- 1Department of Early Prehistory and Quaternary Ecology, University of Tübingen, Tübingen, Germany
- 2Senckenberg Centre for Human Evolution and Palaeoenvironment, University of Tübingen, Tübingen, Germany
- 3The Role of Culture in Early Expansions of Humans (ROCEEH), Heidelberg Academy of Sciences and Humanities at the University of Tübingen, Tübingen, Germany
- 4Geological Sciences, University of KwaZulu-Natal, Durban, South Africa
- 5School of Geography and Environmental Sciences, Ulster University, Coleraine, United Kingdom
The use of marine resources and the settlement of coastal settlements may have influenced the bio-cultural evolution and dispersal of Pleistocene Homo sapiens in Africa. In order to test such scenarios, however, we require evidence for these behaviours deriving from an expanded spatio-temporal archaeological record. The Stone Age of South Africa documents the richest and longest record of coastal adaptations. In contrast to abundant evidence of coastal sites on the western and southern seaboard, the eastern Indian Ocean coast has not played a role in recent discussions. Considering the important and well-known Middle and Later Stone Age (MSA/LSA) record from inland sites of KwaZulu-Natal (KZN), this region may be an underappreciated area for further study. Here we provide a systematic overview of marine resource use and the settlement of coastal landscapes during the Late Pleistocene and Holocene in KZN. For the first time, we contextualize these archaeological findings with a review and new data on the changing marine and coastal geomorphology in the context of GIS modelling and offshore marine geophysical investigations. Our review finds evidence for long-term dietary and non-dietary use of marine resources during the MSA and LSA from a few stratified sites, with many more surface occurrences particularly for the MSA along the modern KZN coastline indicating human habitation. Comparisons to other areas of South Africa, GIS modelling and geological considerations suggest that current data on the eastern seaboard are not reflective of the original extent and nature of the consumption of marine foods and settlement of coastal landscapes. By contextualizing the biased and patchy MSA and LSA record with results on the dynamic marine and coastal geomorphology of KZN, we develop productive lines of future studies to assess open questions on potential coastal adaptations in this region. These research strategies include the identification of areas with high potential for finding new sites within a 10 km transect along the current coastline as well as dedicated off-shore projects including underwater archaeology aided by new marine geological work in the southwest Indian Ocean.
1 Introduction
Humans have a deep-seated connection with oceans, coasts and the organisms inhabiting these ecosystems: today, more than 600 million people live in coastal communities, beaches are highly popular natural attractions to tourists and seafood is a major source of diet found around the globe. Archaeologists and paleoanthropologists have long been interested in the evolutionary roots of this relationship. Coastal foraging by hunter-fisher-gatherers during the Holocene and in recent times has been among the main research topics of archaeology since its inception (Forchhammer et al., 1852; Morse, 1877; Uhle, 1907). In contrast, the prevailing view on the Pleistocene use of beaches and oceans during most of the 20th century has been rather negative. With a few notable exceptions (e.g. Sauer 1962), the majority opinion has been that shorelines were marginal, dangerous and unattractive ecosystems, with many coastlines being uninhabitable and barriers to expansion due to a lack of potable water, resulting in a lack of evidence for a deep-time engagement of people with oceans such as manifested in the form of shell middens (e.g. Binford, 1968; Washburn and Lancaster, 1968; Meighan, 1969; Osborn, 1977; Waselkov, 1987; Yesner, 1987; for discussion see Erlandson, 2001).
A renewed interest in the topic coupled with novel archaeological data has challenged this picture. Over the past 20 years, the earliest habitations of coastal landscapes and the exploitation of seafood have become important topics for understanding the bio-cultural evolution of Homo sapiens during the Pleistocene (Erlandson, 2001; Parkington, 2003; Jerardino and Marean 2010; Marean, 2014; Jerardino 2016; Will et al., 2019). The potential of coastal landscapes to facilitate dispersals of early modern humans from Africa has likewise been a major theme of recent research (e.g., Field and Lahr, 2006; Mellars et al., 2013). While there is still ongoing debate and the actual relevance of these behaviors for human evolution and dispersal have been criticised (see Bailey, 2009; Boivin et al., 2013; Groucutt et al., 2015; Blinkhorn et al., 2017; Rabett, 2018), there is now good evidence for some populations of both Neanderthals and modern humans having adapted to coastal ecosystems and marine food resources in a systematic way over a period of many tens of thousands of years stretching back to the late Middle Pleistocene (e.g. Will et al., 2019; Zilhão et al., 2020).
Throughout the article we follow our recent definition of the term ‘coastal adaptations’ as a multifaceted array of behavioral traits in a population beneficial to their fitness, consisting of the regular and systematic acquisition, consumption and use of marine resources as well as an expansion of settlement systems that included repeated and planned occupation of coastal landscapes (for a more detailed discussion of the concept see Will et al., 2016: 70; Will et al., 2019: 36; for an even broader approach of ‘coastal engagement’ see Faulkner et al., 2021). The majority of evidence on such Pleistocene use of coasts by Homo sapiens has come from the African Middle Stone Age (MSA), with the longest record for the consumption of marine resources deriving from sites in northern and particularly southern Africa (for northern Africa see Steele and Alvarez-Fernández, 2011; Campmas et al., 2016). In South Africa, the oldest known collection of edible shellfish derives from Pinnacle Point Cave 13B at ∼164 ka (Jerardino and Marean, 2010), one of the very few localities in Africa with evidence predating the Eemian interglacial. During Marine Isotope Stage (MIS) 5e, systematic shellfish gathering has been documented at Ysterfontein 1 (120–113 ka; Niespolo et al., 2021) and Hoedjiespunt 1 (130–100 ka; Will et al., 2013; Kyriacou et al., 2014; Tribolo et al., 2022). Multiple localities with ample evidence for coastal adaptations as defined above date to between ∼110 ka and 50 ka, including Klasies River (Thackeray, 1988), Blombos Cave (Langejans et al., 2012), Die Kelders Cave 1 (Grine et al., 1991), Klipdrift Shelter (Henshilwood et al., 2014) and Herolds Bay Cave (Brink and Deacon, 1982) on the southern Indian Ocean coast, an Diepkloof (Klein and Steele, 2013), Varsche Rivier 003 (Steele et al., 2016) and Sea Harvest (Volman, 1978) on the western Atlantic coast. In sum, ample archaeological data exists for coastal adaptations during the Late Pleistocene in sites which escaped high sea level of the Holocene and Eemian interglacials.
Yet, the archaeological record of coastal occupation and resource use is not equally distributed along African shorelines. Vast gaps in knowledge plague the African record, such as in western or eastern Africa (see e.g. Niang et al., 2020; Faulkner et al., 2021), and even in regions that are comparatively well-studied such as South Africa. In contrast to abundant evidence of Pleistocene coastal sites on the western and southern seaboard, the eastern Indian Ocean coastline and the province of KwaZulu-Natal (KZN; Figure 1) in particular have not played any role in recent discussions due to the perceived scarcity of relevant data, and those often deriving from old publications. The recently excavated coastal site of Waterfall Bluff dated to ∼38–10 ka, located in Eastern Pondoland ca. 50 km southwest of KZN, is a notable exception (Esteban et al., 2020; Fisher et al., 2020). Considering this recent evidence and the important MSA and LSA archaeological record from inland sites of this region, the seaward sectors of KZN may be an underappreciated area for further study (Bader and Will, 2017). The specific coastal and marine ecology of KZN likely impacted the currently known archaeological record of this region. Most importantly, the Indian Ocean coast features a different terrestrial biome and lower marine productivity compared to the Fynbos and nutrient-rich waters of the Atlantic ocean coast (e.g. Kyriacou, 2017).
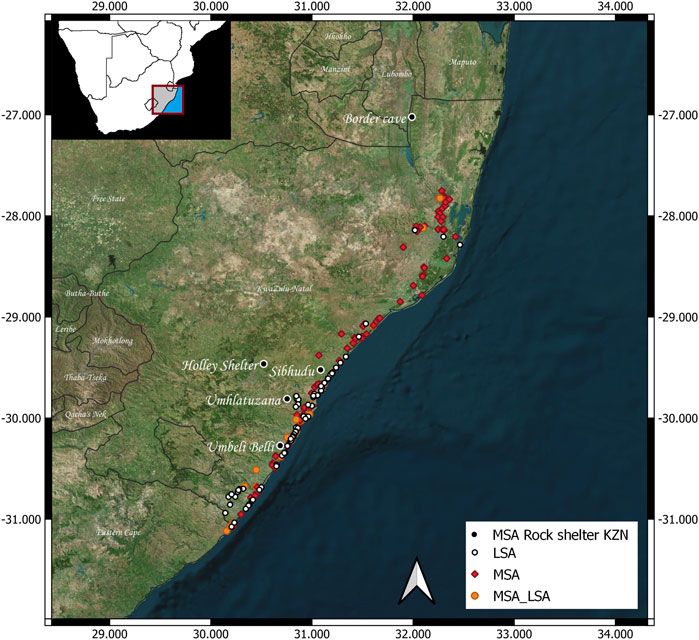
FIGURE 1. Overview map of the province of KwaZulu-Natal in southern Africa (see inset top left) with named key MSA rockshelter sites as well as MSA, LSA and mixed MSA/LSA sites from an exhaustive database of sites within a transect of 20 km from the modern shoreline.
Connected to this research gap are the more general issues of the relevance and spread of coastal adaptations in early humans. Some scholars see the current evidence as geographically isolated behaviors, derived primarily from the highly productive marine ecosystems such as the Atlancic ocean coast in southwestern South Africa (Bailey 2009; Boivin et al., 2013; Blinkhorn et al., 2017). Such a pattern could imply that Pleistocene people were limited in their capability to adapt to variable coastal ecosystems and only able to exploit the most prolific shorelines in an efficient manner. The record is further biased towards specific areas by climate and geology, particularly Pleistocene changes in sea-levels with only few sites close to the ocean having escaped erosion during sea-level highstands (Bailey and Flemming, 2008; Bailey, 2009; Bailey et al., 2015). In order to test scenarios for the spatial bias, selective advantages and dispersal capabilities of coastal adaptations, we require more evidence for these behaviours deriving from an expanded spatio-temporal archaeological record from diverse ecological, geographical and geological settings.
Here we examine the understudied region of KZN which differs in its marine ecology and offshore geology from the well-known record of western and southern South Africa. We are interested in how such areas can contribute to a better understanding and evaluation of the evolutionary relevance of coastal adaptations. This goal requires a joint approach of archaeology, marine geology and geography. The KZN shoreline is an ideal test case. For one, the region possesses a mostly steep offshore bathymetric profile with a mix of narrow and broader continental shelves. As a result, coastal sites in some regions had a chance of escaping erosion by post-glacial sea-level rise due to limited coastline movements across glacial/interglacial cycles. At the same time, the marine ecosystem of this region is less productive compared to the western Atlantic coast. Here we provide a systematic overview of marine resource use and the settlement of coastal landscapes during the Late Pleistocene, considering localities within 20 km of the current coastline of KZN. We contextualise these archaeological findings with a review and both new and existing data on the changing marine and coastal geomorphology in this area in the context of GIS modelling and offshore marine geophysical investigations. We start by introducing the general geological and physiographic setting of the region.
2 Geological and physiographic setting
The KZN continental margin comprises a sheared passive margin that formed during the breakup of Gondwana (Martin and Flemming, 1988). The margin is currently tectonically stable, and has been so since the late Pliocene, following several pulses of hinterland uplift that deposited most of the KZN shelf edge sequences (Green, 2011; Green and Garlick, 2011). The KZN shelf can be divided into three main zones. The southern KZN shelf, from the Mzimkulu River to Durban, is narrow with an average width of 8 km (Bosman, 2012; Green et al., 2018; Pretorius et al., 2019). In some places, this is decreased where submarine canyons intersect the shelf break (Flemming, 1981). From Durban to Port Durnford, the shelf widens to a maximum of ∼40 km offshore the Thukela River (Martin and Flemming, 1988; Engelbrecht et al., 2020), before narrowing to a minimum of 4 km from Richards Bay to the Mozambique border (Green et al., 2022a). The area between Durban and Port Durnford is known as the Natal Bight, and is underlain by a Kimmeridgian to Cenozoic-aged submerged fan delta complex formed by the Thukela River, termed the Thukela Cone (Hicks and Green, 2016). The shelf break throughout KZN occurs at a uniform depth of 100 m, though its expression varies from distinct in the south and north, to relatively gradual in the central areas offshore the Thukela River.
To the south and north of Durban, the acoustic basement comprises Cretaceous-aged siltstones into which several fluvial valleys associated with the Pleistocene lowstands are incised (Green, 2009a; Green, 2011; Pretorius et al., 2019). The acoustic basement of the Natal Bight comprises Pliocene-age siltstones of the Thukela Cone (Hicks and Green, 2016). Throughout KZN, the acoustic basement forms a shallow feature covered by only a thin veneer of Holocene sediment (Flemming, 1978; Flemming, 1981). Unconsolidated sediment cover, up to 30-m-thick, may be found on the inner shelf, decreasing to no more than a few meters at the ∼50 m isobath (Flemming, 1981; Green and Garlick, 2011; Green et al., 2022a). Seaward of the 50 m isobath, the shelf is increasingly sediment starved with mostly exposed bedrock mantled by patches of palimpsest bioclastic gravels (Green, 2009b; Green and Garlick, 2011; Pretorius et al., 2019; Green et al., 2022a).
