- 1Research Institute of Exploration and Development, PetroChina Xinjiang Oilfield Company, Karamay, China
- 2PetroChina Research Institute of Petroleum Exploration and Development—Northwest, Lanzhou, China
- 3Key Laboratory of Deep Oil and Gas, School of Geosciences, China University of Petroleum, Qingdao, China
- 4State Key Laboratory for Mineral Deposits Research, School of Earth Sciences and Engineering, Nanjing University, Nanjing, China
To investigate the mechanisms of deep oil–gas accumulation in superimposed basins in western China, and identify deep oil–gas reserves in the Junggar Basin, this study examined Carboniferous reservoirs in the Central Depression of the basin. We determined the hydrocarbon physical properties and geochemical characteristics, and controlling factors on hydrocarbon accumulation in the deep part of the Central Depression. We present a geological model of oil–gas accumulation based on analog modeling of reservoir formation. The deep hydrocarbons in the study area are mainly light–medium oil and wet natural gas, which were mainly derived from Permian and Carboniferous source rocks. The hydrocarbon source rocks and their thermal evolution led to hydrocarbon accumulation in the study area. Adjacent source–reservoir rocks on both sides of faults were key to vertical hydrocarbon migration and multi-stage charging. Suitable reservoir–cap rock assemblages were also important for reservoir preservation. The hydrocarbon accumulation model involves a source–reservoir link along faults, late charging, and paleo-uplifts. The reservoir types include those linked to source rocks by large-scale faults and those in paleo-uplifts surrounded by oil-generating depressions, which both have good exploration prospects. This accumulation model may be applicable to deep parts of other superimposed basins.
1 Introduction
Deep oil–gas accumulation usually means the hydrocarbon resources buried below 4,500 m in sedimentary basins (Jia and Pang, 2015; Pang et al., 2015). Recent years, the deep parts of sedimentary basins are increasingly important sites for global oil–gas exploration, and attract much attention (Ma et al., 2018; Zheng et al., 2018; Zhu et al., 2021). However, many questions still in confusion, such as the formation and evolution of deep-buried traps, the efficiency of large-scale reservoirs, the types of migration pathways and hydrocarbon accumulation processes. In China, superimposed petroliferous basins have experienced multiple stages of tectonism and sedimentation, which have led to the development of multiple sets of source and reservoir rocks. These complex geological conditions make the hydrocarbon accumulation mechanism in the deep buried strata still unclear. Lateral hydrocarbon migration and accumulation have been affected by faulting and, as such, the hydrocarbon migration and accumulation processes are complex (Zhang et al., 2021).
The Junggar Basin is a typical superimposed petroliferous basin in western China and an important area for onshore oil–gas exploration. Deep strata exploration began in the 1990s, when the first ultra-deep well (6,010 m; the Lu 3 well) was drilled in the basin, specifically in the Luliang Uplift of the Central Depression where deep Carboniferous strata were targeted. Based on this well, the Carboniferous reservoirs in the Shixi area were discovered and developed. However, subsequent deep oil–gas exploration has been limited by technological and economic constraints. In 2005, the deepest onshore exploration well in China at that time (i.e., the Moshen1 well) was drilled in the Carboniferous anticline of the Mosuowan Uplift in the Central Depression to a depth of 7,500 m, which yielded a good oil–gas show. Although there was no oil flow, it was confirmed that effective source rocks and reservoirs occur in (ultra)-deep parts of the Central Depression of the basin. Since 2005, the PetroChina Xinjiang Oilfield Company has increased exploration in many areas of the deep basin, which has led to the establishment of many producing oil–gas wells. In particular, in the past 2 years, discoveries have been made in the Chetan 1, Shatan 1, Kangtan 1, Gaotan 1, and Hutan 1 wells in Carboniferous–Triassic strata around the Shawan and Fukang sags, and in Jurassic–Cretaceous strata in the southern thrust belt (Du et al., 2019a; Du et al., 2019b; Zhi et al., 2019; Hu et al., 2020; He et al., 2021; Zhi et al., 2021). This highlights the considerable exploration potential of the deep basin strata, and necessitates further improvements in our geological understanding of the deep structures, source and reservoir rocks, and hydrocarbon accumulation in such settings. Although previous exploration and studies have indicated the deep Carboniferous strata in the Central Depression are a potential exploration area, key factors such as the state of the oil–gas phases and hydrocarbon accumulation processes remain unclear and hinder hydrocarbon exploration. This is a common problem for (ultra)-deep oil–gas exploration worldwide.
As such, this study investigated deep Carboniferous reservoirs in the Central Depression of the Junggar Basin, China, in what is the first study of the hydrocarbon accumulation mechanisms in this region. To guide deep oil–gas exploration, analog modeling of reservoir formation was undertaken to constrain the processes of oil–gas accumulation and enrichment.
2 Geological setting
The Junggar Basin is part of the Central Asia Orogenic Belts (CAOB) and located in northwestern China, and has an area of 13.6 × 104 km2. The basin has experienced numerous tectonic events from the Carboniferous to Quaternary, and has evolved from a transitional marine–continental facies basin to an intracontinental depression basin with multiple depocenters, a large depression basin that underwent multiple cycles of subsidence, and finally a foreland basin (Chen et al., 2002; Chen et al., 2005; He et al., 2018; Wang et al., 2018). The strata in the Central Depression are Carboniferous to Quaternary in age, and the oldest strata intersected by drilling are early Carboniferous. The Carboniferous rocks consist mainly of andesite, basalt, tuff, and occasional clastic sedimentary rocks. In the early Carboniferous, the Junggar Basin was in a marine environment, in which marine clastic and carbonate rocks were deposited, and volcanic rocks were erupted. In the late Carboniferous, the ocean basin closed and a foreland basin formed (Li et al., 2012; Li et al., 2015a; Li et al., 2015b). At the end of the Carboniferous, the basin was strongly deformed and erosion was widespread (He et al., 2010a; Lu et al., 2013). During the early–middle Permian, the Junggar Basin had multiple depositional and subsidence centers in the Mahu, Shawan, Penyijingxi, Fukang, Jimusar, and Zhangbei–Shishugou regions, in which the best source rocks in the basin were deposited (Wang et al., 2012; Xia et al., 2020a; Xia et al., 2020b; Cao et al., 2020; Tang et al., 2021; Xia et al., 2022). In the Mesozoic, the Junggar Basin were developed as an intracontinental depression due to the compression from northwest and northeast (Allen and Vincent, 1997; Liu et al., 2017). The strata were mainly composed of conglomerate, sandstone, mudstone and so on. From Late Jurassic to Early Neogene, the compression stress became gradually weak and changed to slightly extension, developing NS- and EW-trending normal faults (Liu et al., 2017). The strata also were mainly composed of conglomerate, sandstone and mudstone, with coal beds developed in the Jurassic. From Neogene to Quaternary, the Junggar Basin changed into a rejuvenated foreland basin (Wu et al., 2005).
Based on the extent of exploration and data availability, this study focused on Carboniferous reservoirs in the Penyijingxi Sag and surrounding areas. The Penyijingxi Sag is one of units in the Central Depression, and is surrounded by the Mosuowan, Mobei, Shixi, Dabasong, and Xiayan uplifts. Several large-scale and high-output oil–gas fields, such as the Pen 5 gas field and Shixi oilfield, have been discovered in the uplift belt around the Penyijingxi Sag (Figure 1). The oil and gas are distributed in several Carboniferous–Jurassic reservoirs.
3 Geochemistry and origin of the oil–gas
3.1 Geochemistry and origins of the crude oils
Typical crude oils in Carboniferous reservoirs in the Penyijingxi Sag occur in the Zhongguai, Shixi, and Mosuowan uplifts. The crude oils have a low densityand a medium wax content (Figure 2). The physical properties of the crude oil vary laterally, and the crude oil density decreases with depth (Figure 2). The crude oil at < 3,100 m depth is medium oil, at 3,100–3,900 m is light oil, and below 4,400 m is mainly condensate. Therefore, it can be inferred that condensate and natural gas reservoirs with poorer physical properties exist at greater depth (Figure 2).
