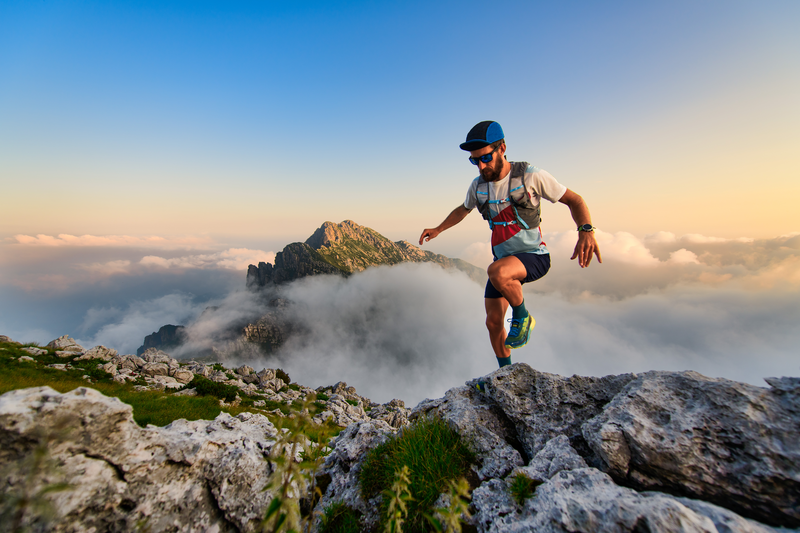
95% of researchers rate our articles as excellent or good
Learn more about the work of our research integrity team to safeguard the quality of each article we publish.
Find out more
BRIEF RESEARCH REPORT article
Front. Earth Sci. , 30 May 2023
Sec. Quaternary Science, Geomorphology and Paleoenvironment
Volume 11 - 2023 | https://doi.org/10.3389/feart.2023.1125302
This article is part of the Research Topic Environmental Change in Drylands: Past, Present, Future - Volume II View all 9 articles
The Lake Qinghai Basin is sensitive to global and regional climate change because of its unique geographical location. It is the hotspot for paleoclimate research in East Asia. In this study, we reconstructed the environmental evolution of the Lake Qinghai since ∼9 ka by using a high-resolution peat and fluvial-lacustrine record (Laoyinggou profile) obtained at the foot of Nanshan Mountain. Based on 8 AMS14C dates and lithology, loss on ignition (LOI), total organic matter (TOC), X-ray fluorescence (XRF) core-scanning measurements, ratio of total organic carbon to nitrogen (TOC/TN), and sediment particle sorting coefficients, we show that during the Middle Holocene (∼9–4.4 ka BP) this region was primarily dominated by the Asian summer monsoon, with a consistent, warm, and humid environment. By contrast, during the late Holocene (4.4 ka to present), the climatic context in this area fluctuated dramatically at the millennial scales. The low TOC content, lower TOC/TN ration and strong hydroclimatic indicate six rapid climate change events, which occurred at ∼4.0 ka, ∼3.6 ka, ∼3.2 ka, ∼2.8 ka, ∼2.1 ka, and ∼1.4 ka, all of which coincided to cold episodes in the North Atlantic Ocean.
The Holocene climate was generally warm and stable at the orbital scale, but numerous studies have demonstrated that the instability of the climate during the Holocene was associated with a number of rapid climate change (RCC) events at the millennial-centennial scales (Bond et al., 1997; Bond et al., 2001; Wang et al., 2005). These RCC events are mainly characterized by cooling in the mid-high latitudes of the Northern Hemisphere and drought in the low-latitude monsoon regions. Paleoclimate communities have comprehensively studied the 8.2 thousand years (ka) event (Alley et al., 1997; Alley and Agustsdottir, 2005) and 4.2 ka event (Weiss, 2019; Utida et al., 2020; An et al., 2021), recognized 2 climate anomalies globally. These 2 RCC events as time nodes could be divided into Early Holocene (11.5–8.2 ka), Middle Holocene (8.2–4.2 ka) and Late Holocene (4.2 ka to precent) (Zanchetta et al., 2016; Ran and Chen, 2019; Walker et al., 2019).
Lake Qinghai, the largest inland lake in China, is located on the northeastern margin of the Qinghai-Tibet Plateau (An et al., 2012). It is located at the front of the Asian summer monsoon and has a high altitude, which makes it very sensitive to the climate change of the East Asian monsoon, Indian monsoon and westerly belt, and has become the hot spot for studying the interaction of these paleoclimate systems (Lister et al., 1991; Shen et al., 2005; An et al., 2012; Liu et al., 2014; Thomas et al., 2014). In the past few decades, many scholars have done a lot of studies on the paleoclimate and environmental evolution of the sediments in the Lake Qinghai Basin. An et al. (2012) revealed that the cold-dry events around 11.0 ka, 10.3 ka, 9.3 ka, 7.2 ka and 5.5 ka, and suggested that the millennial-scale climate change in the Lake Qinghai during the Holocene reflects that Summer Monsoon Index (SMI) variability may be linked to North Atlantic abrupt events associated with the Atlantic’s overturning circulation and sea-ice variations, freshwater forcing played a prominent role in millennial scale climate change. Furthermore, weak monsoon events in the SMI sequence can be correlated with many of the intervals of low solar activity (An et al., 2012). Ji et al. (2005) argued that the 8.2 ka event and the Little Ice Age event were recorded in the Lake Qinghai sediments. Wang et al. (2015) reconstructed the palaeotemperature of the Lake Qinghai using long chain alkenones, exhibits 10.4 ka and 8.8 ka rapid climate changes. Besides lake sediments, aeolian sand and loess surrounding Lake Qinghai areas also record the rapid climate change during the Holocene (Lu et al., 2010; Liu et al., 2012; Shang et al., 2013; E et al., 2015; Lu et al., 2015; Ding et al., 2019; E et al., 2019; Yang et al., 2019). For example, the eastern sandy land of the Lake Qinghai has obvious response to 9.4 ka, 8.2 ka and 4 ka cold events (Liu, 2013; Lv, 2018).
