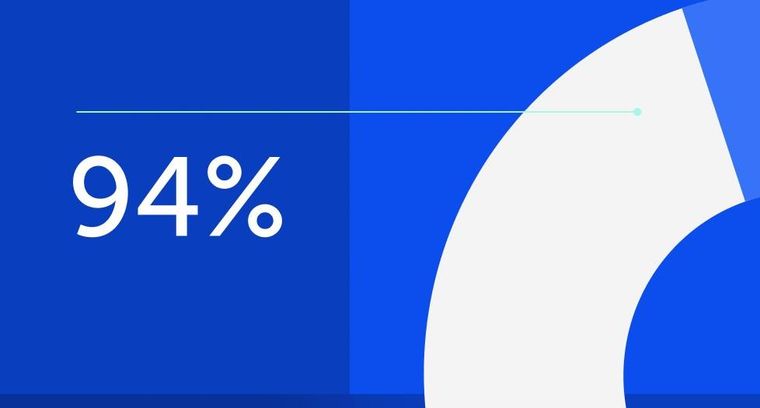
94% of researchers rate our articles as excellent or good
Learn more about the work of our research integrity team to safeguard the quality of each article we publish.
Find out more
ORIGINAL RESEARCH article
Front. Earth Sci., 07 September 2022
Sec. Atmospheric Science
Volume 10 - 2022 | https://doi.org/10.3389/feart.2022.997586
This article is part of the Research TopicAtmospheric Physics, Atmospheric Environment, and Atmospheric Effects on Human HealthView all 12 articles
Using 51 years (1960–2010) of observations from meteorological stations in the Sichuan-Chongqing region, including atmospheric visibility as a proxy for aerosol concentration, relative humidity, air temperature, wind speed and FNL reanalysis data (1°×1°) of air temperature, pressure and wind, and the altitude of each station, a linear trend and multivariate fitting approach was used to explore the effects of the large topography on the atmospheric environment in the Sichuan-Chongqing region. The region mainly consists of two areas: Sichuan Basin (SCB) and Western Sichuan Plateau (WSP; eastern part of the Tibetan Plateau). Visibility was relatively low in the SCB and high in the WSP, indicating the high and low levels of aerosols respectively in the SCB and the WSP. Additionally, visibility and wind speed were positively correlated within the basin (altitude below 750 m), while negatively correlated at stations above 1,500 m, such as on the WSP, indicating that the topography had an influence on the atmospheric environment of the basin. On the one hand, the vertical structures of the wind fields and the vertical profiles of latitudinal deviations in wind speed and air temperature in the basin show that the unique large topography causes a “harbour” effect on the leeward-slope of the WSP, with the SCB being a weak wind region, while the descending air currents in the upper westerlies of the basin form a huge “vault” of air. On the other hand, topographic effects can make the basin more susceptible to the formation of inversion structures near the surface and at high altitudes, thus stabilising the atmosphere. The topographic effects, which is not conducive to horizontal diffusion and convective transport of pollutants, were the most significant in winter, followed by autumn and spring.
The industrialization and urbanization of China over the past few decades have caused serious air pollution problems (Sun et al., 2016; Gui et al., 2020; Zheng et al., 2021), characterized of high loading of fine particulate matter PM2.5 (particulate matter with an aerodynamic diameter equal to or less than 2.5 μm) in ambient air and low horizontal visibility (Che et al., 2007; Deng et al., 2008; Sun et al., 2016), with likely consequences on the human health (Kampa and Castanas, 2008; Shang et al., 2013; Feng et al., 2016; Xing et al., 2016; Liu et al., 2017), ecosystems (Carslaw et al., 2010; Allen et al., 2019), and climate (Carslaw et al., 2013; Kelly and Zhu, 2016). In addition to factors such as high emissions, the role of meteorological conditions associated with large topography is also considered to be a crucial factor (Wang et al., 2013; Wang X. et al., 2018; Liao et al., 2018; Ning et al., 2019; Zhang et al., 2019; Shu et al., 2022b; Hu et al., 2022). It is generally accepted that topography has an important influence on synoptic circulation, atmospheric boundary layer, and local meteorology (Poulos and Pielke, 1994; Hu et al., 2014; Wang X. et al., 2018; Liu et al., 2018; Zhu et al., 2018; Zhang et al., 2019), and therefore alter chemical and physical processes of pollutants.
Topography can alter the thermodynamic and dynamic structure of the atmosphere, resulting in flows representing a systematic characteristic of large topography weather and climate (Zardi and Whiteman, 2013; Wagner et al., 2015; Giovannini et al., 2017), and thus affects the exchange of energy and mass in the atmosphere (Wang H. et al., 2018; Wang et al., 2019; Shu et al., 2022b). One of the main effects is the thermodynamic forcing caused by inhomogeneous surface properties, disturbing horizontal wind flows and altering vertical momentum mixing (Schmidli and Rotunno, 2010). In addition, topographic effects can cause strong temperature inversions (Whiteman et al., 1999), low-level jets (Ting and Wang, 2006; Tuononen et al., 2015), lee-side vortices (Gao and Ping, 2005), and gusts (Letson et al., 2019). These phenomena are of worldwide significance, as nearly a quarter of the Earth’s land mass can be classified as mountainous (Blyth, 2002), such as the European Alps, the Tibetan Plateau (TP) and the Qinling and Taihang Mountains in China, and the Rocky Mountains in North America. Especially, eastern China has been identified as more climatically stable than Europe and the United States in terms of air conditions, partly due to the country’s larger size and more complex terrain (Wang X. et al., 2018).
