- 1Institute of Geology and Paleontology, Linyi University, Linyi, Shandong, China
- 2College of Geosciences, China University of Petroleum, Beijing, China
- 3Petroleum Exploration and Production Research Institute, SINOPEC, Beijing, China
- 4College of Mining Engineering, North China University of Science and Technology, Tangshan, China
- 5Institute of Geomechanics, Chinese Academy of Geological Sciences, Beijing, China
- 6Shandong Province Research Institute of Coal Geology Planning and Exploration, Jinan, Shandong, China
Major breakthroughs have been made in the exploration and development of shale gas in the Longmaxi Formation in the southern Sichuan Basin, China. The previous have adopted various methods to study the fracturability of shale, but the quantitative characterization of the comprehensive properties of shale during hydraulic fracturing is still difficult. For this reason, in this study, taking the Longmaxi Formation shale in the Changning and Luzhou Blocks in the southern Sichuan Basin as an example, the mineral composition, petrophysical properties, rock mechanical properties and in-situ stress of the shale were systematically studied using X-ray diffraction, pulsed porosity-permeability analysis, rock mechanics and in-situ stress tests. Furthermore, the brittle mineral content, elastic modulus, and in -situ stress parameters were calculated, and the Analytic Hierarchy Process (AHP) method was adopted to establish a comprehensive evaluation index of shale fracturability. The research results show that the shale of the Longmaxi Formation in southern Sichuan is dominated by mixed shale facies, and it has the characteristics of high content of brittle minerals, low porosity, large compressive strength and Young’s modulus, and small value of in-situ stress difference coefficient. Therefore, the Longmaxi Formation shale has good fracturing conditions. TOC and quartz contents have important effects on the fracturability of the Longmaxi shale. The analytic hierarchy process was adopted to determine the weight coefficients of the modulus brittleness index, mechanical brittleness index, in-situ stress difference coefficient, rock compressive strength and TOC content. Furthermore, a comprehensive evaluation index of fracturability was constructed. Calculations show that the comprehensive fracability indices of shale in the Changning and Luzhou Blocks are 0.49 and 0.59, respectively. Moreover, the quality of shale in the Luzhou Block is better than that in the Changning Block, which is related to the organic matter and quartz content and the microscopic pore structures inside the shale.
Introduction
In recent years, major breakthroughs have been made in the exploration and development of unconventional natural gas in China (Li, 2022). As an important unconventional oil and gas resource, shale gas has become the main resource for the increase of natural gas reserves and production capacity in China (Xu et al., 2019). After nearly a decade of development, China’s shale gas production has also grown rapidly from 25 billion cubic meters in 2012 (Qiu et al., 2020) to 20 billion cubic meters in 2020 (Zhang et al., 2022). The Sichuan Basin, especially its southern region, has become the main battlefield for shale gas exploration and development in China (Fu et al., 2019). Different from conventional reservoirs, shale gas reservoirs are low-porosity and low-permeability tight reservoirs, which require hydraulic fracturing to achieve efficient development (Yu et al., 2019; Li et al., 2022). The fracturability of shale has become an important parameter for evaluating whether a complex fracture network can be effectively generated. Fracturability intuitively reflects the possibility of shale fracture and the improvement of reservoir seepage capacity (Wu et al., 2018). Generally, the evaluation indicators of shale fracturability mainly include: brittle mineral content (Mullen and Enderlin, 2012), Young’s modulus and Poisson’s ratio (Rickman et al., 2008), brittleness (Guo et al., 2015), and in-situ stress difference coefficient (Xiao et al., 2022). In recent years, more and more scholars have established a coupled multi-parameter comprehensive characterization model of shale fracturability by considering multiple geomechanical factors (Huang et al., 2019; Chen et al., 2021; Dou et al., 2021; Xiao et al., 2022).
At present, the quantitative characterization of the comprehensive properties of shale during hydraulic fracturing is still difficult. For this reason, in this study, taking the Longmaxi Formation shale in the Changning and Luzhou Blocks in the southern Sichuan Basin as an example, the mineral composition, petrophysical properties, rock mechanical properties and in-situ stress of the shale were systematically studied using X-ray diffraction, pulsed porosity-permeability analysis, rock mechanics and in-situ stress tests. Furthermore, the brittle mineral content, elastic modulus, and in-situ stress parameters were calculated, and the Analytic Hierarchy Process (AHP) method was adopted to establish a comprehensive evaluation index of shale fracturability.
Geological background
The Sichuan Basin is located in South China and belongs to the secondary structural unit of the Yangtze Platform (Shi et al., 2021). It is surrounded by mountains and has a NE-trending diamond shape as a whole (Shi et al., 2022). The east of the Sichuan Basin is the Daloushan Fold Belt, the south is the Emeishan Fold Belt, the west is the Longmenshan Fault Zone, and the north is the Qinling-Dabieshan Orogenic Belt. According to the regional tectonic characteristics, the interior of the Sichuan Basin is further divided into six secondary structural units, namely, the low-gentle structural belt in northern Sichuan, the low-steep structural belt in western Sichuan, the gentle structural belt in central Sichuan, the high-steep structural belt in eastern Sichuan, the low-fold structural belt in southwestern Sichuan, and the low-steep structural belt in southern Sichuan (Zhang, 2021; Zhang et al., 2021; Zou et al., 2022). The study area is located in the low-steep structural belt in southern Sichuan (Figure 1).
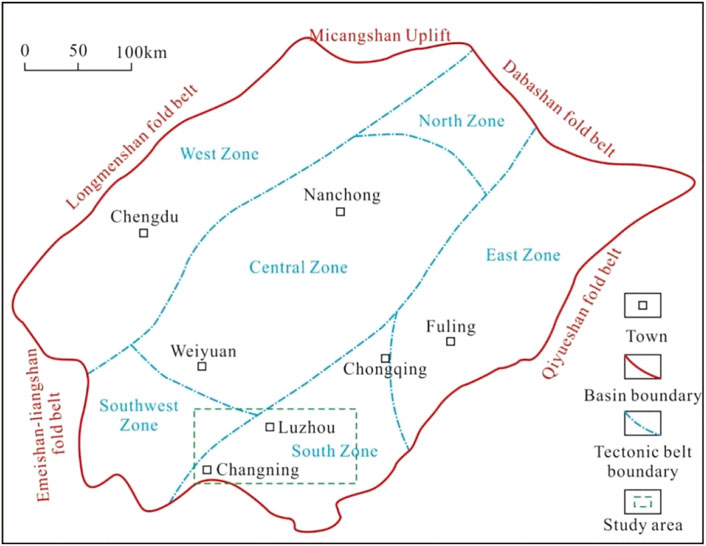
FIGURE 1. Location of the study area (Tang et al., 2020)
On the pre-Sinian metamorphic rock basement, the Sichuan Basin has experienced seven tectonic evolutions successively, including the Caledonian Movement, the Hercynian Movement, the Indosinian Movement, the Yanshan Movement and the Cenozoic Himalayan Movement. From bottom to top, it develops Paleozoic Cambrian, Ordovician, Silurian, Carboniferous and Permian, Mesozoic Triassic, Jurassic and Cretaceous strata (Liu et al., 2021). From the Late Ordovician to the Early Silurian, the Sichuan Basin was in a clastic shelf facies depositional environment. However, affected by the Caledonian Movement, the depth of the water body in the southern and northeastern parts of the Sichuan Basin is relatively large, and the sediments are in a deep-water shelf deposition environment (Xiong et al., 2021). Therefore, the energy of the water body is low, and the sediment as a whole is in an anoxic environment. Furthermore, the thick organic-rich shale deposits from the Ordovician Wufeng Formation to the Silurian Longmaxi Formation were formed (Tang et al., 2020; Liu et al., 2021; Shi et al., 2021). The buried depth of shale in the Longmaxi Formation in the southern Sichuan Basin varies widely. The buried depth of shale in the Longmaxi Formation in Changning Block rangs from 2000 to 3,500 m, while that in the Luzhou Block ranges from 3,500 m to 4,500 m (Chen et al., 2022).
