Corrigendum: “Antarctic on fire”: Paleo-wildfire events associated with volcanic deposits in the Antarctic Peninsula during the Late Cretaceous
- 1Antarctic and Patagonia Paleobiology Laboratory, Chilean Antarctic Institute—INACH, Punta Arenas, Chile
- 2Climatic and Polar Center, Graduate Program in Geography, Universidade Federal do Rio Grande do Sul—UFRGS, Porto Alegre, Brazil
- 3Department of Biology, Paleontology Laboratory of Ribeirão Preto, Universidade de São Paulo—USP, São Paulo, Brazil
- 4Graduate Program in Geology—PPGEO Universidade do Vale do Rio dos Sinos, São Leopoldo, Brazil
- 5Programa de Pós-Graduação em Ambiente e Desenvolvimento, PPGAD, Universidade do Vale do Taquari—Univates, Lajeado, Brazil
- 6Department of Geology and Paleontology, National Museum, Universidade Federal do Rio de Janeiro—UFRJ, Rio de Janeiro, Brazil
The occurrence of paleo-wildfire events during the Campanian age of the Late Cretaceous is demonstrated in this study for the first time with deposits from the King George Island, South Shetland Islands, Antarctic Peninsula. With the aim of providing information that fills important paleoenvironmental and paleobiogeographic gaps about the end of the Cretaceous for the Gondwana, samples of macroscopic charcoal were collected at two different volcanic levels of the Price Point outcrop, King George Island, during the expeditions to the Antarctic Peninsula by the Brazilian Antarctic Program (PROANTAR). The samples of charcoal were treated and later analyzed under a stereomicroscope and scanning electron microscope. The analysis allowed the identification of morphoanatomical structures with potential taxonomic affinity with Podocarpaceae. These conifers were important in temperate forests of high-latitude environments during the Late Cretaceous, and this is in accordance with previous palaeobotanical records from Price Point. The analysis also showed that southern paleofloras were subject to the occurrence of paleo-wildfires much more frequently than previously thought. This indicates that fire and active volcanism were significant modifiers of the ecological niches of austral floras, because even in distal areas, the source of ignition for forest fires often came from contact with a hot volcanic ash cloud, where the vegetation was either totally or partially consumed by fire.
Introduction
Although the occurrence of wildfires has been increasingly haunting contemporary society, evidence of vegetation fires has been found in the geological record since the Silurian (Rowe and Jones, 2000; Cressler, 2001; Edwards and Axe, 2004; Glasspool et al., 2004) and even during the Quaternary, with a notable intensification in the Upper Paleozoic, when swampy forests and vegetation appeared (e.g., Scott, 1989; 2000; 2010; Scott and Glasspool, 2006). Thus, fire has been a present and shaping element of the landscape in the most distinct terrestrial ecosystems over geological time (e.g., Scott and Stea, 2002; Glasspool et al., 2004; Scott and Damblon, 2010), and evidence of the presence of paleo-wildfires can be identified through fossil charcoal preserved in the geological record (Gallowy and Hobday, 1983; Kuhlbusch and Crutzen, 1996; Schmidt and Noack, 2000; Scott and Glasspool (2007); Scott, 2010).
Although many studies have indicated a link between fires and global warming events (Pyne, 1982; Scott, 1989; Jones and Chaloner, 1991), volcanic activities might exert as another potential driver of paleo-wildfires (e.g., Swanson et al., 2013; Jiang et al., 2014; Degani-Schmidt et al., 2015; Kovács et al., 2020). Fires have also been reported as important conditioning factors of the burning and preservation of vegetation in fossil assemblies (e.g., Kemp, 1981; Scott, 2000; Manfroi et al., 2015; Cai et al., 2021).
The Antarctic Peninsula was formed by multiple and complex tectonic movements of a set of small plates that occurred from the end of the Paleozoic (Elliot, 1988; Del Valle and Rinaldi, 1993). Both geological and paleontological evidence have shown the influence of volcanic processes in fossiliferous preservation and demonstrated a greater diversity of life between the end of the Mesozoic and Middle Eocene because of the presence of more favorable climates (Westerhold et al., 2008; Zachos et al., 2008; McInherney and Wing, 2011). In addition, volcanic activities have been pointed out as one of the factors that may lead to the presence of extremely propitious conditions for the occurrence of fires (Svensen et al., 2004; Storey et al., 2007; Moore and Kurtz, 2008).
The Cretaceous is considered a special “high-fire” period in Earth’s history, during which the atmospheric oxygen level was much higher than 16%, thus facilitating combustion (Belcher and McElwain, 2008). This hypothesis has been supported by several authors based on studies carried out in North America, Europe, and Asia (Brown et al., 2012; Scott et al., 2014). Other authors have also sought to find evidence of the occurrence of paleo-wildfires in southern areas of Gondwana (e.g., Martill et al., 2012; Abu Hamad et al., 2016; dos Santos et al., 2016; El Atfy et al., 2016; 2019; Lima et al., 2019). However, when considering the depositional strata of the end of the Cretaceous for austral areas, there is a significant lack of detailed data on the occurrence of paleo-wildfires, an information gap that has been an impediment to the adequate understanding of the paleoenvironmental characteristics during that period.
The Late Cretaceous corresponds to an important period in Earth’s life history, especially for the southern regions, during which the initial break-up of the Gondwana paleocontinent occurred and propelled the emergence of the Antarctic Circumpolar Current. This break-up of Gondwana was not only responsible for significant global climate changes in those times, but it also still controls the climate in the Southern Hemisphere (Barker, 2001; Lawver and Gahagan, 2003; Pfuhl and Mc Cave, 2005). Still, in the Cretaceous, due to the generation of new environments, the floras underwent a significant evolution, which gave place to the appearance and diversification of angiosperms, which later dominated most of the terrestrial niches (Lupia et al., 1999; Barrett and Willis, 2001; Nagalingum et al., 2002). Studies focusing on the natural dynamics involved in the environments where these floras were inserted are of paramount importance, since plants are excellent environmental markers, just as fire is widely accepted as a modeler responsible for evolutionary pressures over paleofloras (Bond and Scott, 2010; Brown et al., 2012; Belcher and Hudspith, 2017; Cai et al., 2021).
Despite their relevance, studies assessing events of paleo-wildfires in the Antarctic Peninsula during the Cretaceous are scarce (Manfroi et al., 2015). These studies are mainly restricted to only five works: three describing carbonized plant fossils, without further analysis, on the Santonian levels of the Kenyon Peninsula (Eklund, 2003; Eklund et al., 2004) and the Coniacian levels of James Ross Island (Kvaček and Sakala, 2011), and two effectively describing the charcoal record for the Campanian age of Nelson Island (Manfroi et al., 2015) and for James Ross Island (Lima et al., 2021), inferred from the morphology of burnt vegetation.
Based on the above, aiming to fill this important knowledge gap regarding the occurrence of paleo-wildfires in the Antarctic Peninsula during the Cretaceous, the present study aimed to reconstruct paleoenvironmental and paleobiogeographic panoramas and, based on new records, to confirm and try to understand the paleo-wildfire events that marked the Campanian times in the Antarctic Peninsula. We analyzed samples of macroscopic charcoal found in materials collected at two different volcaniclastic levels at the Price Point outcrop, Fildes Peninsula (King George Island, South Shetland Islands) (Figure 1).