Among the mountainous areas, atmospheric environment in the Sichuan Basin (SCB) is of particular interest in recent years (Yin et al., 2020; Chang et al., 2021; Kong et al., 2021; Wu et al., 2022), due to both its unique bowl-shaped topography and its high levels of air pollution. The SCB is flanked by mountains-that is, bordered to the west by the TP, to the north by the Daba Mountains, to the east by the Wu mountains, and to the south by the Yunnan-Guizhou Plateau, which together serve to shield the basin from external winds and trap air penetrating the basin via large-scale weather disturbances (Zhang et al., 2019; Guo et al., 2022). The basin is one of the regions with the highest values of atmospheric aerosols and clouds in China (Li et al., 2015; Qiao et al., 2015), with an annual average AOD (550 nm) value of 0.848 from 2000 to 2010, which is very significantly different from that in the TP (about 0.1) (Luo et al., 2014), which is immediately to the west of the basin. Previous studies showed that the average visibility in the SCB from 1973 to 2010 was basically no higher than 20 km, and below 10 km for most of the time in the central and southern regions, while the visibility on the western Sichuan Plateau (WSP) in the immediate west of the region remained above 25 km all year round (Chen and Xie, 2012). The principal causes of the visibility impairment in SCB were identified to be high levels of aerosol concentrations, as well as low wind speed and high relatively humidity conditions due to terrain effects (Wang et al., 2017). Previous studies have examined the influence of terrain effects on haze pollution in the SCB using individual cases of PM2.5 pollution and seasonal PM2.5 pollution aspects (Ning et al., 2018; Ning et al., 2019; Zhang et al., 2019; Shu et al., 2022b). Using sensitivity simulations of topography, Zhang et al. (Zhang et al., 2019) found that the topography could increase near-surface PM2.5 concentrations in the SCB by close to 50 μg/m3 during a severe haze episode in winter 2014, corresponding to an increase of roughly 45%. Shu et al. (Shu et al., 2022b) then went on to investigate the three-dimensional distribution of terrain-induced PM2.5 concentrations over the SCB in different seasons, and they found that the increases in PM2.5 concentrations can increase from 30 μg/m3 in summer to 90 μg/m3 in winter at surface layer and increase from summertime 10 μg/m3 to wintertime 30 μg/m3 in the lower free troposphere.
Although previous studies have manifested the terrain effects of the SCB on regional atmospheric environments, the current understanding is still inadequate, especially from a long-term climate perspective. In the context of the westerly wind belt, the dynamic and thermal effects of the TP significantly influence the regional weather and climate characteristics of China, particularly the SCB region. Therefore, there is a great need for an in-depth analysis of the large topographic dynamics and thermal effects on the long-term spatial and temporal distribution changes of aerosols in the SCB. Due to the lack of long-term aerosol concentration observations, this study uses visibility as a proxy for analysis, and a description of data and method used are presented in Section 2. Section 3 describes the spatial and temporal variability of visibility in relation to topography and analyses the mechanisms by which topography affects it using reanalysis data. Finally, the major conclusions are summarized in Section 4.
This paper uses observations from meteorological stations in the SCB and its surrounding areas (191 stations in total as shown in Figure 1) for a period of 51 years (1960-2010). The meteorological selected for analysis includes atmospheric visibility as a proxy for aerosol concentration, relative humidity, air temperature, and wind speed, as well as the air temperature, pressure, and wind in the FNL reanalysis data from 1999 to 2010. The AOD from MODIS remote sensing data were used. Data from 2010 onwards is avoided because of the substantial impact on air pollution due to the large number of emission reduction measures undertaken in SCB (Liu et al., 2021; Shu et al., 2022a). Visibility observations in China were classified according to 10 classes before 1980, after which they were expressed in kilometers. In order to make the visibility data comparable before and after 1980, we adopt the method of Qin (Qin et al., 2010) to homogenize the data. With the method, the day-by-day visibility data of all stations from 1980 to 2005 are accurately converted into visibility classes, and the visibility distances are averaged under the limits of each visibility class to obtain a more suitable proxy for the visibility distances corresponding to the 10 visibility classes (Table 1).
FIGURE 1. Topography map (shading, unit: m) with the locations of TP, WSP, and SCB. The solid red line marks the basin boundary with a topographic elevation of 750 m. The 191 meteorological stations used to collect visibility and wind speed are marked with red dots.
The Chinese meteorological stations conduct at least three regular visibility observations per day, i.e., 08:00, 14:00, and 20:00 Beijing time (BJT), with an additional observation at 02:00 BJT for the base meteorological stations. Since the visibility observation at 02:00 BJT and 20:00 BJT are carried out at night, the targets selected for observation are different from those during the daytime (i.e., 14:00 BJT), resulting in inconsistent observation data. In addition, the visibility at 08:00 BJT is easily affected by early morning radiation fog. Therefore, only the observations at 14:00 BJT were selected for analysis. Observations of visibility under weather conditions such as fog, high winds, precipitation, and high humidity (relative humidity RH> 90%), which may affect visibility, have been excluded. The filtered visibility data was then revised for humidity according to the method of Rosenfeld (Rosenfeld et al., 2007) to obtain “dry visibility”, based on which the climatic characteristics of atmospheric visibility in the SCB and its surrounding areas were analyzed. In conjunction with the wind, air temperature, humidity, etc. from FNL, the effects of meteorological elements and the potential impact mechanism on the transport and dispersion of pollution in the special topography were investigated.
