- 1Research Institute of Exploration and Development, PetroChina Southwest Oil and Gas Field Company, Chengdu, China
- 2The Key Laboratory of Natural Gas, Southwest Petroleum University, Chengdu, China
- 3Key Laboratory of Deep Oil and Gas, China University of Petroleum (East China), Qingdao, China
Exploration of natural gases in the Middle Permian Qixia Formation has achieved a breakthrough in the central and western Sichuan Basin since 2010. This study discusses the quality of dolomite reservoirs and source rocks, trap types and hydrocarbon charging history and proposes that good accumulation conditions are the basis for the large-scale enrichment of natural gas in the Qixia Formation. The high-quality reservoirs in the Qixia Formation are dolomites that primarily occur along platform margins and in the inner-platform shoals surrounding the Caledonian palaeo-uplifts. The Qixia gases originated mainly from the Cambrian and Permian mudstone source rocks. The overlaying Permian source rocks and the underlying Cambrian source rocks are both widely distributed and could provide sufficient gases for dolomite reservoirs. Additionally, the structural or structural-lithologic traps formed by an overlap of the Qixia dolomites and structurally high are favourable for oil and gas accumulation. The Qixia Formation had experienced four episodes of oil and gas charging from the middle-late Triassic to the Early Cretaceous, and traps located in structural-high zones are most favourable for hydrocarbon accumulation. Thus, the Qixia Formation has excellent accumulation conditions for the formation of large-scale gas fields. The concealed structural belts in the footwall of fault No. 1, the Longmenshan–Daxingchang structural belts and the periphery Gaomo–Shehong palaeo-uplift are the key zones for large-scale gas exploration in the Qixia Formation. The Qixia Formation is an important strategic replacement field for natural gas exploration in the Sichuan Basin following the Ediacaran Dengying Formation and the Cambrian Longwangmiao Formation.
Introduction
In the recent 50 years, the Middle Permian strata in the Sichuan Basin have been serving as important intervals for natural gas exploration. In the early exploration stage, the fractured limestone reservoirs of the Maokou Formation in the southern Sichuan Basin were the main exploration targets (Dai and Ou, 1990; Hu et al., 2012). Well K2 was drilled on the Kuangshanliang structure to explore the porous dolomitic reservoirs of the Qixia Formation in 2003. However, well testing showed water in these reservoirs and not further exploration was attempted. In 2014, the ST1 wildcat well was drilled by PetroChina in the Shuangyushi structure and it successfully gained high-yield industrial gas flow from platform-margin shoal dolomite reservoirs of the Qixia Formation. This ST1 gas well is very significant not only for discovering the Shuangyu gas field but also revealing new exploration targets of the Permian strata in the Sichuan Basin. Recently, many high-gas-production industrial gas wells have been drilled and up to one million square metres of daily gas production has been achieved in the Qixia dolomite reservoirs in the central, northwestern and southwestern Sichuan Basin. Meanwhile, several structural and structural-lithologic gas traps have also been successively discovered, showing the great potential for natural gas exploration in the Qixia Formation in the Sichuan Basin.
The reservoir quality of carbonate rocks has become a risk factor for gas exploration in deep burial settings (Wang et al., 2015; Wang, 2022). The carbonate gas reservoirs of the Permian Qixia Formation are characterised by average burial depths of more than 4500 m, with maximum burial depths in the western Sichuan Basin reaching about 8000 m. Studies regarding such ultra-deep carbonate gas reservoirs are rarely reported, particularly in the Longmenshan fold-thrust structural belt, where the carbonate reservoirs have strong heterogeneity and the thermal evolution degree of the source rock is high. The Longmenshan fold-thrust structural belt has a complex structural evolution history and experienced at least three major tectonic movements after the Middle Triassic occurring during the Indosinian, Yanshan and Himalayan orogenies. Consequently, the conditions for large-scale enrichment of natural gas still need to be further studied. The object of interest is the Middle Permian Qixia Formation in the Sichuan Basin. Based on drilling, testing and petrographic and geochemical data, as well as palaeo-geomorphology, we systematically analysed the quality of the carbonate gas reservoirs and source rocks, as well as the structural characteristics. The oil and gas accumulation conditions of the Qixia Formation are discussed and the favourable zones for natural gas accumulation are proposed to provide support for further exploration of the Middle Permian Qixia Formation in the Sichuan Basin.
Geological setting
The Middle Permian in the Sichuan Basin consists of the Liangshan, Qixia and Maokou Formations (Figure 1). The Sichuan Basin is in the upper Yangtze platform and adjacent to the Bayan Kara Basin to the west. The Caledonian palaeo-uplift was formed in the centre of the basin during the Hercynian tectonic compressional stage. Shallow-water carbonate platforms were developed during the Middle Permian on the inherited palaeo-geomorphology, while high-energy shoals were deposited on the periphery of the palaeo-uplifts and on the margins of the carbonate platform. The Liangshan Formation, Qixia Formation and Maokou Formation were successively developed in the Middle Permian successions from bottom to top. The Qixia Formation has a thickness of ∼100–120 m and can be further divided into the first member, mainly composed of dark grey and grey-black micritic clastic limestone and argillaceous bioclastic limestone, and the second member, deposited in high-energy shoals, that were composed mainly of packstones, grainstones and dolomitized carbonates. As a mainly gas reservoir, the Qixia dolomite has a single thickness of 15–40 m and a cumulative thickness of 60–110 m (Shen et al., 2015; Zhang et al., 2018). Dolomite is mainly distributed in the lower and middle parts of the second member of the Qiaxia Formation. Under the influence of the Caledonian, Liujiang and Yunnan movements, the strata between the Middle Permian and the Cambrian were eroded to a variable degree; thus, in some areas the Middle Permian strata is in direct contact with the Cambrian strata, forming a favourable reservoir-source assemblage for hydrocarbon accumulation (Zhang et al., 2018; Luo et al., 2020). During the Middle Triassic, under the strong compression exerted by the Indosinian movement, the Longmenshan fold and thrust structural belt was formed in the western part of the basin. After the modification by the Yanshan and Himalayan movements, this structural pattern, two uplifts on both sides and one depression in the middle, was formed (Zhang et al., 2020). Several faults and various types of traps were formed by tectonic movement and have played important roles in oil and gas migration and accumulation.
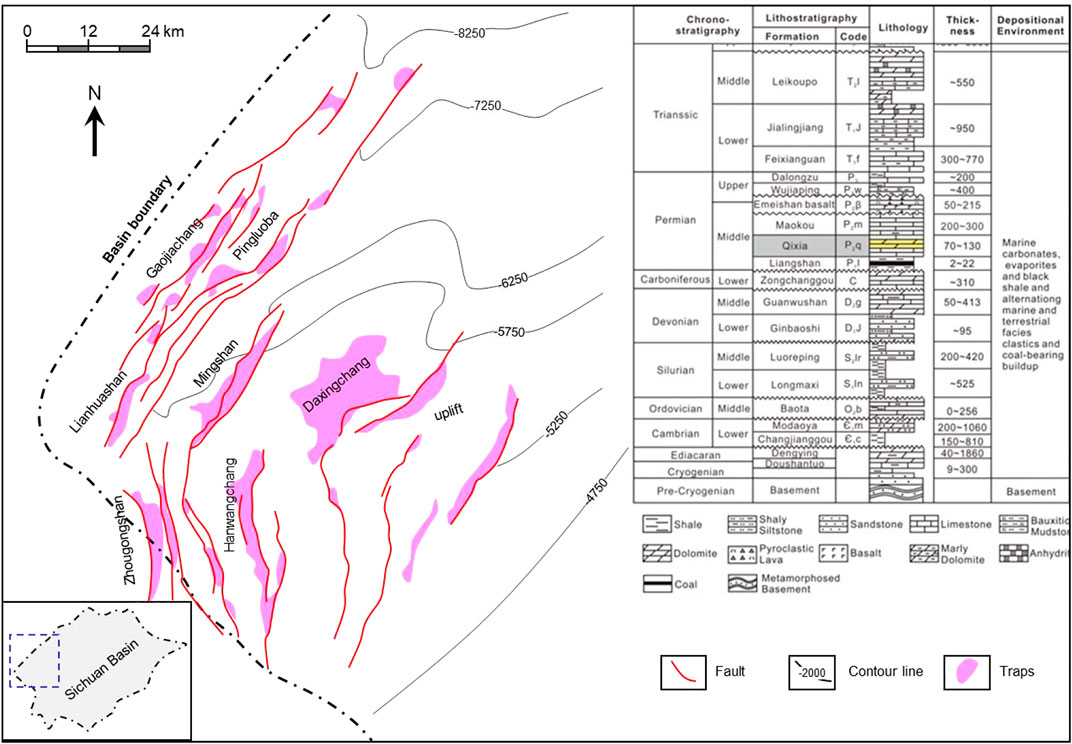
FIGURE 1. Location and generalised stratigraphy of the Sichuan Basin showing its main structures and traps on the top of the Qixia Formation.