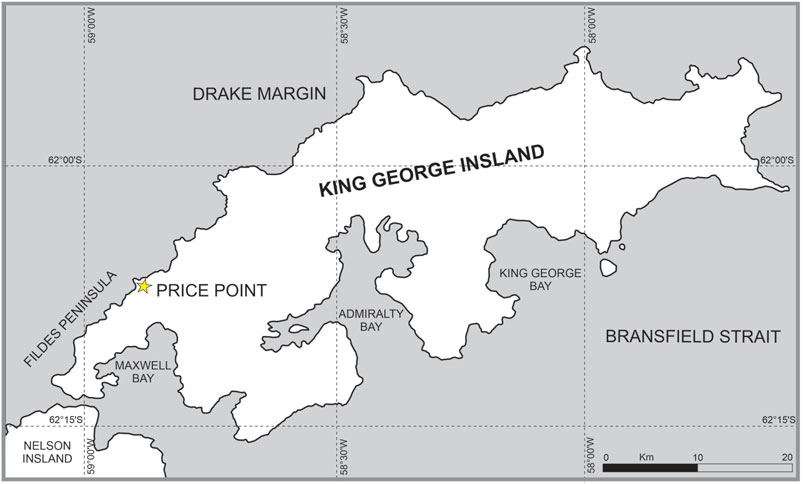
FIGURE 1. Simplified map of the King George Island, one of northern islands of the South Shetlands archipelago in the Antarctic Peninsula. The star highlights the herein studied Price Point locality.
Geological context and taphoflora
The South Shetland archipelago, which is located in the northwestern portion of the Antarctic Peninsula, is approximately 500 km long and has a total area of 3,687 km2. It consists of about eleven islands, including King George Island, which have great territorial significance. A large part of these islands was originally connected to the continental portion of the Peninsula or Graham’s Land, until extension movements that occurred 4 Ma broke these connections and casted the Bransfield Strait, which currently has an approximate width of 100 km (Tokarski, 1991; Yi et al., 2005).
Price Point (62° 15′ 41 “S/59° 49′ 59” W) is located to the east of the Fildes Peninsula and outcrops in an area close to sea level on the coast of the Drake Strait. The set of lithologies and fossiliferous content of Price Point has been assigned to the Campanian age, just as other locations on the Fildes Peninsula Smellie et al. (1984) (Shen, 1994; Dutra, 1997; Dutra et al., 1998; Dutra and Batten, 2000). The deposition of Price Point has been included in the Peninsula Fildes Group, which shows volcanic lithologies, predominantly andesitic, with tufts and shales interspersed and is representative of the oldest levels in the succession of the South Shetland Islands (Smellie et al. (1984)). Tuffite levels with lenticular centimetric coal beds of erosive basal contact are overlaid by debris flows (breccias), which, upwards, give place to tufted clasts, basalts, and conglomerate deposits with large clasts (50 cm), including wood fragments (Figure 2).
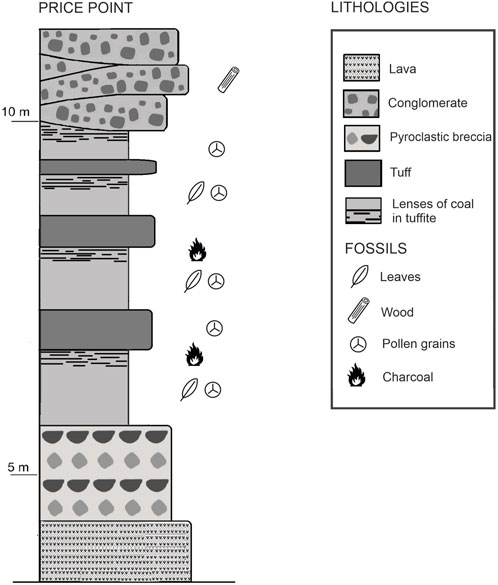
FIGURE 2. Geological section of Price Point and its main volcanic and volcaniclastic levels characterized by tuff beds and tuffites, from where the samples of macroscopic charcoal, the object of this study, were collected (modified from Dutra and Batten, 2000).
Epiclastic-to-intermediate volcaniclastic facies may correspond to floodplains and are associated swamps, similar to what Smith (1991) called low-relief alluvial plain rings, corresponding to a highstand phase among eruptions (Dutra et al., 1996; Dutra and Batten, 2000; Dutra, 2004). This proposal is supported by the presence of massive sandstone layers, of great lateral amplitude and exhibiting load features, seated on pelites. A posterior hydrothermal action is evidenced by the expressive presence of calcite in microfractures and fractures, frequent along the profile. Additionally, post-depositional tectonic movements are reflected by the 15° S dip of the strata (Dutra and Batten, 2000).
Since the Price Point outcrop has few preserved plant fossils (Figure 3) and the assembly of megafossils is limited and poorly preserved. Shen Yanbin (1994) carried a comparison palynological analysis of the material for Price Point with associated with the similar levels of other outcrops in King George Island, we sought to carry out a palynological analysis of the material associated with the levels of other outcrops in King George Island. Such analysis showed associations with the Half Three Point, in the south of King George Island, which allows proposing its inclusion in the Half Three Point Formation, Fildes Peninsula Group.
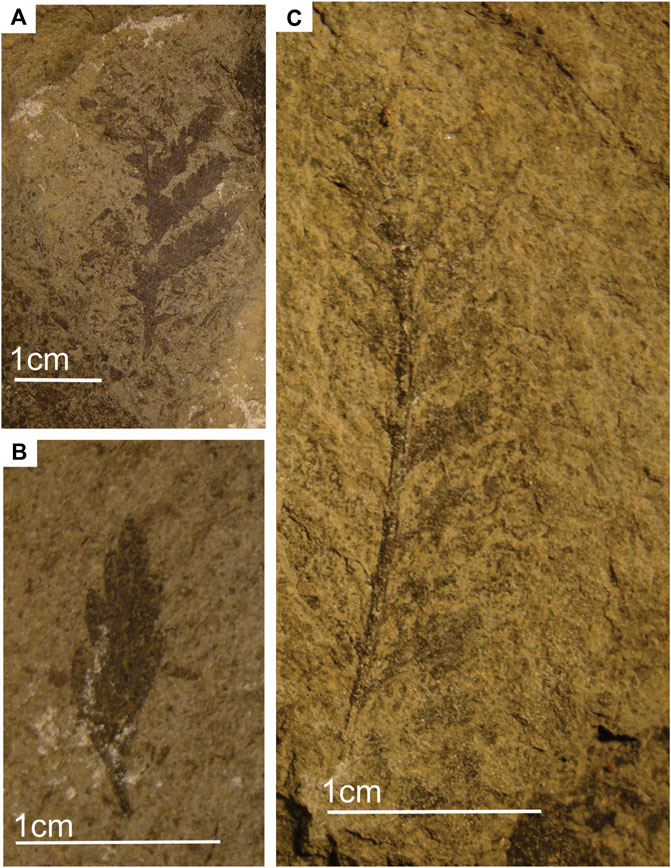
FIGURE 3. Plant fossils of ferns from Price Point belonging to the Chilean Antarctic Institute (INACH) paleobotany collection studied by Leppe et al., 2012: (A) incomplete sterile pinnae of Polypodiales; (B) fragment of sterile pinnule of Polypodiales; (C) fragment of sterile pinnae of Polypodiales.
Pollen assemblages were found to be not only characterized by the dominance of Nothofagus affinity, but they were also represented by the Nothofagidites ancestral pollen type and Podocarpus, accompanied by pteridophyte spores, which evidenced the presence with forms of Leiotriletes, Cyathedites (Cyatheaceae/Dicksoniaceae), Osmundacites, Baculatisporites (Osmundaceae), Kuklisporites, Verrucosisporites, and Laevigatosporites, the latter being associated with Schizeaceae (Dutra et al., 1996; Dutra and Batten, 2000; Leppe et al., 2012). The few macroremains preserved in the outcrop also show the presence of pteridophytes, conifers, and angiosperms, once again represented by primitive forms (Czajkowski and Rösler, 1986; Rohn et al., 1990; Leppe et al., 2012). Despite the poor preservation of plant fossils, paleobotanical studies carried out by different authors have allowed to obtain a synopsis of the taphoflora of Price Point, which comprises leaves of Cladophlebis and Dryopteris (Pteridophyta), foliage of Cycadaceae, Podocarpus tertiaria, Podozamites sp., and Phyllocladus sp (Gymnosperms) (Drinnan & Chambers, 1986; Cantrill, 1991). The leaves of angiosperms are fragmented and represented by Nothofagus sp. and mainly mesophilic leaves with Lauraceae types.