Figure 2 shows the spatial distribution of the AOD over China, aggregated over the years 2001–2010. It can be seen that the SCB is one of the several most polluted regions in China, with the AOD comparable with those in NCP and PRD, and that the interior of the basin (marked with white line in Figure 2) is more polluted than the peripheral areas around the basin.
FIGURE 2. Spatial distribution of the AOD over China, aggregated over the years 2001–2010. Regions with relatively high AOD are marked, i.e., the SCB, the North China Plain (NCP), the Yangtze River Delta (YRD), the Central China (CC), and the Pearl River Delta (PRD).
The higher the AOD, the stronger the extinction of the atmosphere. Therefore, visibility is generally inversely proportional to AOD. Atmospheric visibility data can be used to indirectly evaluate the optical properties of aerosols, although the effects of aerosols, air molecules and water vapor on light attenuation should be considered in the calculation. According to the annual average of atmospheric visibility over the past 51 years in Figure 3A, it can be seen that there is a sharp contrast between the visibility in the SCB and its western plateau region. Visibility within the basin, averaged at 18.9 km, is significantly lower than that in the WSP where the visibility mostly falls within 40–50 km, with the average visibility of about 46.0 km in areas above 1.5 km in altitude. The lowest visibility and highest AOD simultaneously occur in the southern part of the basin, with the former showing a gradual increase from east to west, and the maximum visibility occurs on the WSP, reaching 58.1 km.
FIGURE 3. Distribution of (A) atmospheric visibility and (B) atmospheric visibility variability in the SCB and its surrounding areas over the 51 years (1960-2010). The makers *, ●, and ○ indicate passing the 99%, 95% and 90% confidence tests, respectively.
According to the distribution of atmospheric visibility variability in Figure 3B, 116 of the 191 stations (60.7% of the total number of stations) showed a decreasing trend in visibility, with 77 stations (66.4%) passing the 99% confidence test, indicating a clear trend of decreasing visibility. The stations with reduced visibility were mainly located in the basin, concentrated in southwest of the basin area and the southeast of Chongqing (Figure 3B). The most significant reduction in visibility is in Panzhihua, with a climatic tendency of about -7.73 km per decade. Panzhihua is an important industrial city in the south Sichuan province, where highly developed industries emitting a large amount of air pollutants may be the main reason for the reduction in high visibility. On the other hand, 74 stations (38.7% of the total number of stations), mainly located on WSP, showed an increasing trend in visibility, with 39 stations (52.7%) passing the 99% confidence test. The most significant increase reached about 6.39 km per decade.
According to the linear correlation between the average visibility and altitude of each station from 1960 to 2010 (Figure 4), it can be seen that visibility and altitude are positively correlated, and the correlation coefficient is about 0.90 (R2=0.81, p<0.01), indicating that there is a close relationship between visibility and altitude, and the higher the altitude, the greater the visibility. When the altitude is less than 750 m, the visibility is mostly less than 25 km, while when the altitude is greater than 1500 m, the visibility is mostly more than 40 km. A linear correlation between the visibility and terrain altitude is also given for the different interdecadal periods (Figure 5). The visibility and terrain height in different periods are highly positive correlated as well, with all the correlation coefficients exceeding 0.8 (p<0.01).
FIGURE 4. Scatter plots and correlation coefficient (R) of the visibilities and terrain heights over the years from 1960 to 2010. There are 191 scattered points in the figure, representing the values of 191 meteorological observation stations.
FIGURE 5. Scatter plots and correlation coefficients (R) of the visibilities and terrain heights over the years from (A) 1960 to 1970, (B) 1980 to 1990, and (C) 2000 to 2010, respectively. There are 191 scattered points in each of the three subplots, representing the values of 191 meteorological observation stations.
As the relationship between visibility and wind speed is relatively complex, it is discussed here separately for the SCB (below 750 m elevation) and the WSP (above 1500 m elevation). It reveals that visibility in the SCB was positively correlated with the wind speed (Figure 6A), with a correlation coefficient of 0.57 (p < 0.1), indicating that wind speed is an important factor influencing visibility changes within the basin. On the contrary, visibility and wind speed on the WSP region are basically negatively correlated (Figure 6B), i.e., visibility decreases with increasing wind speed. As a rule of thumb, in heavily emitting areas such as the SCB, higher wind speeds are more conducive to the dispersion and outward transport of pollutants, while in relatively clean areas, higher wind speeds are more conducive to the transport of external pollutants into the local area causing local pollution.
FIGURE 6. Scatter plots and correlation coefficients (R) of the visibilities and wind speeds in the stations with the altitude (A) below 750 m and (B) above 1,500 m.
