- 1Qingdao Institute of Marine Geology, CGS, Qingdao, China
- 2Laboratory for Marine Geology, Pilot National Laboratory for Marine Science and Technology, Qingdao, China
- 3Hubei Key Laboratory of Yangtze Catchment Environmental Aquatic Science, School of Environmental Studies, China University of Geosciences, Wuhan, China
- 4Institute of Marine Science and Technology, Shandong University, Qingdao, China
- 5Chinese Academy of Geological Sciences, Beijing, China
- 6Faculty of Engineering, China University of Geosciences, Wuhan, Hubei, China
Saline groundwater is a valuable unconventional water resource in silty coastal zone, which contain complex and fragile sedimentary and hydrological environments that are widely distributed and vulnerable to human activities. Coastal groundwater salinization along the eastern coast of China since the late Pleistocene is thought to be a response to global environmental change. Previous studies have shown a spatial and temporal link between the development of saline groundwater and deposition of marine strata. However, groundwater salinization is becoming an increasing problem in coastal zone, and the hydrogeochemical characteristics and cause of the groundwater salinization or desalinization remain poorly understood. In this study, we compared the hydrogeochemical characteristics and relationships between saline groundwater and the sedimentary environment in two representative, muddy coastal zone of the Yellow Sea coast (YSC) and Bohai Sea coast (BSC). Our monitoring results show that the groundwater varies from fresh water, to brackish water, saltwater, and brine moving offshore of the BSC, with total dissolved solid (TDS) concentrations of 203–184,196 mg/L. In contrast, brine is absent from the YSC, where the TDS concentrations range from 280 to 41,690 mg/L. The relationships between δ2H and δ18O values indicate that freshwater–seawater–brine and freshwater–seawater mixing are the main processes leading to enrichment in TDS in the BSC and YSC, respectively. Based on the relationship between the major cations, Cl−, and Cl/Br ratios, the results show that the groundwater salinity is primarily due to saltwater intrusion and secondly to water–rock interactions (i.e., evaporitic salt dissolution and cation exchange). The regression curves on plots of Na–Cl and (Ca+Mg)–(CO3+SO4) have negative slopes (−0.33 and −0.34, respectively) for the groundwater samples from the BSC and YSC, indicating the Na in groundwater exchange for Ca due to seawater or residual seawater intrusion. The saline groundwater (or brine) samples with reverse ion exchange indicate that seawater or residual seawater has invaded the aquifer, and in which Na+ is replacing Ca2+ and Mg2+. These results enhance our understanding of the formation and evolution of saline groundwater, and provide insights into groundwaters in other silty coastal zones.
1 Introduction
The interactions between the ocean, land and human activities can affect muddy groundwater environments, making these areas more vulnerable to geological disasters (Wang et al., 2020). Coastal zone can be classified into five types: sandy, silty, bedrock, biological, and artificial coasts (Yang, 2004). Silty coasts are characterized by silty clay and clayey silt (Wang et al., 2003), and have been widely developed and utilized due to their broad expanse and flat terrain. Frequent changes in global sea level have led to the formation of saline groundwaters in silty coastal zone (Han et al., 2014; Larsen et al., 2017). Due to population growth and industrialization, water resources in silty coastal zone are becoming increasingly scarce and urgently needed. Understanding the formation and evolution of saline groundwater might contribute to preventing seawater intrusion and the protection of scarce freshwater resources.
Silty coastal zone has complex hydrogeological environments due to the presence of various types of groundwater, such as freshwater (total dissolved solids [TDS] < 1 g/L), brackish water (TDS: 1–3 g/L), saline groundwater (TDS: 3–50 g/L), and brine (TDS ≥ 50 g/L). For example, the groundwater varies from freshwater to brine on the coast of the Louisiana Gulf in the USA (Hanor and Mercer, 2010), Rhine-Meuse delta in the Netherlands (Griffioen et al., 2016), and the Burdekin delta in Australia (Fass et al., 2007). The complex sedimentary environment, as well as the variable hydrodynamic and hydrogeochemical conditions of silty coastal zone, hinder studies of the salinization mechanisms of groundwater (Cary et al., 2013). The salinization mechanisms of coastal groundwater include: 1) modern seawater intrusion (Reilly and Goodman, 1985; Andersen et al., 2005; Werner et al., 2013); 2) marine transgressive–regressive events associated with ancient sea water intrusion (Kooi et al., 2000; Santucci et al., 2016; Li et al., 2021); (3)evaporitic salt infiltration (Guan et al., 2010; Yang et al., 2016); 4) water–rock interactions (Merchán et al., 2015; Sun et al., 2023); 5) pollution by industrial and domestic waste water (Perrin et al., 2011; Cary et al., 2013); 6) extreme weather events such as storm surges and tsunamis (Yu et al., 2016).
Despite numerous studies of saline groundwater, the formation mechanisms of different types of groundwater remain unclear. Currently, the methods used for identifying the source and migration of water–salt include mainly (bio) geochemical analysis (major and trace elements, microorganisms, and isotopes ratios) and numerical simulations. Although (bio) geochemical analysis can provide information regarding the source, supply, and migration of water and salt, it cannot accurately quantify the contribution from different sources or predict the evolution of groundwater under complex environmental conditions. In contrast, numerical simulation can quantify the hydrodynamic and hydrogeochemical changes on different spatial and temporal scales, but provide limited insights into the formation mechanisms of saline groundwater.
Saline groundwater occurs widely in eastern coastal areas of China, including Hebei, Shandong, and Jiangsu provinces, where groundwater has been utilized for centuries, with brine being used for salt production on the northern coast of Shandong Province (Han et al., 1996). Previous studies have investigated saline groundwaters in these silty coastal zones. Zhao et al. (1993) proposed that changes in the Holocene sea level controlled the distribution of saline groundwater beneath the Jiangsu alluvial plain. Zhang X. et al. (2017) found that deep saline groundwater beneath the Jiangsu alluvial plain was mainly formed by leaching of salt from ancient marine strata. Ge (2018) investigated the salinity sources in porewater from the Jiangsu alluvial plain, and showed that the salinity was mostly derived from Holocene seawater, with the deep porewater salinity due mainly to contributions from late Pleistocene seawater. By simulating the porewater migration, Li et al. (2021) showed that saltwater in weak permeable layers was the potential cause of groundwater salinization.
The Bohai Sea coast (BSC) has more types of groundwater and a more complex evolution as compared with the Yellow Sea coast (YSC). Sun et al. (2006); Sun (2007) found that overexploitation of groundwater had caused various modes of seawater intrusion in BSC, including facial, stripped, veined, and leakage types (Zuo and Wan, 2006; Fei et al., 2009; Shi et al., 2014). Along the southern coast of Laizhou Bay, previous studies have focused mainly on the sedimentary environment (Han et al., 1999; Li et al., 1999; Liu et al., 2003), water–rock interactions (Zhang and Chen, 1996; Sun et al., 2023), and saltwater (brine) intrusion (Han D. et al., 2011; Han F. et al., 2011; Han et al., 2014). Early studies considered that the coastal brine was formed by the evaporation and concentration of ancient seawater stranded by marine transgressive–regressive events since the late Pleistocene (Han and Wu, 1982; Zhang and Chen, 1996; Wang et al., 2003). However, further research has questioned this hypothesis (Han et al., 1996; Xu et al., 2011; Yang et al., 2016; Sun et al., 2023). Although the previous studies linked the source of the saltwater to marine transgressive–regressive events, the proposed formation mechanisms vary widely. From the perspective of regional water circulation, there have been few studies of the saline groundwater (brine) characteristics and salinization, and most researches on groundwater salinization is focused on the two end-members mixing model of saline–fresh water, the mixing of the three end-members (freshwater–seawater–brine) and the hydrogeochemical evolution of saline groundwater remain poorly understood (Han D. et al., 2011; Han F. et al., 2011; Han et al., 2014; Hu et al., 2015; Dang, et al., 2022; Sun et al., 2023). Given this shortcoming, it is necessary to better understand the formation and evolution of saline groundwater in silty coastal zone (Gao et al., 2015).
