- 1Institute of Geomechanics, Chinese Academy of Geological Sciences, Beijing, China
- 2Key Laboratory of Active Tectonics and Geological Safety, Ministry of Natural Resources, Beijing, China
- 3Research Center of Neotectonism and Crustal Stability, China Geological Survey, Beijing, China
- 4School of Earth Sciences and Resources, China University of Geosciences, Beijing, China
The Linxia Basin is located in the transition zone between the Qinghai–Tibet Plateau and the Loess Plateau in China. Collapse–landslide–debris flow geological disasters are particularly prevalent in this region. Taking a debris flow that occurred in Zhangjiayuan gully, Dongxiang County, as an example, the characteristics and formation mechanism were studied through field investigation and remote sensing interpretation methods. The hazard zoning and influence range of the debris flow were analysed under different precipitation conditions using numerical simulations. The results show that the debris flow in Zhangjiayuan gully experienced medium-to small-scale, rapid and catastrophic viscous mud flows, with activity frequencies ranging from medium to low. Because large numbers of landslides, collapses and overland flows developed on the gully bank slope, material sources for debris flows were abundant but were supplied intermittently. Induced by heavy rainfall, the landslides on the bank slipped into the gully, and then the landslide mass blocked the channel and formed a weir dam. As water converged in the gully, the dam body softened to mud, seepage deformation occurred, and the dam then burst to form a debris flow. This disaster mode represents a kind of rainfall-type landslide–blockage–debris flow disaster chain. Under varying conditions in which heavy rainfall occurs once every 10 years or once every 50 years, debris flows may break out in Zhangjiayuan gully, and the Zheda highway and the associated tunnels located below the gully are in medium–high debris flow danger zones. When a debris flow rushes out of the gully mouth and cannot be discharged in time, it accumulates and buries the highway and tunnels. Therefore, attention should be given to preventing such small-scale sudden debris flow disasters and strengthening the early warning capacity of debris flow prevention to ensure the safe operation of highway.
1 Introduction
Debris flows are one of the geomorphic agents carrying large amounts of solid material. Because of sudden initiation and complicated formation processes, debris flows have strong destructive power to cause casualties (Peng et al., 2004; Zhang et al., 2016). The Linxia Basin in Gansu Province China is located at the transitional region between the Tibetan Plateau and the Loess Plateau, where debris flows are highly developed (Li and Zeng 1982). Debris flows in this region often accompany with catastrophic disasters, as exemplified by some cases on 20 July 1964, 7 July 1965 and 21 July 2013 (Qu et al., 2018). In fact, debris flows in the region mainly depend on the rainfall condition, as the material resources is unlimited and mainly from the loess deposits (Zhang and Tang 1994; Liu et al., 2001). However, detailed information about local debris flow event is few, and it is worth us to explore the characteristics and risk potential in rainstorm with varied recurrence period.
Debris flows in Linxia Basin are commonly small scale and low frequency (Wang, 1995; Ma et al., 2006). Due to the concealment, unclear genetic mechanisms and insufficient hazard estimation, local debris flow has been rarely documented. Though the annual precipitation in Linxia Basin is far less than other regions, occasional rainstorm occur in rainy season (Liu et al., 2001; Qu et al., 2018). In the eastern part of the Tibetan Plateau, local debris flows can be triggered not by rainstorm, but a combination of heavy rainfall and earthquakes (Hu and Zhong, 2002; Wei et al., 2002; Su et al., 2004; Tang et al., 2011a; Liu et al., 2011; Ni et al., 2011; Guo et al., 2014; Xu et al., 2017). With the global climate warming and increasing seismic events, extreme weather in Linxia Basin and its surrounding areas may occur in future. Recently, some extreme events that never occur before have been documented. On 21 July 2013, a heavy rain in Hedong, Gansu Province, poured hourly rainfall up to 82.3 mm. From August 17 to 30, 2017, a continuous rainfall lasted 15 days and covered the hilly area from Lanzhou to Jingyuan. From September to October 2021, the southeast part of Gansu province suffered heavy rainfall and caused tremendous landslides. It can be speculated that extreme events could promote the debris flows possibility because slope failures in Linxia Basin are more prone to occur than other mountain regions in eastern marginal boundary of the Tibetan Plateau.
Quantitative evaluation provides an effective way to reduce disaster losses (Pasutoa and Soldati 2004). Many hazard assessment methods have been proposed, such as the analytic hierarchy process, fuzzy comprehensive evaluation, logistic regression model, deterministic distribution model, and geographic information system (Delmonaco et al., 2003; Huggel et al., 2003; Paudel et al., 2020). Numerical simulations analysis can better simulate the movement process and movement characteristics (Chau and Lo 2004; Song et al., 2018; Monia et al., 2020), and determine the runout and deposition of debris flows (Magirl et al., 2010). Among them, FLO-2D is commonly used to analyse the risk potential of mud flow or flood (O’Brien et al., 2009; Bertolo and Wieczorek 2005; Zhang et al., 2014; Liang et al., 2016). Such method can provide the potential inundation areas under given rainstorm with varied recurrence period, which is helpful for risk zoning according to the predicted flow depth, maximum velocity and flow mass (Hungr, 1995; Erena et al., 2018; Chang et al., 2019a; Liu et al., 2020; Wu et al., 2022). In Linxia Basin where debris flows are rarely documented, it is important to delineate the potential inundation areas considering the rainstorm recurrence period. Furthermore, detailed description of debris flows there are also important for risk assessment and hazard mitigation.
The early identification and genetic mechanism of debris flows in the Linxia basin are few, and potential risk evaluation is also rarely reported. On 24 April 2021, a severe mud flow event occurred in the Zhangjiayuan watershed, Dongxiang County, Linxia Hui Autonomous Prefecture, Gansu Province, China. This case provided a valuable chance to know about the characteristics and formation condition of mud flow. In this work, the rainfall triggering condition, material sources and deposits were clearly documented according to the timely investigation by the research team of Institute of Geomechanics, Chinese Academy of Geological Sciences. Then, the potential hazard of mud flows at two rainfall probabilities were numerically assessed by FLO-2D and the hazard zoning were divided by the flow depth and momentum. The results will contribute to comprehend the formation mechanics of mud flow in Loess Plateau, China, and its nearby surroundings, which also provide a reference for hazard mitigation and forecasting for debris flows.
2 Environmental and geological settings
The study area is located in north-western part of Dongxiang County, Linxia Hui Autonomous Prefecture, Gansu Province. As the study area is on the transitional region between the Tibet- and Loess-Plateau (Figure 1A), the tableland area is heavily incised. Tectonically, the Linxia Basin is between the Qilian Mountain and the Qinling orogenic belt (Figure 1B). Upper Pleistocene Malan loess mainly exposed in the study area. In Zhangjiayuan watershed, Neogene rocks expose at the gully bottom and at the valley bottom, and the brick-red-sandy mudstone is nearly horizontal. The Malan loess, which is more than 50 m thick, drapes on the Neogene deposits. The Zhangjiayuan watershed is a fan-shaped watershed located on the tableland of Yellow River. The area of the watershed is 1.36 km2, and the mean channel gradient is 130‰. The main channel, which has a total length of 1.78 km, drains into the Liujiaxia Reservoir. Within the Zhangjiayuan watershed, the highest elevation is 2020 m, and the relative relief of watershed is 251 m (Figure 1C). The watershed outlet is approximately 30 m over the Liujiaxia Reservoir. In the transport section, the channel is 1.21 km long and is incised to V-shape.
