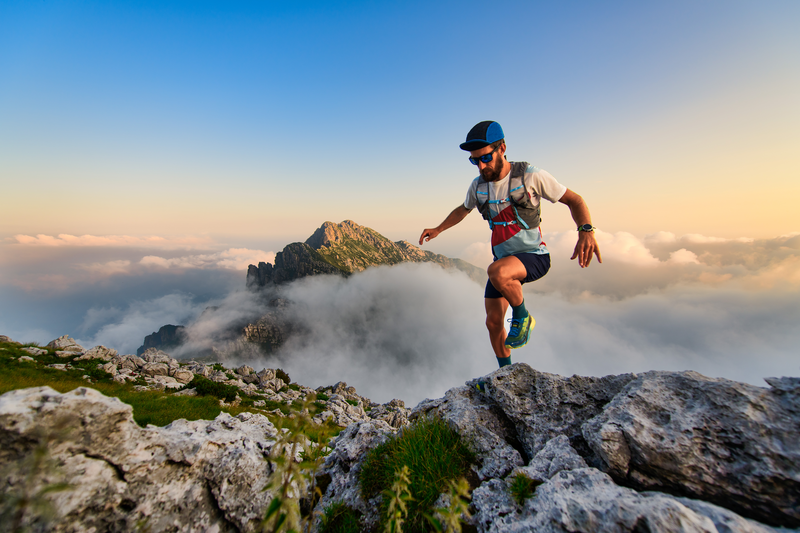
94% of researchers rate our articles as excellent or good
Learn more about the work of our research integrity team to safeguard the quality of each article we publish.
Find out more
REVIEW article
Front. Earth Sci. , 08 September 2022
Sec. Interdisciplinary Climate Studies
Volume 10 - 2022 | https://doi.org/10.3389/feart.2022.988710
This article is part of the Research Topic Climate Change and Associated Impacts View all 16 articles
Determining how climate change affects aquatic organisms, such as fish is vital, since this could directly or indirectly impact food and protein sources that are important for human nutrition. Thus, identifying suitable organisms for studying the impacts of climate change on aquatic species is essential. It is most effective to select model organisms for climate change study and determine how each organism might adapt within the diversity of organisms present. This study aimed to review the current development and frontiers of climate change’s model organism based on the literature. We conducted a scientometric analysis by differentiating between publications on different model species, the number and origin of authors and affiliations involved, the citation analysis, and the most common keywords used. Increased publication numbers for Zebrafish and Medaka were detected during the analysis of the networks. Our results showed that both species are among the most important aquatic model organisms for climate change related research. Furthermore, we found that these model organisms, especially the Zebrafish are becoming increasingly important towards climate change related studies, because of their simple anatomy and established biological studies. Our analysis could be on the forefront for disseminating and communicating scientific knowledge and impactful discoveries to researchers, academics, policymakers, and to the public worldwide for future contribution to the community resources preservation.
Fish are vital sources for human protein and are a basic necessity for a large percentage of the world, especially who lived in the coastal area (Braña et al., 2021; Mamun et al., 2021; Maulu et al., 2021). The future sustainability of aquatic products, such as seafood, is partly related to the human response to climate change. For example, sea surface temperature is changing the distribution of fish stock (Sunday et al., 2012), thus altering where fish can sustainably be caught (Free et al., 2022). Due to the diversity of aquatic organisms, it is more effective to select a few aquatic organisms as model species, and assess how they might adapt to climate change rather than trying to assess many species at once The concept of model organism research started in the early 1960s and 1970s due to the increasing number of techniques available in the molecular biological field (Dietrich et al., 2014). Research organisms or model organisms are also frequently used for laboratory research for specific characterization experiments. This type of research helps to inform on the fundamental mechanisms in other organisms and their behaviour. Some examples of classic model species in biology include mice and chickens (Müller and Grossniklaus, 2010; Tregaskes and Kaufman, 2021); Zebrafish and Medaka can also be considered as model organisms, especially among aquatic species.
There are a few traits which make a species a good candidate for being a model species, including: short generation times or short life cycle and rapid development (Silvertown et al., 2011), anatomically simple and clearly defined biological system, tolerance to various environmental conditions (Morgan et al., 2019), capability for breeding or inbreeding, established domestication (Joubert and Bijlsma, 2010) and complete reference genomic studies (Waldvogel et al., 2020). Zebrafish and Medaka are species living in freshwater and marine water environments (Hsu et al., 2014), thus making them suitable organisms for understanding organism function changes within climate change-related studies in either environment. Zebrafish and Medaka’s ecology and evaluation are well established as natural model organisms, and knowledge of how they should best be managed is vital for various research (Marx, 2021; Chowdhury et al., 2022). In addition, Zebrafish and Medaka also share the same anatomy, genetic, and physiological elements (Hsu et al., 2014; Chowdhury et al., 2022).
Publication trends on model organisms have been assessed by previous authors, however, these authors only focused on a few species, mostly terrestrial (e.g., rat, mouse, common fruit flies, chicken, and frogs) rather than aquatic species (Dietrich et al., 2014). Therefore, comprehensive bibliographic analysis on aquatic model organisms, such as author and affiliation network or citation analysis is needed. Scientometrics is known as the idea of measuring the networks of scientists, institutions and ideas from a large database or number of sources. The fields of scientometrics and bibliometrics are growing, and there is an increased appreciation for their importance in many scientific fields. Previous scientometric analysis also has been done on the global mapping of zebrafish species (Kinth et al., 2013), however, their relationship with climate change was not assessed. There are no available scientometric-based studies for the Medaka species and this study is among the first to identify the different scientometrics for this species. Previous studies showed that various model organisms can be useful for researching climate change related studies, such as climate-induced water temperature changes (Morgan et al., 2019), acidification, and eutrophication (Altshuler et al., 2011) as well as pollution (Vilas-Boas et al., 2020). However, still missing is an analysis that broadly assesses how model organisms can improve our understanding of climate change.