Of special importance to this paper are several aeolianite and beachrock features that are commonplace along the mid to outer shelf of KZN (Cawthra et al., 2012; Green et al., 2018; Pretorius et al., 2018). Beachrocks are lithified beach sediments cemented by high-Mg calcite precipitated in the mixing zone of seawater and meteoric waters (Mauz et al., 2015). They are known to form particularly rapidly along the KZN coastline, often within a span of 80 years or less (Wiles et al., 2018; Falkenroth et al., 2022). The coastal aeolianites of KZN formed through the precipitation of low-Mg calcite from CO2-saturated meteoric waters in coastal dunes (Cooper and Green, 2016). The narrow, steep and bedrock-framed coastline of KZN means that beachrock and aeolianite often occur in tandem with each other due to the limited accommodation space that arises from such coastal topography (Cooper and Flores, 1991; Kelly et al., 2014). Their presence on the shelf reflects inter alia multiple cycles of shoreline inheritance and occupation throughout the mid Pleistocene to Holocene (Bosman, 2012; Pretorius et al., 2016).
Much of the shelf is exposed and presents a relict topography due, in part, to the strong sweeping effects of the Agulhas Current, a fast, poleward-flowing western boundary current (Lutjeharms, 2006). This current hugs the shelf break and periodically impinges into the mid-shelf areas (Flemming, 1978). In tandem with the vigorous wave regime (Smith et al., 2010) that forms a particularly strong wave ravinement (Pretorius et al., 2018), and when coupled to the narrow shelf widths of the area, the current results in the expression of well-exposed hardgrounds and paleo-landsurfaces from the mid shelf to seaward.
3 Materials and methods
3.1 Archaeological database
The review of archaeological data focusses on MSA and LSA sites in KZN with potential evidence of coastal adaptations, including traces of various behaviors such as the regular consumption and systematic acquisition of marine food resources and the expansion of settlement systems to include coastal and near-coastal zones as occupation spots on a regular and planned basis (Will et al., 2016; Will et al., 2019). The presentation of new and published data consists of two parts: 1) Key MSA and LSA sites of KZN with relevant data on coastal resource use deriving from the published literature and our own recent work. 2) An overview of excavated and known Stone Age sites in KZN in a strip of 20 km along the modern coastline from a large database. The latter is a modified version of the KZN Museum database in Pietermaritzburg which was kindly provided by G. Whitelaw. It contains a systematic collection of archaeological sites and surface collections conducted by researchers since the early 20th century. Much of this data comes from early systematic and extensive surveys of the KZN coast by Oliver Davies (1952, Davies (1970) and members of the KZN Museum reporting on ESA, MSA and LSA stone tools among various beach deposits within a strip of ∼10 km along the coastline. Davies had a profound knowledge of southern African archaeology and he dedicated most of his later years towards a comprehensive investigation of KZN, with a focus on coastal areas. More detailed information on the sites and the sources of their data can be found in SOM Supplementary Table S1. We treated Lake St. Lucia (Isimangaliso Wetland Park) like a coastal area as there is clear evidence for the lake being fully marine at certain points within the time frame of this study, indicated by corals growing on the landward shorelines at Listers Point, False Bay (Cooper et al., 2013; Benallack et al., 2016).
3.2 Material and methods of GIS modelling
We investigated the spatiotemporal variations of the continental shelf during the Late Pleistocene and Holocene with a dynamic GIS model. Our approach is based on bathymetry and paleo sea-level reconstructions and thus aims to 1) simulate displacements of the shoreline, 2) estimate the exposed shelf area, and 3) measure the shore-distances of selected coastal sites at sea level lowstands. The modelled area spans the South African East Coast from 28.1 to 31.2°S covering parts of the KZN South Coast, the Durban Basin and the Zululand Basin (Figure 1). The bathymetric model was extracted from a regional dataset produced by de Wet and Compton (2021) from single-beam echo-sounding, which features a high spatial resolution of ∼290 m and has proven suitable for the study of sea level fluctuations on the continental margin. The temporal extent of the model covers the last 119,000 years and is informed by a global sea level reconstruction by Waelbroeck et al. (2002). The dataset provides a total of 81 timesteps for every 1,500 years in our timeframe with information on relative mean sea level as well as upper and lower margins.
Our model is similar to the approaches presented in Fisher et al. (2010) and Bader et al. (2022) relying on the vectorization of isobaths for relevant time steps and their respective relative sea levels. Due to the high tectonic stability (Erlanger et al., 2012) and negligible effects of glacial isostatic adjustment in the region, we consider the passive flood or “bathtub” approach (Anderson et al., 2018) to be a suitable basis for our calculations. Further, the very limited sediment cover seaward of the 50 m isobath provides a reliable paleo-bathymetric surface on which our model is contextualised. The model was realised in the statistical software “R” v. 4.1.2 (R Core Team 2022) using the geospatial libraries “stars” v.0.5–5 (Pebesma 2021) for raster data (bathymetry) and “sf” v.1.0–7 (Pebesma 2018) for vector data handling. In the first step, we generated paleo-shorelines by deriving isobaths from the bathymetric data, which relate to the relative sea levels in the past, and measured their length. Short isobaths, representing either smaller inland depressions or small islands off the coast, were excluded with a threshold. In a second step, we estimated the Euclidean distances between coastal archaeological sites and the closest segment of the modelled paleo-shorelines with the ‘geosphere’ library v.1.5–14 (Hijmans 2021). Due to the properties of the bathymetric model, relative sea levels higher than 1 m were excluded from this study. As a result, the model computes shoreline distance measurements for each site and each paleo sea level lowstand in the time range between 0 and 119,000 years ago. Since the sea level reconstruction by Waelbroeck et al. (2002) reports uncertainty estimates, these were used to calculate shore distance uncertainty. Figure 2 shows the input sea level reconstruction (A) and the resulting shoreline distances (B) for two example sites, Sibhudu and Umbeli Belli.
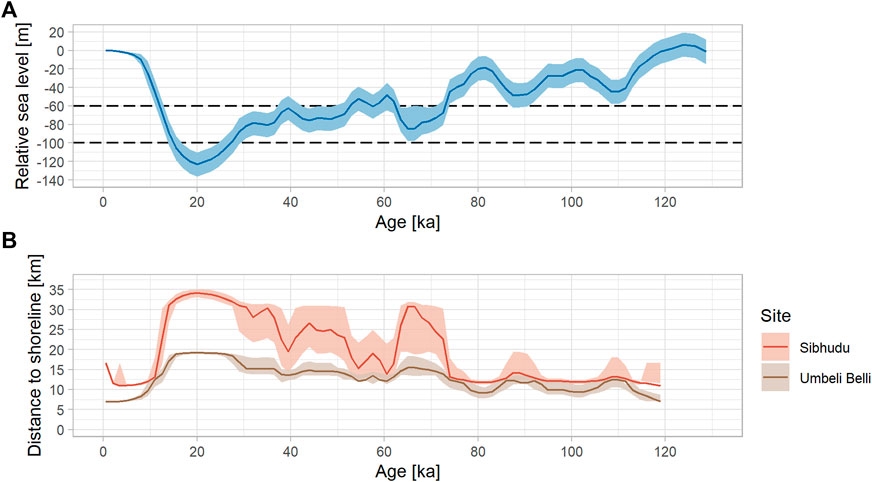
FIGURE 2. Input and output of the GIS model. (A) Sea level curve by Waelbroeck et al. (2002) with error margins. The dashed lines indicate the depths of aeolianite shoreline positions at −60 and −100 m. (B) Shoreline distance variation of two exemplary sites, Sibhudu and Umbeli Belli, in response to sea level lowering. The error margins are derived from the uncertainties of the sea level reconstruction.
3.3 Materials and methods of coastal and offshore geomorphology
The offshore geological and geomorphological data base used in this study stems from data collected using a series of geophysical techniques. Bathymetric data were collected using a WASSP WMB 3250 multibeam sonar system (Green et al., 2018; Pretorius et al., 2019). The positioning was achieved using a Hemisphere VS.330 Real Time Kinematic (RTK) and heading system, with the RTK base correction. Motion correction was applied by a xSens MTi-G motion reference unit mounted at the WMB 3250 transducer. Sound velocity profiles were collected with a Valeport MiniSVP, and the data were tidally corrected using the RTK input. The data were then exported as a 2 × 2 m grid.
Two different types of seismic reflection data are employed in this paper. High-resolution seismic profiles were collected using a 200 J Applied Acoustics boomer, coupled to an 18-element hydrophone array, the data acquisition and processing parameters of which are outlined in Green et al. (2013a). Ultra-high resolution seismic reflection data were collected using a Kongsberg Topas PS18 parametric sub-bottom profiler. Data were collected using a Chirp pulse and match filtered with the Kongsberg SBP utility where transducer motion was also modelled using the inputs from a Kongsberg Seapath 200 Inertial Motion Unit. The final segy data were exported using the secondary low frequency data between 0.5 and 6 kHz, with a resolution of <10 cm. All data were converted from two way travel time to depth using a standard sound velocity of 1500 m/s and 1,600 m/s for water and sediment respectively. Several vibracores were collected to examine the subsurface lithology, the collection and handling of which is outlined in Pretorius et al. (2016). AMS 14C dating on selected deposits representative of palace-land surfaces or processes were carried out, the intricacies of which are further documented in Pretorius et al. (2016; Pretorius et al. (2019).
4 Results
4.1 MSA and LSA archaeology
The few key stratified MSA sites in KZN all derive from rock shelters and encompass Border Cave, Holley Shelter, Sibhudu, Umbeli Belli and Umhlatuzana (Figure 1). None of these sites is closer than 7 km to the current coastline, showing a remarkable absence of excavated localities on the modern Indian Ocean coast. No MSA engagement with marine resources is recorded from Umhlatuzana and Holley Shelter, which are >30 km distant from the modern shoreline. Unfortunately, Umbeli Belli features no organic preservation in the MSA (Bader et al., 2016) potentially erasing any relevant traces, considering that the LSA layers with more favourable taphonomic conditions have yielded shellfish remains (see below). In the following, we first summarise the published work on key MSA and LSA sites on the known evidence, followed by our new data from an exhaustive archaeological database.
The site of Sibhudu (Figure 1) has provided evidence of a low number of edible marine shellfish from a maximum of 8 different species - with the most frequent being Perna perna (MNI=36) coming from its late MSA until pre-Still Bay layers (∼77–48 ka) but also some rare marine animals, fish, crustaceans and turtle (Plug, 2006). In addition, the Still Bay occupations feature a total of eight potential ornamental shell beads with anthropogenic perforation from the marine gastropod Afrolittorina africana and one humanly-modified bead from Nassarius kraussianus deriving from the Late MSA layers, with further marine shells with natural perforations occuring in the Howiesons Poort and pre-Still Bay strata (d’Errico et al., 2008; Vanhaeren et al., 2019). New excavations directed by N. Conard since 2011 have added a small number (NISP=26 specimens >2 cm; plus hundreds of smaller fragments) of unpublished mollusks - both edible and non-edible—from layers spanning the Howiesons Poort until pre-Still Bay occupations (>80–60 ka) that require further zooarchaeological analysis. Combined, the data from Sibhudu indicate long-term engagement with the coasts both for dietary but also non-dietary reasons over the entire MSA occupations and at least during >80–38 ka, even though the distance to the coast during these occupations has fluctuated between 12–38 km to the shoreline. The low quantity of evidence in particular for the consumption of marine foods is likely a function of site-distance to the coast which exceeds the daily foraging radius. At Border Cave (Figure 1), the association of two beads of marine Conus ebraeus shell with the potential burial of a child at ∼74 ka (d'Errico and Backwell, 2016) and the presence of two perforated specimens of Nassarius kraussianus in layers dated to ∼44–42 ka (d’Errico et al., 2012) also indicate the special and planned use of marine resources as ornaments from sites far inland at the time (>50 km). Unfortunately, data on potential marine faunal remains are currently unpublished, precluding further assessment of their dietary use.
Regarding LSA localities, the most prevalent feature is the presence of marine shell ornaments - most often beads - at several inland sites in KZN. These beads (n=78) occur in variable frequency at a minimum of 11 LSA sites that span the time period ∼13.000–300 uncal. BP (Mitchell, 1996). All of these sites are >20 km away from the present coastline, some up to 180 km. While a variety of marine gastropod species were used for ornamental purposes, Nassarius kraussianus accounts for >90% of all specimens (Mitchell, 1996). In general, there are few excavated stratified shell middens or localities of associated shellfish and LSA stone tools. Among the rare published stratified LSA sites along the modern coastline, Davies and MacDonald (1978) report on a shell midden with frequent mussels but also oysters found together with “Smithfield” stone tools dated to ∼500 uncal. BP at Umhlanga Rocks on the modern coastline. About 7 km away from the Indian Ocean, shellfish exploitation for consumption is also evidenced in the LSA layers at Umbeli Belli (1,140±50 and 200±50 uncal. BP; Figure 1) dominated by Perna perna with >95% (Cable, 1984). Most interestingly, Shongweni (Davies, 1975), currently 30 km away from the coast, features shells of Perna Perna in LSA occupations between 4,000–1,000 uncal. BP which are most likely associated with dietary use.
Instead of relying on a biased picture from rock shelters alone, human presence during the Stone Age can be traced on a much broader scale by including open air sites and surface scatters. Our review of archaeological sites from an exhaustive database led to the identification of 228 coastal localities providing evidence for human activities during the MSA and LSA (see SOM Supplementary Table S1). Figure 1 shows the distribution of these sites. In contradiction to our expectations, MSA sites are more common than LSA sites (114 pure MSA sites and 29 MSA/LSA sites vs. 85 pure LSA sites). This seems counterintuitive, considering the large shell middens typically associated with late LSA occupants elsewhere in southern Africa. Furthermore, MSA sites also appear to be more widely distributed along most of the KZN coast, whereas LSA sites are more restricted to the southern part of the province. Since the overall majority of the data from our database derives from Oliver Davies’ work it is hard to imagine that the LSA was simply ‘overlooked’ in the northern areas. In a recent study, Bader et al. (2022) also found comparatively little evidence for late Pleistocene/early Holocene LSA sites in this area, where substantial gaps in occupation after the end of the MSA (∼28.000 cal BP) before the LSA occur, sometimes lasting more than 20.000 years. In sum, the database (SOM Supplementary Table S1) and Figure 1 provide clear evidence that the (modern) coastal area of KZN was frequently visited during both the MSA and the LSA. The data suggest that the majority of the original coastal occupations during the Stone Age may lie beyond this modern border but are currently submerged offshore.