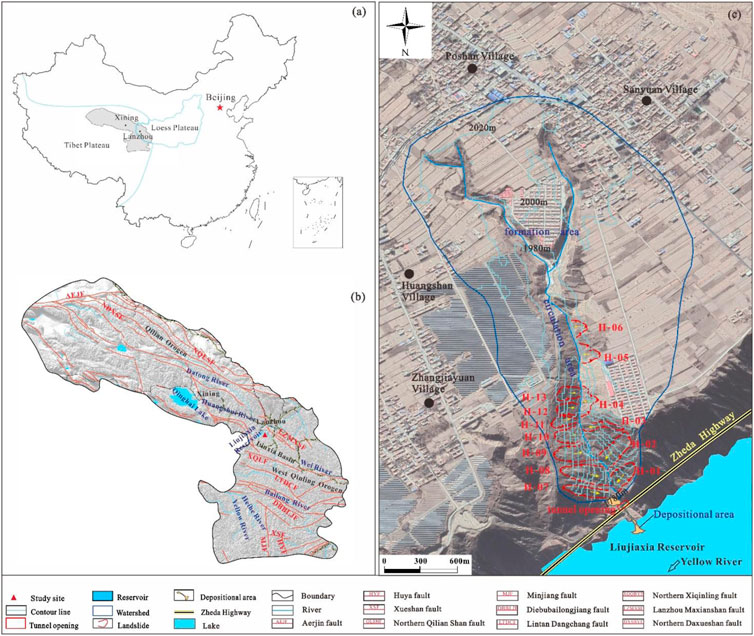
FIGURE 1. Simplified topography and geological setting of the Zhangjiayuan watershed (A) location of the study area in the transitional regions of Tibet Plateau and Loess Plateau, (B) geological setting of the study area and (C) simplified topography of the study area.
This area is located in temperate semiarid climate zone with most rain fallen between July and September. The annual precipitation ranges from 336.5 mm (in 1980) to 707.5 mm (in 2007). According to the meteorological records from 1986 to 2005, the annual mean temperature was 5.6°C and annual mean precipitation was 530 mm. However, the average annual temperature and monthly average temperature of Zhangjiayuan watershed were slightly higher than those of the whole county. Within the study area, the rainy years have a general recurrence pattern of 5–6 years, and the dry years have a recurrence of 8 to 10 years (Figure 2). The maximum daily precipitation was high up to 62.7 mm, and the maximum hourly rainfall was 45.0 mm. In the past 50 years, there were 5 events with daily precipitation >50 mm, and approximately one heavy rainfall event (>50 mm) occurred in 10 years interval.
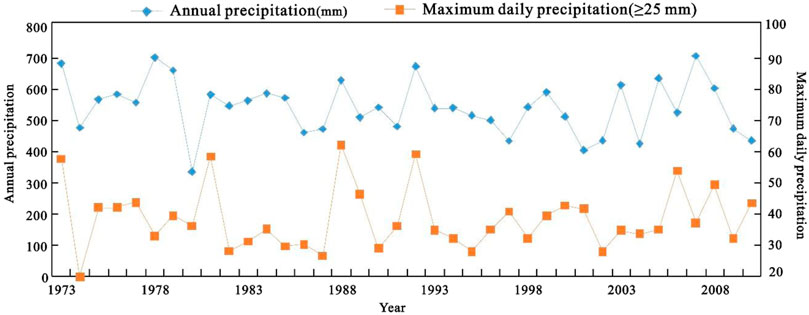
FIGURE 2. Climatic conditions of the study area according to the meteorological station at Dongxiang County.
3 Data and methods
3.1 Data collection
In this work, field investigation and remote sensing image interpretation combine to examine the material resources. Laboratory tests, inspecting the mudflow properties, provide the physical parameters to numerical simulation work of delineating potential inundation areas. The morphological and geometric parameters of the gully, the landslides developed on the bank, and the flow marks of debris flow were measured by hand-held laser rangefinder during field investigation. The distribution of landslides and depositional area is finely interpreted by remote sensing images (Liu et al., 2022b; Qiu et al., 2022). The physical parameters of the debris flow deposits are analysed in laboratory tests.
Debris flow samplings were taken at the depositional area of Zhangjiayuan watershed. During investigation, road departments and debris flow control personnel were interviewed to determine the flow pattern of debris flows. The samples were adjusted to the fluid state at the time of the debris flow outbreak, and the bulk density was calculated after weighing. The consistency limit of the deposits was measured by a liquid–plastic combine tester in the laboratory. Grains >0.05 mm were sorted by sieve analysis, and grains <0.05 mm were treated by hydrometer analysis.
3.2 Setting the simulation conditions and characterization parameters of the debris flow
According to meteorological records from the China National Meteorological Administration and local meteorological bureaus, the maximum daily precipitation of the study area was 62.7 mm on 18 August 1970. During 2011 and 2021, the maximum daily rainfall reached 55.7 mm. Therefore, the maximum 50-year precipitation (probability p =2%) was set to 62.7 mm and the maximum 10-year precipitation (probability p =10%) was set to 55.7 mm.
The parameters of the debris flow calculation were set based on field investigations and lab tests, by referring to existing research results and the FLO-2D user manual. Referencing the FLO-2D manual and previous researches (Zhang et al., 2014; Hou et al., 2021), the laminar flow arrest coefficient (K) was set to 2280 as the debris flows in Zhangjiayuan watershed are typically mud flows. The viscosity coefficient (η) and yield stress (τy) mainly depend on the volume concentration of sediments, which is solved by obtaining the correlation between
Due to the reactivation of the H-08 landslide, the sliding body blocked the channel and formed a barrier dam. The upstream side of the landslide dam was determined as the water catchment point (Figure 1C and Figure 3A). According to the clear water flow line and amplification coefficient
4 Results
4.1 Description of the debris flow event
The debris flow in Zhangjiayuan watershed occurred in the early morning of 24 April 2021, following the rainstorm started in the night of 23 April 2021. There were no meteorological stations near the study area, as it is about 33 km away from the urban area of Linxia County. However, the local inhabitants near the study area told that April this year was sufficient in precipitation, whereas it was not so previously. Owing to increasing extreme weather events in recent years, the rainy seasons occur earlier or later, and heavy rainfall may occur in April or even October subsequent years. In the study area, the rainy season of 2021 started at the end of March (Figure 4). Four heavy precipitation events occurred till the end of April, including the events from March 31 to April 4, April 7 to April 12, April 18 to April 20 and April 23 to April 25. The rainfall totals were 15.4 mm on April 2 and 11.5 mm on April 23, indicating that the rainfall process was long duration.
According to the field investigation and the information from Transport Department of Gansu Province, the debris flow volume was approximately 9, 000 m3, with approximately 2, 000 m3 in tunnel and 7, 000 m3 in channel. Majority of sediments deposited at the tunnel mouth and on the Zheda highway, thus caused damage to the west entrance of Tunnel No. 408 and to the east entrance of Tunnel No. 409. The length of the depositional area in Tunnel No. 408 was 146 m, and the highest mud level was 3.5 m (Figure 5A); however, this length was 25 m in Tunnel No. 409, with a mud level of 3.3 m. Importantly, the debris flow travelled across the road and delivered sediments on the right bank of the Liujiaxia Reservoir (Figure 5B). The depositional fan was 90 m in length, 58 m in width, 1–3 m in thickness, with diffusion angle 120° and magnitude of 2, 500 m3.
