- 1Paleo[Fab]Lab, Earth Science Department, Dipartimento di Scienze della Terra, Università degli Studi di Firenze, Firenze, Italy
- 2Institut Català de Paleontologia Miquel Crusafont, Universitat Autònoma de Barcelona, Cerdanyola del Vallès, Spain
- 3NBFC, National Biodiversity Future Center, Palermo, Italy
Introduction: Paleontological heritage rarely receives the same legislative attention as archeological heritage. Part of the problem seems to be, at least in some contexts (e.g., Italy), the difficulty of acknowledging fossils as distinct assets from other cultural heritage objects and the lack of expertise among the administrative functionaries. This study aims to describe and test an objective analytic operational procedure that could allow for the assessment of the relevance of any fossiliferous site and its potential vulnerability in its present state.
Methods: The estimations were obtained using several quantitative and qualitative parameters that could describe the probability of fossil recovery for each site (Pr) and the scientific and cultural interest of the locality (In). Each parameter has subcategories for better defining. The product of probability and interest results in the scientific value (S), generally expressed as a percentage (S*). The vulnerability index (V) of productive and active sites considers the use of the land (U) and the natural erosion (E). The parameters were thought to apply to any type of fossil locality. We tested the indexes on 22 localities (7 of which were with paleoichnological records and 15 with direct body-fossil evidence), all different from one another.
Results: The results show that the proposed indexes well describe and characterize each locality. Most of the sites are moderately vulnerable (V between 1 and 3) except for large quarry sites (e.g., Pirro Nord, Italy and Solnhofen, Germany) whose vulnerability indexes fell in the maximum degree of risk.
Discussion: The operational procedure presented here is a simple, objective, and remotely applicable method allowing paleontologists and non-expert personnel to categorize localities and, therefore, act as a base to plan actions in paleontological heritage management relative to territorial development and land use.
1 Introduction
Every year, around the world, thousands of square kilometers of land are affected by development projects such as quarries, highways, and new agricultural fields (Hurtt et al., 2011; Merril and Leatherby, 2018; Ellis, 2021). Cultural heritage managers and administrative personnel, thus, must deal with the complex process of overseeing heritage asset spread (e.g., archeological, paleontological, or environmental ones) across the territory and safeguard and protect possible new findings. With the 1992 European Convention on the Protection of the Archaeological Heritage (also known as the “Valletta Treaty” or “Malta Convention”), most European countries have set the protection, preservation, and scientific research of archaeological heritage on top of their political agenda. One major consequence has been the flourishing of collaborative preventive archeology projects (Hofman and Hoogland, 2016), the designing of numerous archeological site evaluation tools (Ólafsdóttir and Dowling, 2009; Kavčič and Peljhan, 2010; Bujok et al., 2015; Goemaere et al., 2015; Sellier, 2016; Lukić et al., 2021), and the theorization of predictive models (Anschuetz et al., 2001; Stančič et al., 2001; Kamermans et al., 2009; Danese et al., 2013; Danese et al., 2014). The effort is not limited to Europe but is also applied globally in extra-European countries (Nikami, 2007; Hadjimitsis et al., 2013).
Unlike archeological patrimony, the paleontological heritage is often overlooked by the legislation of many countries. The main reason for this neglect is strictly linked to the complex dichotomous nature of paleontological heritage. According to the 1970 UNESCO Convention of the Means of Prohibiting the Illicit Import, Export, and Transfer of Ownership of Cultural Property (UNESCO, 1970), the term cultural heritage encompasses several main categories of heritage, such as “rare collections and specimens of fauna, flora, minerals, anatomy, and objects of paleontological interest.” On the other hand, the term “natural heritage” includes “natural sites with cultural aspects such as cultural landscapes and physical, biological, or geological formations” (UNESCO, 2022). Since the fossil record is tightly connected and inseparable from the geological environment, it can be argued that the protection of fossil resources could be framed as part of the natural heritage because fossils are natural objects, and not created by humans (Prado, 2008). Nevertheless, based on the statements within the 1970 UNESCO convention (UNESCO, 1970), many countries’ legislations consider paleontological heritage as included under the definition of “cultural heritage.” For this reason, in legal terms, paleontological resources are equated to objects with different origins and nature, like archeological and historical artifacts (Alcalà and Morales, 1994; Prado, 2008; Guerrero-Arenas et al., 2020).
In addition to the complex nature of fossils and the old-fashioned legislation of many countries (e.g., Italy), we need to add that among the administrative personnel and the heritage managers, professional experts in paleontology are missing (and in most cases, there are not even experts in biological or Earth sciences), thus, the personnel responsible for the site is often unable to manage the paleontological heritage with the appropriate attention and scientific background. All these factors contribute to hindering the proper tutelage of the “immovable” paleontological heritage, both historically known fossil sites or newly discovered fossiliferous localities.
In this sense, it is fundamental to provide government agencies with an operational procedure capable of operatively categorizing sites according to their paleontological relevance and thus offering protection for proper conservation and management in a cost-effective way.
In the past, several models concerning the evaluation of the paleontological heritage have been proposed (Alcalá and Morales, 1994; Morales, 1996; Morales et al., 1999; Oheim, 2007; Hayward, 2009; Mampel et al., 2009; Endere and Prado, 2015; Canudo et al., 2021; Morey and Pons, 2021; García-Ávila et al., 2022). However, they were all designed to be applied to specific countries or specific fossil-rich regions, thus resulting in them often being too specific and/or not useful to be extended to a broader set of different situations/conditions.
This research paper describes a new and innovative analytic operational procedure to measure the potentiality of fossil recovery in one site and, at the same time, assess the risk to which such a site is, or might be, subject. Furthermore, this operational procedure could promote paleontological heritage, raising awareness of its relevance and value and, by doing so, favoring its protection. To define the analytic operational procedure, we selected a number of significant parameters that allow the synthetic yet objective description and estimation of the importance of the paleontological heritage (specifically the scientific, educational, and historical values). Furthermore, the parameters were subdivided into data categories easily retrievable by consulting relevant bibliography and through the use of GIS software. Two sets of parameters were defined to differentiate between track sites (indirect fossil evidence) and localities with body-fossil remains (direct evidence). A value was assigned to each parameter based on predefined categories to characterize the selected fossiliferous locality. The parameters were then merged to calculate four different indexes to make a quick, objective, and comprehensive evaluation of the relevance and vulnerability of the site.
The aim of this project was to define an operational procedure applicable to any fossil locality from anywhere in the world; the parameters were adjusted to be easily evaluated by paleontologists as well as non-specialized technical personnel, thus minimizing the subjective component in the evaluation process. With this operational procedure, it is possible to have a simple and objective starting base to evaluate a site’s relevance and take actions on paleontological heritage management relative to territorial development.
2 Materials and methods
2.1 Experimental base
The necessity of designing an analytic operational procedure to evaluate the importance and vulnerability of each fossil locality arose within a larger project granted by the Regione Toscana and the Università degli Studi di Firenze aiming at the valorization of the Tuscan paleontological heritage and, in particular, the Grosseto province, selected as a test case for its diversity of sites in a single, limited area.
Managing a sizable database to obtain direct information on paleontological aspects of the various fossil localities required the definition of a series of parameters capable of arranging and analyzing the increasing amount of available data and geographical information. The parameters for the scientific value index were selected (Tables 1, 2) taking into consideration the previous experiences of inventory and classification of archeological and paleontological heritage (Alcalá and Morales, 1994; Morales, 1996; Morales et al., 1999; Hayward, 2009; Mampel et al., 2009; Ólafsdóttir and Dowling, 2009; Kavčič and Peljhan, 2010; Bujok et al., 2015; Endere and Prado, 2015; Goemaere et al., 2015; Sellier, 2016; Canudo et al., 2021; Lukić et al., 2021; Morey and Pons, 2021; García-Ávila et al., 2022) but were also based on the requirements identified during the data collection and the survey carried out for this study.
