- 1College of Geosciences and Engineering, North China University of Water Resources and Electric Power, Zhengzhou, China
- 2School of Mining Engineering and Geology, Xinjiang Institute of Engineering, Wulumuqi, China
The Yanqi Basin is a coal-bearing basin in Western China. In order to determine the hydrocarbon generation potential of the Jurassic source rocks in this area, the organic matter composition of coal-measure mudstone, carbonaceous mudstone and coal rocks, the yields of liquid and gaseous hydrocarbons, and the comparison of hydrocarbon sources were studied by means of hydrocarbon-generating thermal simulation experiments. The results showed that the micro-composition of the Jurassic coal rocks in the Yanqi Basin showed the characteristics of rich vitrinite-inertinite and poor chitinite + saprolite components; however, the micro-composition of the mudstone showed the characteristics of poor inertinite and rich vitrinite–chitinite + saprolite components. The Jurassic mudstone has a wide distribution range of hydrogen and oxygen indexes, among which the hydrogen index is distributed between 2 and 266 mg/gTOC, and the oxygen index is distributed between 3 and 183 mg/g TOC. The organic matter in the mudstone belongs to Type II2-III kerogen. The hydrogen index of coal is distributed in the range of 100–300 mg/gTOC, which is obviously larger than that of mudstone. The total liquid hydrocarbon yield of the coal rock is the highest (43.2 mg/gTOC), followed by mudstone (16.4–20.4 mg/gTOC), while that of the carbonaceous mudstone is the lowest (5.36 mg/gTOC). In addition, mudstone has the highest yield of gaseous hydrocarbons, followed by coal, while that of carbonaceous mudstone is the lowest. The oil-source comparison showed that the Jurassic crude oil has a certain affinity with its main source rocks (mudstone, carbonaceous mudstone, and coal). At the same time, the comparison results of steroid and terpene biomarker parameters with crude oil showed that the Middle and Lower Jurassic mudstone, carbonaceous mudstone, and coal rock in this area are all source rocks and the Badaowan Formation mudstone is the main hydrocarbon source.
1 Introduction
The Yanqi Basin is a small coal-bearing basin in Western China. At present, certain breakthroughs in oil and gas exploration have been obtained in the Jurassic coal-measure strata in the Northern Sag. However, due to lack of research on the geochemical characteristics, organic petrological properties, and hydrocarbon generation mechanism of coal-measure source rocks, the prediction of Jurassic oil and gas resources and the evaluation of exploration prospects in the Yanqi Basin are restricted. The Yanqi Basin is located between the three major basins of Tarim, Junggar, and Tuha in China. It is a Mesozoic–Cenozoic sedimentary basin formed on the varixian folded basement (Cai et al., 2008; Guo et al., 2012). In addition to the good distribution of organic matter, there is a relatively high geothermal gradient inside the basin, which provides favorable conditions for the massive generation and accumulation of oil and gas (Li, 2000; He et al., 2013; Li et al., 2019).
The Yanqi Basin has experienced three large-scale lake transgressions since the Mesozoic. Furthermore, three sets of coal-bearing and carbonaceous mudstone lithologic assemblages were formed in the three coal accumulation stages. The three coal accumulation periods are the Triassic, Middle and Late Jurassic Badaowan Formation period, and Jurassic (Yao et al., 2003). Sedimentary facies studies show that the Jurassic is dominated by fluvial, delta, and shore-shallow lacustrine facies, especially the extensive development of shore-lake facies (Yao et al., 2003; Zhao et al., 2013). In order to explore the hydrocarbon generation potential of the Jurassic source rocks in this area, the organic matter composition of the coal-measure mudstone, carbonaceous mudstone and coal, the yields of liquid and gaseous hydrocarbons, and the comparison of hydrocarbon sources were studied by means of hydrocarbon-generating thermal simulation experiments. This study can provide a reference for similar studies worldwide.
2 Databases and methods
2.1 Geological background
The Yanqi Basin is located in the east of the South Tianshan Orogenic Belt and is a Mesozoic–Cenozoic sedimentary basin formed on the Hercynian folded basement. The Yanqi Basin covers an area of 1.3×104 km2 (Figure 1). The sedimentary caprocks of the basin composed of Mesozoic Triassic, Jurassic, Cenozoic Tertiary and Quaternary, and Jurassic is the main exploration target layer (Yao et al., 2004; Zhang et al., 2011).
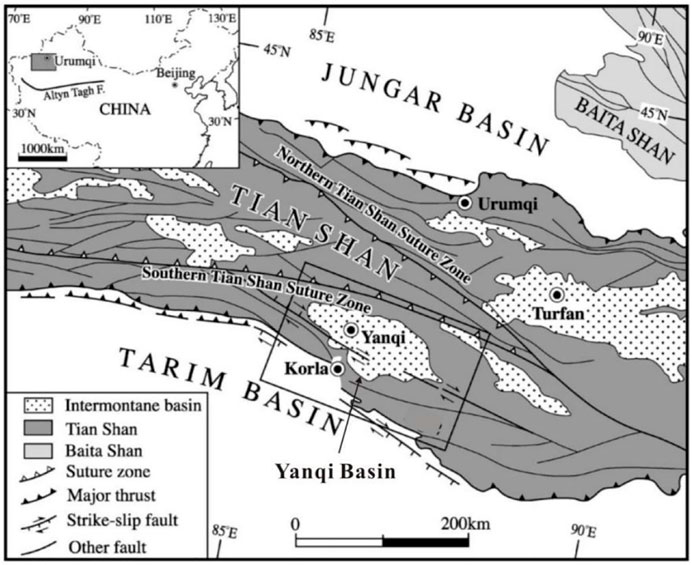
FIGURE 1. Location and regional structural characteristics of the Yanqi Basin, China (Lin et al., 2002).
2.2 Thermal-pressure simulation experiments of coal-measure source rocks
In this study, the Jurassic low-maturity source rocks (dark gray mudstone, carbonaceous mudstone, and coal rock) (Table 1) were used for thermal-pressure simulation experiments under low temperature (360°C), long time (greater than 72 h), and water additions.
The thermal simulation experiment of hydrocarbon generation adopts the golden tube-autoclave thermal simulation system. The experimental conditions of the autoclave thermal simulation are as follows: the samples were heated from room temperature to 150°C within 10 h, and then they were heated to 450°C at 2°C/h. After thermal simulation, the gas in the gold tube was punctured and collected in a special glass vacuum device. The device is connected to an Agilent HP6890N chromatographic instrument, and gaseous products can be drawn into the chromatograph for analysis using an automatic control program. Gaseous hydrocarbon carbon isotopes were analyzed using a Delta V Advantage isotope mass spectrometer. The chromatographic column is a CP-Pora PLOT Q quartz column (30 m×0.32 mm×20 μm). For quantitative analysis of thermally simulated liquid hydrocarbons, an Agilent HP6890 was used as the chromatograph, and the chromatographic column was an HP-5 column (30 m×0.32 mm×0.25 μm).