Our research objectives were as follows: 1) to clarify the relationship between saline groundwater and the sedimentary environment in silty coastal zone; 2) to identify the water and salt sources in saline groundwater and the controlling factors on the hydrochemical evolution; and 3) to compare the formation and evolution of saline groundwater in the YSC and BSC.
2 Materials and methods
2.1 Regional setting
The distribution of saline groundwater in the silty coast of eastern China is mainly concentrated in coastal zone of Hebei, Tianjin, Shandong, and Jiangsu Provinces (Figure 1). These regions have an elevation below 30 m and a slope ratio <0.13‰. The climate is influenced by monsoon variations in BSC, with an average annual temperature of 8°C–12°C, showing warm-dry conditions and high evaporation rates. Specifically, the annual average evaporation is 1–4 times higher than annual average precipitation (Sun et al., 2006). The annual average precipitation of YSC is 1,050 mm, and the annual average evaporation is 951–1,120 mm (Li et al., 2021).
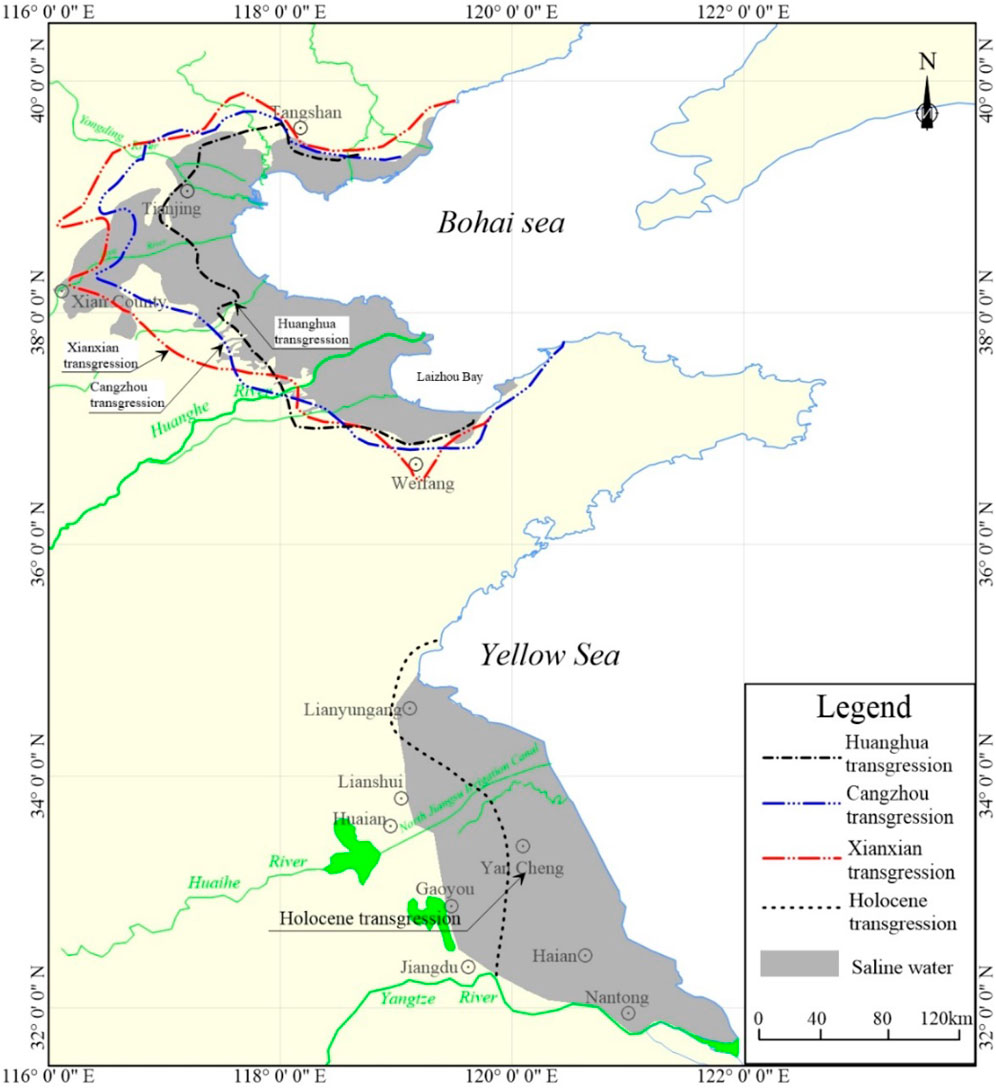
FIGURE 1. Saline groundwater and three marine transgressions in coast of eastern China (Modified from Xue, 2009; Hou et al., 2021).
The North China depression belongs to the China-North Korea Paraplatform, while the Lower Yangtze depression falls within the Yangtze Paraplatform, as determined by their geological structures. The secondary depressions and uplifts are predominantly oriented in the NE direction. Since the Cenozoic period, these structures have been in a decline stage, while the surrounding mountains ranges have experienced relatively upward movement (Li, 1986). Due to extensive sedimentation, all uplifts and depressions have been buried by thick layers of sedimentary formations. In particular, the Quaternary strata are characterized by a thickness of 500–600 m. The major coastal rivers in the study area including the Yellow, Huaihe, Haihe and Luanhe rivers.
The sedimentary environment of saline groundwater in the silty coast is complex, and microscopic hydrogeochemical behavior occurs at the interfaces of seawater/saltwater, saltwater/brine, and freshwater/saltwater. Such behavior could lead to changes in the chemical type of groundwater, which in turn affects the intensity of groundwater circulation. The saline groundwater significantly impedes normal industrial and agricultural production. Conversely, the overexploited fresh groundwater has changed the hydrodynamic conditions of local groundwater. This has resulted in saltwater intrusion into freshwater aquifers, causing geological disasters such as groundwater salinization and soil secondary salinization, which seriously threatens the sustainable development of the coastal ecological environment.
2.2 Sedimentary environment
The BSC and YSC have been the sites of deposition of three marine sediment layers since the last glacial period (Qing and Zhao, 1985). These layers are closely linked to changes in global sea level, and correspond to the Cangzhou (Qp31; 110–70 ka B.P.), Xianxian (Qp33; 40–25 ka B.P.), and Huanghua (Qh2, 7–2.5 ka B.P.) transgressions. The general distribution and thickness of each sediment layer are provided in Table 1. The largest transgressive event occurred during the middle Holocene, with the boundary extending to the west coast of Yellow Sea (i.e., the northern Jiangsu alluvial plain). Coeval ancient or invasive seawater is stored in these alternating continental–marine facies sedimentary strata. The sedimentary environment along these silty coasts varies greatly due to the combined effects of tectonic activity, sea level rise, topography, and paleoclimate. The sedimentary environment has undergone major changes, especially during the early stages of the regressions. As such, the groundwater consists of multiple types and is stratified.
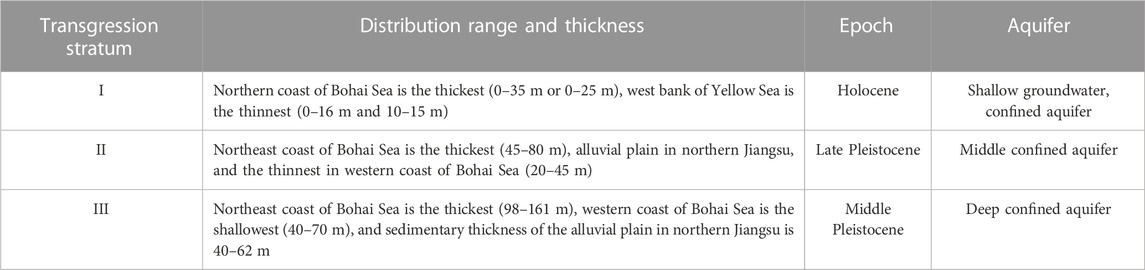
TABLE 1. Distribution range and characteristics of transgression stratum in the Yellow Sea and Bohai Sea (modified by Li, 1986).