Recently, Yang et al. (2021) analyzed the stable isotope record of the Delingha tree rings on the western side of the Lake Qinghai, providing high-resolution Holocene paleoclimate data for the region (Yang et al., 2021). According to Yang et al. (2022) the record does not reflect a significant hydroclimatic transition event occurring around 4.2 ka (Yang et al., 2022). However, Weiss (2022) argued that Delingha tree rings recorded the 4.2 ka event. Therefore, how many Holocene abrupt climate events existed in the Lake Qinghai Basin, especially whether there was a 4.2 ka event, still need more paleoclimatic records to discuss.
Peat has a high content of organic matter, and radiocarbon (14C) dating of peat, which is not easy to be affected by the hard water effect, and can provide independent age-paleoclimatic information different from saltwater lake deposition and eolian deposition records (Geyh et al., 1997; Hatté et al., 2001; Wang et al., 2014; Zhou et al., 2022). As a result, peat is convenient and accurate for 14C dating in paleoclimatology research. In addition, peat deposition has good continuity and high resolution, and a variety of indicators can interpret rich environmental information. Thus, the change of peat deposition comprehensively reflects the change of sedimentary environment and hydrological conditions. To date, However, there exists a limited number of studies linked to the rapid change in climate and hydrological conditions within the Holocene of the Lake Qinghai Basin, utilizing peat as a means of paleoclimatology study. Given this, we selected to utilize the Laoyinggou (LYG) peat deposition profile situated at the northern base of Nanshan Mountain in the southwestern region of Lake Qinghai to reconstruct climate variations dating back to 9 ka BP.
The Lake Qinghai Basin is located in the northeastern part of the Qinghai-Tibet Plateau (Figure 1), with an altitude of 3,100–4,755 m, surrounded by the Datong Mountains in the north, the Riyue Mountains in the east and the Nanshan Mountains in the southwest, covering a total area of 29,000 square kilometers. The climate is plateau continental climate, winter is cold and long, summer is cool and short, and spring is windy with sandstorms, less rainfall, wet and dry seasons are clear. The basin has a variety of habitats, including alpine shrub, cold temperate coniferous forest, temperate grassland, alpine meadow, marsh meadow and sandy vegetation, etc. (Chen, 1993; Chen et al., 2011).
FIGURE 1. Physiographical map of the study site (red solid star, LYG profile, 36°32‘05.07″N, 100°18′15.95″E, 3438 m). (A) Overview map showing locations of the Lake Qinghai Basin, and the dominant circulation systems of the westerlies and the Asian monsoon.; (B) Monthly mean precipitation and temperature changes from 1959 to 2015 (Ding et al., 2018); (C) Location of Lake Qinghai and the LYG profile site.
LYG profile (Figure 1; Figure 2) is located on the east bank of a tributary extending southward from Jiangxi Gully on the southern bank of the Lake Qinghai. This profile is a peat and fluvial-lacustrine deposit cut by a modern river, the coordinates are 36°32‘05.07″N, 100°18′15.95″E, the altitude is 3438 m, and the linear distance from the modern Lake Qinghai shoreline is 13–15 km. The profile is 300 cm thick and can be divided into the two parts according to lithology.
0–135 cm, visible obvious plant roots at the top, black to brown sandy clayey silt, peat layer containing more humus plants, which can be divided into the following layers.
0–30 cm: brownish meadow soil with clayey sandy silt, with more grass roots and occasional shrub roots.
31–40 cm: brownish-black clayey sandy silt.
41–55 cm: black peat layer, with many humus plants.
56–90 cm: brownish-gray sandy clayey silt, with thin layers of peat at 65 cm, 82 cm.
91–105 cm: black peat layer, mostly humus.
106–115 cm: brownish gray sandy clayey silt, weakly developed soil aggregates, brownish patches visible.
116–125 cm: light grey clayey sandy silt.
126–135 cm: black peat layer with mostly humus plants.
136–300 cm: black-brown to grayish-yellow, gravelly clayey sandy silt, which can be divided into the following layers.
136–155 cm: black-brown gravelly sandy clayey silt with weakly developed soil.
156–175 cm: grayish-yellow clayey sandy silt, sand with fine gravels, interspersed with black organic matter layer, oxidized plant roots visible, weakly developed soil-on lacustrine sediments.
176–285 cm: brownish-black gravelly sandy clayey silt with gravels.
286–300 cm: grayish-white clayey sandy silt.
Before sampling, we cleaned the surface layer of the profile, and removed 5–10 cm of soil on its surface to expose the fresh profile, and then used the columnar stainless-steel groove to sample, so that the complete and continuous samples were taken, which was convenient for the X-ray fluorescence (XRF) scanning analysis. The core samples were divided at 1 cm intervals in the laboratory. Samples 101 and 102 (at the depths of 101 and 102 cm) are missing due to compression.
We selected organic matter samples from 6 horizons, plant residues and freshwater snail shells from 3 horizons in the LYG profile to conduct Accelerator Mass Spectrometry (AMS) 14C dating. The results are shown in Table 1. The results of AMS 14C dates were calibrated with IntCal 20 dataset in Calib 8.1 program with 2 sigma uncertainty. The AMS 14C dating of the LYG sediment profile was conducted by the BETA Laboratory and Xi’an-AMS Center.
