- 1School of Geography and Tourism, Qufu Normal University, Rizhao, China
- 2MOE Key Laboratory of Western China’s Environmental Systems & College of Earth and Environmental Sciences, Lanzhou University, Lanzhou, China
- 3Shandong Provincial Eco-environment Monitoring Center, Jinan, China
The Qilian Mountains are a typical active fold-thrust belt. A series of large and elongated drainage basins are oriented almost parallel to the Mountain Chain. Conversely, on North flank of the Qilian Mountains, transverse rivers dominate the drainage network. However, the evolution of these drainage patterns is still poorly understood. Here, we first review the evolutionary history of the drainage pattern of major rivers in the Qilian Mountains. We find that early transverse-dominated river networks are progressively replaced by longitudinal-dominated rivers during mountain building. Because the incision rate of transverse rivers is defeated by the uplift rate of mountains, the transverse rivers would be diverted toward the fold tips and gradually lengthened. Then, we analyze the evolutionary trends of drainage networks using topographic metrics. We suggest that longitudinal rivers, especially the upper reach of longitudinal rivers, will be captured by transverse rivers. Our study shows that the evolution of drainage patterns in active fold-thrust belts has two stages: in the early stage, transverse rivers would be replaced by longitudinal rivers; in the later stage, the upper longitudinal rivers would be captured by transverse tributaries. Moreover, the evolution model of drainage patterns in active fold-thrust belts is validated by using the TopoToolbox Landscape Evolution Model (TTLEM). Tectonics and deformation impart a lasting impression on the planform pattern of drainage networks. However, the drainage network will show different patterns in various evolution stages, even with consistent tectonic conditions. The results of this study may help investigate the drainage network evolution process in other active fold-thrust belts.
1 Introduction
Drainage networks are the dominant external forcing for Earth’s surface landforms, as they carry material from upland mountain belts to the depositional lowlands and sculpt a diversity of landscapes. Beyond its role in shaping the topography, the evolution of drainage networks can reflect tectonics and deformations (Castelltort et al., 2012; Zhang et al., 2012; Willett et al., 2014; Gemignani et al., 2022; Jolivet et al., 2022; Souza et al., 2022). Thus, the drainage network is an effective way to explore the interconnected processes of the Earth system. Rivers are extremely sensitive to active tectonic deformation (Holbrook and Schumm, 1999). The planform patterns of the drainage network can also yield valuable insights into the tectonics underlying the landscape response (Duvall et al., 2020).
The establishment of the evolution processes of drainage networks is the essential precondition for understanding the response of drainage patterns to tectonic movement. The historical evolution of drainage networks can be reconstructed by landform records (Pan et al., 2011; Fan et al., 2018), provenance analyses (Clift et al., 2012; Ma et al., 2020; Zhao et al., 2021), historical records or modern observations (Chen et al., 2012; Shugar et al., 2017; Stokes et al., 2018). The evolutionary trends of the drainage network can be assessed by topographic metrics (Willett et al., 2014; Forte and Whipple, 2018; Lin et al., 2021). Moreover, the evolution of drainage patterns can also be simulated by numerical landscape evolution models or experimental models (Tucker and Slingerland, 1996; Viaplana-Muzas et al., 2019). However, it is difficult to obtain a full process by using a method alone.
As one of the most active tectonic areas, in active fold-thrust belts the drainage pattern is mainly controlled by tectonics. The evolution of drainage patterns in active fold-thrust belts has recently become a topic of considerable interest and some debate. Previous studies have shown that a drainage network may evolve from an early stage characterized by transverse dominant channels perpendicular to the main structures to longitudinal dominant channels parallel to the main structures in response to folding or thrusting (Gupta, 1997; Champel et al., 2002; Ramsey et al., 2008; Keller and DeVecchio, 2013; Cao et al., 2020). However, other studies suggested that a drainage network may evolve from longitudinal to transverse dominated during mountain building (Babault et al., 2012; Viaplana-Muzas et al., 2019). This controversy is mainly caused by the difficulty in understanding the entire drainage network evolution process. Thus, it is necessary to obtain the full process of drainage evolution for a typical active fold-thrust belt using multiple methods.
The Qilian Mountains are a typical active fold-thrust belt located in the Northeastern Tibetan Plateau. The drainage network is dominated by rivers flowing parallel to the mountain chain in the interior of the Qilian Mountains. In contrast, on the North flank of the Qilian Mountains, transverse rivers dominate the drainage network. Moreover, research on drainage evolution in the Qilian Mountains has achieved many results. Ma et al. (2020) reconstructed the history of the drainage evolution of the Datong River using integrated provenance methods. The evolution of the Heihe River has been derived from sedimentary and chronological results (Hetzel et al., 2004; Pan et al., 2016). However, all these studies focused on the formation of single rivers, and the evolution of drainage patterns in the Qilian Mountains is still unclear.
The main goal of this study is to discuss the evolution of drainage patterns in active fold-thrust belts, and we focus on the Qilian Mountains as a case study. We reconstruct the evolutionary history and calculate the evolutionary trends of major drainages in the Qilian Mountains by summarizing morphometric and geological evidence. Then, we propose an evolution model of drainage patterns in active fold-thrust belts that is validated by using a numerical landscape evolution model.
2 Geomorphic and geological setting
The Qilian Mountains, a large fold-thrust belt ∼1,000 km long and 300 km wide, are situated in the Northeastern Tibetan Plateau (Figure 1). The Qilian Mountains is an early Paleozoic orogeny reactivated in the Cenozoic resulting from the India-Asia collision (Zuza et al., 2018; Cheng et al., 2021). The Cenozoic tectonics of the Qilian Mountains are characterized by folds, thrust faults and crustal thickening and shortening (Tapponnier et al., 2001; Zheng et al., 2017). The Qilian Mountains initiated there in the Eocene immediately in response to the Indian-Eurasian plate convergence (Yin et al., 2008; Wu et al., 2021). However, significant exhumation tracked via low-temperature thermochronology, indicates that the Qilian Mountains experienced accelerated uplift in the Miocene (Duvall et al., 2013; Zheng et al., 2017; Yu et al., 2019; Wang et al., 2020; Wu et al., 2021). In the late Miocene, the uplift and deformation of the Qilian Mountains expend to the Hexi Corridor Basin resulted from outward intracontinental growth of the Tibetan Plateau (Zheng et al., 2017; Wang et al., 2020).
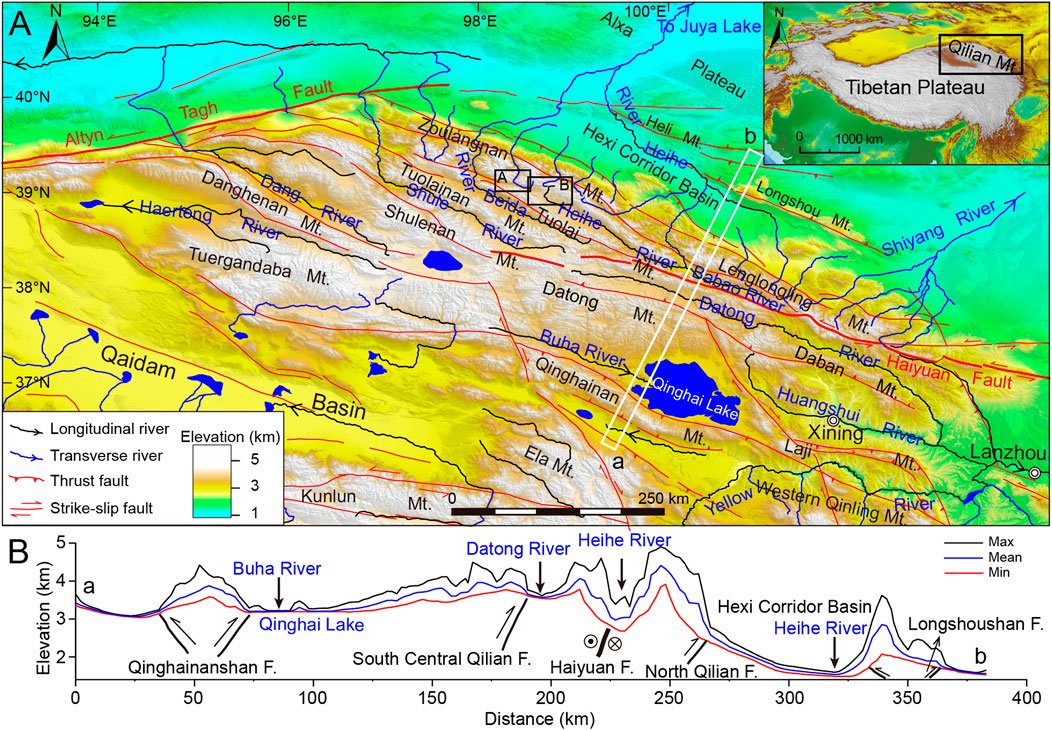
FIGURE 1. (A): Geomorphological and geological characteristics of the Qilian Mountains. The black box in the inset shows the location of the Qilian Mountains in Figure 1A. The A and B black boxes show the locations indicated in Figures 6A,C, respectively. The white box shows the location of the topographic swath profile shown in (B). Locations of major faults are from Deng et al., 2003. (B): Maximum, mean and minimum elevations along a 20 km wide swath window. The swath shows the major topographical and geological features across the Qilian Mountains.
