- 1Archaeology, School of Humanities, The University of Sydney, Camperdown, NSW, Australia
- 2Max Planck Institute for the Science of Human History, Jena, Germany
- 3Department of Anthropology, Texas A&M University, College Station, TX, United States
- 4School of Social Science, The University of Queensland, Brisbane, QLD, Australia
- 5Niche Environment and Heritage, Parramatta, QLD, Australia
- 6Department of Museums and Antiquities, Zanzibar, Tanzania
- 7Department of Anthropology, University of Virginia, Charlottesville, VA, United States
- 8Department of Anthropology, College of William & Mary, Williamsburg, VA, United States
- 9Cultural Heritage Institute, Royal Agricultural University, Cirencester, United Kingdom
- 10Department of Anthropology, Smithsonian National Museum of Natural History, Washington, DC, United States
- 11Department of Anthropology and Archaeology, University of Calgary, Calgary, AB, Canada
The intertidal zone, covering the nearshore fringe of coasts and islands and extending from the high-water mark to areas that remain fully submerged, encompasses a range of habitats containing resources that are as important to modern populations as they were to humans in prehistory. Effectively bridging land and sea, intertidal environments are extremely dynamic, requiring complexity and variability in how people engaged with them in the past, much as they do in the present. Here we review and reconsider environmental, archaeological, and modern socio-ecological evidence from the Zanzibar Archipelago on eastern Africa’s Swahili coast, focusing on marine molluscs to gain insight into the trajectories of human engagement with nearshore habitats and resources. We highlight the potential drivers of change and/or stability in human-intertidal interactions through time and space, set against a backdrop of the significant socio-economic and socio-ecological changes apparent in the archipelago, and along the Swahili coast, during the late Holocene.
1 Introduction
Coastal areas, and by extension the intertidal zone, are of high environmental significance. From an ecological perspective, the coastal zone represents a transitionary area between terrestrial and marine environments, providing the physical connection between land and sea. The intertidal zone represents dynamic and complex systems, largely due to long- and short-term variability in tidal range and amplitude, wave energy, fluctuating salinity levels and temperature, and the effects of high-energy storm activity. Although nearshore coastal habitats present a unique set of challenging conditions for human exploitation as a result of these factors, importantly, the intertidal zone often comprises highly productive ecosystems. This is particularly the case for eastern Africa, which is characterised as containing highly productive coastal habitats and rich biodiversity (Richmond, 2011; Nordlund et al., 2014). Often forming one of the most prevalent groups of taxa inhabiting the intertidal zone, molluscs fill crucial roles within marine ecosystems, being carnivores/predators and herbivores/detritivores operating at all trophic levels. They serve as primary consumers, increase habitat complexity, and improve water quality through filtration.
Human engagement with intertidal habitats extends back minimally 160,000 years in southern Africa. Intertidal foraging and mollusc harvesting are pivotal elements in ongoing debates about human evolution, adaptation to dynamic marine environments, and behavioural modernity (Klein and Bird, 2016; Marean, 2016; Will et al., 2019). Although there is ongoing debate over the timing and nature of initial coastal resource use, when people became coastally adapted, and transitioned to fully maritime societies (Marean, 2014; Fleisher et al., 2015; Jerardino, 2016), of key importance when considering the nature of human engagement with marine environments is that the intertidal zone connects the land, and therefore people, to the sea. Intertidal foraging is thus an important component of marine resource use through time, with intertidal resources providing critical evidence in understanding past human-ecodynamics in coastal settings. Molluscs therefore play a potentially important role in disentangling key elements of human interactions with nearshore environments. This is particularly true on eastern African coasts and islands, where our understanding of intertidal foraging and molluscan resource use is less well developed.
Msemwa (1994) noted in his foundational ethnoarchaeological study of mollusc harvesting on the Tanzanian coast that the literature on shellfish collection across the eastern Africa intertidal zone was particularly limited. While archaeological research has grown substantially since then, archaeomalacology has not significantly progressed in the region, a point echoed in several recent studies. In their review of zooarchaeological evidence from the coast and islands of eastern Africa, for example, Quintana Morales and Prendergast (2018) comment on the richness and productivity of the intertidal zone, but note that their consideration of molluscan evidence is limited due to the lack of regional systematic archaeomalacological analyses. Although their focus is on wild and domesticated terrestrial fauna and fish evidence, they indicate the diversity of economic strategies apparent on the coast and islands, including mollusc gathering, fishing, hunting and animal husbandry.
Greater emphasis has been placed on the archaeological analyses of terrestrial vertebrate fauna and marine fish since the 1980s, with research undertaken in various areas between the Lamu Archipelago on the north Kenyan coast to southwest Madagascar (e.g., Mudida and Horton, 1996; Quintana Morales and Horton, 2014; Prendergast et al., 2016; Prendergast et al., 2017; Douglass et al., 2018; Quintana Morales and Prendergast, 2018; Quintana Morales et al., 2022). Molluscs were little mentioned in the ethnographic and ethnohistoric records on foraging on the eastern African islands as reviewed by Walsh (2007), where emphasis is on terrestrial fauna. To date, systematic assessment of molluscan assemblages is restricted to the ethnoarchaeological work of Msemwa (1994) and Ichumbaki (2014) on the mainland Tanzanian coast, and a handful of studies on Unguja (Zanzibar) Island (Faulkner et al., 2018, 2019a; Sarathi, 2018, 2020), Mafia Island (Christie, 2013; Faulkner et al., 2019b), and southwest Madagascar (Douglass 2017).
Perspectives on the role of molluscs in past economies vary, from a dietary staple to supplementary or starvation food (Erlandson, 2001). Similar interpretations of the relative importance of these resources have occurred in eastern Africa, where they have also been viewed as fallback or starvation food (e.g., Msemwa, 1994; Fleisher, 2003). Although broader syntheses of the molluscan evidence have been hampered by different analytical standards and incompatible data presentation (Fleisher, 2003), recent analyses of specific site assemblages in the Zanzibar and Mafia Archipelagos indicate that the economic importance of molluscs varied greatly through time and space (i.e., Faulkner et al., 2018; Faulkner et al., 2019b; Sarathi, 2020; Rødland, 2021). This suggests that how people engaged with the intertidal zone also changed considerably, and not necessarily unidirectionally, with long-term transitions from hunting and gathering to urban economies.
Extending from this point of view, here we present the first detailed, comparative analyses of molluscan assemblages from the Zanzibar Archipelago. By broadening the spatial and chronological scope within a defined region, we aim to investigate the nature of human interactions with nearshore environments, to identify change or stability in how people foraged and made decisions when engaging with the intertidal zone. We take an ecologically oriented approach to understanding human ecodynamics (following Fitzhugh et al., 2019), considering the nature of palaeoenvironmental shifts and contextualised within patterns of socio-economic/cultural changes, to provide a baseline understanding of intertidal foraging and decision-making through time.
2 Background
2.1 Unguja and Pemba Islands, Zanzibar Archipelago
The Zanzibar Archipelago, adjacent to mainland Tanzania (Figure 1), consists of c.50 islets and two large islands: Unguja at 1,670 km2 and 119 m above sea level, and Pemba at 990 km2 and 96 m above sea level. Unguja is separated from continental Africa by 25–30 km of shallow ocean (Zanzibar Channel, c.35–65 m deep), whereas Pemba sits c.55 km from the mainland surrounded by deeper waters, being separated from the continent by the c.400–800 m deep trench of the Pemba Channel (Agnarsson and Kuntner, 2012; Khamis et al., 2017). Higher precipitation occurs on the western sides of both islands, with deeper soils in these areas being more favourable for agriculture compared with the coral rag and shallow soils of the eastern coasts (Arthurton et al., 1999; Khamis et al., 2017). The vegetation of the coastal lowlands and islands of eastern Africa forms the Zanzibar-Inhambane regional mosaic, containing grassland, shrubland, and a mix of dry and moist forests (Burgess et al., 1998). At present, natural forest areas are largely restricted to protected areas, such as Jozani-Chwaka on Unguja and Ngezi-Vumawimbi on Pemba (Khamis et al., 2017).
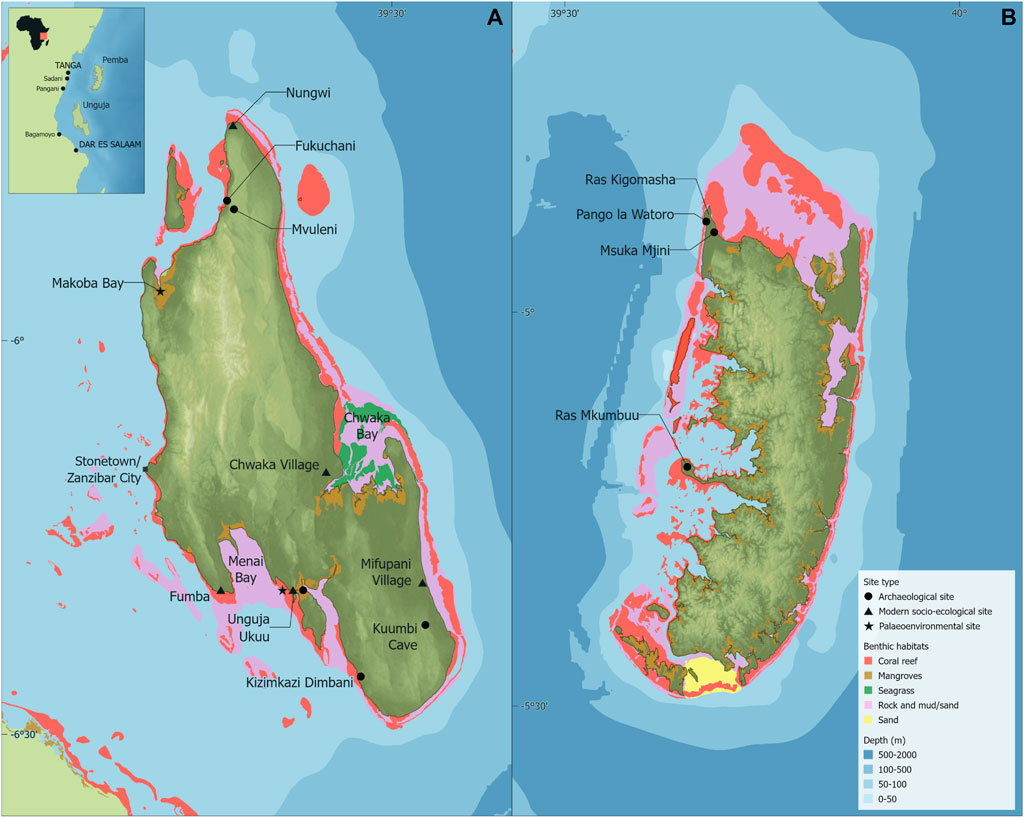
FIGURE 1. The Zanzibar Archipelago study area, showing locations mentioned in the text on Unguja Island (A) and Pemba Island (B) with the distribution of the dominant benthic habitats (Spalding et al., 2010; Khamis et al., 2017 [with permission from Elsevier, order no. 5338180474815]; UNEP-WCMC, WorldFish Centre, World Resources Institute, The Nature Conservancy, 2021; UNEP-WCMC and Short, 2021). Map created using ArcGIS Pro 2.9.1. Bathymetric data from GEBCO Compilation Group. (2021), administrative boundaries from GADM (2022).
The Unguja and Pemba coastlines are characterised by intertidal fringing platforms, commonly extending more than 1 km (in some areas up to 3 km) from the shore, with associated muddy flats, sand flats and beaches, and coral reefs. The northwest and eastern coasts of Pemba, and south and northwest coasts of Unguja, have narrow intertidal zones, with sandy-muddy shores dominating the west coasts. Much of the intertidal platform supports a cover of unconsolidated sediments that form the substrate of seagrass meadows. The platforms and fringing beaches on both islands’ eastern coasts are flanked by sandy beach-ridge plains, the seaward edges of which are marked by prominent storm ridges (Arthurton et al., 1999; Arthurton, 2003; Khamis et al., 2017). In sheltered, shallow embayments, such as those on the south, west and northern coasts of Pemba, and north and southwest coasts and in Chwaka Bay of Unguja, extensive mangrove and seagrass habitats with deeper sand/mud substrates are common. Some of these areas have developed more recently due to ongoing sedimentation and processes of progradation (Arthurton et al., 1999). Unguja’s reefs support a large proportion of regional and global reef-coral diversity (Zvuloni et al., 2010). Living corals cover c.90 km2 across the archipelago, although corals are primarily distributed around the two main islands. Corals form continuous reefs on the eastern coasts, with a patchier distribution on the west, particularly on Unguja (Khamis et al., 2017).
2.2 Archaeological and historical overview
The Zanzibar Archipelago evidences human occupation dating to 20,000 BP at Kuumbi Cave on Unguja. Kuumbi’s pre-Holocene occupation corresponds with a land bridge that connected Unguja to the mainland. Occupation at Kuumbi disappears with the submergence of that connection at the start of the Holocene. Kuumbi was not reoccupied until the mid-first millennium CE (Chami, 2009; Kourampas et al., 2015; Shipton et al., 2016), when ironworking, farming communities expanded from the mainland onto the Zanzibar Archipelago. These communities are associated with a regional ceramic tradition known as Early Tana Tradition/Triangular Incised Ware (ETT/TIW) that marks the Middle Iron Age (MIA, c. 600–1,000 CE) (Fleisher and Wynne-Jones, 2011). Contemporaneous with Kuumbi’s reoccupation included the appearance of small villages across the archipelago such as Fukuchani in north Unguja, and trading ports such as Unguja Ukuu in the south (Juma, 2004). This period was characterised by the rise of Indian Ocean trade on the Swahili coast, linking it with China, South Asia, and the Middle East (e.g., Juma, 2004; LaViolette, 2008; Wood et al., 2016), as well as the introduction of Islam (Crowther et al., 2015; Fitton and Wynne-Jones, 2017; Sarathi et al., 2022).
The abandonment of Unguja Ukuu in the early second millennium CE (Juma, 2004) coincided with new economic and political growth on the coast. The period from c.1000 to 1500 CE saw the emergence of urban centres at localities such as Msuka Mjini, Ras Mkumbuu, and Mvuleni (Horton and Middleton, 2000). These sites, often referred to as “stonetowns”, were marked architecturally by the construction of stone and coral houses, mosques and tombs and housed a merchant elite that controlled trade along the coast and between coast and interior. Some stonetowns, such as the UNESCO World Heritage site of Kilwa off the central Tanzanian coast, gained sufficient wealth and power to function as autonomous city-states (Kusimba, 1999).
The 1500s saw the beginning of the colonial period, when foreign powers sought to dominate the Swahili coast. The Portuguese arrival in the Indian Ocean in the late 15th century was matched by Ottoman expansion into the Red Sea in the 16th century. While the Ottomans claimed theoretical sovereignty over much of the Swahili coast, the Portuguese set up factories and fortresses in some major ports to project power in real terms. On Unguja, the Portuguese period is represented by sites such as one located beneath the Old Fort in Stone Town, and Mvuleni in the northwest. Portuguese-period sites suggest a shift in trade networks as new coastal settlements were established and older ones were abandoned. The great Swahili city of Kilwa, for example, was abandoned after it was attacked by the Portuguese. The decline of Portuguese power in the 18th century coincided with the rise of Omani dominance over much of the Western Indian Ocean. Ultimately, the Omani Empire was partitioned, leading to the establishment of the Sultanate of Zanzibar (1856-1964) which itself became a British protectorate in 1890 (Glassman, 2011).
The year 1964 brought revolution to the archipelago and eventual merger with the Tanganyikan mainland to form modern Tanzania. Since its independence from the Omanis, Zanzibar has modernised in a variety of ways. As a result, modernity and its social, cultural, and economic contexts represent a rupture from the past in many respects. The tourist industry, with its enormous international investments, has severely disrupted traditional modes of subsistence—more so on Unguja than Pemba—with the rise of industrial fishing and increasing availability of motorised boats affecting how fishing and shellfish gathering take place. Traditional modes of subsistence persist, however, in places such as Mifupani on Unguja.
2.3 Study site locations, descriptions and chronology
2.3.1 Northern Pemba: Pango la Watoro and Msuka Mjini
North Pemba supports coral reefs, mangroves, intertidal and subtidal flats with seagrass beds and algal growth. Some areas contain rocky cliffs and intertidal rock flats while others have long sandy beaches. On the western side, numerous embayments are characterised by shallow water, sand banks, seagrass, and mangrove forests. The northern tip (Ras Kigomasha) has a wide intertidal zone with vast seagrass meadows. Fringing coral reefs surround Ras Kigomasha with some interruptions due to input from freshwater channels. Reefs on the western side are similar to that described below for southern Pemba, with reefs on the east having a steep slope and low coral cover due to strong wave action (Richmond, 2014). To Ras Kigomasha’s southeast is a relatively large area of mangroves within Ngezi Forest and at the mouth of a creek and estuary.
