- Tianjin branch of CNLC Logging Co. LTD., Tianjin, China
Natural gas hydrates, which only occur in geological environments with special conditions, are characterized by physical properties unique from those of oil and gas, rendering current methods of measuring saturation, such as the conventional Archie formula or the sonic velocity method, inaccurate. Therefore, to obtain a reliable saturation value of natural gas hydrates, we propose a two-parameter calculation model of resistivity and acoustic interval transit time based on logging data. The saturation results calculated using the two-parameter calculation model were far more accurate than those of the core experiment results, with the proposed model having an average relative error of 7%, whereas that of the conventional Archie formula was 24%. These results indicate that the logging two-parameter model effectively improves the calculation accuracy of gas hydrate saturation. The saturation calculation model is based on objective theory and has wide adaptability, which provides a reliable foundation for future hydrate resource evaluation, exploration, and development in the study area.
Introduction
Natural gas hydrate, also known as solid methane, is an ice-like solid compound formed from hydrocarbon gas molecules, such as methane or water molecules, under low temperature and high pressure. Due to its occurrence under special environmental conditions and physical properties unique from oil and gas, resource evaluation and development of natural gas hydrates is challenging. Specifically, the current quantitative evaluation of gas hydrate reservoir parameters basically follows the theoretical method of oil and gas exploration, which can produce inaccurate results due to the property differences in natural gas hydrates versus oil and gas. Logging technology can directly obtain the information of in-situ formation and is one of the most effective methods used currently to identify and evaluate natural gas hydrate reservoirs (Wang et al., 2003; Murray et al., 2006; Collet, 2013; Zhong et al., 2020). The most common methods for calculating saturation parameters of natural gas hydrates are the resistivity method and the sonic velocity method (Guo et al., 2011; Su et al., 2014; Xiao and Bai., 2015; Zhu et al., 2019). The resistivity method requires the determination of the rock-electrical parameters of the reservoir and then use the Archie formula to calculate the saturation. The sonic velocity method is used to calculate saturation based on sonic velocity measurements of hydrated sediments, which is then combined with empirical or petrophysical models (Wang et al., 2010; Sun et al., 2011). However, natural gas hydrates decompose readily from the in-situ formation, making it difficult to obtain rock electrical parameters through experimentation. In addition to the influence of hydrate saturation, the sonic velocity of hydrate-bearing reservoirs is also sensitive to the limestone composition, compaction degree, and microscopic distribution pattern of hydrate in sediments. Thus, the loose and unconsolidated silty clay distribution of the marine hydrate reservoir lends to possible error. In this study, we propose a two-parameter logging model to calculate natural gas hydrate saturation by studying the logging data of several natural gas hydrates in the South China Sea, in an effort to improve the reliability and accuracy of hydrate saturation parameters.
Logging the response characteristics of natural gas hydrate
Lithology of the hydrate reservoir in the Shenhu area of South China Sea is mainly clay siltstone with loose and unconsolidated strata (Zhang et al., 2017). The range of logging depth is about 30–235 m below the seabed. Conventional logging curves are generally constructed based on parameters such as hole diameter, gamma ray, resistivity, acoustic interval transit time, compensated neutron, and compensated density. Figure 1 shows a partial LWD curve of well SH**in the study area. The hydrate-rich section of the reservoir is located at 133–155 m. Compared with the upper and lower strata without hydrates, the curve response features are as follows: lower gamma ray, significantly higher resistivity, lower compensative neutrons, higher density, and significantly shorter acoustic interval transit time. Additionally, the hydrate-rich stratus is highlighted in the micro-resistivity scanning image. The hydrate saturation data points obtained from the core experiment are also in good agreement with them. These characteristics, along with support from the hydrate saturation data points obtained from the core experiment, clearly indicate the existence of hydrate.
