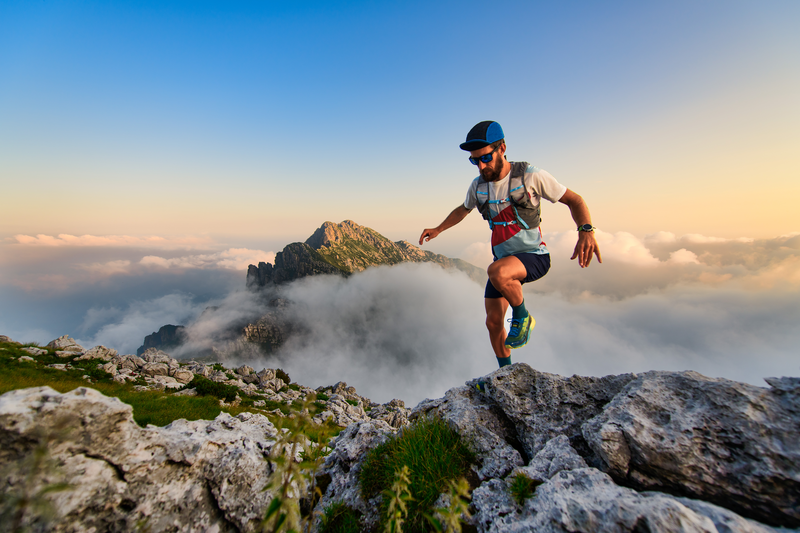
95% of researchers rate our articles as excellent or good
Learn more about the work of our research integrity team to safeguard the quality of each article we publish.
Find out more
ORIGINAL RESEARCH article
Front. Earth Sci. , 07 September 2022
Sec. Economic Geology
Volume 10 - 2022 | https://doi.org/10.3389/feart.2022.995394
This article is part of the Research Topic Exploration, Exploitation, and Utilization of Coal-Measure Gas into the Future View all 16 articles
Based on the results of previous research on the fracture systems of reservoirs of middle- and low-rank coal, we took high-rank coal in the southern Qinshui Basin as the study object in this paper and summarize the characteristics of both macro- and micro-fractures in reservoirs of different rank coals, establish a geometric model of the fracture network for different rank coals, and explore the mechanism of coal reservoir permeability change under different conditions. The study found that the structure of the fracture network of high-rank coal developed unevenly. The high-rank coal had the characteristics of rift created outside, micro-fracture development, and undeveloped endogenous fracture, which can be used to improve the permeability of the coal reservoir, to a certain extent. We concluded that given the absence of a seepage aisle in the high-rank coal, there is a rapid increase in reservoir permeability from low to high rate during the seepage process of the fracture network. However, the seepage rate in other coal rank reservoirs increases smoothly. Due to fracture compression and coal matrix shrinkage, the permeability of the coal reservoir first decreases and then increases during the drainage stage. At the same rate of pressure drop, the permeability of high-rank coal reservoir decreases at the fastest rate, followed by that of low-rank coal reservoir, and that of middle-rank coal reservoir, in that order.
