes reflects deposition at a very similar time, which suggests that debris flow lobes were formed by a single event of glacial advance.
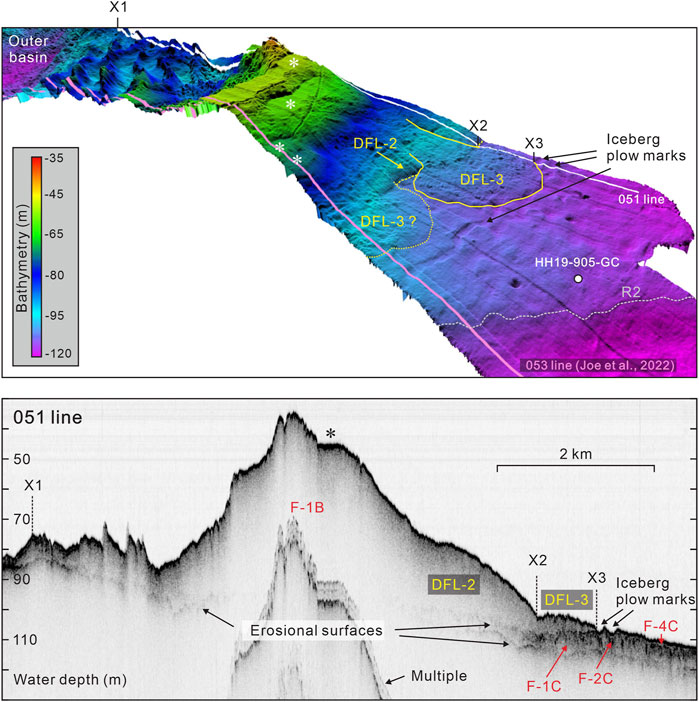
FIGURE 1. Multibeam bathymetry and high-resolution subbottom profiling data from the Terminal Moraine Complex (TMC) and its proximal and distal flanks. Asterisks (*) mark the flattened areas of the crest and distal flank of TMC suggested by Noormets and Kirchner.
Noormets and Kirchner also cast doubt on the age model of core 905 and the stratigraphic relation between TMC-related debris flow lobes (F-1B) and the late Holocene glaciomarine sediments (F-4C) in the distal side of TMC. The age model of core 905 was established using mainly four radiocarbon ages dated to 0.6, 3.6, 10.1 and 11.7 ka, respectively (Figure 7 in Joe et al., 2022). In particular, the late Holocene interval consisting of two lithofacies (Facies 3 and 4) was constrained based on two radiocarbon ages of 0.6 and 3.6 ka. The complex glaciomarine environments may have caused the internal variability in sedimentation rates, leading to the age uncertainty of the interpolated age during this interval. Nevertheless, Facies 3 generally exhibited consistent lithology with bioturbated mud to sandy mud and organic geochemistry with relatively high TOC and δ13C values and low TOC/Norg ratio, except for the clast-rich, uppermost parts. The distal side of TMC was likely characterized by the glacier-distal setting and oceanographic conditions during the late Holocene, suggesting a lack of significant changes in sedimentation rates. Additionally, the overall changes in sedimentation rates in core 905 were similar to those in a nearby site HH12-1209-GC in eastern Storfjorden (Rasmussen and Thomsen, 2014; Nielsen and Rasmussen, 2018). Hence, our age model is thought to be sufficient to reflect environmental changes during the late Holocene. Furthermore, it is worth noting that our interpretation of glacier surges during the late Holocene was based not only on IRD flux but also on variations in terrestrial inputs, as evidenced by TOC, δ13C, and TOC/Norg (Knies and Martinez, 2009), thus not misleading to determine glacier surges based solely on the increase in IRD as proposed by Noormets and Kirchner.