The Qilian Mountains consist of a series of WNW- or NW-trending ranges (e.g., the Zoulangnan Mountain, Tuolai Mountain, Qinghainan Mountain), which are separated by subparallel elongated intermountain basins. Ranges reach elevations of 4–5 km and intermountain basins are 3–4 km in elevation. A series of longitudinal rivers flow in these intermountain basins. Rivers on the Northern flank of the Qilian Mountains drain to the Hexi Corridor Basin (e.g., the Shiyang River, Heihe River, Beida River); along the Southern flank rivers mainly flow into the Qaidam Basin and Qinghai Lake (e.g., the Haerteng River, Buha River). In addition, some river flows out the mountain from the East and West ends (e.g., the Datong River, Huangshui River, Danghe River). The interior of the Qilian Mountains is dominated by longitudinal rivers, and the outer part around the mountains, especially the Northern flank, is dominated by transverse rivers in the present day (Figure 1). Dating studies of river evolution constrain the initiation of major modern river formation to the early Pleistocene in the Qilian Mountains, e.g., the Datong River, Huangshui River, and Heihe River (Zeng et al., 1995; Pan et al., 2016; Ma et al., 2020).
The Datong River basin is one of the most elongated river basins in the world. The total length of the Datong River is ∼560 km, and the drainage basin is only ∼20 km wide. The Datong River originates in the middle segment of the Qilian Mountains and runs in a SE direction through the Eastern Qilian Mountains to the Huangshui River. It is a longitudinal river bounded by Datong Mountain and Daban Mountain to the South and Tuolai Mountain and Lenglongling Mountain to the North (Figure 1).
The Heihe River is the second largest inland river in China. The Heihe River originates from the Qilian Mountains and converges at Juyan Lake, with a total length of ∼810 km. The drainage basin can be divided into three major geomorphological units: the Southern Qilian Mountains, the middle Hexi Corridor Basin and the Northern Alxa Plateau (Li et al., 2001). The upper section of the Heihe River basin is dominated by two longitudinal rivers, the Heihe River and Babaohe River, which are bounded by Tuolai Mountain to the South and Zoulangnan Mountain and Lenglongling Mountain to the North (Figure 1). After the confluence of the Heihe and Babao Rivers, the Heihe River cuts through Zoulangnan Mountain and flows into the Hexi Corridor Basin. In the Hexi Corridor Basin, the Heihe River flows to the Northwest and receives several transverse rivers. Then, the Heihe River cuts through Heli Mountain and finally converges at Juyan Lake.
3 Drainage divide stability assessment method
Recently, Willett et al. (2014) proposed χ-maps as a proxy for determining divide stability and forecasting the direction of divide migration (Willett et al., 2014; Forte and Whipple, 2018). The evolution of a river profile can be described by a stream power model (Willett et al., 2014):
where z is the elevation of a point in a river channel; x is the upstream distance along the channel; t is time; U is the rock uplift; K is an erodibility coefficient; A is drainage area; and m and n are empirical constants.
For the simple case where U and K are constant in space and time, the steady-state solution of Eq. 1 is (Perron and Royden, 2013; Willett et al., 2014):
with
where xb is the downstream end of the analyzed profile, x’ is a dummy variable, and A0 is the referenced drainage area.
When uplift, rock erodibility, climate, and catchment outlet elevation are uniform across both river networks on either side of the divide, the lower value of χ implies a higher erosion rate and indicates that the divide will move to the other side. We calculated χ-maps by using DivideTools (Forte and Whipple, 2018), which was built using TopoToolbox (Schwanghart and Kuhn, 2010). The digital elevation model (DEM) dataset used is the SRTM-DEM, with a spatial resolution of ∼90 m, which is produced by the Shuttle Radar Topography Mission and downloaded from the Chinese Academy of Sciences (http://www.gscloud.cn). The χ-maps were calculated with uniform U and K with a concavity θ of 0.5 and a referenced drainage area A0 of 1 m2. We used a constant outlet elevation of 1700 m to calculate χ for the Datong River and its adjacent drainage basins except the Qinghai Lake watershed. Because the Qinghai Lake area is an inland river basin with a higher local base level, we specifically used an outlet elevation of 3,400 m to calculate χ for the Qinghai Lake watershed and upper Datong River. For the analysis of the Heihe River, the outlet elevation selected was 2000 m.
Moreover, other metrics, such as the mean local relief and mean gradient, are also useful to assess the current status of the drainage network. The resulting across-divide erosion rate contrast would force the divide to move toward the side with lower slopes and erosion rates (Forte and Whipple, 2018). To assess the divide stability for different segments, we divided the Datong River drainage into five sections based on different adjacent drainage basins. To provide an easy visual assessment of divide stability for individual divide segments, we normalized the difference in the mean value of χ (Δχ), local relief and gradient of all streams on either side within 5 km from the divide.
4 Drainage evolution in the Qilian Mountains
4.1 Datong River
Our previous research has shown that the drainage network of the Datong River evolved from an early stage characterized by transverse dominance to longitudinal dominance in the past 1.1 Ma using integrated provenance methods and river terrace dating (Ma et al., 2020). The formation of the Datong River started at ∼1.1 Ma, and the drainage basin was limited to the area of the lower modern Datong River in the initial period. Since then, the river channelized into the Menyuan Basin between ∼0.62 Ma and ∼0.42 Ma, and it captured the upper Datong River between ∼0.13 Ma and ∼0.06 Ma (Ma et al., 2020) (Figure 2). The drainage basin gradually extended through drainage reorganization, and the drainage pattern of the Datong River became increasingly elongated and dominated by longitudinal.