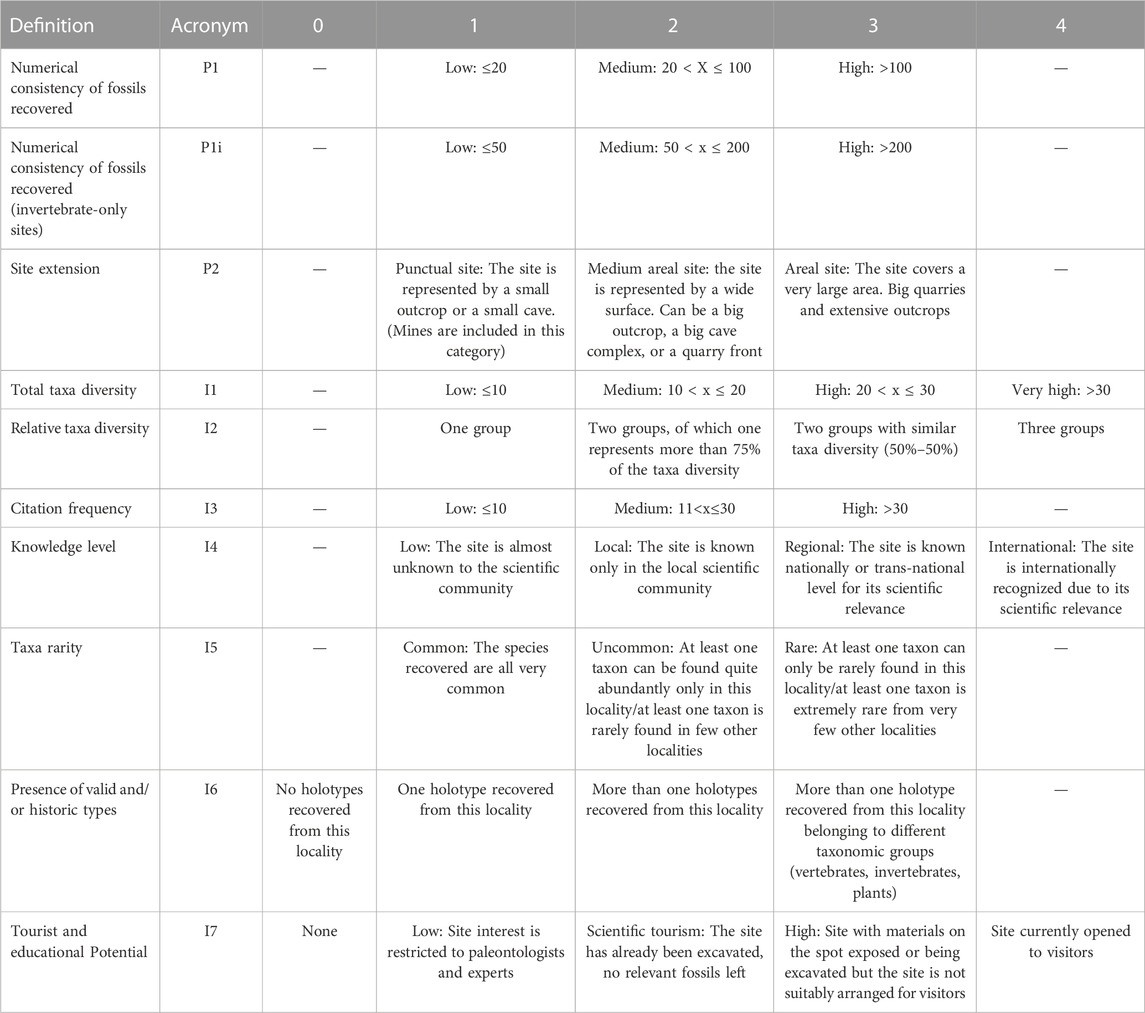
TABLE 1. Variables for the calculation of the site relevance index (R) of sites with fossil remains.
2.2 Proposed methodology
The proposed methodology for the estimation of the scientific value and vulnerability of fossil sites (Figure 1) is composed of 13 different parameters grouped into three different categories (Tables 1–3): discovery probability (Pr), site interest (In), and vulnerability (V). These parameters were combined to calculate four different indexes.
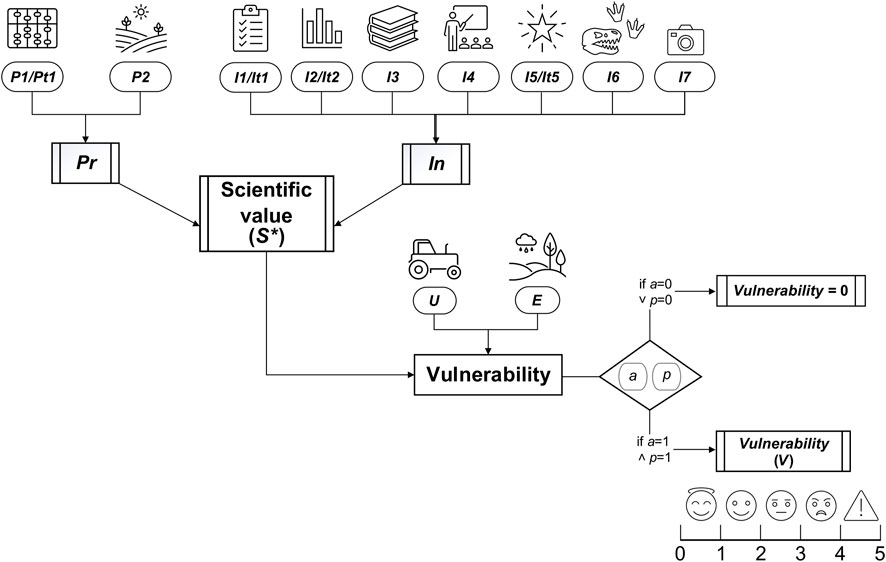
FIGURE 1. Schematic representation of the methodological proposal to assess the scientific value and vulnerability of paleontological sites. For abbreviations, see the text. Caption: in normal characters - the conceptual objectives of the estimations (i.e., the relevance and the vulnerability of each site); in italics - parameters and discriminants (ovals) and indexes (double-lined rectangles); and the rhombus represents a decision affecting the estimation of vulnerability, i.e., depending on the parameters active/inactive (a) and productivity (p). “˅” and “˄” represent logical connectors. The scale below vulnerability (V) schematically expresses the intensity of the vulnerability index, from 0–1 (low concern) to 4–5 (extreme vulnerability).
Despite applying a similar methodology to evaluate track and body-fossil sites, the variable list (Tables 1 and 2) shows some differences in parameters between the two types of fossiliferous localities. Sites yielding skeletal remains differ from those with trace fossils in terms of some of the discovery probability and site interest parameters. Each parameter is, in turn, characterized by a number, usually an integer, that defines the characteristics of the selected fossil site. The parameters that make up the discovery probability (Pr) are the numerical consistency of fossils recovered (P1) and the site extension (P2). For localities with fossil traces, the P1 parameter is modified as the numerical consistency of traces recovered (Pt1).
As the name implies, numerical consistency (P1) is simply the number of single fossil elements or traces recovered from a site. Unidentifiable bone fragments and unidentifiable plant matter are not included in the calculation. The threshold values established to separate the different categories of this parameter were defined in previously published studies (Mampel et al., 2009; Endere and Prado, 2015). In the case of a fossiliferous locality bearing solely invertebrate remains, doubling the threshold values of each category (P1i) was deemed better, thus avoiding giving too much value to the common shell beds we can often find in marine/lacustrine formations while not belittling truly important invertebrate sites.
The site extension (P2) parameter defines the areal extension of the fossiliferous locality. Since it is often difficult to truly quantify the area covered by a site, we did not define the categories using areal units, but we preferred giving a brief and easy-to-interpret description of the different types of fossil localities that can be normally found. Therefore, we described punctual sites as very small outcrops not extending over a few square meters; very small caves also fall into this definition. Medium-sized outcrops, small quarry fronts, and big cave complexes belong to the second category, the medium areal site. We included mines in this second category. The third category, called areal site, includes only very large fossiliferous localities that can be easily mapped in large-scale maps, such as huge quarries or extensive outcrops.
These two parameters add up to calculate the discovery probability index (Pr):
For localities with fossil tracks, the equation simply becomes
Through the discovery probability (Pr), investigators can easily elaborate on the richness and extension of the fossiliferous locality.
The site interest (In) category is composed of seven different parameters (Table 1): total taxa diversity (I1), relative taxa diversity (I2), citation frequency (I3), knowledge level (I4), taxa rarity (I5), presence of valid and/or historic types (the site is a type locality of some taxa) (I6), and tourist and educational potential (I7). For localities with fossil traces, the parameters are (Table 2) variety of ichnogroups and/or ichnotypes (It1), number of trackways (It2), citation frequency (I3), knowledge level (I4), length of trackways (It5), presence of valid and/or historic types (I6), and tourist and educational potential (I7).
The total taxa diversity (I1) defines the number of different taxa identified in a site. Less-specific identifications (e.g., “Cheloniidae index”) are also included in the count. Similarly, the variety of ichnogroups and/or ichnotypes (It1) defines the number of ichnotaxa identified on the site.
For the parameter relative taxa diversity (I2), we divided the fossil remains into invertebrates, vertebrates, and plants and considered their relative abundance in each site. We decided to attribute higher values to localities that present two or more of these groups as they can offer a better ecological insight into the paleoenvironment and, thus, have a higher scientific relevance.
The citation frequency (I3) was used to define how relevant a site is for the scientific community. To do so, a simple and effective way is to use the Google Scholar database. Launched in 2004, this search engine is a useful tool, helping researchers locate in seconds relevant research papers from billions of pages across the Web and in many cases directly retrieve the full text of those studies (Hightower, 2010; Giustini and Kamel Boulos, 2013). A reliable objective figure of the number of studies focused on the site was obtained using online article repositories (e.g., Google Scholar, Web of Science, and Scopus). These websites report the number of references citing each paper; here, we used the first and/or most representative published research paper on the selected locality, divided by the number of years that have passed since the publication of that paper. The obtained estimation is the frequency of citation of that paper and, as a result, how much the scientific interest for a specific locality fluctuated through time. Using a ratio instead of the sheer citation number, we avoided the clear “advantage” that renowned fossiliferous localities have over recently discovered localities. When the study is too old to be found correctly cited in Google Scholar or Scopus (e.g., Solnhofen fauna was described in the 19th century), the most cited research paper or the most representative one can be used instead.
Knowledge level (I4) considers the scientific and public outreach of a fossil site, factoring in the number of articles in national and/or international journals, documentaries, books, or articles in local newspapers.
Taxa rarity (I5) is used to report the occurrence of rare and/or very rare taxa, supporting the cultural and scientific value of the site. Similarly, the presence of valid and/or historic types (I6) allows giving credit to localities that have produced type specimens and are, therefore, historically relevant.
Finally, tourist and educational potential (I7) evaluates the tourist attractiveness of the site, factoring in the accessibility to the site, the presence of guided tours, and the overall public interest in the locality.