Pango la Watoro, the northernmost site in our study, is a small limestone cave that fronts directly onto a sandy beach on the western side of Ras Kigomasha. Excavations in the cave (1 × 2.5 m) by the Sealinks Project in 2012 uncovered low densities of cultural remains throughout the deposits, including c.17th-18th century pottery, fragments of iron implements, charred millet grains, and terrestrial vertebrate faunal remains, as well as a large molluscan assemblage. Although analyses of the non-molluscan excavated components are ongoing, the available evidence suggests that the cave was used only intermittently over the last few hundred years.
Msuka Mjini is a large settlement located at the southeastern end of Ras Kigomasha, c.300 m west of its eastern shoreline. The site covers c.2ha and is known primarily for the ruins of a large, well-preserved 15th century mosque situated at its centre. The mosque famously bears an inscription of the date AH 816 (1413–4 CE) on the inside of its mihrab (Horton, 2017). East of the site is a long sandy beach with a large and expansive sand/mud and rocky intertidal zone fringed by an uninterrupted coral reef. A single 1 × 1 m test trench was excavated at the site in 2016 by the Sealinks Project, uncovering a series of rapidly formed midden deposits. These contained c.13th–15th-century pottery including Middle Eastern and Chinese trade wares, terrestrial vertebrate faunal remains, and fish bone, with analyses of these materials in progress.
2.3.2 Southern Pemba: Ras Mkumbuu
The site of Ras Mkumbuu is located at the end of a long, narrow and low-lying peninsula on the western side of Pemba. Covering 10ha at its zenith, the site boasts one of the most extensive and best-preserved set of ruins on Pemba, and has attracted archaeological attention since the 1930s. Survey and excavations by Kirkman (1959) and Horton (2017) established the presence of two main settlement phases: 10th–12th centuries CE (c.1,050–750 BP) focused on the site’s eastern ridge, and 14th–16th centuries CE (c.550–350 BP) at the site’s western end. Ras Mkumbuu is rich in cultural materials, including diverse imported pottery, glass and stone beads, and metal objects, attesting to its important role as a Swahili trading port and stone town. In 2012, the Sealinks Project excavated four small (1 × 1 m, 2 × 1 m) test trenches at the eastern end of the site. Three of these targeted an area adjacent to the beach at the base of the eastern ridge, where midden material was located. The fourth was placed into the side of a mound on the top of the ridge, which cut through a shallow sequence of compacted house floors. A range of cultural materials, c.10th–12th century in date, were recovered.
Southern Pemba supports coral reefs, mangroves, intertidal and subtidal flats with seagrass beds and algal growth. The continental shelf is narrow and deep waters lie close to the shore. This combination of characteristics has resulted in high coastal species diversity. Around Ras Mkumbuu, the peninsula is surrounded by expansive sand and mudflats fringed by coral reef and containing areas of patch reef. Mangroves are common around the mouths of rivers and creeks and in the sheltered bays of this area. There are rocky outcrops and headlands with small isolated sandy beaches in small bays and rocky inlets. The expansive intertidal sand and mudflats backed by mangrove forests support high taxonomic diversity (Richmond, 2014).
2.3.3 Northern Unguja: Fukuchani and Mvuleni
The northern coast of Unguja is characterised by expansive sand flats and rocky platforms, seagrass beds, patch, and fringing reefs, with little representation of mangrove communities (Richmond, 2014).
The site of Fukuchani is situated on the northwest coast of Unguja, opposite Tumbatu Island. It comprises ten mounded midden deposits (c.2 m × 10 m) running parallel to the coastline and the remains of daub structures (Horton and Middleton, 2000; Horton and Chami, 2018). Three trenches excavated by the Sealinks Project in 2011 (FK10, FK11, FK12) revealed Middle Iron Age occupation evidence, with radiocarbon dates and diagnostic ceramics indicating site use in the 7th–8th centuries CE (c.1,350–1,450 BP). In addition to substantial midden deposits, wild terrestrial vertebrate fauna, near-shore marine fish (primarily reefs and estuarine), Middle Eastern trade wares, Early Tana Tradition/Triangular Incised Ware (ETT/TIW) ceramics, shell and glass beads, and charred millet grains were recovered (Crowther et al., 2016; Prendergast et al., 2017). Fukuchani’s molluscan assemblages were presented in Faulkner et al. (2018), with the initial analyses suggesting a primary focus on rocky habitat taxa akin to reef sweeping. The Fukuchani assemblages are reanalysed here, combining data from the three trenches and reassessing taxonomic composition and habitat at a higher resolution.
Mvuleni is a late 16th–17th-century Portuguese site located at the foot of an escarpment c.750 m inland from Fukuchani with evidence for commercial farming (e.g., tobacco). The site was investigated through detailed mapping and photogrammetry, shovel-test pit (STP) survey, and test and block excavations to identify activities and implications for Portuguese-Swahili interactions in a rural setting (LaViolette and Norman, 2023). A large central house/warehouse dominates, built by Swahili craftsmen of mortared coral-rag, surrounded by a loopholed coral-rag wall. The walling extends north around space where the coralline substrate is at ground surface and a staircase with access to a shallow limestone cave with brackish water; there is local memory of fresh water there. The walling extends south around a 2ha plot of farmland, evidence for earth-and-thatch structures, and latrines and washing spaces. Inside, a small bastion juts out from the seaward wall. STPs and excavations around the bastion revealed the highest density of midden materials at the site, including locally made and European ceramics, a game token and marble, iron knife, crossbow bolt, animal bone (analysis of which is ongoing), and many kgs of burned mussel and crab shell. On the escarpment are 170 m of unmortared coral-rag walls which provided an extraordinary view of activity in Tumbatu and Zanzibar Channels.
2.3.4 Southern Unguja: Unguja Ukuu, Kuumbi Cave and Mifupani
Unguja Ukuu is located on Menai Bay, on the southwest coast of Unguja. Menai Bay is characterised by extensive algal flats and seagrass beds, with patch reefs in deeper parts of the bay, and fringing reefs on the seaward edge of the rocky platforms and small islets. The eastern shore of Menai Bay is a back-reef shoreline, or intertidal shelf, containing incised tidal channels, and extending up to 2 km offshore to the fringing reef system. Mangroves occur in the northern, western, and eastern margins of the bay, with dense forests associated with Uzi Creek and Channel (Berkström et al., 2013; Punwong et al., 2013b; Yahya, 2013; Kotarba-Morley et al., 2022).
Unguja Ukuu is a large proto-urban centre and significant trading port. Based on numerous radiocarbon dates and presence of diagnostic ceramics, occupation there dates to the Middle Iron Age, 7th–10th centuries CE (c.1,350–1,050 BP) (Crowther et al., 2016). Earth-and-thatch structures are associated with significant vertebrate assemblages and middens, as well as evidence for a mix of industrial (lime burning, iron slag) and domestic activities. Similar to Fukuchani, ETT/TIW ceramics and shell and glass beads were recovered, in addition to bead grinders, local and imported trade wares, and African and Asian crops. The vertebrate evidence indicates caprine herding, wild animal exploitation, and the remains of fast-swimming fish (tuna, potentially shark) from deeper waters alongside the reef and estuarine taxa (Juma, 2004; Crowther et al., 2016; Prendergast et al., 2017; Culley et al., 2021). Geoarchaeological evidence from two trenches (UU11, UU14) provide a c.200–350-years sequence of geomorphological change and coastal progradation, combined with increasing human occupation, deposition of anthropogenic debris and potentially land clearance for agricultural activity (Kotarba-Morley et al., 2022; see also Fitton, 2017). Molluscan remains from four of five trenches excavated by the Sealinks Project in 2011–2012 (UU11, UU13, UU14, UU15) were published by Faulkner et al. (2018), focusing on establishing basic assemblage structure and an initial understanding of the mollusc exploitation there. As with Fukuchani, data from the four trenches are combined and reanalysed here to enable robust comparisons with the other assemblages.
Kuumbi Cave is in the Jambiani district of southwest Unguja, c.2.5 km from the present coastline. One of a series of solutional limestone caves within marine limestone terraces of Pleistocene age (Kourampas et al., 2015), from c.20,000 BP the site would have been no more than 7–8 km from the shore due to the steep continental shelf off eastern Unguja (Prendergast et al., 2016). The excavations, stratigraphy, and chronology from the 2012 Sealinks Project excavations have been previously detailed (e.g., Kourampas et al., 2015; Prendergast et al., 2016; Shipton et al., 2016; Faulkner et al., 2019a). The terrestrial and marine molluscan assemblages reported in Faulkner et al. (2019a) focused on the role of these resources in long-term coastal economic adaptations. Highlighted was the use of different intertidal habitats through time relative to postglacial sea level rise, with people using a generalised, sweeping strategy of habitat foraging. Here we restructure and re-analyse the Kuumbi marine molluscan assemblages, with these data falling within four main chronological phases: a late Pleistocene occupation (combining the previously identified Phases 3 and 4) that spans c.20,000–17,000 BP; terminal Pleistocene-early Holocene (Phase 2: c.13,000–11,000 BP); and separating the Phase 1 layers reported in Faulkner et al. (2019a) into the Middle Iron Age (as 1B: c.1,350–1,000 BP) and Late Iron Age (as 1A: c.1,000 to 500 BP) following Prendergast et al. (2016).
On the southeastern coast of Unguja, northeast of Kuumbi Cave, research undertaken in Mifupani village provides a robust sample of modern mollusc harvesting. The people of Mifupani gain their livelihood participating in the tourist industry, fishing, mollusc gathering, horticulture, raising livestock, and some hunting. Sarathi (2020) conducted a 12-months subsistence study of fish and invertebrates gathered there for comparison with archaeofaunal datasets; the marine vertebrate data are not considered in the analyses below as our focus is on intertidal foraging and molluscan assemblages. People can forage along the sandy beach, across the intertidal platform comprised of rock, sandy substrate and seagrass, and onto subtidal reef fringing the intertidal zone c.2–3 km from shore. Although people forage for bivalves (Donax incarnatus) by digging through sand, most mollusc gathering occurs on the intertidal reef flat or just beyond it (Sarathi, 2020). The Mifupani study complements socio-ecological research in Nungwi, Chwaka Bay and Menai Bay (Nordlund et al., 2010; Yahya, 2013; Fröcklin et al., 2014), but is more longitudinal and comprehensive, providing a modern baseline that can be used alongside archaeomalacological datasets on Pemba and Unguja.
2.4 Palaeoenvironmental context
Assessment of relative sea levels in the southwestern Indian Ocean has indicated a high degree of regional complexity in the timing and nature of post-glacial sea levels. Variability in relative sea levels across this broad area is likely due to glacio-isostatic processes, tectonic movement, and changing oceanographic conditions (e.g., Pirazzoli, 1991; Camoin et al., 1997; Woodroffe et al., 2015; Prendergast et al., 2016). Regionally, there appear to be two broad types of relative sea level patterns (Camoin et al., 2004; Woodroffe and Horton, 2005). The first represents a constant rate of sea level rise to 3,000–2,000 cal BP without evidence for a mid-to late Holocene highstand. This pattern appears prevalent on offshore volcanic and coral islands, e.g., Réunion, Mauritius, Mayotte, and the Seychelles (Colonna et al., 1996; Camoin et al., 2004; Woodroffe et al., 2015). The second pattern, following a rapid rise during the terminal Pleistocene-early Holocene, indicates a highstand in the order of 2.5–3 m above present c.6,000 cal BP, with a subsequent slow regression to present levels in the late Holocene. This second pattern has been identified in Madagascar, and on the Kenyan and Mozambican coasts (Camoin et al., 2004; Woodroffe et al., 2015; Accordi and Carbone, 2016).
The recent reconstruction of relative sea levels presented by Prendergast et al. (2016) provides a localised sea level curve for the Zanzibar Archipelago over the last 20,000 years. As Pemba Island effectively lies off the continental shelf, it was isolated during the Last Glacial Maximum (LGM) (and since the early Pliocene; Rowson et al., 2010) due to lower sea levels and the 800 m deep Pemba Channel (Stagna et al., 2022). The Prendergast et al. (2016) model indicates that Unguja was connected to the mainland until c.9,000 BP, with a phase of more rapid sea level rise between c.12,000–9,000 BP (Figure 2; Figure 3A). This accords well with two pulses of rapid sea level rise recorded in Mayotte in the Comoros, dated between 12,800 and 12,400 BP and between 10,100 and 9,600 BP, followed by a gradual increase to roughly present levels from c.7,000 to 1,500 BP (Colonna et al., 1996). The sea level model is also largely supported by mangrove observational data from the Rufiji Delta (Punwong et al., 2013a) on the southern mainland Tanzanian coast, as well as in Unguja Ukuu and Makoba Bay (Punwong et al., 2013b, 2013c; Woodroffe et al., 2015) in the southwest and northwest of Unguja Island. Sea surface temperature (SST) varies through time, with cooler periods from 9,700 to 7,800 cal BP and 5,600–4,200 cal BP, interspersed with warm (7,800–5,600 cal BP) and moderate (4,200–1,350 cal BP) temperatures (Figure 3B). Within these overall trends are high-frequency SST variations at centennial scales (Kuhnert et al., 2014).
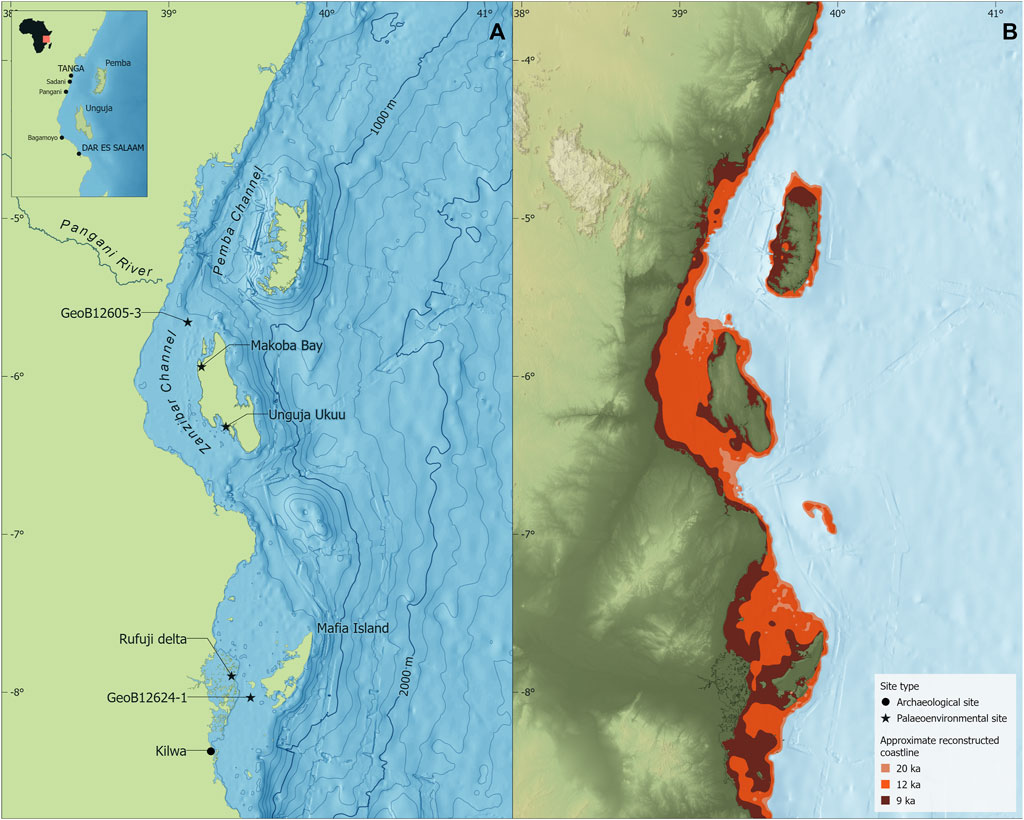
FIGURE 2. Bathymetry of the Tanzanian coast and Zanzibar Archipelago, including the location of the marine sediment cores for sea level and palaeoenvironmental reconstruction (A). Reconstructed sea levels at c. 20,000, 12,000 and 9000 BP (B) after Prendergast et al. (2016). Map created using ArcGIS Pro 2.9.1. Bathymetric data from GEBCO Compilation Group. (2021), administrative boundaries from GADM (2022).