3 Results
3.1 Organic micro-components of coal-measure source rocks
The micro-composition of the Jurassic coal rocks in the Yanqi Basin shows the characteristics of rich vitrinite–inertinite and poor chitinite + saprolite components; however, the micro-composition of the mudstone shows the characteristics of poor inertinite and rich vitrinite–chitinite + saprolite components (Figure 2). The average composition of micro-components in coal rock are as follows: vitrinite 72.0%, inertinite 19.4%, and chitinite + saprolite 8.6%; the average composition of micro-components in the mudstone are as follows: vitrinite 59.5%, inertinite 14.5%, and chitinite + saprolite 26.0%. In the distribution form of the micro components, the vitrinite–inertinite combination showed a distinct band-like distribution. The distribution of micro-components in mudstone is also relatively scattered, with no obvious center, reflecting the diversity of depositional environment and biogenic materials (Curtis et al., 2012; Jiang et al., 2015; Gai et al., 2016).
3.2 Organic geochemical characteristics of source rocks
3.2.1 Organic matter abundance
The organic carbon content of coal-measure mudstone is generally higher than that of lacustrine mudstone. Taking the Qinshui Basin as an example, the TOC of coal-measure mudstone in the Shanxi Formation is usually distributed in the range of 1%–6%, while the TOC of general lacustrine mudstone is usually distributed in the range of 0.1%–4%. However, the hydrocarbon generation potential, soluble organic matter, and total hydrocarbon contents of coal-measure mudstone are generally lower than those of lacustrine mudstone with the same organic carbon content. Therefore, when evaluating the organic carbon abundance of coal-measure mudstone, the evaluation standard of lacustrine mudstone cannot be used.
Tables 2, 3 show the statistical results of the organic matter abundance of the coal measure mudstones in the Badaowan, Sangonghe, and Xishanyao Formations and the coal rocks in the Bohu Depression.
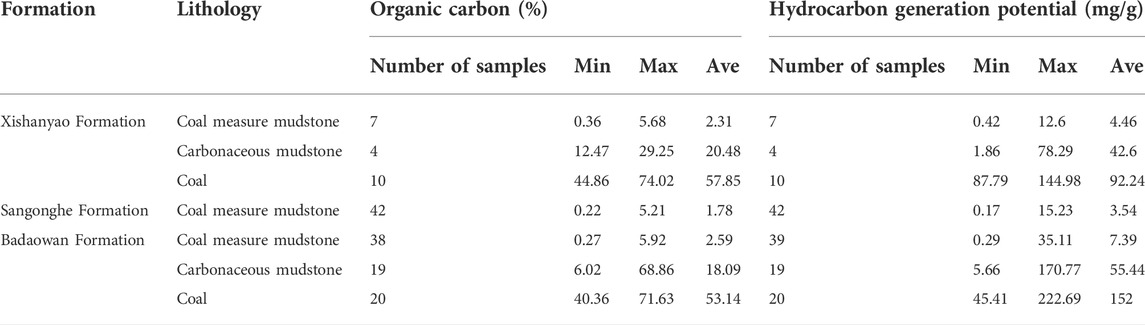
TABLE 2. Test results of organic carbon content and hydrocarbon generation potential of Jurassic source rocks in the Yanqi Basin.
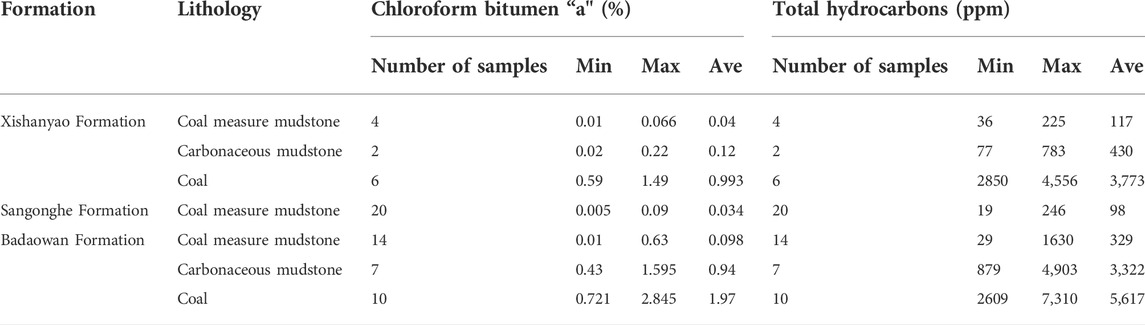
TABLE 3. Test results of chloroform bitumen “A” and total hydrocarbon content of Jurassic source rocks in the Yanqi Basin.
Overall, the Jurassic coal-measure mudstones in the study area belong to medium–good source rocks, which account for 50%–60% of the total samples, while the poor-non-source rocks account for 40%–50% of the total samples. Carbonaceous mudstones belong to medium source rocks, while they account for 45% of the total samples, and the poor source rocks account for 30% of the total samples. Moreover, 55%–65% of the coal rocks belong to poor source rocks, and only 30% are medium source rocks. Among the various Jurassic source rocks, the Lower Jurassic Badaowan Formation is the most important source rock in this area, with the best hydrocarbon generation conditions, followed by the Middle Jurassic Xishanyao Formation. However, the hydrocarbon generation conditions of the Lower Jurassic Sangonghe Formation are relatively poor. Therefore, the hydrocarbon-generating capacity of the coal-measure mudstone is better than that of the coal rock and carbonaceous mudstone. However, due to the high abundance of organic matter, the coal rocks and carbonaceous mudstones have great potential for hydrocarbon generation, and their contribution to oil and gas generation cannot be ignored (Guo et al., 2015; Wang et al., 2015; Yang et al., 2016; Li et al., 2020; Li, 2022).
3.2.2 Elemental composition of C, H, and O in kerogen
Figure 3 is the correlation diagram of H/C and O/C atomic ratios of various source rocks in the Jurassic Xishanyao, Sangonghe, and Badaowan Formations in the Bohu Depression. It reflects that most of the Jurassic source rocks in the Bohu Depression have a H/C atomic ratio of less than 0.8 and an O/C atomic ratio of less than 0.15, indicating that they are Type III parent materials. Only a few source rocks have H/C atomic ratios greater than 0.8, which belong to Type II2 parent materials. By comparison, the Badaowan Formation source rocks are of the best quality, which may be related to the relatively high degree of the thermal evolution of the Badaowan Formation source rocks (Wang et al., 2015; Li et al., 2021).