According to the analysis of visibility and altitude correlation for 1960-2010 and for each inter-decade period (1960-1970, 1980-1990, 2000-2010), it can be seen that visibility and altitude do not vary with the inter-decade period, but show a significant positive correlation, indicating that there is indeed a close influence effect between atmospheric environment and topography, and it can be inferred that the conclusions of this study would be still valid if the data are extended to present. In addition, visibility and wind speed are significantly positively correlated in the SCB area, while are weakly negatively correlated in the WSP area, demonstrating the regional differences in visibility variability, and its relationship with influencing factors, which are tightly affected by the topography. It is needed to understand how topography affects the atmospheric environment. In this section, the characteristics of atmospheric dynamic and thermal characteristics (reflected by wind and temperature, respectively) under the influence of the special topography are studied.
Figure 7 illustrates the vertical profile distribution of the latitudinal zone average (28°N-32°N) of horizontal and vertical wind speeds for different seasons. It can be seen that the atmospheric circulation structure over the region is relatively similar in winter, spring and autumn. During the three seasons, the low-level atmosphere in the SCB, where harbored by the Tibetan Plateau (TP) with its impact on midlatitude westerly winds, is characterized by weak winds and updrafts, while the high-level atmosphere is generally characteristic of strong winds and downdrafts, forming a “harbor” effect over the basin on the leeward slopes of the TP (Xu et al., 2015; Xu et al., 2016). On the contrary, the surface wind speed in the WSP area is substantially higher than those within the basin. A minimal horizontal wind speed zone exists between 700 hPa and 850hPa, where is typically the center of a lee-side vortex of immediately upstream TP (Zhang et al., 2019; Shu et al., 2021). The zero vertical velocity layer is at approximately the same altitude as the WSP. It is worth noting that sinking air aloft is stronger in winter than those in spring and autumn, with the updrafts being suppressed within a lower altitude range and at their weakest strength, forming a more unfavourable vertical structure for the transport and dispersion of pollutants. In contrast, although there are also areas of weak winds over the basin in summer, the atmosphere is almost entirely in upward motion, which is more conducive to the upward transport of pollutants. The pronounced difference in the vertical movement of the atmosphere in winter and summer should be related to the thermal effect of the TP, i.e., acting as a cold source in winter and heat source in summer (Zhang et al., 2000). The dynamical structure of the atmosphere under the influence of large topography may partly explain why haze is the most severe in the basin in winter, followed by spring and autumn, and least severe in summer (Cai et al., 2018). Overall, on the basis of the preceding analysis, diffusion conditions for pollution are poor in the SCB under the particular large topography, and become progressively better with increasing altitude. Besides, higher anthropogenic emissions within the basin would further reduced the visibility in the basin areas.
FIGURE 7. Vertical west–east cross–sections of horizontal (shading) and vertical (contour) wind speed averaged from 28°N to 31°N in (A) winter, (B) spring, (C) summer, and (D) autumn for the period 1999 to 2010. The solid and dashed lines indicate rise (ω<0) and descend (ω>0) of the airflow, respectively.
In order to isolate the effect of large topography on the atmospheric dynamic and thermal structure of the SCB, the latitudinal deviations in wind speed and temperature within the basin area (28°N∼31°N, 104°E-109°E) were calculated. As shown in Figure 8, in terms of average wind speed deviations, the topography reduced the wind speed below 500 hPa in the basin by about 0.5–2 m/s, while increased the wind speeds above 500 hPa significantly. The topography causes the most significant decrease in wind speed over the basin at 700-800 hPa, especially in winter and spring (Figure 8A), which are not conducive to the transport and dispersion of pollutants and tend to cause the accumulation of pollutants in the basin. The large topographic dynamic effects described above are present in different seasons, only to varying degrees. Based on the overall temperature-latitude deviation, it can be seen that, compared with other regions in the same latitudinal zone, the topography reduces the atmospheric temperature below 600 hPa over the basin, with the largest drop of 2.5–3°C at 800 hPa (Figure 8B), which is about the same as the height at which the topography reduces the wind speed over the basin most significantly. This suggests that the topography tends to make this layer of the basin more susceptible to off-ground inversions, which may be closely related to the overall downward warming of the leeward flow over the Tibetan Plateau (Xu et al., 2014). Under the warming effect of the leeward-slope airflow, inversions aloft are prone to form over the basin, with the highest likelihood and frequency of occurrence in winter (Figure 8B). The inversions would make the atmospheric stratification in the basin region more stable and the vertical transport of pollutants would be weakened, leading to the accumulation of pollutants in the basin and thus deteriorating air quality. There is a large seasonal variation in the effect of topography on temperature, with an overall decrease in temperature of a more pronounced magnitude in autumn and winter, and a relatively more pronounced inversion effect at 800-600 hPa. Combining the above analysis of the terrain effects on the wind-temperature structure over the basin shows that the most significant effects are experienced in winter, with both wind speed and temperature changing considerably due to the topography, and that an inversion layer is more likely to be generated than those in the other seasons. It is therefore important to pay more attention to the deteriorating effect of topography on air quality when developing pollution prevention and control in winter.
FIGURE 8. Overall and seasonal deviations of wind speed (A) and temperature (B) from the same latitudinal band for the period 1999 to 2010 in the range 104°E–109°E and 28°N–31°N (corresponding to the SCB region).