Samples and experimental methods
The experimental samples were all taken from the Luzhou and Changning Blocks of the Longmaxi Formation shale in the southern Sichuan region, with a total of 24 samples. All samples are dark shales. Among them, the depth of samples in the Luzhou Block is 3,300–3500 m, and that in the Changning Block is 2,100–2500 m. In order to clarify the fracturability of the Longmaxi Formation shale in the study area, tests such as X-ray diffraction, pulsed porosity and permeability, rock mechanics, and in-situ stress were systematically carried out in this paper.
A SmartLab-9 X-ray diffractometer was used to carry out quantitative analysis of whole-rock minerals in shale samples. The shale samples were ground, dried and passed through a 200 mesh screen. The experimental operation process refers to SYT 5163–2010 “X-ray Diffraction Analysis Method of Clay Minerals and Common Non-Clay Minerals in Sedimentary Rocks”.
The total organic carbon content (TOC) of shale is measured by a K35222 carbon sulfur analyzer. The experimental procedure was carried out with reference to the standard GB/T 19,145–2003 “Determination of total organic carbon in sedimentary rocks”.
The storage properties of shale samples were tested by a TC-200 pulsed porosity and permeability tester. The experimental procedure was carried out with reference to the standard GB/T 29,172–2012 “Core Analysis Method”. First, the shale samples were cut and machined into standard core columns with a diameter of 2.5 cm and a length of about 5.0 cm. Then, an S4800 scanning electron microscope was employed to observe the pore structures of the shale.
The MTS815 rock mechanics analysis system was adopted to complete the shale rock mechanics tests. The test item is a triaxial compression test. The maximum axial load of the compressor is 2,800 kN, the maximum confining pressure is 80 MPa, the maximum pore water pressure is 80 MPa, and the maximum temperature is 200°C. Similarly, the samples were cut into standard core columns 2.5 cm in diameter and 5.0 cm in length. For the five shale samples in the Changning Block, the burial depth is shallow (about 2300 m), and the experimental temperature is set at 75°C, the experimental confining pressure is 49 MPa, and the overlying rock pressure is 61 MPa. For the six shale samples in the Luzhou Block, the burial depth is about 3500 m, the experimental temperature is set at 121°C, the experimental confining pressure is 82 MPa, and the overlying rock pressure is 91 MPa. The rock mechanics parameters tested include the triaxial compressive strength, the young’s modulus and the Poisson’s ratio.
In addition, the in-situ stress tester was used to pressurize from 150 Psi to 10,000 Psi, and the in-situ stress test of shale was completed in three cycles. Changes in strain throughout the compression/decompression process are recorded.
Results
Mineral composition of shale reservoirs
Whole-rock mineral composition analysis of 24 shale samples in the study area shows that the mineral composition of the Longmaxi Formation shale is dominated by clay minerals and quartz, the sum of which is as high as 93.6%. Followed by feldspar and calcite content, in addition, there is a small amount of pyrite in the samples (Table 1). According to the shale facies classification standard (Liang et al., 2014; Xiao et al., 2022), the shale of the Longmaxi Formation in the study area is dominated by mixed shale facies (Figure 2).
Overall, the Longmaxi Formation shale in the southern Sichuan Basin has the highest clay mineral content, ranging from 10.7% to 54.3%, with an average of 35.35%; followed by quartz minerals, the content of which is between 14.5% and 66.5%, and the average value is 35.08%; the mineral content of feldspar ranges from 1.8% to 12.5%, with an average of 6.48%. Moreover, the content of calcite minerals varies widely, ranging from 0% to 56.4%, with an average value of 13.66%. In addition, the content of pyrite is relatively low, which generally does not exceed 5%. Statistics show that the content of pyrite ranges from 0% to 13.9%, with an average content of 3.18% (Figure 3).
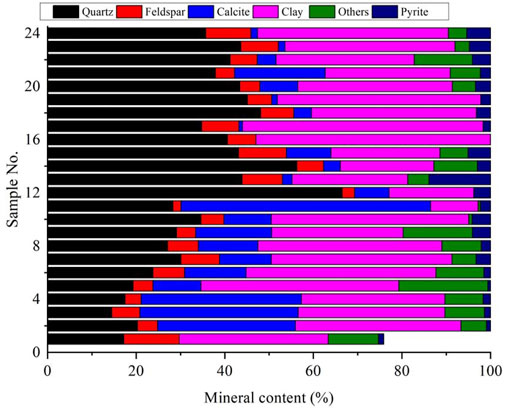
FIGURE 3. Distribution characteristics of whole-rock mineral content in the Longmaxi Formation shale in the study area.
In addition, there is a certain difference in the mineral content of the shale in the Changning and Luzhou Blocks. The content of quartz and feldspar in the shale in the Luzhou Block is significantly higher than that in the Changning area, while the calcite content in the shale is significantly lower than that in the Changning area, and the shale in the two blocks has comparable clay mineral content (Figure 4).
The difference in calcite content in shale in the two regions is mainly caused by the difference in depositional environment. The shale in the Luzhou Block has a relatively deep burial depth and is a product of deep-water shelf facies, while the shale in the Changning area has a relatively shallow burial depth and is a calcareous shallow-water shelf facies deposition (Zou et al., 2022). Statistics show that the clay mineral content of the shale in the Changning area is 10.7%–44.7%, with an average of 34.21%, and the clay mineral content of the shale in the Luzhou Block is 21.1%–54.3%, with an average of 36.50%.
Petrophysical properties of shale reservoirs
According to the analysis results of whole-rock mineral composition of the 24 shale samples in the study area, the porosity of shale is relatively low. The range of porosity is in the range of 1.96%–8.12%, with an average value of 4.80%. And with the increase of depth, the porosity of the shale in the Changning Block gradually increases, while the porosity of the shale in the Luzhou Block does not change significantly (Figure 5A). In comparison, the porosity of the Changning Block shale ranges from 2.36% to 6.91%, with an average of 4.60%. More than 33% of the samples have a porosity greater than 4%, while more than 16.7% of the samples have a porosity greater than 6%; however, the porosity of the shale in the Luzhou Block varies widely, ranging from 1.96% to 8.12%, with an average value of 4.99%. Moreover, more than 37.5% of the samples have a porosity greater than 4%, while more than 12.5% of the samples have a porosity greater than 6% (Figures 5B,C).