Joe et al. (2022) integrated seismostratigraphy with chronostratigraphy of core 905 in the distal side of TMC, assigning acoustic units F-1C, F-2C, and F-4C to the subglacial till during the Younger Dryas (>ca. 12 ka), melt out deposits during the earliest Holocene (12–10.1 ka), and glaciomarine sediment during the late Holocene (<ca. 3.7 ka), respectively (Figure 10 in Joe et al., 2022). As noted in Section 5.1 of Joe et al. (2022), our stratigraphic relation between TMC-related debris lobes (F-1B) and acoustic group C is somewhat ambiguous due to the insufficient vertical resolution of the subbottom profiling data. However, the additional subbottom profile of the 051 line (Figure 1) clearly shows that DFL-2 and DLF-3 lie above F-2C (Figure 1), which encompasses the period from the IRD-rich earliest Holocene to the middle Holocene characterized by the prominent decrease in sedimentation rates (Figure 8 in Joe et al., 2022). This suggests that DFL-2 and DLF-3 were formed at least after the middle Holocene, thus during the late Holocene. However, we cannot determine how much earlier DFL-1 formed than DFL-2 and DFL-3 because our data set does not cover the DFL-1 zone. A further geophysical and geological investigation is necessary to evaluate this speculation.
Regarding the complex morphology of TMC, Noormets and Kirchner argued that the flattened crest areas and terraces at depths up to 70 m on the distal flank of TMC were likely formed by large tabular iceberg scouring. Considering the shallow depth of inner Storfjorden and the submarine ridge (e.g., Mid-ridge), and the northwards increasing gradient of the glacio-isostatic response of the Storfjorden area (Forman et al., 2004 and references therein), they suggested that the large tabular icebergs possibly originated from the Storfjorden ice stream (or potentially ice shelf) that existed during the last deglaciation (cf. Nielsen and Rasmussen, 2018), rather than during the Holocene. If these iceberg scours had formed earlier than the Holocene, we would expect to identify a distinct glaciomarine sediment cover on the top of the flattened features, as exemplified in an acoustic unit F-4C on the distal side of TMC. However, the absence of glaciomarine sediment cover, such as an F-4 type acoustic unit, on top of flattened features (Figure 5 in Joe et al., 2022) argues against the possibility of pre-Holocene iceberg scouring. Furthermore, these flattened features on the distal flank of TMC appear morphologically to be synchronous with or after the formation of DFL-2 (Figure 1) during the late Holocene. In this context, the flattened features can be formed by large icebergs originating from Hambergbreen since the Little Ice Age (LIA). Such huge icebergs existed during LIA and even after AD1900 in the Hambergbukta, as identified by iceberg scours at depths of 70–80 m on the proximal flank of TMC (Figure 2B). We speculate that large icebergs overturned after passing TMC potentially and then formed flattened features, such as kettle holes (cf. Ottesen et al., 2017) on the distal flank of TMC (Figure 2A).