Methods
Petrographic observation and fluid inclusion micro-thermometry were performed on different types of thin sections. Sections were half-stained with Alizarin Red S to distinguish dolomite from calcite. Some sections were impregnated with blue epoxy and pores showed blue under a standard polarising microscope. Microscopic observation and fluid inclusion micro-thermometry were conducted at the Research Institute of Exploration and Development, PetroChina Southwest Oil and Gas Field Company. Microscope observation and scanning used NIKON Ni-U and Leica DM6M automatic scanning microscopes. Additionally, three thin sections were used to conduct scanning electron microscopy and elemental analyses using an EPMA-1720 Electron Microprobe in Shandong Provincial Key Laboratory of Deep Oil and Gas, China University of Petroleum (East China). Homogenisation temperatures were measured using a NIKON Ni-U with a 50× telephoto working lens and THMS 600G cooling-heating table from Linkam. Temperature accuracy was 0.01°C.
Gas chemical compositions were measured using a Hewlett Packard 6890 Ⅱ gas chromatograph (GC) equipped with a flame ionisation detector and a thermal conductivity detector. The stable carbon isotopic values of the gas samples were determined on an Optima isotope ratio mass spectrometer (MS) equipped with the Hewlett Packard 6890 II GC. Accuracy was estimated to be ±0.5‰ with respect to the VPDB standard. An Agilent 7700 inductively coupled plasma mass spectrometer (ICP-MS) was used for rare earth element (REE) analysis at the Beijing Research Institute of Uranium Geology. REE concentrations were normalised to the post-Archean Australian shale.
A JS100007 helium porosimeter and A-10133 nitrogen permeameter were used to measure helium porosity and gas permeability, respectively, at the Research Institute of Exploration and Development, PetroChina Southwest Oil and Gas Field Company. Organic carbon content (TOC), organic type and vitrinite reflectance (Ro) were measured on source rock samples from cores and outcrops. The gas production rate can be obtained from the hydrocarbon generation chart according to the type of organic matter and Ro and the organic carbon recovery coefficient was determined. Thus, the gas generation intensity of point i could be calculated using Eq. 1 along with the thickness of the source rock
where
Noble gas analysis (He and Ar) was conducted as follows: gas was extracted by manual crushing of samples under ultrahigh vacuum (<2 × 10–7 mbar) using modified Swagelok valves with rounded steel tips. Samples were firstly partially crushed until the sample was fragmented and then fully crushed when the valve tip was fully extended. Samples were purified and analysed with the procedure by Li et al. (2020) with a blank and an air standard. The noble gas isotope-time formula used in this study were from Liu and Xu (1993) (Liu and Xu, 1993). 40Ar/36Ar and 3He/4He in natural gas were mathematically processed with the geological age of the source rock and the mathematical expressions between them were regressed: T (Ma) = 544.5 log (40Ar/36Ar) −1362.3 and T (Ma) = −633.3 log (3He/4He) − 3944.1, respectively.
Results
Gas reservoirs of the Qixia Formation are mainly dolostones, which consist of fine to middle dolomite crystals (Figure 2). The dolostones are generally porous (Figures 2A–C) and fabric-destructive with some residual bio-clastics and intra-clasts (Figure 2E). The pores include inter-particle pores (Figure 1E), inter-crystalline pores (Figures 2E–H), vuggy (Figure 2I) and caves (Figure 1A), as well as some fractures. The porosity of Qixia dolostones is in the range of 2%–6% with 3.7% average and the permeability is mainly in the range of 0.01–10 mD. The pore structure is dominated by the middle pore throat with good connectivity. The dolostones mainly occur on the platform margins in the western and along the periphery of the Caledonian palaeo-uplift in the central basin (Figure 3) and the thickness of dolostones tends to decrease from the platform-margin to the intra-platform shoals (Figure 4). Geochemical analysis shows that the Qixia dolostones have the δ13C values of 0.92‰–3.81‰ and the δ18O values of −8.68‰ to −3.43‰. The saddle dolomites show Ce-positive anomalies and extremely Eu-positive anomalies (Figure 5).
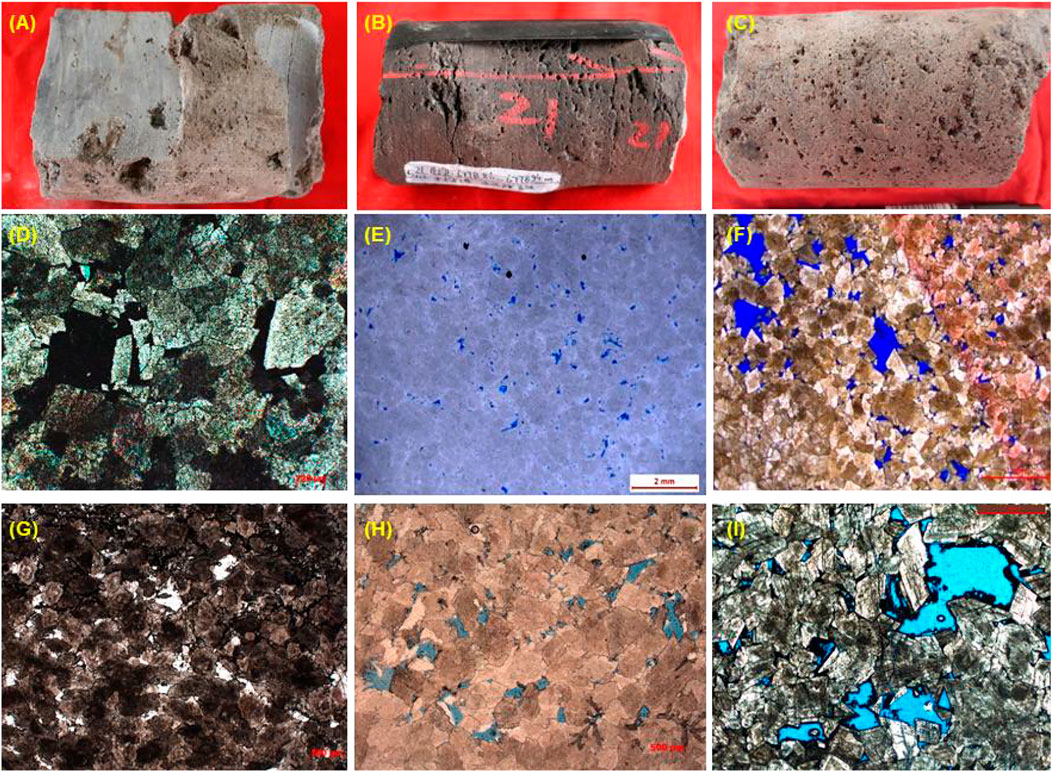
FIGURE 2. Lithology and reservoir space types of the Qixia Formation in the Sichuan Basin. (A) Well MX117, 4603.7–4603.8m, middle crystalline dolomite, vugs are filled with saddle dolomite. (B) Well PT1, 6778.86–6778.94m, finely crystalline dolomite. (C) Well SY132, 7590.6m, light brownish-grey fine-middle crystalline dolomite and pinhole developed. (D) Well MX117, 4603.01 m, middle crystalline dolomite, intergranular pore, intergranular solution pore developed (plane-polarised light). (E) Well K2, 2423.55 m, finely crystalline dolomite, biological particle phantom (plane-polarised light). (F) Well ST12, 7058.16 m, finely crystalline dolomite, inter-crystalline solution pores developed (plane-polarised light). (G) Well PT1, 6735.73 m, medium crystalline dolomite with inter-crystalline pores. (H) Well ST3, 7457.51 m, medium crystalline dolomite with inter-crystalline pores. (I) Well MX108, 4671.80 m, medium crystalline dolomite with vuggy pores.