Rocks form various kinds of fissures during long-term geological action, and the study of their morphological characteristics has important engineering significance, as well as coal as a kind of rock (Salmachi et al., 2016; Zhao et al., 2021). Coal reservoirs have a dual pore-fracture structure, and the connectivity and dimensions of their pore fracture system are crucial to their permeability (Yao et al., 2006; Gao et al., 2017; Shen et al., 2019; Shang et al., 2020). The permeability rate is one of the parameters affecting the effectiveness of the extraction of a coal reservoir’s coalbed methane (CBM). It reflects the ease of fluid seepage within the coal reservoir and determines the transport and output of CBM. The study of characteristics of the pore and fracture development of coal seams is important for in-depth understanding of the micro-mechanism of fluid fugacity and mass transfer processes in coal seams and for the optimization of geological suitability development (Moore., 2012; Giffin S et al., 2013; Liu et al., 2021). At present, substantial progress has been made in the gas production areas of high-rank coal reservoirs represented by the Qinshui Basin, the gas production areas of middle-rank coal represented by the eastern margin of the Ordos Basin, and the gas production areas of low-rank coal represented by the Erlian Basin and the southern margin of the Junggar Basin. These results obtained are credited to the extensive basic scientific research work conducted by previous authors on the gas production mechanisms of reservoirs of different ranks of coal, as well as verification of the existence of different structural characteristics of reservoirs of different ranks of coal and corresponding differences in gas transport in the reservoirs (Liu et al., 2017; Li et al., 2018; Yu et al., 2018). The pore system controls the desorption-diffusion transport of coalbed methane, and the fracture system mainly determines the large-scale seepage output of the gas (Li et al., 2014; Fang et al., 2019; Fang et al., 2020). Due to differences in the development of connected pores, endogenous fractures, and exogenous fractures in the structure of the reservoirs, each factor will make a different contribution to the permeability values of the reservoir. In general, the seepage capacity of connected pores, endogenous fractures, and exogenous fractures decreases from exogenous fractures to endogenous fractures to connected pores in decreasing order (Zhou et al., 2018). Theoretically, the natural fracture development of the coal seam is conducive to increasing the permeability of the coal seam. Domestic and foreign scholars have shown through a large number of experimental tests that the differences in the degree of fracture network development and connectivity in coal seams have a key effect on the permeability of coal seams (Sampath et al., 2019; Wu et al., 2019; Zhang et al., 2019). For reservoirs of higher rank coal, there is a higher contribution of exogenous fractures due to the non-development of endogenous fractures and connected pore spaces. For reservoirs of middle- and low-rank coal, the contribution of endogenous fractures and connected pores to the permeability of the coal reservoir is high, and middle-rank coal is characterized by a relatively high contribution of endogenous fractures, while low-rank coal is characterized by a relatively high contribution of connected pores (Sang et al., 2009).
Previous studies on permeability in high-ranking coal reservoirs have mostly focused on the overall effect, which is limited to the overall size of permeability, while the refinement of the fracture network in high-ranking coal reservoirs and the resulting variation process of permeability size are still lacking. Research in this area will have an important impact on the drainage process in CBM development, where the variation of permeability size determines the selection of drainage parameters.
Therefore, to further illuminate the multi-stage and multi-genesis fracture network system of high-rank coal reservoirs, this paper takes high-rank coal in the southern Qinshui Basin as the research object, describes the characteristics of high-rank coal fracture network (semi-) quantitatively by means of downhole field observation, optical microscope and scanning electron microscope observation, and compared and analyzed with the previous research results of middle and low-rank coal, establishes the geometric model of fracture network of different coal rank coals, highlights the macroscopic and microscopic characteristics of high-rank coal, the macroscopic and microscopic characteristics of fractures in high-rank coal reservoirs are highlighted, their control on reservoir permeability is investigated, and the mechanism of change in permeability of high-rank coal reservoirs under different drainage stages is discussed, with a view to providing a theoretical basis for the optimization of coalbed methane extraction process in the study area.
The object of this research is the 3# coal seam in the area, the coal thickness is relatively stable, roughly between 5.0 and 7.0 m, and shows a trend of gradually increasing from south to north; the burial depth in most areas is between 500 and 1000 m, and the burial depth is relatively shallow; the coal metamorphism in the area is high and belongs to the anthracite stage, and the coal grade shows a significantly increasing trend from N to S. The macroscopic coal rock type is mainly semi-bright coal, followed by bright coal, and locally also intercalated with dull coal stratification; microscopic components are mainly mirror group, common matrix mirror body, followed by the inert group, common semi-filament body, and rare shell group. The average ash is about 12.98%, which is low ash coal, and the average volatile matter (Vdaf) is about 7.08%; the average moisture (Mad) is about 1.34%.
In this study, a combination of field investigation and laboratory observation was adopted to conduct a comprehensive and systematic statistical analysis of the fracture network characteristics of high-order coal in the study area from macroscopic to microscopic. The macroscopic fracture characteristics of high-order coal in the study area were firstly studied by using fresh coal walls from the underground of three pairs of coal mines, including Sihe, Tang’an, and Chengzhuang, combined with the sketch map of four coal rock profiles drawn and the borehole core data collected from 46 CBM parameter wells and some exploration wells; then the microscopic fractures and ultramicroscopic fractures of 40 coal samples collected were observed and counted in the laboratory by using an optical microscope and scanning electron microscope to study The microscopic fracture characteristics of high-order coal were then studied in the laboratory using an optical microscope and scanning electron microscope. See Figure 1 for details of sampling point locations.