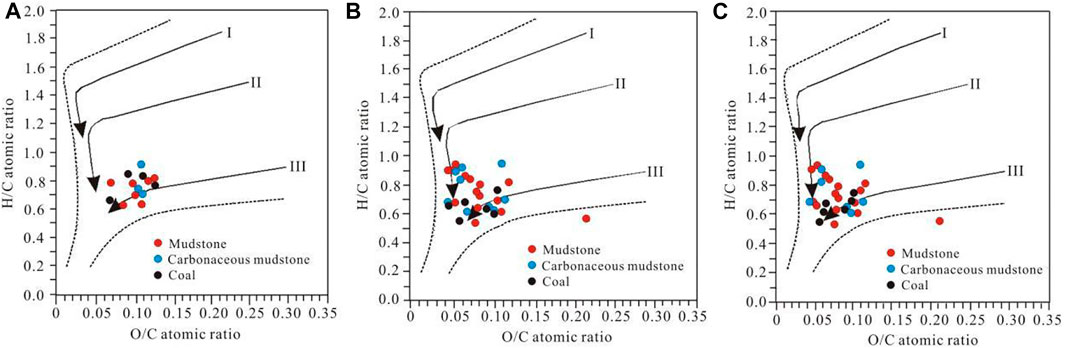
FIGURE 3. Relationship between H/C and O/C atomic ratios of Jurassic source rocks in the study area. Notes: (A) Xishanyao Formation; (B) Sangonghe Formation; (C) Badaowan Formation.
3.2.3 Distribution characteristics of hydrogen and oxygen indexes
The Jurassic mudstone in the study area has a wide distribution range of hydrogen and oxygen indices, with the hydrogen index ranging from 2 to 266 mg/gTOC and the oxygen index ranging from 3 to 183 mg/gTOC (Figure 4). The degradation rate is less than 30%, which reflects that the type of organic matter in the mudstone belongs to Type II2–III. The hydrogen index of coal is distributed in the range of 100–300 mg/gTOC, which is significantly higher than that of mudstone.
According to the abovementioned analysis, the organic matter type of the Jurassic source rocks in the Yanqi Basin is mainly Type III, followed by Type II2. The quality of Jurassic source rocks from high to low is the Badaowan Formation, the Xishanyao Formation, and the Sangonghe Formation. In the same layer, the organic matter type of coal rock and carbonaceous mudstone is slightly better than that of the coal measure mudstone.
3.3 Carbon isotopic characteristics of crude oil
The stable carbon isotopic composition of crude oil is related to the type of parent material, so it can reflect the genetic type of crude oil. Generally, the δ13C of crude oil related to sapropelic parent material is lighter than −27‰, the δ13C of saturated hydrocarbons is lighter than −28.5‰, and the δ13C of aromatic hydrocarbons is lighter than −26.5‰. However, the δ13C of crude oil related to parent material is generally heavier than −27.0‰, and the δ13C of saturated hydrocarbons is heavier than −28.5‰. In this study, only the δ13C value in the crude oil from Well Bonan1 is lighter than −27‰, while that in the other crude oil samples are all heavier than −27.0‰. It reflects the characteristics that the crude oil in this area is dominated by higher plants and rich in heavy carbon isotopes (Loucks et al., 2009; Xiong et al., 2017; He et al., 2020).
3.3.1 Carbon isotopic characteristics of crude oil monomer hydrocarbons
Figure 5 shows the molecular isotopic distribution of 11 crude oils from the Middle and Lower Jurassic in the Yanqi Basin. It can be seen that the molecular isotope of C12–C33 gradually becomes lighter with the increase of carbon number, and the δ13C decreases from −20‰–23‰ to −27‰–34‰. Among them, the molecular isotope of one crude oil showed a slight difference in C30, but the carbon isotope of the other molecules showed almost the same trend of decreasing isotopic mass with the increase of carbon number. The variation trend of the molecular carbon isotope of the crude oil further reveals a similar composition from the perspective of molecular composition (Lu et al., 2015; Wang et al., 2018; Yu et al., 2019; Li et al., 2022).
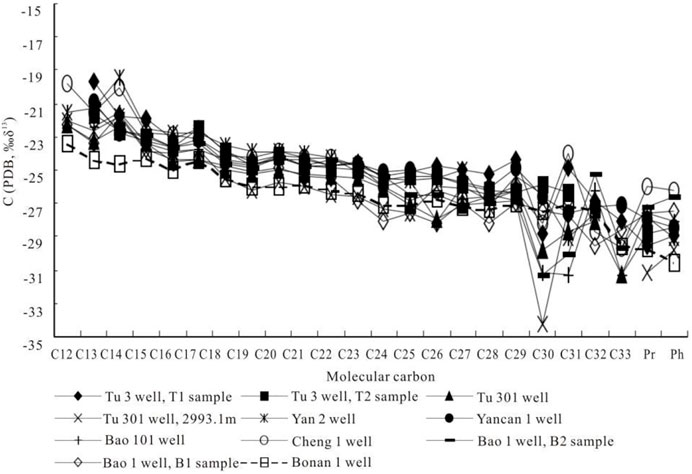
FIGURE 5. Distribution characteristics of carbon isotopes in the Lower Jurassic crude oil molecules in the study area.
3.3.2 Distribution characteristics of carbon isotopes
Figure 6 is the molecular carbon isotope distribution of the possible source rocks (coal-measure mudstone, carbonaceous mudstone, and coal) in this area. The molecular carbon isotope distributions of the Triassic mudstones from Well Baonan1 are somewhat different from those of most samples, while the molecular carbon isotope distributions of most possible source rocks are basically the same. This fact indicates that the abovementioned possible source rocks share common original parental precursors (higher plants) and also reflects that they have common characteristics in carbon isotope fractionation and inheritance effects (Yang et al., 2010; Xu et al., 2019; Zou et al., 2019). Therefore, from the perspective of molecular isotope distribution, coal-measure mudstone, carbonaceous mudstone, and coal in this area have common source rocks for crude oil.
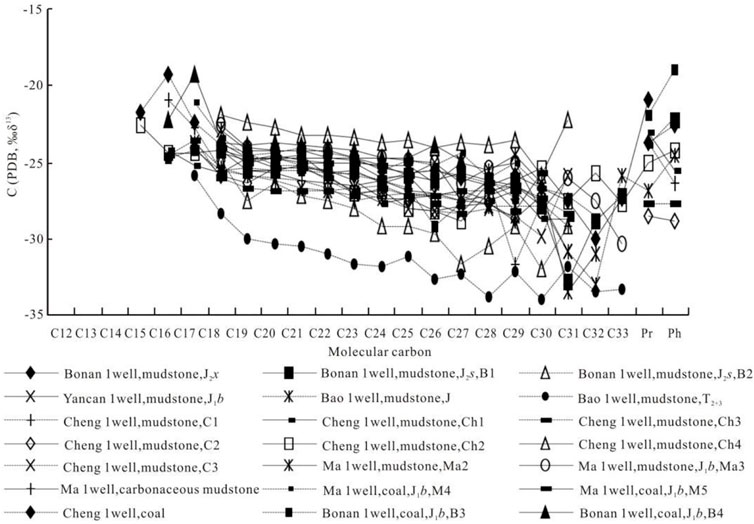
FIGURE 6. Distribution characteristics of molecular carbon isotopes in the J-T source rocks in the study area.