In the case of localities with fossil traces, relative taxa diversity (I2) and taxa rarity (I5) are substituted by the number of trackways (It2) and length of trackways (It5). This choice was made following specific scientific literature: the wider the representation and the variety of trackways, the easier it is to establish comparative trends and reach more solid and proven conclusions (Mampel et al., 2009; Falkingham et al., 2016). This, in turn, reflects the scientific and cultural value of the site.
The length of trackways (It5) is calculated differently according to the nature of the organism that has produced the tracks, following common practices of ichnologists (among others, Melchor and Cardonatto, 2014; Moreau et al., 2021). For vertebrate trackways, the length is based on the number of steps preserved, whereas for invertebrates, the length is based on the metric length of the track. The threshold values for the vertebrate trackways were taken from the work of Mampel et al. (2009), while the quantitative evaluation of the invertebrate trackway length was based on extensive related literature (Buta et al., 2005; Minter et al., 2007; Melchor and Cardonatto, 2014; Moreau et al., 2021).
The sum of these seven parameters produces the site interest index:
For localities with fossil tracks, the equation becomes
The site interest index could summarize the characteristics of a fossiliferous locality with a few simple and easy-to-answer questions.
The combination of the discovery probability index and the site interest index defines the scientific value index as a more comprehensive and exhaustive parameter to evaluate the significance of a site. The maximum value obtainable with the scientific value (S) in our method is 156, but it is usually expressed as a percentage (S*):
The final category contains all the parameters that contribute to defining, together with the indexes defined above, the vulnerability index (Table 3). The parameters included in this index are productivity (p), active/inactive (a), site use (U), and natural erosion (E). These parameters do not change between sites with body-fossil remains and those with fossil traces.
Productivity (p) and active/inactive (a) are discriminants as they can only assume values of 0 or 1. They are needed in the final calculation of the vulnerability index score cases as sites that are no longer productive or accessible. In the case of fossil localities that were completely exploited, while they still maintain historical and scientific value, they do not require any specific protection. Similarly, a fossiliferous locality that, for an anthropic or natural cause, can no longer be reached (e.g., a disused and collapsed mine, an outcrop covered by a landslide, and/or a site already lost due to an urban development project) does not require protection.
Site use (U) defines the actual use of the land where fossiliferous remains or traces have been found and how much its use could affect the integrity and preservation of the fossil remains contained in the site. The value of this parameter varies from 0, in the case of a site completely musealized, to 5 for sites subjected to high anthropic use, such as quarrying or urbanization. Intermediate values refer to sites located in areas with different degrees of anthropization (see Table 3 for the complete list).
Natural erosion (E) is used to describe the intensity of erosion to which the site is subject. Land erosion is often influenced by many different contributing factors, such as lithology, land cover, soil susceptivity, topography, etc.) (Zachar, 1982; Renschler and Harbor, 2002), often resulting in peculiar local effects. In the last decades, several models were developed to assess erosion in different parts of the world, even at different spatial and temporal scales (Tangestani, 2006; Sheik Mujabar and Chandrasekar, 2013; Panagos et al., 2015; Borrelli et al., 2017; Nearing et al., 2017; Uddin et al., 2018; Alewell et al., 2019; Bhattacharya et al., 2019; Ciampalini et al., 2020; Wuepper et al., 2020; Ferreira et al., 2022), especially considering its profound socio-economic impact (Panagos et al., 2018; Tsegaye, 2019). Such evaluation procedures are based on the possibility of retrieving reliable data from third-party, official, and often institutional services. One example is the United Nations Inter-Governmental Technical Panel on Soils of the Global Soil Partnership (https://www.fao.org/global-soil-partnership/itps/en/), a working group made of top experts representing all the regions of the world. Despite the challenges, advantages, and distinctions of the various models, data on factors controlling land erosion are often readily available for many geographic regions (Canuti et al., 2000; Borrelli et al., 2021; Poggio et al., 2021), thus enabling such estimations even for decision-making personnel (Renschler and Harbor, 2002; Borrelli et al., 2021; Ferreira et al., 2022). Models even manage to make predictions of future erosion at different scales (Catani et al., 2010; Borrelli et al., 2020; Panagos et al., 2021). Therefore, despite the well-known issues of the methodologies (Batista et al., 2019), the quantification of erosion remains a key calculable parameter for land-managing and development programs. Even in the vulnerability assessment of a paleontological site, erosion should not be disregarded. Although, compared to the aforementioned methods, simpler categorization could suffice. Indeed, previous studies in paleontology (Oheim, 2007; Mampel et al., 2009) used data available from local institutional administrations and geologic services reporting erosive potentiality at a local or regional scale (Aragón Territorial Centre of Information and Documentation; Mampel et al., 2009). Such estimations include all different sources of erosion, e.g., water, wind, biological, and anthropogenic. This information was converted by these authors into simple qualitative categories.
In our analysis, we directly surveyed some of the sites considered (e.g., Cornate di Gerfalco, Pirro Nord, Dmanisi) and qualitatively evaluated the degree of potential erosion on some considerations (e.g., formation type and climate). Second, we compared our evaluation with institutional data of the area to refine them. Finally, we contrasted our assessment with that of scientific literature and established discrete qualitative categories to be used for the gross assessment of the vulnerability of each site. Non-numerical variables defining erosion also allow adapting different and non-comparable records: the same amount of erosion per year can be considered as a “moderately low” degree of erosion in some regions, while in others, it could be “moderate” or high. For instance, in the central and southern regions of the Italian Peninsula, we have this discrepancy: 5–10 tons per acre per year is a “low” erosion potential in Tuscany, while the same amount is a “moderate” one in Apulia (Montanarella et al., 2000). This being considered, a qualitative definition of natural erosion (E) prevents this incompatibility. The values of parameter E here adopted range from 0 if the site is protected from natural erosion to 3 for sites affected by severe weathering. In the absence of any data for the considered locality, we followed Mampel et al. (2009), defining a minimum value of weathering equal to 0.5.
Based on all the aforementioned and defined parameters and indexes, the vulnerability index (V) can be computed as follows:
The vulnerability scale is subdivided into five different intervals (Table 4) and ranges from 0 for protected and musealized sites to 5 in the case of highly vulnerable sites. The vulnerability index equation can produce values slightly higher than 5, but they are all reconducted to the maximum range of the vulnerability scale, as all values higher than 4 represent high-risk situations that require immediate intervention.

TABLE 4. Ranges of vulnerability calculated with Eq. 6.
2.3 Case studies
To prove the simplicity and effectiveness of the indexes, we tested them on a variety of different fossiliferous localities. We selected 15 localities with fossil remains and seven localities with fossil traces, ranging from the Early Paleozoic to the Late Cenozoic (Figure 2; Tables 5). Seven of the 22 localities considered here were personally visited by the authors and contributed to the definition of the methodology presented. The different localities were selected from all around the world, regardless of their paleontological content, to further test the reliability of the results. All necessary data to compile the previous equations were recovered from bibliographies and through GIS methodologies and, in some of the case studies, direct visits to the sites (Table 5). The complete list of the selected localities is reported in Table 5.
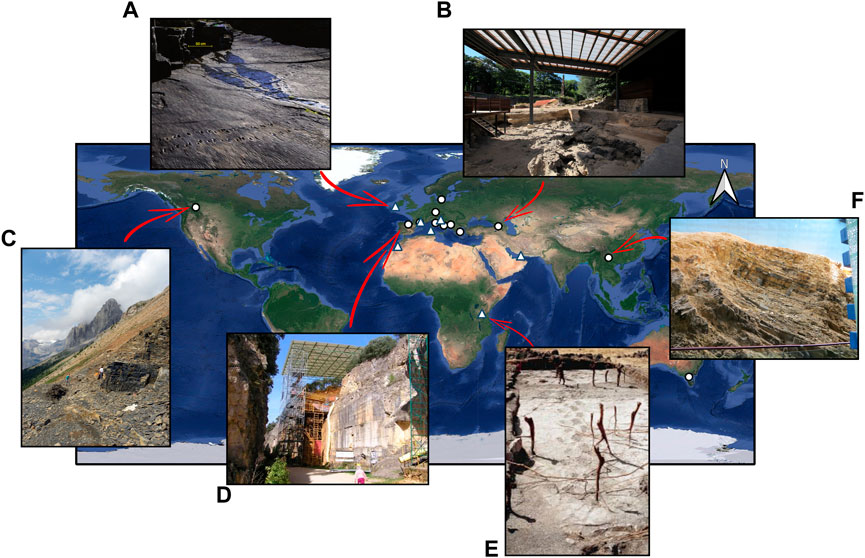
FIGURE 2. Location of the selected localities used to test the new analytical procedure. Caption: white circles: localities with fossil remains; white triangles: tracksites. Photos of some of the localities: (A) Dohilla tracksite (modified from the work of Stössel et al., 2016); (B) Dmanisi (photo by Saverio Bartolini-Lucenti); (C) Walcott quarry (Mark A. Wilson, Department of Geology, The College of Wooster, Public Domain); (D) Gran Dolina site in Atapuerca (photo by Mario Modesto Mata, own work, CC BY-SA 3.0); (E) Laetoli site during the 1994 conservation project (modified from the work of Agnew and Demas, 1998); and (F) Maotianshan musealized site (Martin Smith, own work, CC BY-SA 4.0).