The distribution of saline groundwater along the eastern coast of China is mainly controlled by the topography. Groundwater flow onto alluvial fans at mountain fronts penetrates into the relatively low alluvial plain and finally flows into the river delta. The northern saltwater area is mainly the Luxi Plain, and the southern saltwater area is the low hilly area from southwest Shandong Province to the Yangtze River, which is mainly the alluvial plain in northern Jiangsu Province. Although saltwater in northern Jiangsu Province is not connected with that in the northern China, there is exchange of saline groundwater between the saltwater in northern Jiangsu Province and that in northern China due to the effects of former Yellow River and geological structure.
In Cangzhou in Hebei Province, a saltwater layer along the Binhai–South Canal is sourced from the Holocene to lower Pleistocene strata, and the salt water to the west of the South Canal–Ziya River occurs only in the Holocene and Upper Pleistocene strata. The depth of the saltwater layer ranges from 20 to 280 m. Based on the burial conditions and hydraulic properties, the groundwater is typically divided into shallow and deep saline groundwaters. The shallow groundwater is <60 m deep and mostly Holocene saltwater. The deep groundwater is >60 m deep and mostly late middle Pleistocene saltwater (Li, 1986). From the land to ocean, the groundwater exhibits obvious zonation: HCO3–Ca·Mg → HCO3–Ca·Na → HCO3·Cl–Na·Ca → Cl·SO4–Na → Cl–Na. The shallow groundwater beneath the alluvial plain in northern Jiangsu Province is mainly saltwater. The deeply confined aquifer was mainly recharged by precipitation during the late Pleistocene cold periods, and the shallow groundwater was mainly recharged by precipitation during the Holocene warm period. The salinity is mainly derived from ancient residual seawater that has undergone strong evaporation.
The distribution of saline groundwater is closely associated with coastline changes. For example, since the Holocene transgression, there have been several high sea-level stands (Figure 2). A comparison of the changes of the saline groundwater with the shoreline, seawater intrusion, and stratigraphy (Table 1) shows that the saltwater distribution is related to changes in the seawater intrusion and coastline. For example, the Yellow and Bohai Seas expanded to the surrounding inland plains. The distribution of saltwater (and marine transgressive strata) is greatest in the western BSC and YSC, and smallest along the northeast BSC and YSC (Figure 2). From the mountain front → central alluvial plain → river delta, the saltwater layer occurs in Holocene → late Pleistocene to Holocene → lower Pleistocene to Holocene strata, due to the temporal relationship between seawater intrusion and transgression. There is also a strong correlation between the saltwater chemistry and transgressive strata. From the ocean to land, the hydrochemical characteristics vary as follow: Cl–Na → SO4·Cl–Na·Mg → HCO3–Ca·Na. The TDS concentrations also change from high to low.
2.3 Dating and methods
A total of 139 samples were collected (Figure 3), including 124 groundwater samples, 12 surface water samples, one local rainwater sample, and two seawater samples, during four sampling campaigns from October 2016 to June 2020. Groundwater samples were divided into shallow groundwater samples (depth < 30 m) and deep groundwater samples (depth > 30 m), which were obtained from unconfined and confined aquifers, respectively. The surface water included eight samples from the YSC and four samples from the BSC.
All samples were filtered through a 0.22 μm filtration membrane, cationic analysis samples are pretreated with a 6 mmol/L nitrate water at pH < 2. Stable isotope (δ2H, δ18O) testing samples were filled with 10 mL brown glass bottle, and the hydrochemical test samples were filled with 500 mL plastic bottle.
Groundwater sampling depths and pH values were measured in situ using Eureka Manta+ (Water quality Multiprobe Sonde). The concentrations of K+, Na+, Ca2+, Mg2+, and Br−ions were measured using inductively coupled plasma analysis (ICAP–7400), while SO42– and Cl−ions were determined using ion chromatography (ICS–600). The HCO3− concentrations of samples were measured using titration. The TDS is obtained by evaporating the water at 105°C–110°C and weighing the total amount of the resulting dried residue, or is calculated by all dissolved component concentrations summed minus one-half of the bicarbonate. The water samples were tested at the Experimental & Testing Center of Marine Geology, Ministry of Natural Resources, China, using high-temperature pyrolysis-isotope ratio mass spectrometry. The values of δ18O and δ2H were calculated with respect to Vienna Standard Mean Ocean Water (VSMOW), and the uncertainties in δ2H and δ18O were ± 1.0‰ and ± 0.2‰, respectively.
At the same time, this study collected 239 hydrochemical data and 172 isotope data (δ18O and δ2H) in BSC and YSC published papers (Han et al., 1996; Xue et al., 2000; Han D. et al., 2011; Han F. et al., 2011; Han et al., 2014; Du et al., 2015; Yang et al., 2016; Zhang Y. et al., 2017).
3 Results
3.1 Hydrochemistry
The TDS concentrations of groundwater in the BSC and YSC range from 204 to 184,196 mg/L and 280 to 41,690 mg/L, respectively. The distribution of groundwater chemical types included (Zhou, 2013): freshwater, brackish water, saline water, and brine, with a predominance of freshwater–brackish water–saline water–brine from land to ocean in the BSC, and freshwater–brackish water–saltwater in the YSC. As mentioned earlier, the hydrogeochemical types exhibit distinct zonation in the silty coasts. In the BSC, the freshwater is mainly HCO3–Ca, HCO3–Ca∙Mg, HCO3–Na; brackish water is HCO3–Na and Cl–Na; saltwater and brine is Cl–Na (Figure 4A). In the YSC, freshwater is HCO3–Ca and HCO3–Na, brackish water is HCO3–Na, HCO3–Mg, and Cl–Na, and saltwater is Cl–Na (Figure 4B).
Gibbs diagrams are frequently used to characterize the primary factors that influence the chemical composition of groundwater, including rainfall, mineral weathering, and evaporation (Rajmohan et al., 2021; Yang et al., 2021). Figure 5 illustrates that mineral weathering is the dominant control on freshwater chemistry. Mineral dissolution and precipitation can modify the cationic components during runoff, such as calcium feldspar weathering to kaolinite and sodium feldspar weathering to montmorillonite, resulting in a broad distribution range of (Na+K)/(Na+K+Ca) in fresh groundwater (Figure 5). With increasing TDS concentrations, (Na+K)/(Na+K+Ca) decreases in saline water, indicating that seawater intrusion has progressively become the main factor. Brine samples along the BSC locate in the upper right corner of the Gibbs diagram (Figure 5A), indicating that they are controlled by strong evaporative concentration (Xu et al., 2021).
3.2 Isotopes
Stable isotopes can record the processes of recharge, mixing, and evaporation in coastal groundwaters. The global meteoric water line (GMWL; δ2H = 8∙δ18O + 10) was taken from Craig (1961), and the local meteoric water line (LMWL; δ2H = 6.6 δ18O + 0.3) for the BSC is based on H–O isotope data for rainfall at Tianjin Station, which is located ∼100 km from the study area (IAEA/WMO, 2006). The LMWL (δ2H = 8.5∙δ18O + 17.7) for the YSC is based on data from Nanjing Station (IAEA, 2011) (Figure 6). For the BSC, H–O isotope data deviate to the right of the GMWL and LMWL (Figure 6A), indicating that atmospheric precipitation has undergone evaporation or mixing with other waters before entering the aquifer. Many seasonal rivers, such as the Mihe and Weihe rivers, flow into the Bohai Sea. Affected by evaporation, isotopic fractionation of the river water is likely to occur. Most of the freshwater and brackish water isotopic compositions are similar to those of river water, indicating groundwater is closely linked to surface rivers. The freshwater with stable isotopic compositions that plot at the bottom left of Figure 6 may be related to groundwater recharge under cold conditions during the late Pleistocene (Han D. et al., 2011; Han F. et al., 2011; Li et al., 2017; Dang et al., 2020).