High resistivity and low acoustic interval transit time are the most typical characteristics of a hydrate reservoir. Natural gas hydrate is similar to ice, and the process of freezing may result in a “salt elimination” effect. Therefore, pure natural gas hydrate is not conductive, and the formation of hydrate occupies a certain formation pore, which hinders the migration of conductive fluid in the formation, ultimately resulting in a sharp increase in the resistivity of the hydrate-bearing layer (Li and Xiao., 2013; Ning et al., 2013; Zhou et al., 2020). As shown in the LWD diagram of gas hydrate in the study area (Figure 1), the resistivity of the hydrate-developed interval (133–155 m; the third channel) increased sixfold from 1.0 Ω m at the top of the hydrate-bearing layer to 6.2 Ω m and then fell back to 1.3 Ω m through the bottom of the hydrate layer. In addition, natural gas hydrate has a high acoustic velocity, which should lead to a significant decrease in the acoustic interval transit time of the reservoir. Figure 1 shows that the acoustic interval transit time (the fourth DTCO) of the hydrated layer (13–155 m) decreases from 575 μs/m at the top of the hydrated layer to 380 μs/m followed by an increase to 580 μs/m at the bottom of the hydrated layer.
Data and methods
Establishment of the two-parameter saturation calculation model
Due to the high resistivity of natural gas hydrate, most studies have equated it with oil and gas when calculating saturation. The saturation of natural gas hydrate is commonly calculated using the resistivity measurement value and Archie formula (Eq. 1; Wang et al., 2021; Malinverno. et al., 2008).
where Sh: The saturation of natural gas hydrate, %; Rw: resistivity of formation water, Ω·m; Rt: resistivity of the formation, Ω·m. φ: porosity of formation, %. a, b: Rock-related coefficients, m: cementation index of the formation; n: saturation index.
The Archie formula is the saturation calculation law established from many core experiments, used to obtain saturation parameters for pure sandstone formations with uniform intergranular pores (Zhang et al., 2018; Lei et al., 2022). The marine natural gas hydrate reservoir has the “non-Archie” characteristics of loose and unconsolidated formation, high shale content, and non-uniform saturation distribution. Additionally, natural gas hydrate decomposes easily once it leaves the in-situ formation, making it difficult to accurately obtain the rock electrical parameters a, b, m, and n in the formula through the laboratory (Hyndman. et al., 1999; Goldberg. et al., 2010). Therefore, the Archie formula cannot obtain effective saturation parameters of the hydrate.
The sonic velocity method is mainly used to calculate hydrate saturation using a time average equation (Eq. 2):
where Vp: P-wave velocity of hydrate-bearing sediments, m/s. φ: core porosity, %; S: saturation of hydrate, %; Vw, Vh, and Vm: P-wave velocities of water, pure hydrate, and skeleton, respectively, m/s.
The premise of calculating hydrate saturation using the time average equation is that the theoretical logging acoustic value is composed of pore water, rock skeleton, and hydrate (Chen et al., 2013). However, the actual acoustic value is not only dependent on these media itself, but also on the contact mode between media, such as the degree of formation compaction and the microscopic distribution of hydrate in pores. Therefore, it is necessary to establish a calculation method that can more accurately calculate hydrate saturation.
The proposed model
The formation of natural gas hydrate occurs at a certain temperature, pressure, pore space, and gas source. The occurrence position in the formation depends on the coupling of the above factors, but has no direct relationship with a single factor. However, due to the typical response characteristics of natural gas hydrate with high resistivity and low acoustic interval transit time, it can also be effectively identified by logging data under multi-factor control. Under this premise, the quantitative calculation model of hydrate saturation is constructed using resistivity and acoustic time difference, which collectively is referred to as the tow-parameter saturation model. The calculation formula is as follows:
where RT: deep resistivity logging value of hydrate formation, Ω·m; RTb: deep resistivity of formation without hydrate, Ω·m; AC: acoustic interval transit time logging value of hydrate formation, Us/m; ACb: acoustic interval transit time of formation without hydrate, μs/m; the above resistivity value and acoustic interval transit time can be obtained from the logging curves; A and b: constant coefficients of the two-parameter saturation model.
The coefficients fitting
The coefficients a and b in the two-parameter saturation model are obtained by linear fitting.
