- Department of Geology, University of Georgia, Athens, GA, United States
As a species, we have reached a tipping point for Earth derived from our unsustainable resource use. While conservation efforts occurred early in human civilization, it was not until 1980 that the full force of environmental destruction, including the Santa Barbara oil spill in the 1970s, culminated in the new discipline of conservation biology focused on the biosphere. Similarly, conservation paleobiology, named two decades later, brings the unique perspective of the fossil record to conservation efforts, uniting biosphere and geosphere scientists. To date, conservation history does not include paleontological or geological perspectives. Further, each discipline has a different benchmark—near time—for when Earth’s ecosystems were modified by humans. Accordingly, the history of conservation efforts leading up to conservation biology and conservation paleobiology was examined from a geological and ecological framework. To provide a benchmark for near time, the hominin record and their geo-environmental modifications were also examined and revealed that by the start of the Holocene, all continents except ice-covered Antarctica and Greenland had human-modified ecosystems. Therefore, near time is dispensable when the Holocene Epoch is universally understood and precisely defined as a time when H. sapiens dominated environments. Lastly, a conservation corps is urgently needed, following the long tradition of F.D. R.’s Civilian Conservation Corps of the 1930s and J.F. Kennedy’s Peace Corps of the 1960s, to promote a global network connecting all students and practitioners of conservation disciplines to focus on biotic resilience, recovery, and solutions for the world’s most pressing environmental problems.
“The green mantle of Earth is now being ravaged and pillaged in a frenzy of exploitation by a mushrooming mass of humans and bulldozers. Never in this 500 million years of terrestrial evolution has this mantle we call the biosphere been under such savage attack.”—M. Soulé and B. Wilcox, 1980.
“All conservation issues are embedded in time”–C. Meine, 1999.
Introduction
Hominins (family Hominidae, subfamily Homininae, Tribe Hominini: archaic and modern humans and their closest ancestors; Almécija et al., 2021) have modified their environments and ecosystems starting ∼3 million years ago, a record that began when they selected and extracted stones from riverbeds or rock outcrops and worked them into tools indicative of cognitive innovations. At the start of the Holocene (11.7 ka), hunter-gathering niches were replaced by agricuture and farming that necessitated a less nomadic lifestyle and the development of settlements (Rowley-Conwy and Layton, 2011; Boivin et al., 2016). It is at this time that human populations greatly increased (Bocquet-Appel, 2011) and their effects on ecosystems became globally widespread producing an altered terrestrial biosphere that occurred much earlier than the Industrial Revolution (Ellis, 2011; 2021; Ruddiman, 2013; Boivin et al., 2016; Ellis et al., 2021). By 12,000 years ago, Homo sapiens had dispersed to all continents except Antarctica (Ruddiman, 2003; 2013; Stephens et al., 2019; Ellis, 2021; Ellis et al., 2021). In no other group of mammals had just a single species changed every ecosystem they had lived in from polar to tropical regions. Certainly, megaherbivorous dinosaurs had affected the evolution and diversification of plants (Onstein et al., 2022) and other megafauna altered marine and terrestrial systems (Estes et al., 2016; Malhi et al., 2016) but not to the extent of hominins, especially H. sapiens.
In 2022, the world’s population reached 8 billion people. We have passed our carrying capacity for Earth (Barrett and Odum, 2000) and are now facing the consequences of our runaway population and resource use (Ehrlich and Ehrlich, 2009; Barnovsky et al., 2012; Forman and Wu, 2016). Although we created a “Used Planet”, there are areas of recovery and resilience (Ellis et al., 2013; Morikawa and Palumbi, 2019; Gold and Vermeij, 2023; Schug et al., 2023). Recovery and resilience is what science needs to focus on with all hands on deck, melding all conservation efforts from bio-, geo-, paleo- and archaeological realms.
Conservation biology is a “crisis-based” science (Soulé, 1985) having to act even in the face of uncertainty before all the data are in—like the medical field adapting to its first global bat virus pandemic—to solve pressing environmental, ecological, and natural resource problems for a sustainable Earth. While conservation biology focuses on mostly modern biodiversity issues, it does not necessarily work on modern death assemblages or the fossil record. A related discipline, conservation paleobiology, examines the entire record from modern habitats and death assemblages to the fossil record (Figure 1; Flessa, 2002; Kowalewski, 2004; Dietl and Flessa, 2011; Dietl et al., 2015; Kidwell, 2015; Kosnik and Kowlewski, 2016; Tyler and Schneider, 2018; Dillon et al., 2022).
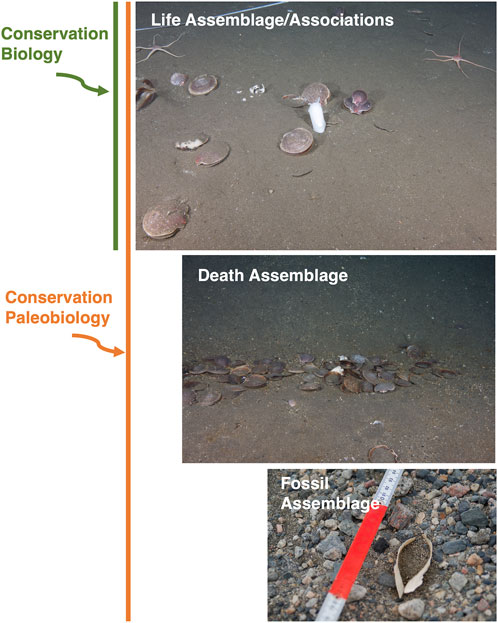
FIGURE 1. Temporal time frame of conservation biology and conservation paleobiology. Conservation biology works mostly on modern life associations and conservation paleobiology works not only with the life assemblage but also modern death assemblages and the fossil record.
Conservation paleobiology was formulated two decades after Soulé and Wilcox’s (1980) seminal call to arms for the conservation movement. Because it is relatively new, there is still a debate about whether it is a discipline or a subdiscipline of conservation biology (Dietl, 2016; Tyler and Schneider, 2018; Dillon et al., 2022). Herein, it is referred to as a discipline: a particular branch of science with its own epistemic goals and training methods (Mazzocchi, 2019).
Conservation paleobiology is a discipline because its development as a science is comparable to the development of conservation biology and it has its own unique goals and tool kit. Stemming from the pressing global biodiversity crisis, Soulé and Wilcox (1980) immediately invoked conservation biology as a discipline in their first edited volume, bringing together ecologic, genetic, and later, management and economic fields. Similarly, conservation paleontology is interdisciplinary, spanning biology, archaeology, paleogenomics, paleoecology, geology, fisheries/forestry, among others (e.g., Bottjer et al., 2006; Rick and Lockwood, 2013; Dietl et al., 2015; Hofman et al., 2015; Kosnik and Kowalewski, 2016). Paleobiology focuses on the entire fossil record of biodiversity crises, such as species originations, extinctions, and recovery from extinctions. Indeed, biodiversity “catastrophes” (extinctions) were first recognized by the vertebrate paleontologist, Georges Cuvier, in the late 1700s long before biologists recognized this concept (Ager, 1993). Lastly, conservation paleobiologists have their own unique tool kit adapted and modified from paleobiology, geology, biology, information sciences and economics, to uniquely use the fossil record for conservation goals.
Here, I review the historical underpinnings of conservation up to the conception of conservation biology and paleobiology, the latter of which is not currently in conservation textbooks. Second, the hominin record of resource is also reviewed to determine when this activity became a global phenomenon that all continents shared, except Antarctica which has the most recent hominin modifications (refer to Figure 1.1 in Ehrenfeld, 1970). That review will provide a prospectus on whether the term near time is needed in the conservation disciplines. Finally, a plea is rendered for more deep-time studies to help solve conservation issues; such studies are largely overlooked in conservationn paleobiology (Dillon et al., 2022). Additionally, the establishment of an interdisciplinary Conservation Corps is needed to solve the world’s pressing environmental problems. Such a corps builds on the work of Franklin Delano Roosevelt’s original WWII conservation efforts and John F. Kennedy’s Peace Corps of the 1960s.
Conservation biology and conservation paleobiology: a geohistorical perspective
Historical treatments for conservation biology are readily available in textbooks and journal articles (Dasmann, 1958; Meine et al., 2006; Cardinale et al., 2020; Sher, A., 2022) but the geologic and paleoecologic context for that history is lacking. Unlike conservation biology, conservation paleobiologists work with the fossil record of life on Earth starting ∼3.8 billion years ago, providing modern conservation studies a rich legacy of past biodiversity in relation to environmental and climatic change. Geologists and paleobiologists have studied these major changes for nearly five centuries, starting with Nicholas Steno in the 1600s, James Hutton and Alexander von Humboldt in the 1700s, Charles Lyell in the 1830s, and Charles Darwin, Alfred Russel Wallace, among many others, in the 1830s–1890s.
In this section, I provide a timeline of critical bio-geohistorical events that led to the modern conservation movement in the 1970s that set the stage for the emergence of conservation paleobiology in the 1990s. The section’s theme is that humans have invoked conservation efforts numerous times over their history, but ultimately, they were not successful in implementing them until the 1970s. Conservation efforts of the 1970s were hard won and always occurred after major crises. Those foundational efforts are still at risk of being repealed by the politicization of bio- and geospheric resources without sustainable solutions. Going forward, humans need to be vigilant, diligent and not ambivalent about fiercely defending a sustainable Earth.
∼10,000 BC–AD 1000s: nature for the spirit and human use
Indigenous peoples had land ethics, but also land modifications, dating to ∼10,000 ka in North and South America (Chapman et al., 1982; Denevan, 1992; Jostad et al., 1994; Martin and Szuter, 1999; Fluery et al., 2014; Piperno et al., 2021) and much earlier in Europe, Africa and Australia (Rose, D.B., 1988; Ikuenobe, P.A., 2014; Ellis et al., 2021; Fletcher et al., 2021). In Asia, conservation had ancient roots in philosophy and spiritual practices of living harmoniously with nature dating back ∼7,000 years (Cardinale et al., 2020; Taylor, 2020; Van Dyke and Lamb, 2020). Plato and other Greek philosophers were the first to write about how humans disrupted nature, specifically soil erosion related to deforestation; the Romans also contributed to extensive deforestation (Hughes, 2011). Thousands of years of land alteration through deforestation also occurred in the Middle East, where oak, cedar and fir forests were altered to scrubland or desert (Mikesell, 1969; Yasuda et al., 2000; Kaplan et al., 2009; Deckers and Pessin, 2017). Unfortunately, these activities continue to this day (Bayram and Öztürk, 2020).
During this time, preservation of nature only occurred for spiritual reasons (Ormsby and Bhagwat, 2010; Brandt et al., 2013) or for hunting or other resource cultivating activity (Schuster et al., 2019; Armstrong et al., 2021). The first known preserved land for hunting started ∼ AD 1000 in England and China but were reserved for the nobility and not for the populace (Van Dyke and Lamb, 2020).
AD 1600–AD 1800s: knowledge of human’s destruction on earth and ineffective laws
From the 1600s–1800s, humans tried to adopt laws to limit environmental destruction (Clepper, 1966; Egerton, 2012). In colonial America, for example, increased population, deforestation, land use and targeting vulnerable sea and land animals led to near ecological collapse in many local and regional areas (Clepper, 1966). Regulations were inacted locally to preserve timber, land animal and fish stocks, but none of those laws were effective because tobacco and fish were major exports, leading to more deforestation, soil erosion, river damming, pollution and, consequently, the fishes’ regional extinction (Clepper, 1966).
In the late 1700s and early-to-mid-1800s, scientists had differing views concerning population increases and resource use. Malthus (1798) acknowledged that the human population needed to be maintained “to the level of means of subsistence” (his p. iii). He further discussed that population increased geometrically, but food only arithmetically. Malthus saw the dangers of increased population but did not think that humans would control this increase although he hoped they would. Even though influenced by Malthus for his natural selection tenets, Darwin (1874, p. 706) did admit, as Malthus did, that human’s “natural rate of increase” led to “obvious evils”, but unlike Malthus, Darwin thought that human population should be left unfettered to allow competition to occur. Darwin’s ideas on population and resource use also differed from his contemporary, Charles Lyell. In his Principles of Geology (1837), Lyell discussed how “the growth of human population cannot take place without diminishing the numbers, or cause the entire destruction, of many animals” (his p. 252). Lyell was also one of the first to address the temporal scope of climate change and the extirpation of many indigenous species after the arrival of Europeans.
Consequently, by the 1800s, it was well known that humans altered the landscape, but conservation efforts were not common, and if instituted, were not enforced. For example, despite the inception of the U.S. Fish Commission in 1871, Pacific salmon were severely exploited at that time and are now facing collapse (Clepper, 1966; Miller et al., 2014; Dadswell et al., 2021). Similarly, wild Atlantic Salmon are nearly extinct today likely from illegal-unreported-and-unregulated high seas fisheries and fitness reduction from interbreeding with farmed salmon (McGinnity et al., 2003; Dadswell et al., 2021). Salmon are critical keystone species in marine and freshwater ecosystems (Hyatt et al., 2000) and their dead carcasses are integral to terrestrial and aquatic ecosystems (Cederholm et al., 1999). Often the solutions to conservation issues are tradeoffs between social, ecologic, and economic factors (Harrison and Gronsleth Gould, 2022), but if this issues dates back nearly 200 years, why have we not protected the salmon?
Starting in the 1840s in eastern North America, sport hunters were the first real conservationists as they began to enforce game protection (Clepper, 1966). These hunters were behind legislation that enacted fish and wildlife protections in the 1860s that led to an increase in conservation societies, laws, and parks such as the first conservation society, the American Fisheries Society in 1870, the 1872 Wild Bird Protection Act in England, and the first conservation park in the United States, Yellowstone Natural Park, in 1872 (Clepper, 1966).
A groundbreaking book, Man and Nature by Marsh (1864), was the first to illustrate humans’ global assault on nature, from introduced species to the complete destruction of landscapes (Ehrenfeld, 1970). In 1886, the Division of Forestry at the U.S. federal level was enacted to manage forest resources (Clepper, 1966). Nevertheless, it was not until the 1900s that conservation laws became actionable.
AD 1900s: environmental crises ushered in conservation movement
In the early 1900s, Aldo Leopold was the main proponent of forestry and wildlife management. By 1940, numerous federal agencies, such as the U.S. Fish and Wildlife Service, were instituted (Clepper, 1966; Meine, 2022). Additionally, the exploitation of fisheries and marine mammals since the 1700s led to regional and international laws in the early 1900s but were not successful. For example, a law in 1911 to protect the Northern Fur Seal from being captured at sea did not protect them on land. This pinniped was previously hunted by Russians starting in the 1780s, then exploited by the United States up to 1984, and today, the seal is still captured on land by indigenous peoples despite population decreases (Veltre and McCartney, 2002; Young, 2009). It was not until after the combined disasters of the Dust Bowl, the Great Depression and World War II that the conservation and environmental movement in the United States was started in earnest.
AD 1930–AD 1940s: compounded crises: the dust bowl, great depression and WWII
The great poverty, human displacement, and environmental deterioration from the Dust Bowl (∼1934–1940) was caused by a series of severe droughts acting on farming methods that did not preserve the topsoil, leading to extensive wind erosion (Cook et al., 2009; McLeman et al., 2014). The Dust Bowl crisis and the Great Depression occurred at the same time, compounding their effects (Crafts and Fearon, 2010). To remedy the poverty and environmental destruction from both crises, the Civilian Conservation Corp (CCC; 1933–1944) was formed. The CCC was the brainchild of Franklin Roosevelt’s New Deal that employed roughly three million men to remediate depleted farm, agricultural and timbered landscapes by planting over three billion trees, emplacing erosion control dams, and building hundreds of state parks (Maher, 2008). The CCC set the seed for remediation and conservation efforts in the 20th and 21st century.
While ancient cultures were likely the first to practice soil conservation using terraces, it is not known if their intent was to prevent soil erosion (Dotterweich, 2013). It was only after the Dust Bowl that major soil conservation efforts began, including the first journal on Soil and Water Conservation (Baveye et al., 2011). Unfortunately, massive soil degradation still occurs across the globe today and needs urgent study, mitigation, and restoration (Baveye et al., 2011). Soil erosion not only affects terrestrial ecosystems but also the health of aquatic ecosystems and the air organisms breathe.
WWII had the first aerial bombardments in Earth’s history that destroyed coastal and inland environments and infrastructure in Europe, North Africa, Britain, Japan, and the Pacific Island region (Leaning, 2000). Also for the first time in Earth’s history, nuclear weapons were used in WWII with horrific consequences for nature and its biotic occupants. Despite that knowledge, nuclear bomb detonation continued after the war with ∼423 atmospheric tests between 1945 and 1957 that devastated Pacific islands where the tests occurred, rendering them unlivable; Caribbean Islands like Puerto Rico were also used as testing grounds (Tate and Hull, 1964; Leaning, 2000; Gerrard, 2015; Thomson and Samuels-Jones, 2020). These islands continue to face the legacy of WWII’s environmental fallout to this day because bioturbation continuously redistributes the radionuclides from these nuclear tests (McMurtry et al., 1985; Thomson and Samuels-Jones, 2020).
The first college textbook on conservation was a direct outgrowth of the environmental devastation of WWII, responding to a need to promote the “wise management of natural resources” for future generations. That textbook, Raymond Dasmann’s Environmental Conservation (1958), covered topics such as soil, water, land and aquatic resource conservation and also advocated limitations on population growth. The increase in human populations was also strongly argued as devastating to the environment a decade later by Anne and Paul Ehrlich, 1968 popular book, The Population Bomb. Criticisms aside (see Ehrlich and Ehrlich, 2009), their book was prescient: The Earth has a finite carrying capacity for humans and their per capita demand for resources (Barrett and Odum, 2000). Barrett and Odum (2000) discovered that the Earth had already reached its carrying capacity for humans by 2000. Although currently debated when Earth’s environmental tipping point will occur (Dakos et al., 2019), Earth already has or will soon likely surpass it (McKay et al., 2022; World Meteorological Organization, 2023).