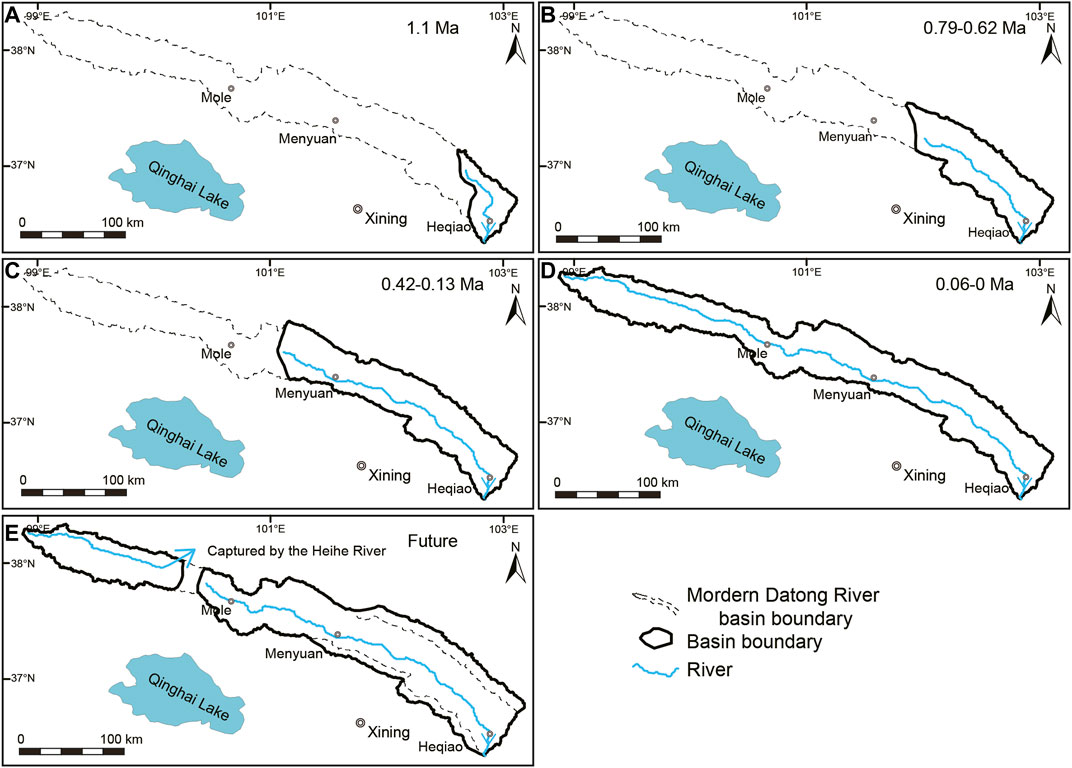
FIGURE 2. Evolution processes of the Datong River [modified from Ma et al. (2020)]. (A–B): The modern Datong River began to form at ∼1.1 Ma, the drainage basin was limited to the area of the lower modern Datong River at the beginning, and since then, it has gradually extended with headward erosion. (C): The Datong River channelized into the Menyuan Basin between ∼0.62 Ma and ∼0.42 Ma (D): The Datong River captured the upper Datong River between ∼0.13 Ma and ∼0.06 Ma (E): In the future, the upper Datong River might be captured by the Heihe River and the basin of lower Datong River will broaden.
Moreover, we can calculate the evolutionary trends of the drainage network of the Datong River using topographic metrics. The χ-map of the Datong River and its adjacent basins shows that each segment of the divide has varied states. Most notably, there are large differences in χ across the divides of the upper Datong River and Heihe River, indicating that the divide is unstable and moving toward the Datong River catchment (Figure 3A, Figure 4A). Moreover, the maximum, mean, and minimum elevations along the swath of Tuolai Mountain show pronounced ridge asymmetry and southward migration of divides (Figure 4B). The Northern slope of the divide is very steep with local relief of more than 1,000 m, whereas the slope on the Southern side is gentler with local relief of only 100 m. Maximum elevations clearly show that the range crest (highest topographic point) of Tuolai Mountain is located on the North flank. In contrast, minimum elevations show that the main divide of the Datong River and Heihe River is located on the South flank of Tuolai Mountain. This finding indicates that the main divide has shifted southward ∼10 km. The topographic profiles clearly show that the local base level of the North-facing slope of Tuolai Mountain is significantly lower than that of the South-facing slope, which indicates that the Northern side has greater erosion potential. These findings also suggest that the divide will continue to migrate Southward, indicating that the upper Datong River might be captured by the Heihe River in the future.
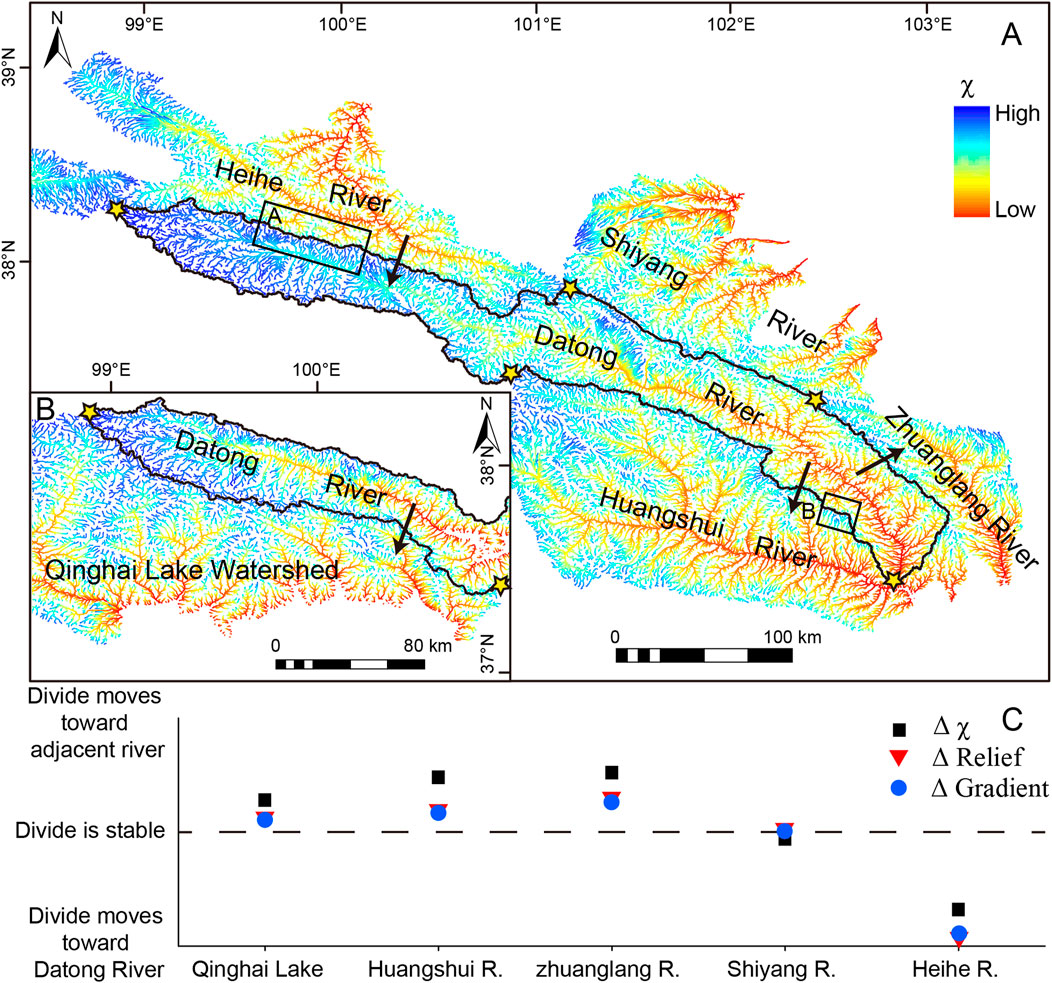
FIGURE 3. (A–B): χ-maps of the Datong River basin and adjacent drainage basins. The black line indicates the divide, and the yellow stars are the boundaries between the divide segments. Black arrows indicate the predicted divide migration directions. (C): Standardized delta plot for the five segments along the divide of the Datong River.