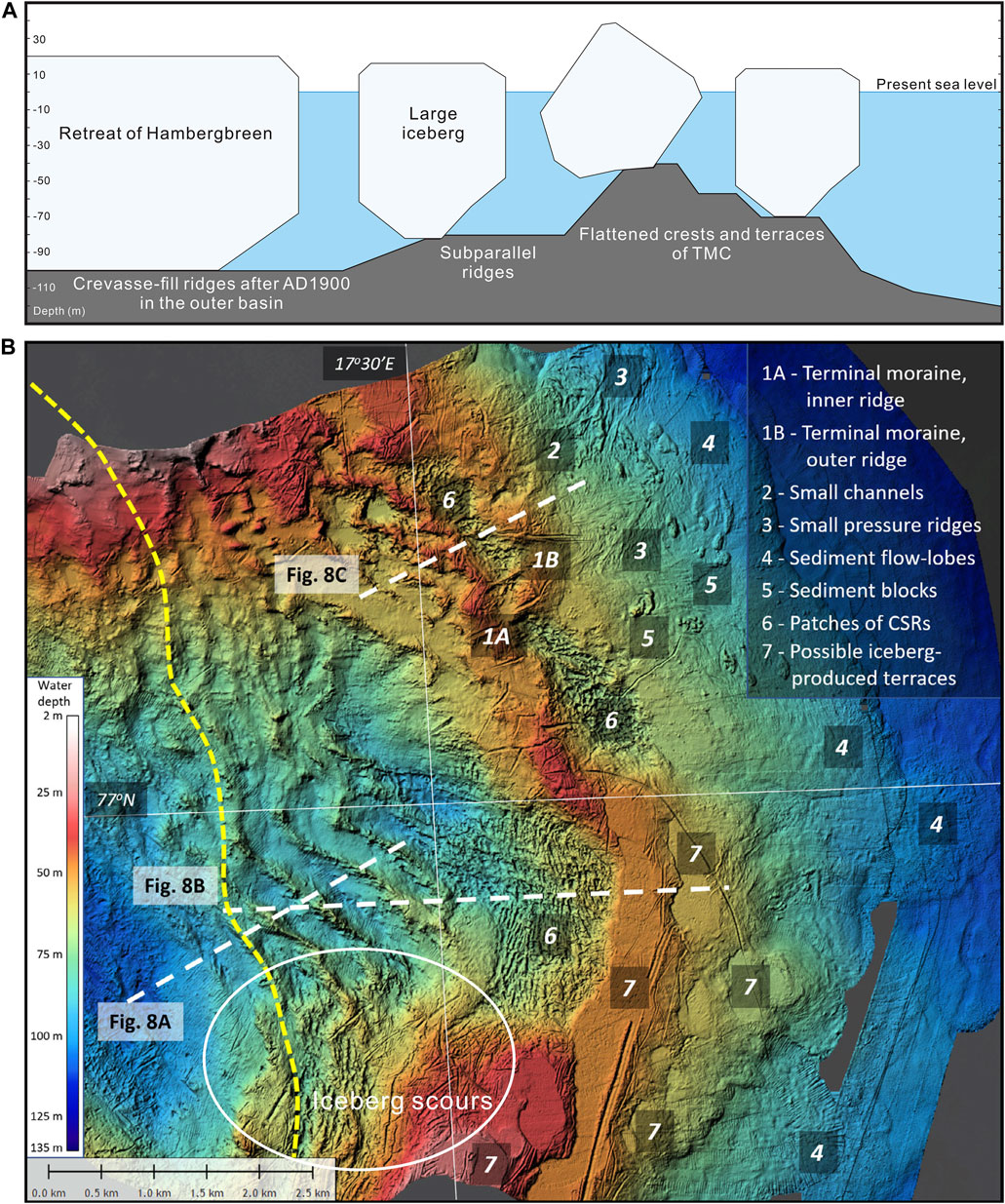
FIGURE 2. Schematic model for the potential formation of flattened crest areas and terraces on the distal flank of TMC (A) and multibeam bathymetry of TMC and its flanks in Figure 7 of Noormets et al. (2021) (B). In a lower panel, a white circle shows iceberg scours indicating the presence of huge icebergs originating from a Hambergbukta tidewater glacier (Hambergbreen).
In summary, our study aimed to understand the glacial history and depositional environments of Little Storfjorden and its tributary, Hambergbukta, since the Younger Dryas. Joe et al. (2022) suggested that the entire TMC was formed by multiple glacial advances (or surges) in agreement with Noormets and Kirchner’s interpretation. However, our geophysical and geological records support the conclusion that the entire TMC formed at least during the late Holocene. In particular, multiple significant advances (or surges) of Hambergbreen occurred after ∼1 ka, leading to the formation of TMC during the latest Holocene, including LIA, similar to northern Spitsbergen (Werner, 1993; Røthe et al., 2015; Røthe et al., 2018). Given the limited radiocarbon age dating and geophysical data set, there is a need for further investigation of the oldest set of debris lobes (DFL-1) on the distal flank of TMC to obtain reliable age data that shed more light on the behavior of Hambergbreen during the late Holocene.
Author contributions
YJJ and S-IN primarily wrote the reply to Noormets and Kirchner’s commentary. YJJ carried out the seismostratigraphic analysis. KJ, MF, and JSL have contributed to editing this reply. All authors contributed to the article and approved the submitted version.
Funding
This research was supported by a National Research Foundation of Korea Grant from the Korean Government (MSIS; the Ministry of Science and ICT) (NRF-2021M1A5A1075512; KOPRI-PN23013).
Conflict of interest
The authors declare that the research was conducted in the absence of any commercial or financial relationships that could be construed as a potential conflict of interest.
Publisher’s note
All claims expressed in this article are solely those of the authors and do not necessarily represent those of their affiliated organizations, or those of the publisher, the editors and the reviewers. Any product that may be evaluated in this article, or claim that may be made by its manufacturer, is not guaranteed or endorsed by the publisher.