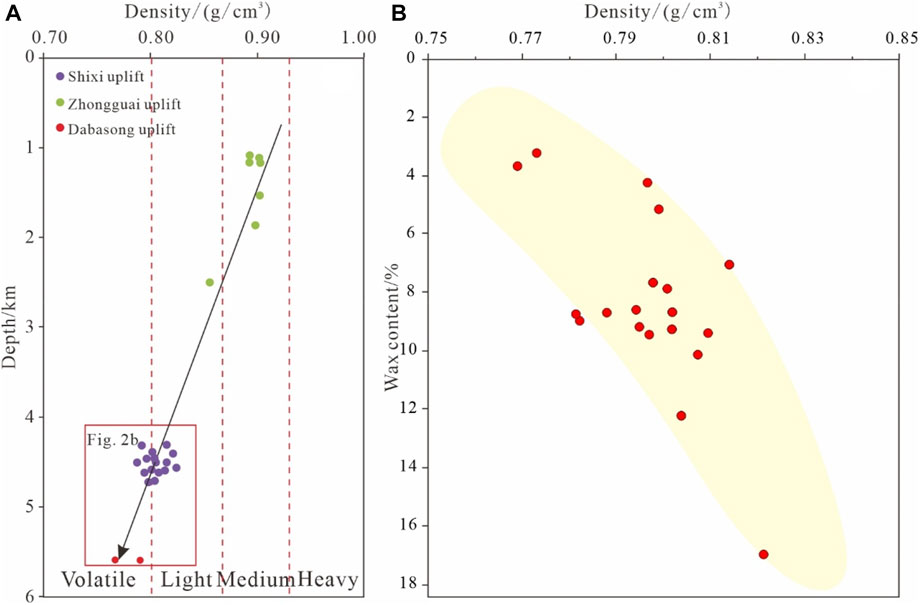
FIGURE 2. Oil density versus (A) depth and (B) wax content for Carboniferous crude oils in the Penyijingxi Sag.
The crude oil from the Zhongguai Uplift was derived from a mixture of Carboniferous and the lower Permian Fengcheng Formation (P1f) source rocks, and those from the Shixi and Mosuowan uplifts were derived from a mixture of Permian and Carboniferous source rocks. In general, the oils from the Zhongguai Uplift have pristane (Pr) with <nC17, phytane (Ph) with <nC18, high Ts/Tm ratios, and moderate β-carotene contents. The Pr and Ph contents are low, with Pr/nC17 < 1 (0.4–1.2), Ph/nC18 < 1 (0.2–1.0), pristane/phytane (Pr/Ph) = 0.8–1.2, and gammacerane index (gammacerane/C30 hopane) values of 0.2–0.6. Crude oils from the Shixi and Mosuowan uplifts have low Pr/Ph ratios, high Ts/Tm ratios, and low gammacerane and β-carotene contents. These crude oils have Pr/Ph < 1, gammacerane index values of < 0.2, and Ts/Tm = 0.4–1.2. Most of the samples do not contain β- or γ-carotene.
In addition, the crude oils contain a wide range of n-alkanes that are normally distributed, with light/heavy n-alkane ratios of >1.5. The n-alkanes exhibit no obvious odd–even predominance and the carbon preference index (CPI) is 1.0–1.2, which is indicative of mature oil. Pr/Ph values of 1.0–2.0 indicate that the source rocks formed in a reducing, lacustrine sedimentary environment. There are no clear differences in Pr/Ph values between the oil fields, although the deep oil in the northern part of the sag has slightly lower Pr/Ph values, Pr/Ph values in the Shixi oilfield are <1.2, and Pr/Ph values of oils from the other areas are 1.2–2.0. The crude oil biomarkers have similar characteristics in all three uplifts. C19 tricyclic terpene contents are lower than C20 and C21 tricyclic terpene contents, with a peak at C20, C21, and C23 tricyclic terpenes. The abundance of C24 tetracyclic terpenes is low and the abundance of the 25-norhopane series is high. The crude oils containing 25-norhopane have higher contents of tricyclic terpenes, Ts, and gammacerane, whereas those not containing 25-norhopane have lower contents of these biomarkers. C29 is the carbon number of the most abundant regular sterane, and the abundance of C28 sterane is slightly lower than that of C29. C27 sterane is low in abundance and diasteranes are almost absent. These biomarker characteristics are typical of Permian crude oil. The carbon isotopic (δ13C) compositions of the crude oils are light, with δ13C values of most crude oils varying from –30‰ to –29‰, but some condensates and light oils have values heavier than –29‰. The biomarker characteristics of the Carboniferous crude oils in this area are different from those in other areas of the Junggar Basin. For example, the crude oil from the northern slope of the Zhongguai Uplift in the western Penyijingxi Sag has high Pr and Ph contents with Pr/Ph ≤ 1, and low Ts and high β-carotene and gammacerane contents (e.g., the Jin 102 well). The crude oil from the southern Zhongguai Uplift has low Pr and Ph, high Ts, low β-carotene, and moderate gammacerane contents (e.g., the Jinlong 103 well). The crude oil in the northern Zhongguai Uplift typically has high β-carotene, low Pr and Ph, low Ts, and high gammacerane contents (e.g., the Jinlong 16 well) (Figure 3).
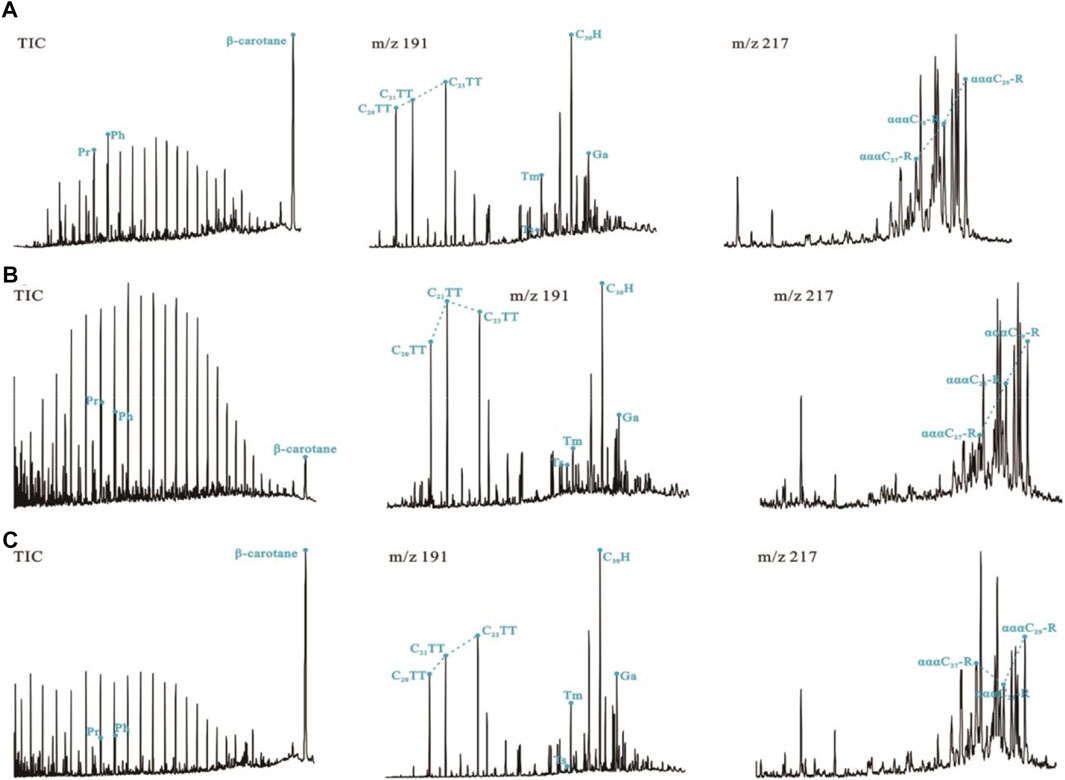
FIGURE 3. Biomarker chromatograms for Carboniferous crude oils in the Penyijingxi Sag. (A) Well Jin102, 1,470–1,510 m, Carboniferous, oil; (B) Well Jinlong103, 3,118–3,130 m, Carboniferous, oil; (C) Well Jinlong16, 2548–2556 m, Carboniferous, oil. Pr is pristane; Ph is phytane; C20TT is C20 tricyclic terpane; C21TT is C21 tricyclic terpane; C23TT is C23 tricyclic terpane; Ts is 18α(H)-22,29,30-trinorneohopane; Tm is 17α(H)-22,29,30-trinorhopane; C30H is C30 hopance; Ga is gammacerane; C27-R is C27 steranes; C28-R is C28 steranes; C29-R is C29 steranes.
3.2 Geochemistry and origins of the natural gas
Methane is the most abundant component in the natural gas in the study area, with contents of 60%–95%. The methane content decreases with increasing depth, and below 4,500 m is <80%. The ethane content of the natural gas is <10% and gradually increases with increasing depth. The propane content is <10%, and mostly <5%. The propane content increases gradually with burial depth. The dryness coefficient is a commonly used parameter to characterize the relative methane contents in natural gas. The dryness coefficient of natural gas in the study area is 0.7–1.0, and decreases gradually with increasing depth. However, the natural gas is generally wet (Figure 4).