Given that the retention time of underground brine along the BSC ranges from 2 to 7 ka B.P. (Han et al., 2014; Dang et al., 2022), the saline groundwater is likely to be due to infiltration of ancient seawater after evaporation and salt concentration during Holocene transgression. In the BSC, the saline groundwater samples have a wide range of δ2H and δ18O values, which plot mostly along a seawater and brine mixing line, indicative of the freshwater–seawater–brine end-members. Fresh groundwater from the YSC plots mainly near the GMWL and LWML (Figure 6B), and the isotopic composition is similar to that of rainwater, indicating the freshwater is mainly derived from atmospheric precipitation. With increasing TDS concentrations, the isotope values gradually become enriched, and the brackish water and saltwater samples plot along the local seawater mixing line (Mao et al., 2020).
Figures 7, 8 show the relationships between Na+, Mg2+, Ca2+, SO42-, Br−, and Cl−concentrations in the water samples. On plots of Na–Cl (Figure 7A), Mg–Cl (Figure 7B), and Br–Cl (Figure 8A), the saltwater samples plot near the seawater–freshwater mixing line, and saltwater with higher TDS concentrations than seawater plots near the seawater evaporation line, indicating that the salinity is related to seawater and residual seawater after evaporation. The Cl/Br ratio of seawater is 655 (Siemann, 2003), and the Cl/Br ratio of terrestrial freshwater is generally <<655. The Cl/Br ratio is often used to identify the salinity source of coastal groundwater (Liu et al., 2017). As shown in Figure 8B, some of the saltwater and brine samples deviate from the seawater Cl/Br ratio line. NaCl precipitation occurs after seawater evaporation reaches halite saturation, and Br is not easy to precipitate, due to its stable chemical properties; thus, the Cl/Br ratio of residual seawater decreases (Figure 8B). However, during recharge by atmospheric precipitation, evaporitic salts in marine strata are easily dissolved into groundwater, resulting in increasing Cl/Br ratios (Cartwright et al., 2004; 2006). In plots of SO4–Cl and Ca–Cl (Figures 7C, D), the water samples deviate from mixing or evaporation lines, particularly the Cl/Br ratios of the freshwater and brackish water samples, which deviate significantly from the seawater ratio (Figure 8B). This indicates that mixing with seawater or concentrated saltwater, in combination with hydrogeochemical effects such as mineral precipitation–dissolution, and ion exchange may have modified the groundwater composition during its formation and evolution.
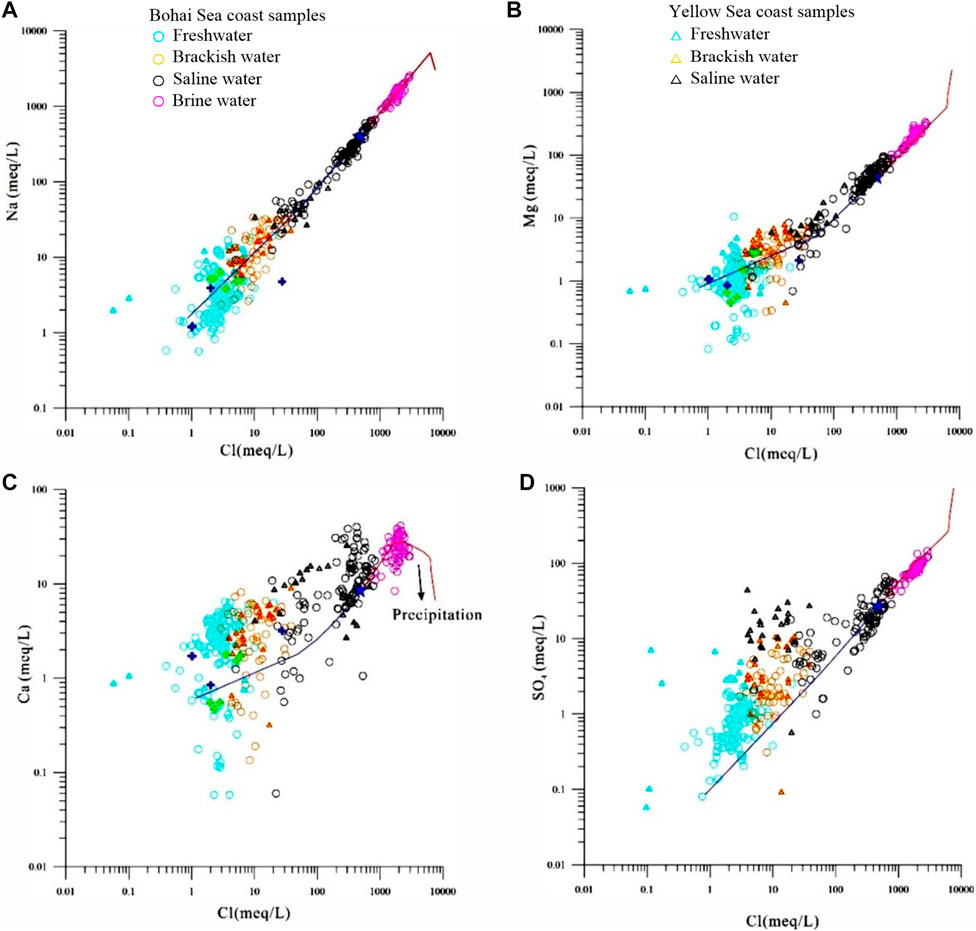
FIGURE 7. Relationship between major ions (A is Na+, B is Mg2+,C is Ca2+, D is SO42−) and chloride ions in various water samples (blue is simulation mixing curve of seawater and fresh water, and the red is evaporation line of seawater in BSC).
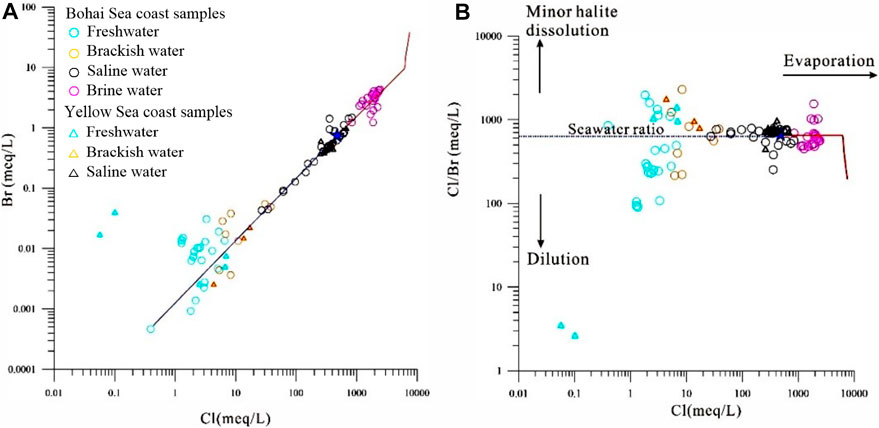
FIGURE 8. Correlation diagram of bromine and chlorine in underground water samples [(A) Bromine and chlorine, (B) Bromine/chlorine and chlorine).