Material and methods
The plant fossils studied were obtained from samples collected during Brazilian expeditions to the Antarctic Peninsula with the support of the Brazilian Antarctic Program (PROANTAR). Samples were collected from the Price Point outcrop, on King George Island, whose age corresponds to the Campanian (Wang and Shen,1994). The samples are stored in the Antarctic Section of the History of Earth and Life Laboratory (LaViGea) at the University of Vale do Rio dos Sinos, Brazil, under the acronym Pbac. Moreover, the material analyzed comes from fifteen samples of charred wood fragments in two subsequent volcaniclastic levels at the Price Point outcrop.
During the field work, it was possible to observe the presence of several small overlapping black lenses, inserted in the tufaceous levels of the outcrop (Figure 2), presenting a shiny and silky appearance, a fact already mentioned by Dutra and Batten (2000) and in agreement with the definitions of charcoal, seen with the naked eye, established by Jones and Chaloner (1991). These lenses, which turned out to be carbonized fossil wood, were mechanically extracted from the sediment with the aid of a geological hammer and spatula from the two fossiliferous levels (Figure 4) using the methodology as applied by Scott (2000), Uhl and Kerp (2003), and Uhl et al. (2004).
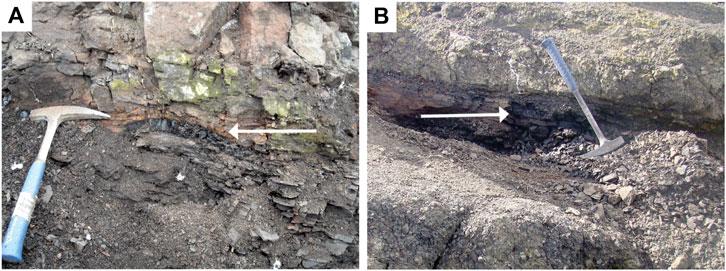
FIGURE 4. Detailed field photographs of part of the Price Point deposition showing the two carbonaceous levels (lenses of charcoal in tuffite) from which the macroscopic charcoal herein studied was collected. (A) is the level closest to the base, and (B) is the level closest to the top.
Subsequently, the collected material was analyzed with a stereomicroscope (Zeiss Stemi 2000-C) with a magnification of ×10 and ×40. Furthermore, the macroscopic charcoal fragments were mounted on standard stubs with LeitC provided with double-sided adhesive tape and metallized with gold (Plano GmbH, www.plano-em.de) for their morphoanatomical analysis with a scanning electron microscope (SEM - Zeiss EVO LS15), which was held at the Science and Technology Park of Vale do Taquari (TECNOVATES), University of Vale do Taquari, Brazil.
After observing and diagnosing the anatomical structures preserved in the macroscopic charcoal obtained from the two different levels, the taxonomic affinities of the carbonized material were determined based on the IAWA Committee (2004), and inferences were made about the possible taphonomic and depositional conditions of the plant fossils and on the reconstruction of the probable paleoenvironment that gave rise to the type of taphoflora.
In addition, a verification was carried out of the macrofossils collected in the same locality by the Chilean Antarctic Institute (INACH), deposited in the collection of Antarctic and Patagonia Paleobiology Laboratory for the purpose of taxonomic comparisons.
Results
Our results showed that the charcoalified plant fossil fragments from the two levels of Price Point are poorly preserved (Figure 5) as a result of a compaction process. Due to poor preservation, only some parts of the plant fossils preserved identifiable morphoanatomical characteristics (Figure 5).
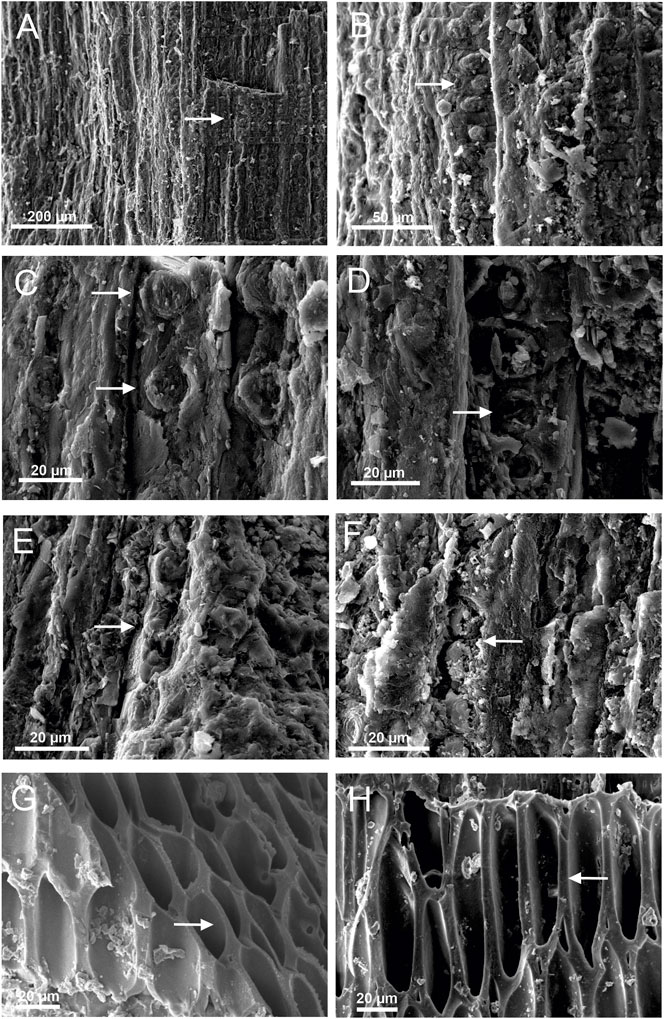
FIGURE 5. SEM images of the charcoalified woods from Price Point outcrop of King George Island exhibiting anatomic features linked to those characterizing the Podocarpaceae: (A) charred wood in radial longitudinal section, with preserved cross-field pits (arrow); (B) detail of cross-field pits, with one or two points per cross-field pit (arrow); (C) charred wood in radial longitudinal section, showing uniseriate spaced pits (arrow); (D) charred wood in radial longitudinal section, with uniseriate, contiguous pits (arrow); (E) charred wood in longitudinal tangential section with uniseriate rays, 8-cells high (arrow); (F) charred wood in longitudinal tangential section with uniseriate rays, 6-cells high (arrow); (G) fragmented tracheids with homogenized cell walls (arrow) from the most basal level (H) (A), as shown in Supplementary Figure S4; fragmented tracheids showing the surface of homogenized cell walls (arrow) often impregnated by minerals from the level closest to the top (B), as shown in Supplementary Figure S4.
The dimensions of the fragments analyzed varied between 1.0 and 3.0 mm in width and between 1.0 and 5.0 mm in length for both depositional levels collected. They showed part of the observable characteristics described as typical of macroscopic charcoal: ≥2.0 mm, silky luster, black color and streak, preserved anatomical details, and homogenized cell walls (Jones and Chaloner, 1991; Scott, 2000; 2010). Regarding the morphoanatomical structures, such as cross-field pits (Figure 5A), one or two points per cross-field pit was diagnosed (Figure 5B). The tracheids, which were 10–26 μm wide and 50–250 μm long, showed abietinoid pitting with circular areolate pits in their radial walls, which were uniseriate and spaced (Figure 5C) or contiguous (Figure 5D) when observed in radial longitudinal sections. Simple transverse bundles of 8–10 μm in width and 8–16 μm in length were detected and the radial uniseriate system, with low rays of six to eight cells in height (Figures 5E, F), was observed in longitudinal tangential sections.