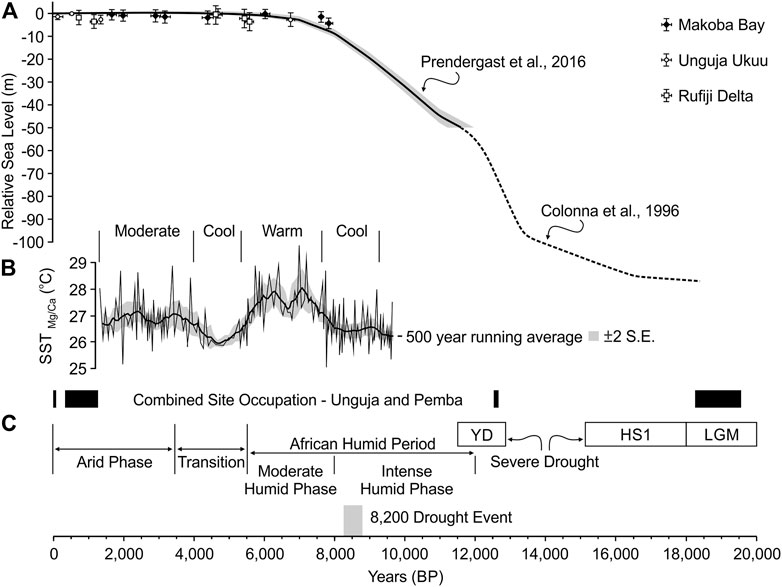
FIGURE 3. Composite sea level curve spanning 20,000 BP to present (A) adapted from Colonna et al. (1996) with permission from John Wiley & Sons (order no. 5334670340538); Prendergast et al. (2016); high resolution SST record, Pemba/Zanzibar Channel (B) adapted from Kuhnert et al. (2014) with permission from Cambridge University Press (order no. 5334730499127); combined site occupation chronology for Unguja and Pemba (see Table 1 for further site-specific chronological and assemblage details) and major climatic phases since the LGM in eastern Africa (C) based on information in Liu et al. (2018).
Importantly, these mangrove data also indicate a phase of early to mid-Holocene sea level rise and late Holocene sea level fluctuation, with sea levels potentially higher than present at c.4,700–4,600 cal BP. Although variable in the rate and timing of sea level rise, it appears that sea levels possibly increased to 0.5–0.6 m above present at Makoba Bay and Unguja Ukuu. This minor increase above present levels is partly supported by the observations of maximum erosional notches on undercut limestone cliffs on Unguja (Arthurton et al., 1999), estimated to approximate that of contemporary higher water levels at spring tides. Mangrove development continued after the mid Holocene, with lower sea levels around c.4,000 cal BP and c.2,000 cal BP, albeit with variability in the nature of fluctuations between locations attributed to local mangrove response to sedimentation and/or erosion (Punwong et al., 2018). For example, at Makoba Bay, sea levels rose after 1,550–1,450 cal BP, whereas at Unguja Ukuu, the mangrove observational and archaeological data suggest sea level was 0.5–1 m below present levels between c.1,300 and 1,000 cal BP, followed by a more recent transgressive episode (Mörner, 1992; Punwong et al., 2013b, 2013c; Prendergast et al., 2016; Punwong et al., 2018). Although mangrove communities have been a feature of the Unguja and Pemba coastlines from c.8,000–7,000 years ago, there is evidence for a reduction in the extent of mangroves (e.g., around Unguja Ukuu) since c.530 cal BP. This reduction has been linked to increasing use of mangrove wood as construction material and fuel (Punwong et al., 2013b).
Modern development of the expansive reef systems fringing Unguja and Pemba was likely initiated in the early Holocene based on sea levels, with reef accretion tracking sea level rise (albeit with a time lag between sea level rise and reef growth) until the reefs reached their near-surface position by the mid-Holocene (Camoin et al., 1997; Camoin et al., 2004). By that time, rising sea levels would have breached the ocean-facing fringing reefs to cover the limestone platforms noted above. The flooding of these platforms would have significantly increased reef system development and intertidal/shallow subtidal habitats around the islands (Arthurton et al., 1999; Arthurton, 2003). In combination with these processes, relatively low sedimentation rates identified for Unguja Ukuu and Makoba Bay (0.3–6.6 mm cal yr−1) on Unguja indicate minor progradation effects in the archipelago (Punwong et al., 2018), at least until the latter half of the first millennium CE with increased anthropogenic impacts associated with coastal settlements (e.g., Fitton, 2017; Kotarba-Morley et al., 2022).
Palaeoclimatic evidence from eastern Africa indicates temporal and spatial complexity since the LGM (Figure 3C). As summarised by Liu et al. (2018) in comparing broader climatic records with a marine sediment core extracted 40 km offshore, from the mouth of the Rufiji River (GeoB12624-1), the LGM is broadly characterised by arid conditions, with two distinctive dry-wet periods (at 15,000–14,500 BP and 11,500–11,000 BP). The latter mark the transition into the African Humid Period of the late Pleistocene-early Holocene. Severe drought would have been experienced in the area north of 8–10°S during the Heinrich Stadial 1 (c.18,000-14,600 BP) and the Younger Dryas (c.12,800–11,500 BP), which includes the Zanzibar Archipelago. The African Humid Period represents a significant phase of climate change across the continent during the Holocene. Based on analyses of marine sediments and benthic foraminifera extracted from an offshore core located c.20 km from the Pangani River mouth, at the western transition between the Pemba and Zanzibar channels (GeoB12605-3), the African Humid Period is represented by two regional phases. The first is an intense humid period from c.12,000 to 8,000 cal BP (with an abrupt drought event around c. 8,200 cal BP), with a second, moderate humid phase from 8,000 to 5,500 cal BP. These humid phases were followed by a transitionary period between 5,500 and 3,500 cal BP and a broad late Holocene arid phase (Romahn et al., 2015; Liu et al., 2017). Evidence of sedimentation rates from this core supports these broad climatic phases, with continuous sedimentation between 9,700 and 1,350 BP, although at a decreasing rate from the core base to the top. The higher sedimentation rates between 9,700 and 8,000 cal BP are attributed to lower sea levels and increased precipitation in the Tanzanian hinterland, followed by a decrease in sedimentary input due to increasing aridity and higher sea levels in the mid to late Holocene (Kuhnert et al., 2014; Liu et al., 2016). More recent climatic fluctuations occur across eastern Africa, with increased aridity from c.950-680 BP, wetter conditions from c.680 to 100 BP, and persistent drought conditions spanning the late 18th and early 19th centuries (Lane and Breen, 2018).
There are gaps in the known occupation sequences for Unguja and Pemba, and critically between c.12,000 and 1,500 BP which represents a period of significant environmental change connected to sea-level rise, separation of Unguja from the mainland and broad climatic fluctuations. Nonetheless, reviewing the baseline paleoenvironmental data provides a clear frame of reference for the available archaeological evidence. Importantly for the late Holocene phases of occupation in the archipelago, even accounting for fluctuating sea levels and associated habitat reconfiguration, the nearshore environments around the islands were largely established by c.3,000–2,000 years ago.
3 Materials and methods
3.1 Identification and abundance
All archaeological molluscan assemblages were analysed at the House of Wonders Museum (Beit-el-Ajaib) or the Peace Memorial Museum (Beit-el-Amani) in Stone Town, Zanzibar, between 2013 and 2019. Identifications were made to the lowest taxonomic level possible (family, genus, or species) based on published keys, descriptions, specimen photographs (e.g., Spry, 1964, 1968; Abbott and Dance, 1998; Carpenter and Niem, 1998; Robin, 2008, 2011; Richmond, 2011), and in comparison with the physical reference collections housed at the University of Sydney. Shell modification was also noted, including burning, damage by marine sponges and polychaete worms, drilling and boring, presence of vermetids (sessile gastropods which may attach to other shells), hermitting by crabs, root etching, and dissolution of the shell (chalkiness). Where they do occur, evidence of burning (2.6%–7.2%), hermit crab alteration (0.1%–0.2%) and predatory modification/epibiont adhesions (0.1%–1.7%) are minimally represented across the assemblages. Evidence of water rolling is also minimal, ranging between 0.1 and 3.0% of assemblages, although there is the possibility of these kinds of taphonomic indicators being obscured by the effects of shell dissolution in these sites. No anthropic modification of the shell (i.e., for ornament production or tool use) was recorded for any of the assemblages. Quantification is based on the calculation of the Minimum Number of Individuals following Harris et al. (2015) and Giovas (2009), using a range of taxon-specific non-repetitive elements (outlined in Faulkner et al., 2018; 2019a). Previously published molluscan data from Unguja Ukuu and Fukuchani (Faulkner et al., 2018) and Kuumbi Cave (Faulkner et al., 2019a) have been reviewed, the taxonomic attributions revised where required, and additional habitat data compiled. Similarly, modern molluscan assemblage data has been compiled for the village of Mifupani in the Jambiani district (Sarathi, 2020). The taxonomic attributions for all archaeological and modern assemblages were checked using the World Register of Marine Species (WoRMS Editorial Board 2022) to ensure that the most up to date nomenclature was recorded.
The following methods are applied to the data at genus and species levels separately to investigate variability in foraging at different taxonomic levels. We do not differentiate between taxa identified as economic or incidental taxa. Economic taxa include those species incorporated into the diet and for the production of tools and ornaments, with incidental taxa defined as those likely to represent harvesting by-products (e.g., juveniles, small-bodied taxa) or natural incorporations into the deposit. As our focus is on investigating the nature of intertidal foraging strategies, we view all taxa as representing the intertidal habitats from which they originated regardless of whether they were harvested incidentally, and as such are considered to provide important information linked to foraging across the intertidal zone. The exceptions to this are the small Batillariidae (mudcreepers) and Vermetidae (worm shells) gastropods, as these taxa largely reflect storm surge deposition or are cemented to other mollusc shells (such as Pinctada spp.). These taxa have the potential to skew results of the habitat analyses as a result and are therefore excluded. The Polyplacophora (chiton) are included as separate categories within each of the analyses as these taxa have been attributed to class level only within each dataset (and are therefore independent categories).
3.2 Richness, nestedness and taxonomic composition
Taxonomic richness is defined as the number of species or other taxonomic category (family, genus) in a sample, and represented as NTAXA (Grayson, 1984; Lyman, 2008). This is a simple and effective measure of assemblage richness, provided that the taxonomic categories identified for analysis are independent, although it can be adversely affected by sample size and may conceal variability in assemblage evenness/dominance. As such, it is important to combine species richness with the measures of heterogeneity focused on dominance and/or evenness discussed further below (Magurran, 2004; Santoro et al., 2017).
In ecology and biogeography, nestedness analyses have been used to investigate species composition, distribution, and interactions across continental and island ecosystems (Ulrich and Almeida-Neto, 2012). First described for insular faunas, such as those found on many islands, nestedness has been related to patterns of colonisation and extinction. Therefore, the general expectation is for an ordered pattern of species presence and richness, where the species composition of a small assemblage is a nested subset of a larger assemblage. Similar trends have been identified at the meta-community level, with strong nestedness reflecting species traits and environmental conditions (Bascompte et al., 2003; Ulrich et al., 2009; Ulrich and Almeida-Neto, 2012). In archaeology, nestedness has been used to complement assessment of the relationship between taxonomic composition and sample size. In these analyses, smaller assemblages with low richness should nest within larger assemblages if they have been derived from the same community (e.g., Wolverton et al., 2015; Faulkner et al., 2021). This also has the potential to shed significant light on past human behaviours, such as foraging strategies, selection, and transportation. This is especially true when considered alongside the available environmental and archaeological contextual information to test whether a series of assemblages distributed through time and space may be derived from similar palaeoecological communities (Peacock et al., 2012; Wolverton et al., 2016).
Nestedness analyses are based on species presence-absence data derived from a site or sampling location. The presence-absence matrix is ordered by the most common species (to the upper rows) and locations with the greatest richness (to the left columns), with nestedness indicated by the concentration of species present in the upper left matrix corner (Ulrich and Almeida-Neto, 2012). The most typically used method of quantifying nestedness is the matrix temperature measure (T), with values ranging between 100° (no nestedness) and 0° (perfect nestedness) (Atmar and Patterson, 1993; Ulrich et al., 2009). As it appears unclear whether the temperature metric should be applied to networks of interacting species (Bascompte et al., 2003), as is effectively the case in investigating archaeological assemblages derived from the interaction of humans with other species of interest, the NODF metric is also calculated (Almeida-Neto et al., 2008; Ulrich et al., 2009). This measure is based on the standardised differences in column and row fills, combined with the overlap of presences in adjacent columns. The NODF values range from 0 (no nestedness) to 100 (perfect nestedness). Nestedness matrices, metrics and significance (Z-scores and p-values) are calculated using the NeD (http://purl.oclc.org/ned) online interface (Strona et al., 2014).
Correspondence analysis and chord distance analysis are used here to explore similarities and differences in the taxonomic composition of the assemblages (e.g., Faith and Lyman, 2019; Harris et al., 2016; Semken and Graham, 1996). Correspondence analysis is a method of ordination that displays the rows and columns of a data matrix as points in corresponding low-dimensional space, such as a bivariate scatterplot. As such, assemblages will plot closer together when similar in taxonomic composition, with taxa plotting closer to those assemblages to which they are more strongly associated (Faith and Lyman, 2019). Chord distance is a metric used for abundance data (as a measure of Euclidean distance between normalised vectors), useful for assessing dissimilarity between samples or assemblages (Legendre and Gallagher, 2001). Chord distance provides a useful measure of dissimilarity as taxa are not heavily weighted when represented by single individuals (Harris et al., 2016). The values derived from chord distance analyses range between 0 (no difference in relative abundance) and
where
The correspondence analyses and chord distance analyses were performed using the PAST Paleontological Statistics Package Version 4.10 (Hammer et al., 2001).
3.3 Diversity indices
Heterogeneity measures can be divided into Type 1 indices that are affected by the occurrence of rare species (richness), and Type 2 indices sensitive to the abundance of the most common species (dominance). Evenness and dominance refer to the distribution of specimens across taxonomic categories. An even assemblage is one where all species are similarly abundant, and an uneven assemblage one where one or few taxa dominate in combination with a high number of rare species (Faith and Du, 2018). The best-known and widely used examples of Type 1 and Type 2 measures in archaeological research are the Shannon (H′) and Simpson (1-D) indices respectively (Lyman, 2008; Magurran, 2004).
The Shannon index (H′) measures diversity based on the relationship between species richness (NTAXA) and the relative abundance (MNI) of each taxonomic category. Using taxonomic richness and abundance, this index calculates the likelihood of predicting the taxonomic affiliation of the next individual drawn from a population, assuming that individuals are sampled randomly from an infinitely large population (Magurran, 2004; Gifford Gonzalez, 2018). The Shannon index is calculated from the equation:
where
Shannon index values usually fall between 1.5 and 3.5 (reflecting lower to higher diversity), rarely increasing beyond 4.0 (Magurran, 2004), and as a measure of heterogeneity, this index is sensitive to both richness and evenness (Faith and Du, 2018).
The Simpson index (1-D) represents the probability that two randomly samples individuals will belong to the same taxon. For zooarchaeological assemblages, which are classified as finite samples (Magurran, 2004; Lyman, 2008; Faith and Du, 2018), the unbiased option of the Simpson index is calculated as:
where
Simpson index values fall between a minimum of 1/NTAXA (where all taxa are equally abundant) to approximately equal to one where an assemblage dominated by a single taxon. The Simpson index is usually expressed as 1-D′ so that larger values are associated with greater evenness (Magurran, 2004; Faith and Lyman, 2019). Importantly, the Simpson index is less sensitive to variations in richness and sample size effects, has high efficiency, has the greatest discriminatory power for uneven communities, and appears to be less sensitive to inter-site differences than the Shannon index (Magurran, 2004; Faith and Du, 2018).
As with the correspondence and chord distance analyses, the diversity analyses were undertaken using the PAST Paleontological Statistics Package Version 4.10 (Hammer et al., 2001). Confidence intervals at 95% for the Shannon and Simpson indices were generated via bootstrapping (9,999 iterations), with diversity permutation tests (9,999 random permutations) used to assess significant differences in each measure (Hammer and Harper, 2007; Harris et al., 2016; Santoro et al., 2017).
3.4 Molluscan zonation and benthic habitat attribution
Recent investigations of molluscan habitats linked to human foraging undertaken by Harris and Weisler (2017) and Rogers and Weisler (2022) in the eastern Pacific Islands form the methodological basis of the analyses of zonation and benthic habitats on Unguja and Pemba. These authors applied hierarchical classification schemes for marine benthic habitats, with mollusc taxa assigned to discrete environmental/geographic zones and habitats. Environmental zone refers to the location of the benthic community (e.g., shoreline intertidal, reef), with habitat referring to the geomorphological structure (e.g., coral, rock/debris, sand). As a similar, detailed classification scheme has not yet been developed for eastern African coastal and island areas, a modified and simplified scheme is applied to the assemblages from Unguja and Pemba. Simplification of the hierarchical classification scheme also suits the geographical and chronological scale of these analyses, providing an opportunity to develop baseline comparative data across the two islands. It also suits the resolution of the information available for molluscan taxa of the Western Indian Ocean, which demonstrates an extremely high degree of species diversity. For example, there are numerous genera of Polyplacophora with c.40 species from more than three families, c.400 gastropod families with at least 2,500 species, and c.50 bivalve families comprised of more than 800 species (Richmond, 2011). While not all these taxa occur within the Zanzibar Archipelago, it highlights the taxonomic complexity of the region.