4.2 Coastal distance modelling
4.2.1 Shelf exposure
We used the GIS model informed with sea level fluctuations to translate the bathymetric data into a map showing the temporal extent of the shelf exposure during sea level lowstands during the last 119,000 years (see Figure 3). According to the sea level curve, the maximum sea level lowstand at 123 m r.m.s.l was reached around 20,000 years ago, which corresponds to the greatest exposed area confined by the steep shelf edge (0 ka isochrone). The geography of the uncovered shelf is dictated by its geological setting. The shelf south of Durban and north of Richards Bay is steep and narrow, so that isochrones trend in narrow and coast-parallel fashion, indicating minor coastline displacements by time increments. The situation is more complex in the Natal Bight between Durban and Richards Bay, with a shallow northern and a deeper southern shelf. The 60 ka isochrones serve as a good indicator, showing that substantial areas of the northern shelf sector, with a shoreline partly (>30 km) displaced from the modern one, were subaerially exposed during 50% of the timeframe investigated. In the southern part of the Natal Bight, larger areas were only uncovered during deeper relative sea levels, but still account for one third of the studied timeframe (40 ka isochrone). Areas with long exposure times (80–100 ka isochrones) are the Aliwal Shoal north of Scottburgh, the Durban Bay and especially the northern Natal Bight in the area offshore the uMlalazi River/Port Durnford.
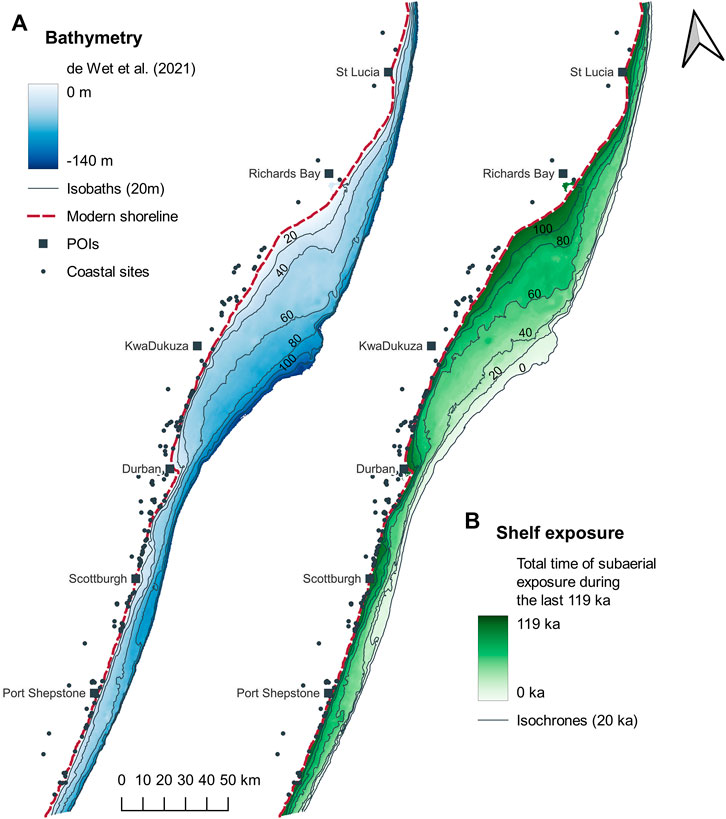
FIGURE 3. How long has the shelf been exposed during the last 119,000? (A) Bathymetric model by de Wet and Compton (2021) shows the modern-day water depths in the study area between 28.1–31.2° S in the sea level affected zone between 0 and 140 m. The result of the GIS model (B) shows the cumulative exposure time of the affected areas. The colours and isochrones indicate how long an area has been uncovered in total during the timeframe between 0–119,000 years ago. Note that the exposure time can be contiguous or the sum of multiple exposures, due to sea level fluctuations derived from the mean sea level curve by Waelbroeck et al. (2002). The isochrones do not indicate absolute dates of paleo-shorelines.
4.2.2 Shoreline distance of MSA and LSA coastal sites
The sea level fluctuations since the Eemian (MIS 5e) have caused considerable displacements of the shoreline. Here we present results of our GIS simulation, showing how these variations have affected the shoreline distance of MSA/LSA coastal sites from our database and thus, the potential access to marine resources during these times. Since many of the sites are surface finds that lack radiometric dates, we could not reconstruct the situation at the time of deposition. However, we were able to define broad geographic regions along the KZN coast based on the variability and timing of their response to sea level fluctuations. We analysed 172 archaeological sites situated within 15 km from the modern shoreline and subdivided these into four regions following our results of the shelf exposure model (see above). These regions include the ‘North Coast’ north of 28.8°S (n=5 sites), the ‘Northern Natal Bight’ between 28.8°–29.3°S (n=19 sites), the ‘Southern Natal Bight’ between 29.3°–29.8°S (n=45 sites) and the ‘South Coast’ south of 29.8°S (n=103 sites). Our results are presented in Figure 4 and show that sites on the North and South Coast lay predominantly within the typical maximum foraging distance of 10 km to the shoreline over the last 119 ka. Even during the LGM (123 m, 20 ka) the average coastal distance of these sites was only ∼14 km (South Coast) to ∼18 km (North Coast) from potential marine resources. Much higher variability is found on the coast of the Natal Bight. In the southern part the average shoreline is only within daily foraging range prior to 72 ka, during a short window around 60 ka, and then again after 12.5 ka. During the LGM, the average site is 24 km from the coast with a maximum of 38 km. In the Northern Natal Bight, the sites are only occasionally situated within foraging distance in the timespan before 77 ka and after 11 ka. During the period between, no known site is in foraging distance and the average distance during the LGM reaches >40 km.
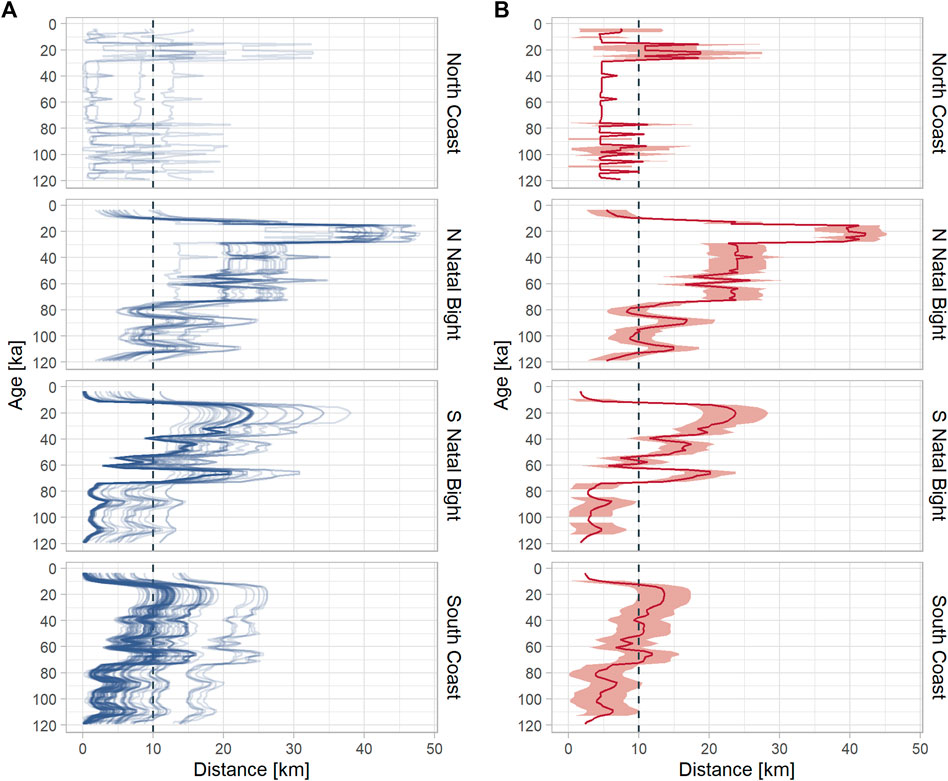
FIGURE 4. Variation of the distance of archaeological sites to the coast over time. (A) Coastal distances for 172 archaeological sites from the North Coast (n=5), Northern Natal Bight (n=19), Southern Natal Bight (n=45), and South Coast (n=103). Light blue lines show the time series of single sites and dark blue lines indicate overlapping of multiple time series. (B) Temporal aggregates of the time series show the mean (dark red line) and one standard deviation (bright red ribbon) coastal distances. The dashed lines at 10 km in (A) and (B) mark the upper boundary of daily foraging range of modern Hunter-Gatherer groups.
4.3 Offshore geology and geomorphology of the KZN shelf: a review and new interpretations
Using a broad array of geophysical data, we examine the morphology and stratigraphy of the shelf offshore the KwaZulu-Natal coast (Figure 5). We focus here on sites that occur adjacent to the MSA occupations of the coast and hinterland, from the Mzimkulu River in the south to KwaDukuza in the north (Figure 5). Between these locations, the shelf widens from 12 km to nearly 30 km offshore the important MSA site of Sibhudu, and houses a variety of submerged landscapes that formed when sea levels were lower than present.
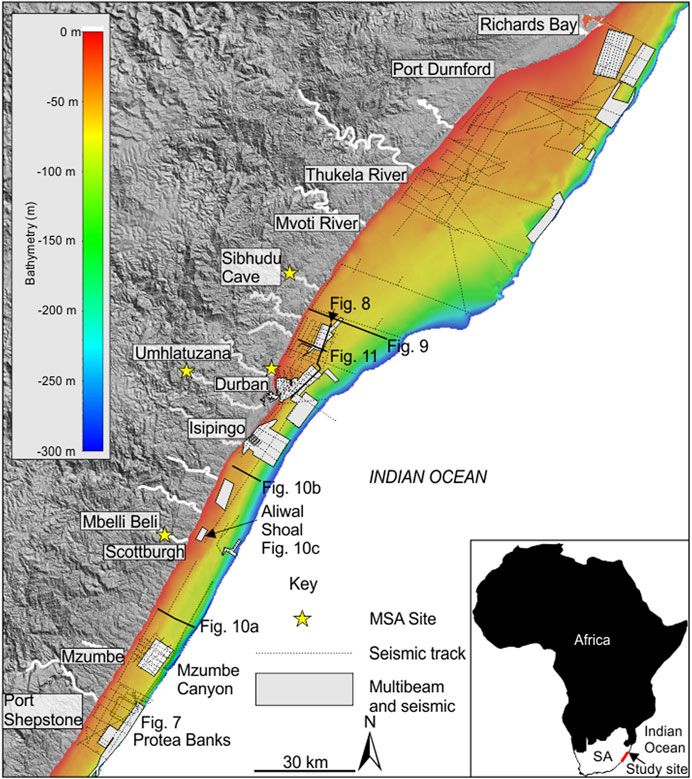
FIGURE 5. Geophysical data coverage of the KwaZulu-Natal shelf, superimposed on the general shelf bathymetry (after de Wet and Compton, 2021). Key data localities discussed in the paper are highlighted in bold.
4.3.1 Paleo-fluvial courses
Many of the rivers observed on the modern coast can be traced seaward of their current mouths where they were incised during the penultimate glacial maximum (MIS6), and the last glacial maximum (LGM) lowstands. During these periods, much of the shelf was exposed to subaerial processes producing a flat coastal plain interspersed with multiple river valleys that terminated at the shelf break where sea level resided (Figures 6, 7). The intervening periods, notably from MIS4 to MIS2 saw the shoreline placed at a minimum of >60 m lower than present, with these rivers forming a series of estuaries and lagoons along the coast in response to higher sea levels drowning the seaward edges of the river valleys. Pretorius et al. (2019) reveal a series of river courses of the Mzimkulu River, some of which are still exposed on the shelf, that were generated during MIS6 and the LGM which have been infilled by estuarine materials (Figure 7). Several key seabed features are: 1) a paleo-lagoon located at a depth of ∼85 m and 2) rivers draining into the head of a submarine canyon to form a coastline analogous to the modern one at Waterfall Bluff, ∼ 95 km to the southwest.
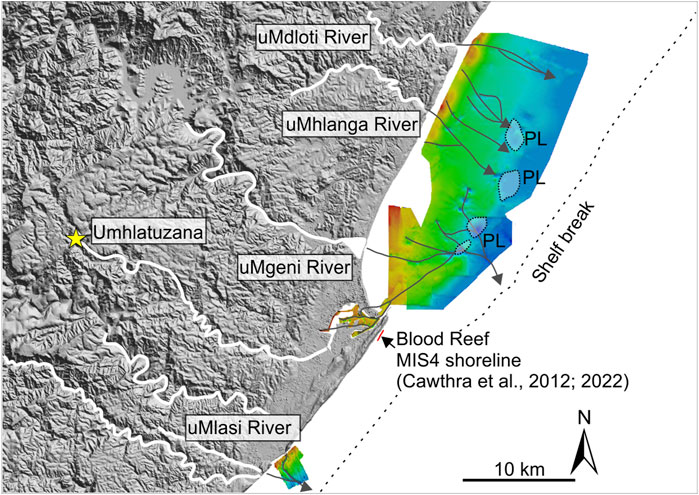
FIGURE 6. Bedrock surface offshore the greater Durban area based on available seismic data. The surface represents several periods of amalgamated subaerial exposure, reflected in the drainage patterns. PL = paleo-lagoon. The MIS 4 shoreline of Cawthra et al. (2012, 2022) is highlighted. Suspected drainage.