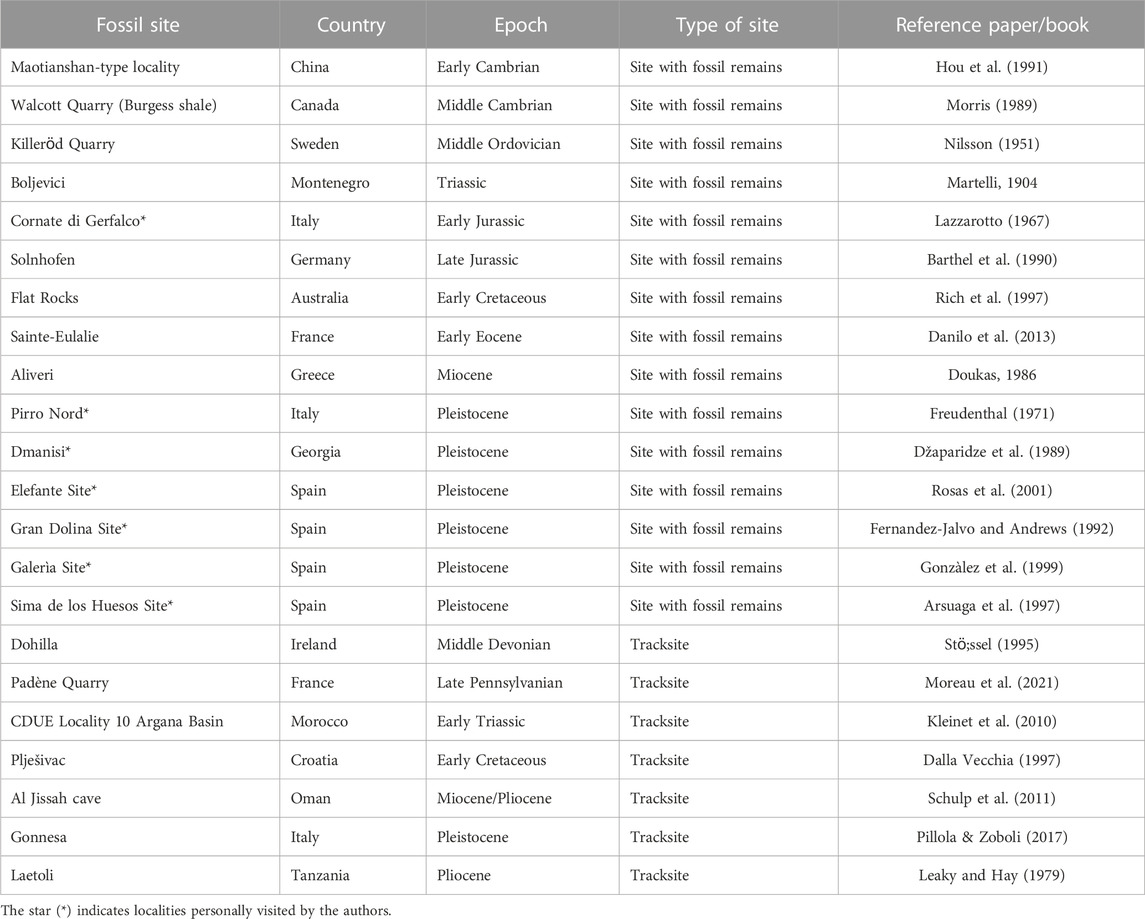
TABLE 5. List of selected localities to test the proposed methodology. The reference paper column reports the studies used to define the historical relevance parameter of each site.
3 Result and discussion
The use of the proposed parameters and the indexes obtained proved consistent and useful to synthesize and organize the relevance and the deterioration risk of each of the selected sites (Tables 6, 7). The results show a wide variety of values, correctly gathering the array of characteristics possessed by each site and expressing them and their influence on the obtained scientific value and vulnerability. On-site observations of some of the localities (Table 5) helped with the calibration of several parameters.
World-renowned localities such as Maotianshan (Chengjiang Biota, Early Cambrian; China), Walcott Quarry (Burgess Shale Biota, Middle Cambrian, Canada), Flat Rocks (Early Cretaceous, Australia), Solnhofen (Late Jurassic, Germany), Dmanisi (Early Pleistocene, Georgia), Laetoli (Plio-Pleistocene, Tanzania), Pirro Nord (Early Pleistocene, Italy), and the four Atapuerca sites (early Middle Pleistocene, Spain) have all produced, as expected, very high scientific value indexes despite being sites with very different characteristics (Barthel et al., 1990; Gould, 1990; Gabunia and Swisher, 2001; Rook, 2013; Hou et al., 2017; Kooland and Lowery, 2020). The variation among the values of these fossiliferous localities is mostly due to the extension of the site and the presence/absence of holotypes. While the musealized site of Maotianshan and the Walcott Quarry are small outcrops, Laetoli, Solnhofen, and Pirro Nord quarries have an area of a few square kilometers. The other sites show a broad range of scientific value indexes (Tables 6-7), from the low values of Cornate di Gerfalco (Early Jurassic, Italy), Al Jissah Caves (Miocene/Pliocene, Oman), Gonnesa (Pleistocene, Italy), and CDUE Locality 10 (Early Triassic, Morocco) to the medium–high values of Padène (Carboniferous, France), Aliveri (Miocene, Greece), Dohilla (Middle Devonian, Ireland), and Galerìa (Pleistocene, Spain). These sites generally have a lower touristic and educational potential, or they have produced a lower variety of fossils/trackways.
The scientific value index partially influences the results of the vulnerability index since a site with a higher relevance must be, in principle, more carefully protected. Nevertheless, each site is subjected to different risk factors, making their vulnerability vary considerably. Musealized sites such as Maotianshan, Dmanisi, and Atapuerca have already been adequately protected. Therefore, their vulnerability index drops to 0. For example, the Pleistocene sites in Sierra de Atapuerca provided exceptional findings for understanding the first steps of human evolution in Europe (Ortega et al., 2014). For this reason, in 2000, the site was included in the UNESCO World Heritage Site list, and, in 2002, the Junta de Castilla y León approved the Guidelines for Using and Managing the property that included specific measures for safeguarding, conservation, research, and promotion of the site (UNESCO, 2021).
The estimation for Aliveri also produced a vulnerability index of 0. Though this is a “positive” figure, this results from the loss of the original fossiliferous outcrop, which was completely covered by the construction of several buildings in the 1990s (Georgalis et al., 2019). Given that the site is no longer active, V, thus, drops to 0.
Other localities such as Cornate di Gerfalco, CDUE Locality 10, Laetoli, and Al Jissah Cave all produced very low vulnerability indexes as they all have low scientific value (except for Laetoli). The Al Jissah site is located in a cave underneath a cliff on the Musandam Peninsula (Oman). According to Schulp et al. (2011), this site is poorly preserved and virtually unreachable if not by a small boat. Even granting the active/inactive parameter to be 1, the vulnerability index remained close to 0 due to the reduced extension of the site and the low touristic and cultural potential of the site. The Cornate di Gerfalco site combines a low scientific value index with the fact that the site is already partially protected, as it is included within a regional natural reserve (the “Riserva Naturale Regionale Cornate e Fosini”), and thus it is characterized by a reduced vulnerability. The on-site observation of the pattern of erosion of this quarry indicates a low but active degree of weathering of the ‘Rosso Ammonitico’ formation (an ammonite-rich limestone). The geological service of Tuscany (Geoscopio, www.regione.toscana.it/-/geoscopio) reports a value of “moderate potential erosion” (∼20 t/ha; 3–4 on a scale of 8). This confirms the qualitative on-field assessment.
Laetoli is probably the most famous tracksite locality in the world (Greshko, 2016; Wei-Haas, 2021). It is best known for the discovery of important fossil remains and ichnological traces of early hominins (White and Suwa, 1987; McNutt et al., 2021), but it has also produced thousands of different vertebrate and invertebrate traces, including several ichnotypes (Meldrum et al., 2011; Genise and Harrison, 2018). The first excavation campaign in Laetoli footprints ended in 1979, and the paleontologist team used the local river sand to rebury the site. The mound of sand was then covered with volcanic boulders to shield it against erosion and the animals. The loose fill and the physical protection and moisture retention provided by the boulders promoted the re-vegetation by acacia trees, which later gave rise to fears over root growth. In 1994, a new conservation project began: the trees and shrubs growing on and near the reburial mound were cut down, and the trackway was re-excavated, stabilized, and reburied with synthetic geotextile materials (Agnew and Demas, 1998). The site is, therefore, well-protected even if not properly musealized yet.
Many localities produced intermediate values of vulnerability, ranging from 1.1 to 2.9 (corresponding to medium–low and medium values of V). These values mark sites that are moderately in danger of destruction. They can be world-renowned localities that are not or cannot be adequately protected (e.g., Walcott Quarry, Flat Rocks, and Dohilla) or less relevant localities that are subjected to stronger natural and/or anthropic erosive phenomena, such as Killerӧd Quarry, Middle Ordovician, Sweden; Boljevici, Triassic, Montenegro; Sainte-Eulalie, Early Eocene, France; Padène Quarry, Late Pennsylvanian, France; and Plješivac, Early Cretaceous, Croatia.
The Walcott Quarry is probably among the most famous Paleozoic fossil localities in the world, having produced the world-renowned Burgess Shale biota, beautifully narrated by the pen of S. J. Gould (Gould, 1990). The site was registered as a World Heritage Site in 1980, and as an IUGS Geological Heritage Site in 2022 (IUGS, 2022). However, the site is still exposed to the strong Canadian atmospheric phenomena. Thus, it could benefit from a covering roof similar to what has been done in the Maotianshan site (Hou et al., 2017), which is also a UNESCO World Heritage Site.