4 Discussion
4.1 Hydrochemical process
In general, Ca dominates terrestrial groundwater, and the Ca/Mg ratio is >1 (Abu-alnaeem et al., 2018). Magnesium in saline groundwater is only exceeded in concentration by Cl and Na, and the Ca/Mg ratio is much lower than that of terrestrial freshwater. Due to seawater intrusion, groundwater Ca/Mg ratios will change significantly. Most of the Ca/Mg ratios are >1 in the fresh groundwater samples (Figure 9A), indicative of mineral weathering and groundwater recharge and runoff. The dissolution of gypsum increases the Ca and SO4 contents, which also explains why the freshwater and brackish water samples are located above the seawater mixing line in plots of SO4–Cl and Ca–Cl (Figures 7C, D); Weathering of silicate minerals such as plagioclase means that some freshwater samples plot above the 1:1 line of Ca-containing minerals (gypsum, calcite, and mica) (Figure 9B). With increasing Cl contents, the Ca/Mg ratios of the brackish water and saltwater samples approach that of seawater (Figure 9A), indicating seawater intrusion. The Ca/Mg ratios of the saltwater and brine samples are less than that of seawater. The salinity of the brine reflects that of residual seawater after evaporation. When the seawater evaporated, Ca-containing minerals such as calcite and muscovite were precipitated (Wang, 2021). The Ca2+ in the residual seawater was consumed, and the Ca/Mg ratios of the residual seawater decreased.
When the salinity changes significantly, such as aquifer desalination or seawater intrusion, there may be ion exchange between the groundwater and clay minerals in the aquifer medium (Eq. 1), and a reverse ion exchange (RIE) process may also occur (Eq. 2) (Nogueira et al., 2019; Rajmohan et al., 2021).
The relationships between Na–Cl and (Ca+Mg)–(HCO3+SO4) can well be indicative of cation exchange. If cation exchange is the cause of enrichment of Na+ or Ca2+ (Mg2+) in groundwater, then the water samples should plot near the equilibrium line with a slope of about −1 (Xiao et al., 2014). As shown in Figure 10A, the slopes of the groundwater regression curves are −0.33 and −0.34 for the BSC and YSC, respectively. The saltwater (brine) samples are located in the RIE field, indicating that seawater or residual seawater has infiltrated down into the aquifer and undergone exchange between Na+ (K+) and Ca2+ (Mg2+) via interactions with clay during seawater intrusion. This also explains why the (Ca+Mg)/(HCO3+SO4) value is >1 for the saltwater and brine samples. The slopes of the regression curves for the freshwater and brackish water samples are −0.69 and −0.63 in the BSC and YSC, respectively (Figure 10B). Most water samples plot in the cation exchange area, indicating that the freshwater and brackish water samples have undergone cation exchange processes. After a regressive event, freshwater (i.e., river water and atmospheric precipitation) can recharge a saltwater aquifer, and Ca2+ (Mg2+) is exchanged with the Na+ adsorbed in the marine sediments, enriching Na+ in the groundwater and resulting in Ca2+ or Mg2+ consumption. The (Ca+Mg)/(HCO3+SO4) values of the freshwater and slightly saline water samples are <1 (Figure 9B).
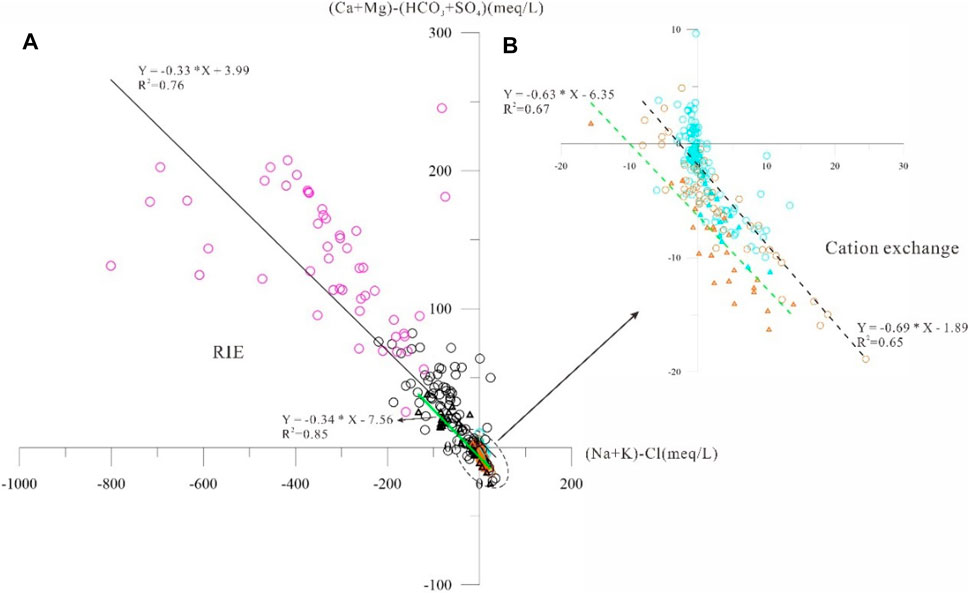
FIGURE 10. Relationship between (Na–Cl) and (Ca+Mg)–(HCO3+SO4) in coastal groundwater [(A). all water samples, (B). fresh and brackish water samples].
4.2 Groundwater formation mechanisms
Douglas et al. (2000) and Han and Currell (2018) proposed that coastal multi-type groundwaters are part of a complex system formed in numerous stages during ocean–land interactions, with mixing between two or three end-members. Chloride ions and oxygen isotopes in groundwater do not participate in reaction processes and can be used to examine mixing processes and saltwater (brine) evolution. Figure 11A shows the relationship between δ18O values and Cl−contents of the various BSC groundwater samples. Seawater–freshwater and brine-freshwater mixing lines were constructed based on conservation of mass. The saltwater and brine samples plot between the two mixing lines, and the water samples with a lower salinity than seawater plot near the seawater–freshwater mixing line. With increasing Cl−, the groundwater samples gradually approach the saltwater–freshwater mixing line.
Since the late Pleistocene, the study area has experienced marine transgression–regression events in the silty coastal zone (Gao et al., 2016). Saltwater intrusion can occur in sand barrier–lagoon facies and other places, and the groundwater was salinized by seawater–freshwater mixing. As the sea level became stable, rivers began to prograde towards the ocean. Due to river sand transport and waves, the sand barrier–lagoon environment changed under the mild–arid paleoclimatic conditions, especially at the delta front. After evaporation, the concentrated saltwater in this semi-closed environment infiltrated the aquifer, and underwent cation exchange and mixing, which increased its salinity. The “seawater-brine-freshwater” end-members mixing occurred in this groundwater aquifer (Dang et al., 2022). Due to a marine regression, lateral infiltration of river waters and fresh groundwater is considered to have been the main saltwater dilution process in the Vietnam delta (Kwong and Jiao, 2016; Larsen et al., 2017; Van Lam et al., 2019). Due to the shallow channels submerged by seawater, frequent flooding events help to wash away ancient saltwater. However, due to mixing with river water and groundwater, mineral dissolution (gypsum) and RIE can occur. The brackish and freshwater samples of the present study have low Cl−contents like the river water samples (Figure 11A), and similar stable isotopic composition.
A plot of δ18O versus Cl−in the YSC shows saltwater plots mainly near the seawater mixing line, and brackish water and freshwater plot close to the river water samples (Figure 11B), indicating that the groundwater has undergone similar salinization and dilution processes. Brine has not yet been documented in the YSC. During a regression, parts of the YSC formed barrier–lagoons (Zhang et al., 2010). However, a large amount of freshwater (Yellow and Yangtze rivers) enters the ocean, which has promoted the transformation of lagoons into freshwater lakes (Ling, 2005). At the same time, the southern Yangtze River provides the material that has formed the tidal sand formations in the YSC (Li et al., 1998). Tidal sands are conducive to cation exchange (Figure 10B). In general, the groundwater is saltwater, but it does not have a high salt concentration due to the lack of stable and semi-enclosed lagoonal evaporitic environments in the YSC.