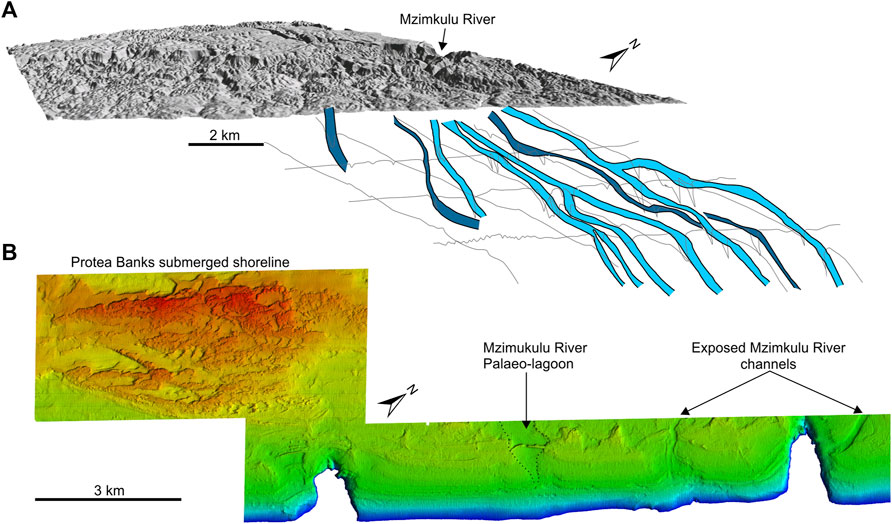
FIGURE 7. (A) 3D representation of the drainage pattern offshore the Mzimkulu River, just to the north of Mpondoland. Dark blue represents an older subaerial channel network, likely MIS 6, the lighter blue represents the LGM drainage system (after Pretorius et al., 2019). (B) Multibeam bathymetry of the Protea Banks shelf immediately offshore the Mzimkulu River. Note the development of a lagoon, with several LGM channels still exposed at the seabed, one of which entering a submarine canyon and likely forming a waterfall akin to the Waterfall Bluff site of Mpondoland.
To the south of Durban and offshore the Isipingo and paleo-Umlasi River mouths, a well-developed meandering river system is evident from seismic reflection data (Figure 6). These river valleys occur two valleys to the south of the Umhlatuzana Rock shelter. The paleo-Umhlatuzana River itself has formed a prominent incised valley in the Durban Bay, where several other smaller rivers merged and exited out to the shelf to combine with a series of incised valleys related to the Mgeni River (Figure 6). These incised valleys and adjoining subaerial paleo-landscape are marked by circular depressions that occur in the subsurface at elevations of between −60 and −70 m (Figure 6). When viewed in profile, these depressions reflect broad topographic lows associated with an older generation of incised valleys, and now filled by a set of reflections that prograde in from the depression margins (Figure 8). Cores reveal this infilling material to comprise a stiff lagoonal clay dated to 39,636 ± 592 cal. BP. This lagoonal sequence is in turn truncated in various parts by LGM-age incised valleys (Pretorius et al., 2016) and overlain by Holocene age aeolianite and beachrock, the surface outcrop of which marks a second lagoon now preserved on the shelf over the MIS 3 example (Green et al., 2013b) (Figure 8).
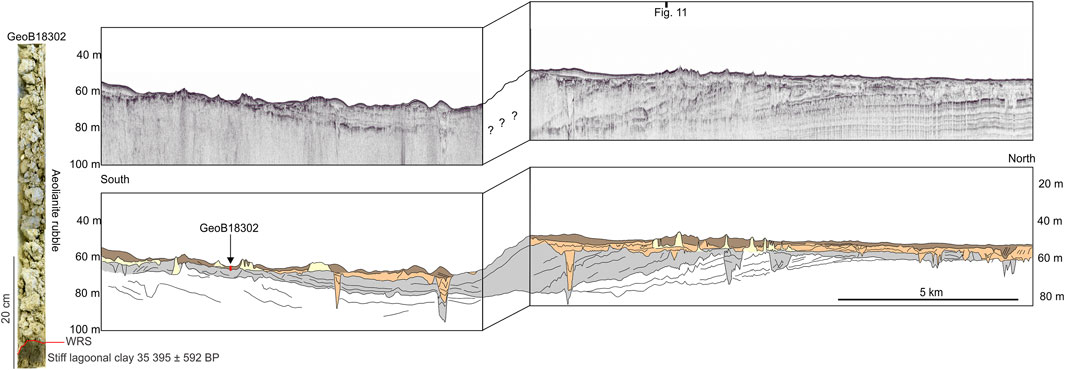
FIGURE 8. Interpreted (top) and raw (bottom) seismic reflection data offshore Durban. The data highlight a series of incisions into paleo-land surfaces. The base of the grey represents an older MIS 3 lagoonal depression (penetrated by GeoB18302-left), which was re-incised by younger LGM channels that later formed younger, Holocene-age lagoons above the older MIS 3 materials.
Across KZN, a similar pattern emerges. In Richards Bay, radiocarbon dates of 39,100 and 45,200 14C yr B.P. (Pta-4142; Pta-4140) from wetland peats (at depths of 46 and 52 m) within a back-barrier sequence provide a maximum indication of lower than present sea levels at that time (R.R. Maud, personal communication, 2000). In the Umlaas canal in Durban, a date of >48,000 14C yr B.P.was obtained from peats and diatomites from -35 m amsl (R.R. Maud, personal communication, 2000) and in Durban Bay, King (1972) reported an age of 24,950 14C yr B.P. (GaK-1390) for a peat at -22 m amsl.
Directly offshore of Sibhudu, seismic profiles reveal a series of compound incised valleys occurring to landward of a subcropping submerged shoreline at between depths of 60 and 70 m (Figure 9). The truncations of each paleo-subaerial surface reveal up to four generations of incision, with subsidiary smaller channels stacked within the associated fills. These smaller channels resemble the tidal channels found in back-barrier estuarine systems of the modern large lagoons of SE Africa (Benallack et al., 2016; Dladla et al., 2019). We view these as a series of incised valleys associated with a back-barrier/estuary/lagoon environment, fronted by a submerged barrier shoreline. Their compound nature implies multiple shoreline occupation at that depth over successive sea level cycles, including the most recent lowstand of the LGM and the lowstands of MIS 12 and 6 too.
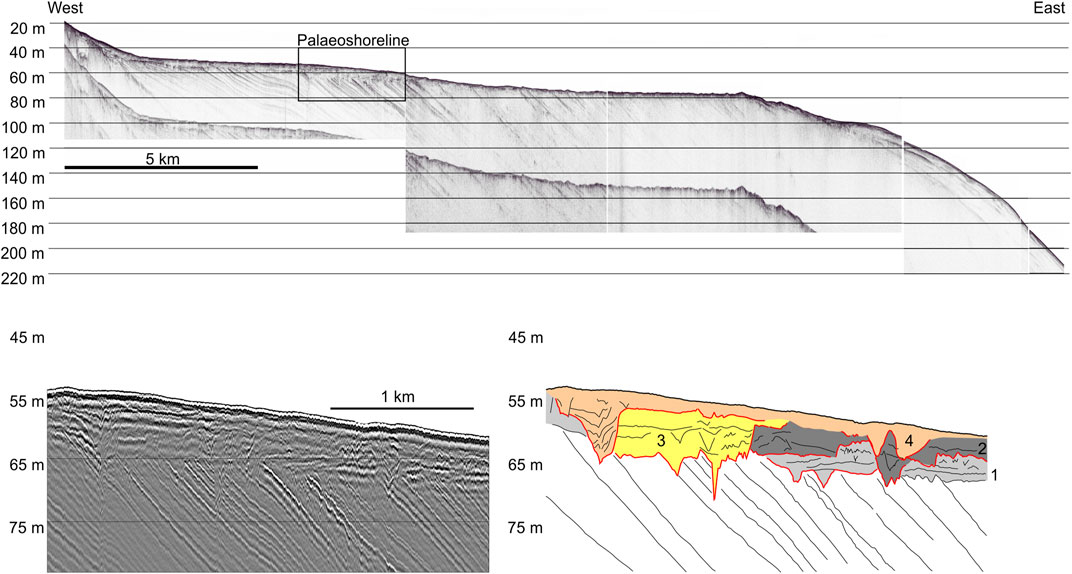
FIGURE 9. Seismic reflection profile offshore Sibhudu Cave. The below interpretation highlights at least four phases of channel incision and lagoon development behind an aeolianite to seaward, spanning a possible MIS 12 to MIS 2 age range.
4.3.2 Coastal aeolianite and beachrock shorelines
Both regional and focused bathymetric and seismic reflection surveys reveal a nearly continuous set of submerged shorelines at depths of 20–30, 60–70 and 90–100 m on the shelf. Offshore the Mzimkulu River, the Protea Banks comprises a large upstanding block of aeolianite, with several small coastal spits and barriers found to seaward of the feature at a depth of 100 m (Pretorius et al., 2019) (Figure 8). Offshore the Mtwalume River, seismic profiles show a prominent aeolianite pinnacle with a basal depth of ∼65 m, fronted to seaward by a flat erosional platform (Figure 10). To landward of the pinnacle, a series of low amplitude, draped reflections onlap the pinnacle and are truncated by a series of small (3 m-deep and 20 m-wide) channels marked by higher acoustic reflection. Likewise, off Amanzimtoti, a similar arrangement is observed, with a well-developed aeolianite onlapped to landward by a prominent incised valley fill.
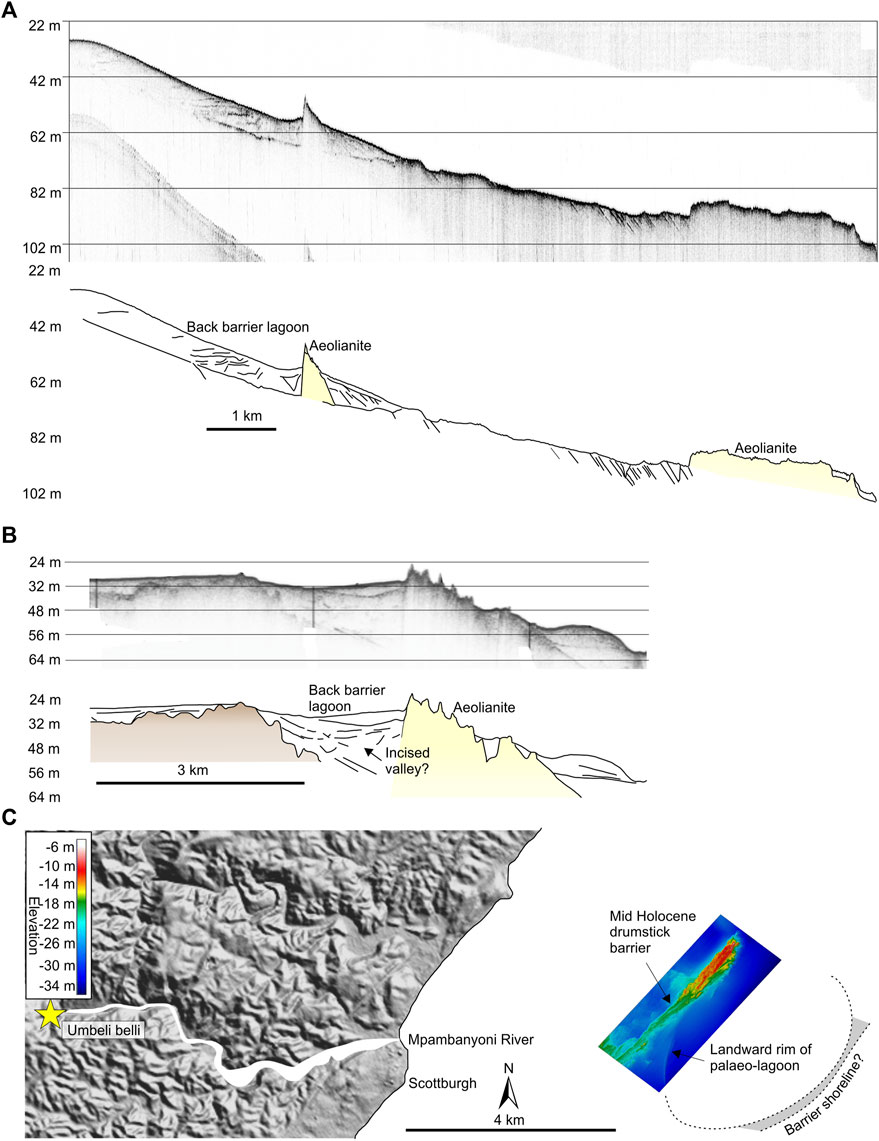
FIGURE 10. (A) Seismic reflection profile from the KZN south coast offshore Mtwalume. Note the two clear phases of aeolianite development at -100 m and 60 m. There is a prominent back barrier lagoon complex housed behind the 60 m shoreline. (B) Seismic reflection profile offshore the Amanzimtoti area. Note the similar seismic architecture to (A) Data adapted from Gribble (2021). (C) Multibeam bathymetry of the Aliwal Shoal, juxtaposed with the location of Umbeli Belli. The shoal forms a drumstick barrier, fringed to seaward by an arcuate ridge that we interpret as the landward rim of a paleo-lagoon.
Bosman (2012) examined a well-developed aeolianite offshore Scottburgh, the Aliwal Shoal, and using OSL dating, placed an early to mid-Holocene age on its development. However, more detailed multibeam mapping shows that the Shoal forms a drumstick barrier that overlies an older arcuate ridge of aeolianite to seaward that is formed at a depth of 30–34 m (Figure 10). These arcuate ridges are commonly associated with paleo-lagoons, forming semi-circular depressions on the seabed bounded by aeolianite and beachrock spits (Green et al., 2013b). This suspected paleo-lagoon occurs 5 km seaward of the Mpambanyoni River, on which the Umbeli Belli site is located, ∼ 12 km directly landward of the Aliwal Shoal.