Flat Rocks is another site with a good scientific value index for its abundant and diversified Barremian fauna (Rich et al., 1997; Kooland and Lowery, 2020). The fossiliferous locality is included in the Bunurong Marine National Park (Intergovernmental Committee on Surveying and Mapping, 2014) and consists of a series of ∼1 m deep excavations on the coastal, wave-eroded rock platform (Herne et al., 2019). Due to its peculiar position, the site is subject to continuous erosional forces by tides, and this makes the site very difficult to preserve. For this reason, since its discovery in 1992, every year, Monash University organizes excavation campaigns, which follow a detailed annual field report (Monash University, 2023).
The other localities, such as Killerӧd Quarry, Boljevici, Sainte-Eulalie, Padène Quarry, and Plješivac, all have intermediate scientific value as they all have local or regional relevance, and their touristic and educational potential is quite low. This can produce different situations of risk, especially when combined with the fact that Killerӧd Quarry and Padène Quarry are abandoned quarries (Månsson, 1995; Moreau et al., 2021) while Boljevici and Sainte-Eulalie (Danilo et al., 2013; Ðaković et al., 2015) are outcrops situated on rockwalls. Moreover, these fossiliferous localities are not included in protected areas and, therefore, could be subjected to urbanization projects in the future.
The Albian Plješivac site is located in the southern part of the main Brioni/Brijun Island (southwestern Istria). It is made up of two wide bed surfaces cropping out along the coast and sloping toward the inner part of the island. The site is included in the Brijun Islands National Park and most probably was an ancient quarry (Dalla Vecchia, 1997; Mezga and Bajraktarević, 2004). The footprints are usually submerged during the highest tides, and in summer, the island and its coasts are famous tourist spots. Compared to what happens on the Flat Rock site, due to its peculiar position, the conservation of the site is complicated. However, as Bajraktarević and Mezga (2004) suggested, the Plješivac site and the other sites on the Brioni/Brijun island can become an important destination for scientific tourism.
Finally, only two fossiliferous localities have produced very high values of the vulnerability index: Pirro Nord and Solnhofen. These two sites are characterized by very high scientific value indexes as they are both among the most renowned fossiliferous localities of Europe.
Pirro Nord lies in the municipality of Apricena (Foggia, southern Italy), in one of the quarries exploiting the Mesozoic “Calcare di Bari” formation. The paleontological record is preserved inside a karst network at the top of the Mesozoic limestones and filled with continental sediments (Abbazzi et al., 1996). The gallery and sinkhole fillings have been extensively investigated in the last 30 years, and after the pioneering report by Freudenthal (1971) and the study by Beaumont (1976), more than 100 taxa have been recognized (Pavia et al., 2012), together with lithic artifacts representing the earliest known human occupation of Europe (Arzarello et al., 2007; 2009; Arzarello and Peretto, 2010; Pavia et al., 2012). The direct observation of Pirro Nord quarry pointed out that erosion in the area is fairly low as “Calcare di Bari” Fm. is subject to limited climatic or natural erosive agents except for the anthropogenic one (i.e., the quarrying activity). Therefore, we opted for a value of E of 1 (“active and low”). When we compared our interpretation with the erosion estimation models applied to the area, we found limited erosion potential for the Pirro Nord area (Montanarella et al., 2000). This confirmed our on-site evaluation.
The main quarry area of Solnhofen lies in the surroundings of the town of Eichstätt, between the villages of Solnhofen, Langenaltheim, and Mörnsheim in the district of Weißenburg-Gunzenhausen (Bavaria, Germany). Here, limestone slabs have been quarried for centuries not only because they are a building stone of high quality, but also because of their use as a lithographic stone and the exceptionally well-preserved fossils they contain (Kölbl-Ebert and Cooper, 2020).
The fossils of the Solnhofen Limestone have been the object of numerous investigations. Among them, the celebrated Archaeopteryx lithographica played a major role in research on the evolution of birds, while the preserved flight membranes of pterosaurs have provided clues on their physiology (Bennet, 1995; Bennet, 2002; Erickson et al., 2009; Foth et al., 2014; Vidovic and Martill, 2014, and references therein). For this reason, the International Union of Geological Sciences (IUGS), in 2022, included the “Jurassic Solnhofen-Eichstätt Archaeopteryx Serial Site” in its selection of 100 “geological heritage sites” around the world (IUGS, 2022). Despite this important acknowledgment, Solnhofen is still an active quarry (as well as Pirro Nord). Such an impactful use of the land threatens the paleontological heritage of these sites.
4 Conclusion
In the near future, local, regional, and national administrations must increase their attention to paleontological heritage management. Issues to be overcome remain, e.g., the contested attribution of the nature of fossils, which in turn leads to obsolete definitions in regional/national laws. Another unresolved issue is the difficulty of the administrative personnel, lacking proper paleontological expertise, in understanding the value of fossil assets and the need for particular actions of tutelage. Objective indexes capable of synthesizing and categorizing fossiliferous localities would be an effective aid in attenuating these problems.
The proposed parameters were selected to be as simple and objective as possible so that administrative personnel and heritage managers, who cannot always directly visit the fossil localities, could use them, even without geological expertise or background. The criteria and indicators proposed here are adequate to be used both remotely and with direct observations. We found that direct observations of ongoing phenomena, such as natural erosion, are confirmed by bibliographic data. A review of the scientific literature of the interested area would suffice to score all the parameters, estimate the scientific value of each site, and rank them according to their vulnerability.
The stepwise methodology proposed here thus becomes a valuable support tool for the decision-making process to help develop reasonable interventions such as emergency fossil excavations, prospecting works, and detecting sites that could be of greater public interest.
The results obtained for the selected localities help quantify the threats affecting them, hopefully prompting actions by local or public administrations to preserve their integrity. Furthermore, the values obtained for each fossiliferous locality should be constantly updated as the characteristics of a site and its vulnerability can change through time.
This new site characterization sets a number of new challenges and opportunities for paleontological heritage protection. The combination of scientific value and vulnerability indexes with data obtained with GIS methods might produce paleontological vulnerability assessment maps, useful when programming land development projects, or even cultural relevance maps. This could altogether fuel scientific and tourist interest in and to local and regional communities.
Data availability statement
The original contributions presented in the study are included in the article/Supplementary Material; further inquiries can be directed to the corresponding authors.
Author contributions
All authors contributed to the study's conception and design. Data collection, methodology, and analysis were performed by AF and SB-L. The first draft of the manuscript was written by AF, and all authors commented on previous versions of the manuscript. All authors contributed to the article and approved the submitted version.
Funding
AF and SB-L were supported by the Tuscany Region funds for young researchers “Giovanisì” (www.giovanisi.it): “POR FSE 2021/27” funds for the “Dottorato Regionale in Scienze della Terra (Programma Pegaso)” to AF and “POR FSC 2014-2020” funds for “Progetti di alta formazione attraverso l’attivazone di Assegni di Ricerca” to SB-L.
Acknowledgments
The authors thank Matteo Belvedere for his useful suggestions. The authors acknowledge the support of NBFC to the University of Florence (Earth Sciences Department), funded by the Italian Ministry of University and Research, PNRR, Missione 4 Componente 2, “Dalla ricerca all’impresa,” Investimento 1.4, Project CN00000033.
Conflict of interest
The authors declare that the research was conducted in the absence of any commercial or financial relationships that could be construed as a potential conflict of interest.
Publisher’s note
All claims expressed in this article are solely those of the authors and do not necessarily represent those of their affiliated organizations, or those of the publisher, the editors, and the reviewers. Any product that may be evaluated in this article, or claim that may be made by its manufacturer, is not guaranteed or endorsed by the publisher.
References
Abbazzi, L., Benvenuti, M., Boschian, G., Dominici, S., Masini, F., Mezzabotta, C., et al. (1996). The neogene and Pleistocene succession, and the mammal faunal assemblages of an area between Apricena and Poggio imperiale (foggia, Italy). Mem. Soc. Geol. It. 51, 383–402.
Agnew, N., and Demas, M. (1998). Preserving the Laetoli footprints. Sci. Am. 279 (3), 44–55. doi:10.1038/scientificamerican0998-44
Alcalá, L., and Morales, I. (1994). “Towards a definition of the Spanish palacontological heritage,” in Geological and landscape conservation. Editors D. O’halloran, C. Creen, M. Hartes, M. Síanley, and K. Knill (London: Geological Society), 57–61.
Alewell, C., Borrelli, P., Meusburger, K., and Panagos, P. (2019). Using the USLE: Chances, challenges and limitations of soil erosion modelling. Int. Soil Water Conserv. Res. 7, 203–225. doi:10.1016/j.iswcr.2019.05.004
Anschuetz, K. F., Wilshusen, R. H., and Scheick, C. L. (2001). An archaeology of landscapes: Perspectives and directions. J. Archaeol. Sci. 9 (2), 157–211. doi:10.1023/a:1016621326415
Arsuaga, J. L., Martınez, I., Gracia, A., Carretero, J. M., Lorenzo, C., Garcıa, N., et al. (1997). Sima de los huesos (Sierra de Atapuerca, Spain). The site. J. Hum. Evol. 33 (2-3), 109–127. doi:10.1006/jhev.1997.0132
Arzarello, M., Marcolini, F., Pavia, G., Pavia, M., Petronio, C., Petrucci, M., et al. (2007). Evidence of earliest human occurrence in Europe: The site of Pirro Nord (southern Italy). Naturwissenschaften 94, 107–112. doi:10.1007/s00114-006-0173-3
Arzarello, M., Marcolini, F., Pavia, G., Pavia, M., Petronio, C., Petrucci, M., et al. (2009). L’industrie lithique du site pléistocène inférieur de Pirro Nord (Apricena, italie du Sud): Une occupation humaine entre 1,3 et 1,7 ma. L’Anthropologie 113, 47–58. doi:10.1016/j.anthro.2009.01.004
Arzarello, M., and Peretto, C. (2010). Out of africa: The first evidence of Italian peninsula occupation. Quat. Int. 223-224, 65–70. doi:10.1016/j.quaint.2010.01.006
Barthel, K. W., Swinburne, N., and Conway Morris, S. (1990). Solnhofen: A study in mesozoic palaeontology. Cambridge: Cambridge University Press.