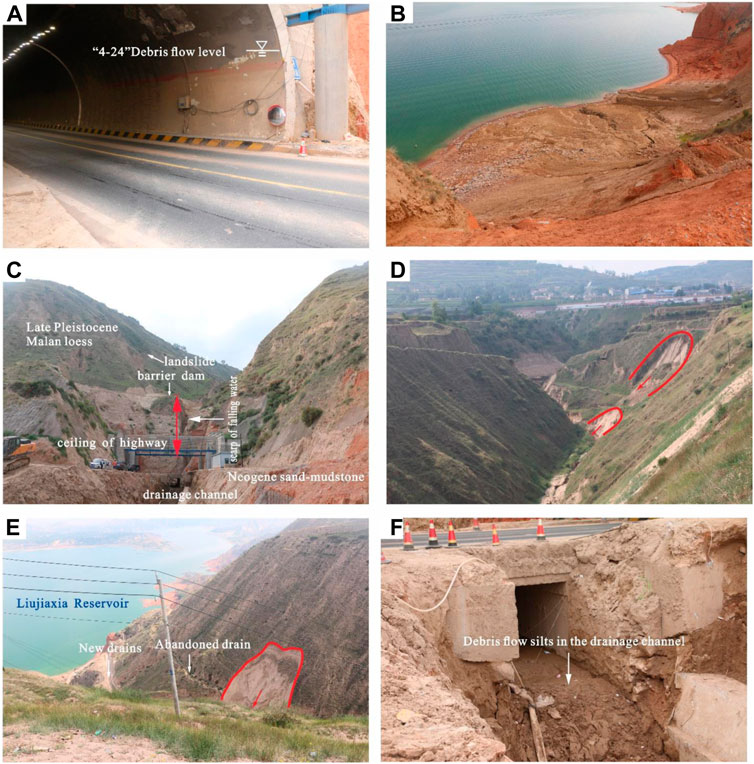
FIGURE 5. Debris flow characteristics and treatment engineering measures applied in Zhangjiayuan gully (A) Mud level at the west entrance of Tunnel 408 lens: NE; General view of the circulation area and debris flow outlet: SEE; (B) Debris flow depositional area lens: NE; (C). Landslides that occurred on the left bank of the gully upstream (H-06) lens: S; (D) Landslide on the right bank in the transportation area lens: NE; (E) Culvert under the highway lens: SE; (F) Retaining wall upstream of the culvert lens: SE.
In accordance with particle component analysis and physical property measurement, the debris flow density is 18.0 kN/m3. The liquid and plastic limit are 25.7% and 18.4%, respectively. The debris flow deposits are mainly composed of fine sand and silt–clay particles (Table 1), and the fine sand accounts for 16.4%, silt 69.0% and clay 14.3%. This indicates that the material sources are mainly from Epipleistocene Malan loess on the gully bank. Therefore, debris flows in Zhangjiayuan watershed belongs to viscous-mud flow.

TABLE 1. Results of the grain analysis performed on samples obtained from the Zhangjiayuan gully debris flow.
4.2 Material sources
The primary material sources of the debris flow are mainly from slope-surface erosion and loess mass movements. As the slope top is relatively flat, the amount of slope erosion is far less than Collapse-landslide mass. On basis of landslide detailed interpretation (Liu et al., 2022a and, Liu et al., 2022b) and field investigation, small collapses, landslides and overland flows mainly developed on the left and right banks of the middle and lower reaches (Figures 5C,D,E), especially within the 500 m channel downstream of the gully mouth. A group of landslides developed (Figure 1 and Table 2) on the right bank of the transportation area in the middle–lower reaches, which consisted of 7 landslides with volumes of 0.4–2.3 ×104 m3. Six small landslides occurred on the left bank, with volumes of 0.3–1.6×104 m3. These 13 landslides were all small-scale and were mainly composed of Pleistocene loess and locally sourced Neogene sands and mudstones. The bed underneath comprise of Pleistocene loess, and Neogene sandstone–mudstone slide beds are exposed near the gully mouth (Figure 5A).
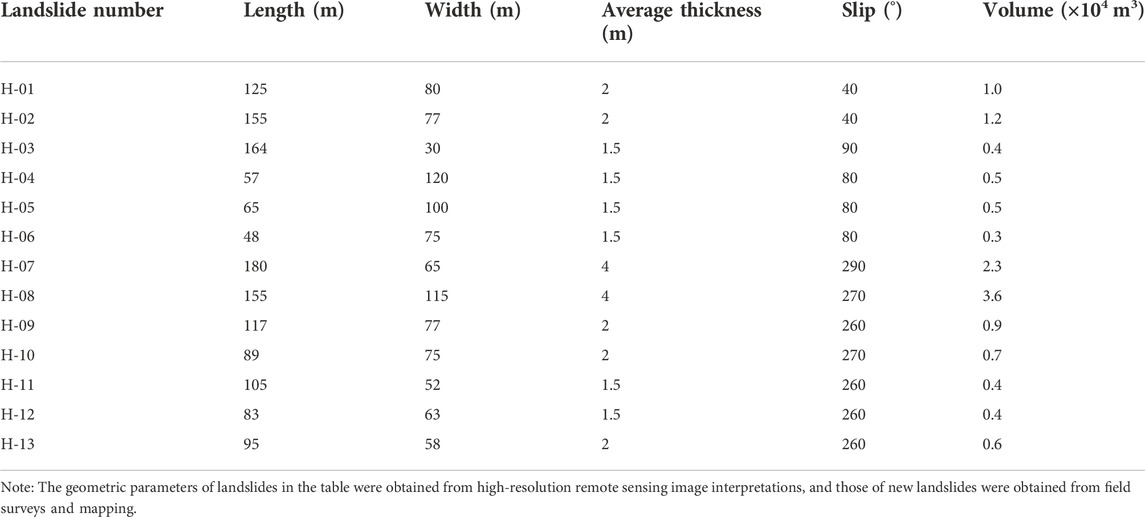
TABLE 2. Statistics of the geometric characteristic parameters of landslides on the Zhangjiayuan gully bank.