3.4 Biomarker composition characteristics of crude oil and various source rocks
3.4.1 Crude oil biomarkers
The tricyclic terpenes in the crude oil of the target layer are not developed, the pentacyclic triterpenes are generally Tm>Ts, and the 17α(H)-rearranged hopane is relatively developed, but the gammacerane is not developed. The sterane composition is generally dominated by C29 stigmasterane, and C29 sterane >> the sum of C27 sterane and C28 sterane, and rearranged sterane is not developed.
3.4.2 Biomarkers of coal-measure mudstone
The biological characteristics of the Middle–Lower Jurassic coal-measure mudstone in this area are relatively complex. The coal-measure mudstone biomarker is represented by Well Yancan1 (3,248.5 m, Badaowan Formation), and its main features are as follows: Tm>>Ts, gammacerane is extremely undeveloped. Both C29 nornehopanes (C29Ts) and C30 rearranged hopanes are less developed, and C29 norhopanes are relatively developed. Due to the development of C29 normotane in this type of rock, its corresponding normotane is also relatively developed, which is also one of the biomarker characteristics of this type of coal-measure mudstone.
3.4.3 Biomarkers of carbonaceous mudstone
There are two biological characteristics of carbonaceous mudstone. One is that the C29 norhopane is larger than the C30 hopane, and the corresponding C29 hopane is also relatively developed. When Tm>>Ts, the gamma pole is not developed, reflecting the characteristics of freshwater deposition. Another characteristic is as follows: when C30 hopane>C29 norhopane, the corresponding C29 nornehopane (C29Ts), and C30 rearranged hopane are relatively developed, and gammacerane is still very low.
3.4.4 Biomarkers of coal
The biomarker characteristics of coal are basically the same as those of carbonaceous mudstone, and there are also two types: one is C30 hopane>C29 hopane, Tm>>Ts, gammacerane is extremely undeveloped, such as the 2767 m (Badaowan Formation) coal of the Well Bonan 1; the other is C29 norhopane>C30 hopane, Tm>>Ts, gammacerane is also very underdeveloped, such as the coal of 2392 m (Badaowan Formation) of the Well Cheng 1.
4 Discussion
4.1 Liquid hydrocarbon yields for thermal-pressure simulations
The thermal evolution of hydrocarbon generation is different for different types of kerogens (Dong et al., 2015). According to the previous research results, when the kerogen type is Type I, the organic matter is gnawed on the edge or inside, and the surface of the pores is smooth. When the volume of kerogen shrinks, then the marginal pores of organic matter will form. They belong to the shrinkage type (Figure 7A). When the kerogen is Type III, the total volume of the organic matter is basically unchanged. The interior of the samples is dominated by the formation of internal pores of organic matter (porous type) (Figure 7B). When the kerogen is Type II, the morphological evolution characteristics of organic matter are between Types I and III, and both marginal pores and internal pores of organic matter are developed. With the increase of the simulated temperature, the organic matter edge pores and the organic matter internal pores continue to increase (Figure 7C). The organic matter pores generated by the kerogen pyrolysis hydrocarbon generation are one of the main reasons for the increase in the internal storage space of mudstone (Cai et al., 2008).
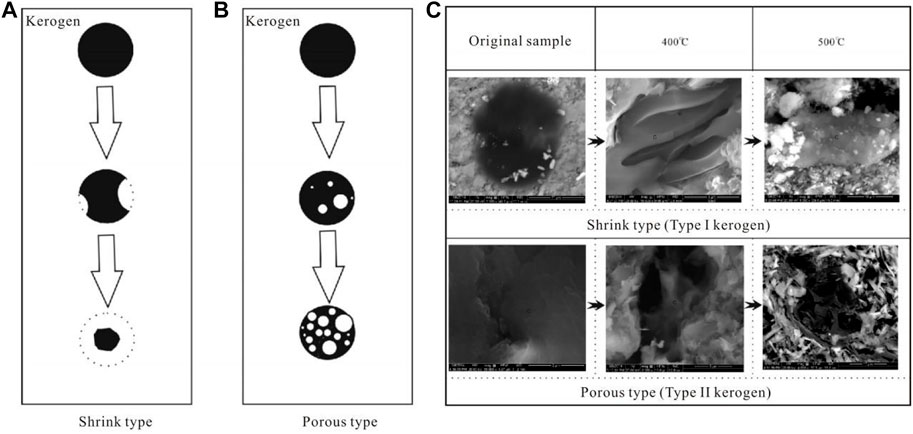
FIGURE 7. Evolution of organic matter morphology and organic matter pores (modified after Dong et al., 2015). Notes: (A) shrinkable organic matter; (B) porous organic matter; (C) thermal evolution mode of organic pores.
4.1.1 Liquid hydrocarbon yield of coal-measure mudstone
The thermal-pressure simulation results of coal-measure mudstone in the Yanqi Basin show that the peak of liquid hydrocarbon generation occurs in the simulated temperature range of 300–340°C, and the Ro value is 0.89–1.22%. It is close to the peak of hydrocarbon generation in the natural evolution of organic matter, indicating that the simulation conditions of low temperature, water content, and long-term thermal pressure are close to the actual underground conditions (Guo et al., 2012). The peak value of chloroform bitumen “A" in the residue appears at the simulated temperature of 210–240°C, and the Ro value is 0.72%–0.78% at this time, which also indicates that there are some early hydrocarbon-generating substances in this type of source rocks. The appearance of the peak value indicates the existence of some early hydrocarbon-generating substances. These substances include resin and matrix vitrinite in the Jurassic coal-measure mudstone with higher plants as the main organic parent material.
The highest liquid hydrocarbon production rate of the dark gray mudstone of the Badaowan Formation in Well Ma1 is 20.4 mg/gTOC, and the maximum liquid hydrocarbon production rate of dark gray mudstone of the Xishanyao Formation in Well Bonan 1 is 16.4 mg/gTOC. Compared with lacustrine mudstone, the liquid hydrocarbon yield in coal measure is not high. However, due to the high abundance of organic matter in coal-measure mudstone, its liquid hydrocarbon production rate is still considerable. On the other hand, it shows that the production rate of liquid hydrocarbons in the source rocks of the Xishanyao Formation in this area is generally lower than that of the mudstones of the Badaowan Formation (Figure 8).