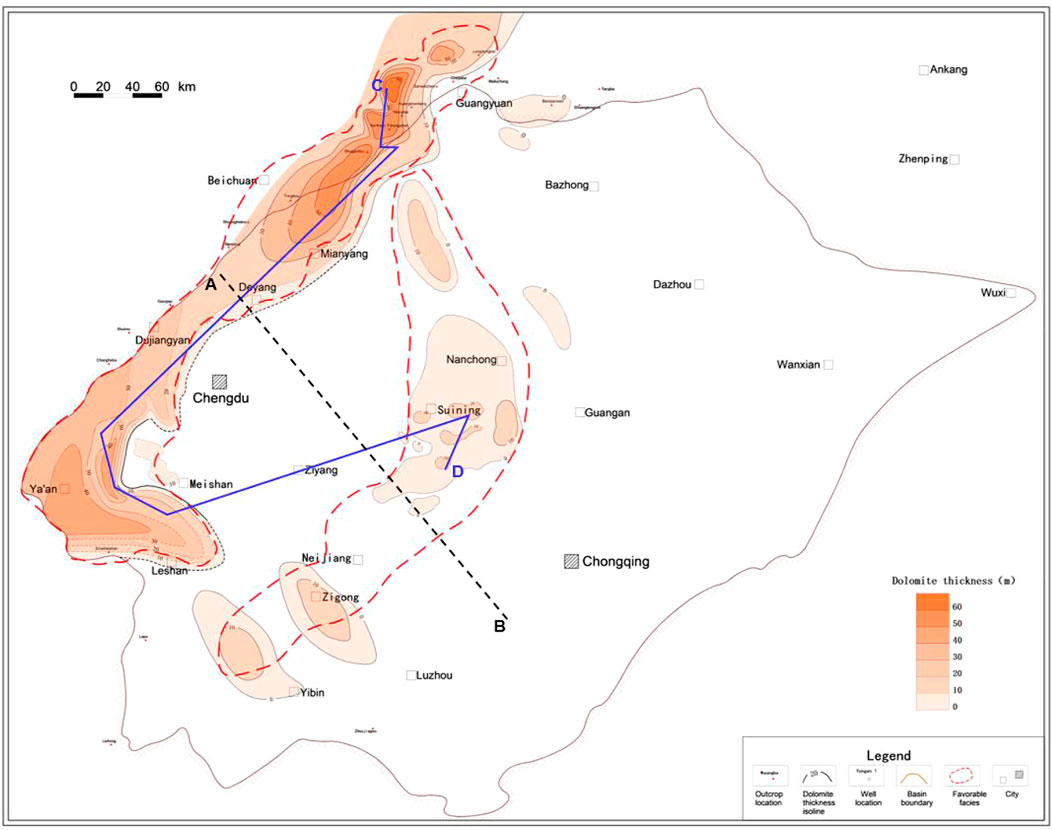
FIGURE 3. Contour map showing the thickness of dolomites in the Qixia Formation, Sichuan Basin. Panels A and B show the location of Figure 9 and C and D show the location of Figure 4.
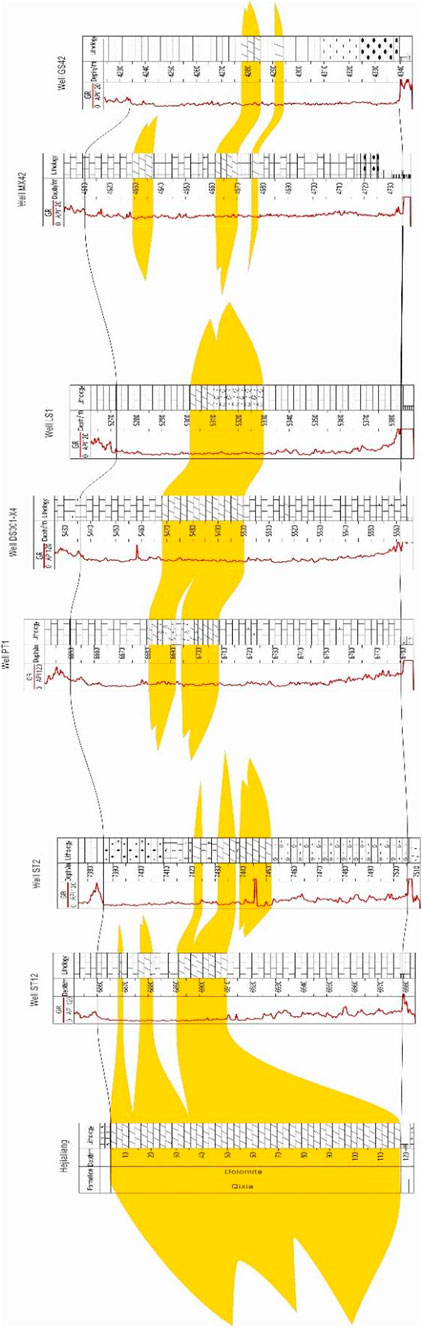
FIGURE 4. Cross section of the Qixia Formation dolomite reservoir (yellow area) in the Sichuan Basin.
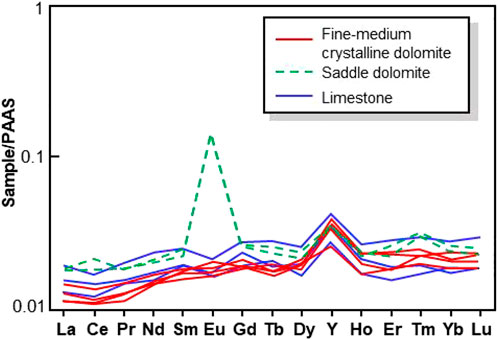
FIGURE 5. Distribution patterns of REE of limestone, fine to medium crystalline dolomite and saddle dolomite in the Qixia Formation. Data for saddle dolomite are from Lu et al. (2020) (Lu et al., 2020).
Natural gases from the Qixia carbonate reservoirs are dominated by methane with values of drying coefficient greater than 0.9. The ln (C1/C2) values are in the range of 6.2–7.1 and the ln (C2/C3) values are 3.1–3.9. Methane hydrogen isotope ratios range from −140‰ to −132‰, carbon C1 isotope values of methane show −32‰ to −29‰ and carbon C2 isotope values of methane are −29‰ to −26‰. The 3He/4He and 40Ar isotopic values of natural gases in the western Sichuan Basin are 2.3 × 10–8 and 2576.2, respectively (Table 1).
The gas generation intensity is 0–160 × 108 m3/km2 with (40–45) × 108 m3/km2 average. In particular, the maximum thickness of the Qiongzhusi source rock in the Deyang-Anyue rift trough exceeds 400 m and the gas generation intensity reaches 140 × 108 m3/km2 (Figure 6A). Among them, the average hydrocarbon generation intensity of the Lower Cambrian source rocks in the favourable facies zone of the Qixia Formation is 60 × 108 m3/km2. The Middle Permian Maokou Formation source rocks are distributed throughout the basin, with 30–220-m thickness (10–60) × 108 m3/km2 hydrocarbon generation intensity and >20 × 108 m3/km2 hydrocarbon generation intensity in most areas. The Middle Permian Qixia source rocks have a thickness of 10–70 m and the hydrocarbon generation intensity is <10 × 108 m3/km2. The hydrocarbon generation centres of the Middle Permian source rocks are mainly located in the northwestern and central-southern areas of the basin, with gas generation intensity reaching (26–44) × 108 m3/km2 (Figure 6B). The average hydrocarbon generation intensity of the Middle Permian source rocks in the favourable facies is 15 × 108 m3/km2.