Thus, the goal of this study was to examine the impact of climate change on two select aquatic model organisms. By employing bibliometric analyses and scientific techniques, we aimed to answer questions about the structure and dynamics of Zebrafish and Medaka as model organisms for climate change related studies. The questions were divided in two different categories, which were 1) the descriptive dataset and 2) the scientometrics-based analysis. The descriptive dataset includes the number of articles published, the citation counts of the articles, the distribution of the countries involved, and the active authors. Meanwhile, for the scientometrics data, the distribution of the cited authors and articles as well as the most abundant keywords used were generated from Citespace software.
The type of bibliometric based studies are also expected to increase the visibility of the field of research and future collaboration between authors and institutions involved. The study is also expected to contribute to the bioclimatology, policymakers, philanthropic and the public worldwide on how to invest limited resources in policies, programs, and research on the impacts of climate change on aquatic species.
Methodological framework for the current study is shown in Figure 1. Web of Science (WOS) Core Collection (WOSCC) was the only database used for the study because it is the general standard for scientometric-based analysis for identifying and monitoring research trends and development. The WOSCC searching index and database includes the “topic” (TS) field (i.e., article titles, abstracts, keywords, KeyWords Plus). There are few steps applied in the review process of the data, including selection of the database, identification of the articles that relate to our study, screening and eligibility criteria, and included documents. The various qualifying and exclusion criteria were considered, the article type, the language used and the source type of the article. Figure 1 shows that there were various types of methods employed in the systematic review process such as scope and coverage (i.e., database, keywords, etc.), topic identification, screening eligibility, merging, duplicate removal and included documents for the scientometric analysis.
FIGURE 1. Flowchart indicating methodological framework where two separate searches were done focusing on: (i) Zebrafish and (ii) Medaka relation with climate change.
The common name as well as the scientific name for both fish species were collected from FishBase, where the current correct information on fishes is provided by various experts and with the support of the European Commission. The climate change related keywords were similar as in Azra et al. (2022). As such, our search strings included the following:
1st searches
Zebrafish:
TS = ((zebrafish) OR (“Danio rerio”) OR (“Girella zebra”) OR (“Pterois russelii”) OR (“Pterois volitans”) OR (“D. rerio”) OR (“G. zebra”) OR (“P. russelii”) OR (“P. volitans”))
AND
Climate change:
TS= ((“climat* chang*”) OR (“global warm*”) OR (“seasonal* variat*”) OR (“extrem* event*”) OR (“environment* variab*”) OR (“anthropogenic effect*”) OR (“greenhouse effect*”) OR (“sea level ris*”) OR (erosio*) OR (“agricult* runoff”) OR (“weather* variab*”) OR (“weather* extrem*”) OR (“extreme* climat*”) OR (“environment* impact*”) OR (“environment* chang*”) OR (“anthropogenic stres*”) OR (“temperature ris*”) OR (“temperature effect*”) OR (“warm* ocean”) OR (“sea surface* temperat*”) OR (heatwav*) OR (acidific*) OR (hurrican*) OR (“el nino”) OR (“el-nino”) OR (“la nina”) OR (la-nina) OR (drought*) OR (flood*) OR (“high precipit*”) OR (“heavy rainfall*”) OR (“CO2 concentrat*”) OR (“melt* of the glacier*”) OR (“melt* ice*”) OR (“therm* stress*”) OR (drought) OR (hypoxia))
2nd searches
Medaka:
TS = [(medaka) OR (“Oryzias latipes”) OR (“Aphyocypris kikuchii”) OR (“O. latipes”) OR (“A. kikuchii”)]
AND
Climate change:
TS = ((climat* chang*) OR (“global warm*”) OR (“seasonal* variat*”) OR (“extrem* event*”) OR (“environment* variab*”) OR (“anthropogenic effect*”) OR (“greenhouse effect*”) OR (“sea level ris*”) OR (erosio*) OR (“agricult* runoff”) OR (“weather* variab*”) OR (“weather* extrem*”) OR (“extreme* climat*”) OR (“environment* impact*”) OR (“environment* chang*”) OR (“anthropogenic stres*”) OR (“temperature ris*”) OR (“temperature effect*”) OR (“warm* ocean”) OR (“sea surface* temperat*”) OR (heatwav*) OR (acidific*) OR (hurrican*) OR (el nino) OR (“el-nino”) OR (“la nina”) OR (la-nina) OR (drought*) OR (flood*) OR (“high precipit*”) OR (“heavy rainfall*”) OR (“CO2 concentrat*”) OR (“melt* of the glacier*”) OR (“melt* ice*”) OR (“therm* stress*”) OR (drought) OR (hypoxia)).
Data from WOSCC were imported directly to the CiteSpace software as a text file (*.txt). The CiteSpace version 6.1.R2 on 64-bit was used for the analysis. CiteSpace is among one of the popular software to analyze scientometric based results (Noor et al., 2021; Feng et al., 2022; Shi et al., 2022). It also shows various types of interactive visualization (i.e., network analysis and visualization), such as most active areas, major related areas, key papers for a given area, critical transitions in the history of the development of the field and its turning points.
The data were divided into the number of authors, number of institutions, countries involved, terminologies used, consistent and most common keywords, cited authors as well as cited references in the field. The threshold setting for CiteSpace was set at “Top 50 N” per slice, which allows the selection of most cited items from each slice to form a network based on the input value and node types. “Pruning” parameter was chosen to prune the network. All term sources in Web of Science, including title, abstract, author keywords, and keywords plus, were chosen for text processing. Three types of scientometric analysis were used in this study, namely; 1) cluster analysis, 2) co-citation analysis and 3) burstness analysis. The explanation for each analysis is given below.
Cluster analysis was used to identify research clusters in focus areas. For this study, variable use for cluster analysis is based on the Articles Cluster. Log-likelihood ratio was used to provide the cluster label as it gives the best results in terms of uniqueness and coverage (Hiekkalinna et al., 2012). The “timeline view” and “cluster view” were used to visualize the articles cluster network’s shape and form. The “timeline view” displayed a vertical range of chronological time periods from left to right, whereas the “cluster view” displayed a spatial network of colour-coded and automatically labeled representations in a landscape format (Chen 2004; Chen and Leydesdorff 2014).