AD 1969: Santa Barbara oil spill, California
Carson (1962) Silent Spring instilled in the public the harms of unregulated pesticides such as DDT as well as polluting industries. Her work spurred grassroot efforts to rectify those issues and later in that decade, the journal, Biological Conservation, was founded, focused on sociological and economic aspects of conservation. Despite those efforts, California had big city smog and raw sewage spewed into coastal waters where children played (Walker, pers. experience). Along U.S. Route 101 near Ventura and Santa Barbara, open-flame methane burned from oil field off gassing and crop dusters sprayed DDT on citrus groves closely associated with residential areas. But, it was not until the Santa Barbara oil spill in January 1969 that was the watershed moment for conservation action.
The Santa Barbara oil spill was an unmitigated disaster, turning miles of coastal beaches black with tar and oil and killing untold thousands of marine organisms and seabirds (Clarke and Hemphill, 2019). Ironically, its environmental effects were never adequately documented (Spezio, 2018), but for the public, the Santa Barbara oil spill was the final straw. Within a year, the first Earth Day was proclaimed on 22 April 1970, ushering in the environmental decade with tougher federal laws to preserve Earth’s finite resources and environmental health.
AD 1970–AD 1980: the environmental decade
Ten years after the publication of Silent Spring and 2 years after the Santa Barbara oil spill, Apollo 17 astronauts photographed the Earth in color for the first time (the “Blue Marble”; Wuebbles, 2012). The Blue Marble image was a stark reminder that Earth is the only planet with known life in our entire Solar System. Consequently, that image became the iconic symbol of the conservation movement (Wuebbles, 2012) and galvanized grassroot efforts calling for federal environmental regulations.
Nixon’s “Environmental Decade” (1970–1980) inacted the first major federal acts and agencies to protect species (including humans) and the environment. As a direct response to the Santa Barbara oil spill, the National Environmental Policy Act and the Environmental Protection Agency (EPA) was founded in 1970. Soon followed the Safe Drinking Water Act, Comprehensive Environmental Response, Compensation and Liability Act, the Clean Air Act, the Coastal Zone Management Act, the Marine Protection Research and Sanctuary Act, the Endangered Species Act, the Wild and Scenic Rivers Act, and the Marine Mammal Protection Act, among many others (Freeman, 2002; Spezio, 2018). Still, although the EPA banned DDT in the United States in 1972, it continues to be used in other regions of the world to detrimental affects (Padayachee et al., 2023).
An important textbook by David Ehrenfeld (1970), Biological Conservation, inspired the teaching and training of students to work toward a sustainable Earth. Ehrenfeld soon followed with a popular book, Conserving Life on Earth (1972), presenting conservation efforts to the public and answering critics who called the new conservation movement “elitist” (Ehrenfeld, 1972, p. xii). In 1974, the Foundation for Environmental Conservation published the first conservation journal, Environmental Conservation. Even though forestry journals date back to the 1800s, the first ecosystem-based journal was started in 1976, Forest Ecology and Management. Nevertheless, it was not until the 1980s that conservation biology became respectable as a discipline, a profession and fundable through granting agencies.
AD 1980s: conservation biology, a full-fledged discipline
Conservation biology immediately became a discipline with the publication of the college textbook, an edited volume, Conservation Biology, by Michael Soulé and Wilcox, (1980). Their goal was to preserve “biological diversity and its evolutionary potential”, which was the first time that conservation biology had an evolutionary basis with a focus on extinction dynamics pertaining to modern species. Their book concerned the tropics because they argued those regions were more prone to environmental devastation. We now know that the entire Earth is experiencing major effects of anthropogenic activities, with the polar regions warming three to four times faster than any other region on Earth (Clem et al., 2020; Rantanen et al., 2022; Hörhold et al., 2023; Molina et al., 2023). Antarctica and Greenland are warming at an alarming rate (Wang et al., 2022; Hörhold et al., 2023; Kusahara et al., 2023). In particular, the Taylor Dry Valley (Figure 2A) is experiencing mass loss of glaciers and land ecosystems are changing (Hoffman et al., 2017; Andriuzzi et al., 2018) and the southern part of Greenland (Figure 2B) is melting at above average rates (Hörhold et al., 2023).
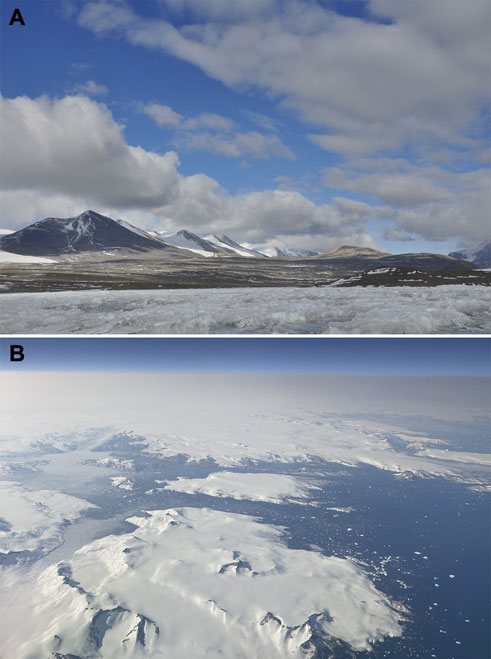
FIGURE 2. Polar regions are heating up faster than any other region of the world. (A), Taylor Dry Valley, Antarctica, in 2008; (B), southern Greenland in 2022.
Soulé and Wilcox’s approachable style and emotional appeal actuated scientists and the public around the world to work in the conservation field (Meine et al., 2006; Noss, 2006; Taylor, 2020). Soulé continued to advocate for conservation science throughout his career (Soulé, 1985; Meine et al., 2006; Taylor, 2020). They justified conservation biology with a cogent call to arms: Because “many habitats, especially tropical ones, are on the verge of total destruction and many large animals are on the verge of extinction”, the intrinsic need for applied applications to save biodiversity is not only necessary but vital. They further changed the mindset about scientific funding, which funded “pure” science at that time but not “applied” subjects like saving habitats and animals. Their new discipline also provided a basis for teaching students, the future stewards for a sustainable Earth. Lastly, they argued that scientists had to engage in science outreach concerning conservation, which was not popular in academia at the time.
The journal Conservation Biology was founded in 1987 with a focus on conserving Earth’s biodiversity through conservation methods and science. Conservation Letters was added much later in 2007. Both journals and the earlier journal, Biological Conservation, are published under the auspices of the Society for Conservation Biology that was established in 1985 after the international success and influence of Soulé and Wilcox 1980 seminal book.
With relatively few exceptions, conservation biology focuses on modern species and ecosystems, allowing species to evolve as naturally as possible as a means to preserve biodiversity and to prevent extinctions (Soulé and Wilcox, 1980; Soulé, 1985). However, evolution does not take place in an ecological vacuum (Boucot, 1983) and the need for an evolutionary-ecological fossil perspective—a geohistorical perspective (Smith and Dietl, 2016)—was needed to also address modern conservation issues.
AD 1990s to today: conservation paleobiology, a full-fledged discipline
Prior to naming conservation paleobiology in 2002, fossils had a long history in conservation efforts. For example, micropaleontologists have used plant pollen to document the fossil and modern history of environmental change (Von Post, 1916; 1924; Janssen, 1967; Brewster-Wingard and Ishman, 1999; Owens, 2020), while others have used singled-celled protists, such as diatoms and foraminifera, and tiny animals such as ostracodes, to reconstruct past aquatic environments, document pollution, and chart the history of sea level and climate change (e.g., Ehrenberg, 1829; Cleve, 1894; Patrick, 1957; Alve, 1991; Culver and Buzas, 1995; Hallock, 2000; Karlsen et al., 2000; Martin, 2000; Zachos et al., 2001; Cronin et al., 2003; Smol, 2009; Smol and Stoermer, 2010). Freshwater mollusks are also used to examine the history and effects of pollution in rivers and lakes starting in the 1800s (Ortman, 1909; Baker, 1922; van der Schalie, 1936; Ingram, 1957) and continues to this day (Dettman and Lohmann, 1993; Goewert et al., 2007; Lundquist et al., 2019; Kusnerik et al., 2022). The first application of stable isotopes (δ18O) for obtaining paleotemperature and climatic records was tested with marine mollusks (Urey et al., 1951; Epstein and Lowenstam, 1953; Valentine and Meade, 1960; Krantz et al., 1987) and as the ability to sample smaller organisms improved, deep-sea foraminifera have given us the best record of Cenozoic climate change (Zachos et al., 2001). Vertebrate paleontology, as well, presented the overkill hypothesis which focused on humans as major hunters of megamammals, leading to their extinction during the Late Pleisotocene (Martin, 1967; 1990) although there were other contributing factors, like climate change (Nagaoka et al., 2018). With the long history of conservation work using fossils—often published in many disciplines like environmental geology, historical geology, paleoecology, and micropaleontology—it was just a matter of time that conservation paleobiology, as a discipline, was coined.
Conservation paleobiology was named by the paleobiologist, Karl Flessa (Flessa, 2002). Along with Michał Kowalewski, his Ph.D. student in the 1990s, (Figure 3), they focused on the historical ecosystem related to the collapse of the Colorado River’s estuary resulting from the lack of freshwater caused by upstream dams and diversions that began in the 1930s. To understand the major changes to the estuary’s ecosystems, they examined fossil shells on the shores of the northern Gulf of California (Figure 4; Kowalewski et al., 2000; Kowalewski, 2001; 2004). Based on the mountains of fossil shells preserved near San Felipe, Baja California, Mexico, they started an extensive research program to “put the dead to work” (after David Jablonski, see Dietl and Flessa, 2011), by using modern death assemblages and fossils to understand the past ecosystem history of the Colorado River’s estuary.
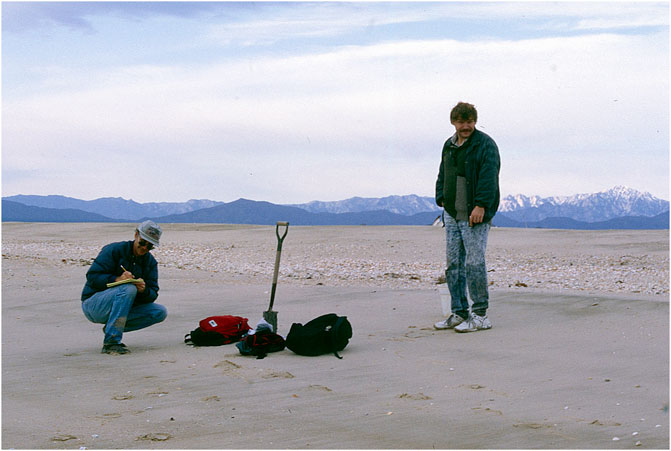
FIGURE 3. Karl Flessa and Michał Kowalewski in San Felipe, Mexico, Feb. 15, 1992. Karl coined conservation paleobiology and together, they provided an early framework for the discipline based on their work on the Colorado River Delta, Mexico.
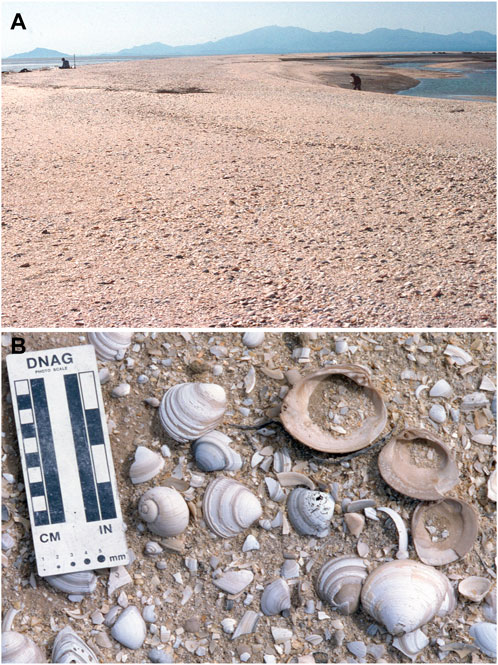
FIGURE 4. Extensive molluscan deposits represent the Colorado River Delta’s former free-flowing water and productivity, San Felipe, Mexico, Feb. 15, 1992. (A), Karl Flessa and Michał Kowalewski working on the extensive shell deposits; (B), Close up of the fossil mollusks that were common in the estuary prior to the damming and diversions of the Colorado River.
Conservation paleobiology uses geohistorical data to complement the modern focus of conservation biology (Kowalewski, 2001; 2004; 2009; Flessa, 2002; Dietl and Flessa, 2009; 2017; Dietl et al., 2015; Kidwell, 2015; Dietl, 2016; Smith and Dietl, 2016; Barnovsky et al., 2017; Tyler and Schneider, 2018; Dillon et al., 2022). Both are multidisciplinary and focus on reducing biodiversity loss, mitigating human alteration of ecosystems, and providing data for sustainable resource management. But there are differences, and that is specifically the use of the fossil record (Kowalewski, 2009; Dietl and Flessa, 2011). Conservation paleobiology bridges the entire record from today to before the time that hominins first perturbed their environment. That is, while conservation biology focuses mostly on living ecosystems, conservation paleobiologists focus on the whole package: from living to fossil assemblages (refer to Figure 1).
Charles Lyell discussed how important it was to examine fossil processes to understand the present. In his Principles of Geology (1837, Vol. 1), he stated, “by these researches into the state of the earth and its inhabitants of former periods, we acquire a more perfect knowledge of its present conditions … ” and further, “…if we enlarge our experience of the present economy of nature, we must investigate the effects of her operations in former epochs” (his p. 1–3). Hence, environmental topics have deep roots in the geological sciences.
Temporal record of hominins as geo- and ecological agents on land and sea
Based on genomic data, hominins diverged from apes between ∼9.3 and ∼6.5 mya in the late Miocene (Figure 5; Moorjani et al., 2016; Almécija et al., 2021). Intense debate is still raging concerning when the hominin lineage split from apes (Moorjani et al., 2016; Almécija et al., 2021). One of the earliest known hominins, Australopithecus, dates to ∼3.5 mya from Chad (Brunet, 1995), but another bipedal hominin from Lake Chad, Sahelanthropus, now extends the hominin fossil lineage to the Late Miocene (∼7 mya; Daver et al., 2022). Our species, H. sapiens, originated in Africa ∼315 ka (Figure 5; late Middle Pleistocene; Hublin et al., 2017) and remarkably, within ∼200,000 years, was on every habitable continent (Figure 6).
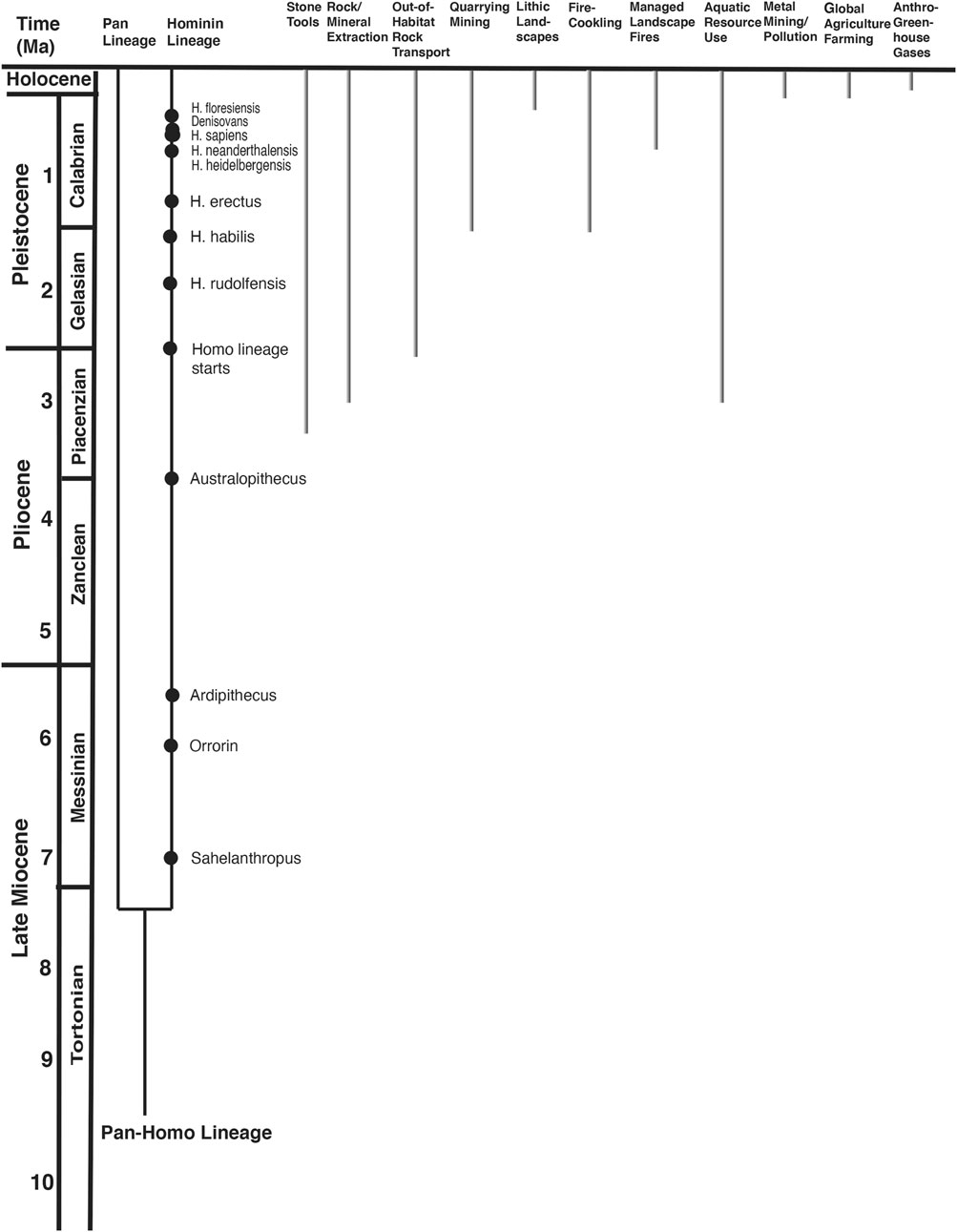
FIGURE 5. Temporal scope of the hominin fossil record and antiquity of their geo-ecosystem perturbations. Time is in millions of years (Ma); references are in text for timing of events.