On 23 April 2021, a new landslide No.H-06 (Figure 5D) occurred on the left bank. It has a length 48 m, width 75 m, thicknesses 1–2 m and volume about 3000 m3. On the right bank, two new landslides occurred. The first one developed from the lower part of old landslide No.H-08 (Figure 5E), with length 85 m, width 65 m, thickness 1–2 m, and volume 5000 m3. The Landslide No. H-13 was 95 m in length, 58 m in width, 2 m in thickness and approximately 6000 m3 in volume. Based on in situ investigations (Table 2), the total and dynamic material storage were calculated, including sediments from landslide (V1) and slope erosion (V2). The total landslide recharge amount can be obtained from the accumulative volume of each landslide, i.e., 1.26 × 105 m3. On the basis of the watershed distribution area (
Referring to appendix I of the Chinese Geological and Mineral Industry standard (DZ/T 0220–2006 Debris flow disaster prevention engineering investigation code) and Appendix F.1 of the geological disaster hazard assessment “Procedure of Gansu Province local standard DB62/T-2009” (in Chinese), the rainfall-flood method was adopted herein to calculate the maximum debris flow outflow amount at a given time:
where
Based on the data of the Chinese National Meteorological Administration, the maximum daily precipitation in the Linxia from June 2011 to June 2021 was 55.7 mm (15 August 2016). According to the 10 years recurrence rain event, the maximum debris flow discharge in Zhangjiayuan gully was calculated to be 61.2 m3/s, and the maximum outflow of a debris flow could reach 1.53 × 104 m3. The above information reveals that the probability of daily precipitation greater than 50 mm is approximately once every 10 years for the rainy- and dry water-year periods in Dongxiang County. Thus, the one-time maximum outburst amount calculated using the 10-year probability occurrence can be considered the dynamic source reserve of Zhangjiayuan gully debris flows.
The field investigation and material interpretation show that the material sources of debris flow in Zhangjiyuan watershed are mainly from the landslides and collapses along gully bank, and the overland flow erosion on the hilly slope. As known to all, the occurrence of mass movements in Loess Plateau, China, closely relates to the annual rainy season precipitation and rainfall duration (Palladino et al., 2018; Ma et al., 2021; Zhou et al., 2022). The material supply from collapse–landslide–overland flow mass is more in rainy years and less in dry years, which leads to the great difference in the size of the provenance dynamic storage provided each year. Therefore, the material source of debris flow in the gully is abundant, but has the characteristics of intermittent supply.
4.3 Hazard simulation results of the analysed debris flow
Figure 6 and Table 4 show the simulation results of the debris flow movement process in Zhangjiayuan gully for precipitation conditions with different probabilities (p =10% and p =2%). Except for the hazard index, the classification of the other indexes was automatically generated by the calculation results.
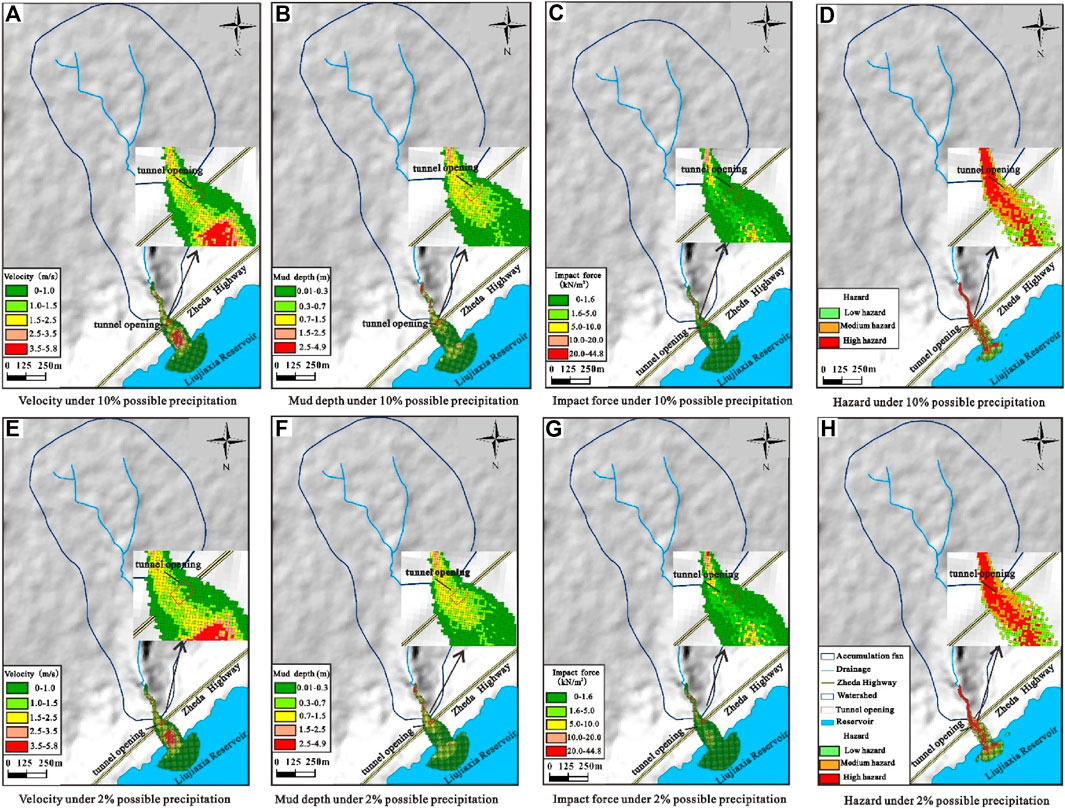
FIGURE 6. Simulation results of debris flow movement characteristics and danger ranges based on FLO-2D under two precipitation conditions.
With reference to the research of Tang et al. (1994), Li et al. (2021), Hou et al. (2021), and the Swiss Disaster Degree Classification (Garcia et al., 2004), the hazard zoning of Zhangjiayuan gully debris flows was carried out on the basis of the velocity (Vc), mud depth (Hc), and other indexes. The area within the hazard range was divided into high-, medium-, and low-hazard zones (Table 3).
According to Figures 6A–D and Table 4, the maximum flow velocity of a debris flow could reach 5.8 m/s in the case of heavy rainfall over 10 years. The zone with flow velocities greater than 3.5 m/s was distributed in the middle of the accumulation body, covering an area of 3750 m2, and the zone with flow velocities less than 1 m/s covered an area of 45,975 m2. The maximum mud depth of the debris flow was 4.8 m, and the area with mud depths greater than 2.5 m was located at the bottom of the circulation area with an area of 800 m2. The maximum impact force of the debris flow was 44.8 kN/m2, and an impact force greater than 20 kN/m2 acted on the bottom of the circulation area; the area with impact forces greater than 10 kN/m2 was only 1675 m2. The high-hazard debris flow area was located from the starting site of the debris flows to the inside channel of the Liujiaxia Reservoir bank, covering an area of 10825 m2. The middle hazard area was located in the middle of the inlet of the Liujiaxia Reservoir and the gully mouth to the northern Zheda highway. The low-hazard area was located outside the accumulation body of the north Zheda highway.