Previous studies have distinguished the coal reservoir fracture system into visualizable exogenous fractures and endogenous fractures (cleats), and micro-fractures visible under an optical microscope (Zhong et al., 2006; Pan et al., 2016). In this paper, we take the high-rank coal of southern Qinshui Basin as the study object to reveal the multi-level and multi genesis fracture network system in high-rank coal reservoirs. This method can provide us with a way to systematically observe the reservoirs of high-rank coal and study them from macro and micro angles. Accordingly, the coal seam can be divided into two categories: macro-fractures and micro-fractures.
Based on the mechanical mechanism of fracture formation in coal, macro-fractures can be divided into exogenous fractures and endogenous fractures, among which endogenous fractures can be classified as face cleat and butt cleat. Statistical analysis of macroscopic cracks in fresh coal walls from underground coal wells and drilled coal cores in Sihe, Tangan and Chengzhuang in the study area was carried out.
The endogenous fractures in the area are generally not developed, and the degree of their development varies at different observation sites. Tracing the direction of the fracture along the coal seam laminate, we know that the main directions of the exogenous fractures in the area are two groups of the NE direction and the NW direction, among which one group of the NE direction corresponds to the NW-SE direction extrusion in the Yanshan period and one group of the NW direction corresponds to the NE-SW direction extrusion in the Xishan period, and the two groups intersect each other and become a network. The average density of the fracture development is 1–5 fractures per meter, and the length of the extension along the laminated surface varies greatly, from several meters to hundreds of meters. The fracture generally cuts through the whole coal seam, sometimes even cutting through the top and bottom plate It is not limited to any coal rock component, and its surface is flat or serrated. It is often filled with coal dust, calcite, and other fillers, which affects the permeability of the coal reservoir in the area.
Endogenous fractures (cleats) mainly develop in bright coal and semi-bright coal, and their development varies from a few millimeters to a few meters. They are mainly comprised of two groups in a dominant development direction. The direction of the first group is roughly between N33°–66°E, and the cleats of this group, which are face cleats, are developed, with a density of 27–120 pieces per meter. The direction of the second group of cleats, which are butt cleats, is roughly between N42°–54°W, but the development of this group’s cleats is relatively weak, with a density of 24–60 pieces per meter. As the cleat density is lower, the cleat of the second group is a butt cleat, whereas the development of the length of the cleat is controlled by the face cleat. Face and butt cleats often intersect at nearly right angles, and the inclination of the cleat surface is generally greater than 70°. Although the width of a cleat varies from a few microns to several hundred microns, most are filled by calcite in a tight state and are not conducive to the permeability of coal reservoirs, meanwhile, the development of endogenous fractures (cleats) is strictly controlled by the components and is mainly seen in the vitrinite band of bright coal, semi-bright coal, or semi-dark coal, and is not developed in dull coal. The density of the cleat development of high-rank coal is much less than that of middle-rank coal (800–1200 pieces per meter).
The scale of micro-fracture development, which can be further divided into two different scale levels, i.e. the micro-fracture that can be observed under an optical microscope and the ultra-micro-fracture that can be observed only under a scanning electron microscope, which mostly develop in vitrain and bright coal, is significantly lower than that of macro-fracture development. In our study, 40 coal samples were collected from coal seam No. 3 in the study area for observation and statistical analysis. The statistical results are detailed in Table 1.