In general, natural gas can be divided into oil-type, coal-type, and mixed gas, based on the hydrocarbon source materials (Dai, 1993). In the Shixi and Mobei oil fields, and Mosuowan gas field (Figure 5) the carbon isotopic compositions of the natural gas are variable. The carbon isotopic compositions of methane (δ13C1) vary from –42‰ to –33‰, and for ethane (δ13C2) from –27.5‰ to –25.0‰, indicating that the natural gas is generally mature.
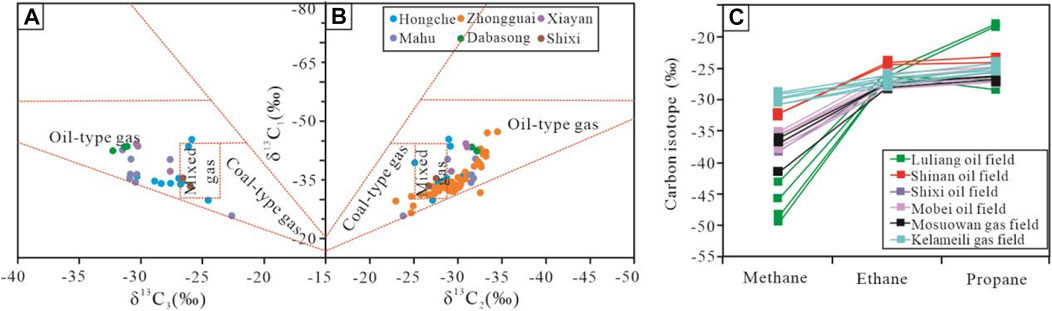
FIGURE 5. (A) Carbon isotopic compositions of propane (δ13C3) and (B) carbon isotopic compositions of ethane (δ13C2) versus carbon isotopic compositions of methane (δ13C1) in Carboniferous and Permian reservoirs in the Penyijingxi Sag; (C) Carbon isotopic compositions of natural gas in Carboniferous and Permian reservoirs in the Penyijingxi Sag.
The source of the natural gas is relatively complex. Based on the light hydrocarbons associated with natural gas in the Mobei and Mosuowan uplifts, it can be inferred the hydrocarbons were mainly derived from a mixture of lacustrine lower organisms and terrestrial higher organisms. The crude oils associated with gas in the Mosuowan Uplift have low Pr and Ph contents, low Ts/Tm ratios, low gammacerane contents, and a V-shaped distribution of C27–C28–C29 cholestanes (Figure 6). The biomarker characteristics of the light hydrocarbons and crude oils associated with the natural gas suggest that the natural gas in the Penyijingxi Sag was mainly derived from the Fengcheng Formation and other Carboniferous source rocks.
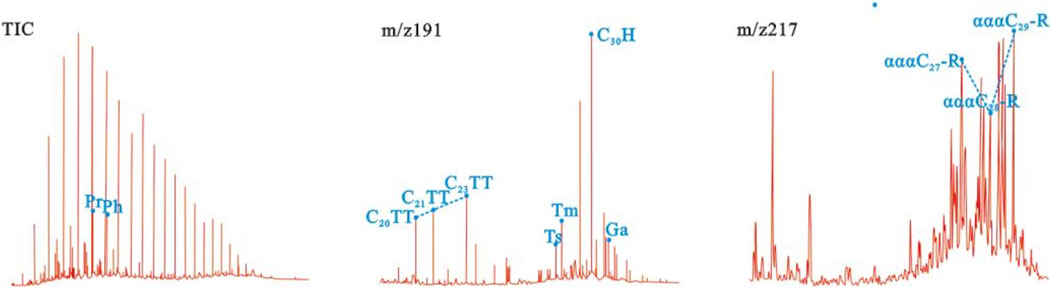
FIGURE 6. Biomarker spectra of natural gas and associated crude oils in the Mosuowan Uplift (Well Moshen1; 7209 m).
4 Factors controlling hydrocarbon accumulation
4.1 Hydrocarbon sources and degree of thermal evolution
Source rocks have a key role in hydrocarbon formation (Kuang et al., 2007; He et al., 2010a; He et al., 2010b; Wang et al., 2010; Zhao et al., 2011; Wang et al., 2012; Yang et al., 2014; Chen et al., 2016). The Penyijingxi Sag is surrounded by paleo-uplifts, which are close to the hydrocarbon generation sag. The oil–gas was derived from deep Carboniferous–Permian source rocks in the sag. In the early stages of exploration, shallow gas reservoirs (e.g., those in the Pen5 and Qianshao wells) were found in the Penyijingxi Sag. The gas was derived from a mixture of Permian and Carboniferous source rocks, and secondary gas reservoirs formed by the upward modification of deeper gas reservoirs (Cao et al., 2007; Ablimiti et al., 2019). More recent exploration of the central area of the sag has identified condensate reservoirs with high gas/oil ratios at depth. In particular, the Shixi 16 well at the eastern margin of the Penyijingxi Sag yielded high oil–gas flows from two Carboniferous intervals, which are large-scale condensate gas reservoirs. This indicates that from the margin to the center of the sag, the maturity of the crude oil increases gradually, the oil changes from light oil to condensate, and the proportion of natural gas increases. Therefore, condensate and gas reservoirs exist at depth. The density of crude oil in the Shixi 16 well is 0.80 g/cm3 and the dryness coefficient is 0.88. The density of crude oil in the Qianshao 2 well in the sag is 0.77 g/cm3 and the dryness coefficient is > 0.93. The gas/oil ratio increases gradually from the uplift area to the sag. As such, the oil–gas in the deep part of the paleo-uplift were affected by the distribution of source rocks and their maturity (Figure 7). In the central area, deep gas reservoirs are more likely to occur due to the high maturity of the source rocks.
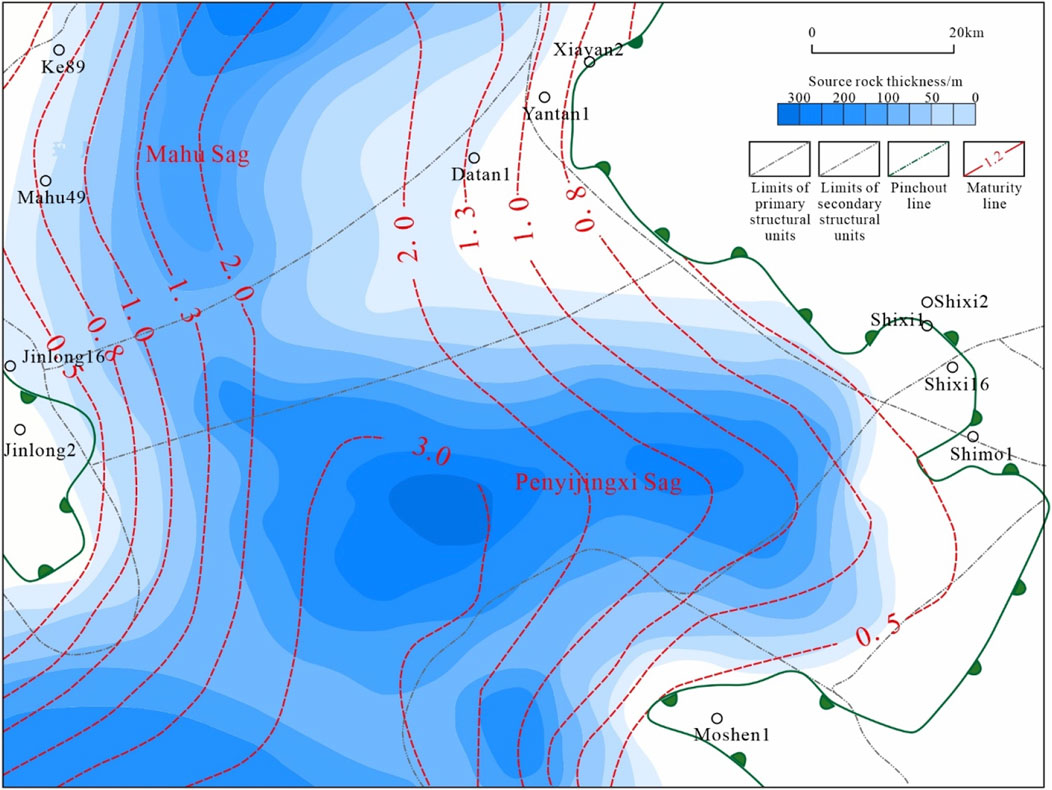
FIGURE 7. Map showing the source rock thickness and maturity in the Fengcheng Formation in the Penyijingxi Sag.