The modularity Q index, average silhouette metric, and centrality metric were used to assess the quality and homogeneity of the document cluster analyses, as well as the detected clusters (Chen et al., 2009; Chen, 2020). The modularity Q index ranges between 0 and 1, where larger index values indicate higher reliability. The average silhouette metric has a value between −1 and 1, where values greater than 0 indicate greater homogeneity. Centrality is a measure of influence that shows the degree to which publications or journals stand between each other, where publications with high centrality would have higher influence on the network as they connect more publications or journals and therefore, more information and paths pass through them.
Co-citation analyses generate a research network consisting of nodes and link and density values to show the main structure of selected variables. For this study, selected variables included 1) Cited Articles analysis and 2) Cited Author analysis. Co-citation analysis will allow us to obtain the cluster of co-citing variables, where a co-citation instance occurs when two articles/authors are cited together in one paper (Boyack and Klavans, 2010). The analysis quality was assessed using degree, betweenness, and sigma (Chen and Song, 2019). The degree value represents the number of citations an article/author receives from another article/author, with a higher degree indicating more citations. Betweenness is a measure of influence that shows the influence to which the same article/author has on others. High betweenness means the article/author has a greater influence on the research areas because it connects other articles/authors, and thus more information and paths pass through them. Centrality is the combination value based on betweenness and burstiness scores (described below), ranging from 0 to 1 where the highest value is associated with high value research articles (Aryadoust et al., 2019).
Citation burstiness were used to determine top keywords related to research areas. Burst detection is defined as a sudden increase in the number of citations for a specific article, or “an abrupt elevation of the frequencies (of citations) over a specific time interval,” as indicated by a red ring around the node (Hou et al., 2018).
This study focused on scientific publications related to climate change and its relationship with zebrafish and medaka species. Research on medaka and climate change started in 1978 (Figure 2), where a total of 177 original articles have been published since then, with an average of 10 publications per year since 2011. On the other hand, the first scientific publication on zebrafish and climate change was only published in Web of Science in 1994. However, zebrafish publications rapidly outnumbered medaka publications, with a total of 1189 papers published since 1994.
FIGURE 2. Number of research articles related to climate change effects on Medaka and/or Zebrafish published annually since 1978.
Article citation is an indicator that shows the impact of a study in its research field. Based on previous studies, the direction of a research field is associated with the articles that are most frequently cited (Chen et al., 2010; Chen, 2020). The top three articles with the highest number of total citations for medaka and climate changes were, 1) “Evaluation of the toxic impact of silver nanoparticles on Japanese medaka (Oryzias latipes)” (210 citations); 2) “Evolutionary dynamics of olfactory receptor genes in fishes and tetrapods” (200 citations); and 3) “Zinc oxide nanoparticles induce oxidative DNA damage and ROS-triggered mitochondria-mediated apoptosis in zebrafish embryos” (137 citations). Tables 1, 2 show the highest citations for climate change research associated with Medaka and Zebrafish, respectively.
TABLE 2. The top ten articles with the highest number of citations for research on Zebrafish and climate change.
For research focusing on Zebrafish and Climate Change, the top 3 most cited publications were: 1) “Toxicity of silver nanoparticles in zebrafish models” (681 citations); 2) “The Oxygen-Rich Postnatal Environment Induces Cardiomyocyte Cell-Cycle Arrest through DNA Damage Response” (437 citations) and 3) “The Serine Protease Matriptase-2 (TMPRSS6) Inhibits Hepcidin Activation by Cleaving Membrane Hemojuvelin” (405 citations).
Research papers in the sample for medaka and climate change research came from 65 countries around the world. Figure 3 shows the distribution of countries working on the Medaka and Zebrafish as a model organism in climate change research and development. China was the most frequent publisher (56 publications), followed by the United States, Japan, Canada, France, Germany, Norway, South Korea, Taiwan and Australia as the top 10 countries that have published in this research area. In the research areas focusing on zebrafish and climate changes, a total of 74 countries contributed between 1994–2022. The United States of America contributed the highest number of articles (342 publications), followed by China, Canada, England, Germany, Australia, France, Italy, Brazil, and Taiwan.
FIGURE 3. The distribution of countries that work on Medaka and Zebrafish as a model organism in climate change research and development.
For the zebrafish subsample, there were 842 authors who published an article related to climate change and zebrafish, but only two authors published more than 10 articles (Figure 3). Rudolf S.S Wu from Education University of Hong Kong contributed the most with 12 articles, followed by Keng Po Lai (10 articles), and Doris Wai Ting Au/Ting Fung Chan (9 articles). Tables 3, 4 indicate the list of top ten most productive authors regarding research on medaka and zebrafish, respectively, and climate change. Within the zebrafish subsample, out of a total of 6015 authors, Steve F. Perry published the most articles (34 articles), followed by Michael G. Jonz (16 articles), and Bernd Pelster (15 articles).
Figure 4 depicts authors in the zebrafish subsample that have a betweenness score greater than 0.05.
FIGURE 4. The distribution of cited authors for research on zebrafish and climate change. Node size and font size increase as centrality score increases.
There were 886 nodes and 3010 connections in the author co-citation network for the zebrafish subsample. The co-citation network density value was 0.0077. Figure 4 depicts authors that have a centrality score greater than 0.1. Pamela A. Padilla from Fred Hutchinson Cancer Research Center was the most interconnected author, with a degree connection of 30, betweenness score of 0.07, and a sigma of 1.81. Andrew Y. Gracey was the second most influential author, with a degree connection of 56, betweenness score 0.11 and Sigma score of 1.65. Even though Andrew Y. Gracey’s degree connection and betweenness score are higher than Pamela A. Padilla’s, his sigma score is lower. This shows that Pamela A. Padilla is located more central in the network and is cited by authors with higher betweenness and degree than Andrew Y. Gracey, making him less influential. Christopher Ton was the third most influential author (Degree Connection: 30; Betweenness: 0.05, Sigma: 1.45). Table 5 shows the details for the top ten authors with the most influence in zebrafish and climate change research.