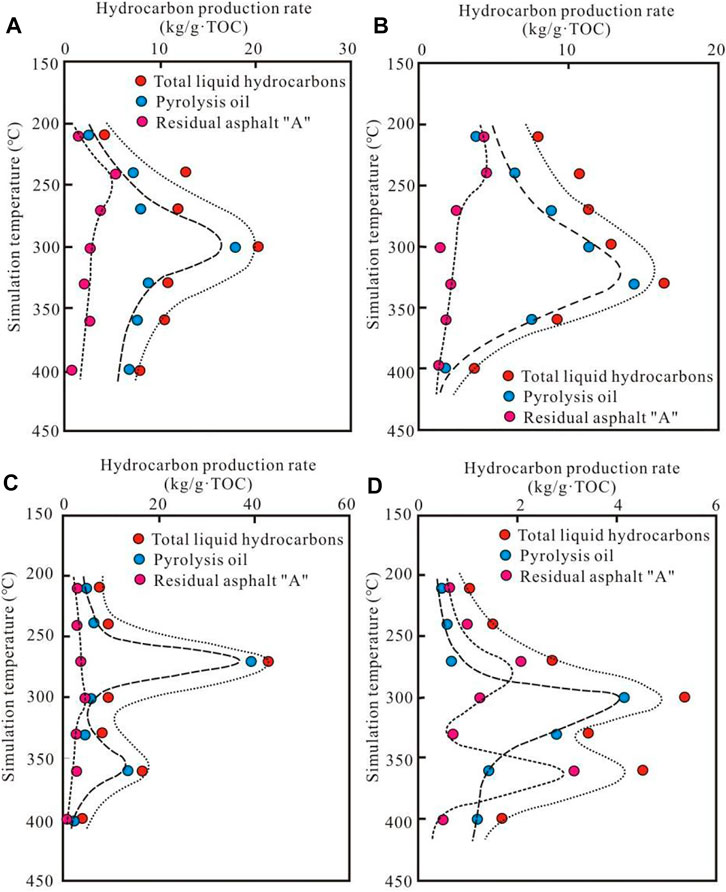
FIGURE 8. Yields of liquid hydrocarbons in thermal pressure simulations of coal rocks, carbonaceous mudstones, and coal-measure mudstones in the Middle and Lower Jurassic in the study area. Notes: (A) Well Ma 1, dark gray mudstone; (B) Well Yannan 1, dark gray mudstone; (C) Hua’an Coal Mine, coal; (D) Well Yan 2, carbonaceous mudstone.
4.1.2 Liquid hydrocarbon yield of carbonaceous mudstone
For the thermo-pressure simulations of carbonaceous mudstone, both total liquid hydrocarbons and residual chloroform “A” showed a distinct bimodal distribution, that is, there are two peaks of hydrocarbon generation between 240 and 300°C and 360°C, the Ro value of the former is 0.8%–0.9%, and the Ro value of the latter is 1.31%. It reflects that the parental composition of the source rock is relatively complex. However, from the change of the pyrolysis oil yield curve, the main peak is still at 300°C, and the Ro value is 0.9%. In the high evolution stage (Ro>1.3%) of carbonaceous mudstone, the residual bitumen “A” and total hydrocarbons still show a peak of hydrocarbon generation, which may be closely related to the adsorption of hydrocarbons by carbonaceous mudstone.
Although the change of the liquid hydrocarbon production curve of carbonaceous mudstone is complicated, the highest total liquid hydrocarbon production rate is only 5.36 mg/gTOC, which is much lower than that of mudstone and coal in this area. It shows that although the carbonaceous mudstone in this area has a high abundance of organic matter, its hydrocarbon generation rate is not high due to the poor type of parent material (Figure 8).
4.1.3 Liquid hydrocarbon yield of coal rock
The residual chloroform bitumen “A” and the total liquid hydrocarbon yield of the coal rock have bimodal distribution characteristics. The two peaks are located in 270°C and 360°C, respectively, and the corresponding Ro values are 0.68% and 1.70%, respectively. Obviously, the appearance of the peak of liquid hydrocarbons at 270°C is the contribution of early hydrocarbon-generating substances such as resin body, suberite, and matrix vitrinite in coal rock. The appearance of the peak of liquid hydrocarbon generation at 360°C may be due to the release of the adsorbed and encapsulated parts in the coal rock under high temperature conditions.
The yield of coal and rock pyrolysis oil is generally 2–3 mg/gTOC. The highest value appears at the temperature step of 300°C. At this time, the Ro value was 0.8%, and the pyrolysis oil yield was 5.2 mg/gTOC. It shows that the hydrocarbon generation potential of coal is equivalent to that of carbonaceous mudstone only by the yield of pyrolysis oil (Jiang et al., 2015).
It is worth mentioning that the total liquid hydrocarbon yield of the Xishanyao Formation coal rock in the Hua’an Coal Mine is as high as 43.2 mg/gTOC, which is double the total liquid hydrocarbon yield of the general Middle–Lower Jurassic coal measure mudstone in the Yanqi Basin. Moreover, the content of residual chloroform bitumen “A” in coal rock is also as high as 3%, which is close to the hydrocarbon expulsion threshold of coal. The total liquid hydrocarbon production rate of coal rocks further supports that the Middle and Lower Jurassic coal rocks in this area can generate a certain quantity of liquid hydrocarbons, and some of them have approached or reached the threshold hydrocarbon concentration for coal rocks to expel liquid hydrocarbons (Figure 8).
The comparison results of the total liquid hydrocarbon production rate show that the Jurassic coal rock in the Yanqi Basin has the highest rate (43.2 mg/gTOC), followed by mudstone (16.4–20.4 mg/gTOC), and the carbonaceous mudstone has the lowest (the maximum liquid hydrocarbon production rate is only 5.36 mg/g TOC).
4.2 Gaseous hydrocarbon yield from thermal-pressure simulations
Figure 9 shows the gaseous hydrocarbon thermo-pressure simulation results of the four samples conducted in this study.
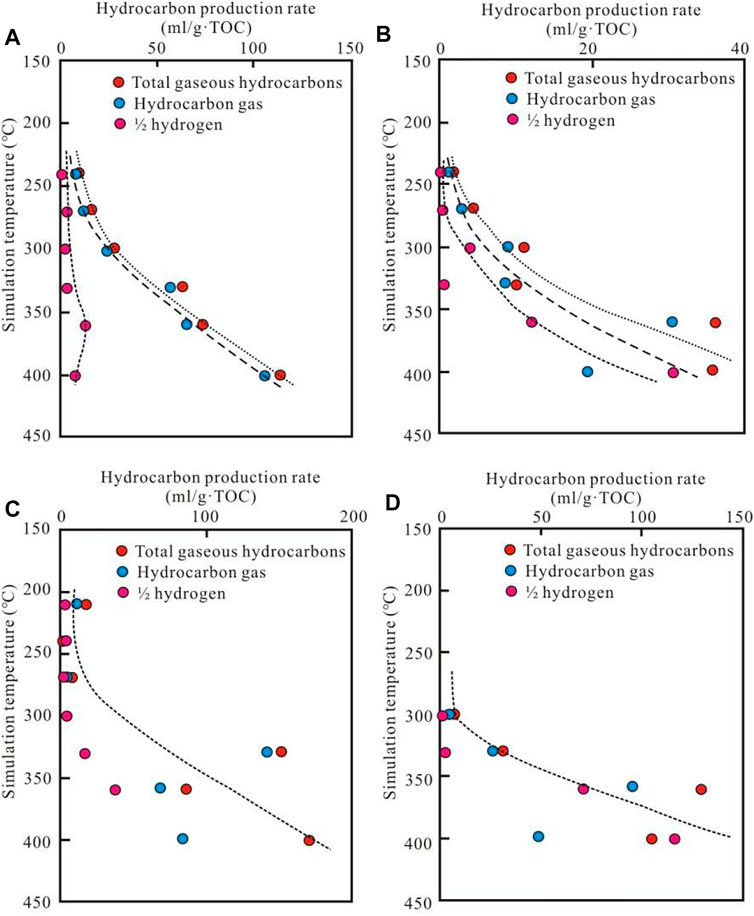
FIGURE 9. Test results of gaseous hydrocarbon yields from thermal pressure simulations of coal rocks, carbonaceous mudstones, and coal-measure mudstones in the study area in the Middle and Lower Jurassic. Notes: (A) Hua’an Coal Mine, coal; (B) Well Yan 2, carbonaceous mudstone; (C) Well Ma 1, dark gray mudstone; (D) Well Yannan 1, dark gray mudstone.