Loss on ignition (LOI) measurements were determined at 1 cm intervals using KSL-1000X-M, weighed the appropriate amount of samples in the crucible, set 550 °C burning 3 h (Heiri et al., 2001; Santisteban et al., 2004). Elemental measurements were determined at 1 cm intervals using an Avaatech XRF scanner (Richter et al., 2006; Liang et al., 2012; Davies et al., 2015; Sun et al., 2016; Guo et al., 2021). TOC concentrations were measured at 4 cm intervals with a Costech ECS4010/4024 cube analyzer, with a measurement uncertainty of <0.1%. Total Nitrogen (TN) content was also measured simultaneously in order to calculate TOC/TN. Grain size analysis was made at 2 cm intervals with a Malvern Mastersizer 3000 laser-diffraction analyzer, which determines grain sizes of 0.01–3000 μm, with an analytical error within 2% (Sun et al., 2006; An et al., 2012). The grain size data were analyzed using the open source software QGrain (Liu et al., 2021). The above experiments were done at the Institute of Earth Environment, Chinese Academy of Sciences. All results are shown in Figure 4.
Nine 14C dating results (Table 1) are generally consistent with the stratigraphic sequence, except for LYG160. We selected two different organic materials (TOC and plant residues) for AMS 14C dating at 190 cm depth in the LYG profile, and the results showed that the 14C age of LYG190 organic matter and plant residues were 3290 ± 30 a BP and 3520 ± 45 a BP, respectively. After calibration, organic matter and plant residues reflected a consistent range of ages. Therefore, we used 8 14C ages except LYG160, and the Bacon model (Blaauw and Christen, 2011) in R software was used for age and depth interpolation and extrapolation to obtain the age results of 0–300 cm for this profile. The deposition rate of 0–300 cm in this profile is calculated in the model, and the results are shown in Figure 3.
The sediment particle sorting coefficient varies from 2.49 to 3.97 (Figure 4), with an average value of 3.00. The LOI varies from 0.83% to 39.26% (Figure 4), with an average value of 12.34%. The TOC/TN varies from 1.22 to 12.42 (Figure 4), with an average value of 8.32. The Rb/Sr ratio varies from 0.14 to 1.04 (Figure 4), with an average value of 0.63.
FIGURE 4. LYG profile sorting coefficient-loss on ignition scatter plot, and sorting coefficient, loss on ignition, TOC, TN, C/N, Rb/Sr plot with depth.
Based on particle sorting coefficient, LOI, XRF results, TOC/TN and Rb/Sr ratio results, combined with the sedimentological observations in the field, the LYG profile can be roughly divided into two units from bottom to top, with the boundary at 135 cm (Figure 4):
1) 136–300 cm, which is the fluvial-lacustrine unit. This sediments unit has high particle size sorting coefficient values, with average value of 3.12. The LOI indicates low organic matter content (average value of 7.25) and good agreement with TOC content. TOC/TN ratio is low than 10. The Rb/Sr ratio is also low, with average value is 0.61.
2) 1–135 cm, a peat sedimentary unit. The sediments are mostly clay, and the particle size sorting coefficient is much lower than that of the fluvial-lacustrine unit, with average value is 2.88. The LOI is significantly higher than the previous unit (average value is 18.49). Average value of TOC/TN ration is 8.40. Rb/Sr rises to the highest value (∼1.04), which also indicates that the intensity of regional weathering in the basin decreases compared to the previous interval.
Some studies have shown that the determination of organic matter in stratigraphic profiles can provide a basis for recovery of past environmental changes to a certain extent (Zhang, 1998). The productivity of organic matter in arid regions is regulated by the impact of precipitation, as noted by An et al. (1993). Chen et al. (2003) have observed that enhanced precipitation not only enhances productivity in lake, but also serves as a crucial factor in the preservation of organic material within the lake. In general, during the humid period, the water level of the lake rose, which became a lacks oxygen environment and conducive to the preservation of organic matter. On the contrary, the content of organic matter in the sediment decreases (Liu, 2012). Moreover, TOC/TN can be used to distinguish endogenous and exogenous organic matter in lake sediments (Meyers, 1990; Talbot and Johannessen, 1992; Meyers, 1994; Lamb et al., 2006). Aquatic phytoplankton are relatively rich in proteins, which are rich in organic nitrogen, so the endogenous C/N from organic matter is low, usually between 4 and 10. Terrestrial vascular plants are mainly lignin and cellulose, which have very low nitrogen content, thus the C/N ratio of exogenous organic matter is often greater than 20 and even as high as 100 (Meyers, 1990). Although the LYG profile has a sedimentary phase change, and the sorting coefficient can better reflect the hydrodynamic changes in the profile. The research shows that the standard deviation of sediment reflects the degree of sorting, the higher value represents the lower the degree of sorting, the stronger the hydrodynamic force (Chen and Wan, 1999). Rb appears inert during weathering and remains in the residue fraction in parent rocks as residual silicate, often accumulating in clay minerals (Koinig et al., 2003). In general, Rb and silicates are carried to basin areas (lakes) by physical weathering. Sr is one of the alkaline Earth metal elements, which can form soluble bicarbonate, chloride and sulfate into aqueous solution. Sr is often attached to the surface of carbonate or organic matter in sediments, and is eventually transferred to lake deposits as ions (Sr2+). Therefore, the chemical weathering intensity of the basin is strong, and the Sr content in the corresponding lake sediments will also increase. Therefore, the Rb/Sr ratio can be used as a proxy to indicate the summer monsoon intensity (Gallet et al., 1996; Chen et al., 2001). It has also been found that low Rb/Sr ratios reflect stronger transport of terrigenous detrital material under weak chemical weathering in the basin (Kalugin et al., 2005).
Here, we use the LOI of the organic matter to represent effective humidity, grainsize sorting coefficient to represent regional hydrodynamics, TOC/TN ratio to represent vegetation changes, and Rb/Sr ration to represent weathering intensity in peats and fluvial-lacustrine records to reconstruct the climate and environment changes since 9 ka in the LYG area. (Figure 5). We divided the study interval into two substages for discussion.