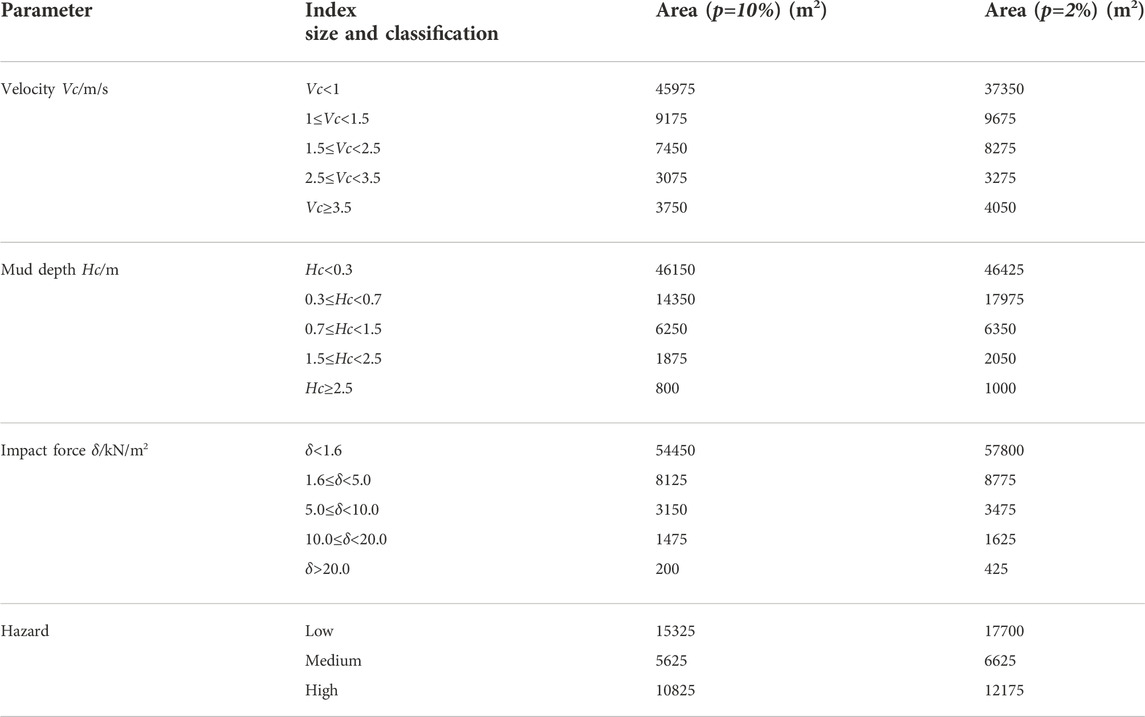
TABLE 4. Simulation and hazard classification results of debris flows in Zhangjiayuan gully under two precipitation conditions.
In the case of a 50-year heavy rainfall event (Figures 6E–H and Table 4), the maximum flow velocity of the debris flow was still 5.8 m/s, and the area of flow velocities greater than 3.5 m/s was 4050 m2. The maximum mud depth of the debris flow was 4.9 m, and the area with mud depths greater than 2.5 m was 1000 m2. The maximum impact force of the debris flow was 50.6 kN/m2, and the area with impact forces greater than 10.0 kN/m2 was only 2050 m2. The high-hazard area of the debris flow was distributed inside the channel from the starting site of the debris flow to the entrance of the Liujiaxia Reservoir, with a distribution area of 12,175 m2, while the medium–low hazard area was basically the same as that obtained under the once-in-a-decade condition.
The simulation results show that debris flows erupt in Zhangjiayuan gully when it encounters heavy rainfall once every 10 years or extreme rainfall once every 50 years. Controlled by the gully morphology and debris flow properties in the circulation area, the debris flows in Zhangjiayuan gully reach their maximum velocity in the middle of the accumulation area, and the strongest impact force of the debris flows is applied to the area from the downstream region of the circulation area to the lower part of the gully mouth. When a debris flow rushes out of the gully, the debris flow begins to accumulate. In the case of a 10-year rainfall event, the mud silting height of the road and tunnel mouth is 1.27 m, the silting width of the east tunnel is 49 m, and that of the west tunnel is 21 m. In the case of a 50-year rainfall event, the maximum silting depth in the tunnel is 1.41 m, the silting width of the east tunnel is 53 m, and that of the west tunnel is 25 m. Under these two precipitation conditions, the tunnels are in the medium–high hazard zone of debris flows, and the silting hazard in the east tunnel is much higher than that in the west. If heavy rainfall occurs once in one hundred years, a large-scale debris flow would occur in the small watershed of Zhangjiayuan gully and may silt and scour the road and tunnel or even destroy the road or tunnel opening.
Based on the above results, three types of hazard-affected objects can be identified: tunnel; pavement and subgrade; and culverts (Figure 5F). Therefore, the susceptibility of the debris flow should be discussed. The susceptibility (C) indicates the resistance degree of the hazard-affected object against debris flow damage. Values for this variable ranges between 0 and 1, where larger susceptibility values indicate a more vulnerable hazard-affected object (Cui and Zou, 2016). According to the studies of Cui et al. (2016) and Li et al. (2021), the C value is mainly affected by two factors: structure susceptibility (C1) and siltation susceptibility (C2). Because the Zhangjiayuan debris flow is mud, the C1 value is too small to be ignored. C2 is calculated by:
where Hc is the mud depth and h is the clearance of the culvert or tunnel. If
The geometric information of the culvert or tunnel was obtained during field investigation. The length × width × height dimensions of the culvert are 10 × 3 × 3 m, and the tunnel section is a semicircular arch with a size of 9 × 10 m.
The hazard zoning results of the Zhangjiayuan debris flow show that for 10-year and 50-year rainfall events, the mud depths of the road and tunnel under the gully mouth are 1.27 m and 1.41 m, respectively, and both the highway and tunnel are in the high hazard zone (Figures 6D,H). However, due to the large bulk density of the debris flow and the slow discharge of the culvert, the tunnel and culvert were buried on 24 April 2021, and the mud depths of the west opening of Tunnel 408 and the east opening of Tunnel 409 were 3.5 m and 3.3 m, respectively. In terms of Eq. 4,
5 Discussion
5.1 Differences in debris flows between the Linxia Basin and the eastern Tibetan Plateau
Differentiating from the viscous debris flows in the eastern Tibetan Plateau, the debris flows in the Linxia Basin are mostly viscous mud flows, as the source materials are derived from the Quaternary loess. Due to the dry climate and uneven rainfall, debris flows generally occur at low frequencies and they are currently in a declining period. The solid sources of debris flows are abundant, and mainly provided by gully bottom erosion and lateral erosion. Most debris flows occur at medium-to high-frequencies and have medium–large magnitudes. Debris flows in the area are triggered by heavy rainfall (Liu et al., 2011; Tie et al., 2013; Chang et al., 2019b) or by both strong earthquakes and heavy rainfall (Cui et al., 2010; Tang et al., 2011b; Ni et al., 2011; Xu et al., 2017). The rainfall thresholds that induce debris flows are generally 25 mm/h∼60 mm/h. In the eastern part of the Qinghai–Tibet Plateau, the types of debris flows are mainly gullies or valleys, with bulk densities of 1.3–2.3 kN/m3. Due to large precipitation in the area and the uneven distribution of rainfall in various watersheds, heavy or extreme rainfall frequently occurs, and debris flows mainly occur at medium-to high-frequency, similar to the currently in flourishing or developing period. Multiple disaster modes, including ravine rainstorm debris flows, collapse–landslide–debris flow disaster chains, and even high-position collapse landslide debris flows have been observed.
5.2 Genetic mechanism of the analysed debris flow
The debris flows in Zhangjiayuan gully mainly form and develop as follows.
Thirteen landslides were identified on the middle and lower banks of the circulation area (Figure 1; Table 2), with channel blockage and poor drainage conditions. These landslides are mainly loess landslides, but some of the landslides on the left bank of the gully cut into the moderately to intensely weathered Neogene sandstone–mudstone zone (Figure 4A). The landslides of the right bank mainly slip along the unloading fissure plane and are mostly shallow landslides. Most of these landslides occurred before 2021. The H-01, H-07 and H-08 landslides, which developed on both banks of the gully mouth, rushed into the gully. Small portions of these landslide masses eroded away by subsequent water flows, but most of the residual parts still accumulated in the gully, resulting in water crossing the section of the gully (Figure 4A) in the narrow outlet of the debris flow; the gully discharge was not smooth.