Batista, P. V., Davies, J., Silva, M. L., and Quinton, J. N. (2019). On the evaluation of soil erosion models: Are we doing enough? Earth-Sci. Rev. 197, 102898. doi:10.1016/j.earscirev.2019.102898
Bennett, S. C. (1995). A statistical study of Rhamphorhynchus from the Solnhofen Limestone of Germany: Year-classes of a single large species. J. Paleontol. 69, 569–580. doi:10.1017/s0022336000034946
Bennett, S. (2002). Soft tissue preservation of the cranial crest of the pterosaur Germanodactylus from Solnhofen. J. Vert. Paleontol. 22 (1), 43–48. doi:10.1671/0272-4634(2002)022[0043:stpotc]2.0.co;2
Bhattacharya, R. K., Das Chatterjee, N., and Das, K. (2019). Multi-criteria-based sub-basin prioritization and its risk assessment of erosion susceptibility in Kansai–Kumari catchment area, India. Appl. Water Sci. 9, 76–30. doi:10.1007/s13201-019-0954-4
Borrelli, P., Alewell, C., Alvarez, P., Anache, J. A. A., Baartman, J., Ballabio, C., et al. (2021). Soil erosion modelling: A global review and statistical analysis. Sci. Total Environ. 780, 146494. doi:10.1016/j.scitotenv.2021.146494
Borrelli, P., Robinson, D. A., Fleischer, L. R., Lugato, E., Ballabio, C., Alewell, C., et al. (2017). An assessment of the global impact of 21st century land use change on soil erosion. Nat. Comm. 8 (1), 2013. doi:10.1038/s41467-017-02142-7
Borrelli, P., Robinson, D. A., Panagos, P., Lugato, E., Yang, J. E., Alewell, C., et al. (2020). Land use and climate change impacts on global soil erosion by water (2015-2070). Proc. Natl. Acad. Sci. U. S. A. 117, 21994–22001. doi:10.1073/pnas.2001403117
Bruijn, H. D., Van der Meulen, A. J., and Katsikatsos, G. (1980). The mammals from the lower miocene of Aliveri (island of evia, Greece). Proc. Koninklijke Nederl. Akademie van Wetenschappen Ser. B Phys. Sci. 83, 241–261.
Bujok, P., Klempa, M., Jelínek, J., Porzer, M., and Rodriguez Gonzalez, M. A. (2015). Industrial tourism in the context of the industrial heritage. Geoj. Tour. Geosites 15 (1), 81–93.
Buta, R. J., Kopaska-Merkel, D. C., Rindsberg, A. K., and Martin, A. J. (2005). “Atlas of Union Chapel Mine invertebrate trackways and other traces,” in Pennsylvanian footprints in the black warrior basin of Alabama. Editors R. J. Buta, D. C. Kopaska-Merkel, and A. K. Rindsberg (Tuscaloosa: Alabama University Press), 277–337. Monograph no. 1.
Canudo, J. I., Badiola, A., Belmonte, A., Cardiel, J., Cuenca-Bescós, G., Díaz-Berenguer, E., et al. (2021). Window onto the Eocene (tertiary): The palaeontological record of the sobrarbe-pirineos UNESCO global geopark (huesca, aragon, Spain). Geoconservation Res. 4 (2), 1–12.
Canuti, P., Casagli, N., Catani, F., and Fanti, R. (2000). Hydrogeological hazard and risk in archaeological sites: Some case studies in Italy. J. Cul. Her. 1, 117–125. doi:10.1016/s1296-2074(00)00158-8
Catani, F., Segoni, S., and Falorni, G. (2010). An empirical geomorphology-based approach to the spatial prediction of soil thickness at catchment scale. Water Resour. Res. 46, W05508. doi:10.1029/2008wr007450
Ciampalini, R., Constantine, J. A., Walker-Springett, K. J., Hales, T. C., Ormerod, S. J., and Hall, L. R. (2020). Modelling soil erosion responses to climate change in three catchments of Great Britain. Sci. Tot. Environ. 749, 141657. doi:10.1016/j.scitotenv.2020.141657
Ðaković, M., Čađenović, D., and Radulović, N. (2015). Anizijska fauna nautiloida iz han buloških krečnjaka crmnice (JI crna gora). Anisian nautiloid fauna from han bulog limestones of crmnica (SE Montenegro). Геолошки гласник. 16, 95–109.
Dalla Vecchia, F. (1997). “Terrestrial tetrapod evidence on the norian (late triassic) and cretaceous carbonate platforms of northern adriatic region (Italy, Slovenia and Croatia),” in Proc. Int. Symp.“Mesozoic vertebrate faunas of central Europe”, sargetia, ser. Sci. Nat. XVII (Deva, 177–201.
Danese, M., Masini, N., Biscione, M., and Lasaponara, R. (2014). Predictive modeling for preventive archaeology: Overview and case study. Open Geosci. 6 (1), 42–55. doi:10.2478/s13533-012-0160-5
Danese, M., Masini, N., and Murgante, B. (2013). Archeological risk and spatial analysis. How to compare urban sprawl and archaeological sensibility maps. EARSel Proc.
Danilo, L., Remy, J. A., Vianey-Liaud, M., Marandat, B., Sudre, J., and Lihoreau, F. (2013). A new Eocene locality in southern France sheds light on the basal radiation of Palaeotheriidae (Mammalia, Perissodactyla, Equoidea). J. Vert. Paleontol. 33 (1), 195–215. doi:10.1080/02724634.2012.711404
De Beaumont, G. (1976). Note sur quelques carnivores (Mammifères) du Quaternaire ancient de la provincie de Foggia (Italie). Bull. Soc. Vaudoise Sci. Nat. 74, 217–226.
Džaparidze, V. B., Nioradze, M., Pavlenisvili, E., Schminke, H. U., and Sologasvili, D. (1989). Der altpaläolithische fundplatz Dmanisi in georgien (kaukasus). Jahr.Römisch-Germanischen Zentralmuseums Mainz 36 (1), 67–116.
Ellis, E. C. (2021). Land use and ecological change: A 12,000-year history. Ann. Rev. Environ. Resour. 46, 1–33. doi:10.1146/annurev-environ-012220-010822
Endere, M. L., and Prado, J. L. (2015). Characterization and valuation of paleontological heritage: A perspective from Argentina. Geoheritage 7, 137–145. doi:10.1007/s12371-014-0124-x
Erickson, G. M., Rauhut, O. W., Zhou, Z., Turner, A. H., Inouye, B. D., Hu, D., et al. (2009). Was dinosaurian physiology inherited by birds? Reconciling slow growth in Archaeopteryx. PLoS One 4 (10), e7390–e7399. doi:10.1371/journal.pone.0007390
Falkingham, P., Marty, D., and Richter, A. (2016). Dinosaur tracks: The next steps (life of the past). Bloomington and Indianapolis: Indiana University Press.
Fernández-Jalvo, Y., and Andrews, P. (1992). Small mammal taphonomy of gran Dolina, Atapuerca (burgos), Spain. J. Archaeol. Sci. 19 (4), 407–428. doi:10.1016/0305-4403(92)90058-b
Ferreira, C. S. S., Seifollahi-Aghmiuni, S., Destouni, G., Ghajarnia, N., and Kalantari, Z. (2022). Soil degradation in the European Mediterranean region: Processes, status and consequences. Sci. Total Environ. 805, 150106. doi:10.1016/j.scitotenv.2021.150106
Foth, C., Tischlinger, H., and Rauhut, O. W. (2014). New specimen of Archaeopteryx provides insights into the evolution of pennaceous feathers. Nature 511 (7507), 79–82. doi:10.1038/nature13467
Gabunia, L. A., Swisher, C. C., Lordkipanidze, D., Vekua, A., Justus, A., and Swisher, C. C. (2001). Dmanisi and dispersal. Evol. Anthropol. Issues, News, Rev. 10 (5), 158–170. doi:10.1002/evan.1030
García-Ávila, M., Ballesteros, B., and Diez, J. B. (2022). Patrimonio paleontológico paleozoico del Geoparque Mundial de la UNESCO Montañas do Courel. Catalogación y puesta en valor. GEOGACETA 71, 19–22.
Genise, J. F., and Harrison, T. (2018). Walking on ashes: Insect trace fossils from Laetoli indicate poor grass cover associated with early hominin environments. Palaeontology 61 (4), 597–624. doi:10.1111/pala.12357
Georgalis, G. L., Villa, A., Ivanov, M., Roussiakis, S., Skandalos, P., and Delfino, M. (2019). Early Miocene herpetofaunas from the Greek localities of Aliveri and Karydia–bridging a gap in the knowledge of amphibians and reptiles from the early Neogene of southeastern Europe. Hist. Biol. 31 (8), 1045–1064. doi:10.1080/08912963.2017.1417404
Giustini, D., and Kamel Boulos, B. (2013). Google Scholar is notenough to be used alone for systematic reviews. Online J. Public Health Inf. 5 (2), 1–9. doi:10.5210/ojphi.v5i2.4623
Goemaere, E., Demarque, S., Dreesen, R., and Declercq, P. (2015). The geological and cultural heritage of the caledonian stavelot-venn massif, Belgium. Geoheritage 8 (3), 211–233. doi:10.1007/s12371-015-0155-y
González, A. P., Aleixandre, T., Pinilla, A., Martínez, A. I. O., and Gallardo, J. C. (1999). “Geología y estratigrafía del relleno de Galería de la Sierra de la Sierra de Atapuerca (Burgos),” in Atapuerca: Ocupaciones humanas y paleoecología del yacimiento de Galería (Valladolid: Junta de Castilla y León, Consejería de Educación y Cultura), 31–42.