The author’s co-citation network for medaka and climate changes had 679 nodes, 2200 connections, and a density of 0.0096. Figure 5 illustrates the knowledge map for the author co-citation analysis, including only authors with betweenness scores greater than 0.05. Table 6 shows the details for the top ten authors involved in the Medaka subsample with the highest influence based on the authors degree, centrality, and sigma score.
Kinoshita Masato from Kyoto University was the most influential author with degree connection = 27, betweenness score = 0.15 and sigma score 1.72. The second and third most influential authors were Angel Amores (degree: 9, centrality: 0.05 and sigma: 1) and Philip L. Munday (degree: 6, centrality: 0.01 and sigma: 1).
Figure 6 illustrates the articles’ co-citation analysis for the zebrafish subsample. The network had 969 nodes and 2824 connections, with a density of 0.006. Only articles with a centrality score greater than 0.1 were listed.
FIGURE 6. Network of Document Co-citation Analysis where articles with a centrality score greater than 0.1 are shown.
Table 7 displays the top ten most influential scientific publications according to the degree, betweenness, and sigma score. “Zebrafish mutants in the von Hippel-Lindau tumor suppressor display a hypoxic response and recapitulate key aspects of Chuvash polycythemia” was the most influential article in zebrafish subsample, with a degree connection of 30, betweenness 0.19 and sigma score of 3.17. “The zebrafish reference genome sequence and its relationship to the human genome” was the second most influential article, with a degree connection of 12, betweenness 0.20 and sigma 2.14. The article “Hypoxia-inducible factor-1 mediates adaptive developmental plasticity of hypoxia tolerance in zebrafish, Danio rerio” was the third most influential article (degree = 21, centrality = 0.12 and sigma = 1.80).
TABLE 7. Top 10 (of 1189) most influential scientific publications related to research on zebrafish and climate change.
Figure 7 shows the majority of highly influential articles based on the article co-citation analysis for the medaka subsample. The network contained 617 nodes and 1695 links. Each node represents an article with the first author’s name and the publication year. The links between nodes represent co-citation relations between two articles. As there were no articles with a betweenness score >0.1, there are no names listed in the figure.
FIGURE 7. Medaka and Climate Change Network of Document Co-citation Analysis, where articles with a centrality score greater than 0.1 are shown.
Table 8 shows the most influential publications (publications according to the degree, betweenness, and sigma score in the medaka subsample. The most influential article is “Epigenetics in teleost fish: From molecular mechanisms to physiological phenotypes” with degree connection of 109, betweenness score 0.04 and Sigma of 1. Interestingly, there are no articles with sigma more than 1, which might indicate there are no highly recognizable articles in this domain.
TABLE 8. Top 10 (of 117) most influential publications related to research on medaka and climate change.
The Modularity Q Index and the Mean Silhouette metric for the zebrafish subsample’s article cluster analysis were 0.8963 and 0.9642 respectively, suggesting more than average reliability and homogeneity for the network. The analysis yielded a total of 17 co-citation clusters which are summarized in Table 9, where each cluster represents a different research topic. The cluster’s size is equal to the number of publications it has. Each of the clusters has range between 4 and 80 publications, with Cluster #0 having the most (95 publications). Cluster silhouette score ranged from 0.927 to 1.000, indicating a high degree of homogeneity among publications in each cluster (silhouette score ranges from −1 to 1, with scores >0 seen as homogenous). This indicator measures the combined strength of structural and temporal properties of a node, namely, its betweeness centrality and citation burst. A composite metric sigma is defined in CiteSpace to measure the combined strength of structural and temporal properties of a node, namely, its betweenness centrality and citation burst. Sigma is computed as (centrality +1) burstness (50), with higher values indicating works with higher influential potential. (Chen et al., 2009).
TABLE 9. Seventeen (17) major clusters from the article co-citation analysis for research on zebrafish and climate changes.
Figure 8 depicts these 17 clusters summarized on a horizontal line, with the cluster label on the right side. The clusters were numbered and ranked in order of size, with #0 being the largest and #39 the smallest. The solid yellow line within each cluster represents the cluster’s lifetime. Text mining and a keyword analysis algorithm in CiteSpace software were used to generate the cluster labels, then the log likelihood ratio was used to name these clusters (LLR).
FIGURE 8. Summary of identified document cluster lifetimes (solid lines). Cluster labels were generated from CiteSpace.
The Modularity Q Index and the Mean Silhouette metric for the Medaka subsample’s cluster analysis were 0.9645 and 0.9980 respectively, suggesting again more than average reliability and homogeneity for the network. The analysis yielded a total of 3 co-citation clusters which are summarized in Table 10. Each cluster ranged between 12 and 35 publications, with Cluster #0 having the most (35 publications). Cluster silhouette score ranged from 0.997 to 1.000, indicating a high degree of homogeneity among publications in each cluster.
TABLE 10. Three (3) major clusters that emerged from the article co-citation analysis for research on medaka and climate change.
Figure 9 depicts these 20 clusters summarized on a horizontal line, with the cluster label on the right side as well as with three major cluster summarized in Table 10. The clusters were numbered and ranked in order of size, with #0 being the largest and #16 the smallest. As before, text mining and keyword analysis algorithms in CiteSpace software was used to generate the cluster labels, and log likelihood ratio was used to name the clusters (LLR).
FIGURE 9. Summary of identified medaka and climate changes cluster lifetimes (solid lines). Cluster labels were generated from CiteSpace.
Table 11 displays the keywords with the keyword citation burst for the zebrafish subsample. There were no burst keywords from the medaka subsample. Bursts reflect the emergence of a keyword within a subject area during a specific time period (the blue line), here from 1994 to 2022, while the red lines represent the burst period. Beginning in 2019 and ending in 2022, the word “temperature” had the highest burst strength (6.81). The following top keywords were “embryonic development” (Strength = 5.5, 2016–2019) and “in vivo” (Strength = 4.78, 2009–2013).
Here, research related to climate change and either zebrafish or medaka was synthesized, revealing the research landscape in terms of the year, journals, authors, countries, keywords, and references. A descriptive analysis was performed on the number of publications, authors, and the countries/regions where authors were affiliated with when papers were published.