Offshore Durban, between Umhlanga and Umdloti, 19 km southeast of Sibhudu, a well-developed rocky shore platform has been mapped for almost 9 km along coastal strike at a depth of 60 m (Figure 11). Seismic reflection profiles reveal this to be the surface expression of an eroded set of prograding moderate amplitude reflections that are distinct from, and located adjacent to, a prominent aeolianite with a basal depth of 60 m. These are likely remnants of an old rocky platform related to debris collapse of a fringing aeolianite (Green et al., 2018). The upstanding aeolianite block overlies the older MIS 3 and Late Pleistocene incised valleys that comprise the same paleo-lagoon complex shown in Figure 7.
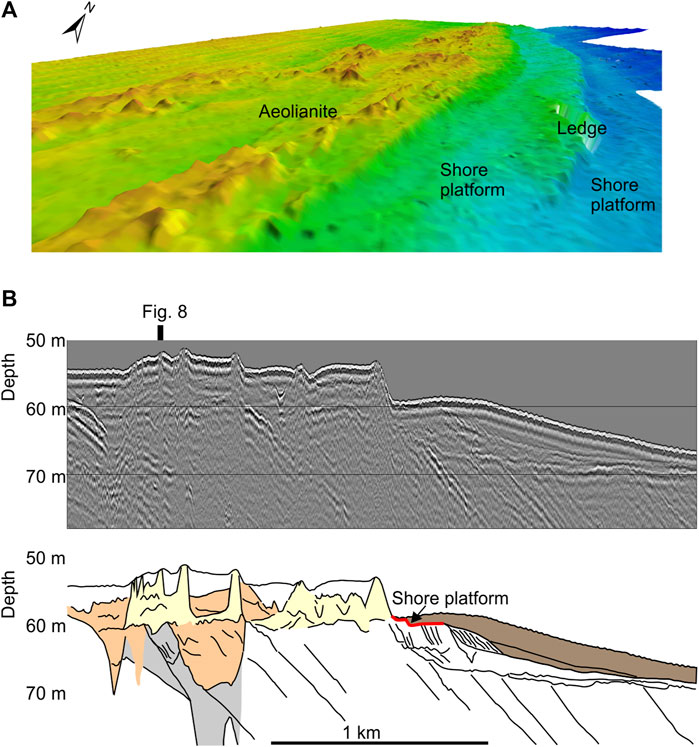
FIGURE 11. (A) 3D multibeam representation of the seabed offshore uMhlanga/uMdloti. Note the landward aeolianite with two shore platforms benched into the rock surface, denoting a rocky shoreline occupation at 60 m (B). Interpreted (top) and uninterpreted (bottom) seismic reflection profiles through the above aeolianite. Note the underlying incised valley, the back barrier system to landward of the aeolianite, and the bench to seaward of the aeolianite, into the fringing material at 60 m.
For almost the entire 160 km length of this study, the same trend emerges where shorelines at ∼60–70 m water depth comprise upstanding blocks of aeolianite fronting an onlapping sequence of lower amplitude reflections that fill an incised valley. We consider these sequences to represent back-barrier lagoonal environments, with small tidal channels that formed behind a substantial coastal dune cordon that had developed on the shelf (cf. Benallack et al., 2016; Dladla et al., 2019; Green et al., 2022b). The shoreline ages are less-well constrained outside of Durban, though it is clear that the shorelines represent multiple phases of shoreline occupation. The majority of work has placed these as Holocene in age, though undoubtedly, the previous phases of shoreline occupation, especially the paleo-lagoons, appear to relate to MIS 3. The barriers may correspond with a stilstand at -60 m at ∼55 ka. According to the core and age data of the submerged lagoons, their presence implies a prolonged period of sea-level stability up to ∼39 ka (Pretorius et al., 2016). In addition, lagoonal remnants crop out as far north as the Thukela River shelf (Green and MacKay, 2016), where they were dated at 40,650 uncal. BP (Flemming, 1978), to which we correlate these deposits.
5 Discussion
5.1 Coastal adaptations in KZN during the Pleistocene and Holocene?
In contrast to the Western and Eastern Cape, little is known about the habitation and use of coastal ecosystems in KZN by modern humans during the Late Pleistocene and Holocene. Here we provide the first systematic review of potential coastal adaptations during the MSA and LSA of the eastern Indian Ocean coast. A review of published sites shows important but rare and mostly low-density evidence through the MSA. Key data regarding dietary use comes from Sibhudu where transport and consumption of marine shell (particularly Perna Perna) but also low numbers of mammals, fish and crustaceans, is apparent. Both Sibhudu and Border Cave feature shell beads, showing that MSA people procured shells from the beaches, wore them as ornaments and left them at the site, sometimes even as potential grave goods. This evidence spans roughly >80–40 ka, suggesting a long-term engagement with coastal and marine ecosystems. Importantly, both sites were up to 30 km (Sibhudu) or >60 km distant during the relevant times as shown in this study. Based on a large database of Stone Age sites in KZN, our study demonstrates that many open-air scatters of MSA stone tools occur along most of the modern coastline, hinting at a much longer and more intense settlement along the shores as currently known from stratified inland Pleistocene sites.
During the LSA, there are few to no shell middens properly excavated and published from KZN, and the ones that do exist all date to the Holocene. This being said, consumption of marine resources - mostly Perna perna - is well-evidenced from sites such as Umbeli Belli and Shongweni within the last 4,000 years. The archaeological database of the region provides evidence for more LSA occupations on the modern shore clustered around the southern part of the province, though interestingly less compared to the MSA. Our shoreline reconstruction over the past 119.000 years has shown that the coast in southern KZN has not dramatically changed during the late Pleistocene and Holocene providing a possible explanation for a multitude of both MSA and LSA occupations along the modern day shoreline. In the northern part of KZN though, shoreline extension was more variable, especially during LSA times (<28.000 cal. BP) during which the coast would have mostly been more than 10 km away from the modern one and thus outside the typical daily foraging radius. The most recurrent pattern of LSA people engaging with coastal resources comes from nearly a dozen inland sites, up to 180 km from the modern shoreline, with evidence for marine shell beads spanning ∼13.000–300 uncal. BP. The consistent and predominant use of Perna perna for food and Nassarius krassanius for beads during both the MSA and LSA shows remarkable consistency in dietary and non-dietary choices across the last ∼80.000 years in the region.
Summing up these observations, KZN has a deep-time record of the use of marine resources and the occupation of coastal landscapes in the Late Pleistocene and Holocene, but one which currently differs in the quantity, quality and diversity from the southern Indian Ocean and Atlantic Ocean coast. In the latter regions, over 10 MSA and dozens of LSA sites indicative of systematic coastal adaptations are known starting already at ∼120 ka, shell middens occur both in the MSA and frequently in the LSA (also as so-called “megamiddens”) and sites are often associated with a wide array of consumed marine mammals, birds and fish from intense occupations directly on the coasts (e.g., Jerardino, 2010; Will et al., 2016). How can we explain these different patterns in the archaeological record? We briefly discuss two potential explanations, though others are conceivable: 1) Behavioral differences based on differences in marine ecology; 2) Research bias due to issues of site preservation, intensity of research and differences in (marine) geology.
Regarding the first hypothesis, the Western Cape and KZN differ strongly in their coastal and marine ecology. Along the Atlantic coast, the Benguela Upwelling system creates highly nutrient-rich waters that sustain a productive marine ecosystem. In contrast, the Indian Ocean coast in KZN is mesotrophic with lower productivity but higher species diversity, driven in part by upwelling along the shelf edge associated with the oligotrophic western boundary of the warmer waters transported by Agulhas Current (De Lecea et al., 2013; Kyriacou et al., 2021). Variable shelf widths and the flow of the Agulhas Current may have changed the nutrient status of the KZN marine ecosystem, though it seems that during lowstands, the Agulhas Current would have been situated close inshore to the shelf edge or paleo-coastline (e.g. Green et al., 2022a) and oligotrophic conditions may have been dominant. The Indian Ocean coast of KZN with its lower marine biomass and different variety of marine food resources might have thus been either unsustainable for intense coastal exploitation, or people in the MSA were not able to efficiently extract resources from such an environment. Modern ethnographic data on coastal foraging in KZN by local subsistence shellfish gatherers show, however, that collecting large amounts of diverse shellfish and crab species is possible (Kyle et al., 1997a; Kyle et al., 1997b). Interestingly, well-defined, stratified Iron Age middens with large amounts of shell (particularly Perna Perna) such as Mpambanyoni (Robey, 1980), Emberton Way (Horwitz et al., 1991), Umhlanga Rocks (Beater and Maud, 1963) or several midden sites around Durban Bluff (Schoute-Vanneck, 1958) also demonstrate the viability of extensive coastal adaptations and extracting large amounts of edible seafood from the Indian Ocean, at least in more recent times by more sedentary societies. Whether MSA and LSA people used it in a comparable manner thus becomes a question requiring further research instead of excluding the option a priori.
Modern ethnographic studies of shellfish exploitation in the nearby Transkei and other regions (Bigalke, 1973; Siegfried et al., 1985; Hockey et al., 1988), have generally found that people living up to 5 km from the sea regularly exploited shellfish while those living 10 km away visit the coast less frequently, possibly on a monthly basis. These visits often coincided with favorable tides, and required transport when available. No sheltered MSA and LSA sites—generally being good sediment traps allowing the accumulation of a longer record and with faunal remains—occurs closer than 7 km to the current shoreline. From ethnographic observations we would expect few marine remains to be transported to these sites, and this matches the archaeological record. On the shore itself there are few to no sheltered localities, with open-air deposits rarely allowing in situ archaeological remains to accumulate and persist. In the Western Cape, almost all sites with good evidence for MSA coastal adaptations come from caves or rock shelters within 10 km from the modern shoreline—such as Klasies River, Blombos or Pinnacle Point - though rare open-air sites exist as well (e.g. Will et al., 2013). Many of the differences between the western and eastern seaboard might be attributable to these differences in relevant sediment traps. Even worse, changes in Pleistocene and Holocene sea-levels along the KZN coastline as modelled here, pushing the shore often 10–40 km from its current location, might have erased or inundated the evidence of open-air deposits on past shorelines. In sum, our key MSA and LSA sites are too far away to record the regular use of marine resources (see also Kyriacou, 2017). More archaeological exploration within a 10 km radius from the shore is needed, especially where the offshore record has identified possible paleo-landscapes that may have provided suitable shelter and/or resources. The lack of caves, rock shelters or sheltered areas on or close to the shoreline and the erosion or flooding of open-air deposits, however, might even require completely new research strategies that we discuss in the next section.
The points made above can be further supported by the example of a recently successful research project on coastal adaptations at the site of Waterfall Bluff along the Indian Ocean coast in Pondoland and only ∼50 km south-west from the KZN border (Esteban et al., 2020; Fisher et al., 2020). Here, excavations at a rockshelter site directly on the modern shoreline below a waterfall created by the run-off of the Mlamobomkulu River into the Indian Ocean has provided ample evidence for coastal adaptations between 36–10 ka. The evidence encompasses not only the systematic collection of marine shellfish and fish but also marine shell beads. The authors ascribe the presence of these archaeological remains, usually scarce in the region, to the narrow continental shelf, with the site thus being close to the ocean during the Late Pleistocene and early Holocene even when sea levels changed. This is likely an important determinant, and given the narrow shelf widths in KZN, such as south of Durban or north of Richards Bay, these too would have been within the ca. 10 km daily foraging radius. The submerged landscapes we discuss below, especially offshore these locations, are thus an especially important future exploration target.
In conclusion, archaeological data and contextual observations suggest that the current evidence on the use of marine resources and coastal landscapes during the MSA and LSA in KZN is more likely the result of a complex research and geological bias instead of an inability of Stone Age hunter-gatherers to exploit these resources efficiently in this specific environment: Sheltered sites with well-known records occur mostly >10 km from the modern coasts where sparse transport of dietary marine resources can be expected, little archaeological fieldwork has been carried out directly on the coasts and much of the evidence that might have once been present would have been erased by changes in sea-level. However, since our archaeological review showed that there is a large untapped potential, the next section aims to highlight how best to exploit this by combining archaeological knowledge with our results on the changing coastline’s position and nature over various sea level cycles.
5.2 Combining archaeology and coastal geomorphology: Pathways to future research
The most fruitful way to further the exploration of potential coastal adaptations during the Stone Age in KZN consists of combining existing archaeological data with results on the GIS modelling of paleo-shorelines and offshore marine geology and geomorphology. Based on the results of the GIS model, the most interesting areas for finding MSA/LSA archaeology on land should be south of Durban and north of Richard’s Bay, both areas of which feature little displacement of the shorelines during the last 120 ka and mostly within <10 km. We especially highlight the Aliwal Shoal region offshore Umbeli Belli, where a proposed lagoon has been identified ∼12 km from the site. Interestingly, this area matches with some of the (scant) excavated, and much of the surface, MSA and LSA archaeological evidence available on land. This is always frequent in the southern part of KZN. Likewise, the series of lagoons and estuaries evident from the seismic record offshore Sibhudu (Figure 9) point to a potential submerged resource in existence since at least MIS 12. Cawthra et al. (2022) recently highlighted an MIS 4 shoreline offshore the Durban coast in shallow waters, and link this to the Sibhudu, providing an interesting comparison of wind conditions and climate for the MSA settlement period that can be done for almost all the sites mentioned here.