Gould, S. J. (1990). Wonderful life: The burgess Shale and the nature of history. WW Norton and Company.
Greshko, M. (2016). Newfound footprints stir debate over our ancestors’ sex lives. Available at: https://www.nationalgeographic.com/history/article/oldest-human-footprints-australopithecus-lucy-tanzania-science.
Guerrero-Arenas, R., Arellano, A., Josefina, F., Alvarado Mendoza, L., and Jiménez-Hidalgo, E. (2020). How is the paleontological heritage of Mexico and other Latin American countries protected? Paleontol. Mex. 9 (2), 93–90.
Hadjimitsis, D., Agapiou, A., Alexakis, D., and Sarris, A. (2013). Exploring natural and anthropogenic risk for cultural heritage in Cyprus using remote sensing and GIS. Int. J. Digit. Earth 6 (2), 115–142. doi:10.1080/17538947.2011.602119
Hayward, B. W. (2009). Protecting fossil sites inNew zealand. PaleoParks—the protection and conservation of fossil sites worldwide. Carnets de Noteb. Geol. Brest Book 3, 49–64.
Herne, M., Nair, J., Evans, A., and Tait, A. (2019). New small-bodied ornithopods (dinosauria, neornithischia) from the early cretaceous wonthaggi formation (strzelecki group) of the Australian-antarctic rift system, with revision of qantassaurus intrepidus rich and vickers-rich, 1999. J. Paleontol. 93 (3), 543–584. doi:10.1017/jpa.2018.95
Hightower, C., and Caldwell, C. (2010). Shifting sands: Science researchers on Google scholar, Web of science, and PubMed, with implications for library collections budgets. Iss. Sci. Tech. Librariansh. 63, 76–94. doi:10.29173/istl2545
Hofman, C. L., and Hoogland, M. L. (2016). Connecting Stakeholders: Collaborative preventive archaeology projects at sites affected by natural and/or human impacts. Caribb. Connect. 5 (1), 1–31.
Hou, X., Ramsköld, L., and Bergström, J. (1991). Composition and preservation of the Chengjiang fauna–a Lower Cambrian soft-bodied biota. Zool. Screen. 20 (4), 395–411. doi:10.1111/j.1463-6409.1991.tb00303.x
Hou, X., Siveter, D. J., Siveter, D. J., Aldridge, R. J., Aldridge, R. J., Cong, P., et al. (2017). The cambrian fossils of chengjiang, China: The flowering of early animal life. Second Edition. Oxford: Wiley Blackwell.
Hurtt, G. C., Chini, L. P., Frolking, S., Betts, R. A., Feddema, J., Fischer, G., et al. (2011). Harmonization of land-use scenarios for the period 1500–2100: 600 years of global gridded annual land-use transitions, wood harvest, and resulting secondary lands. Clim. Change 109 (1), 117–161. doi:10.1007/s10584-011-0153-2
Intergovernmental Committee on Surveying and Mapping (2014). GDA94: Geocentric datum Australia, technical manual version 2.4: Canberra, Australian capital territory, Commonwealth of Australia. Canberra: Anzlic committee on surveying & mapping, 52.
IUGS (2022). The first 100 IUGS geological heritage sites. Spain: International Union of Geological Sciences.
Kamermans, H., van Leusen, M., and Verhangen, P. (2009). Archeological prediction and risk management. Archaeological studies. Leiden: Leiden University Press.
Kavčič, M., and Peljhan, M. (2010). Geological heritage as an integral part of natural heritage conservation through its sustainable use in the Idrija Region (Slovenia). Geoheritage 2 (3), 137–154. doi:10.1007/s12371-010-0018-5
Kleinet, H., Voigt, S., Hminna, A., Saber, H., Schneider, J., and Hmich, D. (2010). Early triassic archosaur-dominated footprint assemblage from the argana basin (Western high atlas, Morocco). Ichnos 17 (3), 215–227. doi:10.1080/10420940.2010.510030
Kölbl-Ebert, M., and Cooper, B. J. (2020). Solnhofener plattenkalk: A heritage stone of international significance from Germany. Geol. Soc. Lond. Spec. Publ. 486 (1), 103–113. doi:10.1144/sp486-2017-324
Kooland, L., and Lowery, M. (2020). Cretaceous vertebrate localities in gippsland. Dinosaur dreaming 2020 field report, 6–7.
Lazzarotto, A. (1967). Geologia della zona compresa fra l’alta Valle del Fiume Cornia ed il Torrente Pavone (Prov. di Pisa e Grosseto). Mem. Soc. Geol. It. 6 (2), 151–197.
Leakey, M. D., and Hay, R. L. (1979). Pliocene footprints in the laetolil beds at Laetoli, northern Tanzania. Nature 278 (5702), 317–323. doi:10.1038/278317a0
Lukić, D., Petrović, M., Radovanović, M., Syromiatnikova, J., and Demirović Bajrami, D. (2021). The evaluation of undiscovered archeological geoheritage potential - the case of Rudna Glava Site (Eastern Serbia). Geoj. Tour. Geosites 36 (2), 654–662. doi:10.30892/gtg.362spl13-695
Mampel, L., Cobos, A., Alcalá, L. L., and Royo-Torres, R. (2009). An integrated system of heritage management applied to dinosaur sites in Teruel (Aragón, Spain). Geoheritage 1 (2), 53–73. doi:10.1007/s12371-009-0005-x
Månsson, K. (1995). Trilobites and stratigraphy of the Middle ordovician killeröd formation, scania, Sweden. GFF 117 (2), 97–106. doi:10.1080/11035899509546206
McNutt, E. J., Hatala, K. G., Miller, C., Adams, J., Casana, J., Deane, A. S., et al. (2021). Footprint evidence of early hominin locomotor diversity at Laetoli, Tanzania. Nature 600, 468–471. doi:10.1038/s41586-021-04187-7
Melchor, R. N., and Cardonatto, M. C. (2014). Insights on the behavior of late paleozoic aquatic Crustaceans (pygocephalomorpha?): Compound trace fossils from western Argentina. Ichnos 21 (2), 76–99. doi:10.1080/10420940.2013.879868
Meldrum, D. J., Lockley, M. G., Lucas, S. G., and Musiba, C. (2011). Ichnotaxonomy of the Laetoli trackways: The earliest hominin footprints. J. Afr. Earth Sci. 60 (1–2), 1–12. doi:10.1016/j.jafrearsci.2011.01.003
Meneghini, G. (1855). Nuovi fossili toscani illustrati dal Prof. G. Meneghini. Firenze: Tipografia Nistri.
Merrill, D., and Leatherby, L. (2018). Here’s how America uses its land. Bloomberg.com. Available at: https://www.bloomberg.com/graphics/2018-us-land-use/#xj4y7vzkg (consulted May 16, 2023).
Mezga, A., and Bajraktarevic, Z. (2004). Cretaceous dinosaur and turtle tracks on the island of Veli Brijun (Istria, Croatia). Geol. Carpathica. 55 (5), 355–370.
Minter, N., Braddy, S., and Davis, R. B. (2007). Between a rock and a hard place: Arthropod trackways and ichnotaxonomy. Lethaia 40 (4), 365–375. doi:10.1111/j.1502-3931.2007.00035.x
Monash University (2023). Field Reports for Dinosaur Dreaming fossil site. Melbourne: Monash Science Centre. Available at: https://rbh49.com/DD2/fieldReports.php (consulted May 16, 2023).
Montanarella, L., Paracchini, R., and Rusco, E. (2000). Indicazione delle aree vulnerabili in Puglia. Settore Programmazione Ufficio Informatico e Servizio Cartografico della Regione Puglia. Bari: Dipartimento Ambiente, Paesaggio e Qualità Urbana, 34.
Morales, J., Azanza, B., and Gomes Ruiz, E. (1999). El patrimonio paleontológico español. Coloquios Paleontol. 50, 53–62.
Morales, J. (1996). “El patrimonio paleontológico. Bases para su definición, estado actual y perspectivas futuras,” in MOPTMA (Madrid): Serie Monografías: El Patrimonio Geológico Bases para su valoración protección y utilización, 39–51.
Moreau, J. D., Gand, G., Fara, E., Galtier, J., Aubert, N., and Fouché, S. (2021). Trackways of arthropleura from the late pennsylvanian of graissessac (hérault, southern France). Hist. Biol. 33 (7), 996–1007. doi:10.1080/08912963.2019.1675055
Morey, B., and Pons, G. X. (2021). El patrimoni paleontològic de Mallorca: Catalogació, caracterització, valoració i propostes de gestió i de conservació. Boll. Soc. Hist. Nat. Balears 64, 101–129.