The topography of the Sichuan-Chongqing region is very complex, i.e. the SCB and the surrounding mountains are highly undulating, especially the TP that lies immediately to the west, resulting in a significant topography-dependent atmospheric environment. Studies have been conducted to quantify the influence of topography on atmospheric pollution in the SCB. In this study, the atmospheric environmental effects of topography in the Sichuan-Chongqing region are examined in terms of long-term changes in visibility, a proxy of aerosol concentration, and its connections with topography.
Visibility in the Sichuan-Chongqing region is significantly and positively correlated with the terrain height. Visibility in the SCB (defined as altitude less than 750 m) shows a significant positive correlation with wind speed, while at higher altitudes (larger than 1,500 m), visibility shows a negative correlation with wind speed. The effects of the large topography on the atmospheric environment of the region are mainly manifested in the following ways: The SCB is located on the leeward slope of the WSP. Under the influence of its special large topography, the leeward-slope effect leads to a weak wind area accompanied by ascending motions at low altitude, and descending motions with westerly winds at high altitude during the winter, spring, and autumn. A zone of minimal wind speed exists between 700 and 800 hPa in winter, spring and autumn, which corresponds to the height of the centre of the leeward-slope vortex. The descending motions could form a huge “vault” over the basin, which are not conducive to vertical transport and dispersion of pollutants. The “vault” effect is strongest in winter, followed by autumn and spring, and disappears in summer, being one of the key factors for the worst winter pollution in the basin. Therefore, more attention should be paid to the exacerbating effect of topography on pollution during winter pollution control. In addition, topography has a significant effect on the temperature structure over the basin, leading to a tendency for inversions to form near the surface of the basin as well as at high altitudes above 800 hPa, particularly at the high altitudes where the intensity of the off-ground inversion is very strong in the winter, autumn, and spring. The inversions will further lead to a more stagnant atmosphere over the basin, which is not conducive to the upward transport and dispersion of pollutants.
This study attempts to investigate the atmospheric effects of topography around the SCB from a long-term climatic perspective, which is important for furthering the understanding of the effects of topography on the atmospheric environment and the protection of the atmospheric environment within the basin. There may exist uncertainties in this study, such as the fact that most of the analysis in this study was carried out using statistical methods, such as the difference in meteorological conditions between areas within the basin and the same latitude zone was used in studying the role of topography, in which the interference of some other factors, such as differences in underlying surface and/or climatic background, may not be excluded. Therefore, future studies could combine a variety of methods, such as conducting long time scale topographic sensitivity tests in conjunction with chemical transport models, to reduce uncertainties and improve the quantitative understanding of the effects of topography in the SCB.
The raw data supporting the conclusion of this article will be made available by the authors, without undue reservation.
LZ and TZ conceived of the presented idea and designed the research framework with support from XG, XX, XZ, YL and LL. LZ and XG reviewed the literature and drafted the manuscript. LZ and YZ led the data analysis and interpreted the results together with KG. ZS provided critical feedback and constructive comments. All authors were involved in the discussion of the results.
This research was funded by National Key Research and Development Program of China (No. 2019YFC0214604), National Natural Science Foundation of China project (grant no. 42030608, 42090030, 41320714, 41975131), the National Key Foundation Study Developing Programs (grant no. 2019YFC0214601, 2019YFC0214801), and the CAMS Basis Research Project (Grant no. 2020Y001).
We are grateful to Sichuan Meteorological Bureau for providing the meteorological data used in this study.
The authors declare that the research was conducted in the absence of any commercial or financial relationships that could be construed as a potential conflict of interest.
All claims expressed in this article are solely those of the authors and do not necessarily represent those of their affiliated organizations, or those of the publisher, the editors and the reviewers. Any product that may be evaluated in this article, or claim that may be made by its manufacturer, is not guaranteed or endorsed by the publisher.
Allen, S., Allen, D., Phoenix, V. R., Le Roux, G., Durántez Jiménez, P., Simonneau, A., et al. (2019). Atmospheric transport and deposition of microplastics in a remote mountain catchment. Nat. Geosci. 12, 339–344. doi:10.1038/s41561-019-0335-5
Blyth, S. (2002). Mountain watch: environmental change & sustainable developmental in mountains. Kenya: UNEP/Earthprint.