Conversely, offshore exploration would be particularly interesting in the wider shelf of the Natal Bight, with large exposures of land now submerged up to 60 km from the modern shoreline. Here, observations on offshore marine geology and geomorphology are a key guide for future research. In many of the submerged shorelines, there is strong potential for cave and rockshelter features to form: Overhangs, notches and caves are common in the submerged beachrocks and aeolianiates of the SE African margin (Green and Uken, 2005; Bosman, 2012), and may have provided similar environments as the Pinnacle Point caves of the southern Cape coast and offshore (Cawthra et al., 2018; 2020). Notable examples include offshore the Thukela River and throughout the Aliwal Shoal, where the foresets of aeolianites weather to form overhangs up to 2 m high and several meters wide (Bosman, 2012; Engelbrecht et al., 2020). Based on their relative stability and ages (from the Holocene to at least MIS 3), the now submerged paleo-shorelines and lagoons around modern-day Durban appear to be further attractive possibilities for uncovering submerged MSA and LSA occupations. Coastal lagoons are productive and biodiversity-rich marine ecosystems, featuring diverse and rich communities of marine fauna, and they provide easy access in their generally shallow (<2 m) water depths (Alvarez-Borrego, 1994; Kjerfve, 1994). Present-day Lake St. Lucia may be seen as a close analogue to these submerged ecosystems in the Late Pleistocene. Our modelling shows the once open marine shorelines of the False Bay region were within 10 km of the closest MSA site, a situation we consider analogous to the submerged environments offshore Durban.
The submerged coastal lagoons along the KZN coast are also relatively close to important MSA and LSA sites on the coast of Durban, such as Sibhudu, Umbeli Belli and Umhlanga Rocks, each of which have good evidence for longer-term occupation and the active use of shellfish and other marine resources. The along-coast shoreline variability of these submerged lagoons should be noted. Offshore Umhlanga Rocks, these transform into paleo-rocky shore coastlines with cliffs and wave-cut platforms, the modern equivalents of which are host to abundant marine shellfish such as the oyster Crassostrea sp, barnacles such as Belanus sp., and the bivalve Perna perna (Cooper and Green, 2016). In addition, fluvial systems were an important attractor for human settlements during the Pleistocene, providing drinking water and access to other animal resources. Many rivers on the modern coast, such as the Isipingo, Mgeni, Mzimkulu and Umlasi Rivers, can be traced offshore via seismic reflection data. These are well-incised due to the overall narrow shelf and shallow shelf break, which was exposed repeatedly during the lowstands of the Pleistocene (Green et al., 2013a). Their paleo-courses, in addition to the other major seaward expressions of KZN’s paleo-drainage, present another attractive target for submerged archeological exploration.
Based on these observations and the foregoing discussion we propose several pathways for further research. In terms of archaeological exploration, the different geomorphic landforms show different likelihood for past settlements, suitability as sediment traps and are associated with certain preservation conditions. The latter relate to lagoon and coastal dune features that represent sediment-abundant conditions, though the two vary in their respective energy regimes. The tranquil back barrier shorelines of the submerged lagoons would seemingly be an attractive area to explore, given the high degree of possible preservation potential of sediment and archaeological archives. Relict rocky coast paleo-shorelines may provide good shelter and foraging potential, however these need to form and then be stranded by falling sea levels so that the shelter potential is not quickly submerged, and the ensuing occupation record remains preserved from marine erosion. Some of the aeolanites offshore the Sibhudu area are important in this regard (Engelbrecht et al., 2020). Other landforms such as present and paleo-river valleys and mouths constitute another primary target for identifying potential Stone Age settlements along the coasts.
Increasingly detailed and high resolution geomorphological mapping, on- and off-shore, may help to identify new sites or prevent surveying archaeological badlands. We in particular identify two regions such as the South Coast and the southern Natal Bight as relevant on-shore and the submerged lagoons, offshore the Protea Banks, Aliwal Shoal and Durban shelf, as relevant off-shore areas for new and better informed explorations. On-shore explorations will be comparatively easy to carry out via foot surveys, informed by local geomorphology and assisted by drones followed by dedicated excavation of documented in situ archaeology or other human traces such as footprints. Similar approaches have shown to be feasible in other coastal areas of South Africa (e.g. Parkington et al., 1988; Morrissey et al., 2020) and recently implemented by us in other areas of KZN. In contrast, off-shore work will be much more demanding in terms of logistics and costs. As a first step, we suggest the initial survey of the identified high-potential areas, using a combined multibeam and side scan sonar approach for underwater detection of lithics wherever possible (see Grøn et al., 2021; Morris et al., 2022). Side scan sonar, with its superior resolution, is especially important to detect lithic artefacts if exposed on seafloors. Given the sediment-denuded character of much of the mid to outer shelf of KZN, this is an especially attractive option. Additional high resolution to ultra-high resolution Chirp seismic reflection data should also be collected to provide stratigraphic context to the ensuing sampling campaign. Small-scale suction dredging for potential stone tools from recovered sediments and localised small core-drillings to identify archaeological traces should follow in this regard. More systematic core drilling over larger areas via research vessels and dedicated underwater archaeology by scuba diver survey and excavation (e.g. Bailey et al., 2015; Bailey et al., 2017; Flemming, 2017; Bailey et al., 2020) on identified Stone Age habitation areas would be a final, much more elaborate step in this exploration. The great potential of KZN offshore areas to yield evidence for Stone Age archaeology associated with early coastal adaptations justifies such an approach in the future.
Other ways to further our current knowledge lie in the interpretation of geomorphological maps as ‘resource maps’, showing past resource spaces with a focus on relevant ecological attributes (e.g. rocky vs. sandy shores; molluscs and sea turtles from marine vs. hippos from estuarine environments), informed by archaeological data. Concerning basic archaeological studies, more detailed analyses are required of both old and new faunal collections from MSA and LSA sites in KZN with a focus on the marine components to assess how far the harvesting of these resources was systematic and optimised (see e.g. Langejans et al., 2012). Re-analysing these assemblages might also uncover more evidence for non-dietary use of shells, with many of the MSA shell beads at Sibhudu only found by in-depth analysis of original material. Outstanding zooarchaeological studies of the large faunal collections from the Conard excavations at Sibhudu since 2011 from the large and small-fraction have great promise to shed further light on the connections of MSA humans to coasts. Likewise, excavated faunal samples from the MSA of Border Cave or the LSA of Shongweni should be studied with a focus on the potential of recovering mollusks derived from dietary use.
6 Conclusion
Present archaeological data indicates that early modern humans during the MSA and LSA were capable of inhabiting the eastern Indian Ocean coast and using its variable resources for dietary and non-dietary purposes, even though marine productivity is comparatively low in this area. The inhabitants engaged with coastal and marine ecosystems in various forms over many thousands of years. The relatively small number of sites and reduced density of shellfish remains could in part be a result of the less productive of the coastal and marine ecosystems in KZN. This being said, current evidence for potential coastal adaptations in KZN appears to be heavily biased in comparison to other regions of South Africa. Chances are therefore high that the current record of the region constitutes only the tip of the iceberg of what was once present. The exact nature and extent of coastal resource use and the settlement of coastal landscapes over the Late Pleistocene by early modern humans thus remains a target for future research. Independent data from archaeology, GIS modelling and offshore geomorphology demonstrate that the shorelines of KZN - both present and past - constitute promising areas for recovering further traces for coastal adaptations in the MSA and LSA. This work can provide key insights from different ecological and geological contexts, and following from the application of novel research strategies. We will only be able to evaluate the full extent and broader relevance of the use of marine resources and the occupation of coastal ecosystems for human evolution in KZN, southern Africa and beyond once new interdisciplinary research on both onshore and offshore areas along the avenues outlined here has been performed.
Data availability statement
The original contributions presented in the study are included in the article/Supplementary Material, further inquiries can be directed to the corresponding author.
Author contributions
MW designed the study; MW; GB performed the archaeological analyses; CS performed the GIS modelling; AG; AC performed the marine geology and geomorphology; MW, GB, and CS; AG wrote the manuscript; all authors commented and proofread the final text version.
Funding
The Funding for the data presented in this paper comes from the Deutsche Forschungsgemeinschaft (grant WI 4978/3-1) and the ROCEEH project (The Role of Culture in Early Expansions of Humans) of the Heidelberg Academy of Sciences. We further acknowledge funds from the BMBF (project MA-RAIN; Grant No. 03F0731A).
Acknowledgments
We acknowledge support by the Open Access Publishing Fund of University of Tübingen. The authors thank Gavin Whitelaw for kindly sharing the KwaZulu-Natal Museum archaeological database on Stone Age sites with us. his paper uses data collected through the scientific surveys with the research vessel Dr Fridtjof Nansen as part of the collaboration between the Food and Agriculture Organization of the United Nations (FAO) on behalf of the EAF-Nansen Programme and South Africa. The EAF-Nansen Programme is a partnership between the FAO, the Norwegian Agency for Development Cooperation (Norad), and the Institute of Marine Research (IMR) in Norway for sustainable management of the fisheries in partner countries and regions. Figure 5 is based on an original draft by Lauren Pretorius, whom we acknowledge accordingly. We further acknowledge Obscape Pty Ltd. for the provision of the multibeam image of the Aliwal Shoal in Figure 8.
Conflict of interest
The authors declare that the research was conducted in the absence of any commercial or financial relationships that could be construed as a potential conflict of interest.
Publisher’s note
All claims expressed in this article are solely those of the authors and do not necessarily represent those of their affiliated organizations, or those of the publisher, the editors and the reviewers. Any product that may be evaluated in this article, or claim that may be made by its manufacturer, is not guaranteed or endorsed by the publisher.
Supplementary material
The Supplementary Material for this article can be found online at: https://www.frontiersin.org/articles/10.3389/feart.2022.964423/full#supplementary-material
References
Alvarez-Borrego, S. (1994). “Secondary productivity in coastal lagoons,” in Elsevier oceanography series (Amsterdam: Elsevier), 60, 287–309.
Anderson, T. R., Fletcher, C. H., Barbee, M. M., Romine, B. M., Lemmo, S., Delevaux, J. M. S., et al. (2018). Modeling multiple sea level rise stresses reveals up to twice the land at risk compared to strictly passive flooding methods. Sci. Rep. 8 (1), 14484. doi:10.1038/s41598-018-32658-x
Bader, G. D., Cable, C., Lentfer, C., and Conard, N. J. (2016). Umbeli Belli rock shelter, a forgotten piece from the puzzle of the Middle stone age in KwaZulu-natal, south Africa. J. Archaeol. Sci. Rep. 9, 608–622. doi:10.1016/j.jasrep.2016.08.038
Bader, G. D., Sommer, C., Conard, N. J., and Wadley, L. (2022). The final MSA of eastern south Africa: A comparative study between Umbeli Belli and Sibhudu. Azania Archaeol. Res. Afr. 57, 197–238. doi:10.1080/0067270X.2022.2078553
Bader, G. D., and Will, M. (2017). “Recent research on the MSA in KwaZulu-natal, south Africa,” in Mitteilungen der Gesellschaft für Urgeschichte (Tübingen: Kerns), 26, 79–108.
Bailey, G. N., Devès, M. H., Inglis, R. H., Meredith-Williams, M., Momber, G., Sakellariou, D., et al. (2015). Blue Arabia: Palaeolithic and underwater survey in SW Saudi Arabia and the role of coasts in Pleistocene dispersals. Quat. Int. 382, 42–57. doi:10.1016/j.quaint.2015.01.002
Bailey, G. N., and Flemming, N. C. (2008). Archaeology of the continental shelf: Marine resources, submerged landscapes and underwater archaeology. Quat. Sci. Rev. 27, 2153–2165. doi:10.1016/j.quascirev.2008.08.012
Bailey, G. N., Sakellariou, D., Alsharekh, A., Al Nomani, S., Devès, M., Georgiou, P., et al. (2017). “Africa-Arabia connections and geo-archaeological exploration in the southern Red Sea: Preliminary results and wider significance,” in Under the Sea: Archaeology and palaeolandscapes of the continental shelf. Editor G. N. Bailey (Dordrecht: Springer), 361–373. Coastal Research Library 20. doi:10.1007/978-3-319-53160-1_23
Bailey, G. (2009). “The Red Sea, coastal landscapes, and hominin dispersals,” in The evolution of human populations in arabia: Paleoenvironments, prehistory and genetics. Editors M. D. Petraglia., and J. L. Rose (New York: Springer), 15–38.
G. Bailey, N. Galanidou, H. Peeters, H. Jöns, and M. Mennenga (Editors) (2020). The archaeology of europe’s drowned landscapes (Cham: Springer), Vol. 35. Coastal Research Library.
Beater, B. E., and Maud, R. R. (1963). The occurrence of a coastal midden near Umhlanga Rocks, Natal. South Afr. Archaeol. Bull. 18, 21. doi:10.2307/3887491
Benallack, K., Green, A. N., Humphries, M. S., Cooper, J. A. G., Dladla, N. N., Finch, J. M., et al. (2016). The stratigraphic evolution of a large back-barrier lagoon system with a non-migrating barrier. Mar. Geol. 379, 64–77. doi:10.1016/j.margeo.2016.05.001
Bigalke, E. H. (1973). “The exploitation of shellfish by coastal tribesmen of the Transkei,” in Annals of the Cape province Museum of natural history (Grahamstown: Cape provincial museums), 9, 159–175.
Binford, L. R. (1968). “Post-Pleistocene adaptations,” in New perspectives in archeology. Editors L. R. Binford., and S. R. Binford (Chicago: Aldine), 313–341.