Both research domains saw the number of articles published increase annually, but the zebrafish subsample saw a higher increase in articles published, with a 10-years average of 92.9 publications per year; whereas medaka subsample only published a 10-years average of 12.7 articles per year. Consequently, the number of papers with high citation counts also differed, where zebrafish had 10 articles with >200 citations whereas medaka only had two articles with >200 citations. Both domains had China and the United States of America as the highest contributors for the number of papers published in the field. The top ten countries and authors varied in terms of region, but were dominated by developed countries and high-ranking institutions. As this result may be due to developed countries and high-ranking institutions having more resources to conduct scientific analyses, we strongly suggest that more international scientific research exchange and collaboration be conducted in the future. The difference in publication numbers and citations indicates that biology as a field has focused more on using zebrafish as a model species when it comes to climate change research, rather than medaka.
Tables 1, 2 showed that most of the top papers published were not related to climate change-related studies. Keywords related to environmental conditions, environmental change, and stress appeared to capture some articles that were not directly related to our objectives regarding climate change. Most of the articles on medaka discussed the ability and roles of the model organism towards various fields of study, such as nanotechnology (i.e., nanomaterials/nanoparticles) (Chae et al., 2009; Zhao et al., 2016) and genetics (Niimura and Nei, 2005). Similarly, articles on zebrafish discussed the species’ ability and role in the fields of nanotechnology (Asharani et al., 2008) and cell metabolism (Silvestri et al., 2008; Puente et al., 2014).
However, there were a few articles on Medaka and Zebrafish that were directly related to climate change issues and elements, such as environmental stress caused by hypoxia (Kong et al., 2008; Wang et al., 2016) and agricultural runoff (Hayasaka et al., 2012) and pollution (Babayigit et al., 2016). Basically, global warming can induce coastal hypoxia by decreasing oxygen solubility and resulting in an increase in the oxygen demand of the aquatic species. Adult marine medaka was used as a model organism for the determination of environmental stress of hypoxia, and the results showed that there were significant alterations in levels of cell proliferation marker and protein in liver conditions of the species, Oryzias melastigma (Kong et al., 2008). Sexually matrure marine medaka, O. melastigma were also affected by hypoxia, causing retarded gonad development, decrease in sperm count and sperm motility of the fish (Wang et al., 2016). Hayasaka et al. (2012) showed the effects of toxic agro-chemicals on the abundance of benthic organisms, such as Medaka, in aquatic paddy communities. They found that adult and juvenile medaka body size were smaller in the treated insecticide pools compared to the controls over the 2 year experiment. In the Zebrafish experiment, environmental pollution caused by the lead and tin impacted the fish embryonic development (Babayigit et al., 2016). These results show that climate change research using medaka or zebrafish as models have largely focused on the effects of agriculture runoff and hypoxia. Specifically, research appears to have focused on how these effects change development and growth within these model species.
There is a huge difference between the most influential author analysis for the zebrafish subsample compared to the medaka subsample. The zebrafish subsample had >10 influential authors with a sigma score >1.0, while the medaka subsample only had one influential author. Even though the medaka subsample started earlier than the zebrafish subsample, the latter was more abundant and had higher influence in the scientific network than the former. From our results, the most influential authors in medaka research are clearly not active anymore as there is hardly any new research being published, indicating low scientific novelty for this domain. This demonstrates the need to change the current approach and introduce more disciplines in this domain. It is critical to start developing experts in these fields and build connections between sub-disciplines or the domain might cease to exist in the future. Our study also suggest that the domain (i.e., medaka and climate change) might not producing enough valuable research, and this could be shows less publication. This is also could be affected that the zebrafish are a better model organism for climate change related studies.
In bibliometrics, co-citation analysis is one way to assess similar articles. Document or article co-citation analysis is a unique method for tracking similar papers that are cited together in journals by many authors; it will lastly identify clusters of research (Boyack and Klavans, 2010). Here, we only found a few articles for both species that fell into categories related to the current objectives of the paper (which is the use of model organisms in climate change research). Instead most of the related studies were on hypoxia and agricultural runoff of chemical pollution (Robertson et al., 2014; Bertram et al., 2015; Lai et al., 2016; Wang et al., 2016). However, few articles have an impact in terms of documents co-citation analysis. For zebrafish species, there were only two articles with a sigma value higher than 2 (which indicates importance of the papers in the field), whereas no articles from the Medaka species reached a sigma value of 2. The studies by Rooijen et al. (2009) and Howe et al. (2013) had sigma values above 2.0. Rooijen et al. (2009) used zebrafish as a model organism to understand cellular response of tumors in humans by exposing fish to hypoxic conditions. Meanwhile, the study by Howe et al. (2013) showed the relationship between the zebrafish reference genome sequence with the human genome. This shows that the zebrafish was not only used for the climate change impacted the aquatic ecosystem or organism, it was also used for the human related studies. This could be impacted the number of researcher using this animals (i.e., zebrafish) rather than the Medaka species. Based on Table 11, there were a total of 15 top keywords generated from the scientometrics analysis. Surprisingly, the top keyword was temperature, in which it was the key driving force in climate change related studies, especially related to the aquatic organisms (Bozinovic and Pörtner, 2015). However, none of the papers or cluster was actually related to the temperature and climate change. This could be attributed to keywords used for researcher when conducted their related studies towards climate change, such as climate-induce water temperature changes or surface temperature (Borges et al., 2019; Azra et al., 2020). As mentioned above, the zebrafish subsample was the most related to climate change studies (Table 11), which indicates the importance of this species towards climate change related research.
Based on the previous scientometric results that was discussed in the present study, the authors found that the Zebrafish and Medaka have been widely used as one of the model species for ecotoxicological based studies. Agriculture runoff is categorized as one of the climate change elements (Azra et al., 2022), and this could directly or indirectly associated with many toxicological chemicals in the environment (Boxall et al., 2009). However, as both Zebrafish and Medaka can be found in the freshwater and seawater ecosystem, future insight into the potential of how these model species could be properly employed to improve our understanding of climate-driven changes are truly needed. For example, climate-induce water temperature changes could be one of the great example on how global warming could impacted the growth and development of both model species. Their results could be compared and future adaptation strategies could be proposed in the future.