4.2.1 Gaseous hydrocarbon yield of coal rock
In Figure 9, the gaseous hydrocarbon yield chart of the coal rock of the Hua’an Coal Mine consists of three curves. One is the change in the yield of hydrocarbon gas with the increase of thermal maturity during the thermal evolution of organic matter. The other is that during the thermal evolution of organic matter, some free hydrogen can be generated due to thermal degradation of kerogen or thermal cracking of heavy hydrocarbons, condensation of aromatic hydrocarbons, or cyclization of paraffins. Since this part of hydrogen comes from organic matter, this study divides its volume by 2 and converts it into the hydrogen fraction of methane and calculates it as hydrocarbons. The third part is the sum of hydrocarbon gases and hydrogen.
The general trend of the change of gaseous hydrocarbon yield in the coal seam of the Hua’an Coal Mine is that it increases with the simulated temperature recovery. The highest gaseous yield in the high-maturity stage is 110.9 ml/g.TOC.
4.2.2 Gaseous hydrocarbon yield of carbonaceous mudstone
In Figure 9, the thermal pressure simulation gaseous hydrocarbon yield of the carbonaceous mudstone in the Sangonghe Formation in Well Yan2 shows that the gaseous hydrocarbon yield of the carbonaceous mudstone in this area is only 36.8 ml/gTOC in the wet gas-condensate stage (Ro is 1.31%). Its gas production is not high.
4.2.3 Gaseous hydrocarbon yield of coal measure mudstone
In Figure 9, the dark gray mudstone of the Badaowan Formation in Well Ma 1 has a gaseous hydrocarbon production rate of 171.6 ml/gTOC when Ro is 1.37%. Also, the dark gray mudstone of the Xishanyao Formation in Well Baoan1 has the highest gaseous hydrocarbon yield of 130.9 ml/gTOC when Ro reaches 1.42%.
In conclusion, the gaseous hydrocarbon yield per unit of organic carbon in source rocks is highest in mudstone, followed by coal rock, and lowest in carbonaceous mudstone.
4.3 Oil source comparison and analysis
Based on the comprehensive analysis of the carbon isotope, molecular carbon isotope, and biomarker characteristics of crude oil and various source rocks, this study focused on a direct oil/rock comparison using biomarkers such as steranes and terpenes.
4.3.1 Triangular diagram of C27, C28, and C29 regular sterane compositions
Figure 10 is a triangular diagram of regular sterane compositions of crude oil and various possible source rocks in this area. It can be seen from Figure 10 that in the affinity circle with crude oil as the core, there are coal-measure mudstone, carbonaceous mudstone, and coal together to form the affinity circle. There is also a considerable amount of mudstone, carbonaceous mudstone, and coal outside this phylogenetic circle. This result indicates that the Middle and Lower Jurassic crude oil series in the Yanqi Basin come from mudstone, carbonaceous mudstone, and coal in this area. The Middle and Lower Jurassic crude oil is not a single oil source but a mixed source oil of the abovementioned three lithologies. It should be noted that, according to the comparison results of the regular sterane composition triangle, coal-measure mudstone, carbonaceous mudstone, and coal all contribute to the source of oil and gas in this area. However, this does not mean that all coal-measure mudstone, carbonaceous mudstone, and coal are source rocks in this area. They are effective source rocks only when they reach the source rock standard in terms of organic matter abundance, hydrocarbon-forming parent material type, and thermal evolution degree.
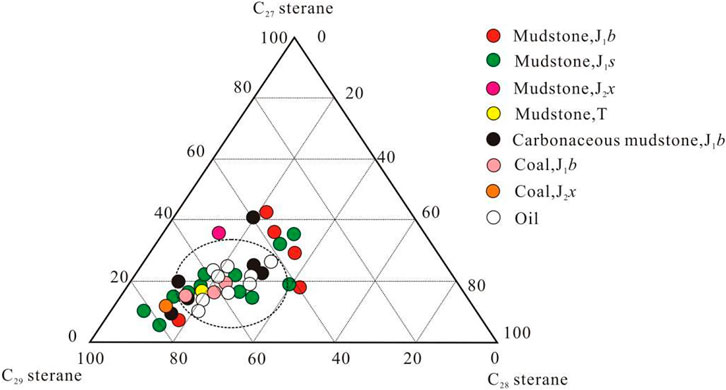
FIGURE 10. Triangular diagram of the composition of crude oil and related steranes in the Middle and Lower Jurassic in the study area.
4.3.2 Relationship between sterane C29αββ/ααα+αββ and sterane C2920S/20S+20R
It is generally believed that sterane C29αββ/ααα+αββ should increase in a linear relationship with sterane C2920S/20S+20R. In the case of self-generation and self-storage reservoirs, the abovementioned parameter distribution of oil/rock should follow a linear relationship. Conversely, in the case of secondary migration reservoirs, the abovementioned parameters do not follow a linear relationship but are far from the range of a linear relationship. From Figure 11, it can be seen that the Middle and Lower Jurassic reservoirs in this area should be self-generating and self-storing oil reservoirs. Also, it also shows that some coal-measure mudstone, carbonaceous mudstone, and coal should be its possible source rocks because, in the vicinity of crude oil, some coal-measure mudstone, carbonaceous mudstone, and coal together form a small-diameter affinity circle. From the perspective of similarity comparison, some of these rocks should be the source rocks in this area.
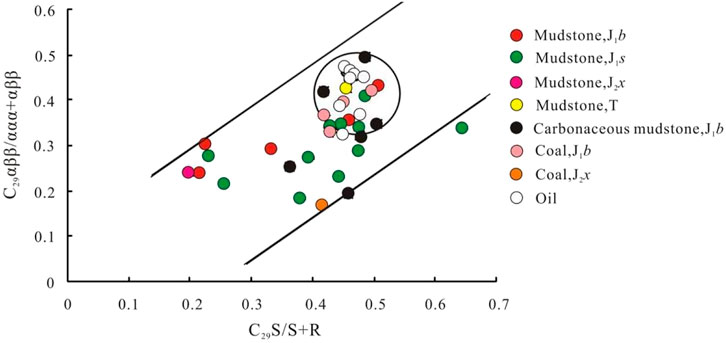
FIGURE 11. Relationship between crude oil and related sterane isomerization parameters in the Middle and Lower Jurassic in the study area.