We use the major tidal areas of the intertidal zone, moving along the gradient from the shoreline to sublittoral as the initial environmental zone designation. Three broad intertidal zones are defined following Richmond (2011): supralittoral (or supratidal), eulittoral and sublittoral (or subtidal). The supralittoral zone is largely dry, being inundated for only a few days a year. The littoral fringe at the seaward edge of the supralittoral zone is only covered by the sea during spring high tides. The eulittoral zone, falling between mean high and low water neap levels, is exposed on every tide. Finally, the sublittoral zone is permanently submerged, with the sublittoral fringe bordering the eulittoral on the landward edge only exposed during or near spring low tides. Geomorphic structures, or substrate characteristics, are designated based on the definitions provided in Harris and Weisler (2017). Taxa are also categorised according to their benthic organism type, either as infaunal (those that live and burrow within the substrate) or epifaunal (those that live on or attached to the outer surface of their environment or other organisms).
The compilation of habitat data was undertaken with reference to Abbott (1959, 1960, 1961), Abbott and Dance (1998), Carpenter and Niem (1998), Cernohorsky (1984), Dekker (2018), Harasewych and Moretzsohn (2017), Harris and Weisler (2017), Healy et al. (2011), Lamprell and Healy (1998), Lamprell and Whitehead (1992), Richmond (2011), Shefer et al. (2012), Willan et al. (2015), and Wilson (1993). Data collection was restricted to taxa at genus and species levels, excluding family-only level taxonomic attributions (11 bivalve, 17 gastropod families) in line with the range of analyses outlined above. Reliable habitat information could not be determined for several taxa, with one bivalve genus (8 MNI), three gastropod genera (4 MNI), and 11 gastropod species (combined 378 MNI). The habitat information and coding per taxon are provided in Supplementary Material S1.
4 Results
4.1 Assemblage characteristics
The characteristics of the molluscan assemblages from the eight sites outlined above are presented in Table 1, with the full list of species per site assemblage provided in Supplementary Material S1. The data from Kuumbi Cave are separated into the distinct chronological phases noted above, with all other sites recorded as individual assemblages (i.e., aggregating data into a single site-based assemblage). The assemblages are listed in Table 1 in approximate chronological order, providing details on the number of taxa and relative abundance (MNI) at family, genus, and species levels. The number of taxa and MNI vary considerably across the assemblages, with the highest MNI and/or NTAXA values irrespective of taxonomic level consistently derived from the modern Mifupani dataset, and lowest values from Kuumbi Cave 1A.
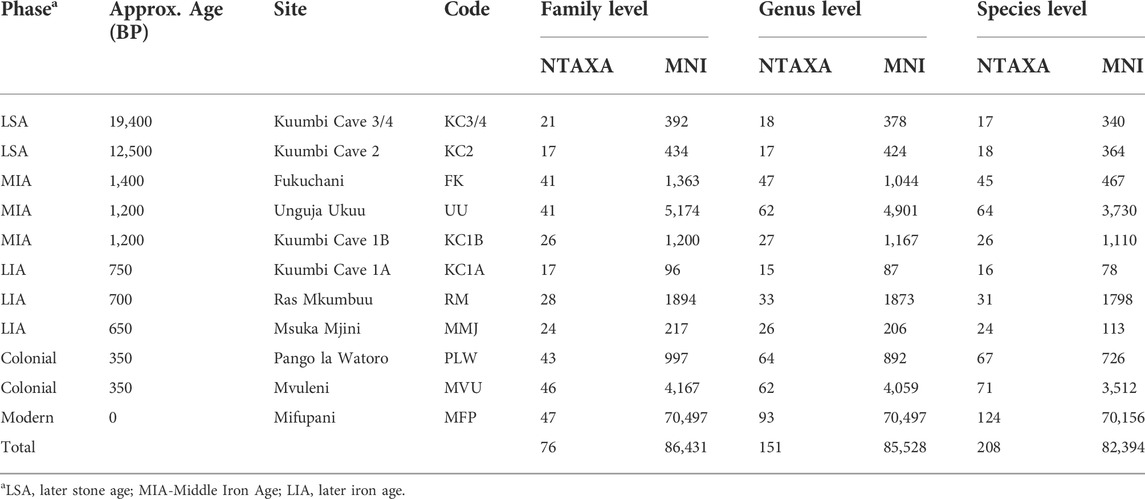
TABLE 1. Assemblage structure per site, showing richness (NTAXA) and relative abundance (MNI) values. Note: Polyplacophora are included in each taxonomic level as an individual category.
To investigate potential sample size effects on assemblage structure and richness, NTAXA values are plotted against MNI at genus (Figure 4A) and species (Figure 4B) levels. At both genus and species levels, the positive relationship indicated by the linear trendline appears to be driven by the large sample size and high richness at Mifupani, although the strength of these relationships is moderate (genus and species R2=0.499). There is little change when removing Mifupani, with a similarly moderate strength of relationship between NTAXA and MNI (genus R2=0.506; species R2=0.519). That said, although individual assessment of the Msuka Mjini, Ras Mkumbuu, Pango la Watoro and Mvuleni assemblages is not currently possible, previous assessment of species area curves for Unguja Ukuu and Fukuchani (Faulkner et al., 2018), and for each chronological phase at Kuumbi Cave (Faulkner et al., 2019a), indicates these assemblages were sampled to redundancy. These results may be seen to indicate that approximately 50% of the variance in NTAXA is explained by sample size, however the degree of data dispersion combined with the differences in site context and chronology (see section 2.3) indicates that a range of human behavioural and environmental factors are likely strong contributors.
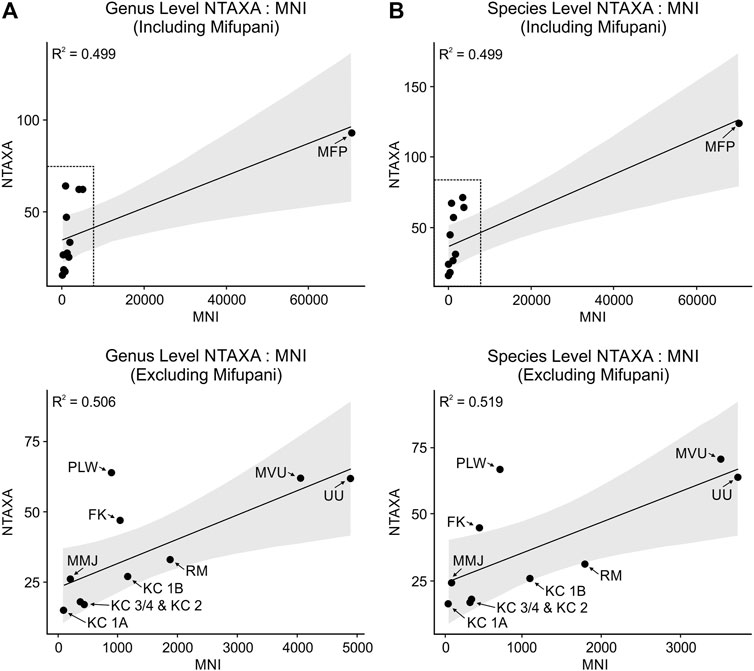
FIGURE 4. NTAXA: MNI plots at genus level (A) and species level (B), with linear regression lines and standard error (grey shading). KC 3/4, Kuumbi Cave 3/4; KC 2, Kuumbi Cave 2; FK, Fukuchani; UU, Unguja Ukuu; KC 1B, Kuumbi Cave 1B; KC 1A, Kuumbi Cave 1A; RM, Ras Mkumbuu; MMJ, Msuka Mjini; PLW, Pango la Watoro; MVU, Mvuleni.
4.2 Richness and nestedness
The nestedness results at genus and species levels are relatively similar. The nestedness metrics and significance statistics for the assemblages at both levels are presented in Supplementary Material S2, with the Z-scores and p-values (all at p < 0.001) for each measure indicating that the assemblages with lower NTAXA values nest within those with higher NTAXA. The nestedness temperature values are low for genus (16.935°) and species (15.532°), indicating a high degree of nestedness for all assemblages. In comparison, the combined NODF values of 57.359 (genus) and 49.611 (species) indicate a moderate rather than high degree of nestedness. These overall NODF values are confirmed by the separate row and column values.
Visual inspection of the genus and species nestedness matrices (Figure 5; with matrix data provided in full in Supplementary Material S3 at genus level and Supplementary Material S4 at species level) provides support for the moderate NODF nestedness results. There is a clear concentration of taxonomic representation in the upper left of each matrix, representing a core group of genera and species common to all assemblages; 27 genera and 25 species occur in 50% or more of the assemblages. The overall distribution of taxa, with what can be described as unexpected absence and presence above and below the diagonal reference lines respectively, indicates imperfect nestedness. This is a result of 124 genera and 183 species being represented in less than 50% of the assemblages. The matrices provide a relative indication of assemblage grouping, with Mifupani separated from Pango la Watoro, Mvuleni and Unguja Ukuu. Fukuchani, Ras Mkumbuu, Kuumbi Cave 1B (MIA) and Msuka Mjini cluster together, with the LSA (KC 3/4 and KC 2) and the LIA (KC 1A) assemblages from Kuumbi Cave falling together.
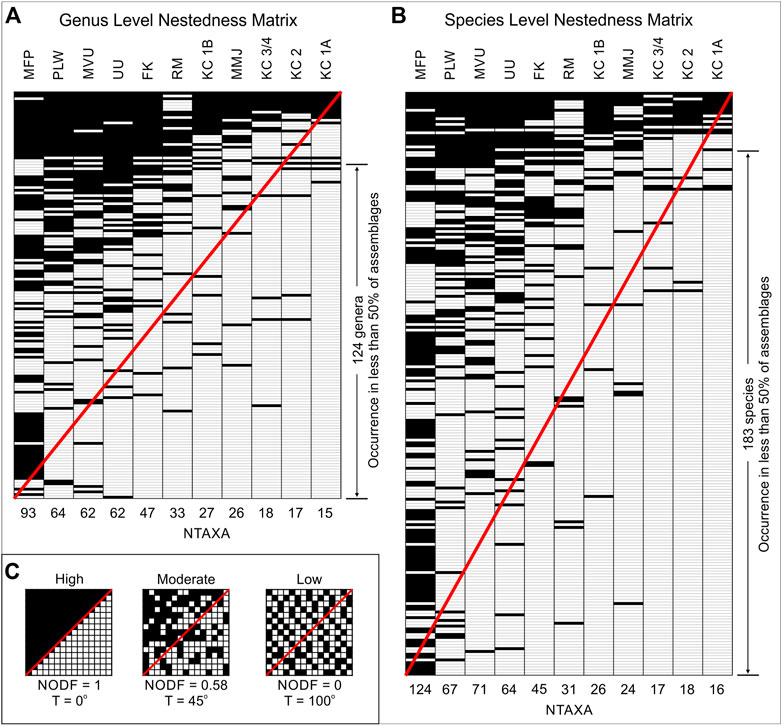
FIGURE 5. Nestedness matrices for the assemblages at genus level (A) and species level (B). Red diagonal lines used for visual reference. Example matrices (C) showing high, moderate, and low nestedness with associated T and NODF values (adapted from Johnson et al., 2013). MFP, Mifupani; PLW, Pango la Watoro; MVU, Mvuleni; UU, Unguja Ukuu; FK, Fukuchani; RM, Ras Mkumbuu; KC 1B, Kuumbi Cave 1B; MMJ, Msuka Mjini, KC 3/4, Kuumbi Cave 3/4; KC 2, Kuumbi Cave two; KC 1A, Kuumbi Cave 1A.
4.3 Taxonomic composition
Consideration of taxonomic composition provides an indication of similarity and difference between assemblages, effectively building on the nestedness results. The contribution of key taxa (at ≥5% by MNI to one or more of the assemblages) at genus level per assemblage is presented in Figure 6.
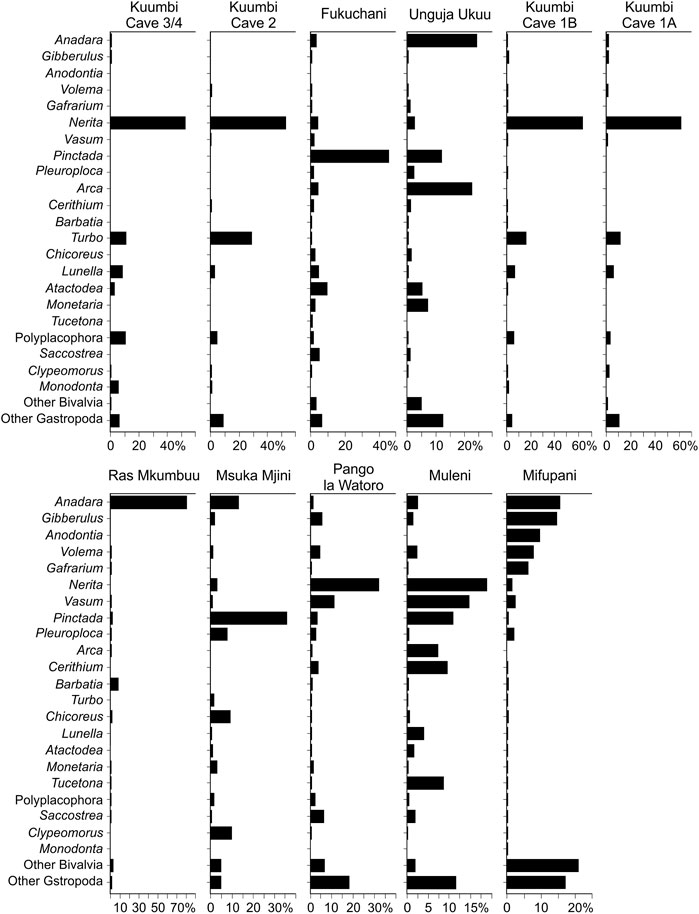
FIGURE 6. Contribution of key taxa (%MNI) at genus level to each assemblage. Each taxon listed contributes ≥5% by MNI to one or more assemblage.
The four Kuumbi Cave assemblages are dominated by gastropods, primarily by Nerita with minor contributions of Turbo, Lunella, the Polyplacophora and Monodonta. Fukuchani and Msuka Mjini have a greater proportional representation of the bivalve Pinctada, with variable but comparatively minor contribution to each assemblage by both bivalve and gastropod taxa. Unguja Ukuu has a similar composition, albeit with an increased proportional representation of the bivalves Anadara and Atactodea, and a lower contribution of a range of other bivalves and gastropods. Pango la Watoro and Mvuleni are dominated by Nerita, with minor contributions from Gibberulus, Vasum and Saccostrea at Pango la Watoro. At Mvuleni, a mix of bivalves and gastropods, including Vasum, Pinctada, Arca, Cerithium and Tucetona, are also strongly represented. In contrast, Ras Mkumbuu is heavily dominated by Anadara (c.80%), and Mifupani exhibiting a more even distribution for Anadara and Gibberulus, followed by Anodontia, Volema and Gafrarium. All other taxa are minor, but more evenly represented in comparison with the other assemblages.
The trends at genus level are largely replicated by species level taxonomic representation (Figure 7), although exclusion of the genus level data forces some reordering depending on level of identifiability. For example, while Pinctada was the dominant genus at Fukuchani, species level attributions for this genus were unattainable for this assemblage. As a result, the contribution of several bivalves (Anadara antiquata, Arca ventricosa, Atactodea striata, Saccostrea cuccullata) and gastropods (Pleuroploca trapezium, Nerita balteata, Lunella coronata) becomes more apparent.
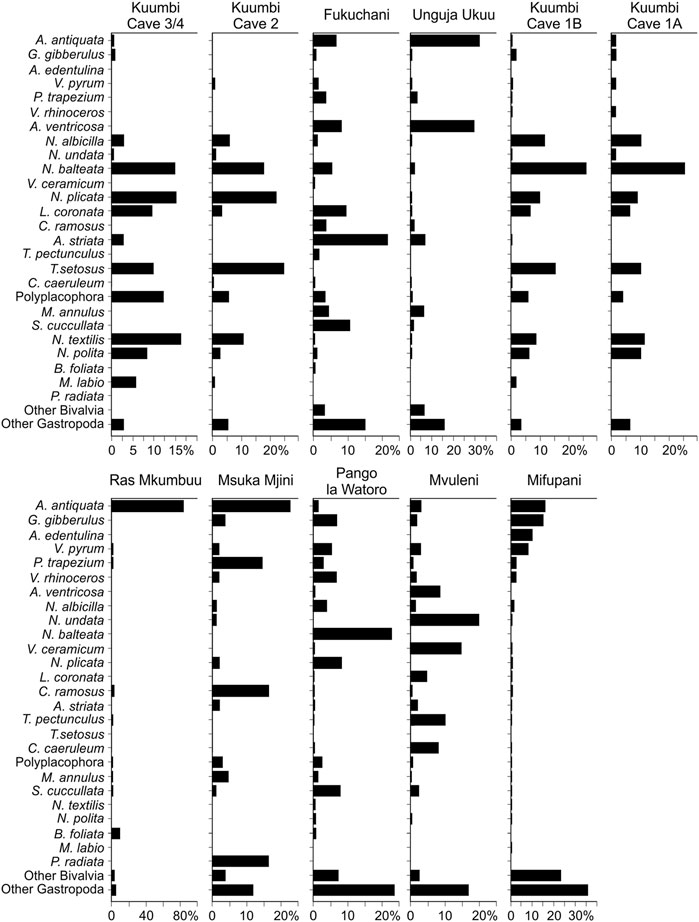
FIGURE 7. Contribution of key taxa (%MNI) at species level to each assemblage. Each taxon listed contributes ≥5% by MNI to one or more assemblage.