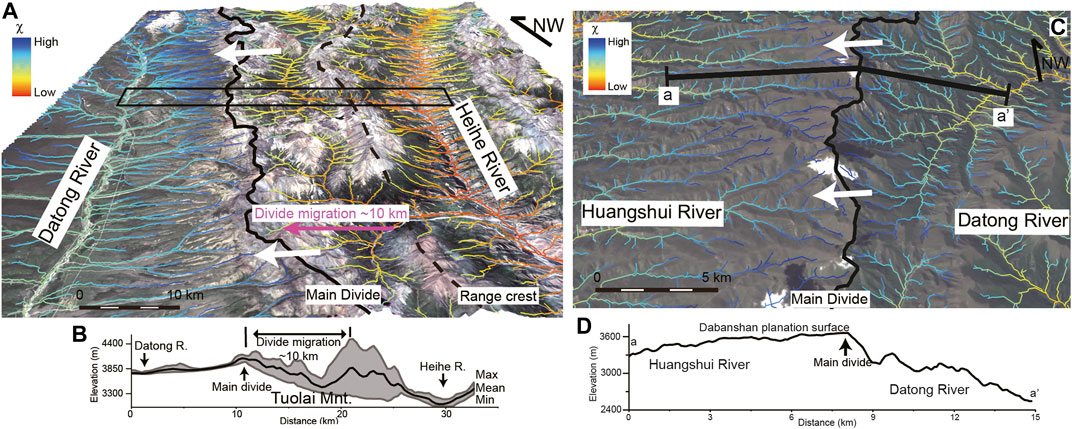
FIGURE 4. (A): χ-map of the upper Datong River and Heihe River. The black line indicates the main divide, and the black dotted line indicates the range crest. The black box shows the position of the swath topographic profile. White arrows indicate the predicted divide migration direction. (B): Swath topographic profile with 3 km width across Tuolai Mountain. Vertical arrows mark the Datong River, Heihe River and the main divide; each profile shows the maximum, mean, and minimum elevations, respectively, and the shaded area marks the local relief. (C): χ-map of the lower Datong River and Huangshui River. The black line indicates the main divide. Line a–a’ shows the position of the topographic profile. White arrows indicate the predicted divide migration direction. (D): Topographic profile across Daban Mountain. Vertical arrows mark the main divide.
In contrast, the Δχ between the Datong River and Qinghai Lake, Huangshui River, and Zhuanglang River suggest that the divide will move toward adjacent drainage basins from the Datong River catchment (Figure 3A,B, Figure 4C). The topographic profile across the divide of the lower Datong River and Huangshui River shows a pronounced ridge asymmetry and Southward migration of the divides (Figure 4D). The Dabanshan planation surface is on the south side of the divide and is a high-elevation, low-relief region; the opposite side is characterized by deep gorges. These geomorphological features also suggest that the divide will continue to migrate southward. The Δχ between the middle Datong River and Shiyang River is fairly low, indicating that the divide is relatively stable (Figure 3C). The results show that the mean local relief and gradient are in good agreement with Δχ (Figure 3C). Overall, the modern elongated Datong River will be disintegrated. The upper Datong River will be progressively replaced by transverse-dominated rivers, and the lower Datong River will be broadened in the future.
4.2 Heihe River
Similar to the Datong River, the evolution process of the middle Heihe River is also characterized by the development of a longitudinal river from transverse rivers. Before 1.1 Ma, the Heihe River cut across Longshou Mountain and flowed Northward (Tan et al., 1998) (Figure 5). Due to the uplift of Longshou Mountain, the Heihe River did not have enough power to maintain the outlet and then left its bed and diverted toward Jiudong Basin after 1.1 Ma (Tan et al., 1998; Pan et al., 2016). Two wind gaps were identified on Heli Mountain, and 21Ne and 10Be exposure dating suggested that the Heihe River left its bed in the eastern and central Heli Mountain at 0.44 Ma and 0.22 Ma, respectively (Hetzel et al., 2004) (Figure 5). In addition, the Heihe River arrived at the current outlet in western Heli Mountain after 0.2 Ma (Hetzel et al., 2004). This result indicated that the Heihe River flowed through central Heli Mountain before 0.44 Ma, and the outlets gradually migrated to the West during the Westward propagation of Heli Mountain. The history of the drainage reorganization also showed that the Heihe River gradually became a longitudinal river.
However, the modern upper Heihe River displays a different characteristic from the past 1.1 Ma. The χ-map of the upper Heihe River shows higher values than its surrounding areas, indicating a migrating trend toward the Heihe River side (Figure 6A). Actually, some transverse rivers have captured the upper reach of longitudinal rivers, such as the Hongshuiba River. The Hongshuiba River has cut through Zoulangnan Mountain, incised into the intermontane basin bounded by Zoulangnan Mountain and Tuolai Mountain and captured the upstream portion of the Zhulongguan River (Figure 6C). The Hongshuiba River has a large bend, where the river abruptly changes directions by ∼100°. In plan view its flow direction is more in line with the Zhulongguan River that flows to the West. This network geometry suggests that the upper Hongshuiba River may have once drained to the Zhulongguan River.
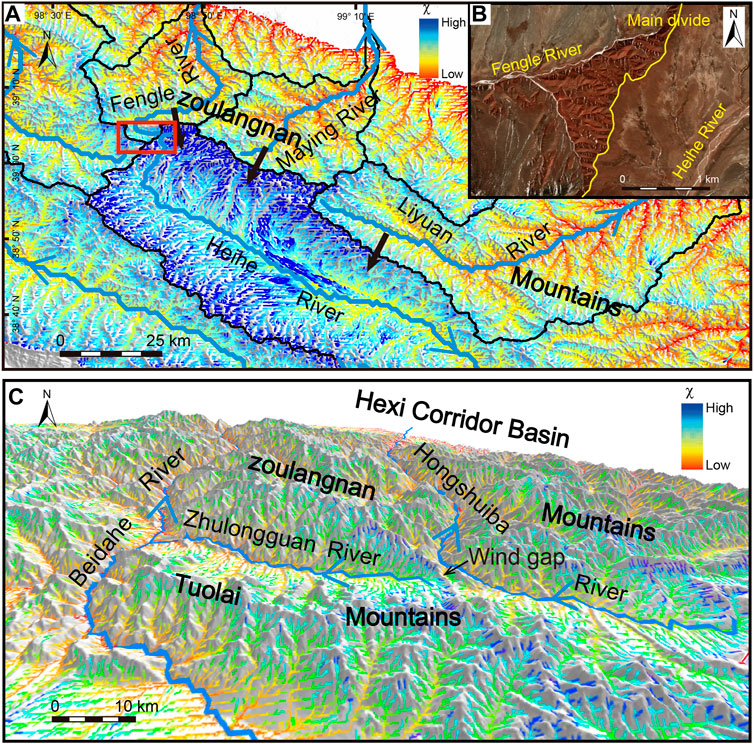
FIGURE 6. (A): χ-map of the upper Heihe River and its surrounding areas, the red box shows the location in Figure 6B. Black arrows indicate the predicted divide migration directions. (B): Google Earth image of the Fengle River and Heihe River. (C): Geomorphic features and χ-map of the Zhulongguan River and Hongshuiba River.
Some transverse rivers have incised into intermontane basins and are going to capture the upper reach of longitudinal rivers, such as the Fengle River. The Fengle River has cut through Zoulangnan Mountain and incised into the intermontane basin. The lower χ value on the Fengle River side indicates that the divide is migrating to the Heihe River side (Figure 6A). Moreover, remote sense images show that the sides of the divide have distinctly different erosion statuses. On the Fengle River side, it is characterized by gullies; conversely, it is characterized by a smooth surface on the Heihe River side (Figure 6B). Stronger erosion occurred on the Fengle River side, and the divide migrated to the Heihe River side. Although some rivers have not yet incised into intermontane basins, the χ-map shows that the divide has a trend of migrating to the intermontane basin side, such as the Maying River and Liyuan River (Figure 6A).
In summary, the middle Heihe River gradually became a longitudinal river in the past 1.1 Ma, and the upper Heihe River will be captured by transverse rivers in the future.
5 Evolution of drainage patterns in active fold-thrust belts
5.1 Early stage -Transverse to longitudinal drainage network evolution
As the two greatest rivers in the Qilian Mountains, the Datong River and middle Heihe River show a coincident evolutionary history, indicating that early transverse dominated river networks are progressively replaced by longitudinal dominated rivers during mountain building.
River response to a growing structure is dependent upon the rate of fluvial erosion versus the rate of uplift (Tucker and Slingerland, 1996; Gupta, 1997). Before the rapid uplift of the mountain, transverse rivers could cut through the structure (Figure 7A). If the mountain grows with a relatively large uplift rate, smaller transverse rivers would be defeated and diverted toward the fold tips. Then, it would gather other laterally adjacent transverse rivers, increasing stream power, and flow out of the mountain in areas of structural weakness (Figure 7B).