Blinkhorn, J., Ajithprasad, P., and Mukherjee, A. (2017). Did modern human dispersal take a coastal route into India? New evidence from palaeolithic surveys of kachchh, Gujarat. J. Field Archaeol. 42 (3), 198–213. doi:10.1080/00934690.2017.1323543
Boivin, N., Fuller, D. Q., Dennell, R., Allaby, R., and Petraglia, M. D. (2013). Human dispersal across diverse environments of asia during the upper Pleistocene. Quat. Int. 300, 32–47. doi:10.1016/j.quaint.2013.01.008
Bosman, C. (2012). The marine geology of the aliwal shoal, Scottburgh, South Africa. Westville: University of KwaZulu-Natal. Doctoral dissertation.
Brink, J. S., and Deacon, H. J. (1982). A study of a last interglacial shell midden and bone accumulation at Herolds Bay, Cape Province, South Africa. Palaeoecol. Afr. 15, 31–40.
Cable, C. (1984). “Economy and technology in the late stone age of southern natal,” in Cambridge monographs in african archaeology (Oxford: BAR publishing).
Campmas, E., Amani, F., Morala, A., Debenath, A., El Hajraoui, M. A., and Nespoulet, R. (2016). Initial insights into Aterian hunter–gatherer settlements on coastal landscapes: The example of Unit 8 of El Mnasra Cave (Témara, Morocco). Quat. Int. 413, 5–20. doi:10.1016/j.quaint.2015.11.136
Cawthra, H. C., Frenzel, P., Hahn, A., Compton, J. S., Gander, L., Zabel, M., et al. (2020). Seismic stratigraphy of the inner to mid Agulhas bank, South Africa. Quat. Sci. Rev. 235, 105979. doi:10.1016/j.quascirev.2019.105979
Cawthra, H. C., Jacobs, Z., Compton, J. S., Fisher, E. C., Karkanas, P., Marean, C. W., et al. (2018). Depositional and sea-level history from MIS 6 (Termination II) to MIS 3 on the southern continental shelf of South Africa. Quat. Sci. Rev. 181, 156–172. doi:10.1016/j.quascirev.2017.12.002
Cawthra, H. C., Jacobs, Z., and Wadley, L. (2022). Winds of change: Climate variability in a mild glacial on the East Coast of South Africa, inferred from submerged aeolianites and the archaeological record of Sibudu. Quat. Int. doi:10.1016/j.quaint.2022.03.014
Cawthra, H. C., Uken, R., and Ovechkina, M. N. (2012). New insights into the geological evolution of the durban Bluff and adjacent blood reef, south Africa. South Afr. J. Geol. 115 (3), 291–308. doi:10.2113/gssajg.115.3.291
Cooper, J. A. G., and Flores, R. M. (1991). Shoreline deposits and diagenesis resulting from two Late Pleistocene highstands near +5 and +6 metres, Durban, South Africa. Mar. Geol. 97, 325–343. doi:10.1016/0025-3227(91)90124-m
Cooper, J. A. G., and Green, A. N. (2016). Geomorphology and preservation potential of coastal and submerged aeolianite: Examples from KwaZulu-natal, south Africa. Geomorphology 271, 1–12. doi:10.1016/j.geomorph.2016.07.028
Cooper, J. A. G., Green, A. N., and Smith, A. M. (2013). Vertical stacking of multiple highstand shoreline deposits from the cretaceous to the present: Facies development and preservation. J. Coast. Res. 65, 1904–1908. doi:10.2112/si65-322.1
d'Errico, F., and Backwell, L. (2016). Earliest evidence of personal ornaments associated with burial: The Conus shells from border cave. J. Hum. Evol. 93, 91–108. doi:10.1016/j.jhevol.2016.01.002
Davies, O. (1975). Excavations at Shongweni south cave: The oldest evidence to date for cultigens in southern africa. Ann. Natal Mus. 22 (2), 627–662.
Davies, O., and MacDonald, J. (1978). Excavation of a shell-midden at urnhlanga rocks, natal. Ann. Natal Mus. 23, 2.
Davies, O. (1952). The place of natal in african archaeology. Theor. A J. Soc. Political Theory 4, 29–35.
De Lecea, A. M., Fennessy, S. T., and Smit, A. J. (2013). Processes controlling the benthic food web of a mesotrophic bight (KwaZulu-Natal, South Africa) revealed by stable isotope analysis. Mar. Ecol. Prog. Ser. 484, 97–114. doi:10.3354/meps10311
de Wet, W. M., and Compton, J. S. (2021). Bathymetry of the South African continental shelf. Geo-Mar. Lett. 41, 40. doi:10.1007/s00367-021-00701-y
d’Errico, F., Backwell, L., Villa, P., Degano, I., Lucejko, J. J., Bamford, M. K., et al. (2012). Early evidence of San material culture represented by organic artifacts from Border Cave, South Africa. Proc. Natl. Acad. Sci. U. S. A. 109 (33), 13214–13219. doi:10.1073/pnas.1204213109
d’Errico, F., Vanhaeren, M., and Wadley, L. (2008). Possible shell beads from the Middle stone age layers of sibudu cave, south Africa. J. Archaeol. Sci. 35, 2675–2685. doi:10.1016/j.jas.2008.04.023
Dladla, N. N., Green, A. N., Cooper, J. A. G., and Humphries, M. S. (2019). Geological inheritance and its role in the geomorphological and sedimentological evolution of bedrock-hosted incised valleys, lake St Lucia, South Africa. Estuar. Coast. Shelf Sci. 222, 154–167. doi:10.1016/j.ecss.2019.04.013
Engelbrecht, L., Green, A. N., Cooper, J. A. G., Hahn, A., Zabel, M., and Mackay, C. F. (2020). Construction and evolution of submerged deltaic bodies on the high energy SE African coastline: The interplay between relative sea level and antecedent controls. Marine Geol. 424, 106170.
Erlandson, J. M. (2001). The archaeology of aquatic adaptations: Paradigms for a new millennium. J. Archaeol. Res. 9, 287–350. doi:10.1023/a:1013062712695
Erlanger, E. D., Granger, D. E., and Gibbon, R. J. (2012). Rock uplift rates in South Africa from isochron burial dating of fluvial and marine terraces. Geology 40 (11), 1019–1022. doi:10.1130/G33172.1
Esteban, I., Bamford, M. K., House, A., Miller, C. S., Neumann, F. H., Schefuß, E., et al. (2020). Coastal palaeoenvironments and hunter-gatherer plant-use at waterfall Bluff rock shelter in Mpondoland (south Africa) from MIS 3 to the early Holocene. Quat. Sci. Rev. 250, 106664. doi:10.1016/j.quascirev.2020.106664
Falkenroth, M., Green, A. N., Cooper, J. A. G., Menzel, M. D., and Hoffmann, G. (2022). Breaking up and making up–reworking of Holocene calcarenite platform into rapidly‐forming beachrock breccias on a high energy coastline (St. Lucia, South Africa). Sedimentology 69, 1339–1364. doi:10.1111/sed.12953
Faulkner, P., Miller, J. M., Quintana Morales, E. M., Crowther, A., Shipton, C., Ndiema, E., et al. (2021). 67, 000 years of coastal engagement at Panga ya Saidi, eastern Africa. Plos one 16 (8), e0256761. doi:10.1371/journal.pone.0256761
Field, J. S., and Lahr, M. (2006). Assessment of the southern dispersal: GIS-based analyses of potential routes at oxygen isotopic stage 4. J. World Prehist. 19, 1–45. doi:10.1007/s10963-005-9000-6
Fisher, E. C., Bar-Matthews, M., Jerardino, A., and Marean, C. W. (2010). Middle and Late Pleistocene paleoscape modeling along the southern coast of South Africa. Quat. Sci. Rev. 29 (11), 1382–1398. doi:10.1016/j.quascirev.2010.01.015
Fisher, E. C., Cawthra, H. C., Esteban, I., Jerardino, A., Neumann, F. H., Oertle, A., et al. (2020). Coastal occupation and foraging during the last glacial maximum and early Holocene at Waterfall Bluff, eastern Pondoland, South Africa. Quat. Res. 97, 1–41. doi:10.1017/qua.2020.26
Flemming, B. W. (1981). Factors controlling shelf sediment dispersal along the southeast African continental margin. Mar. Geol. 42, 259–277. doi:10.1016/0025-3227(81)90166-3
Flemming, B. W. (1978). Underwater sand dunes along the southeast african continental margin—Observations and implications. Mar. Geol. 26 (3-4), 177–198. doi:10.1016/0025-3227(78)90059-2
Flemming, N. C. (2017). “The role of the submerged prehistoric landscape in ground-truthing models of human dispersal during the last half million years,” in Under the Sea: Archaeology and palaeolandscapes of the continental shelf. Editor G. N. Bailey (Dordrecht: Springer), 269–283. Coastal Research Library 20.
Forchhammer, J. G., Steenstrup, J. J. S., and Worsae, J. J. A. (1852). Undersøgelser i geologisk-antiqvarisk Retning. Copenhagen: B. Lunos Bogtrykkeri.
Green, A. N., Dladla, N., and Garlick, G. L. (2013a). Spatial and temporal variations in incised valley systems from the Durban continental shelf, KwaZulu-Natal, South Africa. Mar. Geol. 335, 148–161. doi:10.1016/j.margeo.2012.11.002
Green, A. N. (2009b). Sediment dynamics on the narrow, canyon-incised and current-swept shelf of the northern KwaZulu-Natal continental shelf, South Africa. Geo-Mar. Lett. 29, 201–219. doi:10.1007/s00367-009-0135-9
Green, A. N., Cooper, J. A. G., Leuci, R., and Thackeray, Z. (2013b). Formation and preservation of an overstepped segmented lagoon complex on a high‐energy continental shelf. Sedimentology 60 (7), 1755–1768. doi:10.1111/sed.12054
Green, A. N., Cooper, J. A. G., and Salzmann, L. (2018). The role of shelf morphology and antecedent setting in the preservation of palaeo-shoreline (beachrock and aeolianite) sequences: The SE african shelf. Geo-Mar. Lett. 38 (1), 5–18. doi:10.1007/s00367-017-0512-8
Green, A. N., Flemming, B. W., Cooper, J. A. G., and Wanda, T. F. (2022b). Bedform evolution and dynamics of a geostrophic current-swept shelf, northern KwaZulu-Natal, South Africa. Geo-Mar. Lett. 42 (1), 1. doi:10.1007/s00367-021-00722-7
Green, A. N., and Garlick, G. L. (2011). A sequence stratigraphic framework for a narrow, current-swept continental shelf: The durban bight, central KwaZulu-natal, south Africa. J. Afr. Earth Sci. 60, 303–314. doi:10.1016/j.jafrearsci.2011.03.007
Green, A. N., Humphries, M. S., Cooper, J. A. G., Strachan, K. L., Gomes, M., and Dladla, N. N. (2022a). “The Holocene evolution of Lake St Lucia, Africa's largest estuary: Geological implications for contemporary management,” in Estuarine, coastal and shelf science 266, 107745.
Green, A. N., and MacKay, C. F. (2016). Unconsolidated sediment distribution patterns in the KwaZulu-natal bight, south Africa: The role of wave ravinement in separating relict versus active sediment populations. Afr. J. Mar. Sci. 38, S65–S74. doi:10.2989/1814232x.2016.1145138
Green, A. N. (2009a). Palaeo-drainage, incised valley fills and transgressive systems tract sedimentation of the northern KwaZulu-Natal continental shelf, South Africa, SW Indian Ocean. Mar. Geol. 263, 46–63. doi:10.1016/j.margeo.2009.03.017
Green, A. N. (2011). The late Cretaceous to Holocene sequence stratigraphy of a sheared passive upper continental margin, northern KwaZulu-Natal, South Africa. Mar. Geol. 289, 17–28. doi:10.1016/j.margeo.2011.09.001
Green, A. N., and Uken, R. (2005). First observations of sea-level indicators related to glacial maxima at Sodwana Bay, northern KwaZulu-Natal. South Afr. J. Sci. 101 (5), 236–238.
Gribble, J. (2021). Heritage impact assessment of proposed 2Africa/Gera (East) submarine fibre optic cable system, landing at Amanzimtoti, KwaZulu-Natal. Mtunzini: Acer (Africa) Environmental Consultants, 84. Unpublished report.
Grine, F. E., Klein, R. G., and Volman, T. P. (1991). Dating, archaeology and human fossils from the Middle stone age levels of Die Kelders, south Africa. J. Hum. Evol. 21, 363–395. doi:10.1016/0047-2484(91)90113-a
Grøn, O., Boldreel, L. O., Smith, M. F., Joy, S., Tayong Boumda, R., Mäder, A., et al. (2021). Acoustic mapping of submerged stone age sites—a HALD approach. Remote Sens. 13 (3), 445. doi:10.3390/rs13030445
Groucutt, H. S., Petraglia, M. D., Bailey, G., Scerri, E. M., Parton, A., Clark‐Balzan, L., et al. (2015). Rethinking the dispersal of Homo sapiens out of Africa. Evol. Anthropol. 24 (4), 149–164. doi:10.1002/evan.21455
Henshilwood, C. S., Van Niekerk, K. L., Wurz, S., Delagnes, A., Armitage, S. J., Rifkin, R. F., et al. (2014). Klipdrift shelter, southern Cape, south Africa: Preliminary report on the Howiesons Poort layers. J. Archaeol. Sci. 45, 284–303. doi:10.1016/j.jas.2014.01.033
Hicks, N., and Green, A. N. (2016). Sedimentology and depositional architecture of a submarine delta-fan complex in the Durban Basin, South Africa. Mar. Petroleum Geol. 78, 390–404. doi:10.1016/j.marpetgeo.2016.09.032
Hijmans, R. J. (2021). geosphere: Spherical trigonometry. R package version 1.5-14. Available at: https://CRAN.R-project.org/package=geosphere (Accessed July 19, 2022).