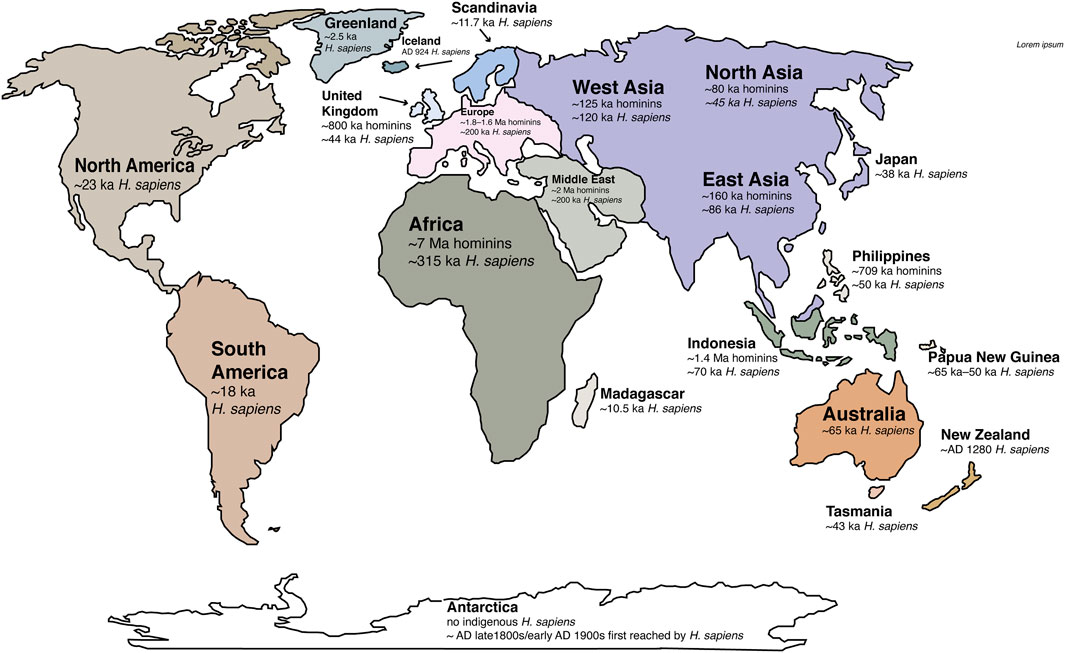
FIGURE 6. Global map with the timing of Homo sapiens and other hominins (mostly archaic humans except for Africa’s 7 Ma hominins) as they arrived around the world. The Earth was untouched by hominins sensu lato prior to their origination and migration into other regions; however, it was the adoption of agriculture and pastorialism at the start of the Holocene Epoch that changed Earth systems forever (see text). References for Homo sapiens first known dates: Africa ∼315 Ma (Richter et al., 2017); Middle East and Europe ∼200 ka (Late Pleistocene; Slimak et al., 2022; Hublin et al., 2017; Hershkovitz, et al., 2018; Scerri et al., 2018; Harvati et al., 2019); Asia (West, East and North Asia combined: ∼120 ka (Liu et al., 2015; 2021; Bae et al., 2017; Freidline et al., 2023); Indonesia ∼70 ka (van den Bergh et al., 2016); Australia ∼65 ka (Clarkson et al., 2017); Papua New Guinea ∼56 ka (Shaw et al., 2020); Philippines ∼50 ka (Ingicco et al., 2018; Arenas et al., 2020); Tasmania ∼43 ka (Turney et al., 2008); United Kingdom ∼44 ka (Higham et al., 2011); Japan ∼38 ka (Ikawa-Smith, 2022); North America ∼23 ka (Goebel et al., 2008; Bennett et al., 2021); South America ∼18 ka (Dillehay et al., 2008); Scandinavia ∼11.7 ka (Günther et al., 2018; Manninen et al., 2021); Madagascar ∼10.5 ka (Hansford et al., 2018); Greenland ∼2.5 ka (Jensen and Gotfred, 2022); Iceland ∼ AD 924 (Sveinbjörnsdóttir et al., 2016); New Zealand ∼ AD 1280 (Wilmshurst et al., 2008); Antarctica had no indigenous hominin population, but reached by Homo sapiens in the 1800s and early 1900s for exploration (Borchgrevink, 2014). Archaic humans arrived in the Arabian Peninsula in the early Pleistocene ∼2 Ma (Foulds et al., 2017); in southern Europe by ∼ 1.6 or 1.8 Ma (including Dmanisi, Georgia) and in northernEurope and the United Kingdom by ∼ 500–800 ka (Key and Ashton, 2022). They may have also occupied Asia and Indonesia by ∼ 1.8 Ma (Forestier et al., 2022; Husson et al., 2022) and the Philippines by 709 ka (Ingicco et al., 2018; Arenas et al., 2020).
Scientists, depending on their discipline, have many terms for the historic record, from environmental history, historical ecology, historical biology, conservation archaeobiology, paleobiology, and so on (see also Rick and Lockwood, 2013). But from a conservation standpoint, none have identified the time when hominins altered their environments such that no “pristine” environments existed on any continent. Knowing and justifying when such a time occurred on the different continents would provide a temporal and spatial framework for conservation studies prior to hominin environmental modifications, especially those of H. sapiens.
Determining the first occurrence of hominin activities is challenging because those occurrences are diachronous across a global landscape (Thompson et al., 2021; Gibbard P. L. et al., 2022). Often, the earliest records are considered regional in scope without global effects (Ellis et al., 2013). Nonetheless, all human perturbations start at the local level and build outward as populations and their resource needs increase. Forensic evidence such as stone tools, burnt artifacts, changes in pollen types across landscapes and anthroturbation (bioturbation by humans, such as mining; Zalasiewicz et al., 2014), among many other factors, all provide clues to the antiquity of hominin-environmental interactions.
In this section, I review the hominin fossil record to determine: 1) When they started modifying their environment from a geologic perspective (among other factors); and 2) when this activity became a global phenomenon that all continents shared.
Rock and mineral extraction
The oldest evidence for hominin-environmental interaction and the oldest hominin artifacts are stone tools discovered in East Africa (Kenya) that were presumably used for hunting and processing animals and/or wood that date to the Pliocene Epoch, 3.3 Ma (Harmand et al., 2015; Lewis and Harmand, 2016). Stone tool technology was widespread in the East Africa Rift Valley starting ∼3 Ma (as Oldowan tools), and by ∼ 2.0 Ma, stone tools were also present in northern and southern Africa (Toth and Schick, 2006; de la Torre, 2011; Plummer et al., 2023). Oldowan stone tools are hammer stones, choppers and scrapers and were a major cognitive breakthrough in stone technology (Toth and Schick, 2006). Hominins had sufficient cognitive ability to select geologically utilitarian rocks and minerals like chert, quartz, and fine-grained volcanics (Stout et al., 2005). In effect, these early hominins were able to discern hardness and composition long before Carl Freidrich Mohs defined the hardness scale for minerals in the early 1800s. Stone tool manufacture also capitalized on another geological characteristc of chert-bearing rocks: conchoidal fracture that produced sharp edges through knapping (de la Torre, 2011). Selecting rocks and tool use occurs in other primates, like Capuchin monkeys and Chimpanzees (Mercader et al., 2002; Falótico et al., 2019). But, unlike hominins, stone tools by other primates is restricted to localized areas and are distinct from hominin worked stone (Boesch and Boesch, 1984; Mercader et al., 2002; Toth and Schick, 2006; de la Torre and Hirata, 2015; Boesch et al., 2019; Falótico et al., 2019).
Out-of-habitat rock transport
Early hominins were transporting stones on a relatively large scale, more so than other non-hominin primates, out of their original collecting region, in some cases up to 13 km (Stout et al., 2005). Out-of-habitat transport also mixed rock types and this became extensive across East Africa starting ∼2.6 Ma (after Stout et al., 2005). Hominins also brought their stones and technology with them as they migrated across the globe. Based on early settlements in Israel, H. sapiens likely brought the Levallois stone tool technology—using stone hammers to produce sharp-flaked tools—out of Africa to northern regions (Prévost and Zaidner, 2020; Slimak et al., 2022). Unfortunately, we still have limited understanding of the amount of stone transported out of habitat in the different geographic regions. If this geoinformation were known, it would provide quantitative estimates for out-of-habitat transport by hominins that brought exotic rocks and minerals into new environments as they migrated and established populations.
Lithic landscapes: the first proposed anthropogenic landscape
Lithic landscapes, environments dominated by a high mount of hominin-worked stone, can have as many as 40,000 lithics per km2 in Plio-Pleistocene archaeological sites (i.e., Koobi Fora; Isaac and Harris, 1981) and more in other hominin-dominated localities (Foley and Mairazón Lahr, 2015). The Messak region of the Central Sahara is one of the first entirely altered anthropogenic landscapes, comprising multiple layers of lithic artifacts dating to the Middle Pleistocene (∼100 ka) and covering an area of ∼15,000 km2 (Foley and Mairazón Lahr, 2015). However, a comprehensive review of ancient lithic landscapes is needed to understand the full extent of how early hominins altered the Earth’s surface as they migrated throughout Africa and elsewhere in the world with their stone repertory.
Mining/quarrying and earliest mining pollution
Anthroturbation (Zalasiewicz et al., 2014) likely started when hominins first extracted rocks from stream beds or outcrops to use in tool making ∼3.3 Ma. Their effects on the environment would likely compound especially if they quarried or mined the same area over thousands or more years forming quarryscapes (e.g., Heldal, 2009). In this manner, hominins are major geologic and geomorphic agents (Hooke, 1994; 2000; Wilkinson, 2005; Price et al., 2011), a process that likely started as soon as they extracted stone to make tools and moving boulders and stone to make seasonal living areas (Hooke, 2000).
The earliest rock quarries were likely chert quarries ∼1.65 Ma in East Africa (Stout et al., 2005). However, major rock quarrying starts ∼300 ka (Verri et al., 2004) and was globally extensive by the beginning of the Holocene (∼11 ka) when hominins were transitioning from hunter-gathers to an agrarian society and needed more sophisticated stone tools and building stones for agriculture, farming, and pastoralism (Zalasiewicz et al., 2014; Grosman and Goren-Inbar, 2016). Massive rock quarrying to build pyramids and other large-scale edifices began in earnest ∼3000 BC (Weiss, 2022). In toto, the antiquity and geographic distribution of ancient rock quarries is still not completely known.
Metals, like copper, were likely used for bead manufacture starting ∼11 ka (Radivojević and Rehren, 2016), but whether these first metals were mined or extracted from river sediments or rock outcrops is unknown. Metal minining may have occurred in Turkey by ∼10 ka (Roberts et al., 2009). Metallurgy (metal smelting using ores from which metals are extracted) began independently in multiple locations in Asia, Europe and Africa between 8 and 6 ka (Höppner et al., 2005; Radivojević and Rehren, 2016; Holl, 2020; but see Roberts et al., 2009). The oldest metal mine, a copper mine, dates to ∼5400 BC in the Balkans (Radivojević and Rehren, 2016) and many others in Europe are known from 4,500–3650 BC (Höppner et al., 2005; Maggie and Pearce, 2005). The amount of copper used, estimated from artifacts, was considerable. For example, the Vinča culture in the Balkans probably used 5 tonnes (5,000 kg) of copper between ∼7.4 and 6.4 ka (Radivojević and Rehren, 2016).
Extraction and transportation of lead, gold, silver, and tin bronzes also occurred across the Middle East, Europe and Asia between ∼8 and 6 ka. The artifacts made from these precious metals could occur “many hundreds of kilometers from the nearest sources of metals” indicating extensive trade (Roberts et al., 2009). The earliest use of iron dates to 5.2 ka in Egypt (hammered iron beads from a meteorite; Rehren, et al., 2013), with many African iron-producing sites known from ∼5 ka (Holl, 2020). Nonetheless, the full extent of metal mining and its detrimental effects on the environment during the early to mid-Holocene are just starting to become known.
The first environmental pollution from toxic metals began when hominins learned to extract precious metals from ores, especially if they selected ores, like copper-arsenic, to make jewelry and eating utensils. Some Eurasian cultures bartered for copper/arsenic alloys (Roberts et al., 2009) and along with lead, these metals were absorbed during the smelting process (Grattan et al., 2002) or entered bone through diagenesis generated by metal offerings during burial (Özdemir et al., 2010). Metals preserved in bones can be used as an environmental indicator of early air, land, and water pollution (Grattan et al., 2002, 2007). These toxic metals from ancient smelting sites are still bioavailable to wildlife today and ancient archaeological sites need to be assessed for their chemical toxicity (Camizuli et al., 2018).
Land modifications from fire to farming and agriculture
Human land use is one of the most fundamental characteristics that has completely altered Planet Earth, affecting ecosystems, water, air and land quality, climate, and biodiversity, making the Earth a very “Used Planet” (Ellis et al., 2013). But when did land removal and modifications by hominins start in the geologic record? Some claim that these modifications started as soon as hominins picked up stones (Price et al., 2011), but hominins became a much larger eco-geological force when they combined stone tools with fire as their populations increased and they migrated across Africa in the Late Pliocene and Early Pleistocene (∼2.8 and 1.5 Ma; Gunz et al., 2009; Ellis, 2011). As they migrated out of Africa multiple times starting ∼1.8 Ma (Prat, 2017), they cleared landscapes of vegetation, hunted, and may have contributed to megafaunal extinctions as the climate changed during the Ice Ages (Kaplan et al., 2010; Ellis, 2011).
In general, biomes modified by humans started to change ∼12 ka when human hunter-gathers cleared land using fire leading to ∼60% seminatural anthromes (Ellis et al., 2020). Seminatural anthromes are defined as inhabited by hominins, but with minor perturbations from densely settled regions and agriculture (Ellis et al., 2020, their Table 1). Between ∼10 and ∼8 ka, agriculture, and pastoralism along with dense human inhabitations became geographically extensive (Ellis et al., 2020). Today, few wild biomes are left that have not been altered by humans (Ellis, 2011; Ellis et al., 2020).
Fire
Fire for cooking dates to the early Pleistocene (∼1.5 Ma; Goren-Inbar et al., 2004; Gowlett, 2016) but was geographically limited. Fire used in landscape modification and for manipulating and capturing food, among other behaviors, is evident by the Middle Pleistocene (∼1.0–0.7 Ma; Bema et al., 2012; Gowlett, 2016). By the Late Pleistocene (∼0.4 Ma), fire artifacts (burnt cobbles, grass, seeds, bone, shells, flint, soil, and large hearths) were globally widespread (Gowlett, 2016) indicating cultural interchange that happened relatively quickly among the populations (MacDonald et al., 2021). In Europe at that time, Neanderthals had excellent technical knowledge on how to use fire to make tools (Roebroeks and Villa, 2011) and may have known how to make fire using the “strike-a-light” method of percussing pyrite on flint (Sorensen et al., 2018).
Fire also allowed the movement of hominins into karstic landscapes, such as caves. What we would now consider cave defacement through breakage of speleothems (stalactites and stalagmites; in archaeology, speleofacts) and graffiti (cave paintings), fire use allowed hominins to move deeper into cave regions. Neanderthals, for instance, broke and then rearranged speleothems to make regular repeating sculptures associated with fire pits deep within Bruniquel Cave, France (Middle Pleistocene ∼176 ka; Jaubert et al., 2016). Cave paintings date to ∼65 ka and are also attributed to Neanderthals (Hoffmann et al., 2018), but the full extent of deep cave modifications is still relatively unknown.
Hunting
Hominins started exterminating large mammals first in Australia in the late Pleistocene ∼50 ka and then later in other localities (Africa, Eurasia, the Americas, Oceania; Ellis et al., 2013). Once large mammals became scarce, hominins broaden their diets to include smaller mammals (Ellis et al., 2013), and as they migrated across the globe, hunting down the food web became more common. Combined with climate change, hominins greatly accelerated the coextinctions of allied organisms and the alteration of their ecosystems (Burney and Flannery, 2005; Ellis et al., 2013; Galetti et al., 2017).
Seed dispersal and farming/agriculture
Because of the loss of megamammal seed dispersers in the Late Pleistocene, plants may have evolved traits like fleshy fruits to attract humans (Spengler, 2020). At that time, hominins transported seeds out of their original habitats and planted them where they settled (Ellis et al., 2013). The earliest cultivated plants by human-hunter gathers date to 23 ka based on an excavated campsite near the Sea of Galilee, Israel (Snir et al., 2015). Over 140 seed species including “proto-weeds” were discovered at that site, considered the earliest disturbed area used for cereal cultivation. Nevertheless, the earliest record of plant and animal husbandry started in the early Holocene (∼11.5 ka; Zeder, 2011).