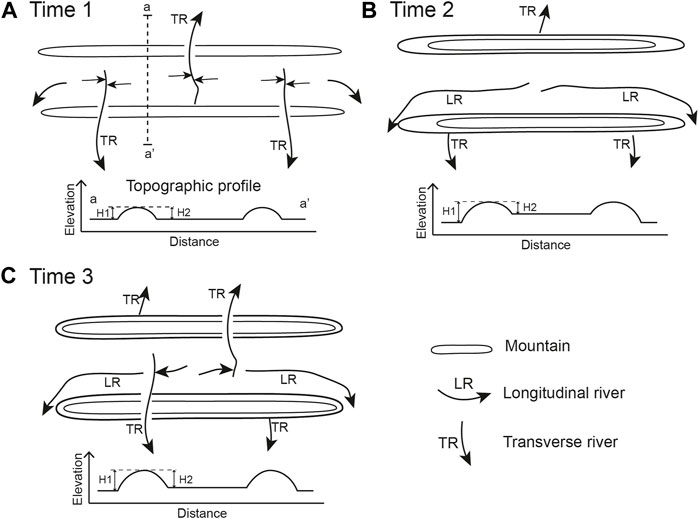
FIGURE 7. Idealized diagram of the model of drainage pattern evolution in active fold-thrust belts. Topographic profiles (a–a’) show the elevation across the fold-thrust belt. (A): At time 1, transverse rivers could cut through the structure. (B): At time 2, transverse rivers would be defeated and diverted toward the fold tips. (C): At time 3, transverse rivers could capture the upper longitudinal rivers.
The process can be supported by other areas in the Qilian Mountains. Provenance analysis showed that erosion materials from the Central and Northern Qilian Mountains can be transported to the Northern Qaidam Basin before the Oligocene (Bush et al., 2016), indicating that this area was dominated by transverse rivers that can cross the Central and Southern Qilian Mountains. Moreover, transverse rivers rise in the Ela Mountains across the region that is currently occupied by Qinghainan Mountain and into the Qinghai Lake Basin before 10 Ma (Zhang et al., 2012). Similarly, transverse rivers rise in the Western Qinling Mountains across the region that is currently occupied by Laji Mountain and into Xining Basin before 20 Ma (Liu et al., 2007). Moreover, integrated field structural and sedimentary evidence suggests that the original N-S direction rivers in the Qilian Mountains changed to W-E flowing rivers since ∼13–8 Ma (Meng et al., 2020). In other words, the area of the Qilian Mountains may have been dominated by transverse rivers before rapid uplift. With the rapid uplift of the Qilian Mountains, transverse rivers with insufficient power would have been defeated and diverted toward the fold tips. The drainage patterns of the Qilian Mountains evolved toward longitudinal rivers, and ultimately formed the current pattern.
Moreover, this evolution model of drainage patterns has been widely detected in other active fold-thrust belts around the world. In the Zagros Mountains, studies have found that as the folds grow in length, the smaller transverse rivers are diverted toward the fold tips to become longitudinal rivers (Ramsey et al., 2008; Walker et al., 2011). In the younger Sub-Himalayan deformation zone, transverse rivers have been dammed by uplift ranges and forced to flow axially in synclinal valleys subparallel to the fold axes (Gupta, 1997; Malik and Mohanty, 2007). With the uplift and lateral propagation of the Wheeler Ridge, drainage deflected parallel to the fold axes, and some wind or water gaps were formed (Keller et al., 1999). In addition, this evolution model of the drainage pattern was validated by a numerical landscape evolution model (Tucker and Slingerland, 1996; Tomkin and Braun, 1999; Collignon et al., 2016).
It is important to note that this evolution model was mainly observed in fold-thrust belts that were in the early stage of development.
5.2 Late stage—Longitudinal to transverse drainage network evolution
In the uplifted fold-thrust belts, the drainage pattern shows a different situation. In the Northern Qilian Mountains, the transverse Hongshuiba River has captured the upper reach of the longitudinal Zhulongguan River. In the upstream reaches of the Heihe River, capture is still ongoing. Some transverse rivers (e.g., Fengle River, Maying River, Liyuan River) are extending their upstream by headward erosion; in fact, the Fengle River has cut across the Zoulangnan Mountain and is going to capture the upper Heihe River. A similar situation is observed in the Datong River catchment, where the divide between the upper Datong River and Heihe River is moving to the South, and the upper longitudinal Datong River will be captured by transverse tributaries of the Heihe River in the future.
Supposing that the rate of uplift of the Qilian Mountains remains unchanged, with uplift, the height difference between the Qilian Mountains and Hexi Corridor Basin would continuously increase. The great height difference (>2000–3,000 m), which was produced by the vast difference in uplift rates between the Qilian Mountains and Hexi Corridor Basin, lowered the base level of erosion and increased the fluvial incision rate of transverse rivers developed on the north flank of the Qilian Mountains. In contrast, the difference in uplift rates between the mountain and intermontane basin is smaller than that between the Qilian Mountains and Hexi Corridor Basin. Therefore, the difference in heights and erosion rates between the longitudinal rivers and the transverse rivers would continuously increase (Figure 7B). Actually, the erosion rates measured by cosmogenic nuclides have shown that the average erosion rate of the Northern Qilian Mountains is several times higher than that of the upper Heihe River (Hetzel, 2013; Hu et al., 2015, 2021). The differential erosion rates between the longitudinal rivers in the intermontane basins and the transverse rivers in the margins made the latter capture longitudinal reaches. Then the drainage pattern controlled by longitudinal rivers would transform into transverse rivers (Figure 7C).
The same phenomenon can be found on the Southeastern Tibetan Plateau. The Anning River, a tributary of the Yangtze River, flows Southward parallel to the main structures located in the Southeastern Tibetan Plateau. The upper course of the paleo-Anning River was captured by the paleo-Dadu River at ∼2 Ma (Yang, et al., 2020). Many studies suggest that the upper Yangtze River once drained south into either the Mekong River or the Red River along the structural valley, and it was later captured by the middle Yangtze River (Clark et al., 2004; Zheng et al., 2013; Chen et al., 2017). This is a common phenomenon where a longer, orogen parallel river is captured by an aggressive steep transverse river in the marginal parts of the Tibetan Plateau. The transformation of the drainage pattern is also due to the uplift of the Tibetan Plateau.
In addition to the Tibetan Plateau, a similar evolution of drainage patterns can be found in other high fold-thrust belts. In the High Atlas of Morocco, which is a still-active linear intracontinental mountain, longitudinal reaches are widely captured by transverse rivers, indicating that the drainage network systematically evolves from longitudinal-dominated to transverse-dominated (Babault et al., 2012). This behavior is similar to the drainage network reorganization observed in other fold-thrust belts, such as the Central Range of the island of New Guinea, the Eastern Cordillera of Colombia and the Pyrenees (Babault et al., 2013; Babault et al., 2018).
It is important to note that this evolution model was mainly observed in fold-thrust belts that were in the late stage of development.
6 Numerical landscape evolution model
Numerical landscape evolution models have been widely used to help understand the processes of landscape evolution. To test the evolution model of drainage patterns in an active fold-thrust belt, we performed numerical landscape evolution modeling using the TopoToolbox Landscape Evolution Model (TTLEM) (Campforts et al., 2017). The TTLEM is a landscape evolution model based on the MATLAB function library TopoToolbox 2 (Schwanghart and Scherler, 2014).
The model occupies a rectangular domain of 100 km long and 60 km wide, with a spatial resolution of 150 m in our study. To simulate the uplift situation of the active fold-thrust belt, we use an uplift pattern subdividing the synthetic domain into three sectors (Figure 8A). The uplift rates of the mountains and intermountain basins were set as 1.75 mm/yr and 1 mm/yr, respectively. The uplift rate of the surrounding areas of the fold-thrust belt was set as 0.5 mm/yr. Moreover, mountains and intermountain basins are set as 85 km long and 10.5 km wide and 85 km long and 6.75 km wide, respectively. The total duration of simulations is 20 Myr with a time step of 0.05 Myr. Other simulation parameters follow defaults, including: erosion coefficient (K) = 3 × 10−6/yr; area exponent (m) = 0.5; slope exponent (n) = 1; hillslope diffusivity = 0.03 m2/year; and drainage area threshold = 0.2 km2.