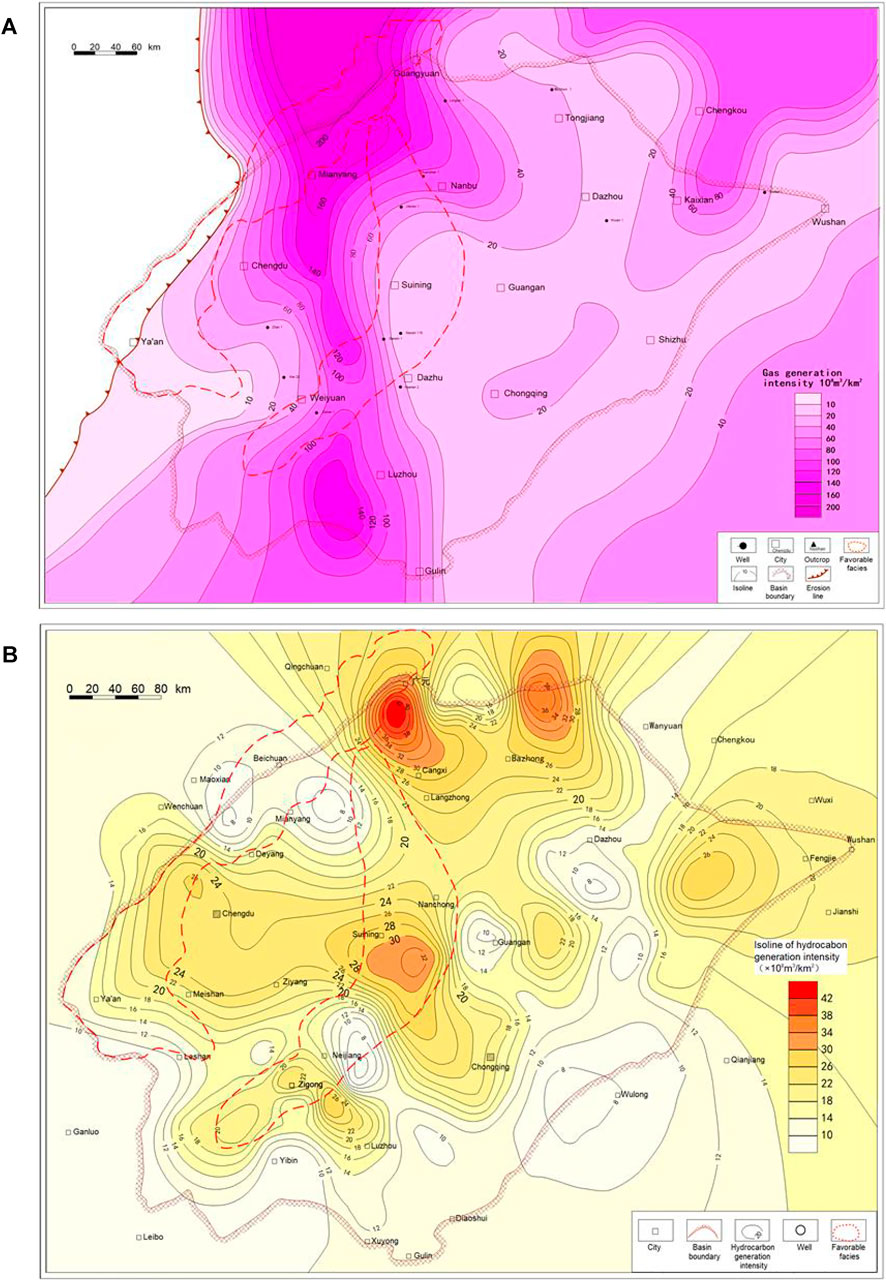
FIGURE 6. Overlapping diagram of hydrocarbon generation intensity of source rocks and favourable facies of the Qixia Formation. (A) Hydrocarbon generation intensity map of the Lower Cambrian source rocks. (B) Hydrocarbon generation intensity map of the Middle Permian source rocks.
Discussion
High-quality shoal dolomite reservoir
During the Early Permian, controlled by the inherited palaeo-geomorphology of the western Sichuan Basin and the Caledonian palaeo-uplift, shallow carbonate deposits were developed in the Qixia Formation in the Sichuan Basin, spanning from the west to the east and forming a basin-slope-platform margin-open platform. The west of the Sichuan Basin developed platform-margin shoals in a belt-like distribution. Along the periphery of the Caledonian palaeo-uplift, the intra-platform shoal facies developed in a ring belt pattern. They together form the ‘one margin and one ring belt’ distribution pattern of the shoal facies of the Qixia Formation in the basin (Figure 3), which ultimately controls the distribution of dolomite reservoirs in the Qixia Formation. Horizontally, the shoal dolomite in the platform margin is distributed in a zonal shape along the line of Hewan Chang-Jinshanliang-Pisces-Ya’an (Figure 3), with thicknesses of 20–60 m and a maximum at 110 m. The intra-platform shoal dolomite exhibits a ring-like pattern along the Gaomo–Nanchong–Shehong line. These widely distributed dolomites are commonly porous, serving as potential reservoirs for gas accumulations.
The analysis shows that the Qixia dolomite is formed by the replacement of penecontemporaneous seawater with later recrystallisation and modification by hydrothermal fluids. The carbon and oxygen isotope values and REE patterns of fine-middle crystalline dolomites are consistent with host limestone, indicating that they are mainly dolomitised by contemporaneous seawater. In addition the relatively low order degree of dolomite (0.6–0.7) indicates that the dolomite of the Qixia Formation was formed earlier and is most likely formed in the penecontemporaneous period, rather than burial dolomitization as proposed in previous work (Chen et al., 2013). The Ce-positive anomalies and extremely Eu-positive anomalies of saddle dolomites (Figure 5) indicate that dolomitising fluids are mantle-derived hydrothermal fluids in a relatively closed system and reducing conditions (Huang et al., 2011; Jiang et al., 2014; Yang et al., 2020). The overall high homogenisation temperature of fluid inclusions (80–240°C) are evidence for the high heat flow environment formed by the initial emplacement of the Early Permian Emeishan mantle plume. This event promoted the (re-)activation of faults and fractures as conduits for vertical migration of hydrothermal fluids into the reservoirs. The relatively high temperatures provided favourable conditions to overcome a dynamic barrier for dolomite precipitation (He and Feng, 1996; Huang et al., 2013; Zhu et al., 2015). Generally it can be summarised that dolomitization consists of thermally driven seawater replacement and superimposed hydrothermal modification in the penecontemporaneous phase. In carbonate successions in the Sichuan Basin gas reservoirs are mainly dolostones, whereas limestones are commonly tight (Wang et al., 2017a; Wang et al., 2022). The higher porosity of dolostones may be related to dolomitization and the rigid rock framework because new pores may be formed during the dolomitization (Wang et al., 2015).
High-quality source rocks
We propose that the natural gas of the Qixia Formation is mainly generated from the Lower Cambrian Qiongzhusi source rocks (Table 2). The gas comes from the thermal cracking of crude oil and a mixed source gas of Cambrian and Permian source rocks. Natural gases in the Qixia Formation mainly comprise methane at a high thermal evolution degree. Solid bitumen occurs pervasively in the pores of the reservoir, which is generally considered the by-product of secondary cracking of crude oil (Sun et al., 2019).
Methane carbon isotope values in the Qixia Formation show that C1 and C2 range from −32‰ to −29‰ and −29‰ to −26‰, respectively, consistent with that of the Dengying Formation in the Gaomo area of the central Sichuan Basin, indicating that the natural gas was generated by the Cambrian source rocks (Sun et al., 2019).