Homo sapiens made a large impact on the Earth during the adoption of farming and agriculture (Ellis, 2011; Price et al., 2011; Doughty, 2013; Ellis et al., 2013; 2021; Waters et al., 2016). The earliest evidence for farming is known from the Middle East ∼23 ka, where cereal grains were processed and presumably cultivated at seasonal shelters (Snir et al., 2015). By ∼11 ka, farming and agriculture became well established with fruit tree cultivation and animal domestication (Smith, 2007). Large scale burial mounds were also being built starting at ∼11 ka (Ellwood et al., 2022), and by ∼6 ka, urbanized areas began in Mesopotamia and Egypt; these regions also had the first canals for irrigation (Hooke, 2000; Mays, 2010). As farming and agriculture spread across the globe ∼10 ka, so did human populations and their consumption of resources (Gowlett, 2016; Freeman et al., 2018). These activities not only led to deforestation, but also widespread soil erosion (Hooke, 2000; Owens, 2020).
Greenhouse gases
With the intensification of agriculture and pastoralism, anthropogenic CO2 built up in the atmosphere ∼8 to 7 ka (Ruddiman, 2003; 2013). Deforestation was much more extensive than in pre-industrial times, contributing to an increase in greenhouse gases, especially around 7 ka (Ruddiman, 2013). Rice farming in Asia also contributed to anthropogenic CH4 beginning ∼5 ka (Ruddiman, 2003). Extensive human land use and ecosystem alteration began in earnest during the Industrial Revolution (∼AD 1760–AD 1860; Price et al., 2011), which led to burning of coal (Price et al., 2011). Greenhouse gases further accelerated during and after the first and second World Wars (Ellis, 2011; Price et al., 2011).
Altered aquatic ecosystems
It is debated whether early hominins may have relied solely on terrestrial organisms for food and later developed the need for aquatic food resources (Walter et al., 2000; Marean et al., 2007). The earliest record of hominins butchering freshwater hippopotamuses occurred in Kenya ∼3.0 to 2.5 Ma (Plummer et al., 2023). Remains of freshwater fish, turtles, frogs, crocodiles, and mollusks appear at hominin sites in the African Rift Valley ∼2.3 to ∼1.1 Ma (Erlandson, 2001). From ∼700 to ∼300 ka, remains from freshwater fish and mollusks became geographically widespread at hominin sites in Eurasia (Erlandson, 2001). In the Americas, coastal and freshwater archaeological deposits date to ∼16.5 ka (Monte Verde, Chile; Dillehay et al., 2008) and by ∼12 to ∼8 ka, freshwater fish, waterbirds, and small mammals (beavers and river otters) occurred in hominin sites in both North and South America (Erlandson, 2001).
For marine systems, the earliest and best evidence comes from coastal South Africa ∼162 ka, where intertidal mollusk remains were found in association with stone tools (Marean et al., 2007). Stone artifacts (bifacial hand axes, obsidian tools) are also reported from Red Sea reef terraces (∼125 ka, Eritrea; Walter et al., 2000). At that locality, the artifacts are near land mammal bones and oyster beds, suggestive of a food processing site. Neanderthals ate intertidal crabs, selecting the larger specimens for cooking (∼50 ka in Portugal; Nabais et al., 2023). Seafood, especially mollusks, became more common at hominin sites in northern Africa and Europe ∼15 ka (Erlandson, 2001, his Table 1). Early hominins appeared to favor easy-to-obtain intertidal mollusks such as mussels (Perna) and gastropods (Turbo; whelks; limpets; Marean et al., 2007; Marean, 2014). Early hominins also may have scavenged whale blubber (Jerardino and Parkinton, 1993). Hominin migrations into and out of Africa through the Red Sea region and eventually into Indonesia and Australia were likely sustained by foraging for marine food resources in estuaries, mangroves and other intertidal zones (Walter et al., 2000; Bulbeck, 2007; Steneck and Pauly, 2019).
Early hominins fed on intertidal species that were easy to catch, but when fishing hooks were created, they could catch larger fish in the open ocean. The oldest preserved fishhooks date to ∼42 ka and are found in association with pelagic tuna remains, indicating that hominins caught these fish using watercraft (O’Conner et al., 2011). Transportation over the open ocean greatly expanded hominins accessibility to other continents and new resources (Kaifu et al., 2020). They must have invented marine transportation by at least 65 ka, enabling them to settle in Australia (Clarkson et al., 2017). Fishing up the food web for tuna or cod using new improved boats and fishing technologies soon led to depletions of favored fish stock, which led to fishing down the food web; these changes were evident from food items preserved in Caribbean archaeological deposits prior to the arrival of Columbus in AD 1492 (Jackson, 1997; Steneck and Pauly, 2019).
Large ocean-going vessels carried extensive plant, animal and other resources between Egypt and its trading partners ∼6 ka, but the Industrial Revolution increased over-ocean transport of resources on a global scale (Carlton, 1999). Coal-fired ships from which fish were caught in all regions and depths of the ocean led to fisheries collapse especially in the 1920s and 1940s (Steneck and Pauly, 2019). Globalization of fishing fleets with long lines and other destructive methods have further devastated fish stocks culminating in major depletions of marine organisms, from whales to kelp, altering oceanic ecosystems (Estes et al., 1998; 2016; Steneck and Pauly, 2019). Environmental effects of shipping also include oil spills, bilge waste release, noise pollution, strikes on marine mammals and birds, and the introduction of invasive marine species (Carlton, 1999; Fabry et al., 2008; Erbe et al., 2019; Walker et al., 2019). Combined with warming seas and ocean acidification, the fate of sea creatures is bleak unless we set limits on the uncontrolled exploitation of marine resources (after Jackson, 1997).
The conundrum of near time
Conservation biology and paleobiology both use historical records to understand how Earth’s environments have changed by hominin use (Meine, 1999; Flessa, 2002; Kowalewski, 2004; Dillon et al., 2022). The term near time was first invoked by conservation biologists to denote the time when H. sapiens originated, the singular species that has caused global extinctions, while deep time refers to all pre-human time (after Meine, 1999). At the time Meine (1999) wrote his article, H. sapiens arose between ∼40,000 and 120,000 years ago. Recent evidence indicates that H. sapiens had a pan-African origin between ∼300,000 and 200,000 years ago, with the oldest fossils discovered in Morocco ∼315, 000 years ago (Hublin et al., 2017). However, the origin of H. sapiens by itself does not justify erecting the term near time unless the term is also connected to the record when humans greatly modified their geo-ecological environments across most continents.
Near time is still not well defined in conservation paleobiology. Early work in conservation paleobiology suggested that the “fossil record of the very recent past (the last centuries to millennia) can serve as an unprecedented baseline for studying ecological changes over time scales not accessible by studying modern conditions alone” (Kowalewski, 2004). This time frame is valuable to compare changes over the last centuries or few millennia for specific questions, however, several millennia is still within the time frame of when H. sapiens had major global affects on Earth—in fact “pristine” or “wilderness” environments likely do not exist for the Holocene (Ellis et al., 2021; Fletcher et al., 2021).
Alternatively, conservation paleobiologists suggested that near time starts at or during the Pleistocene either 2.5 or 2.0 Ma (Dietl et al., 2015; Dietl and Flessa, 2017). The justification for using the Pleistocene as the start of near time is to have “similar species and ecosystems as today” (Tyler and Schneider, 2018 their p. 6, and references therein). Yet, the Pleistocene was very different than today, with climate oscillating between glacial and interglacials that likely exacerbated oceanic and terrestrial extinctions (Raffi et al., 1985; Hayward, 2001; Blois et al., 2010; Kiessling et al., 2012). Near time has also been defined as the Pleistocene, Holocene and Recent (Dillon et al., 2022, p. 12), but without justification. To move forward, both conservation biologists and paleobiologists need to determine what near time represents and whether that term is necessary. To accomplish this, the hominin record and their geo-ecological modifications need to be discussed in relation to when their modifications became global (refer to the temporal record of hominins as geo- and ecological agents on land and sea earlier in this paper).
Based on the review of hominins as geo- and ecological agents in the preceeding section and Figure 5, there are five temporal scenarios for near-time designations that are linked to the following: 1) When hominins evolved; 2) when stone tool use and homiinins became pan-African in distribution along with when the Homo lineage evolved; 3) when lithic assemblages and tool use became common out of Africa in the Middle East and Eurasia; 4) when population growth increased and Homo species started settling across the globe; and 5) when H. sapiens became major geo- and ecosystem engineers through agriculture, farming, and the generation of greenhouse gases across the globe (Table 1).
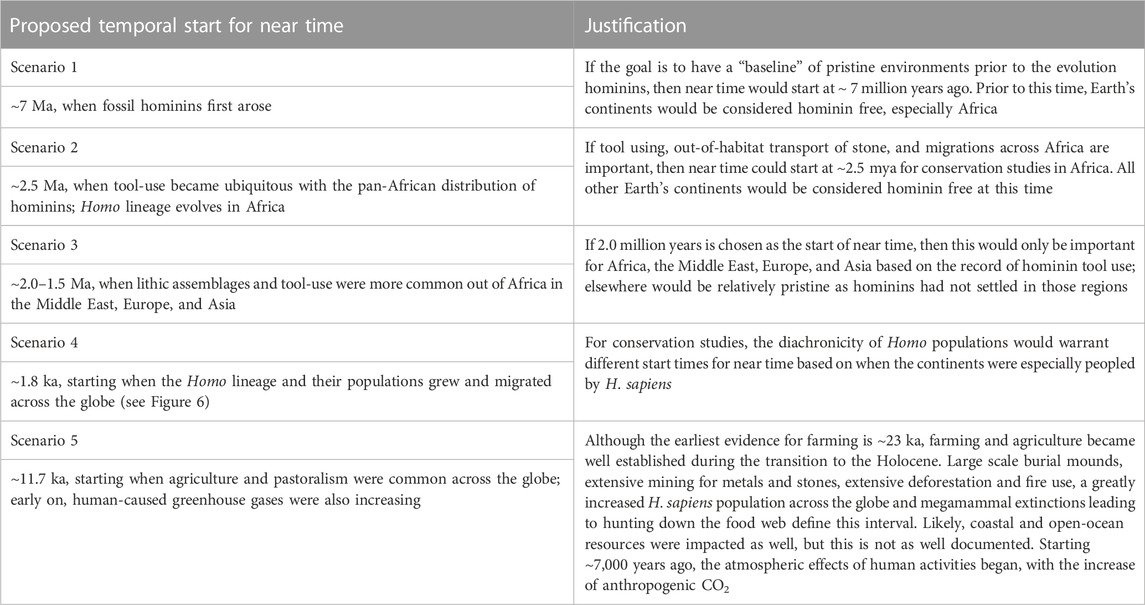
TABLE 1. Scenarios for the designation of near time for conservation biology and conservation paleobiology. The author favors the last one, starting at the beginning of the Holocene Epoch. Refer to references in the paper and Figures 5, 6 for background rationale for these scenarios.
If the evolution of hominins is a priority for a near-time definition, then it would start ∼7 Ma in Africa, as before that time, all continents were hominin free (Table 1: scenario 1). If near time begins at ∼2.5 Ma when stone tool using was ubiquitous across Africa and the Homo lineage evolved, then at that time all continents except Africa were pristine (Table 1: scenario 2). Near time could also start when the Homo lineage migrated out of Africa (∼2.0 and 1.5 Ma) and spread throughout the Middle East and Eurasia bringing their stone tool use and fire technology to modify environments. During that time, North and South America, and Australia were free of hominins (Table 1: scenario 3). Near time could occur when Homo species spread across the globe but would vary depending on when they first occurred on the different continents, from ∼315 ka in Africa to ∼18 ka in South America (Table 1: scenario 4). Those last two scenarios ensure that near time is a diachronous definition for conservation biology and paleobiology practitioners and therefore not universally equivalent across the globe for conservation studies. Lastly, near time could start at the beginning of the Holocene (∼11.7 ka) when agriculture and pastoralism were common across the globe (Table 1: scenario 5). This is also a time of major geolandscape modification, deforestation, megamammal extinctions, metal pollution and anthropogenic greenhouse gas increase, and is the best candidate for near time.
If the Holocene designation is accepted as a substitute for near time, then the use of near time is moot, as the beginning of the Holocene is universally understood and validated. Using the Holocene is also consistent with the decadal to millennial approach of Kowalewski (2004), the prehistoric and historic approach of Flessa (2002), and allows scientists on all continents to have a universally accepted date for the time of extensive hominin modifications to the Earth. This is not an argument for whether the Anthropocene is an Epoch or Event or when it occurred (e.g., Waters et al., 2016; Gibbard P. et al., 2022), rather, it defines a time when hominins were universally modifying the Earth across the globe. Anthrome maps also confirm that ∼60% of the terrestrial surface was semi-natural at ∼ 12 ka (Ellis et al., 2020; 2021), further indicating hominins extensive affects on Earth’s biomes at the start of the Holocene. Conservation scientist can then specify what time of the Holocene they work in, designating today’s environments as the present (with a given time). Deep time would then refer to the pre-Holocene fossil record back to when life evolved on Earth, which is currently known as ∼ 3.8 Ga.
The need for deep-time studies in conservation paleobiology
In a recent survey, Dillon et al. (2022) discovered that many conservation paleontologists work in the Holocene Epoch. Fewer conservation scientists work in older time periods. The relative lack of deep-time studies may be related to temporal scale, as most conservation paleontological efforts focus on familiar time scales from decadal to a few millennia within the Holocene. However, this is the same time that conservation biologists have worked in, as well as paleontologists in the past who have not called themselves conservation paleontologists. That makes sense as the time scales of modern studies are much more comparable to the last few thousand years. Whereas the larger magnitudes of deep time are yet to be adequately scaled to address conservation problems (Kiessling et al., 2019). Perhaps this is a tangible issue for some, but it really depends on the question that is addressed. Paleontologists have the sweep of the entire fossil record to examine the ebb and flow of biodiversity in relation to climate and environmental change which can be adapted to understand resiliency in Earth’s biota.
Flessa (2002) noted that one does not have to argue where the shifting baseline is if the fossil record is used. The fossil record provides nearly 3.8 billion years of ecosystems, while H. sapiens arose only in the last ∼300,000 years. The fossil record has all the data and evidence needed to understand present conservation issues because it is a great repository of physical, chemical, and biotic data waiting to be tapped for conservation efforts. Additionally, all modern organisms have roots in deep time: The majority of animal phyla and plant groups arose at the beginning of the Phanerozoic Eon and microfossils such as cyanobacteria and radiolarians hail from the Archean and Proterozoic Eon, respectively. We need to retool our questions concerning spatial and temporal scale to address the pressing issues that we face (see Benson et al., 2021).
Indeed, paleontology has a long history of using deep time to understand modern biospheric changes. For example, Lyell (1837) discussed how species with broad geographic distributions are less prone to extinction, a topic that today’s paleontologists and conservation biologists work on (e.g., Jablonski, 1986; Payne and Finnegan, 2007; Runge et al., 2015). Lyell (1837) also observed that younger strata have more recent mollusk species than older strata. He, among others, used these observations and data from Deshayes to develop a “numerical proportion of recent to extinct species of fossil shells” for defining time intervals in the Cenozoic Era (Lyell, 1837, Vol. III, p. 373–374; Stanley et al., 1980). His work using “Lyellian percentages” is still foundational for examining extinctions among different geographic regions from the tropics to polar regions (Stanley et al., 1980; Stanley, 1986; Krug et al., 2010) and can be co-opted for conservation paleobiology efforts.
Valentine (1973), like Lyell, argued whole heartedly that the past is the key to the present: Though deep-time organisms may differ, the natural laws that affected them were no different than today. Even though the fossil record might not have all the pieces of past ecosystems, it still has the data to inform on how ecosystems are affected by climate and sea level change and how organisms respond to gradual or rapid change, as well as surviving and recovering from mass extinctions (Valentine, 1989; Valentine and Jablonski, 1991). Using fossil data from the entire Cenozoic Era, temperature change increases extinction rates (Edie et al., 2018), which does not bode well for our animal species, Homo sapiens.
Micropaleontologists use foraminifera to reconstruct major climatic change for the Cenozoic Era, providing scientists with a 65-million-year history (Zachos et al., 2001). Microfossils also reveal rapid climatic change in the past, often combined with major reorientation of oceanographic circulation (Zachos et al., 1993). Foraminifera are also excellent pollution indictors for modern estuarine and coral ecosystems (e.g., Alve, 1995; Hallock, et al., 2003), and some of these changes are known from the fossil record (Alve, 1995). In fact, deep-time microfossils are invaluable for solving many of our current conservation issues (Culver and Rawson, 2000, and references therein).
Lastly, the fossil record provides data on past ecosystem’s carrying capacities, although that topic needs to be addressed. It would be important to know the carrying capacity for different ecosystems through time, especially for changing climate and extinction dynamics. Carrying capacity, in general, is the number of organisms that a habitat can sustain without damage, although there are many ways to define and study it (Barrett and Odum, 2000; Chapman and Byron, 2018). This information is essential for living sustainablity on Earth and could become part of the conservation paleobiology toolkit working alongside of conservation biologists going forward.
Conservation paleobiology in the Anthropocene
Crutzen (2002) christened the term Anthropocene as the most recent time when global greenhouse gases (CO2, CH4) increased in polar ice cores starting in the late 1700s. Today, it is hard to find anything on land or in the oceans that has remained unaltered by human activities (Woodwell et al., 1983; Jackson, 1997; Carlton, 1999; Carlton et al., 1999; Ellis et al., 2020). In fact, Boivin et al. (2016) suggests that the “default expectation” for Earth’s environments is one of anthropogenic alteration even if those habitats appear pristine.