These analyzed characteristics in the wood and consequently their taxonomic botanical affinities were the same for the two levels collected and the homogenized cell walls, confirming that the material we studied was charred (Figure 5G). All the wood materials shared the same highly fragmented tracheids and surfaces of the cell walls (Figure 5H) expected by the taphonomic processes that led to their preservation in distinct lithologies and events involved.
Discussion
As widely demonstrated both in the literature and in this work, plants are excellent paleoenvironmental markers, and when associated with unexpected and cyclic events such as catastrophic paleo-wildfires, they reaffirm that fire is an important element as a modeler of terrestrial systems, because it is closely related to the dynamics of the subjected biota (e.g., Bond and Scott, 2010; Brown et al., 2012; Belcher and Hudspith, 2017; Cai et al., 2021).
The data herein presented represent an important record of the occurrence of paleo-wildfire events during the end of the Cretaceous in the Antarctic Peninsula, a fact so far little reported (Manfroi et al., 2015; Lima et al., 2021). The first record of charcoal in the Antarctic Peninsula was that found at Rip Point (Manfroi et al., 2015), an outcrop located to the north of the neighboring Nelson Island, whose lithologies (tuff, tuffites, conglomerates, and lava) and lithological composition are similar to those of Price Point on King George Island.
The similarity also extends to the taxonomic details of the charcoalified material, which shows abietinoid tracheids, pitting with circular areolate uniseriate spaced or contiguous pits in radial walls, and a similar radial system with homogeneous uniseriate, low rays of six to eight cells in height (Figures 5E, F) and low rays of seven to nine cells. However, regarding the morphoanatomical characteristics of the macroscopic charcoal, the analysis presents some novelties due to the preserved cross-field pits with one or two pits per field pit (Figures 5A, B), allowing to confirm the presence of conifer woods potentially linked with the family Podocarpaceae (Philippe and Bamford, 2008), namely, Podocarpus Persoon (leaf imprints) and Podocarpidites Cookson (pollen grains) identified at Price Point (Leppe et al., 2012). However, due to the fragmentation of the material, a more detailed taxonomic analysis was avoided.
The great novelty in the analyzed fossil content of Price Point is the confirmation of paleo-wildfires, evidenced by the presence of macroscopic charcoal that, despite its poor preservation, shows the same structures and aspects as those described (Jones and Chaloner, 1991; Scott 2000; Scott 2010) at two different levels of the same depositional system (Figure 2).
Recently, El Atfy et al., 2019 reported the recurrence of paleo-wildfires in deposits of the Cenomanian age, Late Cretaceous, from Egypt. Although the Cretaceous is widely considered a “high-fire” period in Earth’s history (Brown et al., 2012; Scott et al., 2014), the repetition records of charcoal at different levels in the same depositional system are rare. Thus, the data presented in this work, in addition to collaborating with the understanding of the paleoenvironmental and paleogeographic panorama of the occurrence of fires in the Late Cretaceous in the southern areas of Gondwana (e.g., Martill et al., 2012; Manfroi et al., 2015; Muir et al., 2015; dos Santos et al., 2016; El Atfy et al. 2016; El Atfy et al. 2019), also draw attention to the repetition records of charcoal at two different levels in the same depositional system, which had not yet been reported for the Antarctic Peninsula.
In addition to the data from the analysis of charcoal, the lithologies of the Price Point profile (Figure 2) are also important, since they confirm the active volcanism in nearby areas (Dutra and Batten, 2000). The association between the plant fossils described (e.g., Dutra et al., 1996, 2000; Leppe et al., 2012) and the lithologies associated with the deposits—in addition to the macroscopic charcoal analysis—allows the reconstruction of a reliable and concrete paleoenvironmental scenario, making it possible to infer the events related to the source of ignition of the paleo-wildfires. The paleoenvironmental inferences from the data in this study, combined with the work already cited, are shown in Figure 6.
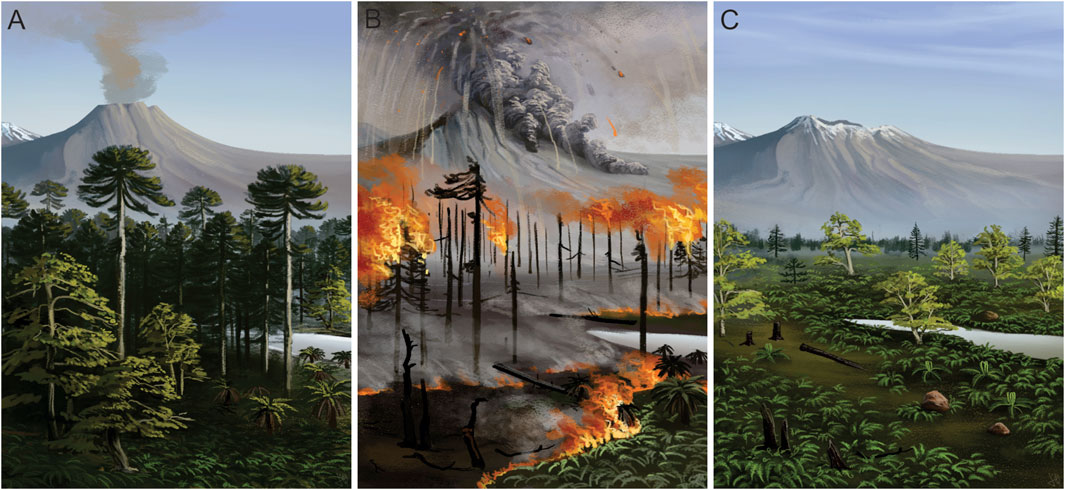
FIGURE 6. Paleoenvironmental reconstruction of austral areas under the influence of paleo-wildfires promoted by the Campanian active volcanism; artistic reconstruction by J. S. d’Oliveira: (A) original wet environments occupied by a forest of conifers, ferns, and mesophilic angiosperms growing near bodies of water and in surrounding high areas; (B) pyroclastic flows reaching the vegetation with hot ash, which burnt the vegetation either partially or totally; (C) the over-time cyclic restoration of the paleofloras and the variable conditions related to intervals between volcanic activities.
The environments of Price Point were occupied by a paleoflora that represented a humid forest of conifers, ferns, and mesophilic angiosperms linked to bodies of water and high areas in the surroundings, with a climate ranging from subtropical to temperate and wet, supported by the near-shore location of the outcrops. Through the fossiliferous record of pollen grains and leaf impressions, it was possible to determine that the assemblage of Price Point was composed of ferns, gymnosperms, and angiosperms (Table 1). In addition, the diagnosis made from the anatomical structures of the burned wood (Figure 5) confirmed the record of conifers of the Podocarpaceae family (Dutra and Batten, 2000; Leppe et al., 2012).