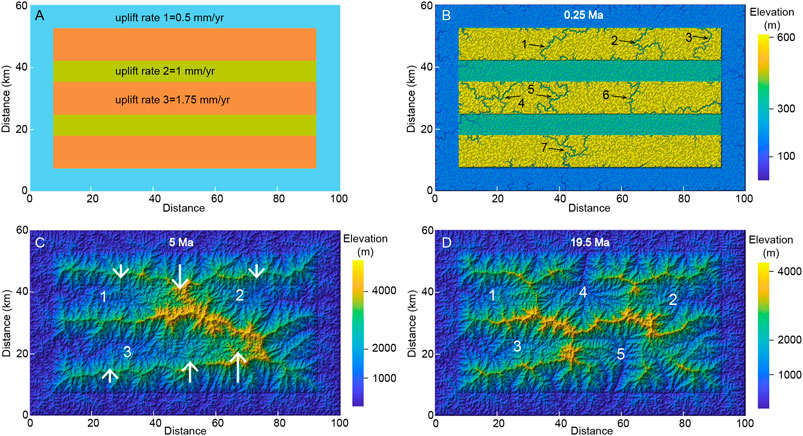
FIGURE 8. Setup of uplift patterns (A) and simulation results for drainage pattern evolution in the active fold-thrust belt (B–D). The numbers in Figure 7B mark the main rivers; the numbers in Figure 8 (C,D) mark the main drainage basins; and the white arrows show the direction and magnitude of divide migration in Figure 8C.
The synthetic landscapes resulting from the experiments are shown in Figures 8B–D. Figure 8B shows that many simulated rivers cut across the mountains in the initial period (e.g., rivers 1–7). However, the incision rates of these rivers are less than the uplift rate of mountains. Then, these transverse rivers are defeated. Meanwhile, the rivers that flow toward mountain tips are gradually lengthened (e.g., drainage basins 1–3 in Figure 7C). A drainage pattern characterized by longitudinal dominant rivers is gradually formed in the middle stage (Figure 7C). It is noteworthy that divides move toward the side of longitudinal drainages when the longitudinal-dominant rivers are formed. The magnitude of divide migration in the upstream basin is significantly greater than that in the other segments (Figure 7C). With the continuous migration of the divide, the catchment of transverse rivers adjacent to the upper reach of longitudinal rivers continues to expand. Finally, these transverse rivers capture the upper longitudinal rivers, and a drainage pattern characterized by longitudinal-dominant rivers is gradually formed (Figure 8D). In summary, the model experiments show that the pattern of the drainage network can evolve from an early stage characterized by transverse-dominant channels to longitudinal-dominant channels. Although the uplift rate is consistent during the simulations, this drainage pattern is unstable, and the upper reaches of longitudinal rivers are captured by transverse rivers. The evolution of the drainage patterns of the numerical simulation result is consistent with that in the Qilian Mountains.
7 Conclusion
In the light of the geological and sedimentary evidence, we reconstructed the evolutionary history of drainage system of the Datong River and the middle Heihe River. Results show that the Datong River and the middle Heihe River have evolved into longitudinal rivers in the past 1.1 Ma. Moreover, the analysis of topographic metrics indicates that the upper Datong River and the upper Heihe River will be captured by transverse rivers in the future. In contrast to previous studies, our study suggests that the evolution of drainage patterns in active fold-thrust belts has two stages: in the early stage, transverse rivers would be replaced by longitudinal rivers; in the later stage, the upper longitudinal rivers would be captured by transverse tributaries. Even if the tectonic setting is constant, the drainage patterns can still be changed in different evolutionary stages of an active fold-thrust belt.
Data availability statement
The original contributions presented in the study are included in the article/Supplementary Material, further inquiries can be directed to the corresponding author.
Author contributions
ZM and TP conceived and designed the experiments; DY, XL, ZF, and QW performed the experiments; ZM, DY, and TP wrote the manuscript with help from the other authors.
Funding
This work was supported by the Natural Science Foundation of Shandong Province, China (Grant No. ZR2021QD005) and the National Natural Science Foundation of China (Grant Nos. 42101156, 41971099).
Conflict of interest
The authors declare that the research was conducted in the absence of any commercial or financial relationships that could be construed as a potential conflict of interest.
Publisher’s note
All claims expressed in this article are solely those of the authors and do not necessarily represent those of their affiliated organizations, or those of the publisher, the editors and the reviewers. Any product that may be evaluated in this article, or claim that may be made by its manufacturer, is not guaranteed or endorsed by the publisher.
References
Babault, J., Teixell, A., Struth, L., Van Den Driessche, J., Arboleya, M. L., Arboleya, M. L., et al. (2013). Shortening, structural relief and drainage evolution in inverted rifts: Insights from the Atlas mountains, the eastern Cordillera of Colombia and the Pyrenees. Geol. Soc. Lond. Spec. Publ. 377 (1), 141–158. doi:10.1144/SP377.14
Babault, J., Van Den Driessche, J., and Teixell, A. (2012). Longitudinal to transverse drainage network evolution in the High Atlas (Morocco): The role of tectonics. Tectonics 31, C4020. doi:10.1029/2011TC003015
Babault, J., Viaplana-Muzas, M., Legrand, X., Van Den Driessche, J., González-Quijano, M., and Mudd, S. M. (2018). Source-to-sink constraints on tectonic and sedimentary evolution of the Western central range and cenderawasih bay (Indonesia). J. Asian Earth Sci. 156, 265–287. doi:10.1016/j.jseaes.2018.02.004
Bush, M. A., Saylor, J. E., Horton, B. K., and Nie, J. (2016). Growth of the Qaidam Basin during Cenozoic exhumation in the northern Tibetan Plateau: Inferences from depositional patterns and multiproxy detrital provenance signatures. Lithosphere 8 (1), 58–82. doi:10.1130/L449.1
Campforts, B., Schwanghart, W., and Govers, G. (2017). Accurate simulation of transient landscape evolution by eliminating numerical diffusion: The TTLEM 1.0 model. Earth Surf. Dynam. 5 (1), 47–66. doi:10.5194/esurf-5-47-2017
Cao, X. L., Geng, H. P., Pan, B. T., and Hu, X. F. (2020). Development of transverse drainages and formation of wind gaps on actively growing fold: Review and case study. Seism. Geo. 42 (3), 670–687. doi:10.3969j.issn.0253/4967.2020.03.009 (in Chinese).