It has yet to be settled as to whether the Anthropocene should be a new geologic age (a new epoch or stage) or an event (Waters et al., 2016; Bauer et al., 2021; Gibbard et al., 2022a; b; Head et al., 2023). For some, an event is more realistic than naming an epoch, given the vagaries in temporal and spatial scale of direct and indirect human-caused perturbations that defy a single golden spike (after Bauer et al., 2021; Gibbard et al., 2022a; b). From a geological perspective—for a Global Stratigraphic Position and Point to mark the start of the new geologic age—there must be an observable and easily assessable stratigraphic area that records fossil species change (e.g., first occurrence of species; extinction of species) without tectonic or chemical alteration, and with a nearly continuous record that can be globally recognized and correlated (Bradner, 1997). However, the GSPP for the beginning of the Holocene does not fit that definition. It took many decades to find the golden spike for the start of the Holocene, which is the end of the Younger Dryas found in the NGRP2 Greenland Ice core, not a sedimentary deposit (Gibbard and Head, 2020). An interdisciplinary group including geologists, paleontologists, geographers and ecologists, have proposed reference sections for the Anthropocene Epoch (Waters et al., 2023), and we may know soon if the Anthropocene Epoch becomes a reality.
Conservation paleobiologists have a unique geohistorical perspective that can help clarify and resolve issues that conservation biologists have about what is a natural state (Dietl and Flessa, 2017) and can facilitate discussions on the Anthropocene going forward. On that note, conservation paleobiologists are pursuing new funding streams (Dietl and Flessa, 2017) and this must continue across scientific societies, industries, and private foundations. We also need more undergraduate and graduate classes offered in conservation paleobiology (see Kelley and Dietl, 2022) that are cross listed with ecology and biology departments. We also need to promote and support a Conservation Biology and Paleobiology Corps (like the Peace Corp of the 1960s and the CCC of the 1930s) that connect researchers from all over the world to work on conservation efforts using the coupled modern and fossil record. Finally, we also need to examine Earth’s fossil biota from a resilience perspective in the face of environmental change (Gold and Vermeij, 2023), and therein lies hope for a sustainable future for Planet Earth.
Summary
1) Conservation efforts have a long history, but it took nearly 7,000 years for humans to inact conservation laws that worked and for the disciplines of conservation biology and conservation paleobiology to be initiated.
2) Conservation biology and conservation paleobiology are complementary disciplines that were formulated after the culmination of a long historical record of geo- and biotic environmental alterations by humans and especially after several major tipping-point crises. The former focuses on modern biota and ecosystems, while the latter also focuses on the fossil archive of biota and paleoecosystems to inform conservation issues.
3) Conservation paleobiology is essential for conservation as the historical record—during hominin’s reign on Earth and before—is housed in the fossil record.
4) Conservation biology and conservation paleobiology overlap as both use the modern and historical record. To that end, both have invoked the term near time but have not justified the term to be useful across disciplines. To provide a justfication, the hominin fossil record and the antiquity of their major geo- and ecologic modifications was reviewed. Based on that review, the author argues that near time is defined as the base of the Holocene, when H. sapiens geo-ecological effects occurred across the globe.
5) Deep-time studies (pre-Holocene and older) are crucial for understanding geo-ecologic change prior to major hominin ecosystem perturbance in the Holocene.
6) Soulé and Wilcox (1980) stated that “the hardest thing to grasp is the geological and historical uniqueness” of what humans are doing to Planet Earth. And now, conservation paleobiology can facilitate that understanding, especially regarding the longevity and resiliency of biota that respond to environmental crises as archived in the fossil record. As we work together, creating interdisciplinary graduate and undergraduate classes in conservation paleobiology, we can also formulate a new interdisciplinary Conservation Biology and Paleobiology Corps that works across the globe to achieve a sustainable future for the Earth’s biota.
Ethics statement
Written informed consent was obtained from the individual(s) for the publication of any identifiable images or data included in this article.
Author contributions
The author confirms being the sole contributor of this work and has approved it for publication.
Acknowledgments
I thank Dr. Bruce Lieberman for inviting me to write this article for Frontiers in Earth Sciences, Roshan Patel for her facilitation of its completion, and two reviewers for their comments that improved this manuscript. I thank Diana Hartle, UGA Science Library, for chasing down enigmatic journal articles and Dr. Karl Flessa for his original paper on conservation paleobiology and editorial assistance and insights on conservation paleobiology’s origins. I also enjoyed discussing “near time” with Adrianne Rankin, Archaeologist for the Barry M. Goldwater Air Force Range, Arizona. I wish to thank my eighth and ninth grade biology teacher, Dr. Muthena Naseri (now emeritus professor of Moorpark College, California), for introducing our class to the major environmental issues facing humanity at that time (and are still relevant today), much to the alarm of our junior high school. Lastly, I thank the support from the Shellebarger Endowment and NSF Polar Programs Grant 1745057.
Conflict of interest
The author declares that the research was conducted in the absence of any commercial or financial relationships that could be construed as a potential conflict of interest.
Publisher’s note
All claims expressed in this article are solely those of the authors and do not necessarily represent those of their affiliated organizations, or those of the publisher, the editors and the reviewers. Any product that may be evaluated in this article, or claim that may be made by its manufacturer, is not guaranteed or endorsed by the publisher.
References
Ager, D. (1993). The New Catastrophism. The importance of the rare event in geologic history. Cambridge: Cambridge University Press.
Almécija, S., Hammond, A. S., Thompson, N. E., Pugh, K. D., Moyà-Sola, S., and Alba, D. M. (2021). Fossil apes and human evolution. Science 372, eabb4363. doi:10.1126/science.abb4363
Alve, E. (1991). Foraminifera, climatic change, and pollution: A study of late holocene sediments in Drammensfjord, southeast Norway. Holocene 1, 243–261. doi:10.1177/095968369100100306
Alve, E. (1995). Foraminiferal responses to estuarine pollution: A review. J. Foram. Res. 25, 190–203. doi:10.2113/gsjfr.25.3.190
Andriuzzi, W. S., Adams, B. J., Barret, J. E., Virginia, R. A., and Wall, D. H. (2018). Observed trends of soil fauna in the Antarctic Dry valleys: early signs of shifts predicted under climate change. Ecology 99, 312–321. doi:10.1002/ecy.2090
Arenas, M., Gorostiza, A., Baquero, J., Campoy, E., Branco, C., Rangel-Villalobos, H., et al. (2020). The early peopling of the Philippines based on mtDNA. Sci. Rep. 10, 4901. doi:10.1038/s41598-020-61793-7
Armstrong, C., Miller, J., McAlvay, A. C., Ritchie, P. M., and Lepofsky, D. (2021). Historical indigenous land-use explains plant functional trait diversity. Ecol. Soc. 26, 6. doi:10.5751/ES-12322-260206
Bae, C. J., Douka, K., and Petraglia, M. D. (2017). On the origin of modern humans: Asian perspectives. Science 358, eaai9067. doi:10.1126/science.aai9067
Baker, F. C. (1922). The molluscan fauna of the Big Vermilion River, Illinois. Ill. Biol. Monogr. 7, 1–126.
Barnovsky, A. D., Hadley, E. A., Gonzalez, P., Head, P. D., Polly, A. M., Lawing, J. T., et al. (2017). Merging paleobiology with conservation biology to guide the future of terrestrial ecosystems. Science 355, eaah4787. doi:10.1126/science.aah4787
Barnovsky, A. D., Hadly, E. A., Bascompte, J., Berlow, E. L., Brown, J. H., Fortelius, M., et al. (2012). Approaching a state shift in Earth’s Biosphere. Nature 486, 52–58. doi:10.1038/nature11018
Barrett, G. W., and Odum, E. R. (2000). The twenty-first century: the world at carrying capacity. BioScience 50, 363–368. doi:10.1641/0006-3568(2000)050[0363:TTFCTW]2.3.CO;2
Bauer, A. M., Edgeworth, M., Edwards, L. E., Ellis, E. C., Gibbard, P., and Merritts, D. J. (2021). Anthropocene: event or epoch? Nature 597, 332. doi:10.1038/d41586-021-02448-z
Baveye, P. C., Rangel, D., Jacobson, A. R., Laba, M., Darnault, C., Otten, W., et al. (2011). From dust bowl to dust bowl: soils are still very much a frontier of science. SSSAJ 25, 2037–2048. doi:10.2136/sssaj2011.0145
Bayram, H., and Öztürk, A. (2020). “Global climate change, desertification, and its consequences in Turkey and the Middle East,” in Climate change and global public health. Editors K. E. Pinkerton, and W. N. Rom (New York: Springer Science+Business Media), 293–305. Respiratory Medicine 7. doi:10.1007/978-1-4614-8417-2_17
Bennett, M. R., Bustos, D., Pigati, J. S., Springer, K. B., Urban, T. M., Holliday, V. T., et al. (2021). Evidence of humans in North America during the last glacial maximum. Science 373, 1528–1531. doi:10.1126/science.abg7586
Benson, R. B. J., Butler, R., Close, R. A., Saupe, E., and Rabosky, D. L. (2021). Biodiversity across space and time in the fossil record. Curr. Biol. 31, R1225–R1236. doi:10.1016/j.cub.2021.07.071
Blois, J. L., McGuire, J. L., and Haldly, E. A. (2010). Small mammal diversity loss in response to late Pleistocene climatic change. Nature 465, 771–774. doi:10.1038/nature09077
Bocquet-Appel, J.-P. (2011). When the world’s population took off: the springboard of the Neolithic demographic transition. Science 333, 560–561. doi:10.1126/science.1208880
Boesch, C., and Boesch, H. (1984). Mental map in wild chimpanzees: an analysis of hammer transports for nut cracking. Primates 25, 160–170. doi:10.1007/BF02382388
Boesch, C., Bombjaková, D., Meier, A., and Mundry, R. (2019). Learning curves and teaching when acquiring nut cracking in humans and chimpanzees. Sci. Rep. 9 (1515), 1515. doi:10.1038/s41598-018-38392-8
Boivin, N. L., Zeder, M. A., Fuller, D. Q., Crother, A., Larson, G., Erlandson, J. M., et al. (2016). Ecological consequences of human niche construction: examining long-term anthropogenic shaping of global species distributions. PNAS 113, 6388–6396. doi:10.1073/pnas.1525200113
Borchgrevink, C. E. (2014). First on the antarctic continent. Cambridge: Cambridge University Press.
Bottjer, D. J., Davidson, E. H., Peterson, K. J., and Cameron, R. A. (2006). Paleogenomics of echinoderms. Science 314, 956–960. doi:10.1126/science.1132310
Boucot, A. J. (1983). Does evolution take place in an ecological vacuum? II. The time has come the walrus said. J. Paleon. 57, 1–30. Available at: https://www.jstor.org/stable/1304605.
Bradner, S. (1997). Best current practice, RCF2119. International Commission on Stratigraphy. Available at: https://stratigraphy.org/gssps/.
Brandt, J. S., Wood, E. M., Pidgeon, A. M., Han, L. X., Fang, Z., and Radeloff, V. C. (2013). Sacred forests are keystone structures for forest bird conservation in southwest China’s Himalayan Mountains. Biol. Conserv. 166, 34–42. doi:10.1016/j.biocon.2013.06.014
Brewster-Wingard, G. L., and Ishman, S. E. (1999). Historical trends in salinity and substrate in central Florida Bay: A paleoecological reconstruction using modern analogue data. Estuaries 22, 369–383. doi:10.2307/1353205
Brunet, M., Beauvilain, A., Coppens, Y., Heintz, E., Moutaye, A. H. E., and Pilbeam, D. (1995). The first australopithecine 2,500 kilometres west of the Rift Valley (Chad). Nature 378, 273–275. doi:10.1038/378273a0
Bulbeck, D. (2007). Where river meets the sea: A parsimonious model for Homo sapiens colonization of the Indian Ocean rim and Sahul. Curr. Anthropol. 48, 315–321. doi:10.1086/512988
Burney, D. A., and Flannery, T. F. (2005). Fifty millennia of catastrophic extinctions after human contact. TREE 20, 395–401. doi:10.1016/j.tree.2005.04.022
Camizuli, E., Scheifler, R., Garnier, S., Monna, F., Losno, R., Gourault, C., et al. (2018). Trace metals from historical mining sites and past metallurgical activity remain bioavailable to wildlife today. Sci. Rep. 8, 3436. doi:10.1038/s41598-018-20983-0
Cardinale, B. J., Primack, R. B., and Murdoch, J. D. (2020). Conservation Biology. New York: Sinauer Associates.
Carlton, J. T., Geller, J. B., Reaka-Kudla, M. L., and Norse, E. A. (1999). Historical extinctions in the sea. Annu. Rev. Ecol. Syst. 30, 515–538. doi:10.1146/annurev.ecolsys.30.1.515
Carlton, J. T. (1999). “The scale and ecological consequences of biological invasions in the world’s oceans,” in Invasive species and biodiversity management. Editors O. T. Sandlund, P. J. Schei, and A. Viken (Dordrecht: Kluwer Academic Publishers), 194–212.
Cederholm, C. J., Kunze, M. D., Murota, T., and Sibatani, A. (1999). Pacific salmon carcasses: essential contributions of nutrients and energy for aquatic and terrestrial ecosystems. Fisheries 24, 6–15. doi:10.1577/1548-8446(1999)024<0006:psc>2.0.co;2
Chapman, E. J., and Byron, C. J. (2018). The flexible application of carrying capacity in ecology. Glob. Ecol. Conserv. 13, e00365. doi:10.1016/j.gecco.2017.e00365
Chapman, J., Delcourt, P. A., Cridlebaugh, P. A., Shea, A. B., and Delcourt, H. R. (1982). Man-land interaction: 10,000 years of American Indian impact on native ecosystems in the lower Little Tennessee River Valley, eastern Tennessee. Southeast. Archaeol. 1, 115–121.
Clarke, K. C., and Hemphill, J. J. (2019). The Santa Barbara oil spill: A retrospective. APCG 64, 157–162. doi:10.1353/pcg.2002.0014
Clarkson, C., Jacobs, Z., Marwick, B., Fullagar, R., Wallis, L., Smith, M., et al. (2017). Human occupation of northern Australia by 65,000 years ago. Nature 547, 306–310. doi:10.1038/nature22968
Clem, K. R., Fogt, R. L., Turner, J., Lintner, B. R., Marshall, G. J., Miller, J. R., et al. (2020). Record warming at the South Pole during the past three decades. Nat. Clim. Chang. 10, 762–770. doi:10.1038/s41558-020-0815-z
Cook, B. I., Miller, R. L., and Seagre, R. (2009). Amplification of the North American “Dust Bowl” drought through human-induced land degradation. PNAS 106, 4997–5001. doi:10.1073/pnas.0810200106
Crafts, N., and Fearon, P. (2010). Lessons from the 1930s Great Depression. Oxf. Rev. Econ. Policy 26, 285–317. doi:10.1093/oxrep/grq030
Cronin, T. M., Dwyer, G. S., Kamiya, T., Schwede, S., and Willard, D. A. (2003). Medieval Warm Period, Little Ice Age, and 20th century temperature variability from Chesapeake Bay. Glob. Planet. Change 36, 17–29. doi:10.1016/s0921-8181(02)00161-3
Culver, S. J., and Buzas, M. A. (1995). The effects of anthropogenic habitat disturbance, habitat destruction, and global warming on shallow marine benthic foraminifera. J. Foram. Res. 25, 204–211. doi:10.2113/gsjfr.25.3.204
Culver, S. J., and Rawson, P. F. (2000). Biotic response to global change, the last 145 million years. Cambridge: Cambridge University Press.
Dadswell, M., Spares, A., Reader, J., McLean, M., McDermott, T., Samways, K., et al. (2021). The decline and impending collapse of the Atlantic Salmon (Salmo salar) population in the Atlantic Ocean: A review and possible causes. Rev. Fish. Sci. Aquac. 30, 215–258. doi:10.1080/23308249.2021.1937044
Dakos, P., Matthews, B., Hendry, A. P., Levine, J., Loeuiville, N., Norberg, J., et al. (2019). Ecosystem tipping points in an evolving world. Nat. Ecol. Evol. 3, 355–362. doi:10.1038/s41559-019-0797-2
Darwin, C. (1874). The descent of man and selection in relation to sex. New York: A.L. Burt Company.
Daver, G., Guy, F., Mackaye, H. T., Likius, A., Boisserie, J.-R., Moussa, A., et al. (2022). Postcranial evidence of late Miocene hominin bipedalism in Chad. Nature 609, 94–100. doi:10.1038/s41586-022-04901-z
de la Torre, I., and Hirata, S. (2015). Percussive technology in human evolution: an introduction to a comparative approach in fossil and living primates. Phil. Trans. R. Soc. B 370, 20140346. doi:10.1098/rstb.2014.0346
de la Torre, I. (2011). The origins of stone tool technology in Africa: A historical perspective. Phil. Trans. Roy. Soc. B Biol. Sci. 366, 1028–1037. doi:10.1098/rstb.2010.0350
Deckers, K., and Pessin, H. (2017). Vegetation development in the Middle Euphrates and upper Jazirah (Syria/Turkey) during the Bronze Age. Quat. Res. 74, 216–226. doi:10.1016/j.yqres.2010.07.007
Denevan, W. M. (1992). The pristine myth: the landscape of the Americas in 1492. Ann. Assoc. Am. Geogr. 82, 369–385. doi:10.1111/j.1467-8306.1992.tb01965.x
Dettman, D. L., and Lohmann, K. C. (1993). “Seasonal change in Paleogene surface water δ18O. Fresh-water bivalves of western North America,” in Climate change in continental isotopic records. American Geophysical Union Monograph. Editors P. K. Swart, K. C. Lohmann, S. McKenzie, and S. Savin, 78, 153–163. doi:10.1029/GM078
Dietl, G. P. (2016). Brave new world of conservation paleobiology. Front. Ecol. Evol. 4, 21. doi:10.3389/fevo.2016.00021
Dietl, G. P., and Flessa, K. W. (2017). Conservation paleobiology science and practice. Chicago: The University of Chicago Press.