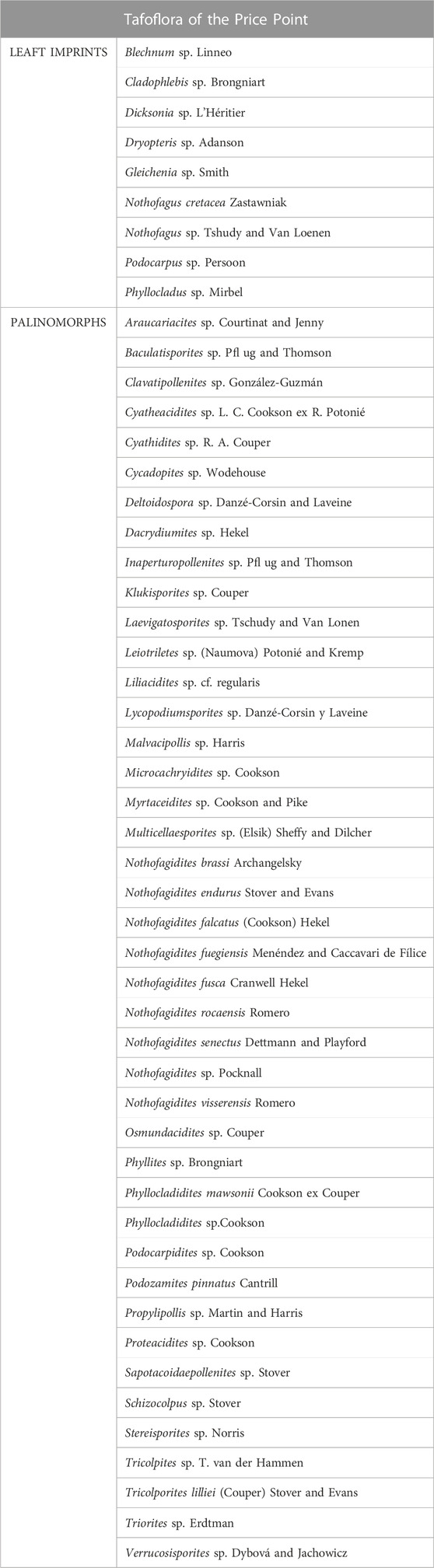
TABLE 1. Taphoflora of Price Point evidencing plant fossils preserved through leaf imprints and palynomorphs (modified from Leppe et al., 2012).
The paleoflora of Price Point was subject to paleo-wildfire events, whose ignition source was hot volcanic ash, which reached the vegetation through pyroclastic flows resembling a cloud of ash. These volcanic events were confirmed by the lithologies identified (tufts of charcoal), which alert to the establishment of intense volcanic activity (Smellie et al., 1984; Shen, 1994), and also by similar records in paleogeographically related areas (Poole et al., 2001; Manfroi et al., 2015).
Pyroclastic surges, also known as nueé ardent (in French), are recognized for their perishing power in relation to the biota. Since these pyroclastic surges have high temperatures (above 700°C) and are normally favored by the topographic unevenness of the volcano, they quickly reach a speed of 200 km/h on average and act over broad regions, driven by the wind (Teixeira et al., 2009; Grotzinger and Jordan, 2013). This destructive characteristic of pyroclastic flows may be the reason for the rare and poor fossil preservation in Price Point when compared with other areas of the Antarctic Peninsula, which show a better-preserved flora favored by the fine sediments of the volcanic ashes (Dutra and Jasper, 2010; Trumper et al., 2021). Additionally, the general incompleteness of the vegetation and its components in the assemblages allows to infer that they were consumed by the fire. In addition, the high temperatures of pyroclastic flows are associated with the cellular behavior of macroscopic charcoal (Figure 5), which allows to infer a burning temperature similar to when the contact of wood with hot volcanic ash at 900°C for 24 h without oxygen was simulated (Scott and Glasspool, 2005; Glasspool and Scott, 2013).
Regarding the poor preservation of the fossil content and the macroscopic charcoal, it is worth mentioning that the environment in which they were deposited suffered multiple forms of disturbance, such as the association with floodplains and low-relief alluvial plains (Smith, 1991). The observed 15°S dip of the strata seems to correspond to a highstand phase between eruptions (Dutra et al., 1996; Dutra and Batten, 2000) and to a post-depositional tectonism (Dutra and Batten, 2000), as well as to processes of pressure and sedimentary compaction, which automatically compacted or fragmented the burnt wood fragments (Figure 4).
Although ferns were not recorded in the macroscopic charcoal studied here, probably because they were completely consumed by fire, they were important elements in the original plant communities of Price Point (Dutra and Batten, 2000; Leppe et al., 2012) (Figure 3) and in other Cretaceous paleoenvironmental contexts (Alvin, 1974; Macdonald et al., 1991; Brown et al., 2012; Sender et al., 2015; Abu Hamad et al., 2016). Since studies have shown that ferns are highly flammable and can fuel fires that spread quickly, they may have been potential sources of fuel for the fires during the Cretaceous (Belcher and Hudspith, 2017), mainly in the Late Cretaceous, when the potential for inflammation/fire would have been greater than today due to the high levels of atmospheric oxygen (Belcher and McElwain, 2008; Belcher et al., 2010).
Besides the paleo-wildfire events that disturbed the deposits studied here, there is also the recomposition of the paleofloras over time, sometimes giving place to the vegetation that recovers and later resurges, even with the same groups or taxa, as shown by the paleoenvironmental reconstructions (Figure 6) and evidenced by the geological session (Figure 2). Based on the interpretations of the data obtained and the related literature, it is also possible to infer that fire was an important element in the dynamics of the paleofloras of the Antarctic Peninsula during the Campanian, as were the fire events that were associated with pyroclastic flows, which determined that much of the vegetation either perished or developed.
This information corroborates and contributes to that proposed by Bond and Scott (2010), Brown et al. (2012), Carpenter et al. (2016), and Belcher and Hudspith (2017), who have suggested that the occurrence of frequent and intense wildfires during the Cretaceous probably played an important role in the evolution of angiosperms and other paleofloras in which they were present, including creating evolutionary strategies to adapt to these events (Shi et al., 2022).
Conclusion
Based on the data presented here, it is possible to draw the following conclusions.
1) The occurrence of paleo-wildfires is reported here for the first time for King George Island, South Shetland Islands, in the northwest of the Antarctic Peninsula, through the macroscopic charcoal record.
2) The potential taxonomic affinity with Podocarpaceae was confirmed by charcoalified conifer woods.
3) Pyroclastic flows were diagnosed as a probable source of ignition for the Campanian wildfire in Price Point, King George Island.
4) The fire events, preserved in the same geological section, are an important record for the Late Cretaceous of Antarctica, which helps to fill the existing gap on the subject for the lands belonging to Gondwana.
5) Fire was an important element in the dynamics of the paleofloras of the Antarctic Peninsula during the Campanian age, as its presence probably controlled the life and survival of the vegetation.
6) The austral paleofloras of the Late Cretaceous were affected by wildfires, which have been shown be more frequent than thought at first.
Data availability statement
The original contributions presented in the study are included in the article/Supplementary Material, further inquiries can be directed to the corresponding author.
Author contributions
First authorship: JM. Equal contribution: CT, TD, MC and FA. Equal contribution and senior authorship: ML, AJ.
Funding
National Council and Technological Development—CNPq/Brazil and Chilean Antarctic Institute—INACH/Chile.
Acknowledgments
JM acknowledges the financial support by National Council and Technological Development—CNPq/Brazil (203277/2020-1). AJ also acknowledges the financial support by CNPq/Brazil (305938/2019-3 and 406992/2022-3). All the authors thank the Brazilian Antarctic Program (PROANTAR) through Project FLORANTAR (442765/2018-5), the Chilean Antarctic Institute—INACH/Chile, the Alexander von Humboldt Foundation—Germany (3.4‒8151/18 025) and the technicians of the Parque Científico e Tecnológico do Vale do Taquari (TECNOVATES-Univates, Brazil). The authors also thank Júlia Soares d’Oliveira for the artistic reconstruction of the past floras and environments presented here.
Conflict of interest
The authors declare that the research was conducted in the absence of any commercial or financial relationships that could be construed as a potential conflict of interest.
Publisher’s note
All claims expressed in this article are solely those of the authors and do not necessarily represent those of their affiliated organizations, or those of the publisher, the editors and the reviewers. Any product that may be evaluated in this article, or claim that may be made by its manufacturer, is not guaranteed or endorsed by the publisher.