The chord distance results at both genus and species levels highlight similarities and differences in taxonomic composition between these assemblages (Supplementary Material S2). At genus level, there is minimal dissimilarity noted for some site pairs; between all Kuumbi Cave assemblages, between Pango la Watoro and Kuumbi Cave 3/4, 1B and 1A, and between Fukuchani and Msuka Mjini. There is moderate dissimilarity between Mvuleni and all Kuumbi assemblages, as well as Mvuleni and Pango la Watoro. Kuumbi Cave 2 exhibits moderate dissimilarity to Pango la Watoro, as does Unguja Ukuu with both Ras Mkumbuu and Msuka Mjini. Moderate dissimilarity is also exhibited between Kuumbi Cave 2 and Pango la Watoro, and between Ras Mkumbuu and Mifupani. All other assemblage pairs indicate moderate to high dissimilarity at genus level. At species level, only the comparisons between the Kuumbi Cave assemblages indicate minimal dissimilarity. Moderate dissimilarity is exhibited between Pango la Watoro and the Kuumbi Cave assemblages, between Unguja Ukuu and both Ras Mkumbuu and Msuka Mjini assemblages, and between Ras Mkumbuu and both Msuka Mjini and Mifupani. As with the genus level data, most assemblage pairs exhibit moderate to higher levels of dissimilarity.
Correspondence analyses exploring the relationship between taxonomic composition and assemblage variability at genus and species levels reinforce the data presented above (Figure 8). For the genus level correspondence analysis (Figure 8A), axes 1 and 2 account for 37.2 and 25.3% of the variance in taxonomic abundance respectively. Mifupani falls close to the origin, indicating that it is less differentiated or distinct relative to the other assemblages based on taxonomic composition. This is highlighted by the clustering of taxa close to the origin, including Anadara, Barbatia, Anodontia, Gafrarium, Gibberulus and Vasum, which occur across several assemblages but are less discriminating. The Kuumbi Cave assemblages fall positively on axes 1 and 2, cluster closely together, and are more strongly associated with Monodonta, Turbo and Nerita. Similarly, Pango la Watoro and Mvuleni exhibit positive axis 1 and 2 scores, but with Mvuleni falling closer to 0 on axis 2. These assemblages are largely discriminated by Nerita, Lunella and Tucetona, although to a lesser degree than Kuumbi Cave. Somewhat similarly, Ras Mkumbuu falls at 0 along axis 1 and is negatively scored on axis 2. This site sits in closer proximity to Mifupani as it is dominated by Anadara and Barbatia. Msuka Mjini, Unguja Ukuu and Fukuchani all score positively on axis 1 and negatively on axis 2, and sit within relative proximity indicating greater similarity (particularly between Msuka Mjini and Unguja Ukuu). These sites are associated with Pinctada, Atactodea, Arca and Monetaria, with other dominant taxa such as Anadara being less discriminating for these assemblages.
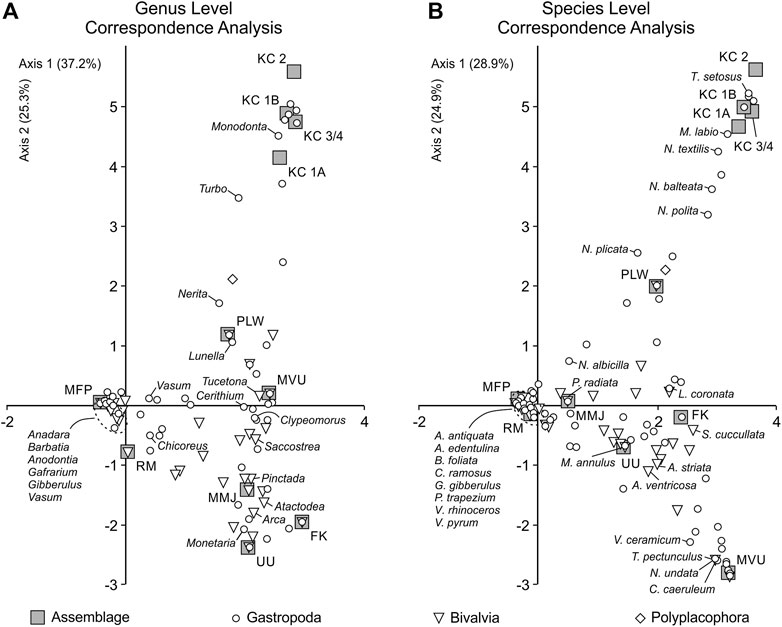
FIGURE 8. Correspondence analysis of taxonomic abundance at genus level (A) and species level (B). Key taxa for the combined and individual assemblages (contributing ≥5% by MNI) are labelled. KC 3/4, Kuumbi Cave 3/4; KC 2, Kuumbi Cave 2; FK, Fukuchani; UU, Unguja Ukuu; KC 1B, Kuumbi Cave 1B; KC 1A, Kuumbi Cave 1A; RM, Ras Mkumbuu; MMJ, Msuka Mjini; PLW, Pango la Watoro; MVU, Mvuleni; MFP, Mifupani.
For the species level correspondence analysis (Figure 8B), axes 1 and 2 account for 28.9 and 24.9% of the variance in taxonomic abundance respectively. Mifupani retains its position close to the origin, with both Ras Mkumbuu and Msuka Mjini now shifting to fall closer to the origin. This indicates greater similarity in assemblage structure and less discrimination by taxonomic composition at species level. The Kuumbi Cave assemblages retain their discrete cluster and association with Turbo setosus, Monodonta labio and several Nerita species, with Pango la Watoro also falling within a relatively similar position to that observed at genus level. The three assemblages that score positively on axis 1 and negatively on axis 2, Unguja Ukuu, Fukuchani and Mvuleni, are associated with different taxa, with Unguja Ukuu falling closer to Msuka Mjini and Ras Mkumbuu (and therefore closer to the origin). Both Fukuchani and Mvuleni are highly differentiated from each other and the other assemblages, with Fukuchani associated with Lunella coronata, Saccostrea cucullata and Atactodea striata, and Mvuleni associated with (and discriminated by) Vasum ceramicum, Tucetona pectunculus, Cerithium caeruleum and Nerita undata.
4.4 Assemblage diversity
The Shannon H′ and Simpson 1-D values are presented in Table 2, along with richness (NTAXA) and MNI values for each assemblage. The NTAXA, H′ and 1-D values are graphed in Figure 9 for visual comparison, with the assemblages in approximate chronological order from the oldest to most recent (left to right).
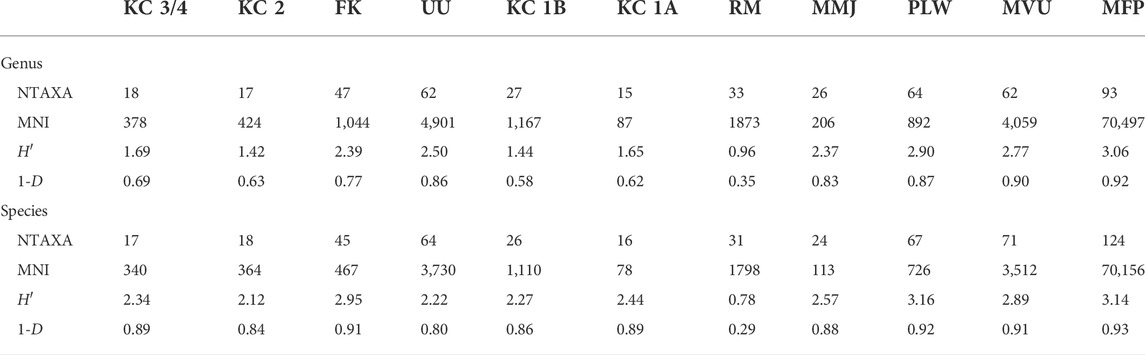
TABLE 2. Genus and species level richness and diversity values by assemblage for Shannon H′ (upper) and Simpson 1-D (lower) indices.
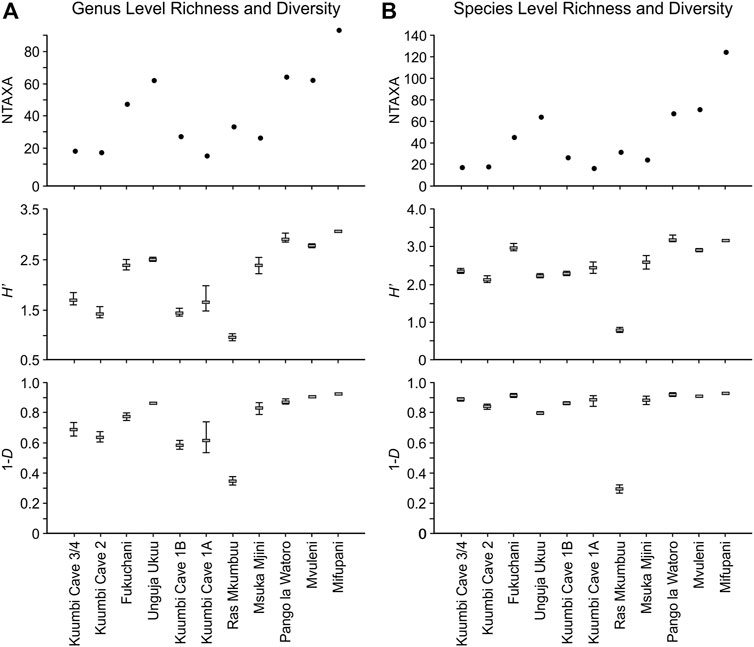
FIGURE 9. Plots of richness (NTAXA) and diversity indices (H′ and 1-D) for each assemblage at genus level (A) and species level (B). Confidence intervals for each measure are the percentiles bootstrapped at 9,999 iterations.
At genus level (Figure 9A), the H′ and 1-D values parallel each other to a large degree. These data indicate a higher degree of diversity for Fukuchani, Unguja Ukuu, Msuka Mjini, Pango la Watoro, Mvuleni and Mifupani, with moderate to high diversity recorded for the four Kuumbi Cave assemblages. Ras Mkumbuu shows the lowest values for both measures of diversity. The results of the diversity permutation tests at genus level (Supplementary Material S2) indicate significant differences for both H′ and 1-D between the majority of assemblages. Kuumbi Cave 1A, 1B and 2 are not significantly different, with Kuumbi Cave 3/4 not differing significantly from 1A alone. For H′, Fukuchani and Unguja Ukuu are significantly different from each other, but neither are significantly different from Msuka Mjini. For 1-D, only Fukuchani is not significantly different from Msuka Mjini. Across both measures of diversity, Unguja Ukuu, Ras Mkumbuu, Pango la Watoro, Mvuleni and Mifupani are significantly different from all other assemblages.
Both diversity index values are consistently high at species level (Figure 9B), although the H′ values show greater variability than those calculated for 1-D. With these data, Fukuchani, Pango la Watoro, Mvuleni and Mifupani demonstrate higher diversity, followed by Kuumbi Cave and Msuka Mjini. For both measures, as with the genus level data, Ras Mkumbuu exhibits substantially lower values in comparison with all other assemblages. The diversity permutation test results at species level (Supplementary Material S2) again indicate significant differences for both H′ and 1-D between most assemblages, although these data are more complex than indicated at genus level. For both measures, Kuumbi Cave 3/4 is not significantly different from Kuumbi Cave 1A and Msuka Mjini, and both Fukuchani and Msuka Mjini are not significantly different from Mvuleni. Kuumbi Cave 1A and 1B do not differ significantly from each other, and Msuka Mjini is not significantly different from Kuumbi Cave 1A. At species level, Ras Mkumbuu and Mifupani are consistently significantly different from the other assemblages.
The diversity index values and results of the permutation tests at both genus and species level indicate, for the most part, lack of a clear, directional chronological trend in richness and diversity. Irrespective of assemblage richness, these data largely indicate a moderate to high or high level of diversity, with a degree of variability apparent across assemblages. Regardless of taxonomic level and diversity measure, Mifupani and Ras Mkumbuu are consistently separated from all other assemblages; Mifupani for its high richness and diversity, Ras Mkumbuu for its low richness and significantly lower levels of diversity.
4.5 Zonation and benthic habitat analysis
Habitat categories identified for taxa at genus and species levels are presented in Figures 10, 11. In both cases, the dominant habitat areas that represent ≥10% by MNI of one or more assemblage are included. At genus level, 10 habitat categories represent between 47% (Mifupani) and 88% (Kuumbi Cave 2) of the total assemblages, and at species level 16 habitat categories represent between 65% (Mifupani) and 99% (Kuumbi Cave 3/4) of the total assemblages.
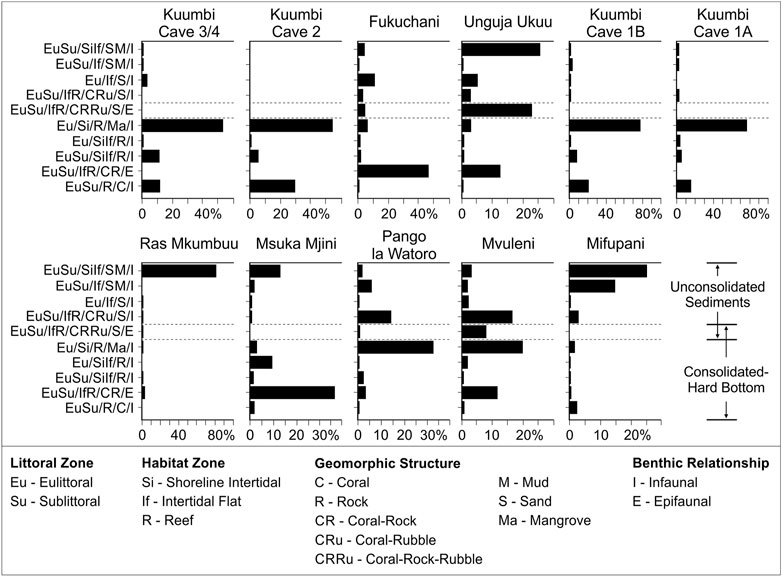
FIGURE 10. Contribution of dominant habitat zones (%MNI) at genus level to each assemblage. Each habitat listed contributes ≥10% by MNI to one or more assemblage. See Supplementary Material S1 for further information on habitat code definitions.
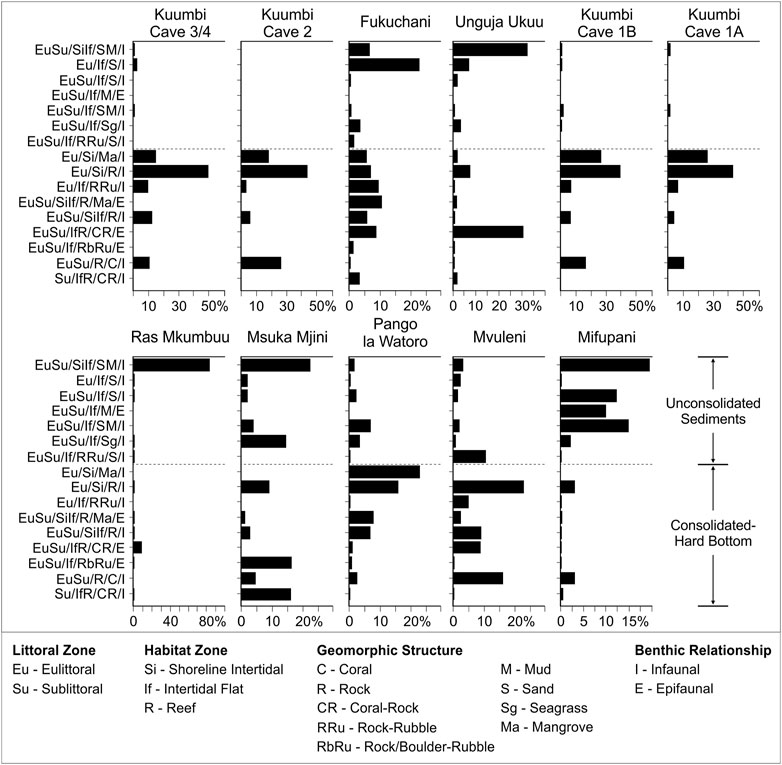
FIGURE 11. Contribution of dominant habitat zones (%MNI) at species level to each assemblage. Each habitat listed contributes ≥10% by MNI to one or more assemblage. See Supplementary Material S1 for further information on habitat code definitions.