Noble gas isotopes in natural gas will accumulate gradually with increasing age. Generally, the older the source rocks are, the more the 40Ar and 4He will be accumulated, and the 40Ar/36Ar ratio increases with a decrease in 3He/4He ratios. Therefore, the isotopic analysis of noble gas can be used for further clarification of the source rock (Wu et al., 2017). The 3He/4He and 40Ar isotopic values of natural gases in the Qixia Formation in the western Sichuan Basin are 2.3 × 10–8 and 2576.2, respectively. The calculated timing for hydrocarbon generation of ∼451–487 Ma approximates the depositional age of the Cambrian source rock (510–570 Ma). Based on the distribution and geochemical characteristics of regional source rocks, it is generally considered that the Qixia Formation natural gas is a mixture co-contributed by the Lower Cambrian and Middle Permian source rocks and mainly comes from the Lower Cambrian Qiongzhusi Formation source rocks.
The Lower Cambrian Qiongzhusi source rocks and Middle Permian source rocks have a high abundance of organic matter and strong hydrocarbon generation capacity. The Lower Cambrian Qiongzhusi Formation is one of the main source rock intervals in the Sichuan Basin, is widely distributed and mainly consists of mudstone and shale. The average thickness of the Qiongzhusi source rock is large. The gas generation intensity is 0–160 × 108 m3/km2 averaging with (40–45) ×108 m3/km2. It is an important hydrocarbon generation kitchen in the basin and provides favourable conditions for the formation of gas reservoirs in the Qixia Formation surrounding the trough (Xu et al., 2014). In the favourable facies of the Middle Permian, source rocks have an average hydrocarbon generation intensity of 15 × 108 m3/km2, which is also an important source for natural gas in the Qixia Formation.
The Qixia dolomite reservoir and the high-quality Lower Cambrian and Middle Permian source rocks together form a variety of accumulation models. The Lower Cambrian source rocks provide gases for the upper Qixia reservoirs, which is referred to as “lower-generation and upper-accumulation”. The Middle Permian source rocks supply the hydrocarbons for the Qixia reservoirs, which is referred to as “side-generation and side-accumulation”. Influenced by the Caledonian and other multi-stage tectonic movements the strata between the Middle Permian and the Lower Cambrian strata in most parts of the basin were eroded and only a few remained. Locally, the Qixia Formation directly covered above the Qiongzhusi Formation, forming a superior hydrocarbon accumulation structure. First, the high-quality dolomite reservoirs of the Qixia Formation are distributed above the hydrocarbon generation centre of the Qiongzhusi Formation in the Deyang–Anyue rift trough in the northwestern Sichuan Basin. The underlying Carboniferous and Devonian strata are connected to the high-quality source rocks of the Qiongzhusi Formation through active faults. The Qixia dolomite reservoirs combined with the Qiongzhusi source rocks constituted an accumulation model of “lower-generation and upper-reservoir” (Figure 7). Simultaneously, as the area under study was in the hydrocarbon generation centre of Middle Permian source rocks, the accumulation condition of “self-generation and self-storage” was favoured (Figure 6B). The Qixia Formation in this area held a significant advantage of near-source accumulation, which rendered it a favourable zone for large-scale enrichment of oil and gas. Second, the Qixia Formation in the southwestern Sichuan Basin is in direct contact with the Qiongzhusi Formation and is adjacent to the hydrocarbon generation centre of the Qiongzhusi source rocks. The accumulation models can be summarised as “side-generation and side-accumulation” and “lower-generation and upper-accumulation” (Figures 6A, 7). The dolomite reservoirs of the Qixia Formation in the central Sichuan Basin are distributed on the periphery of the Caledonian palaeo-uplift, close to the hydrocarbon generation centre of the Qiongzhusi Formation, and the accumulation models of “side-generation and side-accumulation” can be formed through the unconformity surface, making it a favourable zone for oil and gas accumulation in the basin (Figures 5A, 6).
Various types of traps for oil and gas accumulation
The Longmenshan fold and thrust tectonic belt in the western Sichuan Basin has a structural pattern of “two uplifts on both sides and one depression in the middle”. The northern and southern segments of the Longmenshan tectonic belt were relatively uplifted and developed into a structural-high belt, whereas the middle part was relatively subsided and developed into a structural depression belt. The structural-high belt superimposes a high-quality Qixia dolomite reservoir, forming structural, structural-lithologic and other types of traps.
Three-dimensional seismic profiles show that under the influence of multi-stage tectonic movements such as the Indosinian and Himalayan orogenies, the thrusting of the northern part of the Longmenshan tectonic belt was strong and many regional large faults developed. The hanging wall of these faults thrusted and uplifted, while the footwall was compressed and folded, which together constituted the superimposed structural model of the complex fold belt and the thrust structure, forming the footwall of fault No. 1 in the front belt of the thrust structure. Bounded by faults No. 1, No. 2 and No. 10, a “three-step planar structural style” is presented (Figure 8). The anticline belt to the east of fault No. 10 is the third step, showing the anticline structural group divided by several local faults. The anticline belt between fault No. 2 and fault No. 10 is the second step. The small faults dividing the anticline are relatively few and the anticline is relatively complete. The anticline belt between fault No. 1 and fault No. 2 is the first step, the anticline trap is large and buried deep and it is a hidden structural zone located in the footwall of fault No.1 (Liang et al., 2018; Yang et al., 2018). The anticline structure formed by the three steps and the dolomite reservoirs of the Qixia Formation together form a large structural-lithologic trap. This trap is bounded by fault No. 1 to the west, dolomite reservoir area (10 m-thick reservoir) to the east and north and structural isoline to the south. An exploration breakthrough has been made in the Qixia Formation in the Shuangyushi area to the east of the No. 10 fault.
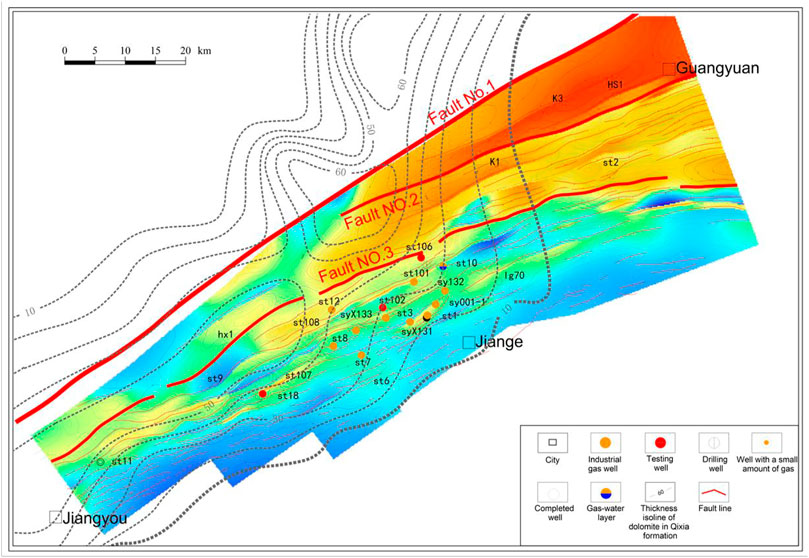
FIGURE 8. Distribution map of structural superimposed dolomite of the Qixia Formation in the northwestern Sichuan Basin.
The southern part of the Longmenshan structural belt can be divided into three structural systems, namely, the Longmenshan piedmont tectonic belt, the Emei–Washan fault blocks and the Daxingchang–Dongguachang structural belt. The Qixia dolomite develops continuously in this area (Zhang et al., 2018). Seismic data and drilling results show that in the Himalayan period, the Emei–Washan fault blocks developed a large fault extending to the surface by the regional compressional stress field and thrusting and, as a result, the gas preservation condition was poor. Consequently, many wells were verified to produce only water in the Qixia Formation. On the contrary, a cap detachment structural style is developed in the Longmenshan fold and thrust structural belt and Daxingchang–Dongguachang structural belt (Figure 9). The upper fault slipped off from the Triassic gypsum strata and was not communicated with overlying faults; therefore, the preservation conditions for gas reservoir were good, which is a favourable area for oil and gas accumulation. Several structural and structural-lithologic traps have developed in the Longmenshan fold and thrust and Daxingchang–Dongguachang structural belts, including Pingluoba structural belt, Dongguachang structural-lithologic trap, Daxingchang structural-lithologic trap and Qionglai north structural-lithologic trap (Figure 10), which all are beneficial for the accumulation of natural gas in the Qixia Formation in the southwestern Sichuan.