5 Conclusion
Saline groundwater occurs in complex and fragile sedimentary environments, and is widely distributed and susceptible to human activities in silty coastal zone. We investigated saline groundwaters in the BSC and YSC. Since the late Pleistocene, there have been three or more large-scale marine transgressive–regressive events along the eastern coast of China. The groundwater composition was found to be controlled by mineral weathering, seawater intrusion, and residual seawater that formed after evaporation. The dissolution of evaporitic salts in marine strata was also a contributor to groundwater salinity. Three end-member mixing (seawater, concentrated salt water (brine), freshwater) occurs in the BSC, whereas saline groundwater is mainly formed by two end-member mixing (seawater and freshwater) in the YSC. Brine has not yet been observed in the YSC, which may be due to the large amount of freshwater and lack of semi-enclosed environments, such as barrier–lagoon systems. The study highlights the complexity of groundwater evolution in silty coastal zones due to ocean-land interactions and human activities. Overall, this study provides valuable insights into the complex geochemical and hydrological processes that govern saline groundwater occurrence in the silty coastal zone. The findings have important implications for managing and conserving freshwater resources in vulnerable coastal zones.
Data availability statement
The datasets presented in this article are not readily available because the authors do not have permission to share data. Requests to access the datasets should be directed to Z2FvbXNoNjZAc29odS5jb20=.
Author contributions
MG performed “Writing–Original Draft and Review”; QS contributed to “Writing–Editing”; XD contributed to “Writing–Editing”; GH contributed to “Investigation”; FG contributed to “Software”; ZL helped perform “Methodology”; XC contributed to “Visualization”. GZ contribution to “Software”. All authors contributed to the article and approved the submitted version.
Funding
This study was financially supported by the National Natural Science Foundation of China (U2106203, 41977173, and 42076070), the National Geological Survey Project of China Geology Survey (No. DD20221775, DD20211401), the China-ASEAN maritime cooperation fund (Cooperative researches on the marine geo-environments and geo-hazards in the Yangtze River and the Red River deltas), and National Natural Science Foundation Shandong Province of China (ZR2020MD079).
Conflict of interest
The authors declare that the research was conducted in the absence of any commercial or financial relationships that could be construed as a potential conflict of interest.
Publisher’s note
All claims expressed in this article are solely those of the authors and do not necessarily represent those of their affiliated organizations, or those of the publisher, the editors and the reviewers. Any product that may be evaluated in this article, or claim that may be made by its manufacturer, is not guaranteed or endorsed by the publisher.
References
Abu-Alnaeem, M. F., Yusoff, I., Ng, T. F., Alias, Y., and Raksmey, M. (2018). Assessment of groundwater salinity and quality in Gaza coastal aquifer, Gaza Strip, Palestine: An integrated statistical, geostatistical and hydrogeochemical approaches study. Sci. Total Environ. 615, 972–989. doi:10.1016/j.scitotenv.2017.09.320
Andersen, M. S., Nyvang, V., Jakobsen, R., and Postma, D. (2005). Geochemical processes and solute transport at the seawater/freshwater interface of a sandy aquifer. Geochimica Cosmochimica Acta 69 (16), 3979–3994. doi:10.1016/j.gca.2005.03.017
Cartwright, I., Weaver, T. R., and Fifield, L. K. (2006). Cl/Br ratios and environmental isotopes as indicators of recharge variability and groundwater flow: An example from the southeast murray basin, Australia. Chem. Geol. 231, 38–56. doi:10.1016/j.chemgeo.2005.12.009
Cartwright, I., Weaver, T. R., Fulton, S., Nichol, C., Reid, M., and Cheng, X. (2004). Hydrogeochemical and isotopic constraints on the origins of dryland salinity, Murray Basin, Victoria, Australia. Appl. Geochem. 19 (8), 1233–1254. doi:10.1016/j.apgeochem.2003.12.006
Cary, L., Casanova, J., Gaaloul, N., and Guerrot, C. (2013). Combining boron isotopes and carbamazepine to trace sewage in salinized groundwater: A case study in cap bon, Tunisia. Appl. Geochem. 34, 126–139. doi:10.1016/j.apgeochem.2013.03.004
Craig, H. (1961). Standard for reporting concentrations of deuterium and oxygen-18 in natural waters. Science 133 (3467), 1833–1834. doi:10.1126/science.133.3467.1833
Dang, X., Gao, M., Wen, Z., Hou, G., Jakada, H., Ayejoto, D., et al. (2022). Saline groundwater evolution in the Luanhe river delta (China) during the Holocene: Hydrochemical, isotopic, and sedimentary evidence. Hydrology Earth Syst. Sci. 26 (5), 1341–1356. doi:10.5194/hess-26-1341-2022
Dang, X., Gao, M., Wen, Z., Jakada, H., Hou, G., and Liu, S. (2020). Evolutionary process of saline groundwater influenced by palaeo-seawater trapped in coastal deltas: A case study in Luanhe river delta, China. Coast. Shelf Sci. 244, 106894. doi:10.1016/j.ecss.2020.106894
Douglas, M., Clark, I. D., Raven, K., and Bottomley, D. (2000). Groundwater mixing dynamics at a Canadian Shield mine. J. Hydrology 235 (1-2), 88–103. doi:10.1016/S0022-1694(00)00265-1
Du, Y., Ma, T., Chen, L., Shan, H., Xiao, C., Lu, Y., et al. (2015). Genesis of salinized groundwater in Quaternary aquifer system of coastal plain, Laizhou Bay, China: Geochemical evidences, especially from bromine stable isotope. Appl. Geochem. 59, 155–165. doi:10.1016/j.apgeochem.2015.04.017
Fass, T., Cook, P. G., Stieglitz, T., and Herczeg, A. L. (2007). Development of saline ground water through transpiration of sea water. Groundwater 45 (6), 703–710. doi:10.1111/j.1745-6584.2007.00344.x
Fei, Y., Zhang, Z., Song, H., Qian, Y., Chen, J., and Meng, S. (2009). Discussion of vertical variations of saline groundwater and mechanism in North China Plain. Water Resour. Proctection 25 (6), 3–10. (in Chinese).
Gao, M., Hou, G., and Guo, F. (2016). Conceptual model of underground brine formation in the silty coast of Laizhou Bay, Bohai Sea, China. J. Coast. Res. 74, 157–165. doi:10.2112/SI74-015.1
Gao, M., Zheng, Y., Liu, S., Wang, S., Kong, X., Zhao, J., et al. (2015). Paleogeographic condition for origin of underground brine in southern coast of Laizhou Bay, Bohai Sea. Geol. Rev. 61 (2), 393–400. (in Chinese).
Ge, Q. (2018). The formation and Geochemical evolution of porewater in the coastal clay-rich aquitards-a case study in north Jiangsu coastal plain. Doctoral dissertation. China University of Geosciences. (in Chinese).