Based on the study of micro-fractures under an optical microscope in high-rank coal reservoirs, we found three common micro-fracture types: shrinkage micro-fractures, hydrostatic micro-fractures, and tectonic micro-fractures. The first two are endogenous fractures. They are strictly restricted by their components which only develop in the vitrinite group. Shrinkage micro-fractures correspond to endogenous fractures (cleats) in macro-fractures. Their main morphological features are short, straight and oriented. There is no displacement on both sides of the fissures, which are largely perpendicular to the laminae and arranged at equal intervals, as in Figure 2A, with lengths ranging between 86.93 and 1636 μm, varying widely, widths ranging between 0.53 and 43.65 μm, usually greater than 1 μm, densities ranging between 44 and 640 pieces/cm2 with average fracture numbers of 177 pieces/cm2. Hydrostatic micro-fractures are affected by the pressure of overlying static rock during their development and are disorderly and undirected. Their main morphological features are short, curved, dense and disorderly, as in Figure 2B, with lengths ranging from 567.9 to 1636 μm, widths ranging from 0.1 to 2 μm or smaller, and densities ranging from 57 to 81 piece/cm2, with an average value of 69 pieces/cm2. The tectonic fissures are not restricted to specific components because their formation is subject to tectonic movements, and they are obliquely intersected by the laminae. From the cutting relationship between the two groups of fractures in the Figure 2C, it can be seen that the relatively long fissures are formed later than the relatively short ones, corresponding to two phases of tectonics. The relatively long fissures are generally 4 mm in height or even larger, while the relatively short fissures range in height from 757.8 to 1760 μm and in width from 2.057 to 8.483 μm, with a density of 49–65 pieces/cm2 and an average of 59 pieces/cm2. At the same time, the density of development of the three micro-fracture types is much more extensive than the endogenous fractures (cleats) and no mineral filling. Under the optical microscope, the micro-fracture of the coal reservoir in the area partially cut through the pore space or cleat with the coal seam cleat, which becomes an important channel to communicate the desorption-diffusion pore space with the cleat fracture network.
FIGURE 2. Development characteristic of micro-fracture in high-rank coal reservoirs. (A) Shrinkage micro-fracture which features are short, straight and oriented (B) Hydrostatic micro-fracture which features are short, curved, dense and disorderly (C) Tectonic micro-fracture which are cutting distribution by tectonic influence.
Based on the study under a scanning electron microscope of ultra-micro-fractures in high-rank coal reservoirs, we found that: ultra-micro-fractures are developed in high-rank coal reservoirs. They are caused by the directional arrangement of the coal molecules and are unique to high-rank coal and absent in middle- and low-rank coal reservoirs. The ultra-micro-fracture usually develops in a group, and the length of the fracture is generally less than 10 μm, the width is generally less than 0.1 μm or even smaller, and it is without mineral filling (Figure 3).
According to the fracture structure development characteristics of the above-mentioned high-rank coal, combined with previous research results in middle- and low-rank coal, (Fu et al., 2001a; Bi et al., 2001; Jia et al., 2015), a structural connectivity network model of coal reservoirs of different coal rank was established (Figures 4, 5). Both exogenous fractures and cleats of different ranks of coal are commonly developed in two groups, and both are roughly vertical in orientationThe coal reservoir is cut into a series of small matrix blocks, in which coalbed methane is mainly transported by seepage, flowing from cleat to exogenous fractures and then transported to the wellbore. The difference is in the different development densities of cleats in different ranks of coal. For dull type coal and semi-dull type coal, the density of the cleats in middle-rank coal and high- or low-rank coal is not very different. The cleats of bright type and semi-bright type coal are mostly developed in middle-rank coal, and are more sparsely developed in high- and low-rank coal, as shown in the left side of Figure 4 and Figure 5, as a whole, the exogenous fracture and microfracture development densities of high-rank coal and middle- and low-rank coal are comparable, and the cleats density development is smaller.
The seepage channels in coal reservoirs of different ranks of coal vary in the structure of the fracture network. The overall diffusion and transport of gas in the coal body gradually moves from the inside to the outside of the coal body through the filling pore, connecting pore, micro-fracture, endogenous fracture, and exogenous fracture. However, in the connecting pore stage, high-rank coal lacks the continuity distribution of the transition pore and the mesopore, and the gas mostly diffuses directly from the micro-pore to the micro-fracture (Zhang et al., 2011), as shown in the right side of Figure 4 and Figure 5, the pore development of the middle- and low-rank coal reservoir is balanced, the high-rank coal develops more microporous and sparse macropores, the transition pores and mesopores are not developed, but some super-microscopic fractures are developed. In the connecting stage from the endogenous fracture to the exogenous fracture, it is mostly connected from the micro-fracture to the exogenous fracture, and the connection from the secondary fracture (cleat) to the exogenous fracture is missing in the middle, which will reduce the transport path of gas in the coal seam and cause the rapid increase of the overall gas seepage rate. High-rank coal reservoirs contain high gas content, and the difficulty of coalbed methane extraction is its unique property, but the thermal evolution and tectonic evolution characteristics of high-rank coal reservoirs have a significant impact on the reservoir structure. The development of the regional thermal metamorphic anthracite pore structure is favorable to reservoir formation, and the tectonic evolution effect is favorable to the development of exogenous fractures in coal seams, which improves the permeability of coal reservoirs.