Supplementary material
The Supplementary Material for this article can be found online at: https://www.frontiersin.org/articles/10.3389/feart.2023.1048754/full#supplementary-material
References
Abu hamad, A. M. B. B., Amireh, H., El Atfy, A., Jasper, A., and Uhl, D. (2016). Fire in a weichselia-dominated coastal ecosystem from the lower cretaceous (barremian) of the kurnub group in NW Jordan. Cretac. Res. 66, 82–93. doi:10.1016/j.cretres.2016.06.001
Barker, P. F. (2001). Scotia Sea regional tectonic evolution: Implications for mantle flow and palaeocirculation. Earth Scie. Rev. 55, 1–39. doi:10.1016/s0012-8252(01)00055-1
Barrett, P. M., and Willis, K. J. (2001). Did dinosaurs invent flowers? Dinosaur–angiosperm coevolution revisited. Biol. Rev. 76, 411–447. doi:10.1017/s1464793101005735
Belcher, C. M., and Hudspith, V. A. (2017). Limits for combustion in low O 2 redefine paleoatmospheric predictions for the mesozoic. Science 321, 1197–1200. doi:10.1126/science.1160978
Belcher, C. M., and McElwain, J. C. (2008). Limits for combustion in low O 2 redefine paleoatmospheric predictions for the mesozoic. Science 321, 1197–1200. doi:10.1126/science.1160978
Belcher, C. M., Yearsley, J. M., Hadden, R.M., Mc Elwain, J.C., and Rein, G. (2010). Baseline intrinsic flammability of Earth’s ecosystems estimated from paleoatmospheric oxygen over the past 350 million years. PNAS 107 (52), 22448–22453. doi:10.1073/pnas.1011974107
Bond, W. J., and Scott, A. C. (2010). Fire and the spread of flowering plants in the Cretaceous. New Phytol. 88, 1137–1150. doi:10.1111/j.1469-8137.2010.03418.x
Brown, S. A. E., Scott, A. C., Glasspool, I. J., and Collinson, M. E. (2012). Cretaceous wildfires and their impact on the Earth system. Cretac. Res. 36, 162–190. doi:10.1016/j.cretres.2012.02.008
Cai, Y. F., Zhang, H., Feng, Z., and Shen, S. Z. (2021). Intensive wildfire associated with volcanism promoted the vegetation changeover in southwest China during the permian-triassic transition. Front. Earth Sci. 9, 615–841. doi:10.3389/feart.2021.615841
Cantrill, D. J. (1991). Broad leafed coniferous foliage from the lower cretaceous otway group, southeastern Australia. Alcheringa 15, 177–190. doi:10.1080/03115519108619017
Carpenter, R. J., Holman, A. I., Abell, A. D., and Grice, K. (2016). Cretaceous fire in Australia: A review with new geochemical evidence, and relevance to the rise of the angiosperms. Aust. J. Bot. 64, 564–578. doi:10.1071/bt16109
Cressler, W. L. (2001). Evidence of earliest known wildfires. Palaios 16, 171–174. doi:10.1669/0883-1351(2001)016<0171:eoekw>2.0.co;2
Czajkowski, S., and Rösler, O. (1986). Plantas fósseis da Península Fildes; Ilha Rei Jorge (Shetlands do Sul): morfografia das impressões foliares. An. Acad. Bras. Cienc. 99–110.
Degani-Schmidt, I., Guerra-Sommer, M., Mendonça, J,O ., Mendonça-Filho, J. G., Jasper, A., Cazzulo-Klepzig, M., et al. (2015). Charcoalified logs as evidence of hypautochthonous/autochthonous wildfire events in a peat-forming environment from the Permian of southern Paraná Basin (Brazil). Int. J. Coal Geol. 146, 55–67. doi:10.1016/j.coal.2015.05.002
Del Valle, R. A., and Rinaldi, C. A. (1993). “Structural features of the northeastern sector of the Antarctic Peninsula,” in Anais Jornadas de comunicaciones sobre investigaciones Antárticas (Argentina: Instituto Antártico Argentino), 261–267.
Dos Santos., A. C. S., Celestino, H. E., De Souza, V., Guerra-Sommer, M., Manfroi, J., Uhl, D., et al. (2016). Evidence of palaeo-wildfire from the upper lower cretaceous (Serra do tucano formation, aptian–albian) of roraima (north Brazil). Cretac. Res. 57, 46–49. doi:10.1016/j.cretres.2015.08.003
Drinnan, A. N., and Chambers, T. C. (1986). Flora of the lower cretaceous koonwarra fossil bed (korumburra group), south gippsland, victoria. Australas. Palaeontol. Mem. 3, 1–77.
Dutra, T. L. (1997). Composição e história da vegetação do Cretáceoe Terciário da ilha Rei George, Península Antártica. Porto Alegre. Universidade Federal do Rio Grande do Sul. Thesis, 481.
Dutra, T. L., and Batten, D. J. (2000). Upper cretaceous floras of king George island, west Antarctica, and their palaeoenvironmental and phytogeographic implications. Cretac. Res. 21, 181–209. doi:10.1006/cres.2000.0221
Dutra, T. L., Hansen, M. A. F., and Fleck, A. (1998). New evidences of wet and mild climates in Northern Antarctic Peninsula at the end of Cretaceous. 3rd Annu. Conf. IGCP Proj. 381, 10–13.
Dutra, T. L., and Jasper, A. (2010). “Paleontologia da Antártica,”. Paleontologia. 3ed. Editor I. (org Carvalho (Brazil: Interciência, Rio de Janeiro), 1, 537–572.
Dutra, T. L., Leipnitz, B., Faccini, U. F., and Lindenmayer, Z. (1996). A non marine upper cretaceous interval in west Antarctica (king George island, northern antarctic peninsula). SAMC (south atlantic mesozoic correlations) news. IGCP Proj. 381 5, 21–22.
Dutra, T. L. (2004). Paleofloras da Antártica e sua Relação com os Eventos Tectônicos e Paleoclimáticos nas Altas Latitudes do Sul. Rev. Bras. Geociênc. 34 (3), 401–410. doi:10.25249/0375-7536.2004343401410
Edwards, D., and Axe, L. (2004). Anatomical evidence in the detection of the earliest wildfires. Palaios 19, 113–128. doi:10.1669/0883-1351(2004)019<0113:aeitdo>2.0.co;2
Eklund, H., Cantrill, D., and Francis, J. E. (2004). Late cretaceous plant mesofossils from table nunatak, Antarctica. Cretac. Res. 25, 211–228. doi:10.1016/j.cretres.2003.11.004
Eklund, H. (2003). First cretaceous flowers from Antarctica. Rev. Palaeobot. Palynol. 127, 187–217. doi:10.1016/s0034-6667(03)00120-9
El Atfy, H., Anan, T., Jasper, A., and Uhl, D. (2019). Repeated occurrence of palaeo-wildfires during deposition of the Bahariya Formation (early Cenomanian) of Egypt. J. Palaeogeogr. 8, 28. doi:10.1186/s42501-019-0042-6
El Atfy, H., Sallam, H., Jasper, A., and Uhl, D. (2016). The first evidence of paleo-wildfire from the campanian (late cretaceous) of north africa. Cretac. Res. 57, 306–310. doi:10.1016/j.cretres.2015.09.012
Elliot, D. (1988). The James Ross basin, northern antartic peninsula. An. V. Congr. Geol. Chil. 39, 225–226.
Galloway, W. E., and Hobday, D. K. (1983). Terrigeneous clastic depositional systems. New York: Springer-Verlag, 423p.
Glasspool, I. J., and Scott, A. (2013). “Identifying past fire events,” in Firephenomena and the Earth system: An interdisciplinary guide to fire science. Editor C. M. Belcher (Chichester: John Wiley and Sons, Ltd.), 179–205.