4.3.3 Comparison of oil sources of terpanes
Figure 12 shows the relationship between gammacerane content and C29 norhopane content in representative crude oil and related coal-measure mudstone, carbonaceous mudstone, and coal in this area. The crude oil in the target layer is basically distributed in the range of C29 norhopane/C30 hopane<1.0 and Gammacerane/C30 hopane<0.2. It forms a small-diameter affinity circle with some coal-measure mudstone, carbonaceous mudstone, and coal in this area. Various kinds of rock in the circle are very likely to be the main source rocks in this area.
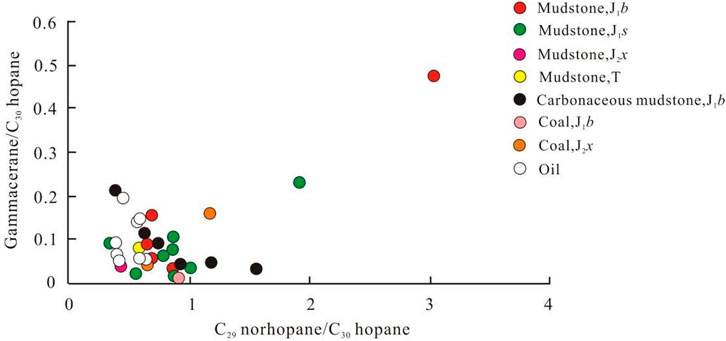
FIGURE 12. Relationship between gammacerane/C30 hopane and C29 norhopane/C30 hopane in Middle and Lower Jurassic crude oil and source rocks in the study area.
Overall, according to the molecular carbon isotopic characteristics of crude oil, the Jurassic crude oil in the coal measure of the study area has a certain affinity with its corresponding source rocks (mudstone, carbonaceous mudstone, and coal rock). At the same time, according to the comparison results of steroid and terpene biomarkers and crude oil, the Middle and Lower Jurassic mudstone, carbonaceous mudstone, and coal rock in this area are all source rocks. There is no doubt that the Badaowan Formation mudstone is the main source rock.
5 Conclusion
1) The micro-composition of the Jurassic coal rocks in the Yanqi Basin shows the characteristics of rich vitrinite–inertinite and poor chitinite + saprolite; however, the micro-composition of the mudstone shows the characteristics of poor inertinite and rich vitrinite–chitinite + saprolite.
2) The Jurassic mudstone has a wide distribution range of hydrogen and oxygen indexes, among which the hydrogen index is distributed between 2 and 266 mg/gTOC and the oxygen index is between 3 and 183 mg/gTOC. The organic matter in the mudstone belongs to Type II2–III kerogen.
3) The hydrogen index of coal is distributed in the range of 100–300 mg/gTOC, which is obviously larger than that of mudstone. The total liquid hydrocarbon yield of the coal rock is the highest (43.2 mg/gTOC), followed by mudstone (16.4–20.4 mg/gTOC), and carbonaceous mudstone is the lowest (5.36 mg/gTOC). In addition, mudstone has the highest yield of gaseous hydrocarbons, followed by coal, while carbonaceous mudstone is the lowest.
4) The oil-source comparison shows that the Jurassic crude oil has a certain affinity with its main source rocks (mudstone, carbonaceous mudstone, and coal). At the same time, according to the comparison results of steroid and terpene biomarker parameters with crude oil, it shows that the Middle and Lower Jurassic mudstone, carbonaceous mudstone, and coal rock in this area are all source rocks and the Badaowan Formation mudstone is the main source rock.
Data availability statement
The original contributions presented in the study are included in the article/Supplementary Material; further inquiries can be directed to the corresponding authors.
Author contributions
YY is responsible for the idea of this paper. FZ, JZ, and JZ are responsible for the experiments.
Funding
This research was funded by the Natural Science Foundation of Xinjiang Uygur Autonomous Region—Surface Project (2019D01A34) “Accumulation Mechanism, Occurrence Characteristics and Basin Dynamics Environment of Shale (Stratification) Gas Source-Reservoir Complex”.
Conflict of interest
The authors declare that the research was conducted in the absence of any commercial or financial relationships that could be construed as a potential conflict of interest.
Publisher’s note
All claims expressed in this article are solely those of the authors and do not necessarily represent those of their affiliated organizations, or those of the publisher, the editors, and the reviewers. Any product that may be evaluated in this article, or claim that may be made by its manufacturer, is not guaranteed or endorsed by the publisher.
References
Cai, J., Wang, H., and Zhao, Z. (2008). Formation process and dynamic mechanism of Bohu depression in Yanqi Basin. Earth Sci. J. China Univ. Geosciences 33 (4), 555–563.
Curtis, M., Cardott, B., Sondergeld, C., and Rai, C. (2012). Development of organic porosity in the Woodford Shale with increasing thermal maturity. Int. J. Coal Geol. 103, 26–31. doi:10.1016/j.coal.2012.08.004
Dong, C., Ma, C., Luo, G., Lin, C., Zhang, X., and Ren, L. (2015). Late pyrolysis simulation experiment and diagenesis evolution pattern of shale. ACTA SEDIMENTOL. SIN. 33 (5), 1053–1061. doi:10.14027/j.cnki.cjxb.2015.05021
Gai, S., Liu, H., He, S., Mo, S., Chen, S., Liu, R., et al. (2016). Shale reservoir characteristics and exploration potential in the target: A case study in the longmaxi formation from the southern sichuan basin of China. J. Nat. Gas Sci. Eng. 31, 86–97. doi:10.1016/j.jngse.2016.02.060
Guo, J., Pang, X., and Liu, D. (2012). In the middle and lower Jurassic in Kuche depression, Jurassic coal measure hydrocarbon expulsion characteristics and resource potential evaluation. Nat. Gas. Geosci. 23 (2), 327–334.
Guo, W., Liu, H., Xue, H., Lan, C., and Tang, X. (2015). Depositional facies of permian Shanxi formation gas shale in the Northern Ordos Basin and its impact on shale reservoir. Acta Geol. Sin. 89 (5), 931–941.
He, D., Li, D., and He, J. (2013). Petroleum geology and exploration enlightenment analogy of kuche depression in Tarim basin and the southwest Tarim depression. Acta Pet. sin. 34 (2), 201–218.
He, X., Zhang, P., He, G., Gao, Y., Liu, M., Zhang, Y., et al. (2020). Evaluation of sweet spots and horizontal-well-design technology for shale gas in the basin-margin transition zone of southeastern Chongqing, SW China. Energy Geosci. 1 (3–4), 134–146. doi:10.1016/j.engeos.2020.06.004
Jiang, S., Xu, Z., Feng, Y., Zhang, J., Cai, D., Chen, L., et al. (2015). Geologic characteristics of hydrocarbon-bearing marine, transitional and lacustrine shales in China. J. Asian Earth Sci. 115, 404–418. doi:10.1016/j.jseaes.2015.10.016
Li, D. (2000). The progress in the petroleum geology of China towards new century. Acta Pet. sin. 21 (2), 1–8.