Castelltort, S., Goren, L., Willett, S. D., Champagnac, J. D., Herman, F., and Braun, J. (2012). River drainage patterns in the New Zealand Alps primarily controlled by plate tectonic strain. Nat. Geosci. 5 (10), 744–748. doi:10.1038/NGEO1582
Champel, B., van der Beek, P., Mugnier, J. L., and Leturmy, P. (2002). Growth and lateral propagation of fault‐related folds in the Siwaliks of Western Nepal: Rates, mechanisms, and geomorphic signature. J. Geophys. Res. 107 (B6), 2111. doi:10.1029/2001JB000578
Chen, Y., Yan, M. D., Fang, X. M., Song, C. H., Zhang, W. L., Zan, J. B., et al. (2017). Detrital zircon U–Pb geochronological and sedimentological study of the simao basin, yunnan: Implications for the early cenozoic evolution of the Red River. Earth Planet. Sci. Lett. 476, 22–33. doi:10.1016/j.epsl.2017.07.025
Chen, Y. Z., Syvitski, J. P. M., Gao, S., Overeem, I., and Kettner, A. (2012). Socio-economic impacts on flooding: A 4000-year history of the yellow river, China. Ambio 41 (7), 682–698. doi:10.1007/s13280-012-0290-5
Cheng, F., Zuza, A. V., Haproff, P. J., Wu, C., Neudorf, C., Chang, H., et al. (2021). Accommodation of India–Asia convergence via strike-slip faulting and block rotation in the Qilian Shan fold–thrust belt, northern margin of the Tibetan Plateau. J. Geol. Soc. Lond. 178 (3), jgs2020–207. doi:10.1144/jgs2020-207
Clark, M. K., Schoenbohm, L. M., Royden, L. H., Burchfiel, B. C., Whipple, K. X., Burchfiel, B. C., et al. (2004). Surface uplift, tectonics, and erosion of eastern Tibet from large-scale drainage patterns. Tectonics 23 (1). doi:10.1029/2002TC001402
Clift, P. D., Carter, A., Giosan, L., Durcan, J., Duller, G., Macklin, M. G., et al. (2012). U-Pb zircon dating evidence for a Pleistocene sarasvati River and capture of the yamuna river. Geology 40 (3), 211–214. doi:10.1130/G32840.1
Collignon, M., Yamato, P., Castelltort, S., and Kaus, B. (2016). Modeling of wind gap formation and development of sedimentary basins during fold growth: Application to the Zagros fold belt, Iran. Earth Surf. Process. Landf. 41 (11), 1521–1535. doi:10.1002/esp.3921
Deng, Q. D., Zhang, P. Z., Ran, Y. K., Yang, X. P., Min, W., and Chen, L. C. (2003). Active tectonics and earthquake activities in China. Earth Sci. Front. 10, 66–73. (in Chinese).
Duvall, A. R., Clark, M. K., Kirby, E., Farley, K. A., Craddock, W. H., Li, C., et al. (2013). Low-temperature thermochronometry along the kunlun and haiyuan faults, NE Tibetan plateau: Evidence for kinematic change during late-stage orogenesis. Tectonics 32, 1190–1211. doi:10.1002/tect.20072
Duvall, A. R., Harbert, S. A., Upton, P., Tucker, G., Flowers, R., and Collett, C. (2020). River patterns reveal two stages of landscape evolution at an oblique convergent margin, Marlborough Fault System, New Zealand. Earth Surf. Dynam. 8 (1), 177–194. doi:10.5194/esurf-8-177-2020
Fan, N., Chu, Z., Jiang, L., Hassan, M., Lamb, M., and Liu, X. (2018). Abrupt drainage basin reorganization following a Pleistocene river capture. Nat. Commun. 9 (1), 3756–6. doi:10.1038/s41467-018-06238-6
Forte, A. M., and Whipple, K. X. (2018). Criteria and tools for determining drainage divide stability. Earth Planet. Sci. Lett. 493, 102–117. doi:10.1016/j.epsl.2018.04.026
Gemignani, L., Mittelbach, B., Simon, D., Rohrmann, A., Grund, M. U., Bernhardt, A., et al. (2022). Response of drainage pattern and basin evolution to tectonic and climatic changes along the dinarides-hellenides orogen. Front. Earth Sci. 10, 821707. doi:10.3389/feart.2022.821707
Gupta, S. (1997). Himalayan drainage patterns and the origin of fluvial megafans in the Ganges foreland basin. Geol. 25, 11–14. doi:10.1130/0091-7613(1997)025<0011:hdpato>2.3.co;2
Hetzel, R. (2013). Active faulting, mountain growth, and erosion at the margins of the Tibetan Plateau constrained by in situ-produced cosmogenic nuclides. Tectonophysics 582, 1–24. doi:10.1016/j.tecto.2012.10.027
Hetzel, R., Tao, M., Niedermann, S., Strecker, M. R., Ivy-Ochs, S., Kubik, P., et al. (2004). Implications of the fault scaling law for the growth of topography: Mountain ranges in the broken foreland of north-east Tibet. Terra Nova. 16 (3), 157–162. doi:10.1111/j.1365-3121.2004.00549.x
Holbrook, J., and Schumm, S. A. (1999). Geomorphic and sedimentary response of rivers to tectonic deformation: A brief review and critique of a tool for recognizing subtle epeirogenic deformation in modern and ancient settings. Tectonophysics 305, 287–306. doi:10.1016/S0040-1951(99)00011-6
Hu, K., Fang, X., Ferrier, K. L., Granger, D. E., Zhao, Z., and Ruetenik, G. (2021). Covariation of cross-divide differences in denudation rate and χ: Implications for drainage basin reorganization in the Qilian Shan, northeast Tibet. Earth Planet. Sci. Lett. 562, 116812. doi:10.1016/j.epsl.2021.116812
Hu, K., Fang, X., and Granger, D. E. (2015). Erosion rates of northern qilian Shan revealed by cosmogenic 10Be. Adv. Earth Sci. 30 (2), 268–275. (in Chinese). doi:10.11867/j.issn.1001-8166.2015.02.0268
Jolivet, M., Cheng, F., Zuza, A. V., Guo, Z. J., and Dauteuil, O. (2022). Large-scale topography of the north Tibetan ranges as a proxy for contrasted crustal-scale deformation modes. J. Geol. Soc. Lond. 179 (4), jgs2021–085. doi:10.1144/jgs2021-085
Keller, E. A., and DeVecchio, D. (2013). Tectonic geomorphology of active folding and development of transverse drainages. Treatise Geomorphol. 5, 129–147. Elsevier Inc. doi:10.1016/B978-0-12-374739-6.00088-9
Keller, E. A., Gurrola, L., and Tierney, T. E. (1999). Geomorphic criteria to determine direction of lateral propagation of reverse faulting and folding. Geol. 27 (6), 515–518. doi:10.1130/0091-7613(1999)027<0515:gctddo>2.3.co;2
Li, X., Lu, L., Cheng, G., and Xiao, H. (2001). Quantifying landscape structure of the Heihe River Basin, north-west China using FRAGSTATS. J. Arid. Environ. 48 (4), 521–535. doi:10.1006/jare.2000.0715
Lin, L., Li, X., and Ma, Z. (2021). Quantifying the geomorphology of the drainage basins along the greater khingan mountains in NE China. Front. Earth Sci. 9, 1228. doi:10.3389/feart.2021.796610
Liu, S., Zhang, G., and Heller, P. L. (2007). Cenozoic basin development and its indication of plateau growth in the Xunhua-Guide district. Sci. China Ser. D-Earth. Sci. 50 (2), 277–291. doi:10.1007/s11430-007-6012-3
Ma, Z., Feng, Z., Peng, T., Liu, S., Li, M., Guo, B., et al. (2020). Quaternary drainage evolution of the Datong River, qilian mountains, northeastern Tibetan plateau, China. Geomorphology 353, 107021. doi:10.1016/j.geomorph.2019.107021
Malik, J. N., and Mohanty, C. (2007). Active tectonic influence on the evolution of drainage and landscape: Geomorphic signatures from frontal and hinterland areas along the northwestern himalaya, India. J. Asian Earth Sci. 29 (5–6), 604–618. doi:10.1016/j.jseaes.2006.03.010
Meng, K., Wang, E., Chu, J. J., Su, Z., and Fan, C. (2020). Late Cenozoic river system reorganization and its origin within the Qilian Shan, NE Tibet. J. Struct. Geol. 138, 104128. doi:10.1016/j.jsg.2020.104128
Pan, B., Chen, D., Hu, X., Cao, X., Chen, J., and Mao, J. (2016). Drainage evolution of the Heihe River in Western Hexi Corridor, China, derived from sedimentary and magnetostratigraphic results. Quat. Sci. Rev. 150, 250–263. doi:10.1016/j.quascirev.2016.08.036
Pan, B., Hu, Z., Wang, J., Vandenberghe, J., and Hu, X. (2011). A magnetostratigraphic record of landscape development in the eastern ordos plateau, China: Transition from late miocene and early pliocene stacked sedimentation to late pliocene and quaternary uplift and incision by the yellow river. Geomorphology 125 (1), 225–238. doi:10.1016/j.geomorph.2010.09.019
Perron, J. T., and Royden, L. (2013). An integral approach to bedrock river profile analysis. Earth Surf. Process. Landf. 38, 570–576. doi:10.1002/esp.3302
Ramsey, L. A., Walker, R. T., and Jackson, J. (2008). Fold evolution and drainage development in the Zagros mountains of fars Province, SE Iran. Basin Res. 20 (1), 23–48. doi:10.1111/j.1365-2117.2007.00342.x
Schwanghart, W., and Kuhn, N. J. (2010). TopoToolbox: A set of matlab functions for topographic analysis. Environ. Model. Softw. 25, 770–781. doi:10.1016/j.envsoft.2009.12.002
Schwanghart, W., and Scherler, D. (2014). Short communication: TopoToolbox 2 – MATLAB-based software for topographic analysis and modeling in Earth surface sciences. Earth Surf. Dynam. 2 (1), 1–7. doi:10.5194/esurf-2-1-2014
Shugar, D. H., Clague, J. J., Best, J. L., Schoof, C., Willis, M., Copland, L., et al. (2017). River piracy and drainage basin reorganization led by climate-driven glacier retreat. Nat. Geosci. 10 (5), 370–375. doi:10.1038/ngeo2932
Souza, D. H., Parra, M., Del Rio, I. A., Sawakuchi, A. O., Pupim, F. N., Hernández-González, J. S., et al. (2022). Late Quaternary drainage rearrangement prevents the vegetation development in the La Tatacoa intermontane basin of the Colombian Andes. Front. Earth Sci. (Lausanne). 423. doi:10.3389/feart.2022.808718
Stokes, M. F., Goldberg, S. L., and Perron, J. T. (2018). Ongoing river capture in the Amazon. Geophys. Res. Lett. 45 (11), 5545–5552. doi:10.1029/2018GL078129
Tan, L. H., Yang, J. C., and Duan, F. J. (1998). Stages of cenozoic tectonic movement in Hexi corridor, gansu Province. Acta Sci. Natur. Univ. Pekin. 34 (4), 523–532. (in Chinese).