FIGURE 5. Comparison of Mid-to Late Holocene Environmental Records and Regional Climate of LYG profile. (A) 30°N Summer Insolation (Laskar et al., 2011); (B) Ice-Rafted Hematite Debris (IRD) (Bond et al., 2001); (C) δ18O from Delingha tree rings (An et al., 2021); (D) Asian summer monsoon index inferred from Lake Qinghai (An et al., 2012); (E) δ13C values of terrestrial plant fragments from the HYXS profile in the Lake Qinghai region (Li et al., 2018); (F) Rb/Sr of LYG profile; (G) sorting coefficient of LYG profile; (H) LOI of LYG profile; (I) TOC/TN of LYG profile.
The first stage in 9–4.4 ka BP is a wet period. During this period, the strong summer solar radiation (Figure 5A), strengthening the summer monsoon (Figure 5D) with sufficient precipitation. Average value of TOC/TN ration in LYG is 8.40, which indicates that the organic matter originated from aquatic plants. At this time, the physical properties of sedimentary profiles with high grainsize sorting coefficients reflect a stronger hydrodynamic. All of these proxies confirm the fluvial-lacustrine deposition environment (Figure 5G). Ma et al. (2011) integrated the multiple records on the fluctuation of the surface of the Lake Qinghai and the δ18O records of the ostracod shells of QH-2000 pore in the Lake Qinghai to show that the surface of the Lake Qinghai was relatively stable at an altitude of 3213 m from 9 to 6 ka, which was ∼20 m higher than the modern surface of the lake water. Liu (2018) reanalyzed the palaeoenvironmental records and climate model simulations of in the Lake Qinghai and found that the Holocene climatic optimum period occurred from 8 to 6 ka. The vegetation in the basin was dominated by forests and grasslands, and the water level of the lake was rising during the middle Holocene (Liu, 2018). Aeolian records located around the Lake Qinghai, for example, Zeku profile (Hu et al., 2022), eastern sandy land profile (Lu et al., 2015; Ding et al., 2019; Chen, 2021), Jiangxigou Profile (E et al., 2013; Yang et al., 2016), Xiala Reservoir in Qinghai Nanshan, and Xiangpi Mountain Loess Profile (Chang, 2016) all indicated that the aeolian activity was weak, and most dunes were stabilized.
From 4.4 ka and continuing to the present day, the second stage has been characterized as a period of low temperatures and reduced precipitation. The decreased summer solar radiation (Figure 5A) during this period weakened the summer monsoon, and the increased Rb/Sr ratio (Figure 5F) also indicates poor regional hydrothermal conditions. The sedimentation profiles and reduced sorting coefficients (Figure 5G) indicate that the shrinkage of watersheds until final disappearance, and LYG profiles shift from fluvial-lacustrine deposition to peat deposition. TOC/TN (Figure 5I) indicates that plant organic matter still originated from aquatic plants. In addition, the weak reducing environment of peat and colder climatic conditions favor the preservation of organic matter, thus the loss on ignition shows high values (Figure 5H). The aeolian sedimentary profile around the Lake Qinghai shows that the aeolian sand activity intensified, the climate deteriorated at ∼4.5 ka, and the whole was mainly cold and dry, with a low degree of chemical weathering (E et al., 2013; Lu et al., 2015; Chang, 2016; Yang et al., 2016; Lv, 2018; Ding et al., 2019; Chen, 2021; Hu et al., 2022).
Although the 8.2 ka cold event was the strongest cold event since 11.5 ka in the Holocene, here the LYG region did not respond strongly to it, and the organic matter only showed a weak trough (drops ∼5%). This is probably because the organic matter content is not high in the first place, and thus the variation is moderate. However, the LYG profile shows strong fluctuations in organic matter during the Late Holocene, with six rapid hydrological changes (blue stripes in Figure 5) recorded at ∼4.0 ka, ∼3.6 ka, ∼3.2 ka, ∼2.8 ka, ∼2.1 ka and ∼1.4 ka, respectively. These six declines in organic matter (from ∼30% to ∼10%) are highly consistent with the peak of the North Atlantic ice rafted debris (IRD) events (Figure 5B). Therefore, we focus on these six rapid hydroclimatic changes in the Late Holocene.
Bond et al. (1997) identified nine IRD events in the North Atlantic during the Holocene based on IRD, which were later gradually referred to as cold events. Among them, the cold events that occurred in the late Holocene were 0.4 ka, 1.4 ka, 2.8 ka and 4.2 ka (Figure 5B), and the corresponding numbers were 0–4 (Bond et al., 1997; Bond et al., 2001). In this study, except for 0.4 ka, the LYG profile responds to the other 3 cold events.
Among those cold events, the LYG profile has the strongest response to the 4.2 ka North Atlantic IRD event at∼4.0 ka. The organic matter (Figure 5H) decreased ∼30%. The summer monsoon in the Lake Qinghai Basin (Figure 5D) weakened, with a corresponding decrease in precipitation. Secondly, the duration of the rapid hydroclimatic change of ∼2.8 ka is longer. The organic matter (Figure 5H) decreases and remains at a low value (the minimum is ∼3.6%). The δ18O value of the Delingha tree ring (Figure 5C) was in the low value zone, with a minimum value of ∼28‰. In addition, the rapid hydroclimatic change in ∼1.4 ka period lasted much longer (∼500 years) and had the strongest impact. During this period, the low value of organic matter (Figure 5H) indicated vegetation coverage decreased, and it did not recover until nearly 100 years ago. Affected by the cold event, terrestrial C3 vegetation in the Lake Qinghai Basin decreased significantly (Figure 5E). The δ18O of Delingha tree ring also shows a continuous decreasing trend (Figure 5C). Although not labelled as specific cold events, both ∼3.2 ka and ∼2.1 ka coincides with the peak of the North Atlantic IRD event (Figure 5B). The Lake Qinghai Basin summer monsoon (Figure 5D) weakened to different degrees in these two periods. Unlike all the above five cold events, there was also a rapid and prolonged hydroclimatic change at 3.6 ka, but this event is not associated with the North Atlantic IRD event (Figure 5B), which may be a regional feature of this region or dating uncertainty.