Large-scale gas accumulation
Combined with the homogenisation temperature of fluid inclusions of dolomite and regional thermal-burial evolution history, it is believed that the Qixia Formation experienced at least four stages of oil and gas charging. The first and second stages of oil and gas charging mainly occurred in the Middle to Late Triassic. The homogenisation temperature of fluid inclusions trapped at that time was 90–95°C and 110–120°C. During that time a large amount of liquid hydrocarbons was generated from the Lower Cambrian source rocks and migrated to the Qixia reservoirs. The Middle Permian source rocks began generating hydrocarbons and there were oil accumulations. In the Early–Middle Jurassic, the Lower Cambrian source rocks mainly produced kerogen-cracking gas. However, the Middle Permian source rocks produced a large amount of liquid hydrocarbons at temperatures of 135°C–140°C, resulting in the third stage of oil and gas charging. Simultaneously, the early-generated liquid hydrocarbons began to crack into gases. The fourth stage of hydrocarbon charging occurred from the Late Jurassic to the Early Cretaceous: when gas was generated from the two sets of source rocks and from the cracking of the early crude oil, with a homogenisation temperature of fluid inclusions of 160°C–180°C, and the charging timing, also the timing of the formation of the palaeo-gas reservoir, can be estimated by projecting onto the thermal evolution curve.
The top boundary of the Qixia Formation in the western Sichuan basin continued to be in the high position of the palaeo-structure at the end of the Middle Triassic and Late Jurassic (Figure 11). Multi-stage oil and gas charging from the Lower Cambrian and Middle Permian source rocks accumulated and was preserved consecutively in the Qixia Formation reservoirs. During the Indonesian-Yanshanian period, the hanging wall of the hidden fault in the Longmenshan complex tectonic belt formed an imbricate thrust structure and the footwall compressional fold formed a low-amplitude large structural-lithologic trap. Several episodes of hydrocarbon generation occurred in the Lower Cambrian and Middle Permian source rocks and subsequently entered the traps and accumulated. During the Himalayan period, the hanging wall was further uplifted and reversed and the lower structural-lithologic trap was complicated by associated faults, but its integrity was not destroyed. At this time, liquid hydrocarbons were cracked into gas in the early stage, forming a large structural-lithologic gas reservoir. Overall, the Qixia Formation has an accumulation evolution process of ‘continuous charging since the Indosinian period, stable maintenance in the Yanshanian period, local adjustment, modification and large-scale accumulation of oil and gas in the Himalayan period’ (Figure 12).
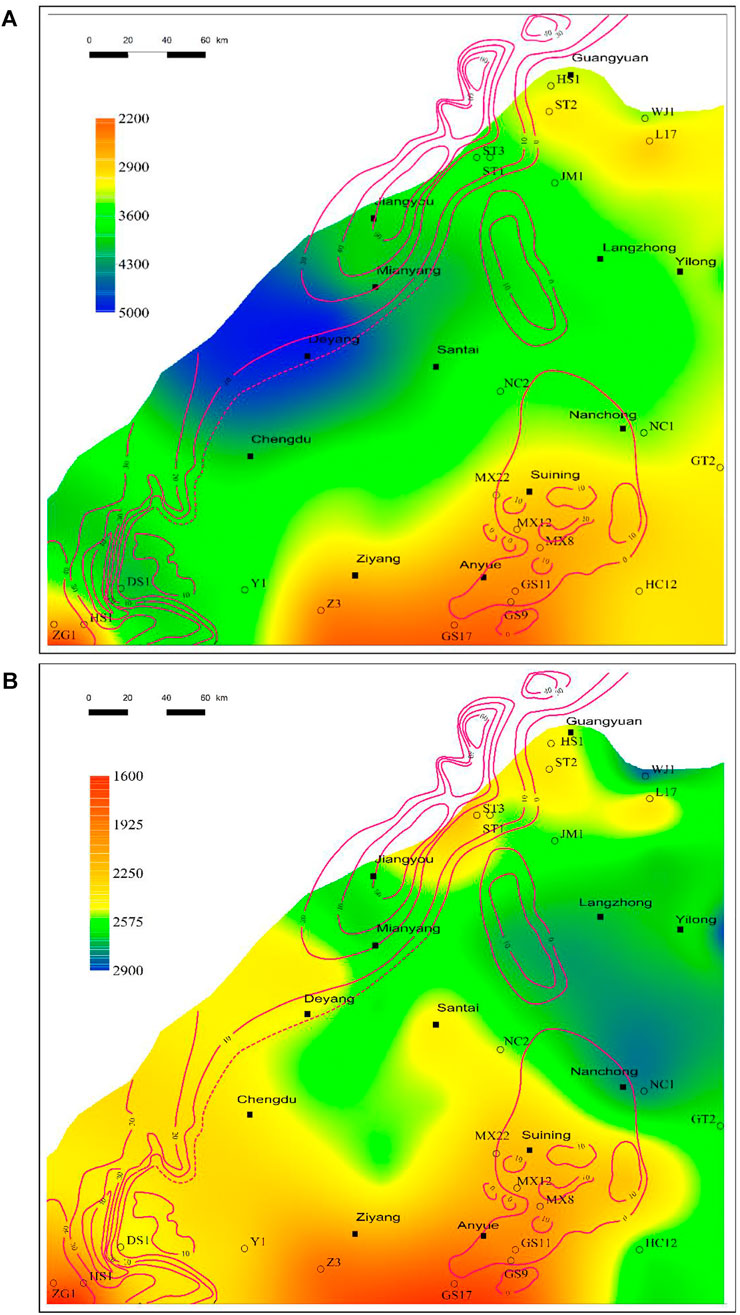
FIGURE 11. (A) Contour map of a dolomite thickness superimposed palaeo-structure of the Qixia Formation at Late Middle Triassic. (B) Contour map of a dolomite thickness superimposed palaeo-structure of the Qixia Formation at the end of Jurassic.