References
Forman, S., Lubinski, D., Ingólfsson, Ó., Zeeberg, J., Snyder, J., Siegert, M., et al. (2004). A review of postglacial emergence on svalbard, franz josef land and novaya zemlya, northern eurasia. Quat. Sci. Rev. 23 (11-13), 1391–1434. doi:10.1016/j.quascirev.2003.12.007
Joe, Y. J., Jang, K., Forwick, M., Laberg, J. S., Kong, G. S., Kang, M.-H., et al. (2022). Glacial history and depositional environments in little Storfjorden and Hambergbukta of Arctic Svalbard since the younger dryas. Front. Earth Sci. 10, 2211. doi:10.3389/feart.2022.1017594
Kempf, P., Forwick, M., Laberg, J. S., and Vorren, T. O. (2013). Late Weichselian and Holocene sedimentary palaeoenvironment and glacial activity in the high-arctic van Keulenfjorden, Spitsbergen. Holocene 23 (11), 1607–1618. doi:10.1177/0959683613499055
Knies, J., and Martinez, P. (2009). Organic matter sedimentation in the Western Barents Sea region: Terrestrial and marine contribution based on isotopic composition and organic nitrogen content. Nor. J. Geology/Norsk Geol. Forening 89.
Nielsen, T., and Rasmussen, T. L. (2018). Reconstruction of ice sheet retreat after the Last Glacial maximum in Storfjorden, southern Svalbard. Mar. Geol. 402, 228–243. doi:10.1016/j.margeo.2017.12.003
Noormets, R., Flink, A., and Kirchner, N. (2021). Glacial dynamics and deglaciation history of Hambergbukta reconstructed from submarine landforms and sediment cores, SE Spitsbergen, Svalbard. Boreas 50 (1), 29–50. doi:10.1111/bor.12488
Ottesen, D., Dowdeswell, J., Bellec, V., and Bjarnadóttir, L. (2017). The geomorphic imprint of glacier surges into open-marine waters: Examples from eastern svalbard. Mar. Geol. 392, 1–29. doi:10.1016/j.margeo.2017.08.007
Plassen, L., Vorren, T. O., and Forwick, M. (2004). Integrated acoustic and coring investigation of glacigenic deposits in Spitsbergen fjords. Polar Res. 23 (1), 89–110. doi:10.1111/j.1751-8369.2004.tb00132.x
Rasmussen, T. L., and Thomsen, E. (2014). Brine formation in relation to climate changes and ice retreat during the last 15,000 years in Storfjorden, Svalbard, 76–78 N. Paleoceanography 29 (10), 911–929. doi:10.1002/2014pa002643
Røthe, T. O., Bakke, J., Støren, E. W., and Bradley, R. S. (2018). Reconstructing Holocene glacier and climate fluctuations from lake sediments in Vårfluesjøen, Northern Spitsbergen. Front. Earth Sci. 6, 91. doi:10.3389/feart.2018.00091
Røthe, T. O., Bakke, J., Vasskog, K., Gjerde, M., D'Andrea, W. J., and Bradley, R. S. (2015). Arctic Holocene glacier fluctuations reconstructed from lake sediments at Mitrahalvøya, Spitsbergen. Quat. Sci. Rev. 109, 111–125. doi:10.1016/j.quascirev.2014.11.017
Keywords: Hambergbukta, terminal moraine, shallow seismic stratigraphy, lithofacies, Little Ice Age, iceberg scouring
Citation: Joe YJ, Jang K, Forwick M, Laberg JS and Nam S-I (2023) Commentary: Reply to commentary by Noormets and Kirchner on “Glacial history and depositional environments in little Storfjorden and Hambergbukta of Arctic Svalbard since the younger dryas” [Frontiers in Earth Science, (2022), 2211]. Front. Earth Sci. 11:1196640. doi: 10.3389/feart.2023.1196640
Received: 30 March 2023; Accepted: 05 May 2023;
Published: 05 June 2023.
Edited by:
Nathan D. Stansell, Northern Illinois University, United StatesReviewed by:
Steven L. Forman, Baylor University, United StatesCopyright © 2023 Joe, Jang, Forwick, Laberg and Nam. This is an open-access article distributed under the terms of the Creative Commons Attribution License (CC BY). The use, distribution or reproduction in other forums is permitted, provided the original author(s) and the copyright owner(s) are credited and that the original publication in this journal is cited, in accordance with accepted academic practice. No use, distribution or reproduction is permitted which does not comply with these terms.
*Correspondence: Seung-Il Nam, sinam@kopri.re.kr