Due to continuous long-term rainfall, the water content of rock‒soil in the basin increases, and the bank surface can be eroded to form overland flows. Alternatively, the reduction in slope stability may lead to the occurrence of landslides and collapses (Yang et al., 2022), such as the new H-06 landslide on the left bank, the revival of the H-08 and H-12 landslides on the right bank, and the landslide body blocking the gully to form a barrier dam after sliding into the gully (Wang et al., 2022). When this region subsequently encountered heavy rainfall, the water gathered and formed a barrier lake.
After rainwater converges, the deposits and landslide rock‒soil mass accumulated in the channel soften and undergo argillization, resulting in dam bursting and the formation of a debris flow induced by short-term heavy rainfall. From the end of March to the end of April 2021, four heavy rainfall events (Figure 5) occurred in the region of Dongxiang, Yongjing and Linxia Counties, including two daily rainfall events exceeding 10 mm. This heavy rainfall led to the rapid increase in the water content and weight of the accumulated rock‒soil mass. When the water content of the deposit exceeded the liquid limit, the soil mass was in the critical plastic flow state, implying that the slurry-making process had been completed. Another rainstorm occurred on the evening of April 23. The water promptly gathered in front of the dam, the water level surged, and the hydrostatic pressure and earth pressure increased (Wu et al., 2013). As the rainwater flowed downstream, the dynamic water pressure also increased. Under the action of the high-water level in the upstream dam, as well as the permeation pressure and fluid self-gravity associated with the debris flow, the shear fractures in the front edge of the landslide accumulation zone were internally eroded, extended and finally connected, and fissure fillings were quickly washed away to form a water inrush channel. When the vertical penetration was more than the effective weight of the soil mass of the dam, parts of the barrier dam experienced soil flow deformation, resulting in the bursting of the dam and the initiation of a debris flow (Zhou et al., 2022). It is worth pointing out that the long-term rainfall ensured sufficient time for rainwater to infiltrate into the rock‒soil mass; this was the premise for softening and argillation of the channel accumulation and collapse-landslide bodies. However, the top of the gully is a plateau tableland, the amount of surface runoff converging into the channel is relatively small, and the fluid of the debris flow was not washed away promptly in the gestation process.
The bulk densities of the debris flows in Zhangjiayuan gully are high. After a debris flow starts, the fluid scours and erodes the channel and slope foot, resulting in the collapse of the bank foot and the lower part of old landslides, thereby increasing the severity and viscosity of the debris flow and enhancing the destructive power of fluid shear.
The circulation area downstream of Zhangjiayuan gully has been blocked by the loose solid materials of landslide bodies for a long time, and the discharge mouth is narrow, thus constituting a bottleneck of debris flow movement. A scarp of falling water is present between the outlet and the road surface below, with a height difference of 14.0 m. The initiation process of a debris flow is similar to the karst water and mud inrush phenomenon that occurs during tunnel excavation (Li et al., 2017), consisting of three parts: the disaster source, debris flow channel and barrier dam. The disaster source is the channel accumulation of the upper dam, the collapse and sliding accumulation body, and the converging water body. The debris flow channels are equivalent to the karst water inrush channels, that is, the migration path of the debris flows. The barrier dam is the eventual barrier that blocks water before the debris flow starts. When the analysed debris flow rushed out of the gully to the Zheda highway, the fluid could not be discharged in a timely manner to the Liujiaxia Reservoir due to the small cross-section (bottleneck) of the culvert under the highway, and the debris flow instead rushed into the tunnels on both sides of the highway until the road was silted and blocked.
6 Conclusion
The goal of this study was to analyse hazards and genetic mechanisms of a debris flow in a small watershed, which was induced by rainfall in the transition zone between the Qinghai-Tibet Plateau and the Loess Plateau. The study area is of loess ridge landform, with vertical and horizontal gullies. The bank slope of the gully is prone to collapse and landslide, and the debris flow sources are abundant. When encountering heavy rainfall or continuous rainfall for a long time, debris flows are prone to outburst in this type of small watershed. As the material sources were mainly loess and the underlying Neogene mudstone weathering material, which were intermittently supplied by previous collapses and landslides on the bank; thus, this kind of debris flow had the characteristics of a the medium-small scale, a medium-low activity frequency, and a viscous mud flow.
The four heavy rainfall events from the end of March to the end of April 2021, and the rainstorm from April 23 to the early morning of April 24 led to the outbreak of the debris flow in Zhangjiayuan gully. This event occurred because the bank slope collapse-landslide-overland flow sequence was induced by long-term rainfall and multiple heavy rainfall processes. The disaster bodies blocked the channel and formed a barrier dam. The water flow converged in front of the dam. The infiltration of water caused the channel deposits to soften and muddy, and led to the seepage deformation of the dam body prior to the debris flow outburst. The disaster formation mode was the rainfall-type landslide-blockage-debris flow disaster chain mechanism.
Under the condition of heavy rainfall once every 10 years or once every 50 years, debris flows will occur in Zhangjiayuan gully. After a debris flow starts, the maximum flow velocity is reached in the middle of the accumulation area, and the strongest impact forces are applied to the area from the downstream region of the circulation area to the lower part of the gully mouth. The Zhida highway and tunnel under the mouth of the gully are in the medium–high hazard zone of debris flow. If a debris flow rushing out of the gully mouth cannot be discharged in time, it will begin to accumulate and bury the highway and tunnel. Although the scale of the debris flow in this small watershed is not large, the debris flows in this region have strong concealment and sudden outbreak characteristics, which may cause a substantial catastrophe. Attention should be given to preventing such ‘small disaster, big catastrophe’ events to ensure the safe operation of highways and tunnels in a timely manner.
Data availability statement
The original contributions presented in the study are included in the article/Supplementary Material, further inquiries can be directed to the corresponding author.
Author contributions
WY, writing; FW, data analysis; SM, data analysis; JQ, programming; CZ, guidance, and HT programming.
Funding
This research is supported by the Second Tibetan Plateau Scientific Expedition and Research Program (2019QZKK0902).
Acknowledgments
The authors would like to thank the editor and two anonymous reviewers for their very helpful comments, which gave us the opportunity to address several issues that were initially overlooked.
Conflict of interest
The authors declare that the research was conducted in the absence of any commercial or financial relationships that could be construed as a potential conflict of interest.
Publisher’s note
All claims expressed in this article are solely those of the authors and do not necessarily represent those of their affiliated organizations, or those of the publisher, the editors and the reviewers. Any product that may be evaluated in this article, or claim that may be made by its manufacturer, is not guaranteed or endorsed by the publisher.
References
Bertolo, L. P., and Wieczorek, G. F. (2005). Calibration of numerical models for small debris flows in Yosemite Valley, California, USA. Nat. Hazards Earth Syst. Sci. 5 (6), 993–1001.