We selected the LYG profile, a peat and lacustrine profile at the northern foot of the Nanshan Mountains, to analyze its organic matter, Rb/Sr ratio changes. We combined the results of AMS 14C dating results to reconstruct the depositional environment of the Lake Qinghai Basin since 9 ka. Based on the experimental results and sedimentary characteristics, we divided the LYG profile into two substages:
1) 9–4.4 ka BP, warm and wet period. This stage is characterized as fluvial-lacustrine deposition and the organic matter content in the sediment is not high.
2) 4.4 ka BP to date, cold and dry period. The LYG profile changes from fluvial-lacustrine deposition to peat deposition, preserving a large amount of organic matter. In addition, the organic matter records 6 obvious rapid hydroclimatic changes, which occurred at ∼4.0 ka, ∼3.6 ka, ∼3.2 ka, ∼2.8 ka, ∼2.1 ka, ∼1.4 ka. These rapid hydrologic changes were highly consistent with the peak of the North Atlantic IRD event.
The original contributions presented in the study are included in the article/supplementary material, further inquiries can be directed to the corresponding author.
CW formal analysis, investigation, data curation, writing original draft, visualization. YQ methodology, resources, data curation, review and editing. FF validation, review and editing. BL and PS conceptualization, validation, review and editing, supervision. DN validation and review. PS conceptualization, writing—review and editing, supervision. All authors contributed to the article and approved the submitted version.
This study was supported by the Fund of Shandong Province (No. LSKJ202203300), the National Natural Science Foundation of China (Nos. 42030512, 42102237), the Strategic Priority Research Program of the Chinese Academy of Sciences (CAS) (Grant No. XDB 40000000). PS also acknowledges the support of the special research assistant project of the Chinese Academy of Sciences.
We thank Guoqing Zhao and Shugang Wu of the Xi’an Accelerator Center, Institute of Earth Environment, Chinese Academy of Sciences, for their guidance and assistance in the experiments. We thank Prof. Hong Wang of Beijing Normal University for the improvements in English.
The authors declare that the research was conducted in the absence of any commercial or financial relationships that could be construed as a potential conflict of interest.
All claims expressed in this article are solely those of the authors and do not necessarily represent those of their affiliated organizations, or those of the publisher, the editors and the reviewers. Any product that may be evaluated in this article, or claim that may be made by its manufacturer, is not guaranteed or endorsed by the publisher.
Alley, R. B., and Agustsdottir, A. M. (2005). The 8k event: Cause and consequences of a major Holocene abrupt climate change. Quat. Sci. Rev. 24, 1123–1149. doi:10.1016/j.quascirev.2004.12.004
Alley, R. B., Mayewski, P. A., Sowers, T., Stuiver, M., Taylor, K. C., and Clark, P. U. (1997). Holocene climatic instability: A prominent, widespread event 8200 yr ago. Geology 25, 483–486. doi:10.1130/0091-7613(1997)025<0483:hciapw>2.3.co;2
An, Z., Porter, S. C., Zhou, W., Lu, Y., Donahue, D. J., Head, M., et al. (1993). Episode of strengthened summer monsoon climate of Younger Dryas age on the Loess Plateau of central China. Quat. Res. 39, 45–54. doi:10.1006/qres.1993.1005
An, Z., Colman, S. M., Zhou, W., Li, X., Brown, E. T., Jull, A., et al. (2012). Interplay between the westerlies and asian monsoon recorded in lake Qinghai sediments since 32 ka. Sci. Rep. 2, 619. doi:10.1038/srep00619
An, J., Kirleis, W., and Jin, G. (2021). Understanding the collapse of the longshan culture (4400-3800 BP) and the 4.2 ka event in the haidai region of China–from an agricultural perspective. Environ. Archaeol., 1–15. doi:10.1080/14614103.2021.2003583
Blaauw, M., and Christen, J. A. (2011). Flexible paleoclimate age-depth models using an autoregressive gamma process. Bayesian anal. 6, 457–474. doi:10.1214/11-ba618
Bond, G., Showers, W., Cheseby, M., Lotti, R., Almasi, P., Demenocal, P., et al. (1997). A pervasive millennial-scale cycle in North Atlantic Holocene and glacial climates. Science 278, 1257–1266. doi:10.1126/science.278.5341.1257
Bond, G., Kromer, B., Beer, J., Muscheler, R., Evans, M. N., Showers, W., et al. (2001). Persistent solar influence on North Atlantic climate during the Holocene. Science 294, 2130–2136. doi:10.1126/science.1065680
Chang, Q. (2016). “Study on photoluminescence chronology of aeolian deposits in Nanshan, Qinghai,” in Journal of salt lake research. Editors Z. L. Baoliang Lu, F. Han, and X. Xie.
Chen, J. A., and Wan, G. (1999). Sediment particle size distribution and its environmental significance in Lake Erhai, Yunnan Province. Chin. J. Geochem. 18, 314–320. doi:10.1007/bf03052905
Chen, J., Wang, Y., Chen, Y., Liu, L., Ji, J., and Lu, H. (2001). Rb and Sr geochemistry characteristics of Chinese loess strata and their monsoon significance. Acta Geol. Sin. 75, 8.