The linear seepage permeability, K0, within a regular fracture is related to the fracture width, w, and spacing, s, as follows (Fu et al., 2001b):
Where: w is the single fracture width (mm), S is the spacing of two adjacent fractures (mm).
The maximum fracture width of coal reservoirs under confining pressure conditions does not exceed a few tens of microns, so when considering the relationship between fracture development characteristics and permeability of different coal rank reservoirs, the fracture width with a very small variation range can be considered a constant value, and only the fracture spacing variation is considered, i.e., the numerical variation of fracture development density is used instead.
Based on the previous analysis of characteristics of fracture network development, the development density of each scale of fracture in reservoirs of different ranks of coal is substituted into Eq. 1, and the mechanism of the permeability change in reservoirs of different ranks of coal under the original state can be obtained (Figure 6). Natural coal samples in reservoirs of high-rank coal have high compressive strength and high brittleness, and are easily ruptured into blocks under the exertion of external forces, and exogenous fractures are developed under the transformation effect of latter stage tectonic movements, and tectonic fractures are developed in micro-fractures. Scanning electron microscope observations and mercury intrusion porosimetry (MIP) indicate that micro-fractures are significantly more developed than endogenous fractures in reservoirs of high-rank coal, while micro-fractures are fully developed in medium- and low-rank coals. Therefore, if the seepage field of middle- and low-rank coal is considered a kind of multi-level road network, then the seepage field of high-rank coal is a network from low rank road directly to high rank road and the transition of secondary road is missing in between. Accordingly, its seepage characteristics are significantly different from those of middle- and low-rank coals, and its seepage rate generally increases with the increase of the fracture width due to the balanced development of fractures in middle- and low-rank coals. While high-rank coal lacks the transition of secondary roads, its seepage rate has a rapid increase between the lower and higher roads.
FIGURE 6. Characteristics of permeability change of different coal rank reservoirs of in original state.
According to the model of permeability change during CBM well drainage, i.e., the Shi-Durucan (S-D) model (Shi and Durucan, 2004; Shi and Durucan, 2010):
Where: Cf is the fracture compression volume factor (MPa-1), σ0 is the effective stress at the original reservoir pressure p0.
Where the effective stresses are as follows:
Assuming that the shrinkage of the substrate and the gas desorption are proportional, then Eq. 3 can be expressed as:
Where: αs is the matrix shrinkage expansion coefficient; V(p) is the adsorption volume at reservoir pressure; b is the Langmuir constant; V0 is the gas adsorption volume at the initial reservoir pressure p0.
The first and second terms in Eq. 4 represent the effects of compression of the fracture and shrinkage of the matrix, respectively. In the drainage gas extraction stage (p< p0), the fracture compresses and the matrix contracts, and the amount of change in σ- s0 depends on the relative amount of change in the interaction of the two factors.
Define
Let
Pequilibrium is the pressure point where the fracture compression and matrix shrinkage effects reach equilibrium, and it can be seen that Pequilibrium is inversely proportional to the initial seam pressure, which means that the higher the original reservoir pressure is when the other properties of the coal seam are the same, the lower the pressure value at which the fracture compression and matrix shrinkage effects on coal seam permeability reach equilibrium, and the longer the time in negative effect.
Find the minimum value of pressure point Pmin corresponding to the minimum value of Δσ:
From the above equation, we can understand that the minimum value is independent of the initial pressure.
In summary, the theoretical trend of permeability change during the drainage process can be obtained (Figure 7). We can understand that: with the minimum value of Pmin as the dividing point, the change of permeability shows the characteristics of first decreasing and then increasing, when P>Pmin, fracture compression plays a dominant role, and the permeability becomes smaller as the pressure decreases; when P<Pmin, matrix contraction plays a dominant role and the permeability starts to rebound and increase.
According to the drainage information of well 1-1 in the area, the dynamic fluid level of the well reached stability after 36 days of drainage, and the drainage data after 36 days were taken for calculation to obtain the permeability change of well 1-1, as shown in Figure 8.