Dietl, G. P., and Flessa, K. W. (2011). Conservation paleobiology: putting the dead to work. TREE 26, 30–37. doi:10.1016/j.tree.2010.09.010
Dietl, G. P., and Flessa, K. W. (2009). Conservation paleobiology: using the past to manage for the future. Paleontological Soc. Pap. 15.
Dietl, G. P., Kidwell, S. M., Brenner, M., Burney, D. A., Flessa, K. W., Jackson, S. T., et al. (2015). Conservation paleobiology: leveraging knowledge of the past to inform conservation and restoration. Annu. Rev. Earth Planet. Sci. 43, 79–103. doi:10.1146/annurev-earth-040610-133349
Dillehay, T. D., Ramírez, C., Pino, M., Collins, M. B., Rossen, J., and Pino-Navarro, J. D. (2008). Monte Verde: seaweed, food, medicine, and the peopling of South America. Science 320, 784–786. doi:10.1126/science.1156533
Dillon, E. M., Pier, J. Q., Smith, J. A., Raja, N. B., Dimitrijević, D., Austin, E. L., et al. (2022). What is conservation paleobiology? Tracking 20 years of research and development. Front. Ecol. Evol. 10. doi:10.3389/fevo.2022.1031483
Dotterweich, M. (2013). The history of human-induced soil erosion: geomorphic legacies, early descriptions and research, and the development of soil conservation—a global synopsis. Geomorph 201, 1–34. doi:10.1016/j.geomorph.2013.07.021
Doughty, C. E. (2013). Preindustrial human impacts on global and regional environment. Annu. Rev. Environ. Resour. 38, 503–527. doi:10.1146/annurev-environ-032012-095147
Edie, S. M., Huang, S., Collins, K. S., Roy, K., and Jablonski, D. (2018). Loss of biodiversity dimensions through shifting climates and ancient mass extinctions. Integr. Compar. Biol. 58, 1179–1190. doi:10.1093/icb/icy111
Egerton, F. N. (2012). Roots of ecology from antiquity to Haeckle. Berkeley: University of California Press. doi:10.1525/california/9780520271746.001.0001
Ehrenberg, C. G. (1829). Die geographische verbreitung der infusionstierchen in Nord-Afrika und West-Asien, beobachtet auf Hemprich und Ehrenbergsreisen. Abh. K. Akad. Wiss, 1–20. Berlin.
Ehrlich, P. R., and Ehrlich, A. H. (2009). The population bomb revisited. Electron. J. Sustain. Dev. 1, 63–71.
Ellis, E. C. (2011). Anthropogenic transformation of the terrestrial biosphere. A. 369, 1010–1035. 3691010–1035. doi:10.1098/rsta.2010.0331
Ellis, E. C., Beusen, A. H. W., and Goldewijk, K. K. (2020). Anthropogenic biomes: 10,000 BCE to 2015 CE. Land 9, 129. doi:10.3390/land9050129
Ellis, E. C., Kaplan, J. O., Fuller, D. Q., Vavrus, S., Goldewijk, K. K., and Verburg, P. H. (2013). Used planet: A global history. PNAS 110, 7978–7985. doi:10.1073/pnas.1217241110
Ellis, E. C., Gauthier, , Klein Goldewijk, K., Bird, R. B., Boivin, N., Diaz, S., et al. (2021). People have shaped most of terrestrial nature for at least 12,000 years. PNAS 118 (17), e2023483118. doi:10.1073/pnas.2023483118
Ellis, E. C. (2021). Land use and ecological change: A 12,000-year history. Annu. Rev. Environ. Resour. 46, 1–33. doi:10.1146/annurev-environ-012220-010822
Ellwood, B. B., Warny, S., Hackworth, R. A., Ellwood, S. H., Tomkin, J. J., Bentley, S. J., et al. (2022). The LSU campus mounds, with construction beginning at ∼11,000 BP, are the oldest known extant man-made structures in the Americas. Amer. J. Sci. 322, 795–827. doi:10.2475/06.2022.02
Epstein, S., and Lowenstam, H. A. (1953). Temperature-shell-growth relations of recent and interglacial Pleistocene shoal-water biota from Bermuda. J. Geol. 61, 424–438. doi:10.1086/626110
Erbe, C., Marley, S. A., Schoeman, R. P., Smith, J. N., Trigg, L. E., and Embling, C. B. (2019). The effects of ship noise on marine mammals—a review. Front. Mar. Sci. 6. doi:10.3389/fmars.2019.00606
Erlandson, J. M. (2001). The archaeology of aquatic adaptations: paradigms for a new millennium. J. Archaeol. Res. 9, 287–350. doi:10.1023/a:1013062712695
Estes, J. A., Tinker, M. T., Williams, T. M., and Doak, D. F. (1998). Killer whale predation on sea otters linking oceanic and nearshore ecosystems. Science 282, 473–476. doi:10.1126/science.282.5388.473
Estes, J. A., Heithaus, M., McCAuley, D. J., Rasher, D. B., and Worm, B. (2016). Megafaunal impacts on structure and function of ocean ecosystems. Annu. Rev. Environ. Resour. 41, 83–116. doi:10.1146/annurev-environ-110615-085622
Fabry, V. J., Seibel, B. A., Feely, R. A., and Orr, J. C. (2008). Impacts of ocean acidification on marine fauna and ecosystem processes. ICES J. Mar. Sci. 65, 414–432. doi:10.1093/icesjms/fsn048
Falótico, T., Proffit, T., Ottoni, E. B., Staff, R. A., and Haslam, M. (2019). Three thousand years of wild capuchin stone tool use. Nat. Ecol. Evol. 3, 1034–1038. doi:10.1038/s41559-019-0904-4
Fletcher, M.-S., Hall, T., and Alexandra, A. N. (2021). The loss of an indigenous constructed landscape following British invasion of Australia: an insight into the deep human imprint on the Australian landscape. Ambio 50, 138–149. doi:10.1007/s13280-020-01339-3
Fluery, S., Malaize, B., Giraudeau, J., Galop, D., Bout-Roumazeilles, V., Martinez, P., et al. (2014). Impacts of Mayan land use on Laguna Tuspán watershed (Petén, Guatemala) as seen through clay and ostracode analysis. J. Archaeol. Sci. 49, 372–382. doi:10.1016/j.jas.2014.05.032
Foley, R. A., and Mirazón Lahr, M. (2015). Lithic landscapes: early human impact from stone tool production on the central saharan environment. PloS ONE 10 (3), e0116482. doi:10.1371/journal.pone.0116482
Forestier, H., Zhou, Y., Sophady, H., Li, Y., Codeluppi, D., Auetrakulvit, P., et al. (2022). The first lithic industry of mainland southeast Asia: evidence of the earliest hominin in a tropical context. L’Anthropologie 126, 102996. doi:10.1016/j.anthro.2022.102996
Forman, R. T. T., and Wu, J. (2016). Where to put the next billion people. Nature 537, 608–611. doi:10.1038/537608a
Foulds, F. W. F., Shuttleworth, A., Sinclair, A., Alsharekh, A. M., Al Ghamdi, S., Inglis, R. H., et al. (2017). A large handaxe from Wadi Dabsa and early hominin adaptations within the Arabian Peninsula. Antiquity 91, 1421–1434. doi:10.15184/aqy.2017.153
Freeman, A. M. (2002). Environmental policy since Earth Day I: what have we gained? JEP 16, 125–146. doi:10.1257/0895330027148
Freeman, J., Baggio, J. A., Robinson, E., Byers, D. A., Gayo, E., Finley, B., et al. (2018). Synchronization of energy consumption by human societies throughout the Holocene. PNAS 115, 9962–9967. doi:10.1073/pnas.1802859115
Freidline, S. E., Wetaway, K. E., Joannes-Boyau, R., Duringer, P., Ponche, J.-L., Morley, M. W., et al. (2023). Early presence of Homo sapiens in southeast Asia by 86–68 kyr at Tam Pa Ling, northern Laos. Nat. Comm. 14, 3193. doi:10.1038/s41467-023-38715-y
Galetti, M., Moleón, M., Jordano, P., Pires, M. M., Guimarães, P. R., Pape, T., et al. (2017). Ecological and evolutionary legacy of megafauna extinctions: anachronisms and megafauna interactions. Biol. Rev. 93, 845–862. doi:10.1111/brv.12374
Gerrard, M. B. (2015). America’s forgotten nuclear waste dump in the Pacific. SAIS Rev 35, 87–97. doi:10.1353/sais.2015.0013/
Gibbard, P. L., Bauer, A. M., Edgeworth, M., Ruddiman, W. F., Gill, J., Merritts, D., et al. (2022a). A practical solution: the Anthropocene is a geological event, not a formal epoch. Episodes 45, 349–357. doi:10.18814/epiiugs/2021/021029
Gibbard, P. L., and Head, M. J. (2020). “The Quaternary Period,” in Geologic time scale 2020, 2. Editors F. M. Gradstein, J. G. Ogg, M. D. Schmitz, and G. M. Ogg (Amsterdam: Elsevier), 1217–1255.
Gibbard, P., Walker, M., Bauer, A., Edgeworth, M., Edwards, L., Ellis, E., et al. (2022b). The Anthropocene as an event, not an epoch. J. Quat. Sci. 37, 395–399. doi:10.1002/jqs.3416
Goebel, T., Waters, M. R., and O’Rourke, D. H. (2008). The Late Pleistocene dispersal of modern humans in the Americas. Science 319, 1497–1502. doi:10.1126/science.1153569
Goewert, A., Surge, D., Carpenter, S., and Downing, J. (2007). Oxygen and carbon isotope ratios of Lampsilis cardium (Unionidae) from two streams in agricultural watersheds of Iowa. Palaeogeogr. Palaeoclimatol. Palaeoecol. 252, 637–648. doi:10.1016/j.palaeo.2007.06.002
Gold, D. A., and Vermeij, G. J. (2023). Deep resilience: an evolutionary perspective on calcification in an age of ocean acidification. Front. Physiol. 14, 1092321. doi:10.3389/fphys.2023.1092321
Goren-Inbar, N., Alperson, N., Kisley, M. E., Simchoni, O., Melamid, Y., Ben-Nun, A., et al. (2004). Evidence of hominin control of fire at Gesher Benot Ya'aqov, Israel. Science 304, 725–727. doi:10.1126/science.1095443
Gowlett, J. A. J. (2016). The discovery of fire by humans: A long and convoluted process. Phil.Trans. Roy. Soc. B Biol. Sci. 37, 20150164. doi:10.1098/rstb.2015.0164
Grattan, J., Huxley, S., Abu Karaki, L., Toland, H., Gibertson, D., Pyatt, B., et al. (2002). Death more desirable than life? The human skeletal record and toxicological implications of ancient copper mining and smelting in Wadi Faynan, southwestern Jordan. Toxicol. Ind. Health 18: 297–307. doi:10.1191/0748233702th153oa
Grattan, J. P., Gilbertson, D. D., and Hunt, C. O. (2007). The local and global dimensions of metalliferous pollution derived from a reconstruction of an eight thousand year record of copper smelting and mining at a desert-mountain frontier in southern Jordan. J. Archael. Sci. 34, 83–110. doi:10.1016/j.jas.2006.04.004
Grosman, L., and Goren-Inbar, N. (2016). Landscape alteration by pre-pottery neolithic communities in the southern Levant-the Kaizer Hilltop quarry, Israel. PLoS ONE 11 (3), e0150395. doi:10.1371/journal.pone.0150395
Günther, T., Malmström, H., Svensson, E. M., Omrak, A., Sánchez-Quinto, F., Kılınç, G. M., et al. (2018). Population genomics of mesolithic Scandinavia: investigating early postglacial migration routes and high latitude adaptation. PloS Biol. 16, e2003703. doi:10.1371/journal.pbio.2003703
Gunz, P., Bookstein, F. L., Mitteroecker, P., Stadlmayr, A., Seidler, H., and Weber, G. W. (2009). Early modern human diversity suggests subdivided population structure and a complex out-of-Africa scenario. PNAS 106, 6094–6098. doi:10.1073/pnas.0808160106
Hallock, P., Lidz, B. H., Cockey-Burkhard, E. M., and Donnely, K. B. (2003). Foraminifera as bioindicators in coral reef assessment and monitoring: the FORAM index. Foraminifera in reef assessment and monitoring. Environ. Monit. Assess. 81, 221–238. doi:10.1023/A:1021337310386
Hallock, P. (2000). Symbiont-bearing foraminifera: harbingers of global change? Micropaleo 46 (1), 95–104.
Hansford, J., Wright, P. C., Rasoamiaramanana, A., Perez, V. R., Godfrey, L. R., Errickson, D., et al. (2018). Early Holocene human presence in Madagascar evidenced by exploitation of avian megafauna. Sci. Advan. 4, eaat6925. doi:10.1126/sciadv.aat6925
Harmand, S., Lewis, J. E., Feibel, C. S., Lepre, C. J., Prat, S., Lenoble, A., et al. (2015). 3.3-million-year-old stone tools from Lomekwi 3, West Turkana, Kenya. Nature 521, 310–315. doi:10.1038/nature14464
Harrison, H. L., and Gronsleth Gould, J. (2022). Big catch, undecided risks: perspectives of risk, reward, and trade-offs in Alaska's salmon enhancement program. N. Am. J. Fish. Manag. 42, 1433–1453. doi:10.1002/nafm.10830
Harvati, K., Röding, C., Bosman, A. M., Karakostis, F. A., Grün, R., Stringer, C., et al. (2019). Apidima Cave fossils provide earliest evidence of Homos sapiens in Eurasia. Nature 571, 500–504. doi:10.1038/s41586-019-1376-z
Hayward, B. W. (2001). Global deep-sea extinctions during the Pleistocene ice ages. Geology 29, 599–602. doi:10.1130/0091-7613(2001)029<0599:GDSEDT>2.0.CO;2
Head, M. J., Zalasiewicz, J. A., Waters, C. N., Turner, S. D., Williams, M., Barnosky, A. D., et al. (2023). The Anthropocene is a prospective epoch/series, not a geological event. Episodes 46, 229–238. doi:10.18814/epiiugs/2022/022025
Heldal, T. (2009). “Constructing a quarry landscape from empirical data,” in General perspectives and a case study at aswan west bank, Egypt” in QuarryScapes: Ancient stone quarry landscapes in the eastern mediterranean. Editors N. Abu-Jaber, E. G. Bloxam, P. Degryse, and T. Heldat (Norway: Geol Surv Norway Spec Pub), 12, 125–153.
Hershkovitz, I., Weber, G. W., Quam, R., Duval, M., Grün, R., Kinsley, L., et al. (2018). The earliest modern humans outside Africa. Science 359, 456–459. doi:10.1126/science.aap8369
Higham, T., Compton, T., Stringer, C., Jacobi, R., Shapiro, B., Trinkaus, E., et al. (2011). The earliest evidence for anatomically modern humans in northwestern Europe. Nature 479, 521–524. doi:10.1038/nature10484
Hoffman, M. J., Fountain, A. G., and Liston, G. E. (2017). Near-surface internal melting: A substantial mass loss on antarctic Dry Valley glaciers. J. Glaciol. 60, 361–374. doi:10.3189/2014JoG13J095
Hoffmann, D. L., Standish, C. D., García-Diez, M., Pettitt, P. B., Milton, J. A., Zilhão, J., et al. (2018). U-Th dating of carbonate crusts reveals Neandertal origin of Iberian cave art. Science 359, 912–915. doi:10.1126/science.aap7778
Hofman, C. A., Rick, T. C., Fleischer, R. C., and Maldonado, J. E. (2015). Conservation archaeogenomics: ancient DNA and biodiversity in the Anthropocene. TREE 30, 540–549. doi:10.1016/j.tree.2015.06.008
Holl, A. F. C. (2020). The origin of African metallurgies. Anthropology: Oxford Research Encyclopedia, 45. doi:10.1093/acrefore/9780190854584.013.63
Hooke, R. LeB. (2000). On the history of humans as geomorphic agents. Geology 28, 843–846. doi:10.1130/0091-7613(2000)028<0843:othoha>2.3.co;2
Höppner, B., Bartelheim, M., Huijsmans, M., Krauss, R., Martinek, K.-P., Pernicka, E., et al. (2005). Prehistoric copper production in the Inn Valley (Austria), and the earliest copper in central Europe. Archaeometry 47, 293–315. doi:10.1111/j.1475-4754.2005.00203.x
Hörhold, M., Münch, T., Weißbach, S., Kipfstuhl, S., Freitag, J., Sasgen, I., et al. (2023). Modern temperatures in central-north Greenland warmest in past millennium. Nature 613, 503–507. doi:10.1038/s41586-022-05517-z
Hublin, J.-J., Ben-Ncer, A., Bailey, S. E., Freidline, S. E., Neubauer, S., Skinner, M. M., et al. (2017). New fossils from Jebel Irhoud, Morocco and the pan-African origin of Homo sapiens. Nature 546, 289–292. doi:10.1038/nature22336
Hughes, J. D. (2011). Ancient deforestation revisited. J. Hist. Biol. 44, 43–57. doi:10.1007/s10739-010-9247-3
Husson, L., Salles, T., Lebatard, A.-E., Zerathe, S., Braucher, R., Noerwidi, S., et al. (2022). Javanese Homo erectus on the move in SE Asia circa 1.8 Ma. Sci. Rep. 12, 19012. doi:10.1038/s41598-022-23206-9
Hyatt, K. D., and Godbout, L. (2000). “A review of salmon as keystone species and their ability as critical indicators of regional and ecosystem integrity” in ed. I. M. Darling, Proceedings of a conference on the biology and management of species and habitats at risk, Kamloops, B.C., 15–19 2, B.C. Ministry of Environment, Lands and Parks, Victoria, Kamloops, B.C: B.C. and the University College of the Cariboo.