Cai, H., Gui, K., and Chen, Q. (2018). Changes in haze trends in the sichuan-chongqing region, China, 1980 to 2016. Atmosphere 9, 277. doi:10.3390/atmos9070277
Carslaw, K., Boucher, O., Spracklen, D., Mann, G., Rae, J., Woodward, S., et al. (2010). A review of natural aerosol interactions and feedbacks within the Earth system. Atmos. Chem. Phys. 10, 1701–1737. doi:10.5194/acp-10-1701-2010
Carslaw, K., Lee, L., Reddington, C., Pringle, K., Rap, A., Forster, P., et al. (2013). Large contribution of natural aerosols to uncertainty in indirect forcing. Nature 503, 67–71. doi:10.1038/nature12674
Chang, L., Wu, Z., and Xu, J. (2021). Contribution of Northeastern Asian stratospheric warming to subseasonal prediction of the early winter haze pollution in Sichuan Basin, China. Sci. Total Environ. 751, 141823. doi:10.1016/j.scitotenv.2020.141823
Che, H., Zhang, X., Li, Y., Zhou, Z., and Qu, J. J. (2007). Horizontal visibility trends in China 1981–2005. Geophys. Res. Lett. 34, L24706. doi:10.1029/2007gl031450
Chen, Y., and Xie, S. (2012). Temporal and spatial visibility trends in the Sichuan Basin, China, 1973 to 2010. Atmos. Res. 112, 25–34. doi:10.1016/j.atmosres.2012.04.009
Deng, X., Tie, X., Wu, D., Zhou, X., Bi, X., Tan, H., et al. (2008). Long-term trend of visibility and its characterizations in the Pearl River Delta (PRD) region, China. Atmos. Environ. 42, 1424–1435. doi:10.1016/j.atmosenv.2007.11.025
Feng, S., Gao, D., Liao, F., Zhou, F., and Wang, X. (2016). The health effects of ambient PM2. 5 and potential mechanisms. Ecotoxicol. Environ. Saf. 128, 67–74. doi:10.1016/j.ecoenv.2016.01.030
Gao, S., and Ping, F. (2005). An experiment study of lee vortex with large topography forcing. Chin. Sci. Bull. 50, 248–255. doi:10.1360/03wd0550
Giovannini, L., Laiti, L., Serafin, S., and Zardi, D. (2017). The thermally driven diurnal wind system of the Adige Valley in the Italian Alps. Q. J. R. Meteorol. Soc. 143, 2389–2402. doi:10.1002/qj.3092
Gui, K., Che, H., Zeng, Z., Wang, Y., Zhai, S., Wang, Z., et al. (2020). Construction of a virtual PM2. 5 observation network in China based on high-density surface meteorological observations using the Extreme Gradient Boosting model. Environ. Int. 141, 105801. doi:10.1016/j.envint.2020.105801
Guo, Q., Wu, D., Yu, C., Wang, T., Ji, M., and Wang, X. (2022). Impacts of meteorological parameters on the occurrence of air pollution episodes in the Sichuan basin. J. Environ. Sci. 114, 308–321. doi:10.1016/j.jes.2021.09.006
Hu, J., Zhao, T., Liu, J., Cao, L., Wang, C., Li, Y., et al. (2022). Exploring the ozone pollution over the western Sichuan Basin, Southwest China: The impact of diurnal change in mountain-plains solenoid. Sci. Total Environ. 839, 156264. doi:10.1016/j.scitotenv.2022.156264
Hu, X. M., Ma, Z., Lin, W., Zhang, H., Hu, J., Wang, Y., et al. (2014). Impact of the loess plateau on the atmospheric boundary layer structure and air quality in the north China plain: a case study. Sci. Total Environ. 499, 228–237. doi:10.1016/j.scitotenv.2014.08.053
Kampa, M., and Castanas, E. (2008). Human health effects of air pollution. Environ. Pollut. 151, 362–367. doi:10.1016/j.envpol.2007.06.012
Kelly, F. J., and Zhu, T. (2016). Transport solutions for cleaner air. Science 352, 934–936. doi:10.1126/science.aaf3420
Kong, D., Ning, G., Wang, S., Cong, J., Luo, M., Ni, X., et al. (2021). Clustering diurnal cycles of day-to-day temperature change to understand their impacts on air quality forecasting in mountain-basin areas. Atmos. Chem. Phys. 21, 14493–14505. doi:10.5194/acp-21-14493-2021
Letson, F., Barthelmie, R. J., Hu, W., and Pryor, S. C. (2019). Characterizing wind gusts in complex terrain. Atmos. Chem. Phys. 19, 3797–3819. doi:10.5194/acp-19-3797-2019
Li, Y., Chen, Q., Zhao, H., Wang, L., and Tao, R. (2015). Variations in PM10, PM2. 5 and PM1. 0 in an urban area of the Sichuan Basin and their relation to meteorological factors. Atmosphere 6, 150–163. doi:10.3390/atmos6010150
Liao, T., Gui, K., Jiang, W., Wang, S., Wang, B., Zeng, Z., et al. (2018). Air stagnation and its impact on air quality during winter in Sichuan and Chongqing, southwestern China. Sci. Total Environ. 635, 576–585. doi:10.1016/j.scitotenv.2018.04.122
Liu, H., Yan, R., and Yang, J. (2021). Credibility and statistical characteristics of CAMSRA and MERRA-2 AOD reanalysis products over the Sichuan Basin during 2003–2018. Atmos. Environ. 244, 117980. doi:10.1016/j.atmosenv.2020.117980
Liu, M., Huang, Y., Ma, Z., Jin, Z., Liu, X., Wang, H., et al. (2017). Spatial and temporal trends in the mortality burden of air pollution in China: 2004–2012. Environ. Int. 98, 75–81. doi:10.1016/j.envint.2016.10.003
Liu, Q., Ding, D., Huang, M., Tian, P., Zhao, D., Wang, F., et al. (2018). A study of elevated pollution layer over the North China Plain using aircraft measurements. Atmos. Environ. 190, 188–194. doi:10.1016/j.atmosenv.2018.07.024
Luo, Y., Zheng, X., Zhao, T., and Chen, J. (2014). A climatology of aerosol optical depth over China from recent 10 years of MODIS remote sensing data. Int. J. Climatol. 34, 863–870. doi:10.1002/joc.3728
Ning, G., Wang, S., Yim, S. H. L., Li, J., Hu, Y., Shang, Z., et al. (2018). Impact of low-pressure systems on winter heavy air pollution in the northwest Sichuan Basin, China. Atmos. Chem. Phys. 18, 13601–13615. doi:10.5194/acp-18-13601-2018
Ning, G., Yim, S. H. L., Wang, S., Duan, B., Nie, C., Yang, X., et al. (2019). Synergistic effects of synoptic weather patterns and topography on air quality: a case of the Sichuan Basin of China. Clim. Dyn. 53, 6729–6744. doi:10.1007/s00382-019-04954-3
Poulos, G. S., and Pielke, R. A. (1994). A numerical analysis of Los Angeles basin pollution transport to the Grand Canyon under stably stratified, southwest flow conditions. Atmos. Environ. 28, 3329–3357. doi:10.1016/1352-2310(94)00127-7
Qiao, X., Jaffe, D., Tang, Y., Bresnahan, M., and Song, J. (2015). Evaluation of air quality in Chengdu, Sichuan Basin, China: are China’s air quality standards sufficient yet? Environ. Monit. Assess. 187, 250–311. doi:10.1007/s10661-015-4500-z
Qin, S., Shi, G., Chen, L., Wang, B., Zhao, J., Yu, C., et al. (2010). Long-term variation of aerosol optical depth in China based on meteorological horizontal visibility observations. Chin. J. Atmos. Sci. 34, 449–456.