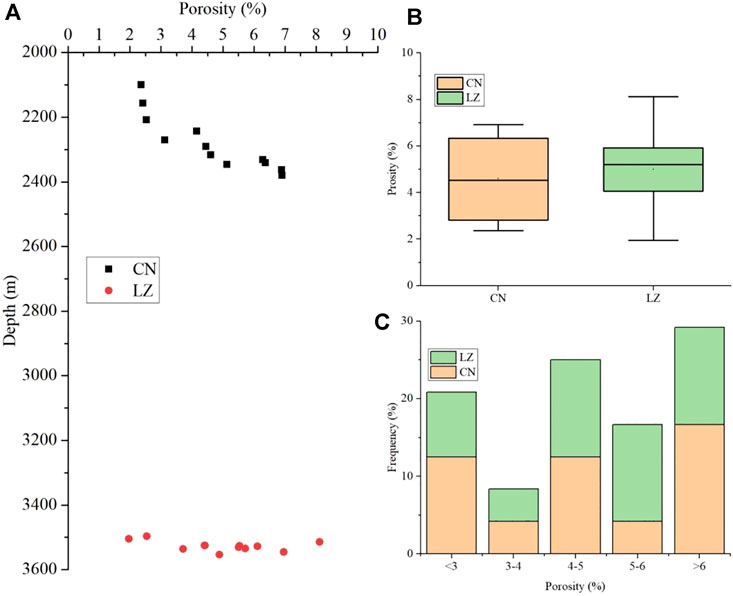
FIGURE 5. Porosity distribution of the Longmaxi Formation shale in the study area. (A) Porosity variation with depth, (B) Box plot of porosity in the Longmaxi Formation shale in Changning and Luzhou blocks, and (C) Cumulative distribution frequency of porosity.
Microscopic pore structures of shale reservoirs
It can be seen from Figure 6 that the shale of the Longmaxi Formation in the southern Sichuan area mainly develops organic pores, mineral intergranular pores, intragranular pores, intercrystalline pores, clay mineral interlayer pores and micro-fractures. In comparison, due to the shallow burial depth of the shale of the Longmaxi Formation in the Changning Block, the shale mainly develops intergranular pores, organic pores and pyrite intercrystalline pores (Figures 6A–C), and micro-fractures are poorly developed. This can explain that the porosity of the shale in the Changning Block gradually increases with depth. However, the shale in the Luzhou Block has a larger burial depth. In addition to a large number of organic pores, the pore types of shale in this area also develop kaolinite interlayer pores and micro-fractures (Figures 6D–F). Therefore, the porosity of shale in this area is relatively high, and the porosity does not change significantly with the increase of burial depth.
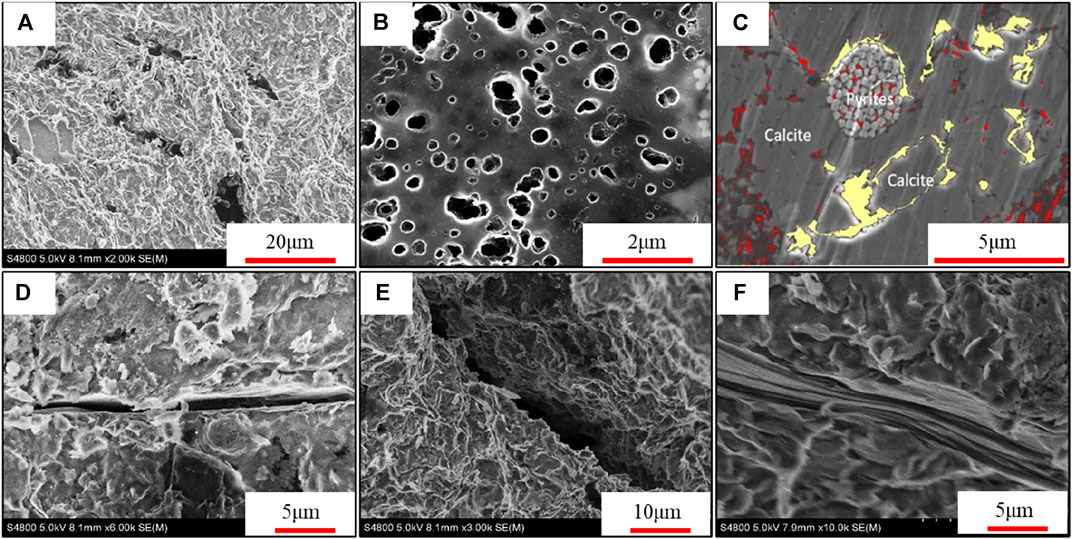
FIGURE 6. SEM images of the pore structure of the Longmaxi Formation shale in the study area. Notes: (A–C) are taken from the Changning Block, and (D–F) are taken from the Luzhou Block. (B) and (C) are from Shi et al., 2022.
Rock mechanical properties of shale reservoirs
Triaxial compression experiments were carried out on 11 samples of the Longmaxi Formation in southern Sichuan, and the test results are shown in Table 2.
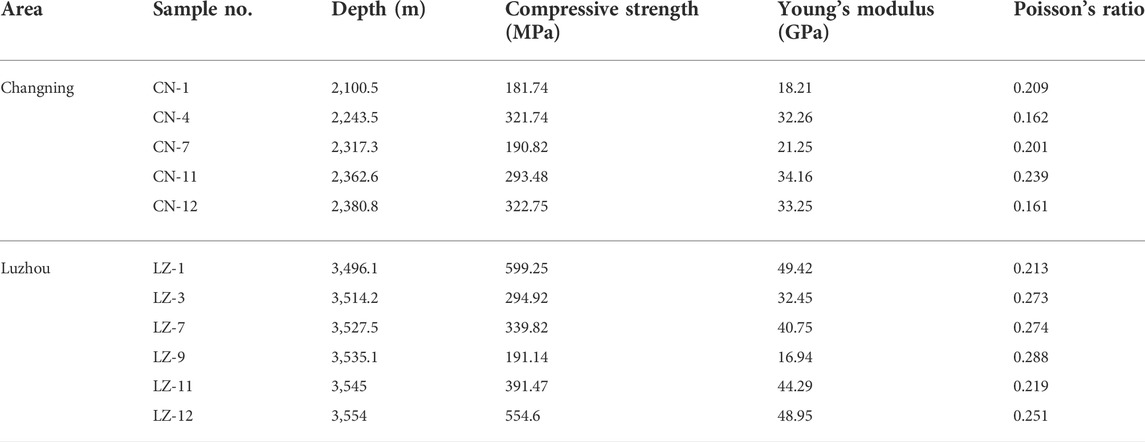
TABLE 2. Test results of triaxial compression experiments of the longmaxi formation shale in the study area.