Studying model organisms helps increase our understanding and knowledge on various types of systems as impacts can be applied to other species. The Zebrafish and Medaka are among one of the most popular aquatic model organisms for studies on climate change. However, our analysis has shown that zebrafish are the most versatile model organism compared to Medaka for climate change as well as in bioindication domain-related studies. Within climate change research, hypoxia and agriculture runoff were common examples for work using these species as models. While the number of publications on these species has increased rapidly, particularly for zebrafish, still missing are more specific studies related to climate change. To understand how other marine or freshwater species will be affected by climate change, particularly climate warming, zebrafish and medaka can be used in experimental studies as model species.
To our knowledge, this is the first study that systematically analyzes and differentiates the data of model-based organisms in climate change studies. However, this survey has some limitations. With respect to our search approach, we included only research articles that were published in English (excluding books, conference papers, abstract, notes, etc.). Additionally our keywords may have been too general, which may be why we saw a high percentage of research not directly related to climate change elements.
For future scientometric work, other databases such as Scopus, Directory of Open Access Journals etc. should be used for article searching and indexing in the future to include a wider array of articles. We also recommend including other alternative climate change species models, such as the Artemia. With respect to primary climate change research, our results indicate that work further investigating the effects of climate-induced hypoxia as well as chemical tolerance on model organisms would be beneficial. Further, we strongly suggest the use of model organisms in climate research to understand more climate induced effects, such as heatwaves, freshwater influx from flooding, or drought conditions that all may influence fish or other organism’s development.
MN: Preliminary idea; First draft; Final draft MM: Preliminary idea; First draft; Final draft MP: Literature search; Critically revised; Software application; Final draft MD: Literature search; Critically revised; Second draft; Final draft MA: Literature search; Critically revised; Second draft; Final draft IZ: Literature search; Critically revised; Final draft MF: Literature search; Critically revised; Second draft; Final draft ZA: Literature search; Critically revised; Supervision; Final draft FP: Preliminary idea; Critically revised; Funding Acquisition; Final draft.
The funding was received through PYPA Initiative managed by the Universiti Teknologi MARA (UiTM).
The authors declare that the research was conducted in the absence of any commercial or financial relationships that could be construed as a potential conflict of interest.
All claims expressed in this article are solely those of the authors and do not necessarily represent those of their affiliated organizations, or those of the publisher, the editors and the reviewers. Any product that may be evaluated in this article, or claim that may be made by its manufacturer, is not guaranteed or endorsed by the publisher.
The Supplementary Material for this article can be found online at: https://www.frontiersin.org/articles/10.3389/feart.2022.988710/full#supplementary-material
Altshuler, I., Demiri, B., Xu, S., Constantin, A., Yan, N. D., and Cristescu, M. E. (2011). An integrated multi-disciplinary approach for studying multiple stressors in freshwater ecosystems: Daphnia as a model organism. Integr. Comp. Biol. 51, 623–633. doi:10.1093/icb/icr103
Aryadoust, V., Tan, H. A. H., and Ng, L. Y. (2019). A scientometric review of rasch measurement: The rise and progress of a specialty. Front. Psychol. 10, 2197. doi:10.3389/fpsyg.2019.02197
Asharani, P. V., Wu, Y. L., Gong, Z., and Valiyaveettil, S. (2008). Toxicity of silver nanoparticles in zebrafish models. Nanotechnology 19, 255102. doi:10.1088/0957-4484/19/25/255102
Azra, M. N., Aaqillah-Amr, M. A., Ikhwanuddin, M., Ma, H., Waiho, K., Ostrensky, A., et al. (2020). Effects of climate‐induced water temperature changes on the life history of brachyuran crabs. Rev. Aquac. 12, 1211–1216. doi:10.1111/raq.12380
Azra, M. N., Noor, M. I. M., Sung, Y. Y., and Ghaffar, M. A. (2022). What evidence exists on the impact of climate change on some of the worst invasive fish and shellfish? A systematic map protocol. Environ. Evid. 11, 19. doi:10.1186/s13750-022-00273-z
Babayigit, A., Thanh, D. D., Ethirajan, A., Manca, J., Muller, M., Boyen, H. G., et al. (2016). Assessing the toxicity of Pb- and Sn-based perovskite solar cells in model organism Danio rerio. Sci. Rep. 6, 18721. doi:10.1038/srep18721
Bertram, M. G., Saaristo, M., Baumgartner, J. B., Johnstone, C. P., Allinson, M., Allinson, G., et al. (2015). Sex in troubled waters: Widespread agricultural contaminant disrupts reproductive behaviour in fish. Hormones Behav. 70, 85–91. doi:10.1016/j.yhbeh.2015.03.002
Borges, J. S., Grande, F. R. D., and Costa, T. M. (2019). Do lower air or water temperatures limit the southern distribution of the white mangrove Laguncularia racemosa in South America? Estuar. Coast. Shelf Sci. 230, 106449. doi:10.1016/j.ecss.2019.106449
Boxall, A. B. A., Hardy, A., Beulke, S., Boucard, T., Burgin, L., Falloon, P. D., et al. (2009). Impacts of climate change on indirect human exposure to pathogens and chemicals from agriculture. Environ. Health Perspect. 117, 508–514. doi:10.1289/ehp.0800084
Boyack, K. W., and Klavans, R. (2010). Co-citation analysis, bibliographic coupling, and direct citation: Which citation approach represents the research front most accurately? J. Am. Soc. Inf. Sci. Technol. 61, 2389–2404. doi:10.1002/asi.21419
Bozinovic, F., and Pörtner, H. O. (2015). Physiological ecology meets climate change. Ecol. Evol. 5, 1025–1030. doi:10.1002/ece3.1403
Braña, C. B. C., Cerbule, K., Senff, P., and Stolz, I. K. (2021). Towards environmental sustainability in marine finfish aquaculture. Front. Mar. Sci. 8, 666662. doi:10.3389/fmars.2021.666662
Chae, Y. J., Pham, C. H., Lee, J., Bae, E., Yi, J., and Gu, M. B. (2009). Evaluation of the toxic impact of silver nanoparticles on Japanese medaka (Oryzias latipes). Aquat. Toxicol. 94, 320–327. doi:10.1016/j.aquatox.2009.07.019
Chen, C., Chen, Y., Horowitz, M., Hou, H., Liu, Z., and Pellegrino, D. (2009). Towards an explanatory and computational theory of scientific discovery. J. Inf. 3, 191–209. doi:10.1016/j.joi.2009.03.004
Chen, C., Ibekwe-SanJuan, F., and Hou, J. (2010). The structure and dynamics of cocitation clusters: A multiple-perspective cocitation analysis. J. Am. Soc. Inf. Sci. Technol. 61, 1386–1409. doi:10.1002/asi.21309
Chen, C., and Leydesdorff, L. (2014). Patterns of connections and movements in dual-map overlays: A new method of publication portfolio analysis. J. Assoc. Inf. Sci. Technol. 65, 334–351. doi:10.1002/asi.22968
Chen, C., and Song, M. (2019). Visualizing a field of research: A methodology of systematic scientometric reviews. Plos One 14, e0223994. doi:10.1371/journal.pone.0223994
Chen, C. (2020). How to use CiteSpace. British Columbia, Canada: Lean Publishing, 246. Available at: https://citespace.podia.com/view/downloads/ebook-how-to-use-citespace.