Chen, C.-T. A., Lan, H.-C., Lou, J.-Y., and Chen, Y.-C. (2003). The dry Holocene megathermal in inner Mongolia. Palaeogeogr. Palaeoclimatol. Palaeoecol. 193, 181–200. doi:10.1016/s0031-0182(03)00225-6
Chen, K., Han, Y., Cao, S., Ma, J., Cao, G., and Lu, H. (2011). The study of vegetation carbon storage in Qinghai Lake Valley based on remote sensing and CASA model. Procedia Environ. Sci. 10, 1568–1574. doi:10.1016/j.proenv.2011.09.249
Chen, G. (1993). “Vegetation and its distribution of Lake Qinghai area,” in Chinese journal of plant ecology. Editor M. Peng.
Chen, D. (2021). “Environmental changes since the middle and late Holocene recorded by He-Lake-Eolian sediments in the sandy land of hudong, qinghai lake,” in Journal of desert research. Editors Z. D. Lurui Jie, and X. Liu.
Davies, S. J., Lamb, H. F., and Roberts, S. J. (2015). “Micro-XRF core scanning in palaeolimnology: Recent developments,” in Micro-XRF studies of sediment cores, 189–226.
Ding, Z., Lu, R., Liu, C., and Chenx, D. (2018). “Characteristics of climate change and its monsoon circulation factors in the Qinghai Lake Rim,” in Advances in earth science.
Ding, Z., Lu, R., Lyu, Z., and Liu, X. (2019). Geochemical characteristics of Holocene aeolian deposits east of Qinghai Lake, China, and their paleoclimatic implications. Sci. Total Environ. 692, 917–929. doi:10.1016/j.scitotenv.2019.07.099
E, C., Cao, G., Hou, G., Sun, Y., Jiang, Y., and Li, F. (2013). Environmental evolution of loess record in Jiangxi gully, qinghai lake, China. Mar. Geol. Quat. Geol. 33, 193–200. doi:10.3724/sp.j.1140.2013.040193
E, C., Lai, Z., Hou, G., Cao, G., Sun, Y., Wang, Y., et al. (2015). Age determination for a Neolithic site in northeastern Qinghai-Tibetan Plateau using a combined luminescence and radiocarbon dating. Quat. Geochronol. 30, 411–415. doi:10.1016/j.quageo.2015.01.007
E, C., Zhang, J., Chen, Z., Sun, Y., Zhao, Y., Li, P., et al. (2019). High resolution OSL dating of aeolian activity at qinghai lake, northeast Tibetan plateau. Catena 183, 104180. doi:10.1016/j.catena.2019.104180
Gallet, S., Jahn, B. M., and Torii, M. (1996). Geochemical, mineralogical characterization of the Luochuan loess-paleosol sequence, China, and paleoclimatic implications. Chem. Geol. 133, 0–88. doi:10.1016/S0009-2541(96)00070-8
Geyh, M. A., Schotterer, U., and Grosjean, M. (1997). Temporal changes of the 14C reservoir effect in lakes. Radiocarbon 40, 921–931. doi:10.1017/s0033822200018890
Guo, F., Clemens, S., Liu, X., Long, Y., Li, D., Tan, L., et al. (2021). Application of XRF scanning to different geological archives. Earth Space Sci. 8, e2020EA001589. doi:10.1029/2020ea001589
Hatté, C., Pessenda, L. C., Lang, A., and Paterne, M. (2001). Development of accurate and reliable 14C chronologies for loess deposits: Application to the loess sequence of nussloch (rhine valley, Germany). Radiocarbon 43, 611–618. doi:10.1017/s0033822200041266
Heiri, O., Lotter, A. F., and Lemcke, G. (2001). Loss on ignition as a method for estimating organic and carbonate content in sediments: Reproducibility and comparability of results. J. Paleolimnol. 25, 101–110. doi:10.1023/a:1008119611481
Hu, M., Ji, T., Zheng, D., Zhuang, J., Sun, W., and Xu, A. (2022). The change characteristics of color parameters of eolian sediments and their environmental evolution in the northeastern qinghai-xizang plateau since 9.4ka. Geoscience 36, 439–448. doi:10.19657/j.geoscience.1000-8527.2022.014
Ji, J., Shen, J., Balsam, W., Chen, J., Liu, L., and Liu, X. (2005). Asian monsoon oscillations in the northeastern Qinghai–Tibet Plateau since the late glacial as interpreted from visible reflectance of Qinghai Lake sediments. Earth Planet. Sci. Lett. 233, 61–70. doi:10.1016/j.epsl.2005.02.025
Kalugin, I., Selegei, V., Goldberg, E., and Seret, G. (2005). Rhythmic fine-grained sediment deposition in Lake Teletskoye, Altai, Siberia, in relation to regional climate change. Quat. Int. 136, 5–13. doi:10.1016/j.quaint.2004.11.003
Koinig, K. A., Shotyk, W., Lotter, A. F., Ohlendorf, C., and Sturm, M. (2003). 9000 years of geochemical evolution of lithogenic major and trace elements in the sediment of an alpine lake – The role of climate, vegetation, and land-use history. J. Paleolimnol. 30, 307–320. doi:10.1023/a:1026080712312
Lamb, A. L., Wilson, G. P., and Leng, M. J. (2006). A review of coastal palaeoclimate and relative sea-level reconstructions using δ13C and C/N ratios in organic material. Earth-Science Rev. 75, 29–57. doi:10.1016/j.earscirev.2005.10.003
Laskar, J., Fienga, A., Gastineau, M., and Manche, H. (2011). La2010: A new orbital solution for the long-term motion of the earth. Astronomy Astrophysics 532, A89. doi:10.1051/0004-6361/201116836