It can be seen from Figure 7 that the compressive strength and Young’s modulus of the Longmaxi Formation shale in the southern Sichuan area are relatively large. Among them, the compressive strength of shale is generally higher than 180 MPa, and the Young’s modulus generally exceeds 18 GPa (Figure 7A). In comparison, the compressive strength and Young’s modulus of the shale in the Luzhou Block with a relatively large burial depth are significantly higher than those in the Changning Block. Among them, the compressive strength of shale ranges from 191.14 to 599.25 MPa with an average value of 395.20 MPa, and the Young’s modulus ranges from 16.94 to 49.42 GPa with an average value of 38.80 GPa. However, the compressive strength of shale in the Changning Block generally does not exceed 350 MPa, which is between 181.74 MPa and 322.75 MPa, and the average is 262.11 MPa; the Young’s modulus generally does not exceed 35 GPa, which is between 18.21 and 33.25 GPa, and the average value is 27.83 GPa (Figure 7B).
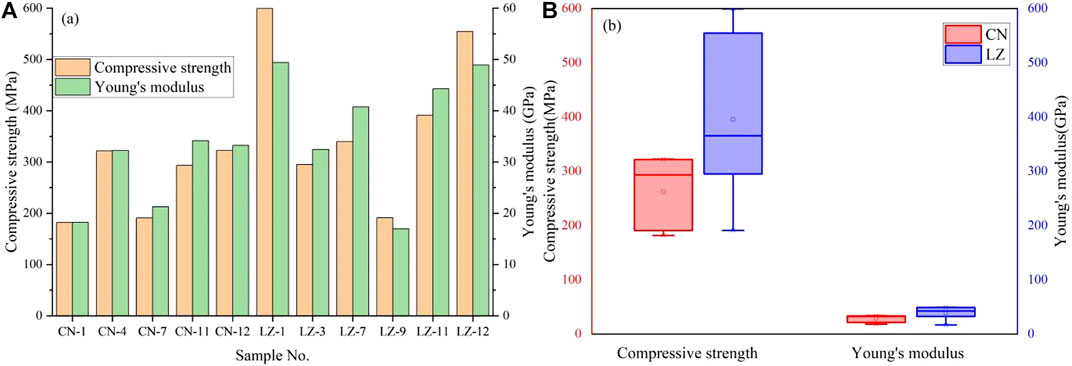
FIGURE 7. Distribution of compressive strength and Young’s modulus of the Longmaxi Formation shale in the study area. Notes: (A) Test results of compressive strength of different samples; (B) Distribution intervals of compressive strength.
In-situ stress characteristics
The in-situ stress test results of the Longmaxi Formation in the study area are shown in Table 3. The results show that the three principal stresses in the Changning Block satisfy σv>σH>σh. The average value of the vertical principal stress is 60.65 MPa, and the average value of its stress gradient is 0.0257 MPa/m; the average value of the maximum horizontal principal stress is 21.15 MPa, and the average value of its stress gradient is 0.0090 MPa/m; the average value of the minimum horizontal principal stress is 20.9 MPa, and the average value of its stress gradient is 0.0089 MPa/m.
The three principal stresses in the Luzhou Block satisfy σH>σv>σh. The average value of the vertical principal stress is 90.15 MPa, and the average value of its stress gradient is 0.0256 MPa/m; the average value of the maximum horizontal principal stress is 94.45 MPa, and the average value of its stress gradient is 0.0268 MPa/m; the average value of the minimum horizontal principal stress is 83.6 MPa, and the average value of its stress gradient is 0.0237 MPa/m.
Discussion
Fracturability analysis based on mineral composition
Brittle minerals in the mineral composition, such as quartz, feldspar, and calcite, are the main internal factors controlling the degree of fracture development, and directly affect the storage space and seepage channels of shale (Chen et al., 2012). Although pyrite is a brittle mineral, its content is generally low, and its contribution to the development of shale fractures is small. Therefore, in general, quartz, feldspar, and calcite are defined as brittle minerals (Xiao et al., 2022). Furthermore, the content of these three minerals as a percentage of the total mineral content is defined as the brittleness index:
In the formula, B1 is the brittleness index, dimensionless; CQ is the percentage of quartz minerals, %; CF is the percentage of calcite minerals, %; CC is the percentage of calcite minerals, %.
The calculation results are shown in Figure 8. The brittleness index of the Longmaxi Formation shale in the study area is generally higher than 0.4. Shales with a brittleness index higher than 0.4 generally have good fracturability (Xiao et al., 2022). Among them, the brittleness index of shale in Changning area is between 0.35 and 0.85, and its average is 0.55; while the brittleness index of shale in Luzhou area is between 0.44 and 0.66, and its average is 0.55. It reflects that the shale of the Longmaxi Formation in the southern Sichuan area has a high content of brittle minerals, and it has good fracturability.
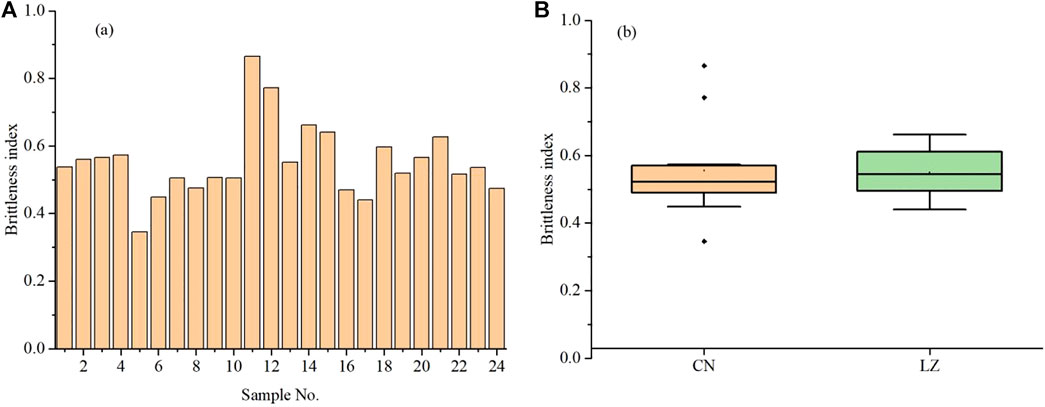
FIGURE 8. Distribution of the brittleness index of the Longmaxi Formation shale in the study area. Notes: (A) Brittleness index distribution for different samples; (B) Distribution range of brittleness index.