4.2 Source–reservoir rocks linked by faults
A comparison of the C29 sterane isomerization parameters for deep and shallow crude oils from the Moshen 1 well in the Mosuowan Uplift (Figure 8) shows that the C29 sterane ααα20S/(20S + 20R) ratios of the deep and shallow crude oils are similar. However, the C29 sterane ββ/(ββ+αα) ratio decreases with increasing depth. The former parameter is mainly affected by the degree of thermal evolution, which increases with increasing thermal maturity. However, the latter is affected not only by the degree of thermal evolution but also hydrocarbon migration. During oil–gas migration, the C29 sterane ββ/(ββ+αα) ratio increases with migration distance. Therefore, the deep and shallow crude oils have a similar maturity and source. However, the C29 sterane ββ/(ββ+αα) ratio increases gradually from the deep to shallow crude oil as a result of oil migration, which was controlled by faults (Figure 9).
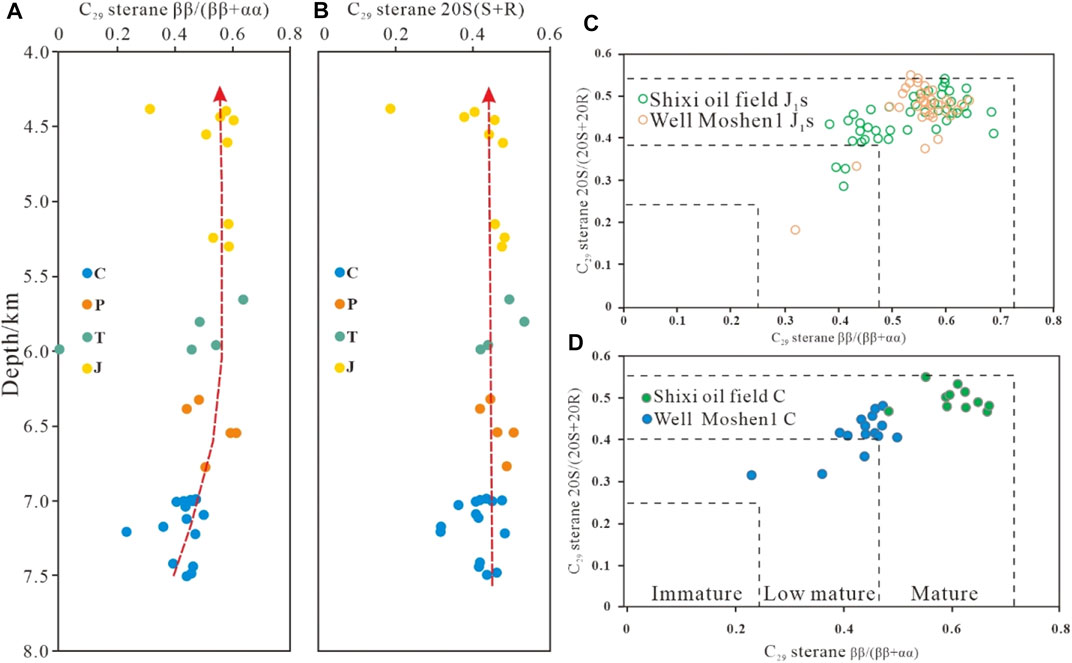
FIGURE 8. Sterane isomerization parameters of crude oils in the Penyijingxi Sag. (A) C29 sterane ββ/(ββ+αα) and (B) C29 sterane ααα20S/(20S + 20R) versus depth for deep and shallow crude oils from the Moshen1 well in the Mosuowan Uplift; C29 sterane ααα20S/(20S + 20R) versus C29 sterane ββ/(ββ+αα) for (C) Jurassic and (D) Carboniferous crude oils from the Shixi oilfield and Well Moshen1.
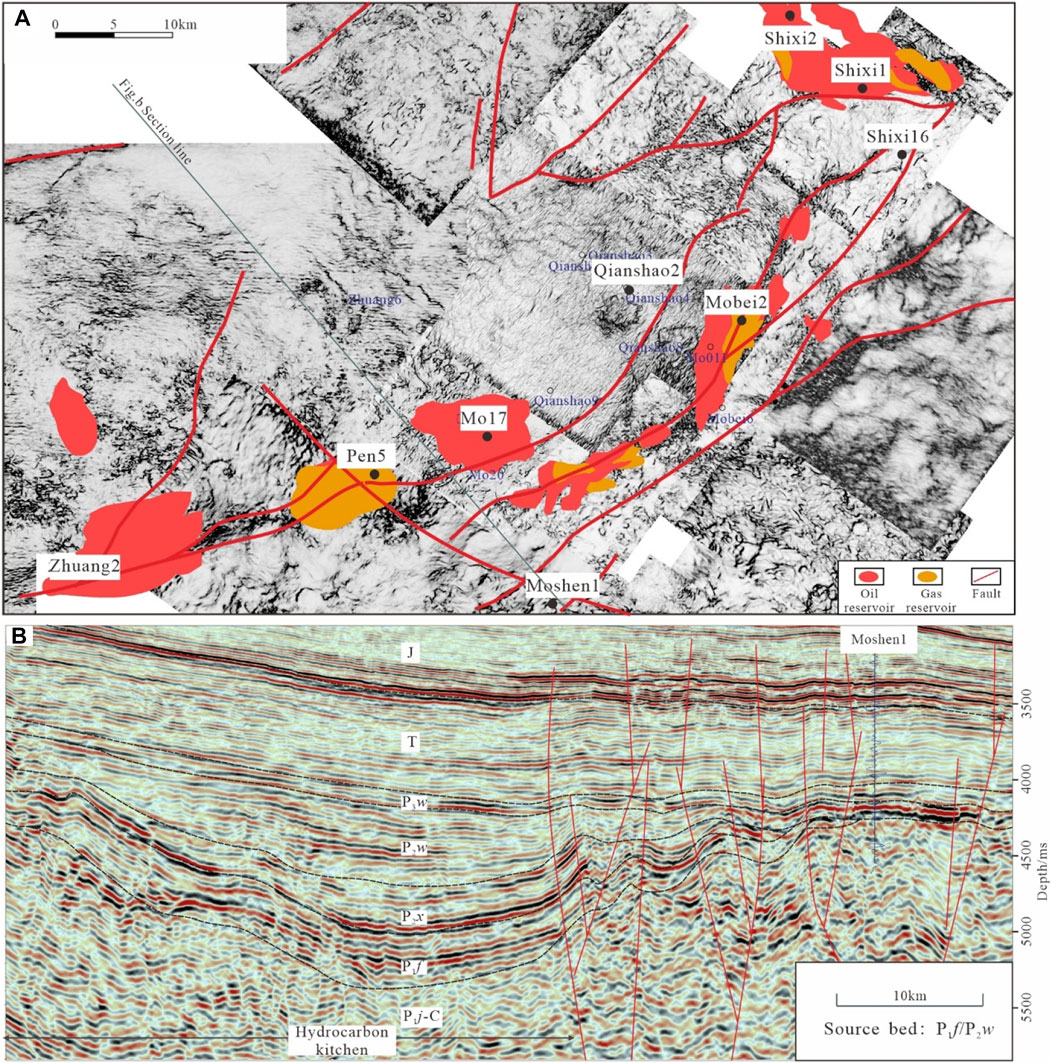
FIGURE 9. (A) Distribution of deep and large faults (basemap is the top of Carboniferous) and reservoir rocks, and an (B) interpreted seismic section of the source rock–fault relationships in the Penyijingxi Sag.
Based on the reservoir distribution and faults identified in previous studies, it is inferred that faults controlled the distribution of oil–gas reservoirs, leading to the formation of a belt of oil reservoirs in the northeastern Penyijingxi Sag (Figure 9A). Based on an analysis of the hydrocarbon accumulation factors in the deep reservoirs of the Shixi, Mobei, and Mosuowan oil–gas fields, the source–reservoir rocks are inferred to have been linked by faults. This linked the source and reservoir rocks and allowed the hydrocarbons to migrate into the reservoirs. Therefore, the reservoirs formed near the source rocks. If no late oil–gas charging occurred along faults, then hydrocarbon accumulation would have been limited. Typical examples of this are the Moshen1 and Shixi 16 wells. The Moshen1 well is located 28 km from the main hydrocarbon source rocks (Figure 9B), and the grains containing oil inclusions (GOI) value of the Carboniferous reservoir in the Moshen1 well are as high as 20%. This indicates that large-scale oil–gas accumulation has occurred. During the Yanshanian orogeny, which occurred after reservoir formation, the oil–gas at depth migrated to shallower levels along faults. Oil–source rock correlations and the timing of hydrocarbon accumulation indicate the crude oil in the Moshen 1 well was derived from the overlying Fengcheng Formation source rocks. There was only one stage of oil–gas charging, and oil–gas at depth were later modified (Wang et al., 2011). This might explain why the Moshen 1 well is not productive.