Ikawa-Smith, F. (2022). “Over the water, into and out of the Japanese archipelago, during the Pleistocene: humans, and lithic techniques” in Maritime prehistory of northeast Asia. The archaeology of asia-pacific navigation, J. Cassidy, I. Ponkratova, and B. Fitzhugh (eds), vol. 6, Singapore: Springer, 51–71. doi:10.1007/978-981-19-1118-7_3
Ikuenobe, P. A. (2014). Traditional African environmental ethics and colonial legacy. Int. J. Philoso. Theol. 2, 1–21. doi:10.15640/ijpt.v2n4a1
Ingicco, T., van den Bergh, G. D., Jago-on, C., Bahain, J.-J., Chacón, M. G., Amano, N., et al. (2018). Earliest known hominin activity in the Philippines by 709 thousand years ago. Nature 557, 233–237. doi:10.1038/s41586-018-0072-8
Ingram, W. M. (1957). “Use and value of biological indicators of pollution: freshwater clams and snails,” in Biological problems in water pollution. Editor C. M. Tarzwell (US Dept. of Health, Education, and Welfare), 94–135.
Isacc, G. L., and Harris, J. (1981). “Stone age visiting cards: approaches to the study of early land use patterns,” in Pattern of the past: Essays in honor of David clarke. Editors I. Hodder, I. Isaac, and N. Hammon (Cambridge: Cambridge University Press), 131–156.
Jablonski, D. (1986). Background and mass extinctions: the alternation of macroevolutionary regimes. Science 231, 129–133. doi:10.1126/science.231.4734.129
Janssen, C. R. (1967). A comparison between the recent regional pollen rain and the subrecent vegetation in four major vegetation types in Minnesota (U.S. A.). Rev. Palaeobot. Palynol. 2, 331–342. doi:10.1016/0034-6667(67)90163-7
Jaubert, J., Verheyden, S., Genty, D., Soulier, M., Cheng, H., Blamart, D., et al. (2016). Early Neanderthal constructions deep in Bruniquel Cave in southwestern France. Nature 534, 111–114. doi:10.1038/nature18291
Jensen, J. F., and Gotfred, A. B. (2022). First people and muskox hunting in northernmost Greenland. Acta Boreal. 39, 24–52. doi:10.1080/08003831.2022.2061763
Jerardino, A., and Parkinton, J. H. (1993). New evidence for whales on archeological sites in the southwestern cape. S. Afr. J. Sci. 89, 6–7.
Jostad, P. M., McAvoy, L. H., and McDonald, D. (1994). Native American land ethics: implications for natural resource management. Soc. Nat. Resour. 9, 565–581. doi:10.1080/08941929609380996
Kaifu, Y., Kuo, T.-H., Kubota, Y., and Jan, S. (2020). Palaeolithic voyage for invisible islands beyond the horizon. Sci. Rep. 10, 19785. doi:10.1038/s41598-020-76831-7
Kaplan, J. O., Krumhardt, K. M., Ellis, E. C., Ruddiman, W. F., Lemmen, C., and Klein, G. (2010). Holocene carbon emissions as a result of anthropogenic land cover change. Holocene 21, 775–791. doi:10.1177/0959683610386983
Kaplan, J. O., Krumhardt, K. M., and Zimmermann, N. (2009). The prehistoric and preindustrial deforestation of Europe. Quat. Sci.Rev. 28, 3016–3034. doi:10.1016/j.quascirev.2009.09.028
Karlsen, A. W., Cronin, T. M., Ishman, S., Willard, D., Kerhin, R., Holmes, C. W., et al. (2000). Historical trends in Chesapeake Bay dissolved oxygen based on benthic foraminifera from sediment cores. Estuaries 23, 488–508. doi:10.2307/1353141
Kelley, P. H., and Dietl, G. P. (2022). Core competencies for training conservation paleobiology students in a wicked world. Front. Ecol. Evol. 10. doi:10.3389/fevo.2022.851014
Key, A., and Ashton, N. (2022). Hominins likely occupied northern Europe before one million years ago. Evol. Anthro. 32, 10–25. doi:10.1002/evan.21966
Kidwell, S. M. (2015). Biology in the Anthropocene: challenges and insights from young fossil records. PNAS 112, 4922–4929. doi:10.1073/pnas.1403660112
Kiessling, W., Raja, N. B., Roden, V. J., Turvey, S. T., and Saupe, E. E. (2019). Addressing priority questions of conservation science with palaeontological data. Phil. Trans. R. Soc. B 374, 20190222. doi:10.1098/rstb.2019.0222
Kiessling, W., Simpson, C., Beck, B., Mewis, H., and Pandolfi, J. (2012). Equatorial decline of reef corals during the last Pleistocene interglacial. PNAS 109, 21378–21383. doi:10.1073/pnas.1214037110
Kosnik, M., and Kowlewski, M. (2016). Understanding modern extinctions in. doi:10.1098/rsbl.2015.0951
Kowalewski, M. (2001). Applied marine paloecolgy: an oxymoron or ralty? Palaios 16, 309–310. doi:10.1669/0883-1351(2001)016<0309:ampaoo>2.0.co;2
Kowalewski, M., Avila Serrano, G. E., Flessa, K., and Goodfriend, G. A. (2000). Dead delta’s former productivity: two trillion shells at the mouth of the Colorado River. Geology 28, 1059–1062. doi:10.1130/0091-7613(2000)028<1059:ddsfpt>2.3.co;2
Kowalewski, M. (2004). “Conservation paleobiology,” in McGraw-hill 2004 Yearbook of science and technology. Editor E. Geller (New York: McGraw-Hill), 60–62.
Kowalewski, M. (2009). “The youngest fossil record and conservation biology: holocene shells as eco-environmental recorders,” in Conservation paleobiology: Using the past to manage the future. Paleontological society short course. Editors G. P. Dietl, and K. Flessa (The Paleontological Society Papers), 15, 1–23.
Krantz, D. E., Williams, D. F., and Jones, D. S. (1987). Ecological and paleoenvironmental information using stable isotope profiles from living and fossil molluscs. Palaeogeogr. Palaeoclimatol. Palaeoecol. 58, 249–266. doi:10.1016/0031-0182(87)90064-2
Krug, A. Z., Roy, K., Jablonski, J., and Beu, A. (2010). Differential Extinction and the Contrasting Structure of Polar Marine Faunas. PloS ONE 5 (12), e15362. doi:10.1371/journal.pone.0015362
Kusahara, K., Tatebe, H., Hajima, T., Saito, F., and Kawamiya, M. (2023). Antarctic sea ice holds the fate of Antarctic Ice-shelf basal melting in a warming climate. J. Clim. 36, 713–743. doi:10.1175/JCLI-D-22-0079.1
Kusnerik, K. M., Means, G. H., Portell, R. W., Kannai, A., Monroe, M. M., Means, R., et al. (2022). Long-Term Shifts in Faunal Composition of Freshwater Mollusks in Spring-Fed Rivers of Florida. Front. Ecol. Evol. 10. doi:10.3389/fevo.2022.851499
Lewis, J. E., and Harmand, S. (2016). An earlier origin for stone tool making: implications for cognitive evolution and the transition to Homo. Phil. Trans. Roy. Soc. B Biol. Sci. 371, 20150233. doi:10.1098/rstb.2015.0233
Liu, W., Matinón-Torres, M., Cai, Y. J., Xing, S., Tong, H. W., Pei, S. W., et al. (2015). The earliest unequivocally modern humans in southern China. Nature 526, 696–699. doi:10.1038/nature15696
Liu, Y., Mao, X., Krause, J., and Fu, Q. (2021). Insights into human history from the first decade of ancient human genomics. Science 373, 1479–1484. doi:10.1126/science.abi8202
Lundquist, S. P., Worthington, T. A., and Aldridge, D. C. (2019). Freshwater mussels as a tool for reconstructing climate history. Ecol. Indic. 101, 11–21. doi:10.1016/j.ecolind.2018.12.048
MacDonald, K., Scherjon, F., van Veen, E., and Roebroeks, W. (2021). Middle Pleistocene fire use: the first signal of widespread cultural diffusion in human evolution. PNAS 118 (31), e2101108118. doi:10.1073/pnas.2101108118
Maggie, R., and Pearce, M. (2005). Mid-fourth-millennium copper mining in Liguria, north-west Italy: the earliest known copper mines in Western Europe. Antiquity 79, 66–77. doi:10.1017/S0003598X00113705
Maher, N. (2008). Nature’s new deal: The civilian conservation corps and the roots of the American environmental movement. New York: Oxford University Press.
Malhi, Y., Doughty, C. E., Galetti, M., Smith, F. A., Svenning, J.-C., and Terborgh, J. W. (2016). Megafauna and ecosystem function from the Pleistocene to the Anthropocene. PNAS 113, 838–846. doi:10.1073/pnas.1502540113
Malthus, T. (1798). An essay on the principle of population and its affects on the furture improvement of society with remarks on the speculation of Mr. Godwin, M. Condorcet, and other writers. London. J. Johnson, in St. Paul’s Church Yard.
Manninen, M. A., Damlien, H., Kleppe, J. I., Knutsson, K., Murashkin, A., Niemi, A. R., et al. (2021). First encounters in the north: cultural diversity and gene flow in Early Mesolithic Scandinavia. Antiquity 95, 310–328. doi:10.15184/aqy.2020.252
Marean, C. W., Bar-Matthews, M., Bernatchez, J., Fisher, E., Goldberg, P., Herries, A. I. R., et al. (2007). Early human use of marine resources and pigment in South Africa during the Middle Pleistocene. Nature 449, 905–908. doi:10.1038/nature06204
Marean, C. W. (2014). The origins and significance of coastal resource use in Africa and Western Eurasia. J. Hum. Evol. 77, 17–40. doi:10.1016/j.jhevol.2014.02.025
Marsh, G. (1864). Man and nature, or, physical geography as modified by human action. New York: Charles Scribner and Co.
Martin, P. S. (1990). 40,000 years of extinctions on the “planet of doom”. Palaeogeogr. Palaeoclimatol. Palaeoecol. 82, 187–201. doi:10.1016/S0031-0182(12)80032-0
Martin, P. S. (1967). “Prehistoric overkill,” in Pleistocene extinctions. Editors P. S. Martin, and H. E. Wright (New Haven: Yale University Press), 75–120.
Martin, P. S., and Szuter, C. R. (1999). War zones and game sinks in Lewis and Clark’s West. Conserv. Biol. 13, 36–45. doi:10.1046/j.1523-1739.1999.97417.x
Martin, R. E. (2000). Environmental Micropaleontology: The application of microfossils to environmental geology. Cambridge: Cambridge University Press.
Mays, L. W. (2010). “A brief history of water technology during antiquity: before the Romans,” in Ancient water technologies. Editor L. Mays (Dordrecht: Springer), 1–28. doi:10.1007/978-90-481-8632-7
Mazzocchi, F. (2019). Scientific research across and beyond disciplines: challenges and opportunities of interdisciplinarity. EMBO Rep. 20, e47682. doi:10.15252/embr.201947682
McGinnity, P., Prodöhl, P., Ferguson, A., Hynes, R., Maoileidigh, N. O., Cotter, D., et al. (2003). Fitness reduction and potential extinction of wild populations of Atlantic salmon, Salmo salar, as a result of interactions with escaped farm salmon. Proc. Roy. Soc. B Biol. Sci. 270, 2443–2450. doi:10.1098/rspb.2003.2520
McKay, D. I., Staal, A., Abrams, J. F., Winkelmann, R., Sakschewski, B., Loriani, S., et al. (2022). Exceeding 1.5 °C global warming could trigger multiple climate tipping points. Science 377, eabn7950. doi:10.1126/science.abn7950
McLeman, R. A., Dupre, J., Ford, L. B., Ford, J., Gajewski, K., and Marchildon, G. (2014). What we learned from the Dust Bowl: lessons in science, policy, and adaptation. Popu. Environ. 35, 417–440. doi:10.1007/s11111-013-0190-z
McMurtry, G. M., Schneider, R. C., Colin, P. L., Buddemeier, R. W., and Suchanek, T. H. (1985). Redistribution of fallout radionuclides in Enewetak Atoll lagoon sediments by callianassid bioturbation. Nature 313, 674–677. doi:10.1038/313674a0
Meine, C. (1999). It’s about time: conservation biology and history. Conserv. Biol. 13, 1–3. doi:10.1046/j.1523-1739.1999.013001001.x
Meine, C. (2022). Land, ethics, justice, and Aldo Leopold. Ecol. Pract. Res. 4, 167–187. doi:10.1007/s42532-022-00117-7
Meine, C., Soulé, M., and Noss, R. F. (2006). A mission-driven discipline: the growth of conservation biology. Conserv. Biol. 20, 631–651. doi:10.1111/j.1523-1739.2006.00449.x
Mercader, J., Panger, M., and Boesch, C. (2002). Excavation of a chimpanzee stone tool site in the African Rainforest. Science 296, 1452–1455. doi:10.1126/science.1070268
Mikesell, M. W. (1969). The deforestation of Mount Lebanon. Geogr. Rev. 59, 1–28. doi:10.2307/213080
Miller, K. M., Teffer, A., Tucker, S., Li, S., Schulze, A. D., Trudel, M., et al. (2014). Infectious disease, shifting climates, and opportunistic predators: cumulative factors potentially impacting wild salmon declines. Evol. Appl. 7, 812–855. doi:10.1111/eva.12164
Molina, A. N., Pulgar, J. M., Rezende, E. L., and Carter, M. J. (2023). Heat tolerance of marine ectotherms in a warming Antarctica. Glob. Change Biol. 298, 179–188. doi:10.1111/gcb.16402
Moorjani, P., Amorim, C. E. G., Arndt, P. F., and Przeworski, M. (2016). Variation in the molecular clock of primates. PNAS 113, 10607–10612. doi:10.1073/pnas.1600374113
Morikawa, M. K., and Palumbi, S. R. (2019). Using naturally occurring climate resilient corals to construct bleaching-resistant nurseries. PNAS 116, 10586–10591. doi:10.1073/pnas.1721415116
Nabais, M., Dupont, C., and Zilhao, J. (2023). The exploitation of crabs by Last Interglacial Iberian Neanderthals: the evidence from Gruta da Figueira Brava (Portugal). Front. Environ. Archaeol. 2. doi:10.3389/fearc.2023.1097815
Nagaoka, L., Rick, T., and Wolverton, S. (2018). The overkill model and its impact on environmental research. Ecol. Evol. 8, 9683–9696. doi:10.1002/ece3.4393
Noss, R. (2006). Is there a special conservation biology? Ecography 22, 113–122. doi:10.1111/j.1600-0587.1999.tb00459.x
O’Conner, S., Ono, R., and Clarkson, C. (2011). Pelagic Fishing at 42,000 Years Before the Present and the Maritime Skills of Modern Humans. Science 334, 1117–1121. doi:10.1126/science.1207703
Onstein, R. E., Kissling, W. D., and Linder, H. P. (2022). The megaherbivore gap after the non-avian dinosaur extinctions modified trait evolution and diversification of tropical palms. Proc. Roy. Soc. B Biol. Sci. 289, 20212633. doi:10.1098/rspb.2021.2633
Ormsby, A. A., and Bhagwat, S. A. (2010). Sacred forests of India: a strong tradition of community-based natural resource management. Environ. Conserv. 37, 320–326. doi:10.1017/S0376892910000561
Ortman, A. E. (1909). The destruction of the freshwater fauna in western Pennsylvania. Proc. Amer. Phil. Soc. XLVII, 90–110.
Owens, P. (2020). Soil erosion and sediment dynamics in the Anthropocene: a review of human impacts during a period of rapid global environmental change. J. Soils Sediments 20, 4115–4143. doi:10.1007/s11368-020-02815-9
Özdemir, K., Selim Erdal, Y., and Demirci, Ş. (2010). Arsenic accumulation on the bones in the Early Bronze Age İkiztepe population, Turkey. J. Archael. Sci. 37, 1033–1041. doi:10.1016/j.jas.2009.12.004
Padayachee, K., Reyolds, C., Mateo, R., and Amar, A. (2023). A global review of the temporal and spatial patterns of DDT and dieldrin monitoring in raptors. Sci. Total Environ. 858, 159734. doi:10.1016/j.scitotenv.2022.159734
Patrick, R. (1957). “Diatoms as indicators of changes in environmental conditions,” in Biological problems in water pollution. Editor C. M. Tarzwell (US Dept. of Health, Education, and Welfare), 71–83.