However, brittle mineral content represents only one aspect of shale fracability. The final determination of fracturability also takes into account its mechanical properties, such as bulk modulus. The bulk modulus of a mineral represents the energy required for its deformation or rupture. The higher the bulk modulus, the smaller the capacity required to reflect the deformation or rupture of the mineral, and the stronger the fracturability; on the contrary, the weaker the fracturability of the mineral. According to Fjaer et al. (2008), the bulk moduli of quartz, feldspar, and calcite minerals are 37.5, 76, and 74 GPa, respectively. Quartz minerals have the smallest bulk modulus and contribute the most to shale fracturability. Therefore, the bulk modulus contributions of quartz, feldspar and calcite are defined as 1.0, 49, and 0.51, respectively. Furthermore, the modulus brittleness index is defined as:
In the formula, BM is the modulus brittleness index, dimensionless; CQ is the percentage of quartz minerals, %; CF is the percentage of calcite minerals, %; CC is the percentage of calcite minerals, %; MQ, MF, and MC are the bulk modulus contributions of quartz, feldspar, and calcite, respectively, dimensionless, which are defined as 1.0, 0.49, and 0.51, respectively.
The calculation results of the modulus brittleness index of the Longmaxi Formation shale in the study area are shown in Figure 9. The modulus brittleness index of the Longmaxi shale is generally higher than 0.30. Among them, the modulus brittleness index of shale in the Changning area is between 0.27 and 0.72, with an average value of 0.41; while that in the Luzhou Block is between 0.39 and 0.61, and its average value is 0.46. It reflects that the Longmaxi Formation in southern Sichuan area has good fracturability, and the shale fracturability in the Luzhou Block is significantly better than that in the Changning Block. This characteristic is not reflected by the brittle mineral content alone.
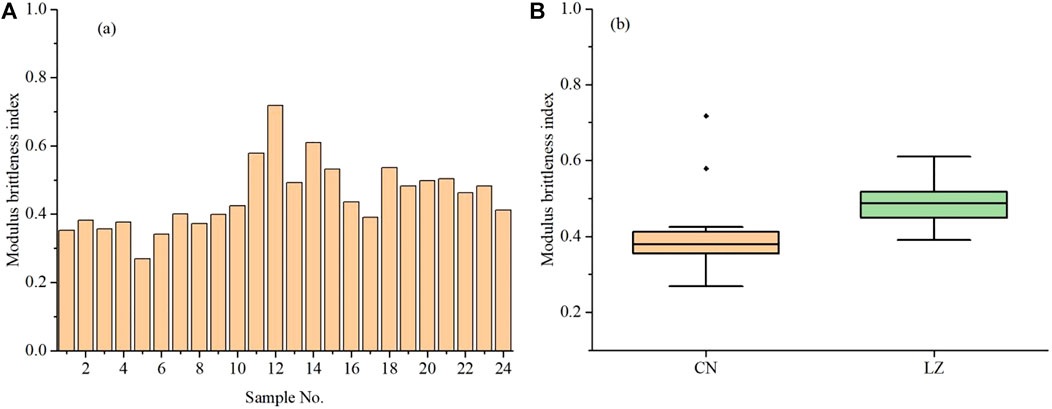
FIGURE 9. Distribution of the modulus brittleness index of the Longmaxi Formation shale in the study area. Notes: (A) Modulus brittleness index distribution for different samples; (B) Distribution range of modulus brittleness index.
Previous studies have shown that the fracturability of shale is related to factors such as quartz content, natural fractures, and diagenesis (Tang et al., 2012). It can be seen from Figure 10 that the modulus brittleness index has a good positive correlation with shale TOC and quartz content, but has a poor correlation with shale maturity Ro and shale porosity. Among them, the shale Ro is between 2.1% and 2.6%, reflecting that the shale of the Longmaxi Formation in the study area is in the late diagenetic stage (Warpinki et al., 2009), so the diagenesis of the shale has little effect on its brittleness. The organic carbon content can reflect the hydrocarbon generation ability of shale. The higher the TOC, the lower the density of shale, the more developed fractures (Cui et al., 2019), and the higher the brittleness index of shale. Quartz has the smallest bulk modulus and requires the least amount of energy to deform or fracture. Therefore, the higher the quartz content in the shale, the higher the brittleness index of the shale, and the better the fracturability of the shale. However, the porosity has little effect on the shale fracturability. Because the porosity only represents the percentage of pore space or the size of reservoir space, and is related to the related to mineral arrangement instead of the mineral composition. The essence of shale fracturing is the ability to produce micro-fractures in shale, which is essentially related to shale mineral composition and rock mechanical properties, and has nothing to do with the size of its reservoir space.
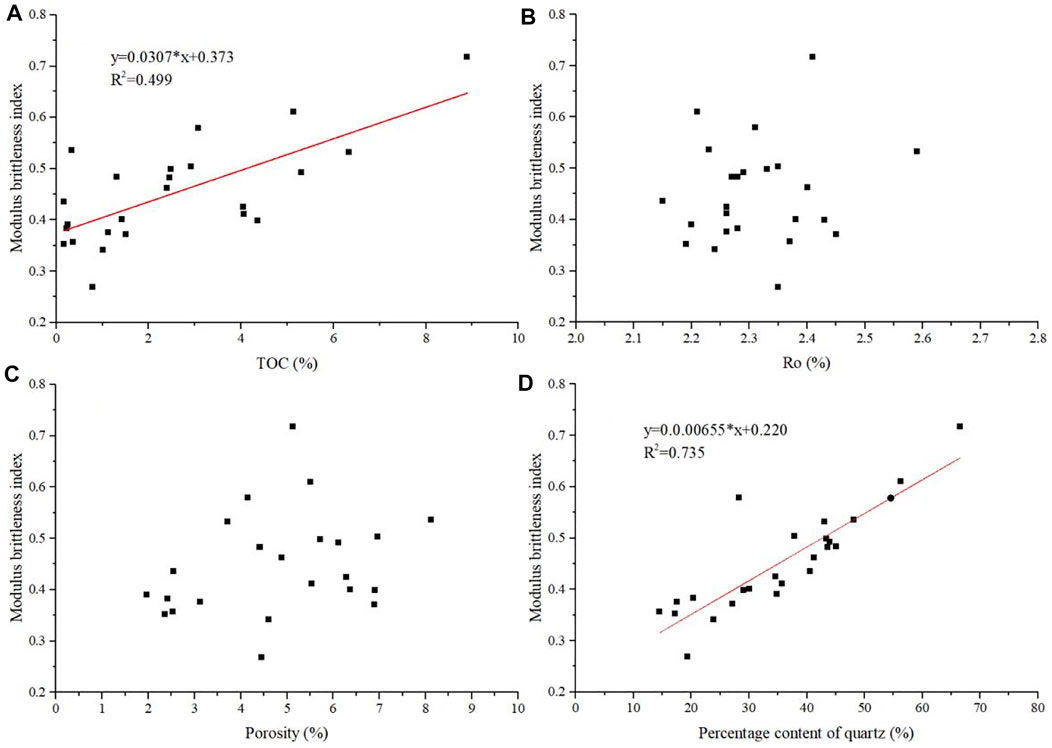
FIGURE 10. Relationship between the modulus brittleness index and TOC (A), Ro (B), porosity (C), and quartz content (D) of the Longmaxi Formation shale in the study area, respectively.