The Shixi Uplift underwent multiple stages of oil–gas charging from a variety of source rocks. The early oil–gas that accumulated in the Carboniferous reservoirs were also modified by the Yanshanian orogeny. High-mature oil–gas from the Lower Wuerhe Formation also charged and accumulated in the Carboniferous reservoirs. The maturity of the Carboniferous crude oil in the Shixi Uplift is much higher than that in the Mosuowan Uplift (i.e., the Moshen1 well). The residual Carboniferous oil–gas in the Moshen 1 well were low-mature in the early stages of charging and there was no later charging of high-mature oil–gas. Late-stage hydrocarbon accumulation involved near-source charging of mature and high-mature hydrocarbons.
4.3 Reservoir–cap rock relationships
The deep Carboniferous strata contain mainly volcanic rock reservoirs, which are less affected by compaction as compared with clastic and dolomitic reservoirs. The widely developed unconformity at the top of these strata, which formed by meteoric water leaching, improved the reservoir properties (Yao et al., 2011; Lei et al., 2013; He et al., 2017; Jin et al., 2018; Ablimiti et al., 2019). Porosity and permeability data show that the volcanic reservoirs at the top of the Carboniferous strata have the best physical properties, and the properties degrade strongly with increasing depth. The hydrocarbon reservoirs with porosity of >10% and permeability of >0.1 mD are located at a depth that is within 150 m of the unconformity at the top of the Carboniferous strata. The physical properties of the weathering crust on the volcanic rocks has affected the oil–gas distribution. A statistical analysis shows that oil production in different test intervals is positively correlated with the reservoir physical properties. For example, the daily oil production of the Shixi 2 and Shi00 5 wells is 19.49 and 31.89 t, respectively, whereas the daily oil production of the Xiayan 2 well is 11.36 t. The reservoir properties of the Shixi2 and Shi005 wells are much better than those of the Xiayan 2 well. In summary, reservoirs consisting of volcanic rocks with a weathering horizon were favorable for deep oil–gas enrichment in the Penyijingxi Sag of Central Depression, and good reservoir quality is essential for high production levels of oil–gas.
Most of the deep oil–gas reservoirs have experienced multi-stage tectonism, and contain light oil–gas. The cap rocks and extent of reservoir preservation controlled the deep oil–gas accumulation. A statistical analysis and comparison of the lithologies, physical properties, and thicknesses of the overlying cap rocks of discovered oil–gas reservoirs and unproductive wells in the western Central Depression shows that the overlying cap rocks of the high-yielding oil–gas reservoirs are thick mudstone. Most of the unproductive wells do not have mudstone cap rocks, and their reservoirs are directly overlain by sandstone, conglomerate, and volcanic rocks. These latter cap rocks have a poor sealing ability, and thus the oil–gas was not preserved in the reservoirs. For the Xiayan 2 well in the Xiayan Uplift, the two Carboniferous test intervals yielded 11.4 and 12.7 t/day of oil, and the Carboniferous reservoirs are directly overlain by 60 m of mudstone and marl of the Fengcheng Formation. The mudstone cap rocks of the Fengcheng Formation and Carboniferous volcanic reservoir rocks form a good reservoir–cap rock assemblage. The four Carboniferous test intervals in the Shixi 1 well yielded 82.9, 67.4, 41.5, and 16.8 t/day of oil. The cap rock of the volcanic reservoir in this well comprises 112 m of mudstone of the Karamay Formation.
In summary, deep reservoir formation and the reservoir types were controlled by the thermal evolution of the source rocks, which generated oil–gas. In addition, source–reservoir linkages and vertical hydrocarbon migration through faults were important for efficient hydrocarbon accumulation in the Carboniferous reservoirs. A comparison of the Shixi oilfield, Shixi 16 gas reservoir, Shimo1 gas field, Pan 5 gas field, and Moshen 1 well confirmed that the migration distance between the source rocks and hydrocarbon traps also affected the accumulation of high-mature oil–gas, due to differences in the accumulation process and multi-stage charging.
5 Hydrocarbon migration and accumulation patterns
5.1 Analog modeling of reservoir formation
Based on the above analysis of the factors controlling hydrocarbon accumulation and the example of the Shixi Uplift, analog modeling of deep oil–gas migration and accumulation were undertaken to investigate the formation of deep oil- and gas-bearing reservoirs. The experiments were conducted at the PetroChina Research Institute of Petroleum Exploration and Development—Northwest Branches. These experiments are the basis for the establishment of a deep hydrocarbon accumulation model.
Using the Penyijingxi Sag and northeastern Shixi and Mobei uplifts as examples, analog modeling of hydrocarbon injection and accumulation was designed at scale of 1:10000 based on the actual structural geology (Figures 10A,B). The oil–gas injection point corresponds to the Penyijingxi Sag; the F1 oil–gas migration pathway corresponds to the Shixi Fault; the F2 oil–gas migration pathway corresponds to the Mobei Fault; the No. 1 volcanic rock body represents the Shixi oil field; the No. 2 volcanic rock body represents the oil–gas reservoir in the Shixi 16 well; and the No. 3 volcanic rock body represents the uncharged trap in the Shimo1 well. In the sandbox model, 400 mesh glass beads were used to represent the Carboniferous weathering crust reservoir and fault channels (particle size of 0.038 mm and 15% porosity); silica powder was used to represent the cap rocks; and brown corundum powder was used to represent the overlying strata. The initial experimental model was saturated with CaCl2 with a salinity of 21,303.6 mg/L, similar to the formation waters in the Shixi 16 well. The sandbox model was left to settle for 12 h, and then oil injection was carried out. The white oil was stained with Sudan red. The oil density was 824 kg/cm3, oil viscosity was 6.266 mPa s, oil surface tension was 27.8 mN/m, oil injection rate was 2.0 ml/min, and oil injection duration was 5 h. Subsequent gas injection (i.e., air) began after 5 h at a rate of 2.0 ml/min. The process of oil–gas migration and accumulation were recorded by taking photographic images during the experiment.
Oil and gas accumulation in the Shixi Uplift involved early oil charging and late high-mature natural gas charging. Therefore, the analog modeling of oil–gas migration and accumulation were conducted in two stages. At the beginning of the experiments, the sandbox reservoir was saturated with formation waters and the source channel was saturated with crude oil, which was charged at a constant rate of 2.0 ml/min. After 50 min, the oil migrated upwards along the F1 and F2 faults. After 100 min, the F1 and F2 faults were filled with oil, and crude oil began to accumulate in the No. 2 rock body. After 150 min, the F1 fault gradually became saturated with crude oil, and the No. 1 rock body began to charge. The No. 1 and 2 rock bodies exhibited oil–water displacement and differentiation, with crude oil preferentially migrating along the bottom of the trap. After 200 min, the No. 2 rock body was filled with oil. After 250 min, the No. 2 rock body was saturated with crude oil and oil began to overflow from the trap, and the No. 1 rock body was nearly filled with oil. The No. 3 rock body was not charged with crude oil during the entire process (Figure 11). The gas injection began at 300 min. The gas migration rate was much higher than that of the crude oil, and gas migrated rapidly along the top of the reservoir and hanging wall of the fault. After 350 min, the color of the upper part of the F1 fault and entire F2 fault became lighter due to gas filling. In addition, the color at the top of the No. 2 rock became lighter, but gas was not saturated in the rock body. After 400 min, the color of the No. 2 rock body became lighter, the degree of gas filling increased, the gas–oil interface migrated downward, the height of the gas column increased, and an oil ring formed. The color of the No. 1 rock body began to become lighter. After 450 min, the color of the No. 2 rock body stabilized and gas was saturated in the rock body. The gas charging in the No. 1 rock body continued, but the gas migration efficiency and saturation of the trap were low. With further gas filling, the model changed little and became stable, and the experiment was stopped.