Li, H. (2022). Research progress on evaluation methods and factors influencing shale brittleness: A review. Energy Rep. 8, 4344–4358. doi:10.1016/j.egyr.2022.03.120
Li, H., Qin, Q. R., Zhang, B. J., Ge, X. Y., Hu, X., Fan, C. H., et al. (2020). Tectonic fracture formation and distribution in ultradeep marine carbonate gas reservoirs: A case study of the maokou Formation in the jiulongshan gas field, sichuan basin, southwest China. Energy fuels. 34 (11), 14132–14146. doi:10.1021/acs.energyfuels.0c03327
Li, H., Tang, H. M., Qin, Q. R., Zhou, J. L., Qin, Z. J., Fan, C. H., et al. (2019). Characteristics, formation periods and genetic mechanisms of tectonic fractures in the tight gas sandstones reservoir: A case study of xujiahe Formation in YB area, sichuan basin, China. J. Petroleum Sci. Eng. 178, 723–735. doi:10.1016/j.petrol.2019.04.007
Li, H., Wang, Q., Qin, Q. R., and Ge, X. Y. (2021). Characteristics of natural fractures in an ultradeep marine carbonate gas reservoir and their impact on the reservoir: A case study of the maokou formation of the JLS structure in the sichuan basin, China. Energy fuels. 35 (16), 13098–13108. doi:10.1021/acs.energyfuels.1c01581
Li, J., Li, H., Yang, C., Wu, Y. J., Gao, Z., and Jiang, S. L. (2022). Geological characteristics and controlling factors of deep shale gas enrichment of the Wufeng-Longmaxi Formation in the southern Sichuan Basin, China. Lithosphere 2022, 4737801. doi:10.2113/1970/4737801
Lin, A., Fu, B., Kano, K., Maruyama, T., and Guo, J. (2002). Late Quaternary right-lateral displacement along active faults in the Yanqi Basin, southeastern Tian Shan, northwest China. Tectonophysics 354, 157–178. doi:10.1016/s0040-1951(02)00288-3
Loucks, R., Reed, R., Ruppel, S., and Jarvie, D. (2009). Morphology, genesis, and distribution of nanometer-scale pores in siliceous mudstones of the mississippian barnett shale. J. Sediment. Res. 79, 848–861. doi:10.2110/jsr.2009.092
Lu, J., Ruppel, S. C., and Rowe, H. D. (2015). Organic matter pores and oil generation in the Tuscaloosa marine shale. Am. Assoc. Pet. Geol. Bull. 99, 333–357. doi:10.1306/08201414055
Wang, G., Ju, Y., Yan, Z., and Li, Q. (2015). Pore structure characteristics of coal–bearing shale using fluid invasion methods: A case study in the huainan–huaibei coalfield in China. Mar. Petroleum Geol. 62, 1–13. doi:10.1016/j.marpetgeo.2015.01.001
Wang, P., Chen, Z., Jin, Z., Jiang, C., Jia, Z., Guo, Y., et al. (2018). Shale oil and gas resources in organic pores of the Devonian Duvernay Shale , Western Canada Sedimentary Basin based on petroleum system modeling. J. Nat. Gas. Sci. Eng. 50, 33–42. doi:10.1016/j.jngse.2017.10.027
Xiong, F., Jiang, Z., Li, P., Wang, X., Bi, H., Li, Y., et al. (2017). Pore structure of transitional shales in the Ordos Basin, NW China: Effects of composition on gas storage capacity. Fuel 206, 504–515. doi:10.1016/j.fuel.2017.05.083
Xu, H., Zhou, W., Zhang, R., Liu, S., and Zhou, Q. (2019). Characterizations of pore, mineral and petrographic properties of marine shale using multiple techniques and their implications on gas storage capability for Sichuan Longmaxi gas shale field in China. Fuel 241, 360–371. doi:10.1016/j.fuel.2018.12.035
Yang, C., Zhang, J., Tang, X., Ding, J., Zhao, Q., Dang, W., et al. (2016). Comparative study on micro-pore structure of marine, terrestrial, and transitional shales in key areas, China. Int. J. Coal Geol. 171, 76–92. doi:10.1016/j.coal.2016.12.001
Yang, H., Li, S. X., and Liu, X. Y. (2010). Characteristics and resource prospects of tight oil and shale oil in Ordos Basin. Acta Pet. Sin. 34 (1), 1–11.
Yao, Y., Liu, C., and Zhao, Z. (2003). Tectonic evolution and hydrocarbon accumulation in Yanqi Basin. Petroleum Geol. Xinjiang 24 (2), 115–117.
Yao, Y., Zhang, Y., and Fu, D. (2004). Relationship between the formation of paleo geotemperature and oil and gas in Bohu depression of Yanqi Basin. Petroleum Explor. Dev. 31 (1), 24–27.
Yu, K., Shao, C., Ju, Y., and Qu, Z. (2019). The Genesis and controlling factors of micropore volume in transitional coal-bearing shale reservoirs under different sedimentary environments. Mar. Petroleum Geol. 102, 426–438. doi:10.1016/j.marpetgeo.2019.01.003
Zhang, H., Wang, R., and Jin, B. (2011). Jurassic hydrocarbon accumulation model in Bohu depression, Yanqi Basin, Xinjiang. Petroleum Geol. Xinjiang 32 (6), 613–615.
Zhao, W., Hu, S., Wang, H., Bian, C., Wang, Z., and Wang, Z. (2013). Large-scale accumulation and distribution of medium-low abundance hydrocarbon resources in China. Petroleum Explor. Dev. 40 (1), 1–14. doi:10.1016/s1876-3804(13)60001-4
Keywords: Yanqi Basin, urassic source rock, hydrocarbon generation potential, organic carbon, oil-source correlation.
Citation: Yao Y, Zhao F, Zhang J and Zhao J (2022) Evaluation of organic geochemical characteristics and experiments of thermal pressure simulation of Jurassic coal measure source rocks in the Yanqi Basin. Front. Earth Sci. 10:992013. doi: 10.3389/feart.2022.992013
Received: 12 July 2022; Accepted: 01 August 2022;
Published: 31 August 2022.
Edited by:
Hu Li, Southwest Petroleum University, ChinaReviewed by:
Shun He, Southwest Petroleum University, ChinaShaoke Feng, Chengdu University of Technology, China
Copyright © 2022 Yao, Zhao, Zhang and Zhao. This is an open-access article distributed under the terms of the Creative Commons Attribution License (CC BY). The use, distribution or reproduction in other forums is permitted, provided the original author(s) and the copyright owner(s) are credited and that the original publication in this journal is cited, in accordance with accepted academic practice. No use, distribution or reproduction is permitted which does not comply with these terms.
*Correspondence: Yaming Yao, yaoyaming321@163.com