As the drainage proceeds, the fluid pressure in the coal reservoir decreases and the effective stress increases, resulting in the gas permeability decreasing first and reaching a low point, and after 250 days of drainage, the gas permeability rises rapidly due to the dominant role of coal matrix contraction. The total permeability of coal reservoirs generally decreases and then increases with time. The reservoir permeability is low at the beginning of drainage and the permeability decreases, and with further drainage, the positive effect of coal matrix shrinkage increases, and the reservoir permeability increases. The course of the total permeability and the trend of the theoretical permeability are in good agreement (Figure 9).
Therefore, the permeability variation in reservoirs of different ranks of coal can be deduced from Eq. 7. Previous studies have shown that the Langmuir pressure tends to decrease gradually with increasing reflectance of the vitrinite group (Zhang et al., 2004), i.e., the Langmuir constant is greatest for high-rank coals. Young’s modulus shows a trend of decreasing and then increasing and is lowest in the middle-rank coal stage (Shen and Zhang, 2000). Using the above trend in combination with Eq. 7 we can see that: the value of Pmin in reservoirs of high-rank coal is the largest, and under the same pressure drop rate conditions, the Pmin pressure point is reached first and the permeability drop rate is the fastest. The reservoir of middle-rank coal has the smallest value of Pmin and reaches the minimum value the latest, with a slower rate of permeability decline, while the low-rank coal is in between. The result corresponds to the contribution of fracture network structure to reservoir permeability for different ranks of coals as described in the previous section, where the permeability of reservoirs of higher rank coal depends on exogenous fractures, which are also necessarily most influenced by fracture compression.
The structure of the high-rank coal fracture network is unevenly developed, and is characterized by the development of exogenous fractures and micro-fractures, but not endogenous fractures; the development of unique ultra-micro-fractures can improve the permeability of the reservoir, to a certain extent.
There is a corresponding absence of seepage channels for high-rank coals, and in the original state, with the increase of fracture width, the seepage rate of middle- and low-rank coals shows a smooth increasing trend in general, while there is a process of rapid increase from low to high seepage rate due to the lack of endogenous fractures.
Under the influence of fracture compression and the coal matrix shrinkage effect, the permeability of the coal reservoir in the drainage stage has a trend of first decreasing and then increasing. Under the same pressure drop rate condition, the permeability of the high rank coal reservoir decreases the fastest, followed by low-rank coal, and middle-rank coal, in that order.
The original contributions presented in the study are included in the article/Supplementary material, further inquiries can be directed to the corresponding author.
YL, ZX, and XZ contributed to the conception and design of the study. YL organized the database and performed the statistical analysis, and also wrote the first draft of the manuscript. ZX and XZ wrote sections of the manuscript. All authors contributed to manuscript revision, read, and approved the submitted version.
The author(s) disclosed receipt of the following financial support for the research, authorship, and/or publication of this article: This work was supported by National Natural Science Foundation of China (Special Characterization of Tectonic Coal Structure and Its Control Mechanism on Coalbed Methane Occurrence and Migration) (No. 41072113), National Natural Science Foundation of China (Dynamic change law and control mechanism of reservoir permeability during surface mining of coalbed methane) (No. 41372162).
The authors declare that the research was conducted in the absence of any commercial or financial relationships that could be construed as a potential conflict of interest.
All claims expressed in this article are solely those of the authors and do not necessarily represent those of their affiliated organizations, or those of the publisher, the editors and the reviewers. Any product that may be evaluated in this article, or claim that may be made by its manufacturer, is not guaranteed or endorsed by the publisher.