The analog modeling of oil–gas migration and accumulation exhibited some similarities and differences with observations in the Shixi Uplift. Vertical migration of crude oil occurred along the faults in the model, but lateral migration was limited. The migration efficiency of the natural gas was high, and involved rapid and unidirectional diffusion. The migration of the deep oil and natural gas showed characteristics of near-source accumulation. After early oil has accumulated and formed charged reservoirs, late natural gas charging can drive oil migration if the cap rock is effective. This gradually transforms the oil reservoir into a gas reservoir. In general, deep oil–gas accumulation produced large-scale oil reservoirs in higher traps, which are underlain by oil–gas reservoirs. Large-scale gas reservoirs occur at depth, close to the source rocks.
5.2 Model of hydrocarbon accumulation and implications for exploration
Based on the factors that controlled hydrocarbon accumulation and the analog modeling of migration and accumulation, the paleo-uplifted regions around the Penyijingxi Sag are inferred to have been favorable sites for hydrocarbon accumulation. We propose a model of deep reservoir formation for the western Central Depression. Most of the deep oil–gas migrated laterally and converged in the paleo-uplifts, which may have moved upward due to tectonism. The preservation conditions of the early oil–gas reservoirs and whether there was large-scale, late oil–gas charging were key factors for the deep accumulation of oil–gas. Based on differences of source-reservoir relationship in the different structural belts, Carboniferous oil–gas accumulation in the Penyijingxi Sag and adjacent strata can be divided into two types.
The first type involves the source and reservoir rocks being connected over a long distance by faults, in which the source rocks have a large hydrocarbon window. Typical examples of this are the Mosuowan, Mobei, and Shixi uplifts, which are connected to hydrocarbon generation sags by large faults. These faults directly link Carboniferous reservoir and Permian source rocks, which was favorable for hydrocarbon accumulation. The hydrocarbon window of the Carboniferous reservoir and Fengcheng Formation source rocks in the Shixi Uplift encompasses 700 m, and that of the lower Wuerhe Formation source rocks is 500 m. The oil–gas generated by these two sets of source rocks migrated and accumulated in Carboniferous volcanic reservoirs. The large hydrocarbon windows in the Mosuowan and western Mobei uplifts are also similar to this type (Figure 12A). For this type of accumulation, the hydrocarbon window of the source rocks is large and the hydrocarbon charging is strong. After the early oil–gas was dispersed, later oil–gas charging occurred again. The Shixi oilfield reservoir in the Shixi Uplift is a typical example of a late-charging reservoir. The reservoir and source rocks on the western side of the Mosuowan Uplift show a much better lateral alignment than those in the Moshun 1 well.
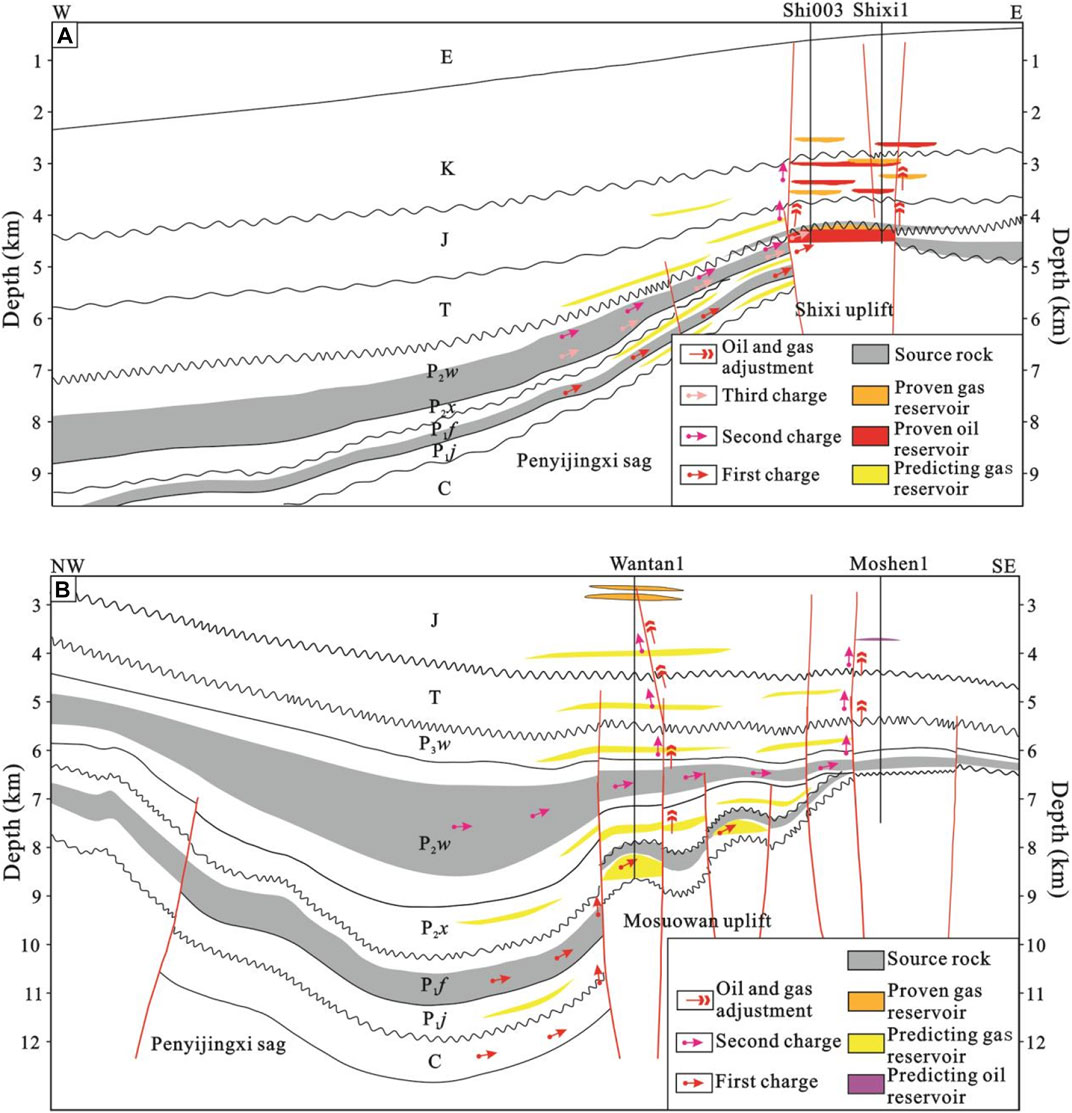
FIGURE 12. Patterns of oil–gas accumulation in deep Carboniferous reservoirs of the Junggar Basin. (A) Shixi Uplift; (B) Mosuowan Uplift. See Figure 1 for location.
The second type is where a paleo-uplift is bounded by hydrocarbon-generating sags. For example, the Dabasong Uplift is located between two hydrocarbon-rich sags (i.e., the Mahu and Penyijingxi sags; Figure 12B). Large faults that controlled the structural development occur on both sides of the uplift, and the reservoirs are laterally connected to the Carboniferous source rocks (i.e., the Jiamuhe, Fengcheng, and Lower Wuerhe formations). Large-scale oil–gas reservoirs formed due to the long-term presence and high abundance of oil–gas. In this type of accumulation, hydrocarbons are supplied to the paleo-uplift from two sides, and earlier charged hydrocarbons may have been displaced upwards and dissipated due to the effects of the Hercynian orogeny. Subsequently, hydrocarbon charging occurred again, cap rocks formed, and the oil–gas preservation conditions were good.
There are five paleo-uplifts developed around the Penyijingxi Sag, which are the Zhongguai, Mosuowan, Mobei, Shixi, and Dabasong uplifts. The Carboniferous source rocks in the nose-salient and depression area are connected over a wide area. The hydrocarbon source rocks in the depression areas generated oil–gas, and these areas were intersected by deep faults and surrounded by paleo-uplifts. This situation was favorable for lateral, near-source hydrocarbon accumulation. High-resolution seismic data indicate a favorable exploration area of nearly 700 km2 over six anticlinal structures. At present, these targets have not all been explored, except for the Shixi uplift and Shixi 16 well. In addition to the advantage of near-source accumulation and direct linkage via deep and large faults, the Permian and Carboniferous source rocks in the Penyijingxi Sag have entered the large-scale gas generation stage. Based on the thermal and hydrocarbon generation history of source rocks in the Fengcheng Formation, the gas generation at depths of >6,500 m might be as high as 20 × 108 m3/km2. The source rocks in the Lower Wuerhe Formation are mainly a humic type, with a lower maturity than the Fengcheng Formation and a greater capacity for gas generation. The early low-mature to mature crude oil and middle–late high-maturity light oil–gas were continuously charged into the Carboniferous paleo-nose salient to form light condensate reservoirs. Due to rapid burial in the Jurassic, anomalously high pressure exists in pre-Jurassic strata in the Penyijingxi Sag, which makes the Carboniferous oil–gas reservoirs highly productive and facilitates effective development. In 2021, the Shixi 161H horizontal well was drilled around the Shixi 16 well. The horizontal section of the well runs along the reservoir at the top of the Carboniferous strata. The horizontal section is 500 m long and, after fracturing, the daily oil–gas production is 2000 t. This indicates that the Carboniferous structures are important exploration targets, despite their great burial depth.