Chang, M., Dou, X. Y., and Tang, C. (2019a). Hazard assessment of typical debris flow induced by rainfall intensity. Earth Sci. 44 (8), 2794–2802. doi:10.3799/dqkx.2017.547
Chang, M., Liu, Y., Zhou, C., and Che, H. (2019b). Hazard assessment of a catastrophic mine waste debris flow of Hou Gully, Shimian, China. Eng. Geol. 275, 105733. doi:10.1016/j.enggeo.2020.105733
Chau, K. T., and Lo, K. H. (2004). Hazard assessment of debris flows for Leung King Estate of Hong Kong by incorporating GIS with numerical simulations. Nat. Hazards Earth Syst. Sci. 4 (4), 103–116. doi:10.5194/nhess-4-103-2004
Cui, P., Zhuang, J. Q., and Chen, X. C. (2010). Characteristics and countermeasures of debris flow in Wenchuan area after the earthquake. J. Sichuan Univ. Sci. Ed. 42 (5), 10–19. doi:10.15961/j.jsuese.2010.05.004
Cui, P., Zou, Q., Xiang, L. Z., and Zeng, C. (2016). Risk assessment of simultaneous debris flows in mountain townships. Prog. Phys. Geogr. 37 (4), 516–542. doi:10.1177/0309.1333.1349.1445
Cui, P., and Zou, Q. (2016). Theory and method of risk assessment and risk management of debris flows and flash floods. Prog. Geogr. 35 (2), 137–147. doi:10.18306/dlkxjz.2016.02.001
Delmonaco, G., Leoni, G., Margottini, C., Puglisi, C., and Spizzichino, D. (2003). Large scale debris-flow hazard assessment: A geotechnical approach and gis modelling. Nat. Hazards Earth Syst. Sci. 3 (3), 443–455. doi:10.5194/nhess-3-443-2003
Erena, S. H., Worku, H., and De Paola, F. (2018). Flood hazard mapping using FLO-2D and local management strategies of Dire Dawa city, Ethiopia[J]. J. Hydrology Regional Stud. 19, 224–239. doi:10.1016/j.ejrh.2018.09.005
Garcia, R., Rodriguez, J. J., and O'Brien, J. S. (2004). “Hazard zone delineation for urbanized alluvial fans,” in Proceedings of the ASCE World Water and Environmental Resources Congress - Arid Lands Symposium, Salt Lake City, Utah.
Guo, F. Y., Meng, X. M., and Yin, N. W. (2014). formation mechanism and risk assessment of debris flow of “5.10” in eryang gully of minxian county, Gansu province. J. Lanzhou Univ. Nat. Sci. 50 (5), 628–632. doi:10.13885/j.issn.0455-2059.2014.05.008
Hou, S. S., Cao, P., and Liang, C. (2021). Debris flow hazard assessment of the Eryang River watershed based on numerical simulation. Hydrogeology Eng. Geol. 48 (2), 143–151. doi:10.1088/1755-1315/861/6/062002
Hu, X. W., and Zhong, P. L. (2002). Debris flow gully distribution and its nonlinear property of evolution in Jiangjiagou area, Yunnan province. Resour. Environ. Yangtze Basin 11 (1), 94–97. doi:10.3969/j.issn.1004-8227.2002.01.021
Huggel, C., Kaab, A., Haeberli, W., and Krummenacher, B. (2003). Regional-scale GIS-models for assessment of hazards from glacier lake outbursts: Evaluation and application in the Swiss alps. Nat. Hazards Earth Syst. Sci. 3 (3), 647–662.
Hungr, O. (1995). A model for the runout analysis of rapid flow slides, debris flows, and avalanches. Can. Geotech. J. 32 (4), 610–623.
Li, H. L., and Zeng, S. W. (1982). Lanzhou institute of glaciology and cryopedology, academia sinica, Traffic science institute of Gansu province, China, Debris flow in Gansu province. Beijing: Publishing House of People’s Transportation.
Li, S. C., Wang, K., and Li, L. P. (2017). Mechanical mechanism and development trend of water-inrush disasters in karst tunnels. Chin. J. Theor. Appl. Mech. 49 (1), 22–30. doi:10.6052/0459-1879-16-345
Li, Y., Su, L. J., Zou, Q., and Wei, X. l. (2021). Risk assessment of glacial debris flow on alpine highway under climate change: A case study of aierkuran gully along karakoram highway. J. Mt. Sci. 18 (6), 1458–1475. doi:10.1007/s11629-021-6689-3
Liang, H. X., Shang, M., and Xu, X. (2016). Research on the influence factors of flow and deposition of debris flow based on the FLO-2D simulation. J. Eng. Geol. 24 (2), 228–234. doi:10.13544/j.cnki.jeg.2016.02.008
Liu, C. Z., Miao, T. B., and Chen, H. Q., (2011). Basic feature and origin of the “8.8” mountain torrent-debris flow disaster happened in Zhouqu County, Gansu, China. Geol. Bull. China 30 (1), 141–150. doi:10.3969/j.issn.1671-2552.2011.01.015
Liu, J. J., Ma, C., and Li, C. Y. (2020). Fundamental problems and prospects in the study of deposition dynamics of viscous debris flow in the gully-river junction. J. Geomechanics 26 (4), 544–555. doi:10.12090/j.issn.1006-6616.2020.26.04.047
Liu, Y. L., Guan, H. P., and Li, J. L. (2001). Time-and-Space distribution and prevention of debris flow in Lanzhou district. J. Lanzhou Railw. Univ. Nat. Sci. 20 (1), 30–35. doi:10.3969/j.issn.1001-4373.2001.01.005
Liu, Y., Qiu, H. J., Yang, D. D., Liu, Z., Ma, S., Pei, Y., et al. (2022a). Deformation responses of landslides to seasonal rainfall based on InSAR and wavelet analysis. Landslides 19, 199–210.
Liu, Z. J., Qiu, H. J., Zhu, Y. R., Liu, Y., Yang, D., Ma, S., et al. (2022b). Efficient identification and monitoring of landslides by time-series InSAR combining single- and multi-look phases. Remote Sens. 14, 1026.
Ma, D. T., Cui, P., and Zhang, J. S., (2006). Mudflow hazards and prevention countermeasures on the loess plateau. Res. soil water conservation 13 (4), 19–21. doi:10.3969/j.issn.1005-3409.2006.04.007
Ma, S. Y., Qiu, H. J., Hu, S., Yang, D. D., and Liu, Z. (2021). Characteristics and geomorphology change detection analysis of the Jiangdingya landslide on July 12, 2018, China. Landslides 18, 383–396.
Magirl, C. S., Griffiths, P. G., and Webb, R. H. (2010). Analyzing debris flows with the statistically calibrated empirical model LAHARZ in southeastern Arizona, USA. Geomorphology 119 (1/2), 111–124.
Monia, C., Valeria, M., Vania, M., Sciarra, N., and Miccadei, E. (2020). Rockfall and Debris Flow Hazard Assessment in the SW Escarpment of Montagna del Morrone Ridge (Abruzzo, Central Italy). Water 12, 1206. doi:10.3390/w12041206
Ni, H. Y., Zheng, W. M., and Tang, Y. Q., (2011). Formation, characteristics and trend of the group debris flows occurred on August 13 in Qingping, Mianzhu County. Hydrogeology Eng. Geol. 38 (3), 133–138. doi:10.16030/j.cnki.issn.1000-3665.2011.03.006
O’Brien, J. S., Julien, P., and Fullerton, W. (1993). Two-dimensional water flood and mudflow simulation. J. Hydraul. Eng. 119 (2), 244–261.