Hockey, P. A. R., Bosman, A. L., and Siegfried, W. R. (1988). Patterns and correlates of shellfish exploitation by costal people in Transkei: An enigma of protein production. J. Appl. Ecol. 25, 353. doi:10.2307/2403631
Horwitz, L., Maggs, T., and Ward, V. (1991). Two shell middens as indicators of shellfish exploitation patterns during the first millennium AD on the Natal north coast. South. Afr. Humanit. 3 (10), 1–28.
Jerardino, A., and Marean, C. W. (2010). Shellfish gathering, marine paleoecology and modern human behavior: Perspectives from cave PP13B, pinnacle point, south Africa. J. Hum. Evol. 59, 412–424. doi:10.1016/j.jhevol.2010.07.003
Jerardino, A. (2016). On the origins and significance of Pleistocene coastal resource use in southern Africa with particular reference to shellfish gathering. J. Anthropol. Archaeol. 41, 213–230. doi:10.1016/j.jaa.2016.01.001
Jerardino, A. (2010). Prehistoric exploitation of marine resources in southern africa with particular reference to shellfish gathering: Opportunities and continuities. Pyrenae 41, 7–52.
Kelly, C. S., Green, A. N., Cooper, J. A. G., and Wiles, E. A. (2014). Beachrock facies variability and Sea level implications: A preliminary study. J. Coast. Res. 70, 736–742. doi:10.2112/si70-124.1
King, L. C. (1972). The coastal plain of southeast Africa: Its form, deposits and development. Z. Geomorph 16, 239–251.
Klein, R. G., and Steele, T. E. (2013). Archaeological shellfish size and later human evolution in Africa. Proc. Natl. Acad. Sci. U. S. A. 110 (27), 10910–10915. doi:10.1073/pnas.1304750110
Kyle, R., Pearson, B., Fielding, P. J., Robertson, W. D., and Birnie, S. L. (1997a). Subsistence shellfish harvesting in the maputaland marine reserve in northern KwaZulu-natal, south Africa: Rocky shore organisms. Biol. Conserv. 82 (2), 183–192. doi:10.1016/s0006-3207(97)00022-0
Kyle, R., Robertson, W. D., and Birnie, S. L. (1997b). Subsistence shellfish harvesting in the maputaland marine reserve in northern KwaZulu-natal, south Africa: Sandy beach organisms. Biol. Conserv. 82 (2), 173–182. doi:10.1016/s0006-3207(97)00021-9
Kyriacou, K. (2017). Intertidal shellfish as a source of protein and energy for the Middle Stone Age inhabitants of the southwestern Cape and northern KwaZulu-Natal, South Africa. Quat. Int. 438, 30–39. doi:10.1016/j.quaint.2017.01.032
Kyriacou, K., Parkington, J. E., Marais, A. D., and Braun, D. R. (2014). Nutrition, modernity and the archaeological record: Coastal resources and nutrition among Middle stone age hunter-gatherers on the western Cape coast of south Africa. J. Hum. Evol. 77, 64–73. doi:10.1016/j.jhevol.2014.02.024
Langejans, G. H. J., van Niekerk, K. L., Dusseldorp, G. L., and Thackeray, J. F. (2012). Middle stone age shellfish exploitation: Potential indications for mass collecting and resource intensification at Blombos cave and Klasies River, south Africa. Quat. Int. 280, 80–94. doi:10.1016/j.quaint.2011.09.003
Marean, C. W. (2014). The origins and significance of coastal resource use in Africa and Western Eurasia. J. Hum. Evol. 77, 17–40. doi:10.1016/j.jhevol.2014.02.025
Martin, A. K., and Flemming, B. W. (1988). “Physiography, structure and geological evolution of the Natal continental shelf,” in Lecture notes on coastal and estuarine studies, 26, 11–46. doi:10.1007/978-1-4757-3908-4_2
Mauz, B., Vacchi, M., Green, A., Hoffmann, G., and Cooper, A. (2015). Beachrock: A tool for reconstructing relative sea level in the far-field. Marine Geol. 362, 1–16.
Meighan, C. W. (1969). “Molluscs as food remains in archaeological sites,” in Science in archaeology. Editors D. R. Brothwell., and E. S. Higgs (London: Thames and Hudson), 415–422.
Mellars, P., Gori, K. C., Carr, M., Soares, P. A., and Richards, M. B. (2013). Genetic and archaeological perspectives on the initial modern human colonization of southern Asia. Proc. Natl. Acad. Sci. U. S. A. 110, 10699–10704. doi:10.1073/pnas.1306043110
Mitchell, P. J. (1996). Prehistoric exchange and interaction in southeastern southern Africa: Marine shells and ostrich eggshell. Afr. Archaeol. Rev. 13, 35–76. doi:10.1007/bf01956132
Morris, M. A., Krysl, P., Rivera-Collazo, I. C., and Hildebrand, J. A. (2022). The resonant acoustic signatures of lithic debitage. J. Archaeol. Sci. Rep. 41, 103266. doi:10.1016/j.jasrep.2021.103266
Morrissey, P., Knight, J., and Stratford, D. J. (2020). Early Marine Isotope Stage 5 sea levels, coastal dune palaeoenvironments, and human occupation on the southeast coast of South Africa. Quat. Sci. Rev. 245, 106504. doi:10.1016/j.quascirev.2020.106504
Niang, K., Blinkhorn, J., Ndiaye, M., Bateman, M., Seck, B., Sawaré, G., et al. (2020). The Middle stone age occupations of tiémassas, coastal west africa, between 62 and 25 thousand years ago. J. Archaeol. Sci. Rep. 34, 102658. doi:10.1016/j.jasrep.2020.102658
Niespolo, E. M., Sharp, W. D., Avery, G., and Dawson, T. E. (2021). Early, intensive marine resource exploitation by Middle Stone Age humans at Ysterfontein 1 rockshelter, South Africa. Proc. Natl. Acad. Sci. U. S. A. 118 (16), e2020042118. doi:10.1073/pnas.2020042118
Osborn, A. (1977). “Strandloopers, mermaids, and other fairy tales: Ecological determinants of marine resource utilization—The Peruvian case,” in For theory building in archaeology. Editor L. R. Binford (New York: Academic Press), 157–205.
Parkington, J. E. (2003). Middens and moderns: Shellfishing and the Middle stone age of the western Cape, south Africa. South Afr. J. Sci. 99, 243–247.
Parkington, J. E., Poggenpoel, C. A., Buchanan, B., Robey, T., Manhire, A. H., and Sealy, J. C. (1988). “Holocene coastal settlement patterns in the Western Cape,” in The archaeology of prehistoric coastlines. Editors G. N. Bailey., and J. E. Parkington (Cambridge: Cambridge University Press), 22–41.
Pebesma, E. (2021). stars: Spatiotemporal arrays, raster and vector data cubes. R package version 0.5-5. Available at: https://CRAN.R-project.org/package=stars (Accessed July 19, 2022).
Pebesma, E. (2018). Simple features for R: Standardized support for spatial vector data. R J. 10 (1), 439. doi:10.32614/RJ-2018-009
Plug, I. (2006). Aquatic animals and their associates from the Middle stone age levels at sibudu. South. Afr. Humanit. 18, 289–299.
Pretorius, L., Green, A., and Cooper, A. (2016). Submerged shoreline preservation and ravinement during rapid postglacial sea-level rise and subsequent “slowstand”. Geol. Soc. Am. Bull. 128 (7-8), 1059–1069. doi:10.1130/b31381.1
Pretorius, L., Green, A. N., and Andrew Cooper, J. (2018). Submerged beachrock preservation in the context of wave ravinement. Geo-Mar. Lett. 38, 19–32. doi:10.1007/s00367-017-0503-9
Pretorius, L., Green, A. N., Cooper, J. A. G., Hahn, A., and Zabel, M. (2019). Outer-to inner-shelf response to stepped sea-level rise: Insights from incised valleys and submerged shorelines. Mar. Geol. 416, 105979. doi:10.1016/j.margeo.2019.105979
R Core Team (2022). R: A language and environment for statistical computing (4.1.5). [Computer software]. R Foundation for Statistical Computing. Available at: https://www.r-project.org/.
Rabett, R. J. (2018). The success of failed Homo sapiens dispersals out of Africa and into Asia. Nat. Ecol. Evol. 2, 212–219. doi:10.1038/s41559-017-0436-8
Robey, T. (1980). Mpambanyoni: A late Iron age site on the natal South Coast. Ann. Natal Mus. 24 (1), 147–164.
Schoute-Vanneck, C. A. (1958). The shell middens on the durban Bluff. South Afr. Archaeol. Bull. 13, 43. doi:10.2307/3886895
Siegfried, W. R., Hockey, P. A., and Crowe, A. A. (1985). Exploitation and conservation of Brown mussel stocks by coastal people of Transkei. Environ. Conserv. 12 (4), 303–307. doi:10.1017/s037689290003441x
Smith, A. M., Mather, A. A., Bundy, S. C., Cooper, J. A. G., Guastella, L. A., Ramsay, P. J., et al. (2010). Contrasting styles of swell-driven coastal erosion: Examples from KwaZulu-natal, south Africa. Geol. Mag. 147, 940–953. doi:10.1017/s0016756810000361
Steele, T. E., and Alvarez-Fernández, E. (2011). “Initial investigations into the exploitation of coastal resources in north africa during the late Pleistocene at grotte des contrebandiers, Morocco,” in Trekking the shore: Changing coastlines and the antiquity of coastal settlement. Editors N. Bicho, J. A. Haws, and L. G. Davis (New York: Springer), 383–403.
Steele, T. E., Mackay, A., Fitzsimmons, K. E., Igreja, M., Marwick, B., Orton, J., et al. (2016). Varsche rivier 003: A Middle and later stone age site with still Bay and howieson's Poort assemblages in southern namaqualand, south Africa. Paleo Anthropol. 2016, 100–163.
Thackeray, J. F. (1988). Molluscan fauna from Klasies River, south Africa. South Afr. Archaeol. Bull. 43, 27. doi:10.2307/3887610
Tribolo, C., Mercier, N., Martin, L., Taffin, N., Miller, C. E., Will, M., et al. (2022). Luminescence dating estimates for the coastal MSA sequence of Hoedjiespunt 1 (South Africa). J. Archaeol. Sci. Rep. 41, 103320. doi:10.1016/j.jasrep.2021.103320
Vanhaeren, M., Wadley, L., and d'Errico, F. (2019). Variability in Middle stone age symbolic traditions: The marine shell beads from Sibudu Cave, South Africa. J. Archaeol. Sci. Rep. 27, 101893. doi:10.1016/j.jasrep.2019.101893
Volman, T. (1978). Early archeological evidence for shellfish collecting. Science 201, 911–913. doi:10.1126/science.201.4359.911
Waelbroeck, C., Labeyrie, L., Michel, E., Duplessy, J. C., McManus, J. F., Lambeck, K., et al. (2002). Sea-level and deep water temperature changes derived from benthic foraminifera isotopic records. Quat. Sci. Rev. 21 (1), 295–305. doi:10.1016/s0277-3791(01)00101-9
Waselkov, G. A. (1987). Shellfish gathering and shell midden archaeology. Adv. Archaeol. Method Theory 10, 93–210. doi:10.1016/b978-0-12-003110-8.50006-2
Washburn, S. L., and Lancaster, C. S. (1968). “The evolution of hunting,” in Man the hunter. Editors R. B. Lee., and I. DeVore (Chicago: Aldine), 293–303.
Wiles, E., Green, A. N., and Cooper, J. A. G. (2018). Rapid beachrock cementation on a South African beach: Linking morphodynamics and cement style. Sediment. Geol. 378, 13–18. doi:10.1016/j.sedgeo.2018.10.004
Will, M., Kandel, A. W., and Conard, N. J. (2019). Midden or molehill: The role of coastal adaptations in human evolution and dispersal. J. World Prehist. 32, 33–72. doi:10.1007/s10963-018-09127-4
Will, M., Kandel, A. W., Kyriacou, K., and Conard, N. J. (2016). An evolutionary perspective on coastal adaptations by modern humans during the Middle Stone Age of Africa. Quat. Int. 404, 68–86. doi:10.1016/j.quaint.2015.10.021
Will, M., Parkington, J. E., Kandel, A. W., and Conard, N. J. (2013). Coastal adaptations and the Middle stone age lithic assemblages from Hoedjiespunt 1 in the western Cape, south Africa. J. Hum. Evol. 64, 518–537. doi:10.1016/j.jhevol.2013.02.012
Yesner, D. R. (1987). “Life in the “Garden of Eden”: Constraints of marine diets for human societies,” in Food and evolution. Editors M. Harris., and E. Ross (Philadelphia: Temple University Press), 285–310.
Keywords: middle stone age, later stone age, marine geomorphology, GIS model, offshore & marine, paleolithic archaeology, palaeolithic
Citation: Will M, Bader GD, Sommer C, Cooper A and Green A (2022) Coastal adaptations on the eastern seaboard of South Africa during the Pleistocene and Holocene? Current evidence and future perspectives from archaeology and marine geology. Front. Earth Sci. 10:964423. doi: 10.3389/feart.2022.964423
Received: 08 June 2022; Accepted: 13 July 2022;
Published: 15 August 2022.
Edited by:
Francisca Martinez-Ruiz, Spanish National Research Council (CSIC), SpainReviewed by:
Michael Lazar, University of Haifa, IsraelHuw Groucutt, Max Planck Institute for Chemical Ecology, Germany
Copyright © 2022 Will, Bader, Sommer, Cooper and Green. This is an open-access article distributed under the terms of the Creative Commons Attribution License (CC BY). The use, distribution or reproduction in other forums is permitted, provided the original author(s) and the copyright owner(s) are credited and that the original publication in this journal is cited, in accordance with accepted academic practice. No use, distribution or reproduction is permitted which does not comply with these terms.
*Correspondence: Manuel Will, manuel.will@uni-tuebingen.de