6 Conclusion
The crude oil in the deep Carboniferous reservoirs of the Junggar Basin is mainly light–medium oil, and the natural gas is wet. The hydrocarbons were derived from Permian and Carboniferous source rocks. The source rocks and their thermal evolution controlled deep hydrocarbon accumulation, and source–reservoir rock linkages via faults allowed vertical hydrocarbon migration and multiple stages of hydrocarbon charging. Good reservoir-cap rock assemblages were important for the preservation of the deep oil–gas reservoirs. The deep Carboniferous paleo-uplifts in the Central Depression of the Junggar Basin were favorable sites for oil–gas accumulation.
The preservation conditions of the early oil–gas reservoirs and whether later, large-scale oil–gas charging occurred were key factors for the deep oil–gas accumulation. The Junggar Basin contains two types of deep reservoir: those linked to source rocks by large-scale faults and those in paleo-uplifts surrounded by oil-generating depressions. Potential exploration targets are the Mosuowan, Shixi, and Dabasong uplifts, although these are high risk.
Data availability statement
The original contributions presented in the study are included in the article/supplementary material, further inquiries can be directed to the corresponding author.
Author contributions
YA: Writing—original draft, Data curation, and Formal analysis. WH: Methodology, and Data curation. NL: Resources. DM: Methodology, and Resources. HL: Visualization, and Investigation. BB: Resources. XD: Methodology, and Data curation. MJ: Resources. JW: Resources. JC: Writing—review and; editing, Conceptualization, Data curation, Funding acquisition, and Supervision.
Acknowledgments
We thank editors and reviewers for insightful comments in improving the manuscript. This work was funded by PetroChina Science and Technology Major Project (Grant Nos. 2019E-2602 and 2021DJ0108).
Conflict of interest
YA, WH, NL, HL, BB, MJ, and JW was empolyed by PetroChina Xinjiang Oilfield Company.
The remaining authors declare that the research was conducted in the absence of any commercial or financial relationships that could be construed as a potential conflict of interest.
Publisher’s note
All claims expressed in this article are solely those of the authors and do not necessarily represent those of their affiliated organizations, or those of the publisher, the editors and the reviewers. Any product that may be evaluated in this article, or claim that may be made by its manufacturer, is not guaranteed or endorsed by the publisher.
References
Ablimiti, Y. M., Zha, M., Yang, F., Yin, H., Ding, X. J., and Bian, B. L. (2019). Carboniferous igneous reservoir distribution and its controlling factors in Mahu-Dabasong Area, Junggar Basin. Xinjiang Pet. Geol. 40, 564–569. doi:10.7657/XJPG20190509
Allen, M. B., and Vincent, S. J. (1997). Fault reactivation in the junggar region, northwest China: The role of basement structures during mesozoic-cenozoic compression. J. Geol. Soc. Lond. 154, 151–155. doi:10.1144/gsjgs.154.1.0151
Cao, J., Hu, W. X., Yao, S. P., Zhang, Y. J., Wang, X. L., Zhang, Y. Q., et al. (2007). Carbon, oxygen and strontium isotope composition of calcite veins in the carboniferous to Permian source sequences of the Junggar Basin: Implications on petroleum fluid migration. Acta Sedimentol. Sin. 25, 722–729.
Cao, J., Xia, L., Wang, T., Zhi, D., Tang, Y., and Li, W. (2020). An alkaline lake in the late paleozoic ice age (lpia): A review and new insights into paleoenvironment and petroleum geology. Earth-Science Rev. 202, 103091. doi:10.1016/j.earscirev.2020.103091
Chen, F. J., Wang, X. W., and Wang, X. W. (2005). Prototype and tectonic evolution of the Junggar Basin, northwestern China. Earth Sci. Front. 12, 77–89. doi:10.3321/j.issn:1005-2321.2005.03.010
Chen, J. P., Wang, X. L., Deng, C. P., Liang, D. G., Zhang, Y. Q., Zhao, Z., et al. (2016). Geochemical features of source rocks and crude oil in the Junggar Basin, Northwest China. Acta Geol. Sin. 90, 37–67. doi:10.3969/j.issn.0001-5717.2016.01.003
Chen, X., Lu, H. F., Shu, L. S., Wang, H. M., and Zhang, G. Q. (2002). Study on tectonic evolution of Junggar basin. Geol. J. China Univ. 8, 257–267. doi:10.3969/j.issn.1006-7493.2002.03.003
Dai, J. X. (1993). Carbon and hydrogen isotope characteristics of natural gas and identification of different types of natural gas. Nat. Gas. Geosci. 4, 1–40.
Du, J. H., Zhi, D. M., Li, J. Z., Yang, D. S., Tang, Y., Qi, X. F., et al. (2019a). Major breakthrough of Well Gaotan 1 and exploration prospects of lower assemblage in southern margin of Junggar Basin, NW China. Petroleum Explor. Dev. 46, 216–227. doi:10.1016/s1876-3804(19)60003-0
Du, J. H., Zhi, D. M., Tang, Y., Jia, C. M., Xu, Y., and Ablimiti, Y. M. (2019b). Prospects in upper permian and strategic discovery in shawan sag, Junggar basin. China Pet. Explor. 24, 24–35. doi:10.3969/j.issn.1672-7703.2019.01.004
He, D. F., Chen, X. F., Kuang, J., Yuan, H., Fan, C., Tang, Y., et al. (2010b). Distribution of Carboniferous source rocks and petroleum systems in the Junggar Basin. Petroleum Explor. Dev. 37, 397–408. doi:10.1016/s1876-3804(10)60041-9
He, D. F., Chen, X. F., Kuang, J., Yuan, H., Wu, X. Z., Du, P., et al. (2010a). Characteristics and exploration potential of Carboniferous hydrocarbon plays in Junggar Basin. Acta Pet. Sin. 31, 1–11. doi:10.7623/syxb201001001
He, D. F., Zhang, L., Wu, S. T., Li, D., and Zhen, Y. (2018). Tectonic evolution stages and features of the Junggar Basin. Oil Gas Geol. 39, 845–861. doi:10.11743/ogg20180501
He, H. Q., Zhi, D. M., Tang, Y., Liu, C. W., Chen, H., Guo, X. G., et al. (2021). A great discovery of well kangtan 1 in Fukang sag in the Junggar basin and its significance. China Pet. Explor. 26, 1–11. doi:10.3969/j.issn.1672-7703.2021.02.001
He, X. Y., Liu, Y., Xu, X. L., Liu, B. X., and Zhang, S. C. (2017). Controlling factors of carboniferous volcanic reservoirs and favorable reservoir prediction in Xiquan area, Junggar Basin. Lithol. Reserv. 29, 42–51. doi:10.3969/j.issn.1673-8926.2017.03.006
Hu, S. Y., Wang, X. J., Cao, Z. L., Li, J. Z., Gong, D. Y., and Xu, Y. (2020). Formation conditions and exploration direction of large and medium gas reservoirs in the Junggar Basin, NW China. Petroleum Explor. Dev. 47, 266–279. doi:10.1016/s1876-3804(20)60045-3
Jia, C. Z., and Pang, X. Q. (2015). Research processes and main development directions of deep hydrocarbon geological theories. Acta Pet. Sin. 12, 1457–1469. doi:10.7623/syxb201512001
Jin, J., Wang, J., Yang, Z., Liu, J., Ji, H. C., Jia, H. B., et al. (2018). Well logging identification of Carboniferous volcanic inner buried-hill reservoirs in Ke-Bai fault zone in Junggar Basin. Lithol. Reserv. 30, 85–92. doi:10.12108/yxyqc.20180210
Kuang, L. C., Xue, X. K., Zou, C. N., and Hou, L. H. (2007). Oil accumulation and concentration regularity of volcanic lithostratigraphic oil reservoir: A case from upper-plate carboniferous of KA-Bai fracture zone, Junggar basin. Petroleum Explor. Dev. 34, 285. doi:10.3321/j.issn:1000-0747.2007.03.003
Lei, D. W., Yang, D. S., Chen, Z. H., Gu, X. P., Liu, W., and Luo, X. P. (2013). Reservoir space characteristics of carboniferous volcanic rocks in the central-east part of Junggar basin, NW China. Xinjiang Geol. 31, 231–235. doi:10.3969/j.issn.1000-8845.2013.03.017
Li, D., He, D. F., Santosh, M., Ma, D. L., and Tang, J. Y. (2015a). Tectonic framework of the northern Junggar basin part I: The eastern Luliang uplift and its link with the east junggar terrane. Gondwana Res. 27, 1089–1109. doi:10.1016/j.gr.2014.08.015
Li, D., He, D. F., Santosh, M., and Ma, D. L. (2015b). Tectonic framework of the northern Junggar Basin Part II: The island arc basin system of the Western Luliang Uplift and its link with the West Junggar terrane. Gondwana Res. 27, 1110–1130. doi:10.1016/j.gr.2014.08.019
Li, D., He, D. F., Tang, Y., Fan, C., and Kong, Y. H. (2012). Genesis of early carboniferous volcanic rocks of the di'nan uplift in Junggar basin: Constraints to the closure time of kalamaili ocean. Acta Petrol. Sin. 28, 2340–2354.