Fracturability analysis based on rock mechanical properties
According to the theory of geomechanics, the fracturability of shale can also be quantitatively evaluated by parameters such as compressive strength, Young’s modulus, and Poisson’s ratio (Tan et al., 2019). Young’s modulus and Poisson’s ratio of rock can reflect the difficulty of deformation or rupture of rock after being stressed. The higher the Young’s modulus and the larger the Poisson’s ratio, the more likely the rock is to fracture (Rickman et al., 2008). The expressions of rock brittleness uses rock mechanical parameters are shown in Eqs (3–5):
where BD is the mechanical brittleness index, En and μn are the normalized Young’s modulus and Poisson’s ratio, respectively, dimensionless; E is the Young’s modulus, and Emax and Emin are the maximum and minimum Young’s modulus of shale, respectively; μ is the Poisson’s ratio, and μmax and μmin are the maximum and minimum Poisson’s ratio of shale, respectively.
Because the rock mechanics parameters of the shale in the Luzhou and Changning Blocks are quite different, the maximum and minimum values are calculated respectively, and the calculation results are shown in Figure 11. It can be seen from Figure 11A that the mechanical brittleness index of shale in the southern Sichuan area is between 0.15 and 0.77, with an average value of 0.47, indicating that the shale fracturability is generally good. In addition, the mechanical brittleness index of shale in the Luzhou Block is significantly higher than that in the Changning Block. It shows that the shale fracturability in the Luzhou Block is better than that in the Changning Block. Moreover, the mechanical brittleness index of shale has a certain positive correlation with its quartz content (Figure 11B), indicating that quartz minerals have a great contribution to the fracturability of shale.
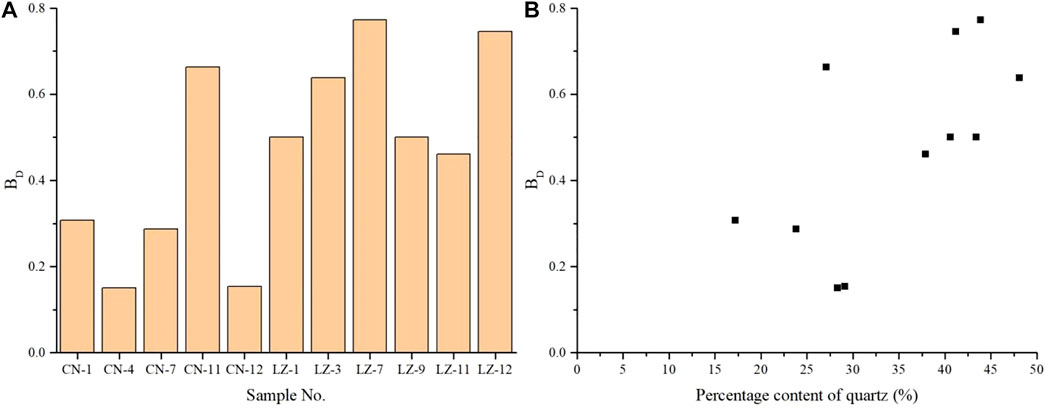
FIGURE 11. Distribution of the brittleness index of the Longmaxi Formation shale in the study area. (A) Brittleness index for different samples and (B) the relationship between the percentage content of quartz and brittleness index.
Fracturability analysis based on in-situ stress characteristics
The level of in-situ stress has an important influence on the distribution of fractures in shale and the difficulty of forming complex fracture networks. In general, the in-situ stress difference coefficient is adopted to characterize the in-situ stress:
In the formula, DF is the in-situ stress difference coefficient, dimensionless; σH is the maximum horizontal principal stress, MPa; σh is the minimum horizontal principal stress, MPa.
The calculation results are shown in Table 3. It can be seen that the in-situ stress difference coefficient of the Longmaxi Formation in the study area is between 0.010 and 0.131, with an average value of 0.071. The difference coefficient of in-situ stress is much less than 0.3, indicating that the shale in this area is prone to form a complex fracture network under the action of stress, and its fracturability is good.
Influence of shale microstructure on fracturability
The comparison indexes of the Longmaxi Formation shale in the two blocks are shown in Table 4. It can be found that the TOC, porosity, and quartz content of the shale in the Luzhou Block are significantly higher than those in the Changning Block, and the degree of microfracture development is higher, while the Ro difference is not significant. Therefore, it can be considered that the fracturability of the Longmaxi Formation shale is mainly controlled by the organic matter content, quartz content and pore structures of the shale reservoir. The higher the organic matter content, the lower the density of shale, and the more developed fractures. The higher the quartz content, the better the brittleness of the shale. However, the pore structures of the reservoir firstly control the petrophysical properties and micro-fracture development characteristics of the shale, and then controls the fracturability of the shale.

TABLE 4. Statistical results of the average value of factors affecting the fracturability of the longmaxi formation shale in the study area.
The microstructure of shale reservoir affects the occurrence state and storage characteristics of shale gas, and is the key factor controlling the fracturability of shale. The microstructure of the shale reservoir first controls the petrophysical properties of the shale reservoir. It can be seen from Figure 12 that there is a certain positive correlation between the porosity of the Longmaxi Formation shale in the southern Sichuan area and the content of siliceous minerals. With the increase of siliceous mineral content, the shale porosity increases significantly, but there are obvious differences between the Changning and Luzhou blocks. Among them, the porosity of the Changning Block shale has a good positive correlation with the content of siliceous minerals. This is mainly because the shale in the Changning Block mainly develops intergranular pores (Figure 6A–C). The higher the content of siliceous minerals, the easier the formation of intergranular pores. However, the porosity of the shale in the Luzhou Block has a relatively poor positive correlation with the content of siliceous minerals. This is mainly due to the relatively developed micro-fractures in the shale of the Luzhou Block (Figures 6D–F). Furthermore, it leads to the contribution of micro-fractures in the shale pore space of this block in addition to the contribution of intergranular pores of siliceous minerals.
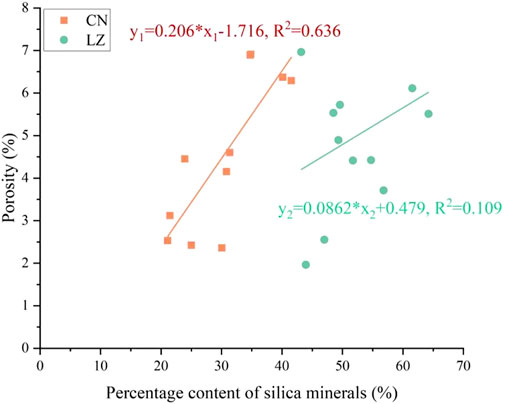
FIGURE 12. Relationship between siliceous mineral content and porosity in the Longmaxi Formation shale in the study area.
The influence of shale microscopic pore structure on the fracturability of shale is not only reflected in the control of reservoir characteristics, but also has an important influence on the characteristics of shale deformation or fracture after being stressed. Previous studies have shown that shale with natural fractures is more likely to develop micro-fractures along the fragile surface of the fracture after being stressed, and it is easier to connect to form a complex fracture network. However, shale without natural fractures will randomly generate micro-fractures after being stressed, and it requires large stress to form a connected fracture network. This is the fundamental reason why the fracturability of the shale in the Luzhou Block is significantly better than that in the Changning Block.