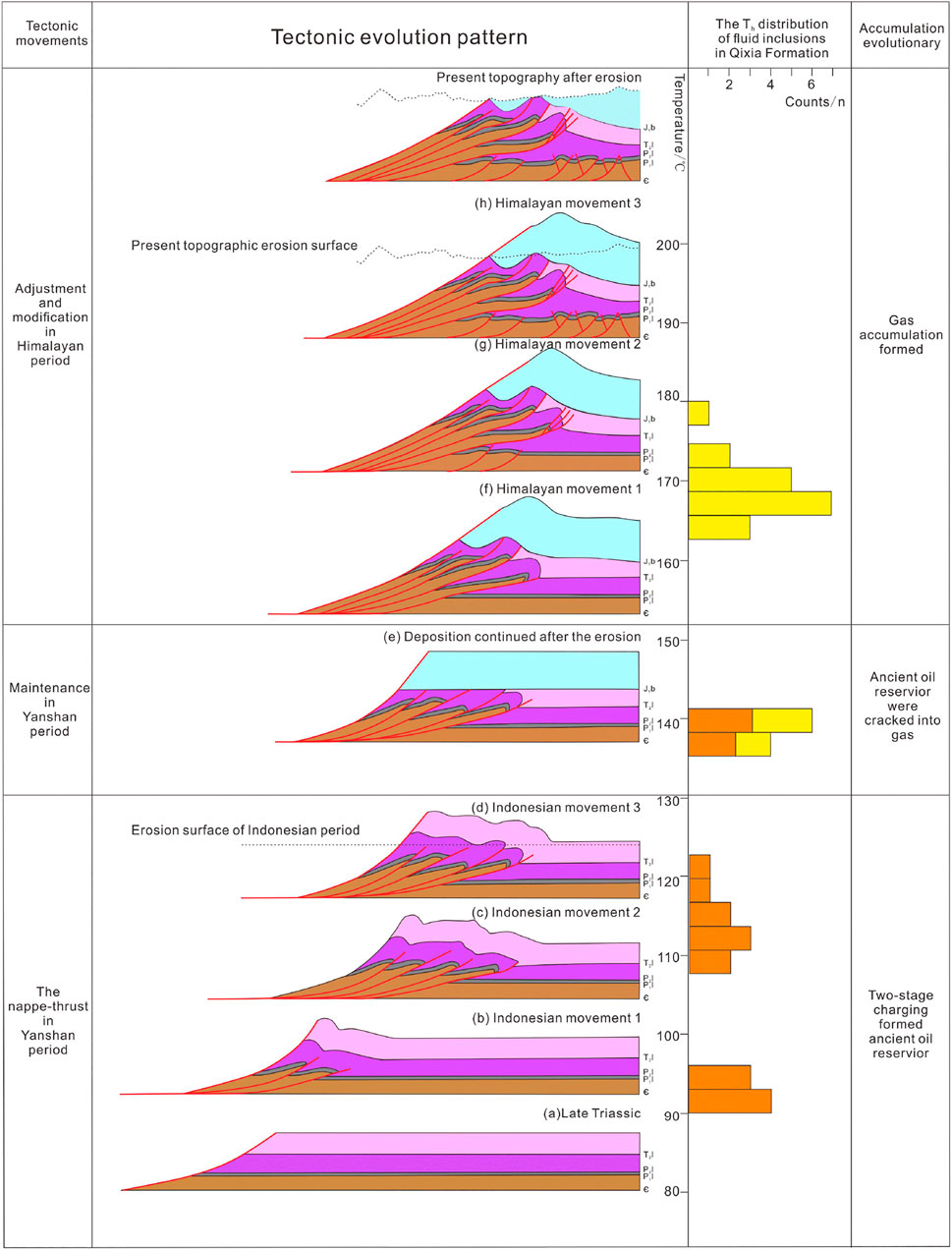
FIGURE 12. Matching relationship between hydrocarbon charging and palaeo-structural evolution of the Qixia Formation in Sichuan shows patterns of oil and gas charging and structural recovery.
Exploration potential
In the Sichuan Basin large volumes of gas accumulation in the Qixia Formation is beneficial from the following factors: wide distribution of high-quality dolomite reservoirs, good hydrocarbon source, superior relationship between the source-reservoir association and excellent matching relationship between the gas accumulation and structural evolution. Dolomites are more compaction-resistant and cementation than other lithology in deep burial settings (Wang et al., 2017b; Zhou et al., 2022). Thus, it has a great potential for gas exploration in the Qixia dolomite reservoirs. The platform margin shoal dolomite reservoirs of the Qixia Formation in the northwestern Sichuan Basin are widely developed, which are in the hydrocarbon generation centre of the Qiongzhusi source rocks and in the high position of the structure during hydrocarbon accumulation. Large structural-lithologic traps are developed in the footwall of fault No. 1, with a favourable exploration area of 2800 km2, in which the breakthrough area of the Shuangyushi area is 1600 km2.
The hidden structure composed of the first and second steps has a favourable exploration area of 1200 km2, with the estimated resources to be 400 billion square metres, which is an important field for increasing reserves and production of the Qixia Formation. Large-scale dolomite reservoirs were developed in the Longmenshan fold and thrust tectonic belt and Daxingchang–Dongguachang structural belt in the southwestern Sichuan Basin, and many structural, structural-lithologic traps with good preservation conditions were formed, with a favourable exploration area of approximately 1100 km2. At present, exploration has been made in the Pingluoba structural belt, but the gas-bearing scale still requires further verification. This is the key area in the Qixia Formation in terms of searching for large-scale reservoirs and expanding the exploration field. Additionally, the favourable exploration area of the platform shoal dolomite reservoir in the Qixia Formation surrounding the Caledonian palaeo-uplift is 5200 km2. However, the lack of exploration makes it a new field for the large-scale exploration of the Qixia formation in the basin.
Conclusion
1) The Qixia Formation in the Sichuan Basin has a fair hydrocarbon source, good-quality reservoirs and favourable source-reservoir assemblages as well as excellent accumulation conditions for forming medium-sized and/or large-sized gas fields. Continuous hydrocarbon is supplied from several sets of widely covered high-quality source rocks. Structural and structural-lithologic traps were developed in inherited structural-high belts.
2) Dolomite reservoirs are distributed on a large scale, occurring primarily in platform margins and in the inner-platform shoals surrounding palaeo-uplifts. The Qixia dolomites formed by seawater through enecontemporaneous dolomitization and the early formed dolomites were recrystallised and modified by later hydrothermal fluids.
3) The hidden structural belt in the footwall of fault No. 1 in the northwest of the basin and Longmenshan thrust and fold structural belt and Daxingchang structural belt in the southwest is a favourable area for large-scale gas exploration in the Qixia Formation. In the central Sichuan Basin, the area around palaeo-uplifts is favourable for gas exploration in the Qixia Formation, and it is a new field for large-scale exploration.
Data availability statement
The original contributions presented in the study are included in the article/supplementary material, further inquiries can be directed to the corresponding author.
Author contributions
ZY, CS, and WL contributed to conception and design of the study. ZY, QL and ZB organized the database. ZY, WH, XC and QY performed the analysis. ZY and WG wrote the first draft of the manuscript. QL wrote sections of the manuscript. All authors contributed to manuscript revision, read, and approved the submitted version.
Funding
This study was supported by the National Natural Science Foundation of China (41902130).
Acknowledgments
Special thanks are extended to Associate Editor Senhu Lin and three reviewers for their critical and constructive reviews.
Conflict of interest
The authors YZ, SC, LW, BZ, HW, CX, XZ, RL and YQ were employed by PetroChina Southwest Oil and Gas Field Company.
The remaining authors declare that the research was conducted in the absence of any commercial or financial relationships that could be construed as a potential conflict of interest.
Publisher’s note
All claims expressed in this article are solely those of the authors and do not necessarily represent those of their affiliated organizations, or those of the publisher, the editors and the reviewers. Any product that may be evaluated in this article, or claim that may be made by its manufacturer, is not guaranteed or endorsed by the publisher.
References
Chen, X., Zhao, W. Z., Liu, Y. H., Zhou, H., and Jiang, Q. C. (2013). Characteristics and exploration strategy of the Middle Permian hydrothermal dolomite in southwestern Sichuan Basin. Acta Pet. Sin. 34 (3), 460–466. doi:10.7623/syxb201303006
Dai, T. S., and Ou, Z. Z. (1990). Fracture traps and their exploration method. Nat. Gas. Ind. 10 (4), 1–6.
He, Y. B., and Feng, Z. Z. (1996). Origin of fine-to coarse-grained dolostones of Lower Permain in Sichuan Basin and its peripheral regions. J. Jianghan Petroleum Inst. 18, 5–20.
Hu, M. Y., Hu, Z. G., Wei, G. Q., Yang, W., and Liu, M. C. (2012). Sequence lithofacies paleogeography and reservoir prediction of the Maokou Formation in Sichuan basin. Petroleum Explor. Dev. 39 (1), 45–55. doi:10.1016/S1876-3804(12)60014-7
Huang, S. J., Lv, J., Lan, Y. F., Huang, K. K., and Wang, C. M. (2011). The main texture of dolomite of middle permain, Western Sichuan Basin: Concurrently on the difference with upper permian-triassic, northeast Sichuan Basin. Acta Petrol. Sin. 27 (8), 2253–2262.