Tapponnier, P., Xu, Z., Roger, F., Meyer, B., Arnaud, N., Wittlinger, G., et al. (2001). Oblique stepwise rise and growth of the Tibet Plateau. Science 294, 1671–1677. doi:10.1126/science.105978
Tomkin, J. H., and Braun, J. (1999). Simple models of drainage reorganisation on a tectonically active ridge system. N. Z. J. Geol. Geophys. 42 (1), 1–10. doi:10.1080/00288306.1999.9514827
Tucker, G. E., and Slingerland, R. (1996). Predicting sediment flux from fold and thrust belts. Basin Res. 8 (3), 329–349. doi:10.1046/j.1365-2117.1996.00238.x
Viaplana-Muzas, M., Babault, J., Dominguez, S., Van Den Driessche, J., and Legrand, X. (2019). Modelling of drainage dynamics influence on sediment routing system in a fold-and-thrust belt. Basin Res. 31 (2), 290–310. doi:10.1111/bre.12321
Walker, R. T., Ramsey, L. A., and Jackson, J. (2011). Geomorphic evidence for ancestral drainage patterns in the Zagros Simple Folded Zone and growth of the Iranian plateau. Geol. Mag. 148 (5–6), 901–910. doi:10.1017/S0016756811000185
Wang, W. T., Zheng, D. W., Li, C. P., Wang, Y., Zhang, Z. Q., Pang, J. Z., et al. (2020). Cenozoic exhumation of the qilian Shan in the northeastern Tibetan plateau: Evidence from low-temperature thermochronology. Tectonics 39, e2019TC005705. doi:10.1029/2019TC005705
Willett, S. D., McCoy, S. W., Perron, J. T., Goren, L., and Chen, C. Y. (2014). Dynamic reorganization of river basins. Science 343, 1248765. doi:10.1126/science.1248765
Wu, C., Zuza, A. V., Li, J., Haproff, P. J., Yin, A., Chen, X. H., et al. (2021). Late Mesozoic–Cenozoic cooling history of the northeastern Tibetan Plateau and its foreland derived from low-temperature thermochronology. Geol. Soc. Am. Bull. 133 (11–12), 2393–2417. doi:10.1130/B35879.1
Yang, R., Suhail, H. A., Gourbet, L., Willett, S., Fellin, M., Lin, X., et al. (2020). Early Pleistocene drainage pattern changes in Eastern Tibet: Constraints from provenance analysis, thermochronometry, and numerical modeling. Earth Planet. Sci. Lett. 531, 115955. doi:10.1016/j.epsl.2019.115955
Yin, A., Dang, Y. Q., Wang, L. C., Jiang, W. M., Zhou, S. P., Chen, X. H., et al. (2008). Cenozoic tectonic evolution of Qaidam basin and its surrounding regions (Part 1): The southern Qilian Shan-Nan Shan thrust belt and northern Qaidam basin. Geol. Soc. Am. Bull. 120 (7–8), 813–846. doi:10.1130/B26180.1
Yu, J., Pang, J., Wang, Y., Zheng, D., Liu, C., Wang, W., et al. (2019). Mid-Miocene uplift of the northern Qilian Shan as a result of the northward growth of the northern Tibetan Plateau. Geosph. (Boulder). 15 (2), 423–432. doi:10.1130/GES01520.1
Zeng, Y. N., Ma, H. Z., Li, Z., and Li, L. Q. (1995). A study on terrace formation and development of the Huangshui River in Xining Area. Sci. Geogr. Sin. 15, 253–258. (in Chinese).
Zhang, H. P., Zhang, P. Z., Zheng, D. W., Zheng, W. J., Cheng, Z. W., and Wang, W. T. (2012). Tectonic geomorphology of the qilian Shan: Insights into late cenozoic landscape evolution and deformation in the northeastern Tibetan plateau. Quat. Sci. 32 (5), 907–920. (in Chinese). doi:10.3969/j.issn.1001-7410.2012.05.08
Zhao, X., Zhang, H., Hetzel, R., Kirby, E., Duvall, A., Whipple, K., et al. (2021). Existence of a continental-scale river system in eastern Tibet during the late Cretaceous–early Palaeogene. Nat. Commun. 12 (1), 7231–7310. doi:10.1038/s41467-021-27587-9
Zheng, D., Wang, W., Wan, J., Yuan, D., Liu, C., Zheng, W., et al. (2017). Progressive northward growth of the northern qilian Shan-Hexi corridor (northeastern tibet) during the cenozoic. Lithosphere 9, 408–416. doi:10.1130/L587.1
Zheng, H., Clift, P. D., Wang, P., Tada, R., Jia, J., He, M., et al. (2013). Pre-miocene birth of the Yangtze River. Proc. Natl. Acad. Sci. U. S. A. 110 (19), 7556–7561. doi:10.1073/pnas.1216241110
Keywords: drainage pattern, divide migration, fold-thrust belt, landscape evolution model, qilian mountains
Citation: Ma Z, Yang D, Li X, Feng Z, Wang Q and Peng T (2022) Evolution of drainage patterns in active fold-thrust belts: A case study in the Qilian Mountains. Front. Earth Sci. 10:980928. doi: 10.3389/feart.2022.980928
Received: 29 June 2022; Accepted: 29 July 2022;
Published: 16 August 2022.
Edited by:
Hema Achyuthan, Anna University, Chennai, IndiaReviewed by:
János Kovács, University of Pécs, HungaryChen Wu, Institute of Tibetan Plateau Research (CAS), China
Copyright © 2022 Ma, Yang, Li, Feng, Wang and Peng. This is an open-access article distributed under the terms of the Creative Commons Attribution License (CC BY). The use, distribution or reproduction in other forums is permitted, provided the original author(s) and the copyright owner(s) are credited and that the original publication in this journal is cited, in accordance with accepted academic practice. No use, distribution or reproduction is permitted which does not comply with these terms.
*Correspondence: Zhenhua Ma, Z2VvbWF6aGhAMTYzLmNvbQ==