Li, X., Liu, X., He, Y., Liu, W., Zhou, X., and Wang, Z. (2018). Summer moisture changes in the Lake Qinghai area on the northeastern Tibetan Plateau recorded from a meadow section over the past 8400 yrs. Glob. Planet. Change 161, 1–9. doi:10.1016/j.gloplacha.2017.11.016
Liang, L., Sun, Y., Yao, Z., Liu, Y., and Wu, F. (2012). Evaluation of high-resolution elemental analyses of Chinese loess deposits measured by X-ray fluorescence core scanner. Catena 92, 75–82. doi:10.1016/j.catena.2011.11.010
Lister, G. S., Kelts, K., Zao, C. K., Yu, J. Q., and Niessen, F. (1991). Lake Qinghai, China: Closed-basin like levels and the oxygen isotope record for ostracoda since the latest pleistocene. Palaeogeogr. Palaeoclimatol. Palaeoecol. 84, 141–162. doi:10.1016/0031-0182(91)90041-o
Liu, X., Lai, Z., Yu, L., Sun, Y., and Madsen, D. (2012). Luminescence chronology of aeolian deposits from the Qinghai Lake area in the Northeastern Qinghai-Tibetan Plateau and its palaeoenvironmental implications. Quat. Geochronol. 10, 37–43. doi:10.1016/j.quageo.2012.01.016
Liu, X., Colman, S. M., Brown, E. T., Henderson, A. C., Werne, J. P., and Holmes, J. A. (2014). Abrupt deglaciation on the northeastern Tibetan plateau: Evidence from Lake Qinghai. J. Paleolimnol. 51, 223–240. doi:10.1007/s10933-013-9721-y
Liu, Y., Liu, X., and Sun, Y. (2021). QGrain: An open-source and easy-to-use software for the comprehensive analysis of grain size distributions. Sediment. Geol. 423, 105980. doi:10.1016/j.sedgeo.2021.105980
Liu, B. (2013). “Millennial-scale climate change in Holocene revealed by trace elements from eolian sediments in northeastern Tibetan Plateau,” in Journal of palaeogeography(Chinese edition). Editors Z. S. Heling Jin, Z. Su, and C. Zhang.
Liu, X. (2018). Impact of temperature and precipitation changes in winter and summer on Holocene environmental change in Qinghai Lake, China. J. Salt Lake Res. 26, 11.
Lu, H., Zhao, C., Mason, J., Yi, S., Zhao, H., Zhou, Y., et al. (2010). Holocene climatic changes revealed by aeolian deposits from the Qinghai Lake area (northeastern Qinghai-Tibetan Plateau) and possible forcing mechanisms. Holocene 21, 297–304. doi:10.1177/0959683610378884
Lu, R., Jia, F., Gao, S., Shang, Y., Li, J., and Zhao, C. (2015). Holocene aeolian activity and climatic change in Qinghai Lake basin, northeastern Qinghai–Tibetan Plateau. Palaeogeogr. Palaeoclimatol. Palaeoecol. 430, 1–10. doi:10.1016/j.palaeo.2015.03.044
Lv, Z. (2018). “Changes of aeolian sand activities since Holocene recorded in the East sandy land of Lake Qinghai lake, China,” in Arid land geography. Editors X. L. Ruijie Lu, J. Du, L. Chen, and T. Li.
Ma, Y., Zhang, J., Liu, X., and Lai, Z. (2011). Lake changes since Qinghai Lake's last deglaciation. J. Salt Lake Res. 19, 7.
Meyers, P. A. (1990). Impacts of late Quaternary fluctuations in water level on the accumulation of sedimentary organic matter in Walker Lake, Nevada. Palaeogeogr. Palaeoclimatol. Palaeoecol. 78, 229–240. doi:10.1016/0031-0182(90)90216-t
Meyers, P. A. (1994). Preservation of elemental and isotopic source identification of sedimentary organic matter. Chem. Geol. 114, 289–302. doi:10.1016/0009-2541(94)90059-0
Ran, M., and Chen, L. (2019). The 4.2 ka BP climatic event and its cultural responses. Quat. Int. 521, 158–167. doi:10.1016/j.quaint.2019.05.030
Richter, T. O., Van Der Gaast, S., Koster, B., Vaars, A., Gieles, R., De Stigter, H. C., et al. (2006). The Avaatech XRF core scanner: Technical description and applications to NE atlantic sediments. Geol. Soc. Lond. Spec. Publ. 267, 39–50. doi:10.1144/gsl.sp.2006.267.01.03
Santisteban, J. I., Mediavilla, R., Lopez-Pamo, E., Dabrio, C. J., Zapata, M., Garcia, M., et al. (2004). Loss on ignition: A qualitative or quantitative method for organic matter and carbonate mineral content in sediments? J. Paleolimnol. 32, 287–299. doi:10.1023/b:jopl.0000042999.30131.5b
Shang, Y., Lu, R., Jia, F., Tian, L., Tang, Q., Chen, Y., et al. (2013). Paleoclimatic evolution indicated by major geochemical elements from aeolian sediments on the east of Qinghai Lake. Sci. Cold Arid Regions 5, 301–308. doi:10.3724/SP.J.1226.2013.00301
Shen, J., Liu, X. Q., Wang, S. M., and Matsumoto, R. (2005). Palaeoclimatic changes in the Qinghai Lake area during the last 18,000 years. Quat. Int. 136, 131–140. doi:10.1016/j.quaint.2004.11.014
Sun, Y., Lu, H., and An, Z. (2006). Grain size of loess, palaeosol and Red Clay deposits on the Chinese Loess Plateau: Significance for understanding pedogenic alteration and palaeomonsoon evolution. Palaeogeogr. Palaeoclimatol. Palaeoecol. 241, 129–138. doi:10.1016/j.palaeo.2006.06.018
Sun, Y., Liang, L., Bloemendal, J., Li, Y., Wu, F., Yao, Z., et al. (2016). High-resolution scanning XRF investigation of Chinese loess and its implications for millennial-scale monsoon variability. J. Quat. Sci. 31, 191–202. doi:10.1002/jqs.2856