Rosenfeld, D., Dai, J., Yu, X., Yao, Z., Xu, X., Yang, X., et al. (2007). Inverse relations between amounts of air pollution and orographic precipitation. Science 315, 1396–1398. doi:10.1126/science.1137949
Schmidli, J., and Rotunno, R. (2010). Mechanisms of along-valley winds and heat exchange over mountainous terrain. J. Atmos. Sci. 67, 3033–3047. doi:10.1175/2010jas3473.1
Shang, Y., Sun, Z., Cao, J., Wang, X., Zhong, L., Bi, X., et al. (2013). Systematic review of Chinese studies of short-term exposure to air pollution and daily mortality. Environ. Int. 54, 100–111. doi:10.1016/j.envint.2013.01.010
Shu, Z., Liu, Y., Zhao, T., Xia, J., Wang, C., Cao, L., et al. (2021). Elevated 3D structures of PM<sub>2.5</sub> and impact of complex terrain-forcing circulations on heavy haze pollution over Sichuan Basin, China. Atmos. Chem. Phys. 21, 9253–9268. doi:10.5194/acp-21-9253-2021
Shu, Z., Liu, Y., Zhao, T., Zhou, Y., Habtemicheal, B. A., Shen, L., et al. (2022a). Long-term variations in aerosol optical properties, types, and radiative forcing in the Sichuan Basin, Southwest China. Sci. Total Environ. 807, 151490. doi:10.1016/j.scitotenv.2021.151490
Shu, Z., Zhao, T., Liu, Y., Zhang, L., Ma, X., Kuang, X., et al. (2022b). Impact of deep basin terrain on PM2.5 distribution and its seasonality over the Sichuan Basin, Southwest China. Environ. Pollut. 300, 118944. doi:10.1016/j.envpol.2022.118944
Sun, K., Liu, H-N., Ding, A-J., and Wang, X-Y. (2016). WRF-Chem simulation of a severe haze episode in the Yangtze River Delta, China. Aerosol Air Qual. Res. 16, 1268–1283. doi:10.4209/aaqr.2015.04.0248
Ting, M., and Wang, H. (2006). The role of the North American topography on the maintenance of the Great Plains summer low-level jet. J. Atmos. Sci. 63, 1056–1068. doi:10.1175/jas3664.1
Tuononen, M., Sinclair, V., and Vihma, T. (2015). A climatology of low‐level jets in the mid‐latitudes and polar regions of the Northern Hemisphere. Atmos. Sci. Lett. 16, 492–499. doi:10.1002/asl.587
Wagner, J., Gohm, A., and Rotach, M. (2015). The impact of valley geometry on daytime thermally driven flows and vertical transport processes. Q. J. R. Meteorol. Soc. 141, 1780–1794. doi:10.1002/qj.2481
Wang, D., Miao, J., and Tan, Z. (2013). Impacts of topography and land cover change on thunderstorm over the Huangshan (Yellow Mountain) area of China. Nat. Hazards (Dordr). 67, 675–699. doi:10.1007/s11069-013-0595-0
Wang, H., Shi, G., Tian, M., Zhang, L., Chen, Y., Yang, F., et al. (2017). Aerosol optical properties and chemical composition apportionment in Sichuan Basin, China. Sci. Total Environ. 577, 245–257. doi:10.1016/j.scitotenv.2016.10.173
Wang, H., Tian, M., Chen, Y., Shi, G., Liu, Y., Yang, F., et al. (2018a). Seasonal characteristics, formation mechanisms and source origins of PM<sub>2.5</sub> in two megacities in Sichuan Basin, China. Atmos. Chem. Phys. 18, 865–881. doi:10.5194/acp-18-865-2018
Wang, X., Dickinson, R. E., Su, L., Zhou, C., and Wang, K. (2018b). PM2.5 pollution in China and how it has been exacerbated by terrain and meteorological conditions. Bull. Am. Meteorological Soc. 99, 105–119. doi:10.1175/bams-d-16-0301.1
Wang, X-C., Klemeš, J. J., Dong, X., Fan, W., Xu, Z., Wang, Y., et al. (2019). Air pollution terrain nexus: A review considering energy generation and consumption. Renew. Sustain. Energy Rev. 105, 71–85. doi:10.1016/j.rser.2019.01.049
Whiteman, C. D., Bian, X., and Zhong, S. (1999). Wintertime evolution of the temperature inversion in the Colorado Plateau Basin. J. Appl. Meteor. 38, 1103–1117. doi:10.1175/1520-0450(1999)038<1103:weotti>2.0.co;2
Wu, K., Wang, Y., Qiao, Y., Liu, Y., Wang, S., Yang, X., et al. (2022). Drivers of 2013–2020 ozone trends in the Sichuan Basin, China: Impacts of meteorology and precursor emission changes. Environ. Pollut. 300, 118914. doi:10.1016/j.envpol.2022.118914