At genus level (Figure 10), Kuumbi Cave shows a consistently high representation across all occupation phases of taxa from mangrove, rock and coral substrates across shoreline intertidal to intertidal flat habitats (76%–88% MNI), with minimal representation of taxa from sand and/or mud substrates from shoreline intertidal, intertidal flat and reef habitats (2%–4% MNI). Kuumbi Cave 2 only contains taxa from consolidated/hard substrates. Fukuchani (46% MNI) and Msuka Mjini (37% MNI) are both dominated by taxa from coral and rock substrates in intertidal flat-reef habitats, with molluscs from a range of habitats and soft substrates comprising 21 and 17% MNI at these sites respectively. Pango la Watoro and Mvuleni exhibit similar distributions, with both containing proportionally higher intertidal flat-reef coral and rubble taxa (14 and 16% MNI respectively) and shoreline intertidal rock/mangrove taxa (32 and 20% MNI respectively). Unguja Ukuu contains a higher proportion of shoreline intertidal-intertidal flat sand and/or mud taxa (26% MNI), intertidal flat-reef coral, rock, rubble and sand taxa (23% MNI), and intertidal flat-reef coral and rock taxa (12% MNI). Ras Mkumbuu and Mifupani exhibit very different patterns of habitat representation. Mifupani contains a combined 40% MNI of taxa from shoreline intertidal-intertidal flat sand/mud habitats, with a lower proportional representation across all other habitat categories. Ras Mkumbuu contains 81% MNI of taxa from shoreline intertidal-intertidal flat sand and/or mud substrates, being the assemblage most skewed towards a single habitat zone.
As with the results of taxonomic representation above, the trends in proportional habitat designations at genus level are largely reproduced at species level (Figure 11). The exclusion of the genus only level data shifts the proportions of some habitat zones, as well as expanding on the range of identified habitat categories, moving from more inclusive genus to more exclusive or explicit species categories. That said, the distributional patterns are similar across the taxonomic levels. This is particularly the case with the degree of skew in the data from the four Kuumbi Cave occupation phases, Ras Mkumbuu, and Mifupani. The habitat distributions from Fukuchani, Msuka Mjini, Pango la Watoro, and Mvuleni are still concentrated within particular habitat areas, but there is now a slightly flatter distribution, with the peaks observable in the genus level data being slightly reduced or redistributed. In contrast, the species level habitat distribution for Unguja Ukuu now shows a greater proportional representation in the shoreline intertidal-intertidal flat sand and/or mud habitat (33% MNI) and the intertidal flat-reef coral/rock habitat (30% MNI), with all other habitat zones being substantially reduced.
The chord distance results for habitat representation highlights several key similarities (Supplementary Material S2). Kuumbi Cave demonstrates minimal dissimilarity between all occupation phases regardless of whether habitat representation is viewed at genus or species level. Similarly, there is moderate dissimilarity when comparing Unguja Ukuu with Fukuchani, Ras Mkumbuu and Msuka Mjini. Moderate dissimilarity is noted between Pango la Watoro and Mvuleni, and between both sites relative to all Kuumbi Cave phases. Mifupani exhibits moderate dissimilarity when compared with Unguja Ukuu, Ras Mkumbuu and Pango la Watoro. The main differences in chord distance are slightly increasing values from genus to species when comparing Mvuleni with both Fukuchani and Unguja Ukuu, with slightly decreasing values exhibited from genus to species when comparing Msuka Mjini with both Ras Mkumbuu and Mifupani. The major difference in habitat representation occurs between Fukuchani and Msuka Mjini, increasing from minimal to moderate-high dissimilarity from genus to species. All other comparisons present moderate to higher levels of dissimilarity.
5 Discussion
In their review of the Swahili coast zooarchaeological record, Quintana Morales and Prendergast (2018) highlight a chronological trajectory in the exploitation of terrestrial and marine fauna. The earliest coastal records indicate a focus on wild terrestrial game, fishing and mollusc harvesting, with marine resource use continuing with the incorporation of food production while wild terrestrial hunting reduced through time. Between the first and second millennium CE, as socio-economic structures shifted concomitant with increasing urbanism, social changes and long-distance trade, people focused more intensively on domesticated animals and offshore marine environments. Interestingly, this perspective connects to terrestrial vertebrates and marine fish resources more than mollusc foraging (Quintana Morales et al., 2022), as any shift away from the shoreline and broader intertidal zone is not reflected in the archaeological or modern socio-ecological data. Although available data indicate the maintenance of intertidal foraging as an economic activity in the Zanzibar Archipelago, as people engaged with the intertidal zone in complex ways, key differences in nearshore marine foraging occur through time and space.
The Kuumbi Cave data indicate different behaviours to those seen in the other assemblages from Unguja and Pemba. Across the four occupation phases there is a pattern of low richness and moderate to high diversity in assemblage structure, representing a small number of key taxa harvested from across the intertidal gradient, but with an emphasis on mangrove, rock, and reef hard-substrate gastropod species (e.g., Nerita spp, Turbo spp. And Lunella coronata). Interestingly, these characteristics are consistent across all occupation phases irrespective of chronology, changing sea levels or climatic fluctuation, being evident in the two LSA phases at c.19,400 (KC 3/4) and 12,500 BP (KC 2), and in the MIA and LIA phases at c.1200 (KC 1B) and 750 BP (KC 1A). This indicates accessibility of shoreline, intertidal flat and reef habitats on Unguja’s eastern coast before separation of the island from the mainland c.9,000 BP, and into the late Holocene. Due largely to the steep bathymetry off the eastern Unguja coast, in a broad sense there has been comparatively minimal change in the structure of the dominant coastal habitats through time. Environmental composition and availability of taxa, however, are not the primary determinants of the Kuumbi Cave assemblage characteristics, as we would expect a range of intertidal flat habitats to have been accessible throughout the last 20,000 years. As in situ processing of harvested molluscs on the coast is likely, followed by differential transportation (sensu Bird et al., 2002; Meehan 1982), the nature of intertidal foraging during the LSA and prior to island formation cannot be easily identified, although the MIA/LIA assemblages give some indication of likely foraging behaviours that connect to the two late Holocene Kuumbi Cave occupation phases. The re-analyses of the Kuumbi Cave data and comparison with other Unguja/Pemba assemblages reinforce the initial interpretation of Faulkner et al. (2019b), that these assemblages primarily reflect a combination of foraging and transportation, with the latter being the key driver explaining the high similarity in assemblage structure and composition through time. Similar issues were flagged in South Africa (e.g., Plug, 2006; Steele and Klein, 2013) and at Panga ya Saidi in Kenya (Faulkner et al., 2021). The Kuumbi Cave assemblages therefore reflect selectivity for transportation, with the movement of key taxa from the coast in the order of 7–8 km during the Pleistocene, and c.2.5 km over the last 2,000 years.
Ethnographic data collection at Mifupani (Sarathi 2020) took place between June 2018 and June 2019 and involved the direct observation of shellfish and fish harvested by c.30 male and female informants on individual gathering trips. Mifupani represents a true generalised foraging strategy (Szabó, 2009; Harris et al., 2016; Harris and Weisler, 2017), with the assemblage characterised by high richness and high diversity, and although there is a proportionally greater representation of species from a variety of nearshore unconsolidated substrate habitats, people access all available intertidal habitat areas from the shoreline to fringing reef. Canoe use combined with wadable shallow waters created by the fossil reef system allows for the exploitation of multiple habitats. Significant factors absent in the archaeological past influence marine faunal exploitation at Mifupani, including the major impact of the tourist industry on maritime subsistence activities. The participation of Mifupani’s residents in a market economy also greatly affected their subsistence decisions, e.g., the availability of meat for purchase having impact on how fishing and shellfish gathering took place in the village.
On Unguja, recent socio-ecological studies in Nungwi (Nordlund et al., 2010), Chwaka Bay (Fröcklin et al., 2014), and Menai Bay (Yahya, 2013) have focused on understanding critical elements of intertidal foraging primarily by women, and the increasing impacts of human predation pressure, anthropogenic habitat disturbance and climate change. These studies highlight differences in species abundance, richness, and diversity between areas under intensive collection and those subjected to minimal harvesting. Interestingly, 224 harvested species were identified by respondents at Nungwi in the qualitative interviews, however only 10 taxa were recorded during the biological field sampling (Nordlund et al., 2010). This is similar to data from Menai Bay, albeit to a lower degree, with 26 taxa recorded during the biological surveys and 34 taxa from the catch assessments (Yahya, 2013). In contrast, at Chwaka Bay the higher numbers of taxa were recorded during the biological surveys (39 taxa) compared to 21 taxa from the catch assessments (Fröcklin et al., 2014).
On the mainland, Msemwa (1994) focused on areas encompassing protected embayments with fringing reefs or coral islands, with areas of straight coastline excluded based on stronger wave action. Ethnographic observation was restricted to September-October, combining informal and formal interviews with first-hand observation. Emphasis was placed on collection during periods of spring tides, which correlate with productive fishing. The nature of harvesting and the taxa being collected were largely determined by shoreline characteristics and habitat structure, which were associated with species diversity and availability. Based on these data, it is suggested that mollusc collection around Dar es Salaam is a secondary activity used to cushion periods of fish scarcity. In this context, Msemwa (1994) recorded only 17 commonly harvested taxa, including six bivalves and 11 gastropods, with collection driven by factors including subsistence, use as bait, and commercial sale. Although most taxa could be collected year-round, Anadara antiquata was the dominant taxon harvested due to meat yield and accessibility, with individuals concentrated/clustering within the intertidal zone.
The full Mifupani study revealed the exploitation of many fish and invertebrate taxa totaling 281 individual species and incorporating 126 mollusc taxa. Most fishing and foraging took place in the shallows created by Mifupani’s fossil reef, with minimal influence from tool use or modes of transportation. Collection was for subsistence, with no clear seasonal pattern of harvest for most taxa, although future analyses may reveal seasonal variation in exploitation patterns. Unlike Msemwa (1994), Sarathi (2020) concludes that fishing and mollusc gathering served as important sources of meat rather than as “crisis” foods. The most important factor affecting what was harvested is the availability of taxa, although this is now influenced by increased touristic activity in the intertidal area, climate change, and levels of human predation.
Fukuchani, Unguja Ukuu, Msuka Mjini, Pango la Watoro and Mvuleni exhibit similarities and differences across the range of comparative analyses. Fukuchani, Unguja Ukuu, Pango la Watoro and Mvuleni are all characterised by moderate species richness and moderate to high diversity, with Msuka Mjini also demonstrating moderate to high diversity but low species richness. There is variability in the diversity metrics, and which assemblages are significantly different from each other, depending on the taxonomic level being assessed. Evaluation of taxonomic and habitat representation using chord distance indicates a generally moderate to high distance value in comparing each of these five assemblages, although again these results vary depending on whether the assessment is at genus or species level. Correspondence analysis indicates greater similarity between Msuka Mjini, Unguja Ukuu and Fukuchani at species level, with Mvuleni and Pango la Watoro falling separately, but in closer proximity to these three assemblages than to Kuumbi Cave, Ras Mkumbuu or Mifupani. In both taxonomic and habitat representation there are broad similarities, but in many respects these are moderate. What draws these assemblages together is the proportional distribution of taxa and habitats, with broadly similar patterns loosely grouping the MIA-LIA assemblages from Fukuchani, Unguja Ukuu and Msuka Mjini, and grouping the colonial period Pango la Watoro and Mvuleni assemblages. The Fukuchani and Unguja Ukuu assemblages were previously interpreted as reflecting generalised foraging influenced by local habitat structure linked to species richness and diversity within the environment (Faulkner et al., 2018). The higher resolution analyses undertaken here reinforce this interpretation, with Msuka Mjini demonstrating similar trends. All three sites therefore reflect a general foraging pattern as one component of a broad-based economic structure that encompasses hunting and trapping, fishing, and food production to greater or lesser degrees. Pango la Watoro and Mvuleni are somewhat similar, but indicate a higher degree of selectivity and focus on hard substrate habitats across the intertidal gradient. This is unlikely to be purely determined by environmental factors and habitat distribution, particularly given the proximity of Mvuleni to Fukuchani and Pango la Watoro to Msuka Mjini, and the nearshore characteristics of both locations. In these cases, foraging strategies may be influenced by broader socio-economic transitions on the coast associated with the colonial period. As such, all sites exhibit somewhat generalised foraging strategies with variability mediated by the nature of their physical and social environmental contexts.
Extending from this last group of assemblages, Ras Mkumbuu provides evidence for a different set of foraging behaviours, deviating substantially from the other MIA-LIA assemblages in the archipelago, as well as the modern Mifupani data. Located on a long narrow peninsula, the site represents an urban complex bordered to the north and south by expansive intertidal sand and mud flats, patch and fringing reefs. These habitat zones are interspersed with patches of sandy beach and rocky headlands, indicating a mosaic of environments that would have supported a rich and diverse fauna. The reef systems in this area are particularly productive, supporting high taxonomic diversity. The molluscan assemblage, however, is characterised by a moderate degree of richness and very low diversity, being overwhelmingly dominated by the shoreline intertidal-intertidal flat sand/mud infaunal bivalve Anadara antiquata (84% MNI). Although the intertidal flats are prominent, the focused gathering of A. antiquata to the exclusion of all other taxa and available habitats indicates that foraging strategies and species selection were not determined by environmental structure. In addition, the fish assemblages, although small, indicate a focus on coral, rocky and outer reef taxa, with less exploitation of estuarine and inshore zones, and no sandy/muddy floor species (Quintana Morales and Horton, 2014). The Ras Mkumbuu evidence appears to conform more to Msemwa’s (1994) modern urban foraging data, as well as the predictions proposed by Fleisher (2003) for the nature of mollusc foraging in complex urban sites. At Ras Mkumbuu, intertidal foraging and mollusc harvesting appears to reflect a strategy of highly focused gathering of an accessible and abundant species as a supplementary resource.
6 Conclusion
The longitudinal, multi-site comparative analyses of the Zanzibar Archipelago molluscan assemblages clearly highlight that the environment, habitat configuration, taxonomic distribution and density all provide the parameters for foraging across the intertidal zone. Complex relationships between these factors vary spatially and chronologically, dependant on broader environmental processes and how these manifest locally. This does not mean, however, that there is a simple, deterministic relationship between environment and human foraging behaviour, with the nature of intertidal foraging mediated by human choice embedded within specific socio-economic contexts. Given the complexities in the ways people engage with the dynamic conditions of the intertidal zone, it is not surprising that there is no single pattern of foraging behaviour across Unguja and Pemba.
The available data for the Zanzibar Archipelago have limitations, principally the lack of evidence for occupation between the terminal Pleistocene and mid to late Holocene, as well as our ability to incorporate broader economic data for each of the sites discussed here. For example, while high-resolution terrestrial and marine vertebrate data and archaeobotanical evidence are currently available for Kuumbi Cave, Unguja Ukuu and Fukuchani, further detailed analyses are required (or are ongoing) for the other archaeological sites incorporated into the analyses above. That said, in approaching the available data in a systematic manner, incorporating archaeomalacological and ecologically oriented modes of analysis across multiple archaeological contexts, we are able to move beyond interpretations constrained to individual sites. The analyses and results presented here provide robust baseline data for future investigations of human-ecodynamics on the coast and islands of eastern Africa, highlighting combinations of stability and variability in foraging behaviours that effectively argue against a single trajectory of foraging behaviour within the intertidal zone.
Data availability statement
The original contributions presented in the study are included in the article/Supplementary Material, further inquiries can be directed to the corresponding authors.
Ethics statement
The studies involving human participants were reviewed and approved by the University of Madison-Wisconsin Institutional Review Board (2016-1353). The patients/participants provided their written informed consent to participate in this study.
Author contributions
PF, AS, and AC contributed to conception and design of the study. PF, AS, TS, MH, AA, and OH collected the assemblage data. PF performed the analyses and wrote the first draft of the manuscript. AS, AL, NN, and AC wrote sections of the manuscript. MH produced Figures 1, 2. PF, AS, AC, TS, MH, AA, OH, AL, NN, MH, and NB contributed to manuscript revision, read, and approved the submitted version.