Morris, S. C. (1989). Burgess Shale faunas and the cambrian explosion. Science 246, 339–346. doi:10.1126/science.246.4928.339
Nearing, M. A., Xie, Y., Liu, B., and Ye, Y. (2017). Natural and anthropogenic rates of soil erosion. Int. Soil Water Cons. Res. 5 (2), 77–84. doi:10.1016/j.iswcr.2017.04.001
Nikami, K. A. (2007). A stochastic model to simulate and predict archaeological landscape taphonomy: Monitoring cultural landscapes values based on an iranian survey project. Archeol. Calcolatori 18, 101–120.
Nillson, R. (1951). Till kannedom om Ordovicium i sydostra Skåne. Geol. Fören. Stockh. Förh. 73 (4), 682–694.
Oheim, K. B. (2007). Fossil site prediction using geographic information systems (GIS) and suitability analysis: The Two Medicine Formation, MT, a test case. Palaeogeog. Palaeoclimatol. Palaeoecol. 251, 354–365. doi:10.1016/j.palaeo.2007.04.005
Ólafsdóttir, R., and Dowling, R. (2009). Geotourism and geoparks — a tool for geoconservation and rural development in vulnerable environments: A case study from Iceland. Geoheritage 6 (4), 71–87. doi:10.1007/s12371-013-0095-3
Ortega, A. I., Benito-Calvo, A., Pérez-González, A., Carbonell, E., Bermúdez de Castro, J. M., and Arsuaga, J. L. (2014). “Atapuerca karst and its palaeoanthropological sites,” in Landscapes and landforms of Spain. Editors F. Gutiérrez, and M. Gutiérrez (Springer Science and Business), 101–110.
Panagos, P., Ballabio, C., Himics, M., Scarpa, S., Matthews, F., Bogonos, M., et al. (2021). Projections of soil loss by water erosion in Europe by 2050. Environ. Sci. Policy 124, 380–392. doi:10.1016/j.envsci.2021.07.012
Panagos, P., Borrelli, P., Poesen, J., Ballabio, C., Lugato, E., Meusburger, K., et al. (2015). The new assessment of soil loss by water erosion in Europe. Environ. Sci. Poicy. 54, 438–447. doi:10.1016/j.envsci.2015.08.012
Panagos, P., Standardi, G., Borrelli, P., Lugato, E., Montanarella, L., and Bosello, F. (2018). Cost of agricultural productivity loss due to soil erosion in the European Union: From direct cost evaluation approaches to the use of macroeconomic models. Land Degrad. Dev. 29 (3), 471–484. doi:10.1002/ldr.2879
Pavia, M., Zunino, M., Coltorti, M., Angelone, C., Arzarello, M., Bagnus, C., et al. (2012). Stratigraphical and palaeontological data from the early Pleistocene Pirro 10 site of Pirro Nord (puglia, southeastern Italy). Quat. Int. 267, 40–55. doi:10.1016/j.quaint.2010.12.019
Pillola, G. L., and Zoboli, D. (2017). Dwarf mammoth footprints from the Pleistocene of Gonnesa (southwestern sardinia, Italy). Boll. Soc. Paleontol. Ital. 56 (1), 57–64.
Poggio, L., De Sousa, L. M., Batjes, N. H., Heuvelink, G., Kempen, B., Ribeiro, E., et al. (2021). SoilGrids 2.0: Producing soil information for the globe with quantified spatial uncertainty. Soil 7 (1), 217–240. doi:10.5194/soil-7-217-2021
Prado, J. L. (2008). “Patrimonio paleontológico,” in Patrimonio, ciencia y comunidad. Un abordaje preliminar en los partidos de Azul, olavarría y tandil: Argentina. Editors M. L. Endere (Buenos Aires: INCUAPA/Universidad Nacional del Centro de la Provincia de Buenos Aires), 111–141.
Renschler, C. S., and Harbor, J. (2002). Soil erosion assessment tools from point to regional scales—The role of geomorphologists in land management research and implementation. Geomorphology 47, 189–209.
Rich, T. H., Vickers-Rich, P., Constantine, A., Flannery, T. F., Kool, L., and Van Klaveren, N. (1997). A tribosphenic mammal from the Mesozoic of Australia. Science 278, 1438–1442. doi:10.1126/science.278.5342.1438
Rook, L. (2013). Special issue: The vertebrate fauna of Pirro Nord (Apricena, Apulia, southern Italy). Palaeontographica abteilung A, band 298, Lieferung, Stuttgart: Schweizerbart and Borntraeger science publishers 1–6.
Rosas, A., Pérez-Gonzàlez, A., Carbonell, E., van der Made, J., Sánchez, A., Laplana, C., et al. (2001). Le gisement pléistocène de la «Sima del Elefante å (Sierra de Atapuerca, Espagne). L'anthropologie 105 (2), 301–312. doi:10.1016/s0003-5521(01)80018-2
Schulp, A. S., Belvedere, M., Nasir, S., and Wheeler, M. (2011). An artiodactyl tracksite at Musandam peninsula, sultanate of Oman. Ichnos 18 (4), 192–196. doi:10.1080/10420940.2011.632659
Sellier, D. (2016). A deductive method for the selection of geomorphosites: Application to Mont Ventoux (Provence, France). Geoheritage 8 (1), 15–29. doi:10.1007/s12371-015-0144-1
Sheik Mujabar, P., and Chandrasekar, N. (2013). Coastal erosion hazard and vulnerability assessment for southern coastal Tamil Nadu of India by using remote sensing and GIS. Nat. Hazards 69, 1295–1314. doi:10.1007/s11069-011-9962-x
Stančič, Z., Veljanovski, T. O., and Podobnikar, T. (2001). Archaeological predictive modelling for highway construction planning. Brit. Archaeol. Rep. Int. Ser. 391, 233–238.
Stössel, I. (1995). The discovery of a new Devonian tetrapod trackway in SW Ireland. J. Geol. Soc. 152 (2), 407–413. doi:10.1144/gsjgs.152.2.0407
Stössel, I., Williams, E. A., and Higgs, K. T. (2016). Ichnology and depositional environment of the Middle Devonian Valentia Island tetrapod trackways, south-west Ireland. Palaeogeogr. Palaeoclimatol. Palaeoecol. 462, 16–40. doi:10.1016/j.palaeo.2016.08.033
Tangestani, M. H. (2006). Comparison of EPM and PSIAC models in GIS for erosion and sediment yield assessment in a semi-arid environment: Afzar Catchment, Fars Province, Iran. J. Asian Earth Sci. 27, 585–597. doi:10.1016/j.jseaes.2005.06.002
Tsegaye, B. (2019). Effect of land use and land cover changes on soil erosion in Ethiopia. Int. J. Agric. Sci. Food Technol. 5 (1), 026–034. doi:10.17352/2455-815X.000038
Uddin, K., Matin, M. A., and Maharjan, S. (2018). Assessment of land cover change and its impact on changes in soil erosion risk in Nepal. Sustain 10, 4715. doi:10.3390/su10124715
UNESCO (2021). Archaeological site of Atapuerca. Available at: https://whc.unesco.org/en/list/989/ (consulted april 15th, 2023).
UNESCO (1970). Convention on the Means of prohibiting and preventing the Illicit Import, Export and transfer of ownership of cultural property. Paris: Tratto da UNESCO. Available at: https://en.unesco.org/about-us/legal-affairs/convention-means-prohibiting-and-preventing-illicit-import-export-and.
UNESCO (2022). Natural Heritage. UNESCO Istitute for Statistics. Available at: http://uis.unesco.org/node/3079775.
Vidovic, S. U., and Martill, D. M. (2014). Pterodactylus scolopaciceps meyer, 1860 (pterosauria, pterodactyloidea) from the upper jurassic of bavaria, Germany: The problem of cryptic pterosaur taxa in early ontogeny. PLoS ONE 9 (10), 1106466–e110716. doi:10.1371/journal.pone.0110646
Wei-Haas, M. (2021). Ancient footprints hint at mysterious human relative with a strange walk. Available at: https://www.nationalgeographic.com/science/article/ancient-footprints-hint-at-mysterious-human-relative-with-a-strange-walk.
White, T. D., and Suwa, G. (1987). Hominid footprints at Laetoli: Facts and interpretations. Am. J. Phys. Anthropol. 72 (4), 485–514. doi:10.1002/ajpa.1330720409
Wuepper, D., Borrelli, P., and Finger, R. (2020). Countries and the global rate of soil erosion. Nat. Sustain. 3 (1), 51–55. doi:10.1038/s41893-019-0438-4
Keywords: geopaleontological heritage protection, vulnerability assessment, site ranking indexes, fossil sites, preventive paleontology, geodiversity, biodiversity through time
Citation: Faggi A, Bartolini-Lucenti S and Rook L (2023) Assessing the scientific value and vulnerability of paleontological sites: a new analytic operational procedure. Front. Earth Sci. 11:1163280. doi: 10.3389/feart.2023.1163280
Received: 10 February 2023; Accepted: 09 May 2023;
Published: 23 May 2023.
Edited by:
Marcelo Cohen, Federal University of Pará, BrazilReviewed by:
Gloria Cuenca-Bescos, University of Zaragoza, SpainPaulo Pereira, University of Minho, Portugal
Copyright © 2023 Faggi, Bartolini-Lucenti and Rook. This is an open-access article distributed under the terms of the Creative Commons Attribution License (CC BY). The use, distribution or reproduction in other forums is permitted, provided the original author(s) and the copyright owner(s) are credited and that the original publication in this journal is cited, in accordance with accepted academic practice. No use, distribution or reproduction is permitted which does not comply with these terms.
*Correspondence: Saverio Bartolini-Lucenti, saverio.bartolinilucenti@unifi.it; Lorenzo Rook, lorenzo.rook@unifi.it