Xing, Y-F., Xu, Y-H., Shi, M-H., and Lian, Y-X. (2016). The impact of PM2. 5 on the human respiratory system. J. Thorac. Dis. 8, E69–E74. doi:10.3978/j.issn.2072-1439.2016.01.19
Xu, X., Wang, Y., Zhao, T., Cheng, X., Meng, Y., and Ding, G. (2015). “Harbor” effect of large topography on haze distribution in eastern China and its climate modulation on decadal variations in haze. Chin. Sci. Bull. 60, 1132–1143. doi:10.1360/N972014-00101
Xu, X., Zhao, T., Liu, F., Gong, S. L., Kristovich, D., Lu, C., et al. (2016). Climate modulation of the Tibetan Plateau on haze in China. Atmos. Chem. Phys. 16, 1365–1375. doi:10.5194/acp-16-1365-2016
Xu, X., Zhao, T., Lu, C., Guo, Y., Chen, B., Liu, R., et al. (2014). An important mechanism sustaining the atmospheric "water tower" over the Tibetan Plateau. Atmos. Chem. Phys. 14, 11287–11295. doi:10.5194/acp-14-11287-2014
Yin, D., Zhao, S., Qu, J., Yu, Y., Kang, S., Ren, X., et al. (2020). The vertical profiles of carbonaceous aerosols and key influencing factors during wintertime over western Sichuan Basin, China. Atmos. Environ. 223, 117269. doi:10.1016/j.atmosenv.2020.117269
Zardi, D., and Whiteman, C. D. (2013). “Diurnal mountain wind systems,” in Mountain weather research and forecasting (Dordrecht: Springer), 35–119.
Zhang, D., Fengquan, L., and Jianmin, B. (2000). Eco-environmental effects of the qinghai-tibet plateau uplift during the quaternary in China. Environ. Geol. 39, 1352–1358. doi:10.1007/s002540000174
Zhang, L., Guo, X., Zhao, T., Gong, S., Xu, X., Li, Y., et al. (2019). A modelling study of the terrain effects on haze pollution in the Sichuan Basin. Atmos. Environ. 196, 77–85. doi:10.1016/j.atmosenv.2018.10.007
Zheng, Y., Che, H., Xia, X., Wang, Y., Yang, L., Chen, J., et al. (2021). Aerosol optical properties and its type classification based on multiyear joint observation campaign in north China plain megalopolis. Chemosphere 273, 128560. doi:10.1016/j.chemosphere.2020.128560
Keywords: Sichuan Basin, atmospheric environment, visibility, climate, topographic effects
Citation: Zhang L, Guo X, Zhao T, Xu X, Zheng X, Li Y, Luo L, Gui K, Zheng Y and Shu Z (2022) Effect of large topography on atmospheric environment in Sichuan Basin: A climate analysis based on changes in atmospheric visibility. Front. Earth Sci. 10:997586. doi: 10.3389/feart.2022.997586
Received: 19 July 2022; Accepted: 09 August 2022;
Published: 07 September 2022.
Edited by:
Meng Gao, Hong Kong Baptist University, Hong Kong, SAR, ChinaReviewed by:
Jinhui Gao, Chengdu University of Information Technology, ChinaCopyright © 2022 Zhang, Guo, Zhao, Xu, Zheng, Li, Luo, Gui, Zheng and Shu. This is an open-access article distributed under the terms of the Creative Commons Attribution License (CC BY). The use, distribution or reproduction in other forums is permitted, provided the original author(s) and the copyright owner(s) are credited and that the original publication in this journal is cited, in accordance with accepted academic practice. No use, distribution or reproduction is permitted which does not comply with these terms.
*Correspondence: Tianliang Zhao, dGx6aGFvQG51aXN0LmVkdS5jbg==
Disclaimer: All claims expressed in this article are solely those of the authors and do not necessarily represent those of their affiliated organizations, or those of the publisher, the editors and the reviewers. Any product that may be evaluated in this article or claim that may be made by its manufacturer is not guaranteed or endorsed by the publisher.
Research integrity at Frontiers
Learn more about the work of our research integrity team to safeguard the quality of each article we publish.