Huang, S. J., Pan, X. Q., Lv, J., Qi, S. C., Huang, K. K., Lan, Y. F., et al. (2013). Hydrothermal dolomitization and subsequent retrograde dissolution in Qixia formation, west sichuan: A case study of incomplete and halfway-back dolomitization. J. Chengdu Univ. Technol. Sci. Technol. Ed. 40 (003), 288–300. doi:10.3969/j.issn.1671-9727.2013.03.09
Jiang, Q. C., Hu, S. Y., Wang, Z. C., Wang, T. S., Li, Q. F., and Qu, X. F. (2014). Genesis of medium-macro-crystalline dolomite in the middle permian of Sichuan basin. Oil Gas Geol. 35 (4), 503–510 doi:10.11743/ogg201409in Chinese with English abstract
Liang, H., Xiao, F. S., Ran, Q., Han, S., Chen, X., Di, G. D., et al. (2018). Accurate structural modeling on the northern section of the complex structural belt at Longmenshan piedmont in the Sichuan Basin and its implications for oil and gas exploration. Nat. Gas. Ind. 38 (11), 26–32. in Chinese with English abstract. doi:10.3787/j.issn.1000-0976.2018.11.003
Liu, W. H., and Xu, Y. C. (1993). Significance of helium argon isotopic composition in natural gas. Chin. Sci. Bull. 38 (9), 818–821.
Lu, F., Tan, X., Zhong, Y., Luo, B., Zhang, B., Zhang, Y., et al. (2020). Origin of the penecontemporaneous sucrosic dolomite in the permian Qixia formation, northwestern Sichuan basin, SW China. Petroleum Explor. Dev. 47, 1218–1234. doi:10.1016/s1876-3804(20)60131-3
Luo, B., Wen, L., Zhang, Y., Xie, Z., Cao, J., Xiao, D., et al. (2020). Differential gas accumulation process of the middle permian Qixia formation, northwestern Sichuan basin. Oil Gas Geol. 41 (2), 393–406. doi:10.3787/j.issn.1000-0976.2020.07.004
Shen, P., Zhang, J., Song, J. R., Hong, H. T., Tang, D. H., Wang, X. J., et al. (2015). Significance of breakthrough in and favorable targets of gas exploration in the Middle Permian system, Sichuan Basin. Geol. Explor. 35 (7), 1–9 doi:10.3787/j.issn.1000-0976.2015.07.001in Chinese with English abstract
Sun, Y. T., Tian, X. W., Ma, K., Peng, H. L., Dai, H. M., Wang, H., et al. (2019). Carbon, and hydrogen isotope characteristics and source of natural gas in Shuangyushi gas reservoir, northwestern Sichuan Basin. Nat. Gas. Geosci. 30 (10), 1477–1486. doi:10.11764/j.issn.1672-1926.2019.10.011
Wang, G. W., Chang, X. C., Yin, W., Li, Y., and Song, T. T. (2017). Impact of diagenesis on reservoir quality and heterogeneity of the Upper Triassic Chang 8 tight oil sandstones in the Zhenjing area, Ordos Basin, China. Mar. Petroleum Geol. 83, 84–96. doi:10.1016/j.marpetgeo.2017.03.008
Wang, G. W. (2022). Dolomitization and dolomite pore formation: Insights from experimentally simulated replacement. Acta Sedimentol. Sin., org/doi:10.14027/j.issn.1000-0550.2022.062
Wang, G. W., Hao, F., Chang, X. C., Lan, C. J., Li, P. P., and Zou, H. Y. (2017). Quantitative analyses of porosity evolution in tight grainstones: A case study of the triassic feixianguan Formation in the jiannan gas field, Sichuan basin, China. Mar. Petroleum Geol. 86, 259–267. doi:10.1016/j.marpetgeo.2017.05.021
Wang, G. W., Hao, F., Zhang, W. B., Zou, H. Y., and Li, P. P. (2022). Characterization and origin of micropores in tight gas grainstones of the lower triassic feixianguan formation in the jiannan gas field, Sichuan basin. Mar. Petroleum Geol. 139, 105609. doi:10.1016/j.marpetgeo.2022.105609
Wang, G. W., Li, P. P., Hao, F., Zou, H. Y., and Yu, X. Y. (2015). Dolomitization process and its implications for porosity development in dolostones: A case study from the lower triassic feixianguan formation, jiannan area, eastern Sichuan basin, China. J. Petroleum Sci. Eng. 131, 184–199. doi:10.1016/j.petrol.2015.04.011
Wu, Z. T., Liu, X. W., Li, X. F., Wang, X. F., and Zheng, J. J. (2017). The application of noble gas isotope in the gas-source correlation of Yuanba reservoir, Sichuan Basin. Nat. Gas. Geosci. 28 (7), 1072–1077. doi:10.11764/j.issn.1672-1926.2017.06.005
Xu, C. C., Shen, P., Yang, Y. M., Luo, B., Huang, J. Z., Jiang, X. F., et al. (2014). Accumulation conditions and enrichment patterns of natural gas in the lower Cambrian longwangmiao fm reservoirs of the Leshan-longnose paleo-high, Sichuan basin. Nat. Gas. Ind. 34 (3), 1–7. doi:10.3787/j.issn.1000-0976.2014.03.001
Yang, Y. M., Chen, C., Wen, L., Chen, X., Liang, H., Liu, R., et al. (2018). Characteristics of buried structures in the northern Longmenshan mountains atheirits significance to oil and gas exploration in the Sichuan Basin. Nat. Gas. Ind. 38 (8), 8–15. doi:10.3787/j.issn.1000-0976.2018.08.002
Yang, Y. M., Yang, Y., Weng, L., Zhang, X. H., Chen, C., Chen, K., et al. (2020). New exploration progress and the prospect of middle Permian natural gas in the Sichuan basin. Nat. Gas. Ind. 40 (7), 10–22. in Chinese with English abstract. doi:10.3787/j.issn.1000-0976.2020.07.002
Zhang, B. J., Ying, H., Li, R. R., Xie, Z., Wang, X. X., Pei, S. Q., et al. (2020). Breakthrough of natural gas exploration in the Qixia Formation of middle Permian by well Pingtan 1 in the southwestern Sichuan basin and its implications. Nat. Gas. Ind. 40 (7), 34–41. doi:10.3787/j.issn.1000-0976.2020.07.004
Zhang, J., Zhou, G., Zhang, G. R., Li, G. H., and Wang, H. (2018). Geological characteristics and exploration orientation of Mid-Permian natural gas in the Sichuan Basin. Nat. Gas. Ind. 38 (1), 10–20. in Chinese with English abstract. doi:10.3787/j.issn.1000-0976.2018.01.002
Zhou, L., Wang, G. W., Hao, F., Xu, R., Jin, Z., Quan, L., et al. (2022). The quantitative characterization of stylolites in the limestone reservoirs of the Lower Triassic Feixianguan Formation, northeastern Sichuan Basin: Insights to the influence of pressure solution on the quality of carbonate reservoirs. Mar. Petroleum Geol. 139, 105612. doi:10.1016/j.marpetgeo.2022.105612
Keywords: one margin and one ring belt, Cambrian, middle-late Triassic to early Cretaceous, qixia formation, Sichuan basin
Citation: Zhang Y, Chen S, Wen L, Zhang B, Wang H, Xiao D, Xie C, Zhang X, Quan L, Liu R, Qiao Y and Wang G (2022) Natural gas accumulation conditions and exploration prospects of the Middle Permian Qixia Formation in the Sichuan Basin. Front. Earth Sci. 10:980490. doi: 10.3389/feart.2022.980490
Received: 28 June 2022; Accepted: 16 August 2022;
Published: 09 September 2022.
Edited by:
Senhu Lin, Research Institute of Petroleum Exploration and Development (RIPED), ChinaReviewed by:
Yanyan Chen, Research Institute of Petroleum Exploration and Development (RIPED), ChinaMeng Xiao, Research Institute of Petroleum Exploration and Development (RIPED), China
Xiangwen Kong, Research Institute of Petroleum Exploration and Development (RIPED), China
Copyright © 2022 Zhang, Chen, Wen, Zhang, Wang, Xiao, Xie, Zhang, Quan, Liu, Qiao and Wang. This is an open-access article distributed under the terms of the Creative Commons Attribution License (CC BY). The use, distribution or reproduction in other forums is permitted, provided the original author(s) and the copyright owner(s) are credited and that the original publication in this journal is cited, in accordance with accepted academic practice. No use, distribution or reproduction is permitted which does not comply with these terms.
*Correspondence: Ya Zhang, emhhbmd5YTA4QHBldHJvY2hpbmEuY29tLmNu