Glasspool, I. J., Edwards, D., and Axe, L. (2004). Charcoal in the Silurian as evidence for the earliest wildfire. Geology 32, 381–383. doi:10.1130/g20363.1
Jiang, B., Harlow, G., Wohletz, K., Zhou, Z., and Meng, J. (2014). New evidence suggests pyroclastic flows are responsible for the remarkable preservation of the Jehol biota. Nat. Comm. 5, 3151–51. doi:10.1038/ncomms4151
Jones, T. P., and Chaloner, W. G. (1991). Fossil charcoal, its recognition and palaeoatmospheric significance. Palaeogeogr. Palaeoclimatol. Palaeoecol. 97, 39–50. doi:10.1016/0031-0182(91)90180-y
Kemp, E. M. (1981). “Pre-Quaternary fire in Australia,” in Fire and the Australian biota. Editors A. M. GILL, R. A. GROVES, and I. R. NOBLE (Camberra, Australia: Australian Academy of Science), 03–21.
Kovács, J., Nemeth, K., Szabó, P., Kocsis, L., Kereszturic, K., Újvárie, G., et al. (2020). Volcanism and paleoenvironment of the pula maar complex: A pliocene terrestrial fossil site in central Europe (Hungary). Palaeogeogr. Palaeoclimatol. Palaeoecol. 537, 109398–398. doi:10.1016/j.palaeo.2019.109398
Kuhlbusch, T. A. J., and Crutzen, P. J. (1996). in Black carbono the global carbono cycle, and atmospheric carbon dioxide in Biomas Burning and Global Change. Editor J. S. Levine (Cambridge: MIT Press), 161–169.
Kvaček, J., and Sakala, J. (2011). Late cretaceous flora of James Ross island (Antarctica) – preliminary report. Czech. Pol. Rep. 1 (2), 96–103. doi:10.5817/cpr2011-2-9
Lawver, A., and Gahagan, L. M. (2003). Evolution of cenozoic seaways in the circum-antarctic region. Palaeogeogr. Palaeoclimatol. Palaeoecol. 198, 11–37. doi:10.1016/S0031-0182(03)00392-4
Leppe, M., Mihoc, M., Varela, N., Sinnesbeck, W., Mansilla, H., Bierma, H., et al. (2012). Evolution of the Austral-Antarctic flora during the Cretaceous: New insights from a paleobiogeographic perspective. Rev. Chil. Hist. Nat. 85, 369–392. doi:10.4067/s0716-078x2012000400002
Lima, F. J. de., Sayão, J. M., Ponciano, L. C. M. de O., Weinschütz, L. C., Figueiredo, R. G., Rodrigues, T. M., et al. (2021). Wildfires in the campanian of james ross Island: A newmacrocharcoal record for the antarctic Peninsula. Polar Rev. 40, 1–10. doi:10.33265/polar.v40.5487
Lima, F. J., Pires, E. F., Jasper, A., Uhl, D., Saraiva, A. A. F., and Sayao, J. M. (2019). Fire in the paradise: Evidence of repeated palaeo-wildfires from the araripe fossil lagerstätte (araripe basin, aptian-albian), northeast Brazil. Paleobiodivers. Paleoenviron. 99, 367–378. doi:10.1007/s12549-018-0359-7
Lupia, R., Lidgard, S., and Crane, P. R. (1999). Comparing palynological abundance and diversity: Implications for biotic replacement during the cretaceous angiosperm radiation. Paleobiology 25, 305–340. doi:10.1017/s009483730002131x
Macdonald, G. M., Larsen, C. P. S., Szeics, J. M., and Moser, K. A. (1991). The reconstruction of boreal forest fire history from lake sediments-a comparison of coal. Palaeogeogr. Palaeoclimatol. Palaeoecol. 291, 11–39.
Manfroi, J., Dutra, L. T., Gnaedinger, S. C., Uhl, D., and Jasper, A. (2015). The first report of a Campanian palaeo-wildfire in the West Antarctic peninsula. Palaeogeogr. Palaeoclimatol. Palaeoecol. 418, 12–18. doi:10.1016/j.palaeo.2014.11.012
Martill, D. M., Loveridge, R. F., Mohr, B. A. R., and Simmond, S. E. (2012). A wildfire origin for terrestrial organic debris in the cretaceous santana formation fossil lagerstätte (araripe basin) of north-east Brazil. Cretac. Res. 34, 135–141. doi:10.1016/j.cretres.2011.10.011
Mcinherney, F. A., and Wing, S. (2011). A perturbation of carbon cycle, climate, and biosphere with implications for the future. Earth Scie. Rev. 39, 489–516.
Moore, E., and Kurtz, A. C. (2008). Black carbon in paleocene-eocene boundary sediments: A test of biomass combustion as the petm trigger. Palaeogeogr. Palaeoclimatol. Palaeoecol. 267 (1-2), 147–152. doi:10.1016/j.palaeo.2008.06.010
Muir, R. A., Bordy, E. M., and Prevec, R. (2015). Lower cretaceous deposit reveals first evidence of a post-wildfire debris flow in the Kirkwood formation, Algoa Basin, Eastern Cape, South Africa. Cretac. Res. 56, 161–179. doi:10.1016/j.cretres.2015.04.005
Nagalingum, N. S., Drinnan, A. N., Lupia, R., and Mcloughlin, S. (2002). Fern spore diversity and abundance in Australia during the Cretaceous. Rev. Palaeobot. Palynol. 119, 69–92. doi:10.1016/s0034-6667(01)00130-0
Pfuhl, H. A., and McCave, I. N. (2005). Evidence for late oligocene establishment of the antarctic circumpolar current. Earth Planet. Sci. Lett. 235, 715–728. doi:10.1016/j.epsl.2005.04.025
Poole, I., Hunt, R. J., and Cantrill, D. J. (2001). A fossil wood flora from king George island: Ecological implications for an antarctic Eocene vegetation. Ann. Bot. 88, 33–54. doi:10.1006/anbo.2001.1425
Pyne, S. J. (1982). Fire in America: A cultural history of wildland and rural fire. New Jersey, USA: Princeton University Press, 257.
Rohn, R., Rösler, O. y., and Ciguel, J. H. (1990). Megafósseis vegetais do Terciãrio inferior coletados durante a Operação Antártica V (Península Fildes, Ilha Rei George). Localidades III e IV: Pontal Winkel e Pontal Price. Paleobotanica Latinoamericana Circular Informativa da Asociación Latinoamericana de Paleobotánica y Palinología 9 (1), 51.
Rowe, N. P., and Jones, T. P. (2000). Devonian charcoal. Palaeogeogr. Palaeoclimatol. Palaeoecol. 164, 331–338. doi:10.1016/s0031-0182(00)00191-7
Schmidt, M. W. I., and Noack, A. G. (2000). Black carbon in soils and sediments: Analysis, distribution, implications, and current challenges. Glob. Biogeochem. Cycles. 14, 777–793. doi:10.1029/1999gb001208
Scott, A. C., Bowman, D. M. J. S., Bond, W. J., Pyne, S. J., and Alexander, M. E. (2014). Fire on Earth: An introduction. New york, USA: wiley and sons, 413.