First, let
Formula (4) belongs to the form of linear function
The core experimental data of hydrate saturation of five wells in Shenhu Sea of the study area were selected to fit the coefficients. RT, RTb, AC, and ACb are the logging values of these five wells, and Sh is the core experimental data of hydrate saturation. According to the above logging values and core experimental values, a correlation diagram (Figure 2) was established with lg△t/lgR as the abscissa and Sh/lgR as the ordinate. According to the correlation, five groups of fitting coefficient values could be obtained. By calculating the arithmetic average, the values of coefficient a and b are 0.2 and 2.6, so the two-parameter saturation calculation model of natural gas hydrate in this area can be rewritten as:
This saturation calculation model is very simple and widely applicable. First, based on the objective change of reservoir physical characteristics caused by hydrate development, the hydrate saturation can be calculated by using resistivity and acoustic interval transit time logging values. Second, this method avoids the difficult process of obtaining the empirical coefficients in the laboratory, and reduces the influence of strata, lithology, and microscopic factors on measurement outputs. In the specific application process, the correlation coefficient needs to be established according to the actual characteristics. In addition, due to the influence of deposition and structure, lithology and physical properties usually change in a well, and the base value can be segmented to improve the calculation accuracy of hydrate saturation. In terms of coefficient fitting, when more wells are selected, the more accurate the coefficients a and b, and the more reliable the saturation parameters.
Discussion
Analysis of calculation accuracy
To verify the reliability of the two-parameter saturation calculation model, the model calculation results of five wells in the study area were compared with the core experimental values and the values calculated by Archie formula (Figures 3–5, respectively). The rock-electrical parameters in Archie formula were derived from the data of Zhao et al., 2021. The comparison results show that the average relative error of saturation calculated by the two-parameter model is 7%, which is highly consistent with the core experiment results (Figures 3, 5), whereas the average relative error calculated by the conventional Archie formula is 24%, which is significantly larger than that of the core experiment results (Figure 4). Therefore, the two-parameter saturation model can effectively improve the calculation accuracy of gas hydrate saturation.
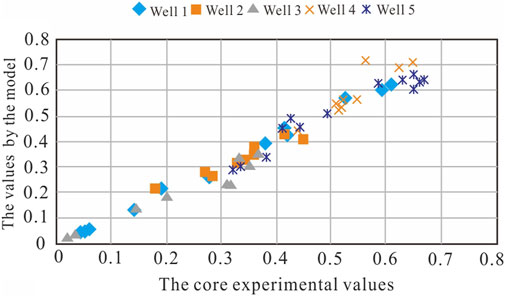
FIGURE 3. Comparison between saturation calculated by two-parameter model and core experimental data.
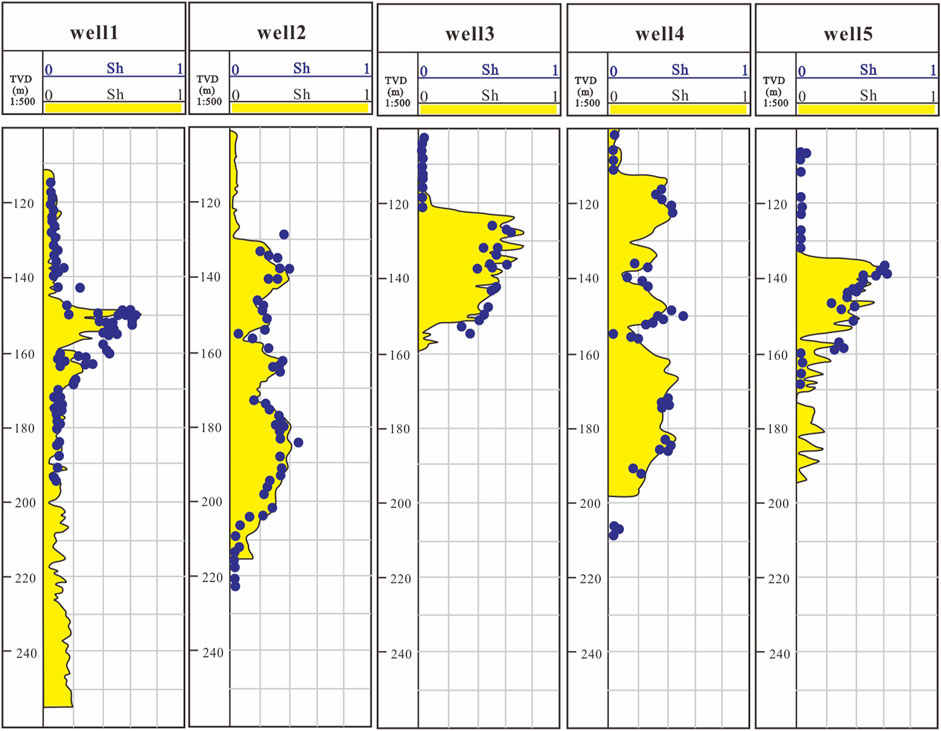
FIGURE 5. Comparison between calculation results of two-parameter saturation model and core experimental data of five wells in the study area.