Griffioen, J., Verweij, H., and Stuurman, R. (2016). The composition of groundwater in Palaeogene and older formations in The Netherlands. A synthesis. Neth. J. Geosciences 95 (3), 349–372. doi:10.1017/njg.2016.19
Guan, H., Love, A. J., Simmons, C. T., Makhnin, O., and Kayaalp, A. S. (2010). Factors influencing chloride deposition in a coastal hilly area and application to chloride deposition mapping. Hydrology Earth Syst. Sci. 14 (5), 801–813. doi:10.5194/hess-14-801-2010
Han, D., and Currell, M. J. (2018). Delineating multiple salinization processes in a coastal plain aquifer, northern China: Hydrochemical and isotopic evidence. Hydrology Earth Syst. Sci. 22 (6), 3473–3491. doi:10.5194/hess-22-3473-2018
Han, D., Kohfahl, C., Song, X., Xiao, G., and Yang, J. (2011a). Geochemical and isotopic evidence for palaeo-seawater intrusion into the south coast aquifer of Laizhou Bay, China. Appl. Geochem. 26 (5), 863–883. doi:10.1016/j.apgeochem.2011.02.007
Han, D. M., Song, X. F., Currell, M. J., Yang, J. L., and Xiao, G. Q. (2014). Chemical and isotopic constraints on evolution of groundwater salinization in the coastal plain aquifer of Laizhou Bay, China. J. Hydrology 508, 12–27. doi:10.1016/j.jhydrol.2013.10.040
Han, F., Wu, J., Zhang, Y., Lu, Y. m., Liu, L. l., Chen, Y. x., et al. (2011b). Melatonin ameliorates ischemic-like injury-evoked nitrosative stress: Involvement of HtrA2/PED pathways in endothelial cells. J. Nanjing Univ. Nat. Sci. 47 (3), 281–291. (in Chinese). doi:10.1111/j.1600-079X.2010.00838.x
Han, M., Zhao, M., Li, D., and Cao, X. (1999). Study on the ancient channels and the relationship between the ancient channels and the sea (salt) water intrusion of the south coastal plain of Laizhou Bay. J. Nat. Disasters 8 (2), 73–80. (in Chinese).
Han, Y., Meng, G., and Wang, S. (1996). Quaternary underground brine in the coastal areas of the northern China. Beijing: Science Press. (in Chinese).
Han, Y., and Wu, H. (1982). The origin of underground brine in the coastal plain of Laizhou Bay. Geol. Rev. 28 (2), 126–131. (in Chinese).
Hanor, J. S., and Mercer, J. A. (2010). Spatial variations in the salinity of pore waters in northern deep water Gulf of Mexico sediments: Implications for pathways and mechanisms of solute transport. Geofluids 10 (1-2), 83–93. doi:10.1111/j.1468-8123.2009.00271.x
Hou, G., Gao, M., Dang, X., and Chen, G. (2021). Water and salt sources and salinization of shallow saline groundwater in the coastal area of Yancheng, Jiangsu. Mar. Geol. Quat. Geol. 41 (4), 48–59. (in Chinese).
Hu, Y., Li, H., Li, Y., Shi, P., Yang, J., Hu, Z., et al. (2015). Hydrogeochemical recognization of seawater intrusion process at the typical profile in Laizhou Bay. Geol. Surv. Res. 38 (1), 41–50. (in Chinese).
IAEA (International Atomic Energy Agency) (2011). Global network of isotopes in precipitation. Available at: http://www-naweb.iaea.org/napc/ih/IHS_resources_gnip.ht-ml.
IAEA/WMO: Global Network of Isotopes in Precipitation (2006). Global network of isotopes in precipitation. The GNIP database. Vienna. Available at: http://www-naweb.iaea.org/napc/ih/IHS_resources_gnip.html.
Kooi, H., Groen, J., and Leijnse, A. (2000). Modes of seawater intrusion during transgressions. Water Resour. Res. 36 (12), 3581–3589. doi:10.1029/2000WR900243
Kwong, H. T., and Jiao, J. J. (2016). Hydrochemical reactions and origin of offshore relatively fresh pore water from core samples in Hong Kong. J. Hydrology 537, 283–296. doi:10.1016/j.jhydrol.2016.03.050
Larsen, F., Tran, L. V., Van Hoang, H., Tran, L. T., Christiansen, A. V., and Pham, N. Q. (2017). Groundwater salinity influenced by Holocene seawater trapped in incised valleys in the Red River delta plain. Nat. Geosci. 10 (5), 376–381. doi:10.1038/ngeo2938
Li, C., Zhang, J., and Yang, S. (1998). Characteristics and paleoenvironmental evolution of onshore tidal sandbodies in North Jiangsu Plain. Sci. China 28 (5), 418–424. (in Chinese).
Li, D., Han, M., Zhao, M., and Jiang, A. (1999). A study of the shallow-buried paleochannel zones and their relations with sea(salt)-water intrusion on the south coast plain of Laizhou Bay. Acta Oceanol. Sin. 21 (6), 64–71. (in Chinese).
Li, F. (1986). Discussion on underground saline water and sea intrusion in coastal plain of Yellow Sea and Bohai Sea. Acta Oceanol. Sin. 8 (4), 456–465. (in Chinese).
Li, J., Gong, X., Liang, X., Liu, Y., Yang, J., Meng, X., et al. (2021). Salinity evolution of aquitard porewater associated with transgression and regression in the coastal plain of Eastern China. J. Hydrology 603, 127050. doi:10.1016/j.jhydrol.2021.127050
Li, J., Liang, X., Jin, M., Yang, J., Ma, B., and Ge, Q. (2017). Origin and evolution of aquitard porewater in the Western coastal plain of Bohai Bay, China. Groundwater 55 (6), 917–925. doi:10.1111/gwat.12590
Ling, S. (2005). Study on models of the changes about sheyang lake in historical period. J. Chin. Hist. Geogr. 20 (3), 73–79. (in Chinese).
Liu, E., Zhang, Z., Shen, J., and Yang, L. (2003). Sedimentary characteristic of Weihe palaeochannel since late Pleistocene and their control to modern salt-water intrusion. Geol. J. China Univ. 9 (1), 47–53. (in Chinese).
Liu, S., Tang, Z., Gao, M., and Hou, G. (2017). Evolutionary process of saline-water intrusion in Holocene and late Pleistocene groundwater in southern Laizhou Bay. Sci. Total Environ. 607, 586–599. doi:10.1016/j.scitotenv.2017.06.262
Mao, C., Tan, H., Song, Y., and Rao, W. (2020). Evolution of groundwater chemistry in coastal aquifers of the Jiangsu, east China: Insights from a multi-isotope (δ2H, δ18O, 87Sr/86Sr, and δ11B) approach. J. Contam. Hydrology 235, 103730. doi:10.1016/j.jconhyd.2020.103730
Merchán, D., Auqué, L. F., Acero, P., Gimeno, M. J., and Causapé, J. (2015). Geochemical processes controlling water salinization in an irrigated basin in Spain: Identification of natural and anthropogenic influence. Sci. Total Environ. 502, 330–343. doi:10.1016/j.scitotenv.2014.09.041
Nogueira, G., Stigter, T. Y., Zhou, Y., Mussa, F., and Juizo, D. (2019). Understanding groundwater salinization mechanisms to secure freshwater resources in the water-scarce city of Maputo, Mozambique. Sci. Total Environ. 661, 723–736. doi:10.1016/j.scitotenv.2018.12.343
Perrin, J., Mascré, C., Pauwels, H., and Ahmed, S. (2011). Solute recycling: An emerging threat to groundwater quality in southern India. J. Hydrology 398 (1-2), 144–154. doi:10.1016/j.jhydrol.2010.12.024
Qing, W., and Zhao, S. (1985). The sedimentary model and quaternary transgression in the continental shelf of China. Quat. Sci. 01, 27–34.
Rajmohan, N., Masoud, M. H., and Niyazi, B. A. (2021). Impact of evaporation on groundwater salinity in the arid coastal aquifer, Western Saudi Arabia. Catena 196, 104864. doi:10.1016/j.catena.2020.104864
Reilly, T. E., and Goodman, A. S. (1985). Quantitative analysis of saltwater-freshwater relationships in groundwater systems—a historical perspective. J. Hydrology 80 (1-2), 125–160. doi:10.1016/0022-1694(85)90078-2
Santucci, L., Carol, E., and Kruse, E. (2016). Identification of palaeo-seawater intrusion in groundwater using minor ions in a semi-confined aquifer of the Río de la Plata littoral (Argentina). Sci. Total Environ. 566, 1640–1648. doi:10.1016/j.scitotenv.2016.06.066
Shi, J., Li, G., Liang, X., Chen, Z., Shao, J., and Song, X. (2014). Evolution mechanism and control of groundwater in the North China plain. Acta Geosci. Sin. 35 (5), 8–18. (in Chinese).