Bi, J., Su, X., Han, D., et al. (2001). The relation between cleat frequency and coal rank. J. China Coal Soc. 26 (4), 346–349. doi:10.3321/j.issn:0253-9993.2001.04.003
Fang, H. H., Sang, S. X., and Liu, S. Q. (2020). A methodology for characterizing the multiscale pores and fractures in anthracite and semi-anthracite coals and its application in analysis of the storage and permeable capacity of coalbed methane. SPE Reserv. Eval. Eng. 23 (1), 177–186. doi:10.2118/195672-pa
Fang, H. H., Sang, S. X., Liu, S. Q., and Du, Y. (2019). Methodology of three-dimensional visualization and quantitative characterization of nanopores in coal by using FIB-SEM and its application with anthracite in Qinshui basin. J. Petroleum Sci. Eng. 182, 106285. doi:10.1016/j.petrol.2019.106285
Fu, X., Qin, Y., Jiang, B., et al. (2001b). Compress experiment of coal cleat and mathematical simulation of coal reservoir permeability. J. China Coal Socirty 26 (6), 573–577. doi:10.3321/j.issn:0253-9993.2001.06.003
Fu, X., Qin, Y., and Li, G. (2001a). Advance in study of coal reservoir Perme AtingAbility. J. Liaoning Tech. Univ. Nat. Sci. 20 (6), 739–743. doi:10.3969/j.issn.1008-0562.2001.06.002
Gao, W., Yi, T., Jin, J., et al. (2017). Pore integrated fractal characteristics of coal sample in western Guizhou and its impact to porosity and permeability. J. China Coal Soc. 42 (5), 1258–1265. doi:10.13225/j.cnki.jccs.2016.0869
Giffin, S., Littke, R., Klaver, J., and Urai, J. (2013). Application of BIB–SEM technology to characterize macropore morphology in coal. Int. J. Coal Geol. 114, 85–95. doi:10.1016/j.coal.2013.02.009
Jia, J., Zhang, H., Jia, Q., et al. (2015). Status and prospect: Study on the cleat system in coal reservoir. Nat. Gas. Geosci. 26 (9), 1621–1628. doi:10.11764/j.issn.1672-1926.2015.09.1621
Li, G., Meng, Z., and Wang, B. (2014). Diffusion and seepage mechanisms of high rank coal-bed methane reservoir and its numericalsimulation at early drainagerate. J. China Coal Soc. 39 (9), 1919–1926. doi:10.13225/j.cnki.jccs.2014.8024
Li, S., Tang, D., Pan, Z., Xu, H., Tao, S., Liu, Y., et al. (2018). Geological conditions of deep coalbed methane in the eastern margin of the Ordos Basin, China: Implications for coalbed methane development. J. Nat. Gas Sci. Eng. 53, 394–402. doi:10.1016/j.jngse.2018.03.016
Liu, S., Sang, S., Wang, G., Ma, J., Wang, X., Wang, W., et al. (2017). FIB-SEM and X-ray CT characterization of interconnected pores in high-rank coal formed from regional metamorphism. J. Petroleum Sci. Eng. 148, 21–31. doi:10.1016/j.petrol.2016.10.006
Liu, S., Wang, H., Wang, R., et al. (2021). Research advances on characteristics of pores and fractures in coal seams. Acta Sedimentol. Sin. 39 (01), 212–230. doi:10.14027/j.issn.1000-0550.2020.064
Moore, T. A. (2012). Coalbed methane: A review. Int. J. Coal Geol. 101, 36–81. doi:10.1016/j.coal.2012.05.011
Pan, J. N., Wang, K., Hou, Q. L., Niu, Q., Wang, H., and Ji, Z. (2016). Micro-pores and fractures of coals analysed by field emission scanning electron microscopy and fractal theory. Fuel 164, 277–285. doi:10.1016/j.fuel.2015.10.011
Salmachi, A., Rajabi, M., Reynolds, P., Yarmohammadtooski, Z., and Wainman, C. (2016). The effect of magmatic intrusions on coalbed methane reservoir characteristics: A case study from the hoskissons coalbed, gunnedah basin, Australia. Int. J. Coal Geol. 165, 278–289. doi:10.1016/j.coal.2016.08.025
Sampath, K. H., Perera, M. S., Ranjith, P. G., Matthai, S., and Dong-yin, L. (2019). Qualitative and quantitative evaluation of the alteration of micro-fracture characteristics of supercritical CO2-interacted coal. J. Supercrit. Fluids 147, 90–101. doi:10.1016/j.supflu.2019.02.014
Sang, S., Liu, H. h., Li, Y. m., Li, M. x., and Li, L. (2009). Geological controls over coal-bed methane well production in southern Qinshui basin. Procedia Earth Planet. Sci. 1 (1), 917–922. doi:10.1016/j.proeps.2009.09.142
Shang, J., Liu, H., Sang, S., et al. (2020). Coupling analysis on permeability and pore fracture development in high rank coal reservoirs of southern Qinshui Basin. Saf. Coal Mines 51 (6), 184–190. doi:10.13347/j.cnki.mkaq.2020.06.040