Conclusion
Unlike conventional oil and gas reservoirs, marine natural gas hydrate reservoirs are characterized by loose and unconsolidated formation, solid hydrate, and easy decomposition. It is difficult to obtain rock-electrical parameters in the laboratory, and there are many influencing factors of logging acoustic value, resulting in relatively poor reliability of hydrate saturation parameters calculated by Archie formula or the sonic velocity method. According to the objective phenomenon of obvious changes in reservoir physical properties caused by hydrate development, a logging two-parameter calculation model based on resistivity and acoustic interval transit time was proposed, and the continuous acquisition of hydrate saturation parameters based on logging data was realized. By comparing results with those of the core experimental data, the calculation results of the two-parameter model were significantly better than those of the Archie formula, indicating that the two-parameter model effectively improves the calculation accuracy of gas hydrate saturation. The method is simple and has wide adaptability, but in the specific application process, the correlation coefficient should be adjusted according to the characteristics of the actual area, and segmented values should be taken to obtain reliable saturation calculation values.
Data availability statement
The original contributions presented in the study are included in the article/Supplementary Material, further inquiries can be directed to the corresponding author.
Author contributions
HL: Investigation, data analysis. JL: Results supervision. CQ: Research and article writing. HS: Logging data processing and interpretation. XZ: Figure drawing.
Conflict of interest
The authors were employed by CNLC Logging Co. LTD.
Publisher’s note
All claims expressed in this article are solely those of the authors and do not necessarily represent those of their affiliated organizations, or those of the publisher, the editors and the reviewers. Any product that may be evaluated in this article, or claim that may be made by its manufacturer, is not guaranteed or endorsed by the publisher.
References
Chen, Y. F., Li, D. L., Liang, D. Q., Zhou, X. B., and Wu, N. Y. (2013). Relationship between gas hydrate saturation and resistivity in sediments of the South China Sea. Acta Pet. Sinca 34 (3), 509–512.
Collet, T. S. (2013). A review of well-log analysis techniques used to assess gashydrate-bearing reservoirs. Geophys. Monogr. Ser. 124, 189–210.
Goldberg, D. S., Kleinberg, R. L., Weinberger, J. L., Malinverno, A., and Collett, T. S. (2010). “Evaluation of natural gas-hydrate systems using borehole logs[M],” in Geophysical characterization of gas hydrates (Tulsa: Society of Exploration Geophysics), 239–261.
Guo, Y., Qiao, S., and Lu, W. (2011). Vertical distribution of gas hydrate in Shenhu area of the South China sea based on acoustic velocity. Mar. Geol. Front. 27 (7), 8–11. doi:10.16028/j.1009-2722.2011.07.001
Hyndman, R. D., Yuan, T., and Moran, K. (1999). The concentration of deep sea gas hydrates from downhole electrical resistivity logs and laboratory data. Earth Planet. Sci. Lett. 172 (1/2), 167–177. doi:10.1016/s0012-821x(99)00192-2
Lei, Y. N., Wu, S. G., Sun, J., and Wang, G. J. (2022). Review on comprehensive geophysical identification methods of marine natural gas hadrate. J. Central South Univ. Sci. Technol. 53 (3), 864–878. doi:10.11817/j.issn.1672-7207.2022.03.009
Li, X., and Xiao, L. Z. (2013). Geophysical characteristics and logging evaluation of natural gas hydrate. Beijing: Petroleum Industry Press, 15–84.
Malinverno, A., Kastner, M., Torres, M. E., and Wortmann, U. G. (2008). Gas hydrate occurrence from pore water chlorinity and downhole logs in a transect across the northern Cascadia margin (Integrated Ocean Drilling Program Expedition 311). J. Geophys. Res. 113 (B8), B08103. doi:10.1029/2008jb005702
Murray, D. R., Fukuhara, M. R., Osawa, O. R., and Endo, T. R. (2006). Namikawa. Saturation acoustic properties growth habit and state of stress of a gashydrate reservoir from well logs. Petrophysics 47 (2), 129–137.