Comprehensive evaluation of shale fracturability
According to this study, the fracturability of shale can be evaluated from the aspects of mineral composition, rock mechanical properties and in-situ stress conditions. Moreover, the fracturability of shale is mainly controlled by multiple factors such as quartz content, TOC, mechanical properties of shale, and in-situ stress conditions, while Ro and petrophysical properties of shale have little effect on its fracturability. Therefore, the calculation formula of the comprehensive evaluation index of shale fracturability is defined as:
In the formula, B is the comprehensive evaluation index of shale fracturability, BM is the modulus brittleness index, dimensionless; BD is the mechanical brittleness index, dimensionless; DF is the in-situ stress difference coefficient, dimensionless; σc, σmax are the compressive strength and maximum compressive strength, MPa, respectively; TOC, TOCmax, and TOCmin are the organic carbon content, the maximum and minimum values of the organic carbon content, respectively; k1, k2, k3, k4, and k5 are the weight coefficients of the above five evaluation parameters, dimensionless, and k1+k2+k3+k4+k5=1.
It can be seen from Eq. 7 that the comprehensive evaluation index of shale fracturability is calculated based on the weighting of mineral modulus components, elastic modulus, in-situ stress, compressive strength and TOC parameters. Finally, k1, k2, k3, k4, and k5 are determined to be 0.3, 0.2, 0.2, 0.2, and 0.1, respectively. The comprehensive evaluation results are shown in Table 5, indicating that the fracturability of the shale in the southern Sichuan area is better, and the fracturability of the shale in the Luzhou Block is significantly better than that in the Changning Block.

TABLE 5. Comprehensive evaluation results of fracturability of the longmaxi shale in the study area.
Conclusion
(1) In this study, taking the Longmaxi Formation shale in the Changning and Luzhou Blocks in the southern Sichuan Basin as an example, the mineral composition, petrophysical properties, rock mechanical properties and in-situ stress of the shale were systematically studied using X-ray diffraction, pulsed porosity-permeability analysis, rock mechanics and in-situ stress tests. Furthermore, the brittle mineral content, elastic modulus, and in-situ stress parameters were calculated, and the Analytic Hierarchy Process (AHP) method was adopted to establish a comprehensive evaluation index of shale fracturability.
(2) The shale of the Longmaxi Formation in southern Sichuan is dominated by mixed shale facies, and it has the characteristics of high content of brittle minerals, low porosity, large compressive strength and Young’s modulus, and small value of in-situ stress difference coefficient. Therefore, the Longmaxi Formation shale has good fracturing conditions. TOC and quartz content have important effects on the fracturability of the Longmaxi shale.
(3) The analytic hierarchy process was adopted to determine the weight coefficients of the modulus brittleness index, mechanical brittleness index, in-situ stress difference coefficient, rock compressive strength and TOC. Furthermore, a comprehensive evaluation index of fracturability was constructed.
(4) The comprehensive fracability indices of shale in the Changning and Luzhou Blocks are 0.49 and 0.59, respectively. Moreover, the quality of shale in the Luzhou Block is better than that in the Changning Block, which is related to the organic matter and quartz content and the microscopic pore structures inside the shale.
Data availability statement
The original contributions presented in the study are included in the article/supplementary material, further inquiries can be directed to the corresponding author.
Author contributions
YG and KZ are responsible for the idea and writing of this paper and DW, XH, XS, and SZ are responsible for the experiments and calculation and simulation of the data.
Funding
This work was supported by the Scientific Research Foundation of the North China University of Science and Technology (BS201827), Science Foundation of Chinese Academy of Geological Sciences (DZLXJK20181201) and Linyi University (LYDX2017BS019).
Conflict of interest
Authors DW and XS were employed by the Petroleum Exploration and Production Research Institute, SINOPEC, and author SZ was employed by the Shandong Province Research Institute of Coal Geology Planning and Exploration
The remaining authors declare that the research was conducted in the absence of any commercial or financial relationships that could be construed as a potential conflict of interest.
Publisher’s note
All claims expressed in this article are solely those of the authors and do not necessarily represent those of their affiliated organizations, or those of the publisher, the editors and the reviewers. Any product that may be evaluated in this article, or claim that may be made by its manufacturer, is not guaranteed or endorsed by the publisher.
References
Chen, J., Wang, L., Wang, C., Yao, B., Tian, Y., and Wu, Y. S. (2021). Automatic fracture optimization for shale gas reservoirs based on gradient descent method and reservoir simulation. Adv. Geo-Energy Res. 5 (2), 191–201.
Chen, S. B., Zhu, Y. M., and Wang, H. Y. (2012). Structure characteristics and accumulation significance of nanopores in Longmaxi shale gas reservoir in the southern Sichuan Basin. J. China Coal Soc. 37 (3), 438–444. doi:10.13225/j.cnki.jccs.2012.03.007
Chen, Y., Tang, H. M., and Liao, J. J. (2022). Analysis of shale pore characteristics and controlling factors based on variation of buried depth in the Longmaxi Formation, Southern Sichuan Basin. Geol. China 49 (2), 472–484. doi:10.12029/gc20220209
Cui, C. L., Dong, Z. G., and Wu, D. S. (2019). Rock mechanics study and fracability evaluation for Longmaxi Formation of Baoj ing block in Hunan Province. Nat. Gas. Geosci. 30 (5), 626–634. doi:10.11764/j.issn.1672-1926.2018.12.021
Dou, L. B., Yang, H. J., and Xiao, Y. J. (2021). Probability study of formation brittleness and new quantitative evaluation of fracability for shale reservoirs. Prog. Geophys. 36 (2), 576–584. doi:10.6038/pg2021EE0460
Fjaer, E., Holt, R. M., and Horsrud, P. (2008). Petroleum related rock mechanics. Dev. Petroleum Sci. 53, 491–492. 2nd Edition. Elsevier.