Chen, C. (2004). Searching for intellectual turning points: Progressive knowledge domain visualization. Proc. Natl. Acad. Sci. U. S. A. 101, S5303–S5310. doi:10.1073/pnas.0307513100
Chowdhury, K., Lin, S., and Lai, S. L. (2022). Comparative study in Zebrafish and Medaka unravels the mechanisms of tissue regeneration. Front. Ecol. Evol. 10, 78381. doi:10.3389/fevo.2022.783818
Dietrich, M. R., Ankeny, R. A., and Chen, P. M. (2014). Publication trends in model organism research. Genetics 198, 787–794. doi:10.1534/genetics.114.169714
Feng, L., Shang, S., Feng, X., Kong, Y., and Bai, J. (2022). Evolution and trend analysis of research hotspots in the field of pollution-intensive industry transfer—based on literature quantitative empirical study of China as world factory. Front. Environ. Sci. 10, 732734. doi:10.3389/fenvs.2022.732734
Free, C. M., Cabral, R. B., Froehlich, H. E., Battista, W., Ojea, E., O'Reilly, E., et al. (2022). Expanding ocean food production under climate change. Nature 605, 490–496. doi:10.1038/s41586-022-04674-5
Hayasaka, D., Korenaga, T., Suzuki, K., Saito, F., Sánchez-Bayo, F., and Goka, K. (2012). Cumulative ecological impacts of two successive annual treatments of imidacloprid and fipronil on aquatic communities of paddy mesocosms. Ecotoxicol. Environ. Saf. 80, 355–362. doi:10.1016/j.ecoenv.2012.04.004
Hiekkalinna, T., Goring, H. H. H., and Terwilliger, J. D. (2012). On the validity of the likelihood ratio test and consistency of resulting parameter estimates in joint linkage and linkage disequilibrium analysis under improperly specified parametric models. Ann. Hum. Genet. 76, 63–73. doi:10.1111/j.1469-1809.2011.00683.x
Hou, J., Yang, X., and Chen, C. (2018). Emerging trends and new developments in information science: A document co-citation analysis (2009–2016). Scientometrics 115, 869–892. doi:10.1007/s11192-018-2695-9
Howe, K., Clark, M. D., Torroja, C. F., Torrance, J., Berthelot, C., Muffato, M., et al. (2013). The zebrafish reference genome sequence and its relationship to the human genome. Nature 496, 498–503. doi:10.1038/nature12111
Hsu, H. H., Lin, L. Y., Tseng, Y. C., Horng, J. L., and Hwang, P. P. (2014). A new model for fish ion regulation: Identification of ionocytes in freshwater- and seawater-acclimated medaka (Oryzias latipes). Cell. Tissue Res. 357, 225–243. doi:10.1007/s00441-014-1883-z
Joubert, D., and Bijlsma, R. (2010). Interplay between habitat fragmentation and climate change: Inbreeding affects the response to thermal stress in Drosophila melanogaster. Clim. Res. 43, 57–70. doi:10.3354/cr00883
Kinth, P., Mahesh, G., and Panwar, Y. (2013). Mapping of Zebrafish research. A global outlook. Zebrafish 10, 510–517. doi:10.1089/zeb.2012.0854
Kong, R. Y. C., Giesy, J. P., Wu, R. S. S., Chen, E. X. H., Chiang, M. Wl, Lim, P. L., et al. (2008). Development of a marine fish model for studying in vivo molecular responses in ecotoxicology. Aquat. Toxicol. 86, 131–141. doi:10.1016/j.aquatox.2007.10.011
Lai, K. P., Li, J. W., Tse, C. K., Chan, T. F., and Wu, R. S. S. (2016). Hypoxia alters steroidogenesis in female marine medaka through miRNAs regulation. Aquat. Toxicol. 172, 1–8. doi:10.1016/j.aquatox.2015.12.012
Mamun, A. A., Murray, F. J., Sprague, M., McAdam, B. J., Roos, N., de-Roos, B., et al. (2021). Pounds Am little DCExport-driven, extensive coastal aquaculture can benefit nutritionally vulnerable people. Front. Sustain. Food Syst. 5, 713140. doi:10.3389/fsufs.2021.713140
Marx, V. (2021). Model organisms on roads less traveled. Nat. Methods 18, 235–239. doi:10.1038/s41592-021-01086-7
Maulu, S., Nawanzi, K., Abdel-Tawwab, M., and Khalil, H. S. (2021). Fish nutritional value as an approach to children's nutrition. Front. Nutr. 8, 780844. doi:10.3389/fnut.2021.780844
Morgan, R., Sundin, J., Finnoen, M. H., Dresler, G., Vendrell, M. M., Dey, A., et al. (2019). Are model organisms representative for climate change research? Testing thermal tolerance in wild and laboratory zebrafish populations. Conserv. Physiol. 7, coz036. doi:10.1093/conphys/coz036
Müller, B., and Grossniklau, U. (2010). Model organisms — a historical perspective. J. Proteomics 73, 2054–2063. doi:10.1016/j.jprot.2010.08.002
Niimura, Y., and Nei, M. (2005). Evolutionary dynamics of olfactory receptorgenes in fishes and tetrapods. Proc. Natl. Acad. Sci. U. S. A. 102, 6039–6044. doi:10.1073/pnas.0501922102