Talbot, M. R., and Johannessen, T. (1992). A high resolution palaeoclimatic record for the last 27,500 years in tropical West Africa from the carbon and nitrogen isotopic composition of lacustrine organic matter. Earth Planet. Sci. Lett. 110, 23–37. doi:10.1016/0012-821x(92)90036-u
Thomas, E. K., Huang, Y., Morrill, C., Zhao, J., Wegener, P., Clemens, S. C., et al. (2014). Abundant C4 plants on the Tibetan plateau during the lateglacial and early Holocene. Quat. Sci. Rev. 87, 24–33. doi:10.1016/j.quascirev.2013.12.014
Utida, G., Cruz, F. W., Santos, R. V., Sawakuchi, A. O., Wang, H., Pessenda, L. C., et al. (2020). Climate changes in Northeastern Brazil from deglacial to Meghalayan periods and related environmental impacts. Quat. Sci. Rev. 250, 106655. doi:10.1016/j.quascirev.2020.106655
Walker, M., Gibbard, P., Head, M. J., Berkelhammer, M., Björck, S., Cheng, H., et al. (2019). Formal subdivision of the Holocene series/epoch: A summary. J. Geol. Soc. India 93, 135–141. doi:10.1007/s12594-019-1141-9
Wang, Y., Cheng, H., Edwards, R. L., He, Y., Kong, X., An, Z., et al. (2005). The Holocene asian monsoon: Links to solar changes and North Atlantic climate. Science 308, 854–857. doi:10.1126/science.1106296
Wang, Z., He, J., and Chen, Y. (2014). The reservoir effect of radiocarbon dating in lake sediment system. J. DESERT Res. 34, 683–688. doi:10.7522/j.issn.1000-694X.2014.000
Wang, Z., Liu, Z., Zhang, F., Fu, M., and An, Z. (2015). A new approach for reconstructing Holocene temperatures from a multi-species long chain alkenone record from Lake Qinghai on the northeastern Tibetan Plateau. Org. Geochem. 88, 50–58. doi:10.1016/j.orggeochem.2015.08.006
Weiss, H. (2019). “The 4.2 Ka BP Event in the Northern North Atlantic.” Climate of the Past. doi:10.5194/cp-2018-162-RC2
Weiss, H. (2022). The East Asian summer monsoon, the Indian summer monsoon, and the midlatitude westerlies at 4.2 ka BP. Proc. Natl. Acad. Sci. 119, e2200796119. doi:10.1073/pnas.2200796119
Yang, L., Sun, Y., E, C., Zhao, Y., and Lu, S. (2016). Geochemical element characteristics and paleoenvironmental significance of No. 1 Eolian section in Jiangxi Gully. J. Salt Lake Res. 24, 10.
Yang, L., Long, H., Cheng, H., He, Z., and Hu, G. (2019). OSL dating of a mega-dune in the eastern Lake Qinghai basin (northeastern Tibetan Plateau) and its implications for Holocene aeolian activities. Quat. Geochronol. 49, 165–171. doi:10.1016/j.quageo.2018.02.005
Yang, B., Qin, C., Bräuning, A., Osborn, T. J., Trouet, V., Ljungqvist, F. C., et al. (2021). Long-term decrease in Asian monsoon rainfall and abrupt climate change events over the past 6,700 years. Proc. Natl. Acad. Sci. 118, e2102007118. doi:10.1073/pnas.2102007118
Yang, B., Qin, C., Bräuning, A., Osborn, T. J., Trouet, V., Ljungqvist, F. C., et al. (2022). Reply to Weiss: Tree-ring stable oxygen isotopes suggest an increase in Asian monsoon rainfall at 4.2 ka BP. Proc. Natl. Acad. Sci. 119, e2204067119. doi:10.1073/pnas.2204067119
Zanchetta, G., Regattieri, E., Isola, I., Drysdale, R. N., Baneschi, I., and Hellstrom, J. C. (2016). The so-called “4.2 event” in the central Mediterranean and its climatic teleconnections. Alp. Mediterr. Quat. 29, 5–17.
Zhang, J. (1998). “Response of numerical fluctuation of loss on ignition to past climate and environment characteristics in Beijing area,” in Acta ecologica sinica. Editor N. D. Zhaochen Kong.
Keywords: Lake Qinghai basin, high-resolution record, rapid climate change (RCC), late Holocene, cold events
Citation: Wang C, Qiu Y, Fan F, Li B, Niu D and Shu P (2023) Rapid environmental changes in the Lake Qinghai basin during the late Holocene. Front. Earth Sci. 11:1125302. doi: 10.3389/feart.2023.1125302
Received: 16 December 2022; Accepted: 16 May 2023;
Published: 30 May 2023.
Edited by:
Zhuolun Li, Lanzhou University, ChinaReviewed by:
Jingran Zhang, Nanjing Normal University, ChinaCopyright © 2023 Wang, Qiu, Fan, Li, Niu and Shu. This is an open-access article distributed under the terms of the Creative Commons Attribution License (CC BY). The use, distribution or reproduction in other forums is permitted, provided the original author(s) and the copyright owner(s) are credited and that the original publication in this journal is cited, in accordance with accepted academic practice. No use, distribution or reproduction is permitted which does not comply with these terms.
*Correspondence: Peixian Shu, c2h1cHhAaWVlY2FzLmNu
Disclaimer: All claims expressed in this article are solely those of the authors and do not necessarily represent those of their affiliated organizations, or those of the publisher, the editors and the reviewers. Any product that may be evaluated in this article or claim that may be made by its manufacturer is not guaranteed or endorsed by the publisher.
Research integrity at Frontiers
Learn more about the work of our research integrity team to safeguard the quality of each article we publish.