Payne, J. L., and Finnegan, S. (2007). The effect of geographic range on extinction risk during background and mass extinction. PNAS 105, 10506–10511. doi:10.1073/pnas.0701257104
Piperno, D. R., McMichael, C. H., Pitman, N. C. A., Andino, J. E. G., Guevara Andino, J. E., Rios, M., et al. (2021). A 5,000-year vegetation and fire history for tierra firme forests in the Medio Putumayo-Algodón watersheds, northeastern Peru. PNAS 118 (40), e2022213118. doi:10.1073/pnas.2022213118
Plummer, T. W., Oliver, J. S., Finestone, J. S., Finestone, E. M., Ditchfield, P. W., Bishop, L. C., et al. (2023). Expanded geographic distribution and dietary startegies of the earliest Oldowan homnins and Paranthropus. Science 379, 561–566. doi:10.1126/science.abo7452
Prat, S. (2017). First hominin settlements out of Africa. Tempo and dispersal mode: review and perspectives. Comptes Rendus Palevol 17, 6–16. doi:10.1016/j.crpv.2016.04.009
Prévost, M., and Zaidner, Y. (2020). New insights into early MIS 5 lithic technological behavior in the Levant: Nesher Ramla, Israel as a case study. PloS ONE 15, e0231109. doi:10.1371/journal.pone.0231109
Price, S. J., Ford, J. R., Cooper, A. H., and Neal, C. (2011). Humans as major geological and geomorphological agents in the Anthropocene: the significance of artificial ground in Great Britain. Phil. Trans. Roy. Soc. A 369, 1056–1084. doi:10.1098/rsta.2010.0296
Radivojević, M., and Rehren, T. (2016). Paint it black: the rise of metallurgy in the Balkans. J. Archaeol. Method Theory 23, 200–237. doi:10.1007/s10816-014-9238-3
Raffi, S., Stanley, S. M., and Marasti, R. (1985). Biogeographic patterns and Plio-Pleistocene extinction of Bivalvia in the Mediterranean and southern North Sea. Paleobiology 11, 368–388. doi:10.1017/s0094837300011684
Rantanen, M., Karpechko, A. Y., Lipponen, A., Nordling, K., Hyvärinen, O., Ruosteenoja, K., et al. (2022). The Arctic has warmed nearly four times faster than the globe since 1979. Earth Environ. 3, 168. doi:10.1038/s43247-022-00498-3
Rehren, T., Belgya, T., Jambon, A., Káli, G., Kasztovszky, Z., Kis, Z., et al. (2013). 5,000 years old Egyptian iron beads made from hammered meteoritic iron. J. Archael. Sci. 40, 4785–4792. doi:10.1016/j.jas.2013.06.002
Richter, D., Grun, R., Johannes-Boyau, R., Steele, T. E., Amani, F., Rue, M., et al. (2017). The age of hominin fossils from Jebel Irhoud, Morocco, and the origins of the Middle Stone Age. Nature 546, 293296. doi:10.1038/nature22335
Rick, T., and Lockwood, R. (2013). Integrating Paleobiology, Archeology, and History to Inform Biological Conservation: Paleobiology, Archeology, and History. Conserv. Biol. 27, 45–54. doi:10.1111/j.1523-1739.2012.01920.x
Roberts, B. W., Thornton, C. P., and Pigott, V. C. (2009). Development of metallurgy in Eurasia. Antiquity 83, 1012–1022. doi:10.1017/S0003598X00099312
Roebroeks, W., and Villa, P. (2011). On the earliest evidence for habitual use of fire in Europe. PNAS 108, 5209–5214. doi:10.1073/pnas.1018116108
Rose, D. B. (1988). Exploring an aboriginal land ethic. Meanjin 47, 378–387. doi:10.3316/informit.580106961985695
Rowley-Conwy, P., and Layton, R. (2011). Foraging and farming as niche construction: stable and unstable adaptations. Phil. Trans. R. Soc. B. Biol. Sci. 366, 849–862. doi:10.1098/rstb.2010.0307
Ruddiman, W. F. (2013). The Anthropocene. Annu. Rev.of Earth Planet. Sci. 41, 45–68. doi:10.1146/annurev-earth-050212-123944
Ruddiman, W. F. (2003). The anthropogenic greenhouse era began thousands of years ago. Clim. Change 61, 261–293. doi:10.1023/B:CLIM.0000004577.17928.fa
Runge, C. A., Tulloch, A., Hammill, E., Possingham, H. P., and Fuller, R. A. (2015). Geographic range size and extinction risk assessment in nomadic species: geographic range dynamics of nomadic birds. Conserv. Biol. 29, 865–876. doi:10.1111/cobi.12440
Scerri, E. M. L., Thomas, M. G., Manica, A., Gunz, J., Stock, T., Stringer, C., et al. (2018). Did our species evolve in subdivided populations across Africa, and why does it matter? TREE 33, 582–594. doi:10.1016/j.tree.2018.05.005
Schug, G. R., Buikstra, J. E., DeWitte, S. N., Baker, B. J., Berger, E., Buzon, M., et al. (2023). Climate change, human health, and resilience in the Holocene. PNAS 120, e2209472120. doi:10.1073/pnas.2209472120
Schuster, R., Germain, R. R., Bennett, J. R., Reo, N. J., and Arcese, P. (2019). Vertebrate biodiversity on indigenous-managed lands in Australia, Brazil, and Canada equals that in protected areas. Environ. Sci. Policy 101, 1–6. doi:10.1016/j.envsci.2019.07.002
Shaw, B., Coxe, S., Kewibu, V., Haro, J., Hull, E., and Hawkins, S. (2020). 2500-year cultural sequence in the Massim region of eastern Papua New Guinea reflects adaptive strategies to small islands and changing climate regimes since Lapita settlement. Holocene 30, 1075–1090. doi:10.1177/0959683620908641
Sher, A. A. (2022). An introduction to conservation biology. Massachusetts: Oxford University Press, Sinauer Associates.
Slimak, L., Zanolli, C., Higham, T., Frouin, M., Schewenninger, J-L., Arnold, L. J., et al. (2022). Modern human incursion into Neanderthal territories 54,000 years ago at Mandrin, France. Sci. Adv. 8 (6), eabj9496. doi:10.1126/sciadv.abj9496
Smith, B. D. (2007). The ultimate ecosystem engineers. Science 315, 1797–1798. doi:10.1126/science.1137740
Smith, J. A., and Dietl, G. P. (2016). The value of geohistorical data in identifying a recent human-induced range expansion of a predatory gastropod in the Colorado River delta, Mexico. J. Biogeogr. 43, 791–800. doi:10.1111/jbi.12644
Smol, J. P. (2009). Pollution in lakes and rivers: A paleoenvironmental perspective. Malden: Blackwell Publishing.
Smol, J. P., and Stoermer, E. F. (2010). The diatoms: Applications for the environmental and earth sciences. Cambridge: Cambridge University Press. doi:10.1017/CBO9780511763175
Snir, A., Nadel, D., Groman-Yaroslavski, , Melamed, Y., Bar-Yosef, O., Weiss, E., et al. (2015). The origin of cultivation and proto-weeds long before Neolithic farming. PloS ONE 10 (7), e0131422. doi:10.1371/journal.pone.0131422
Sorensen, A. C., Claud, E., and Soressi, M. (2018). Neandertal fire-making technology inferred from microwear analysis. Sci. Rep. 8, 10065. doi:10.1038/s41598-018-28342-9
Soulé, M., and Wilcox, B. A. (1980). Conservation biology: An evolutionary-ecological Perspective. Massachusetts: Sinauer Associates, Inc.
Spengler, R. N. (2020). Anthropogenic seed dispersal: rethinking the origins of plant domestication. Trends Plant Sci 25, 340–348. doi:10.1016/j.tplants.2020.01.005
Spezio, T. S. (2018). The Santa Barbara oil spill and its effect on United States environmental policy. Sustainability 10, 2750. doi:10.3390/su10082750
Stanley, S. M., Addicott, W. O., and an Chinzei, K. (1980). Lyellian curves in paleontology: possibilities and limitations. Geology 8, 422–426. doi:10.1130/0091-7613(1980)8<422:LCIPPA>2.0.CO;2
Stanley, S. M. (1986). Population size, extinction, and speciation: the fission effect in Neogene Bivalvia. Paleobiology 12, 89–110. doi:10.1017/s0094837300003006
Steneck, R. S., and Pauly, D. (2019). Fishing through the Anthropocene. Curr. Biol. 29, R987–R992. doi:10.1016/j.cub.2019.07.081
Stephens, L., Fuller, D., Boivin, N., Rick, T., Gauthier, N., Kay, A., et al. (2019). Archaeological assessment reveals Earth’s early transformation through land use. Science 365, 897–902. doi:10.1126/science.aax1192
Stout, D., Quade, J., Semaw, S., Rogers, M. J., and Levin, N. E. (2005). Raw material selectivity of the earliest stone toolmakers at Gona, Afar, Ethiopia. J. Hum. Evol. 48, 365–380. doi:10.1016/j.jhevol.2004.10.006
Sveinbjörnsdóttir, A. E., Ramsey, C. B., and Heinemeier, J. (2016). The Settlement Date of Iceland Revisited: evaluation of 14C Dates from Sites of Early Settlers in Iceland by Bayesian Statistics. Radiocarbon 58, 235–245. doi:10.1017/RDC.2016.2
Tate, M., and Hull, D. M. (1964). Effects of nuclear explosions on Pacific Islanders. Pac. Hist. Rev. 33, 379–393. doi:10.2307/3636039
Taylor, B. (2020). Michael Soulé (1936–2020) on spirituality, ethics, and conservation biology. Conserv. Biol. 34, 1426–1432. doi:10.1111/cobi.13634
Thompson, J. C., Wright, D. K., Ivory, S. J., Choi, J.-H., Nightingale, S., Mackay, A., et al. (2021). Early human impacts and ecosystem reorganization in southern-central Africa. Sci. Adv. 7 (19), eabf9776. doi:10.1126/sciadv.abf9776
Thomson, R., and Samuels-Jones, T. (2020). Toxic colonialism in the territorial isles: A geospatial analysis of environmental crime across U.S. territorial islands 2013–2017. Int. J. Offender Ther. Comp. Criminol. 66, 470–491. doi:10.1177/0306624x20975161
Toth, N., and Schick, K. (2006). “An overview of the Oldowan Industrial Complex: the sites and the nature of their evidence,” in The Oldowan: Case studies into the earliest stone age. Editors N. Toth, and K. Schick (Gosport: Stone Age Institute Press), 3–42.
Turney, C. S. M., Fannery, T. F., Roberts, R. G., Reid, C., Fifield, L. K., Higham, T. F. G., et al. (2008). Late-surviving megafauna in Tasmania, Australia, implicate human involvement in their extinction. PNAS 105, 12150–12153.
Tyler, C. L., and Schneider, C. L. (2018). “An overview of conservaton paleobiology,” in Marine conservation paleobiology. Editors C. L. Tyler, and C. L. Schneider (Springer), 47, 1–10. doi:10.1007/978-3-319-73795-9_1Top. Geobiol.
Urey, H. C., Lowenstam, H. A., Epstein, S., and McKinney, C. R. (1951). Measurement of paleotemperatures and temperature of the Upper Cretaceous of England, Denmark, and the southeastern United States. Geol. Soc. Am. Bull. 62, 399–416. doi:10.1130/0016-7606(1951)62[399:mopato]2.0.co;2
Valentine, J. W. (1973). Evolutionary paleoecology of the marine biosphere. New Jersey: Prentise-Hall.
Valentine, J. W., and Jablonski, D. (1991). Biotic effects of sea level change: the Pleistocene test. J. Geophys. Res. 96, 6873–6878. doi:10.1029/90JB00602
Valentine, J. W., and Meade, R. F. (1960). Isotopic and Zoogeographic Paleotemperatures of Californian Pleistocene Mollusca. Science 132, 810–811. doi:10.1126/science.132.3430.810
Valentine, J. W. (1989). Phanerozoic marine faunas and the stability of the earth system. Palaeogeogr. Palaeclimatol., Palaeoecol. 75, 137–155. doi:10.1016/0031-0182(89)90188-0
Van den Bergh, G. D., Li, B., Brumm, A., Grun, R., Yurnaldi, D., Moore, M. W., et al. (2016). Earliest hominin occupation of Sulawesi, Indonesia. Nature 529, 208–211. doi:10.1038/nature16448
van der Schalie, H. (1936). Contributing factors in the depletion of Naiades in eastern United States. Basteria 3, 51–57.
Van Dyke, F., and Lamb, R. (2020). Conservation biology, foundations, concepts and applications. Switzerland: Springer Nature Switzerland AG.
Veltre, D. W., and McCartney, A. P. (2002). Russian exploitation of Aleuts and fur seals: the archaeology of eighteenth-and early-nineteenth-century settlements in the Pribilof Islands, Alaska. Hist. Arch. 36, 8–17. doi:10.1007/BF03374356
Verri, G., Barkai, R., Bordeanu, C., Gopher, A., Hass, M., Kaufman, A., et al. (2004). Flint mining in prehistory recorded by in situ-produced cosmogenic 10Be. PNAS 101, 7880–7884. doi:10.1073/pnas.0402302101
Von Post, L. (1916). Forest tree pollen in south Swedish peat bog deposits. Pollen Spores 9, 375–401.
Von Post, L. (1924). Ur de sydsrenska skogarnas regionala historia under post-arktisk tid. Geol. Foren. Forh. 46, 83–128. doi:10.1080/11035892409444880
Walker, T. R., Adebambo, O., Del Aquila Feijoo, M. C., Elhaimer, E., Hossain, T., Johnson-Edward, S., et al. (2019). “Environmental effects of marine transportation,” in World seas: An environmental evaluation, 111, 505–530. Ecological Issues and Environmental Impacts. doi:10.1016/B978-0-12-805052-1.00030-9
Walter, R. C., Buffler, R. T., Henrich Bruggemann, J., Guillaume, M. M. M., Berhe, S. M., Negassi, B., et al. (2000). Early human occupation of the Red Sea coast of Eritrea during the last interglacial. Nature 40-15, 65–69. doi:10.1038/35011048
Wang, J., Luo, H., Yang, Q., Liu, J., Yu, L., Shi, Q., et al. (2022). An unprecedented record low Antarctic sea-ice extent during austral summer 2022. Adv. Atmos. Sci. 39, 1591–1597. doi:10.1007/s00376-022-2087-1
Waters, C. N., Turner, S. D., Zalasiewicz, J., and Head, M. D. (2023). Candidate sites and other reference sections for the Global boundary Stratotype Section and Point of the Anthropocene Series. Anthropocene Rev. 10, 3–24. doi:10.1177/20530196221136422
Waters, C. N., Zalasiewicz, J., Summerhayes, C., Barnosky, A. D., Poirier, C., Galuszka, A., et al. (2016). The Anthropocene is functionally and stratigraphically distinct from the Holocene. Science 351, aad2622. doi:10.1126/science.aad2622
Weiss, H. (2022). Pyramid building and collapse. PNAS 119 (37), e2212483119. doi:10.1073/pnas.2212483119
Wilkinson, B. H. (2005). Humans as geologic agents: A deep-time perspective. Geology 33, 161–164. doi:10.1130/G21108.1
Wilmshurst, J. M., Anderson, A. J., Higham, T. F. G., and Worthy, T. H. (2008). Dating the late prehistoric dispersal of Polynesians to New Zealand using the commensal Pacific rat. PNAS 105, 7676–7680. doi:10.1073/pnas.0801507105
Woodwell, G. M., Hobbie, J. E., Houghton, R. A., Melillo, J. M., Moore, B., Peterson, B. J., et al. (1983). Global deforestation: contribution to atmospheric carbon dioxide. Science 222, 1081–1086. doi:10.1126/science.222.4628.1081
World Meterological Organization (2023). Global annual to decadal climate update. World Meteorological Organization, 2023–2027. Available at: https://library.wmo.int/index.php?lvl=notice_display&id=22272.
Wuebbles, D. J. (2012). Celebrating the “Blue Marble”. EOS, Trans. Amer. Geophys. Union. 93, 509–510. doi:10.1029/2012EO490001
Yasuda, Y., Kitagawa, H., and Nakagawa, T. (2000). The earliest record of major anthropogenic deforestation in the Ghab Valley, northwest Syria: a palynological study. Quat. Int. 73–74, 127–136. doi:10.1016/S1040-6182(00)00069-0
Young, O. R. (2009). The political economy of the Northern Fur Seal. Polar Rec. 20, 407–416. doi:10.1017/S0032247400003612
Zachos, J. C., Lohmann, K. C., Walker, J. C. G., and Wise, S. W. (1993). Abrupt climate change and transient climates during the Paleogene: A marine perspective. J. Geol. 101, 191–213. doi:10.1086/648216
Zachos, J., Pagani, M., Sloan, L., Thomas, E., and Billups, K. (2001). Trends, rhythms, and aberrations in global climate 65 Ma to present. Science 292, 686–693. doi:10.1126/science.1059412
Zalasiewicz, J., Waters, C. N., and Williams, M. (2014). Human bioturbation, and the subterranean landscape of the Anthropocene. Anthropocene 6, 3–9. doi:10.1016/j.ancene.2014.07.002
Keywords: conservation biology, conservation paleobiology, hominins, near time, deep time, Holocene
Citation: Walker SE (2023) Conservation biology and conservation paleobiology meet the Anthropocene together: history matters. Front. Earth Sci. 11:1166243. doi: 10.3389/feart.2023.1166243
Received: 15 February 2023; Accepted: 11 September 2023;
Published: 28 September 2023.
Edited by:
Bruce S. Lieberman, University of Kansas, United StatesReviewed by:
Pamela Hallock Muller, University of South Florida, United StatesCarrie Tyler, University of Nevada, United States
Copyright © 2023 Walker. This is an open-access article distributed under the terms of the Creative Commons Attribution License (CC BY). The use, distribution or reproduction in other forums is permitted, provided the original author(s) and the copyright owner(s) are credited and that the original publication in this journal is cited, in accordance with accepted academic practice. No use, distribution or reproduction is permitted which does not comply with these terms.
*Correspondence: Sally E. Walker, swalker@gly.uga.edu