Noor, M. I. M., Azra, M. N., Lim, V. C., Zaini, A. A., Dali, F., Hashim, I. M., et al. (2021). Aquaculture research in Southeast Asia - a scientometric analysis (1990-2019). Int. Aquatic Res. 13, 271–288. doi:10.22034/IAR.2021.1932503.1166
Puente, B. N., Kimura, W., Muralidhar, S. A., Moon, J., Amatruda, J. F., Phelps, K. L., et al. (2014). The oxygen-rich postnatal environment induces cardiomyocyte cell-cycle arrest through DNA damage response. Cell. 24, 565–579. doi:10.1016/j.cell.2014.03.032
Robertson, C. E., Wright, P. A., Koblitz, L., and Bernier, N. J. (2014). Hypoxia-inducible factor-1 mediates adaptive developmental plasticity of hypoxia tolerance in zebrafish, Danio rerio. Proc. R. Soc. B 281, 20140637. doi:10.1098/rspb.2014.0637
Rooijen, E. V., Voest, E. E., Logister, I., Korving, J., Schwerte, T., Schulte-Merker, S., et al. (2009). Zebrafish mutants in the von Hippel-Lindau tumor suppressor display a hypoxic response and recapitulate key aspects of Chuvash polycythemia. Blood 113, 6449–6460. doi:10.1182/blood-2008-07-167890
Shi, X., Zhang, J., Lu, S., Wang, T., and Zhang, X. (2022). China carbon neutralization research status and research frontier tracking. Front. Environ. Sci. 10, 896524. doi:10.3389/fenvs.2022.896524
Silvertown, J., Cook, L., Cameron, R., Dodd, M., McConway, K., Worthington, J., et al. (2011). Citizen science reveals unexpected continental-scale evolutionary change in a model organism. PLoS ONE 6, e18927. doi:10.1371/journal.pone.0018927
Silvestri, L., Pagani, A., Nai, A., Domenico, I. D., Kaplan, J., and Camaschella, C. (2008). The serine Protease matriptase-2 (TMPRSS6) Inhibits Hepcidin activation by cleaving Membrane Hemojuvelin. Cell. Metab. 8, 502–511. doi:10.1016/j.cmet.2008.09.012
Sunday, J. M., Bates, A. E., and Dulvy, N. K. (2012). Thermal tolerance and the global redistribution of animals. Nat. Clim. Chang. 2, 686–690. doi:10.1038/nclimate1539
Tregaskes, C. A., and Kaufman, J. (2021). Chickens as a simple system for scientific discovery: The example of the MHC. Mol. Immunol. 135, 12–20. doi:10.1016/j.molimm.2021.03.019
Vilas–Boas, J. A., Cardoso, S. J., Senra, M. V. X., Rico, A., and Dias, R. J. P. (2020). Ciliates as model organisms for the ecotoxicological risk assessment of heavy metals: A meta–analysis. Ecotoxicol. Environ. Saf. 199, 110669. doi:10.1016/j.ecoenv.2020.110669
Waldvogel, A. M., Schreiber, D., Pfenninger, M., and Feldmeyer, B. (2020). Climate change genomics calls for standardized data reporting. Front. Ecol. Evol. 8, 242. doi:10.3389/fevo.2020.00242
Wang, S. Y., Lau, K., Lai, K. P., Zhang, J. W., Tse, A. C. K., Li, J. W., et al. (2016). Hypoxia causes transgenerational impairments in reproduction of fish. Nat. Commun. 7, 12114. doi:10.1038/ncomms12114
Keywords: bibliometrics, Citespace, environmental sciences, global warming, hypoxia number of articles affiliations
Citation: Nor Azra M, Mohd Noor MI, Pau Tan M, Dawood M, Amin M, Zekker I, Fuad Abdullah M, Abd Latif Z and Pardi F (2022) Zebrafish and Medaka as model organisms for climate change research: Global literature scientometric analysis. Front. Earth Sci. 10:988710. doi: 10.3389/feart.2022.988710
Received: 07 July 2022; Accepted: 01 August 2022;
Published: 08 September 2022.
Edited by:
Huopo Chen, Institute of Atmospheric Physics (CAS), ChinaReviewed by:
Liqiang Zhao, Guangdong Ocean University, ChinaCopyright © 2022 Nor Azra, Mohd Noor, Pau Tan, Dawood, Amin, Zekker, Fuad Abdullah, Abd Latif and Pardi. This is an open-access article distributed under the terms of the Creative Commons Attribution License (CC BY). The use, distribution or reproduction in other forums is permitted, provided the original author(s) and the copyright owner(s) are credited and that the original publication in this journal is cited, in accordance with accepted academic practice. No use, distribution or reproduction is permitted which does not comply with these terms.
*Correspondence: Faezah Pardi, ZmFlemFocGFyZGlAdWl0bS5lZHUubXk=; Mohd Iqbal Mohd Noor, bW9oZGlxYmFsbW5AdWl0bS5lZHUubXk=
Disclaimer: All claims expressed in this article are solely those of the authors and do not necessarily represent those of their affiliated organizations, or those of the publisher, the editors and the reviewers. Any product that may be evaluated in this article or claim that may be made by its manufacturer is not guaranteed or endorsed by the publisher.
Research integrity at Frontiers
Learn more about the work of our research integrity team to safeguard the quality of each article we publish.