Shen, W., Shao, L., Tian, W., Chen, G., Chen, F., Hou, H., et al. (2019). Study on geological controls and enrichment models of coalbed methane in the Wuwei Basin in eastern North Qilian, northwestern China. Energy Explor. Exploitation 37 (1), 429–452. doi:10.1177/0144598718803693
Shen, W., and Zhang, B. (2000). Testing study on mechanical parameters of coal. Chin. J. Rock Mech. Eng. 19, 860–862. doi:10.3321/j.issn:1000-6915.2000.z1.008
Shi, J., and Durucan, S. (2004). Drawdown induced changes in permeability of coalbeds:A new interpretation of the reservoir response to primary recovery. Transp. Porous Media 56, 1–16. doi:10.1023/b:tipm.0000018398.19928.5a
Shi, J., and Durucan, S. (2010). Exponential growth in San Juan Basin Fruitland Coalbed permeability with reservoir drawdown:model match and new insights. SPE Reserv. Eval. Eng. 6, 914–925. doi:10.2118/123206-pa
Wu, H., Zhou, Y., Yao, Y., and Wu, K. (2019). Imaged based fractal characterization of micro-fracture structure in coal. Fuel 239, 53–62. doi:10.1016/j.fuel.2018.10.117
Yao, Y., Liu, D., Huang, W., et al. (2006). Research on the pore-fractures system properties of coalbed methane reservoirs and recovery in Huainan and Huaibei coal-fields. J. China Coal Socirty 31 (2), 163–168. doi:10.3321/j.issn:0253-9993.2006.02.007
Yu, K., Qu, Z., Ju, Y., et al. (2018). Burial and thermal history of coal-bearing strata in Shengli coalfield, Erlian basin. Acta Sedimentol. Sin. 36 (5), 903–913. doi:10.14027/j.issn.1000-0550.2018.064
Zhang, Q., Zhang, Q., and Zhang, H. (2004). Adsorption characteristics of different rank coals in different areas China. Coal Geol. Explor. 32, 68–72. doi:10.3969/j.issn.1001-1986.2004.z1.015
Zhang, X., Liu, Y., Sang, S., et al. (2011). Study of the gas diffusion mechanism in high-rank coal reservoirs. J. China Univ. Mining& Technol. 40 (01), 43–48.
Zhang, Y., Lebedev, M., Jing, Y., Yu, H., and Iglauer, S. (2019). In-situ X-ray micro-computed tomography imaging of the microstructural changes in water-bearing medium rank coal by supercritical CO2 flooding. Int. J. Coal Geol. 203, 28–35. doi:10.1016/j.coal.2019.01.002
Zhao, Z., Wang, H., Cui, Z., et al. (2021). Experimental study on mode-I fracture toughness using chevron straight-notched semi-circular bend (CSNSCB) method. Theor. Appl. Fract. Mech. 116, 103093. doi:10.1016/j.tafmec.2021.103093
Zhong, L., Zhang, Q., Xie, G., et al. (2006). MT/T 968-2005 Description methodology of fracture in coal. Beijing: China Coal Industry Publishing House.
Keywords: high-rank coal reservoirs, fracture network, gas seepage, mechanism analysis, reservoir permeability
Citation: Liu Y, Xiong Z and Zhang X (2022) Fracture network characterization of high-rank coal and its control mechanism on reservoir permeability. Front. Earth Sci. 10:995394. doi: 10.3389/feart.2022.995394
Received: 15 July 2022; Accepted: 08 August 2022;
Published: 07 September 2022.
Edited by:
Mingjun Zou, North China University of Water Resources and Electric Power, ChinaReviewed by:
Wang Hongjian, North China University of Water Conservancy and Electric Power, ChinaCopyright © 2022 Liu, Xiong and Zhang. This is an open-access article distributed under the terms of the Creative Commons Attribution License (CC BY). The use, distribution or reproduction in other forums is permitted, provided the original author(s) and the copyright owner(s) are credited and that the original publication in this journal is cited, in accordance with accepted academic practice. No use, distribution or reproduction is permitted which does not comply with these terms.
*Correspondence: Zuqiang Xiong, ZmNjd2FuZ21oMkB6enUuZWR1LmNu
Disclaimer: All claims expressed in this article are solely those of the authors and do not necessarily represent those of their affiliated organizations, or those of the publisher, the editors and the reviewers. Any product that may be evaluated in this article or claim that may be made by its manufacturer is not guaranteed or endorsed by the publisher.
Research integrity at Frontiers
Learn more about the work of our research integrity team to safeguard the quality of each article we publish.