Siemann, M. G. (2003). Extensive and rapid changes in seawater chemistry during the phanerozoic: Evidence from Br contents in basal halite. Terra 15 (1), 243–248. doi:10.1046/j.1365-3121.2003.00490.x
Sun, Q., Gao, M., Wen, Z., Hou, G., Dang, X., Liu, S., et al. (2023). Hydrochemical evolution processes of multiple-water quality interfaces (fresh/saline water, saline water/brine) on muddy coast under pumping conditions. Sci. Total Environ. 857, 159297. doi:10.1016/j.scitotenv.2022.159297
Sun, X. (2007). Sustainable utilization study of groundwater resources in Circum-Bohai-Sea region. Doctoral dissertation. China University of Geosciences. (in Chinese).
Sun, X., Xu, J., Yang, Q., Shi, P., Zhong, X., Zhang, S., et al. (2006). Character and prevention strategies of sea (saline) water intrusion in Circum-Bohai-Sea region. Geol. Surv. Res. 29 (003), 203–211. (in Chinese).
Van Lam, N., Van Hoan, H., and Duc Nhan, D. (2019). Investigation into groundwater resources in southern part of the Red River’s delta plain, Vietnam by the use of isotopic techniques. Water 11 (10), 2120. doi:10.3390/w11102120
Wang, X. (2021). Equilibrium between dolomitization and dedolomitization of a global set of surface water samples: A new theoretical insight on the dolomite inorganic formation mechanism. Mar. Chem. 235, 104017. doi:10.1016/j.marchem.2021.104017
Wang, Y., Gan, Y., Deng, Y., and Xie, X. (2020). Land-ocean interactions and their eco-environmental effects in the coastal zone: Current progress and future perspectives. Bull. Geol. Sci. Technol. 39 (1), 1–10. doi:10.19509/j.cnki.dzkq.2020.0101
Wang, Z., Meng, G., and Wang, S. (2003). Geochemistry modeling of quaternary subsurface brine in the south coast of the Laizhou Bay, Bohai Sea. Mar. Geol. Quat. Geol. 23 (1), 49–53. (in Chinese).
Werner, A. D., Bakker, M., Post, V. E., Vandenbohede, A., Lu, C., Ataie-Ashtiani, B., et al. (2013). Seawater intrusion processes, investigation and management: Recent advances and future challenges. Adv. water Resour. 51, 3–26. doi:10.1016/j.advwatres.2012.03.004
Xiao, G., Yang, J., Hu, Y., Du, D., Xu, Q., Qin, Y., et al. (2014). Hydrogeochemical recognition of seawater intrusion processes in Yang River and Dai River coastal plain of Qinhuangdao city. Saf. Environ. Eng. 21 (02), 32–39. (in Chinese).
Xu, B., Yu, Z., Liu, D., Jiang, X., Chen, H., Yao, Q., et al. (2011). Study of formation of high dissolved uranium in underground brines at the south coast of Laizhou Bay. Periodical Ocean Univ. China 41 (11), 5. (in Chinese).
Xu, X., Xiong, G., Chen, G., Fu, T., Yu, H., Wu, J., et al. (2021). Characteristics of coastal aquifer contamination by seawater intrusion and anthropogenic activities in the coastal areas of the Bohai Sea, eastern China. J. Asian Earth Sci. 217, 104830. doi:10.1016/j.jseaes.2021.104830
Xue, C. (2009). Historical changes of coastlines on west and south coasts of Bohai Sea since 7000 a B.P. Sci. Geogr. Sin. 29 (2), 217–222. (in Chinese).
Xue, Y., Wu, J., Ye, S., and Zhang, Y. (2000). Hydrogeological and hydrogeochemical studies for salt water intrusion on the south coast of Laizhou Bay, China. Groundwater 38 (1), 38–45. doi:10.1111/j.1745-6584.2000.tb00200.x
Yang, F., Liu, S., Jia, C., Gao, M., Chang, W., and Wang, Y. (2021). Hydrochemical characteristics and functions of groundwater in southern Laizhou Bay based on the multivariate statistical analysis approach. Estuar. Coast. Shelf Sci. 250, 107153. doi:10.1016/j.ecss.2020.107153
Yang, Q., Wang, R., Xu, S., Li, W., Wang, Z., Mei, J., et al. (2016). Hydrogeochemical and stable isotopic characteristics of brine in Laizhou Bay. Geol. Rev. 62 (2), 343–352. (in Chinese).
Yu, X., Yang, J., Graf, T., Koneshloo, M., O'Neal, M. A., and Michael, H. A. (2016). Impact of topography on groundwater salinization due to ocean surge inundation. Water Resour. Res. 52 (8), 5794–5812. doi:10.1002/2016WR018814
Zhang, X., Miao, J., Hu, B. X., Liu, H., Zhang, H., and Ma, Z. (2017a). Hydrogeochemical characterization and groundwater quality assessment in intruded coastal brine aquifers (Laizhou Bay, China). Environ. Sci. Pollut. Res. 24, 21073–21090. doi:10.1007/s11356-017-9641-x
Zhang, Y., and Chen, H. (1996). Characteristics of sedimentary seawater and its formation environment in post-late Pleistocene strata on the south of Laizhou Bay. Acta Oceanol. Sin. 18 (6), 8–16. (in Chinese).
Zhang, Y., Fu, C., Mao, L., Gong, X., and Li, X. (2017b). Hydrochemical characteristic and formation mechanism of the groundwater in Yancheng, Jiangsu Province. Resour. Environ. Yangtze Basin 26 (4), 598–605. (in Chinese).
Zhang, Z., Xie, L., and Zhang, Y. (2010). Sedimentary records of the MIS3 marine transgression event in the North Jiangsu Plain, China. Quat. Sci. 30 (5), 883–891. (in Chinese).
Zhao, J., Liang, J., and Cai, S. (1993). Relationship between brackish water formation and water-bearing medium in northern Jiangsu alluvial Plain. Hydrogeology Eng. Geol. (03), 25–27. (in Chinese).
Zhou, X., Li, Y., Liu, S., Yang, Q., Su, X., Zhou, L., et al. (2013). Ultra-deep sequencing enables high-fidelity recovery of biodiversity for bulk arthropod samples without PCR amplification. Hydrogeology Eng. Geol. 40 (5), 4–10. (in Chinese). doi:10.1186/2047-217X-2-4
Keywords: silty coast, saline groundwater, hydrogeochemical characteristic, TDS, seawater intrusion
Citation: Gao M, Sun Q, Dang X, Hou G, Guo F, Liu Z, Chang X and Zhao G (2023) Hydrogeochemical characteristic and recognization of saline groundwater formation and evolution in silty coast of the Yellow Sea and Bohai Sea, Eastern China. Front. Earth Sci. 11:1186661. doi: 10.3389/feart.2023.1186661
Received: 15 March 2023; Accepted: 09 June 2023;
Published: 21 June 2023.
Edited by:
Ying Zhao, Ludong University, ChinaReviewed by:
Tianyuan Zheng, Ocean University of China, ChinaXuejing Wang, Tianjin University, China
Copyright © 2023 Gao, Sun, Dang, Hou, Guo, Liu, Chang and Zhao. This is an open-access article distributed under the terms of the Creative Commons Attribution License (CC BY). The use, distribution or reproduction in other forums is permitted, provided the original author(s) and the copyright owner(s) are credited and that the original publication in this journal is cited, in accordance with accepted academic practice. No use, distribution or reproduction is permitted which does not comply with these terms.
*Correspondence: Maosheng Gao, Z2FvbXNoNjZAc29odS5jb20=