Fu, Y., Jiang, Y., Wang, Z., Hu, Q., Xie, J., Ni, G., et al. (2019). Non-connected pores of the Longmaxi shale in southern Sichuan Basin of China. Mar. Petroleum Geol. 110, 420–433. doi:10.1016/j.marpetgeo.2019.07.014
Guo, J. C., Luo, B., Zhu, H. Y., Wang, Y. H., Lu, Q. L., and Zhao, X. (2015). Evaluation of fracability and screening of perforation interval for tight sandstone gas reservoir in Western Sichuan Basin. J. Nat. Gas Sci. Eng. 25, 77–87. doi:10.1016/j.jngse.2015.04.026
Huang, C., Xu, T., Ju, Y., Zhu, H., Ju, L., and Li, W. (2019). Fracability evaluation of shale of the Wufeng-Longmaxi Formation in the changning area, Sichuan Basin. Acta Geol. sinica-. Engl. Ed. 93 (4), 996–1004. doi:10.1111/1755-6724.13877
Li, L. (2022). Development of natural gas industry in China: Review and prospect. Nat. Gas. Ind. B 9 (2), 187–196. doi:10.1016/j.ngib.2022.03.001
Li, Z., Li, G., Yu, H., Jiang, Z., Li, H., and Gong, H. (2022). Fracability evaluation based on the three-dimensional geological numerical simulation of in situ stress: Case study of the Longmaxi Formation in the weirong shale gas field, southwestern China. Math. Geosci. 54, 1069–1096. doi:10.1007/s11004-022-10001-5
Liang, C., Jiang, Z. X., Zhang, C. M., Guo, L., Yang, Y., and Li, J. (2014). The shale characteristics and shale gas exploration prospects of the lower silurian Longmaxi shale, Sichuan Basin, south China,Sichuan Basin,south China. J. Nat. Gas Sci. Eng. 21, 636–648. doi:10.1016/j.jngse.2014.09.034
Liu, W., Wu, J., Jiang, H., Zhou, Z., Luo, C., Wu, W., et al. (2021). Cenozoic exhumation and shale-gas enrichment of the Wufeng-Longmaxi formation in the southern Sichuan basin, Western China. Mar. Petroleum Geol. 125, 104865. doi:10.1016/j.marpetgeo.2020.104865
Mullen, M., and Enderlin, M. (2012). Fracability index–more than just calculating rock properties//SPE Annual Technical Conference and Exhibition. doi:10.2118/159755-MS
Qiu, Z., Zou, C., Wang, H., Dong, D., Lu, B., Chen, Z., et al. (2020). Discussion on the characteristics and controlling factors of differential enrichment of shale gas in the Wufeng-Longmaxi formations in south China. J. Nat. Gas Geoscience 5 (3), 117–128. doi:10.1016/j.jnggs.2020.05.004
Rickman, R., Mullen, M. J., and Petre, J. E. (2008). A practical use of shale petrophysics for stimulation design optimization: All shale plays are not clones of the Barnett Shale//SPE annual technical conference and exhibition. doi:10.2118/115258-MS
Shi, X., Luo, C., Cao, G., He, Y., Li, Y., Zhong, K., et al. (2021). Differences of main enrichment factors of S1l11-1 sublayer shale gas in southern Sichuan Basin. Energies 14 (17), 5472. doi:10.3390/en14175472
Shi, Z., Zhou, T., Wang, H., and Sun, S. (2022). Depositional structures and their reservoir characteristics in the wufeng–longmaxi shale in southern Sichuan Basin, China. Energies 15 (5), 1618. doi:10.3390/en15051618
Tan, Y., Pan, Z., Feng, X. T., Zhang, D., Connell, L. D., and Li, S. (2019). Laboratory characterisation of fracture compressibility for coal and shale gas reservoir rocks: A review. Int. J. Coal Geol. 204, 1–17. doi:10.1016/j.coal.2019.01.010
Tang, L., Song, Y., Jiang, S., Li, L., Li, Z., Li, Q., et al. (2020). Sealing mechanism of the roof and floor for the Wufeng-Longmaxi shale gas in the southern Sichuan Basin. Energy fuels. 34 (6), 6999–7018. doi:10.1021/acs.energyfuels.0c00983
Tang, Y., Xing, Y., and Li, L. Z. (2012). Influence factors and evaluation methods of the gas shale fracability. Earth Sci. Front. 19 (5), 356–363.
Warpinki, N. R., Mayerhof, M. J., Vinct, M. C., Cipolla, C. L., and Lolon, E. P. (2009). Stimulating unconventional reservoirs: Maximizing network growth while optimizing fracture conductivity. J. Can. Petroleum Technol. 48 (10), 39–51. doi:10.2118/114173-MS
Wu, J., Zhang, S., Cao, H., Zheng, M., Sun, P., and Luo, X. (2018). Fracability evaluation of shale gas reservoir-A case study in the Lower Cambrian Niutitang formation, northwestern Hunan, China. J. Petroleum Sci. Eng. 164, 675–684. doi:10.1016/j.petrol.2017.11.055
Xiao, J. F., Hu, P. J., and Han, L. X. (2022). Mechanical properties and compressibility evaluation of Qiongzhusi shale in Weiyuan area in southern Sichuan. Drill. Prod. Technol. 45 (2), 61–66. doi:10.3969/J.ISSN.1006-768X.2022.02.11
Xiong, J., Liu, K., Shi, C., Liu, X., and Huang, L. (2021). Logging prediction and evaluation of fracture toughness for the shales in the Longmaxi Formation, southern Sichuan Basin. Petroleum 7 (3), 254–262. doi:10.1016/j.petlm.2020.10.003
Xu, H., Zhou, W., Zhang, R., Liu, S., and Zhou, Q. (2019). Characterizations of pore, mineral and petrographic properties of marine shale using multiple techniques and their implications on gas storage capability for Sichuan Longmaxi gas shale field in China. Fuel 241, 360–371. doi:10.1016/j.fuel.2018.12.035
Yu, H., Taleghani, A. D., and Lian, Z. (2019). On how pumping hesitations may improve complexity of hydraulic fractures, a simulation study. Fuel 249, 294–308. doi:10.1016/j.fuel.2019.02.105
Zhang, D. W. (2021). Development prospect of natural gas industry in the Sichuan Basin in the next decade. Nat. Gas. Ind. B 41 (8), 119–131. doi:10.1016/j.ngib.2021.08.025
Zhang, J., Shi, M., Wang, D., Tong, Z., Hou, X., Niu, J., et al. (2022). Fields and directions for shale gas exploration in China. Nat. Gas. Ind. B 9 (1), 20–32. doi:10.1016/j.ngib.2021.08.014
Zhang, L. H., He, X., and Li, X. G. (2021). Shale gas exploration and development in the Sichuan Basin: Progress, challenge and countermeasures. Nat. Gas. Ind. 41 (8), 143–152. doi:10.3787/j.issn.1000-0976.2021.08.013
Keywords: mineral composition, rock mechanical properties, longmaxi formation, shale reservoir, fracturability, southern sichuan area
Citation: Guo Y, Wang D, Han X, Zhang K, Shang X and Zhou S (2022) Evaluation of fracturability of shale reservoirs in the longmaxi formation in southern sichuan basin. Front. Earth Sci. 10:993829. doi: 10.3389/feart.2022.993829
Received: 14 July 2022; Accepted: 27 July 2022;
Published: 17 August 2022.
Edited by:
Wenlong Ding, China University of Geosciences, ChinaReviewed by:
Qingan Zhou, Changqing Oil Field Company, ChinaWei Liao, PetroChina Xinjiang Oilfield Company, China
Copyright © 2022 Guo, Wang, Han, Zhang, Shang and Zhou. This is an open-access article distributed under the terms of the Creative Commons Attribution License (CC BY). The use, distribution or reproduction in other forums is permitted, provided the original author(s) and the copyright owner(s) are credited and that the original publication in this journal is cited, in accordance with accepted academic practice. No use, distribution or reproduction is permitted which does not comply with these terms.
*Correspondence: Kaixun Zhang, emhhbmdrYWl4dW5AMTI2LmNvbQ==