Funding
PF was funded by a Faculty of Arts and Social Sciences Research Support Scheme grant and Tom Austen Brown Research funding (University of Sydney), and by the Max Planck Society. PF is an Australian Research Council Future Fellow (FT200100464). AS was funded by grants from the Fulbright-Hays Program (award#P022A170037), the National Geographic Society (award#EC-227-18), the Department of Anthropology at the University of Wisconsin-Madison, and the University of Wisconsin-Madison Graduate School. AC is an Australian Research Council Future Fellow (FT200100179) and was funded by postdoctoral fellowships from the British Academy and The University of Queensland. AL and NN were supported by Springer/Nature and the British Institute in Eastern Africa. NB was funded by a European Research Council grant for the SEALINKS Project (grant no. 206148). Radiocarbon dates for the Sealinks Project were provided by a UK Natural Environment Research Council grant (NF/2012/2/4).
Acknowledgments
We are grateful to the Zanzibar Department of Museums and Antiquities and the Office of Chief Government Statistician (Zanzibar Research Committee) for supporting our archaeological fieldwork and providing permission to conduct the research. PF, AC, MH, and NB extend their appreciation to the local communities on Pemba and Unguja, particularly in Makangale, Fukuchani, Unguja Ukuu, and Jambiani. AL and NN thank PF for his invitation to contribute to this paper. They are deeply grateful to AA for his magnanimity in all aspects of this research; the student crews for their many contributions, and the communities in Nungwi, Fukuchani and Mvuleni for their gracious assistance. AS is especially grateful to the people of Mifupani for sharing their subsistence activities with researchers daily for more than a year. Special thanks are due to Muhidini Mgana and Ameir Jecha for facilitating research at Mifupani. Finally, we thank the guest editorial team of Manuel Will, Andrew Green, Jon Erlandson, Ximena Suarez Villagran and Antonieta Jerardino for their invitation to contribute to this special issue.
Conflict of interest
The authors declare that the research was conducted in the absence of any commercial or financial relationships that could be construed as a potential conflict of interest.
Publisher’s note
All claims expressed in this article are solely those of the authors and do not necessarily represent those of their affiliated organizations, or those of the publisher, the editors and the reviewers. Any product that may be evaluated in this article, or claim that may be made by its manufacturer, is not guaranteed or endorsed by the publisher.
Supplementary material
The Supplementary Material for this article can be found online at: https://www.frontiersin.org/articles/10.3389/feart.2022.982694/full#supplementary-material
References
Abbott, R. T., and Dance, S. P. (1998). Compendium of seashells: A color guide to more than 4,200 of the world's marine shells. El Cajon, California: Odyssey Publishers.
R. T. Abbott (Editor) (1959). Introduction and the family Vasidae in the Indo-Pacific. Indo-Pacific Mollusca 1, 1–32
R. T. Abbott (Editor) (1961). The genus Lambis in the Indo-Pacific. Indo-Pacific Mollusca. 1, 147–174.
R. T. Abbott (Editor) (1960). The genus Strombus in the Indo-Pacific. Indo-Pacific Mollusca 1, 33–144
Accordi, G., and Carbone, F. (2016). Evolution of the siliciclastic-carbonate shelf system of the northern Kenyan coastal belt in response to Late Pleistocene-Holocene relative sea level changes. J. Afr. Earth Sci. 123, 234–257. doi:10.1016/j.jafrearsci.2016.08.003
Agnarsson, I., and Kuntner, M. (2012). “The generation of a biodiversity hotspot: Biogeography and phylogeography of the Western Indian ocean islands,” in Current topics in phylogenetics and phylogeography of terrestrial and aquatic systems. Editors K. Anamthawat-Jónsson, and IntechOpen, 33–82. doi:10.5772/1947
Almeida‐Neto, M., Guimaraes, P., Guimaraes, P. R., Loyola, R. D., and Ulrich, W. (2008). A consistent metric for nestedness analysis in ecological systems: Reconciling concept and measurement. Oikos 117 (8), 1227–1239. doi:10.1111/j.0030-1299.2008.16644.x
Arthurton, R. S., Brampton, A. H., Kaaya, C. Z., and Mohamed, S. K. (1999). Late quaternary coastal stratigraphy on a platform-fringed tropical coast: A case study from Zanzibar, Tanzania. J. Coast. Res. 15 (3), 635–644.
Arthurton, R. (2003). The fringing reef coasts of eastern Africa—Present processes in their long-term context. West. Ind. oc. J. Mar. Sci. 2 (1), 1–14. doi:10.4314/wiojms.v2i1.28424
Atmar, W., and Patterson, B. D. (1993). The measure of order and disorder in the distribution of species in fragmented habitat. Oecologia 96 (3), 373–382. doi:10.1007/bf00317508
Bascompte, J., Jordano, P., Melián, C. J., and Olesen, J. M. (2003). The nested assembly of plant–animal mutualistic networks. Proc. Natl. Acad. Sci. U. S. A. 100 (16), 9383–9387. doi:10.1073/pnas.1633576100
Berkström, C., Lindborg, R., Thyresson, M., and Gullström, M. (2013). Assessing connectivity in a tropical embayment: Fish migrations and seascape ecology. Biol. Conserv. 166, 43–53. doi:10.1016/j.biocon.2013.06.013
Bird, D. W., Richardson, J. L., Veth, P. M., and Barham, A. J. (2002). Explaining shellfish variability in middens on the Meriam Islands, Torres Strait, Australia. J. Archaeol. Sci. 29 (5), 457–469. doi:10.1006/jasc.2001.0734
Burgess, N. D., Clarke, G. P., and Rodgers, W. A. (1998). Coastal forests of eastern Africa: Status, endemism patterns and their potential causes. Biol. J. Linn. Soc. 64 (3), 337–367. doi:10.1111/j.1095-8312.1998.tb00337.x
Camoin, G. F., Colonna, M., Montaggioni, L. F., Casanova, J., Faure, G., and Thomassin, B. A. (1997). Holocene sea level changes and reef development in the southwestern Indian Ocean. Coral Reefs 16 (4), 247–259. doi:10.1007/s003380050080
Camoin, G. F., Montaggioni, L. F., and Braithwaite, C. J. R. (2004). Late glacial to post glacial sea levels in the Western Indian Ocean. Mar. Geol. 206 (1-4), 119–146. doi:10.1016/j.margeo.2004.02.003
K. E. Carpenter, and V. H. Niem (Editors) (1998). The living marine resources of the Western Central Pacific: Volume 1. Seaweeds, corals, bivalves and gastropods. FAO species identification guide for fishery purposes (Rome: Food and Agriculture Organization of the United Nations).
Cernohorsky, W. O. (1984). Systematics of the family Nassariidae (Mollusca: Gastropoda). Bull. Auckland Inst. Mus., 14, 1–356.
Chami, F. (2009). Zanzibar and the Swahili Coast from c. 30,000 years ago. Dar es Salaam, Tanzania: E&D Vision Pub.
Christie, A. C. (2013). “Exploring the social context of maritime exploitation in Tanzania between the 14th-18th c. AD: Recent research from the Mafia archipelago,” in. Prehistoric marine resource use in the Indo-Pacific regions. Editors R. Ono, A. Morrison, and D. Addison, 39, 97–122. Terra Aust.: Canberra: ANU Press
Colonna, M., Casanova, J., Dullo, W. C., and Camoin, G. (1996). Sea-level changes and δ18O record for the past 34, 000 yr from Mayotte Reef, Indian Ocean. Quat. Res. 46 (3), 335–339. doi:10.1006/qres.1996.0071
Crowther, A., Lucas, L., Helm, R., Horton, M., Shipton, C., Wright, H. T., et al. (2016). Ancient crops provide first archaeological signature of the westward Austronesian expansion. Proc. Natl. Acad. Sci. U. S. A. 113 (24), 6635–6640. doi:10.1073/pnas.1522714113
Crowther, A., Veall, M. A., Boivin, N., Horton, M., Kotarba-Morley, A., Fuller, D. Q., et al. (2015). Use of zanzibar copal (Hymenaea verrucosa Gaertn.) as incense at Unguja Ukuu, Tanzania in the 7–8th century CE: Chemical insights into trade and Indian ocean interactions. J. Archaeol. Sci. 53, 374–390. doi:10.1016/j.jas.2014.10.008
Culley, C., Janzen, A., Brown, S., Prendergast, M. E., Wolfhagen, J., Abderemane, B., et al. (2021). Collagen fingerprinting traces the introduction of caprines to island Eastern Africa. R. Soc. open Sci. 8 (7), 202341. doi:10.1098/rsos.202341
Dekker, H. (2018). A note on the genus Rubitrochus (Gastropoda, Vetigastropoda, Trochidae), with descriptions of five new species. Basteria 82 (1-3), 1–14.
Douglass, K., Antonites, A. R., Quintana Morales, E. M., Grealy, A., Bunce, M., Bruwer, C., et al. (2018). Multi-analytical approach to zooarchaeological assemblages elucidates Late Holocene coastal lifeways in southwest Madagascar. Quat. Int. 471, 111–131. doi:10.1016/j.quaint.2017.09.019
Douglass, K. (2017). The diversity of late Holocene shellfish exploitation in Velondriake, southwest Madagascar. J. Isl. Coast. Archaeol. 12 (3), 333–359. doi:10.1080/15564894.2016.1216480
Erlandson, J. M. (2001). The archaeology of aquatic adaptations: Paradigms for a new millennium. J. Archaeol. Res. 9 (4), 287–350. doi:10.1023/a:1013062712695
Faith, J. T., and Du, A. (2018). The measurement of taxonomic evenness in zooarchaeology. Archaeol. Anthropol. Sci. 10 (6), 1419–1428. doi:10.1007/s12520-017-0467-8
Faulkner, P., Harris, M., Ali, A. K., Haji, O., Crowther, A., Horton, M. C., et al. (2018). Characterising marine mollusc exploitation in the eastern African Iron Age: Archaeomalacological evidence from Unguja Ukuu and Fukuchani, zanzibar. Quat. Int. 471, 66–80. doi:10.1016/j.quaint.2017.08.051
Faulkner, P., Harris, M., Haji, O., Ali, A. K., Crowther, A., Shipton, C., et al. (2019a). Long-term trends in terrestrial and marine invertebrate exploitation on the eastern African coast: Insights from Kuumbi Cave, Zanzibar. J. Isl. Coast. Archaeol. 14 (4), 479–514. doi:10.1080/15564894.2018.1501442
Faulkner, P., Harris, M., Haji, O., Crowther, A., Horton, M. C., and Boivin, N. L. (2019b). Towards a historical ecology of intertidal foraging in the Mafia archipelago: Archaeomalacology and implications for marine resource management. J. Ethnobiol. 39 (2), 182–203. doi:10.2993/0278-0771-39.2.182
Faulkner, P., Miller, J. M., Quintana Morales, E. M., Crowther, A., Shipton, C., Ndiema, E., et al. (2021). 67, 000 years of coastal engagement at Panga ya Saidi, eastern Africa. PloS ONE 16 (8), e0256761. doi:10.1371/journal.pone.0256761
Fitton, T. (2017). Pushing the boat out: A study of spatial organisation and harbour spaces in the early Swahili ports of the Zanzibar archipelago, 550–1100 CE. PhD dissertation. York: University of York.
Fitton, T., and Wynne-Jones, S. (2017). Understanding the layout of early coastal settlement at Unguja Ukuu, Zanzibar. Antiquity 91 (359), 1268–1284. doi:10.15184/aqy.2017.141
Fitzhugh, B., Butler, V. L., Bovy, K. M., and Etnier, M. A. (2019). Human ecodynamics: A perspective for the study of long-term change in socioecological systems. J. Archaeol. Sci. Rep. 23, 1077–1094. doi:10.1016/j.jasrep.2018.03.016
Fleisher, J. B. (2003). Viewing stonetowns from the countryside: An archaeological approach to Swahili regional systems, AD 800–1500. PhD dissertation. Charlottesville: University of Virginia.
Fleisher, J., Lane, P., LaViolette, A., Horton, M., Pollard, E., Quintana Morales, E., et al. (2015). When did the Swahili become maritime? Am. Anthropol. 117 (1), 100–115. doi:10.1111/aman.12171
Fleisher, J., and Wynne-Jones, S. (2011). Ceramics and the early Swahili: Deconstructing the early Tana tradition. Afr. Archaeol. Rev. 28, 245–278. doi:10.1007/s10437-011-9104-6
Fröcklin, S., de la Torre-Castro, M., Håkansson, E., Carlsson, A., Magnusson, M., and Jiddawi, N. S. (2014). Towards improved management of tropical invertebrate fisheries: Including time series and gender. PLoS One 9 (3), e91161. doi:10.1371/journal.pone.0091161
GADM (2022). Global administrative areas version 4.0.4. Berkeley: University of California. [digital geospatial data]. Available online: http://www.gadm.org [20/06/2022.
Giovas, C. M. (2009). The shell game: Analytic problems in archaeological mollusc quantification. J. Archaeol. Sci. 36 (7), 1557–1564. doi:10.1016/j.jas.2009.03.017
Glassman, J. (2011). War of words, war of stones: Racial thought and violence in colonial zanzibar. Bloomington: Indiana University Press.
Grayson, D. K. (1984). Quantitative zooarchaeology: Topics in the analysis of archaeological fauna. Orlando, Florida: Academic Press.
Hammer, Ø., Harper, D. A., and Ryan, P. D. (2001). Past: Paleontological statistics software package for education and data analysis. Palaeontol. Electron. 4 (1), 9
Hammer, Ø., and Harper, D. A. T. (2007). Paleontological data analysis. Malden, MA: Blackwell Publishing.
Harris, M., Lambrides, A. B., and Weisler, M. I. (2016). Windward vs. leeward: Inter-site variation in marine resource exploitation on Ebon Atoll, Republic of the Marshall Islands. J. Archaeol. Sci. Rep. 6, 221–229. doi:10.1016/j.jasrep.2016.02.012
Harris, M., Weisler, M., and Faulkner, P. (2015). A refined protocol for calculating MNI in archaeological molluscan shell assemblages: A Marshall Islands case study. J. Archaeol. Sci. 57, 168–179. doi:10.1016/j.jas.2015.01.017
Harris, M., and Weisler, M. (2017). Intertidal foraging on atolls: Prehistoric forager decision-making at Ebon Atoll, Marshall Islands. J. Isl. Coast. Archaeol. 12 (2), 200–223. doi:10.1080/15564894.2016.1167140
Healy, J., Norman, M., Potter, D., and Willan, R. (2011). “Molluscs,” in Wild guide to Moreton Bay and adjacent coasts. Queensland Museum). Editors P. Davie, and Brisbane. 2nd edition, Vol. 2, 119–196
Horton, M., and Chami, F. (2018). “Swahili origins,” in The Swahili World. Editors S. Wynne-Jones, and A. LaViolette (Milton: Routledge), 135
Horton, M. C., and Middleton, J. (2000). The Swahili: The social landscape of a mercantile society. Oxford: Blackwell Publishers.
Horton, M. C. (2017). Zanzibar and Pemba: The archaeology of an Indian Ocean trading archipelago. London: British Institute in Eastern Africa.
Ichumbaki, E. B. (2014). Archaeological and ethnographic evidence for the historic consumption of fish and shellfish along the coast of East Africa in Tanzania. J. Indian Ocean Archaeol. 10, 1–18
Jerardino, A. (2016). On the origins and significance of Pleistocene coastal resource use in southern Africa with particular reference to shellfish gathering. J. Anthropol. Archaeol. 41, 213–230. doi:10.1016/j.jaa.2016.01.001
Johnson, S., Domínguez-García, V., and Muñoz, M. A. (2013). Factors determining nestedness in complex networks. PloS ONE 8 (9), e74025. doi:10.1371/journal.pone.0074025
Juma, A. M. (2004). Unguja Ukuu on Zanzibar: An archaeological study of early urbanism. PhD dissertation. Uppsala: Department of Archaeology and Ancient History, Uppsala University.
Khamis, Z. A., Kalliola, R., and Käyhkö, N. (2017). Geographical characterization of the Zanzibar coastal zone and its management perspectives. Ocean Coast. Manag. 149, 116–134. doi:10.1016/j.ocecoaman.2017.10.003
Kirkman, J. (1959). Excavations at Ras Mkumbuu on the island of Pemba. Tanganyika Notes Rec. 53, 161–178
Klein, R. G., and Bird, D. W. (2016). Shellfishing and human evolution. J. Anthropol. Archaeol. 44, 198–205. doi:10.1016/j.jaa.2016.07.008
Kotarba-Morley, A. M., Kourampas, N., Morley, M. W., MacAdams, C., Crowther, A., Faulkner, P., et al. (2022). Coastal landscape changes at Unguja Ukuu, Zanzibar: Contextualizing the archaeology of an early Islamic port of trade. J. Isl. Coast. Archaeol., 1–35. doi:10.1080/15564894.2022.2030441
Kourampas, N., Shipton, C., Mills, W., Tibesasa, R., Horton, H., Horton, M., et al. (2015). Late Quaternary speleogenesis and landscape evolution in a tropical carbonate island: Pango la Kuumbi (Kuumbi Cave), Zanzibar. Int. J. Speleol. 44 (3), 293–314. doi:10.5038/1827-806x.44.3.7
Kuhnert, H., Kuhlmann, H., Mohtadi, M., Meggers, H., Baumann, K. H., and Pätzold, J. (2014). Holocene tropical Western Indian Ocean sea surface temperatures in covariation with climatic changes in the Indonesian region. Paleoceanography 29 (5), 423–437. doi:10.1002/2013pa002555
Lamprell, K., and Whitehead, T. (1992). Bivalves of Australia, Vol. 1. Bathurst, NSW: Crawford House Press.