Liu, Y., Wu, K. Y., Wang, X., Liu, B., Guo, J. X., and Du, Y. N. (2017). Architecture of buried reverse fault zone in the sedimentary basin: A case study from the hong-che fault zone of the Junggar basin. J. Struct. Geol. 105, 1–17. doi:10.1016/j.jsg.2017.11.002
Lu, H. T., Xia, H. P., Chen, Z. H., Lai, S. X., Zhang, Y., and Mao, H. B. (2013). Stratigraphic division, correlation and distribution of the Carboniferous system in the Junggar Basin. J. Stratigr. 37, 353–360.
Ma, Y. S., Cai, X. Y., and Zhao, P. R. (2018). China's shale gas exploration and development: Understanding and practice. Petroleum Explor. Dev. 45, 589–603. doi:10.1016/s1876-3804(18)30065-x
Pang, X. Q., Jia, C. Z., and Wang, W. Y. (2015). Petroleum geology features and research developments of hydrocarbon accumulation in deep petroliferous basins. Pet. Sci. 12, 1–53. doi:10.1007/s12182-015-0014-0
Tang, Y., Cao, J., He, W. J., Guo, X. G., Zhao, K. B., and Li, W. W. (2021). Discovery of shale oil in alkaline lacustrine basins: The late paleozoic Fengcheng Formation, Mahu sag, Junggar basin, China. Petroleum Sci. 18, 1281–1293. doi:10.1016/j.petsci.2021.04.001
Wang, B., Wu, M., Wang, X. L., Zhang, Y. Q., and Cao, J. (2011). Source rock features and evaluation of Triassic strata in the central Junggar basin. J. Southwest Petroleum Univ. 33, 12–20. doi:10.3863/j.issn.1674-5086.2011.02.002
Wang, X. L., Zha, M., Xia, H. P., Chen, Z. H., Kong, Y. H., and Jiang, R. F. (2012). Forecast and assessment of oil and gas resources in Carboniferous, northern Xinjiang. Adv. Earth Sci. 27, 80–85.
Wang, X. L., Zhao, M. J., Xiang, B. L., Da, J., Jiang, Y. Q., and Liu, C. M. (2010). Carboniferous source rocks in the Ludong-Wucaiwan area, Junggar basin, NW China. Petroleum Explor. Dev. 37, 523–530. doi:10.1016/s1876-3804(10)60052-3
Wang, Y. J., Jia, D., Pan, J. G., Wei, D. T., Tang, Y., Wang, G. D., et al. (2018). Multiple-phase tectonic superposition and reworking in the Junggar Basin of northwestern China-Implications for deep-seated petroleum exploration. Am. Assoc. Pet. Geol. Bull. 102, 1489–1521. doi:10.1306/10181716518
Wu, K. Y., Zha, M., Wang, X. L., Qu, J. X., and Chen, X. (2005). Further researches on the tectonic evolution and dynamic setting of the Junggar basin. Acta Geosci. Sin. 26, 217–222. doi:10.3321/j.issn:1006-3021.2005.03.004
Xia, L., Cao, J., Bian, L., Hu, W., Wang, T., Zhi, D., et al. (2022). Co-evolution of paleo-environment and bio-precursors in a Permian alkaline lake, Mahu mega-oil province, Junggar Basin: Implications for oil sources. Sci. China Earth Sci. 65, 462–476. doi:10.1007/s11430-021-9861-4
Xia, L., Cao, J., Lee, C., Stüeken, E. E., Zhi, D., and Love, G. D. (2020a). A new constraint on the antiquity of ancient haloalkaliphilic green algae that flourished in a ca. 300 Ma Paleozoic lake. Geobiology 19, 147–161. doi:10.1111/gbi.12423
Xia, L., Cao, J., Stüeken, E. E., Zhi, D., Wang, T., and Li, W. (2020b). Unsynchronized evolution of salinity and pH of a permian alkaline lake influenced by hydrothermal fluids : A multi-proxy geochemical study. Chem. Geol. 541, 119581. doi:10.1016/j.chemgeo.2020.119581
Yang, H. B., Xiang, B. L., Bao, H. J., and Pang, H. (2014). Hydrocarbon resources potential in carboniferous igneous inner reservoirs in Ludong Area of Junggar Basin. Xinjiang Pet. Geol. 35, 1–4.
Yao, W. J., Fan, C. H., Dang, Y. F., Qin, Q. R., and Zhao, L. (2011). Characteristics and main control factors of Carboniferous volcanic reservoirs of Zhongguai uplift in the northeastern margin of Junggar Basin. J. Oil Gas Technol. 33, 32–36. doi:10.3969/j.issn.1000-9752.2011.09.007
Zhang, D. D., Liu, W. H., Wang, X. F., Luo, H. Y., Wang, Q. T., Li, Y. N., et al. (2021). Genetic types and characteristics of deep oil and gas plays. Oil Gas Geol. 42, 1169–1180. doi:10.11743/ogg20210514
Zhao, M. J., Wang, X. L., Da, J., Xiang, B. L., Song, Y., and Qin, S. F. (2011). Genetic origin of natural gas and its filling history in Dinan Uplift-Wucaiwan of Junggar Basin. Nat. Gas. Geosci. 22, 595–601.
Zheng, M., Li, J. Z., Wu, X. Z., Wang, S. J., Guo, Q. L., Yu, J. D., et al. (2018). China's conventional and unconventional natural gas resources: Potential and exploration targets. J. Nat. Gas Geoscience 3, 295–309. doi:10.1016/j.jnggs.2018.11.007
Zhi, D. M., Song, Y., He, W. J., Jia, X. Y., Zou, Y., and Huang, L. L. (2019). Geological characteristics, resource potential and exploration direction of shale oil in middle-lower permian, Junggar basin. Xinjiang Pet. Geol. 40, 389–401. doi:10.7657/XJPG20190402
Zhi, D. M., Tang, Y., He, W. J., Guo, X. G., Zheng, M. L., and Huang, L. L. (2021). Orderly coexistence and accumulation models of conventional and unconventional hydrocarbons in lower permian Fengcheng Formation, Mahu sag, Junggar basin. Petroleum Explor. Dev. 48, 43–59. doi:10.1016/s1876-3804(21)60004-6
Keywords: hydrocarbon accumulation mechanism, deep strata, analog modeling, fault, Carboniferous, Junggar Basin
Citation: Ablimiti Y, He W, Li N, Ma D, Liu H, Bian B, Ding X, Jiang M, Wang J and Cao J (2022) Mechanisms of deep oil–gas accumulation: New insights from the Carboniferous Central Depression, Junggar Basin, China. Front. Earth Sci. 10:987822. doi: 10.3389/feart.2022.987822
Received: 06 July 2022; Accepted: 30 August 2022;
Published: 21 September 2022.
Edited by:
Hao Zou, Chengdu University of Technology, ChinaReviewed by:
Xiaoqi Wu, SINOPEC Petroleum Exploration and Production Research Institute, ChinaPiotr Krzywiec, Institute of Geological Sciences (PAN), Poland
Copyright © 2022 Ablimiti, He, Li, Ma, Liu, Bian, Ding, Jiang, Wang and Cao. This is an open-access article distributed under the terms of the Creative Commons Attribution License (CC BY). The use, distribution or reproduction in other forums is permitted, provided the original author(s) and the copyright owner(s) are credited and that the original publication in this journal is cited, in accordance with accepted academic practice. No use, distribution or reproduction is permitted which does not comply with these terms.
*Correspondence: Jian Cao, jcao@nju.edu.cn; Yiming Ablimiti, ablmt@petrochina.com.cn