Palladino, M. R., Vieroc, A., Turconi, L., Brunetti, M., Peruccacci, S., Melillo, M., et al. (2018). Rainfall thresholds for the activation of shallow landslides in the Italian alps: The role of environmental conditioning factors. Geomorphology 303 (1), 53–67. doi:10.1016/j.geomorph.2017.11.009
Pasutoa, A., and Soldati, M. (2004). An integrated approach for hazard assessment and mitigation of debris flows in the Italian Dolomites. Geomorphology 61 (1), 59–70. doi:10.1016/j.geomorph.2003.11.006
Paudel, B., Fall, M., and Daneshfar, B. (2020). GIS based assessment of debris flow hazards in Kulekhani Watershed, Nepal. Nat. Hazards (Dordr). 101 (9), 143–172. doi:10.1007/s11069-020-03867-3
Peng, J. B., Ma, R. Y., and Lu, Q. Z., (2004). Geological hazards of uplift of Qinghai-Tibet plateau. Adv. earth Sci. 19 (3), 457–466. doi:10.3321/j.issn:1001-8166.2004.03.018
Qiu, H. J., Zhu, Y. R., Zhou, W. Q., Sun, H., He, J., and Liu, Z. (2022). Influence of DEM resolution on landslide simulation performance based on the Scoops3D model. Geomatics, Nat. Hazards Risk 13 (1), 1663–1681.
Qu, R., Li, Z. X., and He, Z. W., (2018). Characteristics and causes of low frequency debris flow induced by heavy rainfall in Dagou village, Tianshui city, Gansu province, China. Mt. Res. 36 (3), 488–495. doi:10.16089/j.cnki.1008-2786.000345
Song, B., Shen, J. H., and Li, J. Y., (2018). Application of RAMMS model on simulation of debris flow in the Basha Gully [J]. J. Sediment Res. 43 (1), 32–37. doi:10.16239/j.cnki.0468-155x.2018.01.006
Su, P. C., Liu, X. L., and Wang, Q. C., (2004). Debris flow characteristics and hazard evaluation in Qiongshan gully of Danba County of Sichuan. J. Geol. Hazards Environ. Preserv. 15 (1), 9–12. doi:10.3969/j.issn.1006-4362.2004.01.003
Tang, C., Li, W. L., and Ding, J., (2011). Field investigation and research on giant debris flow on August 14, 2010 in yinxiu town, epicenter of wenchuan earthquake. Earth Science- J. China Univ. Geosciences 36 (1), 172–180. doi:10.3799/dqkx.2011.018
Tang, C., Zhou, J. Q., and Zhu, J., (1994). A study on the risk zonging of debris flow on alluvial fans by applying technology of numerical sumulation. J. Catastrophology 9 (4), 7–13.
Tang, C., Zhu, J., Ding, J., Cui, X. F., Chen, L., and Zhang, J. S. (2011). Catastrophic debris flows triggered by a 14 August 2010 rainfall at the epicenter of the Wenchuan earthquake. Landslides 8 (4), 485–497.
Tie, Y. B., Zhou, H. F., and Ni, H. Y. (2013). Formation of low frequency debris flow induced by short-time heavy rainfall in mountain area of southwest, China—take Lengmu debris flow as an example, Baoxing, Sichuan province. J. Catastrophology (04), 110–113. doi:10.3969/j.issn.1000-811X.2013.04.020
Wang, L. Y., Qiu, H. J., Zhou, W. Q., Zhu, Y., and Liu, Z., (2022). The post-failure spatiotemporal deformation of certain translational landslides may follow the pre-failure pattern. Remote Sens. 14, 2333.
Wang, W. Y. (1995). Debris flow and its control countermeasures in loess hilly and gully region of eastern Qinghai Province[J]. Chin. soil water conservation 02 (1), 11–13.
Wang, Y. Y., Zhan, Q. D., and Han, W. L., (2003). Stress-strain properties of viscous debris flow and determination of volocity parameter[J]. Chin. J. Geol. Hazard Control 14 (1), 9–13. doi:10.3969/j.issn.1003-8035.2003.01.002
Wei, F. Q., Hu, K. H., and Cui, P., (2002). Characteristics and origing debirs flow of Jiangjiagou valiey blodking. J. Soil Water Conservation 16 (6), 71–75. doi:10.13870/j.cnki.stbcxb.2002.06.021
Wu, J. H., Zhang, C. S., and Yang, W. M., (2022). Risk assessment of debris flow in Zhujiagully watershed based on entropy weight coefficient of variation fusion algorithm and FLO-2D[J]. J. Nat. disasters 31 (1), 81–91. doi:10.13577/j.jnd.2022.0108
Wu, Y. H., Liu, K. F., and Chen, Y. C. (2013). Comparison between FLO-2D and Debris-2D on the application of assessment of granular debris flow hazards with case study. J. Mt. Sci. 10 (2), 293–304.
Xu, Q., Li, H. J., and He, Y. S., (2017). Quantitative evaluation of engineering treatments for prevention of debris flow at Wenjia gully. J. Eng. Geol. 25 (4), 1046–1056. doi:10.13544/j.cnki.jeg.2017.04.019
Yang, D., Qiu, H., Ma, S., Liu, Z., Du, C., and Zhu, Y. (2022). Slow surface subsidence and its impact on shallow loess landslides in a coal mining area[J]. Catena 209, 105830. doi:10.1016/j.catena.2021.105830
Zhang, L. F., and Tang, S. Q. (1994). Debris flow and its countermeasures in Gansu province. J. Arid Land Resour. Environ. 8 (4), 27–34. doi:10.13448/j.cnki.jalre.1994.04.004
Zhang, P., Ma, J. Z., and Shu, H. P., (2014). Numerical simulation of erosion and deposition debris flow based on FLO-2D Model. J. Lanzhou Univ. Nat. Sci. 50 (3), 363–368. doi:10.13885/j.issn.0455-2059.2014.03.011
Zhang, Y. S., Guo, C. B., and Yao, X., (2016). Research on the geohazard effect of active fault on the eastern Margin of the Tibetan plateau. Acta Geosci. Sin. 37 (3), 277–286. doi:10.3975/cagsb.2016.03.03
Keywords: debris flow, hazarda assessment, formation mechanism, small watershed, western gully of zhangjiayuan
Citation: Yang W, Wan F, Ma S, Qu J, Zhang C and Tang H (2023) Hazard assessment and formation mechanism of debris flow outbursts in a small watershed of the Linxia Basin. Front. Earth Sci. 10:994593. doi: 10.3389/feart.2022.994593
Received: 15 July 2022; Accepted: 26 September 2022;
Published: 09 January 2023.
Edited by:
Haijun Qiu, Northwest University, ChinaReviewed by:
Chao Ma, Beijing Forestry University, ChinaLingzhi Xiang, Chongqing Jiaotong University, China
Copyright © 2023 Yang, Wan, Ma, Qu, Zhang and Tang. This is an open-access article distributed under the terms of the Creative Commons Attribution License (CC BY). The use, distribution or reproduction in other forums is permitted, provided the original author(s) and the copyright owner(s) are credited and that the original publication in this journal is cited, in accordance with accepted academic practice. No use, distribution or reproduction is permitted which does not comply with these terms.
*Correspondence: Weimin Yang, snon_72@163.com