Ning, F. L., Liu, L., Li, S., Zhang, K., Jiang, G. S., Wu, N. Y., et al. (2013). Well logging assessment of natural gas hydrate reservoirs and relevant influential factors. Acta Pet. Sin. 34 (3), 591–606. doi:10.7623/syxb201303026
Su, Z., C, Y. C., Yang, R., Wu, N. Y., Chen, D. F., Yang, S. X., et al. (2014). Research on the formation model of gas hydrate deposits in the Shenhu Area, northern South China Sea. Chin. J. Geophys. 57 (5), 1664–1674. (in Chinese). doi:10.6038/cjg20140529
Sun, Z. D., Jia, C. Z., and Li, X. F. (2011). Unconventional oil and gas exploration and development (volume II). Beijing: Petroleum Industry Press, 1285–1286.
Wang, X. J., Wu, S. G., Liu, X. W., Guo, Y. Q., Lu, J. A., Yang, S. X., et al. (2010). Estimation of gas hydrate saturation based on resistivity logging and analysis of estimation error. Geoscience 24 (5), 993–999. doi:10.19657/j.geoscience.1000-8527.2010.05.001
Wang, Y. J., Wei, W., Xing, C. L., Han, W. F., and Gao, L. (2021). Measurement of hydrate saturation in sediments based on dual parameters from TDR. Adv. new Renew. energy 9 (5), 403–410. doi:10.3969/j.issn.2095-560X.2021.05.006
Wang, Z. W., Li, Z. B., and Liu, J. H. (2003). Logging identification and evalution methods for gas hydrate. Mar. Geol. Quat. Geol. 23 (2), 97–101. doi:10.16562/j.cnki.0256-1492.2003.02.015
Xiao, G., and Bai, Y. (2015). The key technology of gas hydrate exploration and development. Wuhan: Wuhan University Press, 1–20.
Zhang, J., Luo, J., Xia, Y., Hu, W. L., Zhang, G. D., He, C. Y., et al. (2018). Limitation analysis and modification of the Archic equation. Chin. J. Geophys. 61 (1), 311–322. (in Chinese).
Zhang, W., Liang, J. Q., Lu, J. A., Wei, J. G., Su, P. B., Fang, Y. X., et al. (2017). Accumulation features and mechanisms of high saturation natural gas hydrate in Shenhu Area, northern South China Sea. Petroleum Explor. Dev. 44 (5), 708–719. doi:10.1016/s1876-3804(17)30082-4
Zhao, J., Shi, Z. F., Li, Y. P., Xiang, X. R., Li, J., and Wei, N. (2021). Simulation of conductivity characteristics of gas hydrate reservoirs and its saturation calculation. Nat. Gas. Geosci. 32 (9), 1261–1269.
Zhong, G. F., Zhang, D., and Zhao, L. X. (2020). Ourrent states of well-logging evaluation of deep-sea gas hydrate-bearing sedimenb by international scientific ocean drilling (DSDP/ODP/IODP) programs. Nat. Gas. Ind. 40 (8), 25–44.
Zhou, J., Song, Y. J., Jiang, Y. J., Sun, Q. S., and Jing, Y. Q. (2020). The research progress of well logging evaluation of marine natural gas hydrate. J. Southwest Petroleum Universty Sci. Technol. Ed. 42 (2), 85–92. doi:10.11885/j.issn.1674-5086.2019.10.10.01
Keywords: natural gashydrates, saturation, logging, resistivity, acoustic interval transit time
Citation: Li H, Liu J, Qu C, Song H and Zhuang X (2022) A method for calculating gas hydrate saturation by dual parameters of logging. Front. Earth Sci. 10:986647. doi: 10.3389/feart.2022.986647
Received: 05 July 2022; Accepted: 12 August 2022;
Published: 09 September 2022.
Edited by:
Pibo Su, Guangzhou Marine Geological Survey, ChinaReviewed by:
Changcheng Han, Xinjiang University, ChinaCunfei Ma, China University of Petroleum, Huadong, China
Weifeng Zhu, Dagang oilfield, China
Copyright © 2022 Li, Liu, Qu, Song and Zhuang. This is an open-access article distributed under the terms of the Creative Commons Attribution License (CC BY). The use, distribution or reproduction in other forums is permitted, provided the original author(s) and the copyright owner(s) are credited and that the original publication in this journal is cited, in accordance with accepted academic practice. No use, distribution or reproduction is permitted which does not comply with these terms.
*Correspondence: Cuixia Qu, MzI5ODY1NzA4QHFxLmNvbQ==