Lane, P. J., and Breen, C. P. (2018). “The eastern African coastal landscape,” in The Swahili World. Editors S. Wynne-Jones, and A. LaViolette (Milton: Routledge), 19–35.
LaViolette, A., and Norman, N. (2023). “The archaeology of Portuguese agricultural outposts in the 17th-century Zanzibar countryside,” in Modern worlds and archaeological records in the Indian ocean. Editors J. Haines, and M. Hauser (Gainesville, FL: University of Florida Press)
LaViolette, A. (2008). Swahili cosmopolitanism in Africa and the Indian Ocean world, AD 600–1500. Arch. 4, 24–49. doi:10.1007/s11759-008-9064-x
Legendre, P., and Gallagher, E. D. (2001). Ecologically meaningful transformations for ordination of species data. Oecologia 129 (2), 271–280. doi:10.1007/s004420100716
Liu, X., Rendle-Bühring, R., and Henrich, R. (2018). High-and low-latitude forcing of the East African climate since the LGM: Inferred from the elemental composition of marine sediments off Tanzania. Quat. Sci. Rev. 196, 124–136. doi:10.1016/j.quascirev.2018.08.004
Liu, X., Rendle-Bühring, R., Kuhlmann, H., and Li, A. (2017). Two phases of the Holocene East African Humid Period: Inferred from a high-resolution geochemical record off Tanzania. Earth Planet. Sci. Lett. 460, 123–134. doi:10.1016/j.epsl.2016.12.016
Liu, X., Rendle-Bühring, R., Meyer, I., and Henrich, R. (2016). Holocene shelf sedimentation patterns off equatorial East Africa constrained by climatic and sea-level changes. Sediment. Geol. 331, 1–11. doi:10.1016/j.sedgeo.2015.10.009
Marean, C. W. (2014). The origins and significance of coastal resource use in Africa and Western Eurasia. J. Hum. Evol. 77, 17–40. doi:10.1016/j.jhevol.2014.02.025
Marean, C. W. (2016). The transition to foraging for dense and predictable resources and its impact on the evolution of modern humans. Phil. Trans. R. Soc. B 371 (1698), 20150239. doi:10.1098/rstb.2015.0239
Mörner, N. (1992). “Ocean circulation, sea level changes and east African coastal settlements,” in Urban origins in eastern Africa: Proceedings of the 1991 workshop in zanzibar. Editors P. J. J. Sinclair, and A. Juma (Stockholm: Swedish Central Board of National Antiquities), 256–266
Msemwa, P. J. (1994). An ethnoarchaeological study on shellfish collecting in a complex urban setting. PhD dissertation. Providence, Rhode Island: Brown University.
Mudida, N., and Horton, M. (1996). “Subsistence at Shanga: The faunal record,” in Shanga: The archaeology of a Muslim trading community on the coast of East Africa. Editor M. Horton (London: British Institute in Eastern Africa), 378–393
Nordlund, L., Erlandsson, J., de la Torre-Castro, M., and Jiddawi, N. (2010). Changes in an east African social-ecological seagrass system: Invertebrate harvesting affecting species composition and local livelihood. Aquat. Living Resour. 23 (4), 399–416. doi:10.1051/alr/2011006
Nordlund, L. M., de la Torre-Castro, M., Erlandsson, J., Conand, C., Muthiga, N., Jiddawi, N., et al. (2014). Intertidal zone management in the western Indian Ocean: Assessing current status and future possibilities using expert opinions. Ambio 43 (8), 1006–1019. doi:10.1007/s13280-013-0465-8
Plug, I. (2006). Aquatic animals and their associates from the Middle Stone Age levels at Sibudu. South. Afr. Humanit. 18 (1), 289–299
Prendergast, M. E., Quintana Morales, E. M., Crowther, A., Horton, M. C., and Boivin, N. L. (2017). Dietary diversity on the Swahili coast: The fauna from two Zanzibar trading locales. Int. J. Osteoarchaeol. 27 (4), 621–637. doi:10.1002/oa.2585
Prendergast, M. E., Rouby, H., Punwong, P., Marchant, R., Crowther, A., Kourampas, N., et al. (2016). Continental island formation and the archaeology of defaunation on Zanzibar, eastern Africa. PloS one 11 (2), e0149565. doi:10.1371/journal.pone.0149565
Punwong, P., Marchant, R., and Selby, K. (2013a). Holocene mangrove dynamics and environmental change in the Rufiji Delta, Tanzania. Veg. Hist. Archaeobot. 22 (5), 381–396. doi:10.1007/s00334-012-0383-x
Punwong, P., Marchant, R., and Selby, K. (2013b). Holocene mangrove dynamics from Unguja Ukuu, Zanzibar. Quat. Int. 298, 4–19. doi:10.1016/j.quaint.2013.02.007
Punwong, P., Marchant, R., and Selby, K. (2013c). Holocene mangrove dynamics in Makoba Bay, Zanzibar. Palaeogeogr. Palaeoclimatol. Palaeoecol. 379, 54–67. doi:10.1016/j.palaeo.2013.04.004
Punwong, P., Selby, K., and Marchant, R. (2018). Holocene mangrove dynamics and relative sea-level changes along the Tanzanian coast, East Africa. Estuar. Coast. Shelf Sci. 212, 105–117. doi:10.1016/j.ecss.2018.07.004
Quintana Morales, E. M., Craig, O. E., Prendergast, M. E., Walshaw, S., Cartaciano, C., Mwebi, O., et al. (2022). Diet, economy, and culinary practices at the height of precolonial Swahili urbanism. J. Anthropol. Archaeol. 66, 101406. doi:10.1016/j.jaa.2022.101406
Quintana Morales, E. M., and Horton, M. (2014). Fishing and fish consumption in the Swahili communities of East Africa, 700–1400 CE. Internet Archaeology 37. doi:10.11141/ia.37.3
Quintana Morales, E. M., and Prendergast, M. E. (2018). “Animals and their uses in the Swahili World,” in The Swahili World. Editors S. Wynne-Jones, and A. LaViolette (Milton: Routledge), 335–349.
Richmond, M. (2014). Coastal profile for zanzibar 2014 region volume II. Dar es Salaam, Tanzania: Samaki consultants, World Bank, Nordic Development Fund.
Richmond, M. D. (2011). A field guide to the seashores of eastern Africa and the western Indian ocean islands. Dar es Salaam, Tanzania: SIDA, WIOMSA.
Robin, A. (2011). Encyclopedia of marine bivalves, including scaphopods, Polyplacophora and cephalopods. Hackenheim, Germany: ConchBooks.
Rødland, H. (2021). Swahili social landscapes: Material expressions of identity, agency, and labour in Zanzibar, 1000–1400 CE. PhD dissertation. Uppsala: Department of Archaeology and Ancient History, Uppsala University.
Rogers, A. J., and Weisler, M. I. (2022). Risk, reliability, and the importance of small-bodied molluscs across the Hawaiian windward-leeward divide. Hum. Ecol. 50 (1), 141–165. doi:10.1007/s10745-021-00297-y
Romahn, S., Mackensen, A., Kuhlmann, H., and Pätzold, J. (2015). Benthic foraminiferal response to late glacial and holocene sea level rise and rainfall variability off East Africa. Mar. Micropaleontol. 119, 34–48. doi:10.1016/j.marmicro.2015.05.003
Rowson, B., Warren, B. H., and Ngereza, C. F. (2010). Terrestrial molluscs of Pemba Island, Zanzibar, Tanzania, and its status as an “oceanic” island. ZooKeys 70, 1–39. doi:10.3897/zookeys.70.762
Santoro, C. M., Gayo, E. M., Carter, C., Standen, V. G., Castro, V., Valenzuela, D., et al. (2017). Loco or no loco? Holocene climatic fluctuations, human demography, and community based management of coastal resources in northern Chile. Front. Earth Sci. (Lausanne). 5, 16. doi:10.3389/feart.2017.00077
Sarathi, A. (2020). Glocalized Indian ocean foodscapes in the Swahili World: An ethnoarchaeological and zooarchaeological case study of Unguja Island (Zanzibar), c.13kya–1600ce. PhD dissertation. Madison: University of Wisconsin-Madison.
Sarathi, A., Kenoyer, J. M., and Walz, J. R. (2022). An early islamic rock crystal amulet seal from Unguja Ukuu, Zanzibar. J. Afr. Arch. 1, 1–13. doi:10.1163/21915784-bja10013
Sarathi, A. (2018). “Shellfish exploitation at Kuumbi cave, zanzibar (c. 11kya–20th cen. CE): A preliminary study,” in Early maritime cultures in east Africa and the western Indian ocean: Papers from a conference held at the university of Wisconsin-Madison (African Studies Program) 23-24 october 2015. Editor A. Sarathi (Oxford: Archaeopress), 171–184.
Semken, H. A., and Graham, R. W. (1996). Paleoecologic and taphonomic patterns derived from correspondence analysis of zooarchaeological and paleontological faunal samples, a case study from the North American prairie-forest ecotone. Acta Zool. Cracoviensia 39 (1), 477–490.
Shefer, S., Feldstein, T., Mienis, H. K., Rittner, O., and Gur, A. (2012). First records of Mimachlamys sanguina (Linnaeus, 1758) from the Mediterranean coast of Israel (Bivalvia, pectinidae). Triton (25), 1–2
Shipton, C., Crowther, A., Kourampas, N., Prendergast, M. E., Horton, M., Douka, K., et al. (2016). Reinvestigation of Kuumbi Cave, Zanzibar, reveals later Stone Age coastal habitation, early Holocene abandonment and Iron Age reoccupation. Azania Archaeol. Res. Afr. 51 (2), 197–233. doi:10.1080/0067270x.2016.1173308
Spalding, M., Kainuma, M., and Collins, L. (2010). A collaborative project of ITTO.” in. World atlas of mangroves (version 3.1) Cambridge, United KingdomLondon: ISME, FAO, UNEP-WCMC, UNESCO-MAB, UNU-INWEH and TNCEarthscan. doi:10.34892/w2ew-m835
Spry, J. F. (1964). the Sea shells of Dar es Salaam: Part II pelecypoda (bivalves).” in Reprinted from Tanganyika notes and records, 63. Dar es Salaam: Tanzania Society.
Spry, J. F. (1968). the Sea shells of Dar es Salaam. Part I. Gastropods.” in Reprinted from Tanganyika notes and records, 56. Dar es Salaam: Tanzania Society.
Stagna, M. D., Maselli, V., Grujic, D., Reynolds, P., Reynolds, D., Iacopini, D., et al. (2022). Structural controls on slope evolution and sediment dispersal pathways along the northern Tanzania continental margin, Western Indian Ocean. Mar. Geol. 443, 106662. doi:10.1016/j.margeo.2021.106662
Steele, T. E., and Klein, R. G. (2013). The Middle and Later Stone Age faunal remains from Diepkloof Rock Shelter, Western Cape, South Africa. J. Archaeol. Sci. 40 (9), 3453–3462. doi:10.1016/j.jas.2013.01.001
Strona, G., Galli, P., Seveso, D., Montano, S., and Fattorini, S. (2014). Nestedness for dummies (NeD): A user-friendly web interface for exploratory nestedness analysis. J. Stat. Softw. 59, 1–9. doi:10.18637/jss.v059.c03
Szabó, K. (2009). “Molluscan remains from Fiji,” in The early prehistory of Fiji. Editors G. Clark, and A. J. Anderson. Terra Aust.: Canberra: ANU E Press
Ulrich, W., Almeida‐Neto, M., and Gotelli, N. J. (2009). A consumer's guide to nestedness analysis. Oikos 118 (1), 3–17. doi:10.1111/j.1600-0706.2008.17053.x
Ulrich, W., and Almeida‐Neto, M. (2012). On the meanings of nestedness: Back to the basics. Ecography 35 (10), 865–871. doi:10.1111/j.1600-0587.2012.07671.x
Unep-Wcmc, , and Short, F. T. (2021). Global distribution of seagrasses (version 7.1). Seventh update to the data layer used in Green and Short. Cambridge, United Kingdom: UN Environment World Conservation Monitoring Centre. doi:10.34892/x6r3-d211
UNEP-WCMC, WorldFish Centre, World Resources Institute, The Nature Conservancy (2021). “Global distribution of warm-water coral reefs, compiled from multiple sources including the Millennium Coral Reef Mapping Project.,” in Includes contributions from IMaRS-USF and IRD (2005), IMaRS-USF (2005) and Spalding et al. (2001) (Cambridge, United Kingdom: UN Environment World Conservation Monitoring Centre). doi:10.34892/t2wk-5t34
Walsh, M. T. (2007). Island subsistence: Hunting, trapping and the translocation of wildlife in the western Indian ocean. Azania Archaeol. Res. Afr. 42 (1), 83–113. doi:10.1080/00672700709480452
Will, M., Kandel, A. W., and Conard, N. J. (2019). Midden or molehill: The role of coastal adaptations in human evolution and dispersal. J. World Prehist. 32 (1), 33–72. doi:10.1007/s10963-018-09127-4
Willan, R. C., Bryce, C., and Slack-Smith, S. M. (2015). Kimberley marine biota. Historical data: Molluscs. Rec. West. Aust. Mus. Sup. 84, 287–343. doi:10.18195/issn.0313-122x.84.2015.287-343
Wilson, B. (1993). Australian marine shells. Prosobranch gastropods volumes 1 and 2. Kallaroo. Kallaroo, Western Australia: Odyssey Publishing.
Wolverton, S., Nagaoka, L., and Rick, T. C. (2016). Applied zooarchaeology: Five case studies. New York: Eliot Werner Publications.
Wolverton, S., Otaola, C., Neme, G., Giardina, M., and Gil, A. (2015). Patch choice, landscape ecology, and foraging efficiency: The zooarchaeology of late Holocene foragers in Western Argentina. J. Ethnobiol. 35 (3), 499–518. doi:10.2993/etbi-35-03-499-518.1
Wood, M., Panighello, S., Orsega, E. F., Robertshaw, P., van Elteren, J. T., Crowther, A., et al. (2016). Zanzibar and Indian Ocean trade in the first millennium CE: The glass bead evidence. Archaeol. Anthropol. Sci. 9 (5), 879–901. doi:10.1007/s12520-015-0310-z
Woodroffe, S. A., and Horton, B. P. (2005). Holocene sea-level changes in the Indo-Pacific. J. Asian earth Sci. 25 (1), 29–43. doi:10.1016/j.jseaes.2004.01.009
Woodroffe, S. A., Long, A. J., Punwong, P., Selby, K., Bryant, C. L., and Marchant, R. (2015). Radiocarbon dating of mangrove sediments to constrain Holocene relative sea-level change on Zanzibar in the southwest Indian Ocean. Holocene 25 (5), 820–831. doi:10.1177/0959683615571422
WoRMS Editorial Board (2022). World register of marine species. Available at: http://www.marinespecies.org (Accessed May 24, 2022).
Yahya, B. M. (2013). The status of intertidal molluscan resources collected by women of Fumba and Unguja Ukuu, Zanzibar. Dar es Salaam, Tanzania: University of Dar es Salaam.
Keywords: archaeomalacology, intertidal foraging, marine subsistence, human-environment interactions, eastern Africa
Citation: Faulkner P, Sarathi A, Crowther A, Smith T, Harris M, Ali AK, Haji O, LaViolette A, Norman NL, Horton M and Boivin N (2022) Human-ecodynamics and the intertidal zones of the Zanzibar Archipelago. Front. Earth Sci. 10:982694. doi: 10.3389/feart.2022.982694
Received: 30 June 2022; Accepted: 12 September 2022;
Published: 03 October 2022.
Edited by:
Manuel Will, University of Tübingen, GermanyReviewed by:
Emma Loftus, Ludwig Maximilian University of Munich, GermanyEsteban Alvarez Fernández, University of Salamanca, Spain
Copyright © 2022 Faulkner, Sarathi, Crowther, Smith, Harris, Ali, Haji, LaViolette, Norman, Horton and Boivin. This is an open-access article distributed under the terms of the Creative Commons Attribution License (CC BY). The use, distribution or reproduction in other forums is permitted, provided the original author(s) and the copyright owner(s) are credited and that the original publication in this journal is cited, in accordance with accepted academic practice. No use, distribution or reproduction is permitted which does not comply with these terms.
*Correspondence: Patrick Faulkner, patrick.faulkner@sydney.edu.au; Nicole Boivin, boivin@shh.mpg.de