Scott, A. C. (2010). Charcoal recognition, taphonomy and uses in palaeoenvironmental analysis. Palaeogeogr. Palaeoclimatol. Palaeoecol. 291, 11–39. doi:10.1016/j.palaeo.2009.12.012
Scott, A. C., and Damblon, F. (2010). Charcoal: Taphonomy and significance in geology, botany and archaeology. Palaeogeogr. Palaeoclimatol. Palaeoecol. 291, 1–10. doi:10.1016/j.palaeo.2010.03.044
Scott, A., and Glasspool, I. (2005). Charcoal reflectance as a proxy for the emplacement temperature of pyroclastic flow deposits. Geol. Soc. Am. 33 (7), 589–592. doi:10.1130/G21474.1
Scott, A. C., and Glasspool, I. (2006). Observations and experiments on the origin and formation of inertinite group macerals. J. Coal Geol. 70, 53–66. doi:10.1016/j.coal.2006.02.009
Scott, A. C., and Glasspool, I. (2007). Observations and experiments on the origin and formation of inertinite group macerals. Int. J. Coal Geol. 70, 53–66. doi:10.1016/j.coal.2006.02.009
Scott, A. C. (1989). Observations on the nature and origin of fusain. J. Coal Geo.l 12, 443–475. doi:10.1016/0166-5162(89)90061-x
Scott, A. C., and Stea, R. (2002). Fire sweep across the mid-cretaceous landscape of nova scotia. The. Geosc 12, 3–6.
Scott, A. C. (2000). The pre-quaternary history of fire. Palaeogeogr. Palaeoclimatol. Palaeoecol. 164, 281–329. doi:10.1016/s0031-0182(00)00192-9
Sender, L. M., Villanueva-Amadoz, U., Pons, D., Diez, J. B., and Ferrer, J. (2015). Singular taphonomic record of a wildfire event from middle Albian deposits of Escucha Formation in northeastern of Spain. Hist. Biol. 27, 442–452. doi:10.1080/08912963.2014.895827
Shen, Y. (1994)., udivision and correlation of cretaceous to paleogene volcano sedimentary sequence from Fildes peninsula. King George Isl. Antarct. Monograph Science Press, 3. Beijing, China: 1–36.S
Shi, C., Wang, S., Cai, H., Zhang, H., Long, X., Tihelka, E., et al. (2022). Fire-prone rhamnaceae with South African affinities in cretaceous Myanmar amber. Nat. Plants. 8, 125–135. doi:10.1038/s41477-021-01091-w
Shi, C., Wang, S., Cai, H., Zhang, H., Long, X., Tihelka, E., et al. (2022). Fire-prone rhamnaceae with South African affinities in cretaceous Myanmar amber. Nat. Plants. 8, 125–135. doi:10.1038/s41477-021-01091-w
Smellie, J. L., Pankhurst, R. J., Thomson, M. R. A., and Davies, R. E. S. (1984). “Stratigraphy, geochemistry and evolution,” in The geology of the south shetland islands. (Cambridge: British Antarctic Survey scientific reports), , 1–85.
Smith, R. C. (1991). Landscape response to a major ignimbrite eruption, Taupo volcanic center, New Zealand.Sediment. Volcan. Setting. SEPM Special Publication, 45. 123–137.
Storey, M., Duncan, R. A., and Swisher, C. C. (2007). Paleocene-eocene thermal maximum and the opening of the northeast atlantic. Science 316 (5824), 587–589. doi:10.1126/science.1135274
Svensen, H., Planke, S., Malthe-Sørenssen, A., Jamtveit, B., Myklebust, R., Eidem, T., et al. (2004). Release of methane from a volcanic basin as a mechanism for initial Eocene global warming. Nature 429 (6991), 542–545. doi:10.1038/nature02566
Swanson, S. F., Jones, J,A., Crisafulli C, M., and Lara, A. (2013). Effects of volcanic and hydrologic processes on forest vegetation: Chaitén Volcano, Chile. Andean Geol. 40 (2), 359–391. doi:10.5027/andgeov40n2-a10
Teixeira, W., Fairchild, T. R., Toledo, M. C. M., and Taioli, F. (2009). Decifrando a terra. São paulo. 2a ed. Companhia Editora Nacional, 623.
Tokarski, A. K. (1991). “The late cretaceous-cenozoic structural history of king George Island, south shetlands, and its palte-tectonic setting,” in Geological evolution of Antarctica. Editor M. R. A. Thonnson, J. A. Crame, and J. W. Thonnson (Cambridge University Press), 493–498.
Trumper, S., Noll, R., Luthardt, L., and Robler, R. (2021). Environment and taphonomy of an intrabasinal upland flora preserved in lower Permian volcaniclastic sediments. N. Jb. Geol. Paläont. Abh. 300 (3), 303–344. doi:10.1127/njgpa/2021/0992
Uhl, D., and Kerp, H. (2003). Wildfires in the late palaeozoic of central Europe – the zechstein (upper permian) of NW – hesse (Germany). Palaeo 199, 1–15. doi:10.1016/s0031-0182(03)00482-6
Uhl, D., Lausberg, S., Noll, R., and Stapf, K. R. G. (2004). Wildfires in the late palaeozoic of central Europe: An overview of the rotliegend (upper carboniferous lower permian) of the saar-nahe basin (SW-Germany). Palaeogeogr. Palaeoclimatol. Palaeoecol. 207, 23–35. doi:10.1016/j.palaeo.2004.01.019
Wang, Y. X., and Shen, Y. (1994). “Rb-Sr isotopic dating and trace element, REE geochemistry of Late Cretaceous volcanic rocks from King George Island, Antarctica,” in Stratigraphy and palaeontology of fildes Peninsula, king George Island, Antarctica. Editor Y. B. Shen Brazil: Monograph), 109–131.
Westerhold, T., Röhl, U., Raffi, I., Fornaciari, E., Monechi, S., Reale, V., et al. (2008). Astronomical calibration of the Paleocene time. Palaeogeogr. Palaeoclimatol. Palaeoecol. 257, 377–403. doi:10.1016/j.palaeo.2007.09.016
Yi, S., Batten, D. J., and Lee, S. J. (2005). Provenance of recycled palynomorph assemblages recovered from surficial glaciomarine sediments in Bransfield Strait, offshore Antarctic Peninsula. Cretac. Res. 26, 906–919. doi:10.1016/j.cretres.2005.06.004
Keywords: Campanian, coniferous wood, King George Island, macroscopic charcoal, pyroclastic flows
Citation: Manfroi J, Trevisan C, Dutra TL, Jasper A, Carvalho MDA, Aquino FE and Leppe M (2023) “Antarctic on fire”: Paleo-wildfire events associated with volcanic deposits in the Antarctic Peninsula during the Late Cretaceous. Front. Earth Sci. 11:1048754. doi: 10.3389/feart.2023.1048754
Received: 19 September 2022; Accepted: 27 March 2023;
Published: 14 April 2023.
Edited by:
Martin Daniel Ezcurra, Museo Argentino de Ciencias Naturales Bernardino Rivadavia, ArgentinaReviewed by:
Karoly Nemeth, Institute of Earth Physics and Space Sciences, HungaryNannan Li, Xiamen University, China
Jacek Szwedo, University of Gdansk, Poland
Copyright © 2023 Manfroi, Trevisan, Dutra, Jasper, Carvalho, Aquino and Leppe. This is an open-access article distributed under the terms of the Creative Commons Attribution License (CC BY). The use, distribution or reproduction in other forums is permitted, provided the original author(s) and the copyright owner(s) are credited and that the original publication in this journal is cited, in accordance with accepted academic practice. No use, distribution or reproduction is permitted which does not comply with these terms.
*Correspondence: Joseline Manfroi, jmanfroi@inach.cl
†ORCID: Joseline Manfroi, https://orcid.org/0000-0002-9242-549X; Cristine Trevisan, https://orcid.org/0000-0001-5550-2380; Tânia Lindner Dutra, https://orcid.org/0000-0002-2227-020X; André Jasper, https://orcid.org/0000-0001-8143-9733; Marcelo De Araujo Carvalho, https://orcid.org/0000-0001-6088-5718; Francisco Eliseu Aquino, https://orcid.org/0000-0003-2993-1100; Marcelo Leppe, https://orcid.org/0000-0002-1545-8167