- 1Dipartimento di Scienze della Terra, Università degli Studi diTorino, Turin, Italy
- 2Centro Interdipartimentale sui Rischi Naturali in Ambiente Montano e Collinare—NatRisk, Turin, Italy
Detecting precursory signals before an eruption is one of the main objectives of applied volcanology. Among these signals, the variation of the emitted heat flux is certainly an important indicator of a state of disequilibrium within the magmatic system. Here we report the results of a detailed analysis of VIIRS (Visible Infrared Imaging Radiometer Suite) imaging bands (at 375 m spatial resolution) focused on measuring the Volcanic Radiative Power (VRP) emitted by the fumarole field of La Fossa crater (Vulcano Island, Italy) over the past decade (2012–2022). The analysis reveals that the long-term, steady-state VRP (baseline ∼0.17 MW) was perturbed in 2020–2021 when a prolonged period of lower than normal (<-2σ) radiant flux preceded the major unrest phase that began in mid-September 2021. By early October the anomalous VRP had peaked at ∼1.2 MW (6–8 times the baseline) then started to gradually decline in the following months. A subsequent thermal pulse was recorded in May–July 2022 and was accompanied by a period of seawater discoloration that affected the Baia di Levante (a shallow sea bay ∼1.4 km north of La Fossa crater). The concomitance of these phenomena suggests the occurrence of a second pressurization phase driven by the arrival of deep magmatic fluids within both the central and distal degassing fumarolic zones. These results provide a complementary, important contribution to the understanding of the unrest of La Fossa crater and highlight the potential of VIIRS in detecting pre-eruptive signals at other poorly-monitored volcanoes characterized by high-temperature fumarolic activity.
Introduction
Together with ground monitoring networks (e.g., geochemistry, geophysics, geodesy), space-based thermal data are increasingly used for detecting volcanic unrest at quiescent volcanoes characterized by background fumarolic activity (Reath et al., 2016; Furtney et al., 2018; Ramsey et al., 2021). Thermal remote sensing offers an effective and safe tool to detect thermal anomalies and allows the heat flux to be measured safely and homogeneously, now for decades (Harris, 2013). However, the ability to detect the fumarolic heat emissions from space strongly depends on the features of the thermal anomalies (i.e., number, size and temperature) as well as on the spatial, temporal, and spectral resolutions of the sensors used (Harris, 2013). Sensors like OLI (Operational Land Iager; onboard of Landsat 8) or ASTER (Advanced Spaceborne Thermal Emission and Reflection Radiometer), which use bands in the Thermal Infrared (TIR: 8–14 μm) with spatial resolutions of 90–100 m, have been proved to be well suited for mapping worldwide fumarole fields (Vaughan et al., 2012; Braddock et al., 2017; Caputo et al., 2019; Reath et al., 2019; Silvestri et al., 2019; Ramsey and Flynn 2020; Way et al., 2022) as well as for quantifying the long-term thermal flux at La Fossa volcano (Italy; Harris and Stevenson 1997a, b; Mannini et al., 2019). However, their temporal resolution (1 image every 10–16 days approximately), together with the cloud coverage issues (about 55% of ASTER nighttime images are covered by clouds over Vulcano Island) make their use still limited for near-real-time monitoring purposes (e.g., Wadge & Aspinall, 2014; Wadge et al., 2014; Mothes et al., 2017).
On the other hand, moderate-temporal and-spatial resolution sensors like AVHRR (Advanced Very High Resolution Radiometer), MODIS (Moderate Resolution Imaging Spectroradiometer) or VIIRS (Visible Infrared Imaging Radiometer Suite), offer an image frequency suitable for daily monitoring of global volcanic (magmatic) activity by providing up to four images per day (Coppola et al., 2020). Some pioneering studies (Harris and Stevenson 1997a,b) have demonstrated the ability of AVHRR to detect thermal anomalies associated with degassing of La Fossa volcano, but their quantification remained difficult because of the coarse resolution of these sensors (about 1 km). As a result, there is currently no operative, satellite hot-spot detection system capable of automatic daily monitoring of a fumarole field.
In this work, we show that the data provided by the VIIRS Imaging Bands (375 m resolution in the middle infrared; MIR: 3–5 μm) can bridge this gap, and allow for monitoring radiant flux of La Fossa crater fumarolic areas (Vulcano Island, Italy) from space. In particular, we demonstrate for the first time how these data can automatically track and follow the background thermal activity of this volcano, and allow the timely detection of the anomalous heat flux that characterize the ongoing unrest, started in September 2021.
The island of Vulcano is the southernmost of the Aeolian islands (Figure 1) and in historical times it has been characterized by frequent eruptions of moderate size (Frazzetta et al., 1983; De Astis et al., 2013; Di Traglia et al., 2013). The last eruptive period dates back to 1873 AD when explosive events, separated by periods of intense degassing, occurred at La Fossa cone, the most recent eruptive center of the volcano (Figure 1). This activity culminated with the 1888–1890 AD eruption when La Fossa was the site of a violent explosive activity, currently known as the archetypal “Vulcanian eruption” (Mercalli 1907). Since then, fumarolic activity has been established on the summit crater, called Gran Cratere (Figure 1B), with gas emissions reaching temperatures up to >500°C (Diliberto 2017, 2021). Between 1987 and 1993 an unrest episode was characterized by an increase in fumarolic activity accompanied by significant variations in CO2 flux in the crater area and the peripheral systems at the base of the cone (Barberi et al., 1991; Chiodini et al., 1996; Montalto 1996). The unrest included the occurrence of shallow volcano-seismic crisis, which however did not culminate in an eruption (Barberi et al., 1991). The seismic sequences was interpreted as the result of an increasing magmatic component of the hydrothermal fluids causing pressurization of the hydrothermal system located 0.5–1.5 km below La Fossa crater (Alparone et al., 2010). Other minor unrest phases were also recorded in 2004–2005, 2009, and 2017 (Granieri et al., 2006; Paonita et al., 2013; Ricci et al., 2015; Selva et al., 2020), but were characterized by weak or not significant intensifications in superficial micro-seismicity and likely involved a modest increase in the total contribution of magmatic fluids to the volcanic system (Aiuppa et al., 2005; Selva et al., 2020). A polybaric system, with several magmatic ponding zones, is supposed to exist under Vulcano, with the shallowest storage zone located at 1–2 km beneath La Fossa cone (Selva et al., 2020 and references therein). Above this zone a very shallow hydrothermal system feeds the main fumarole field located in the crater area, while a second area of exhalations is located in the “Baia di Levante“, located ∼1.4 km north of the crater (Chiodini et al., 1995; Figure 1B).
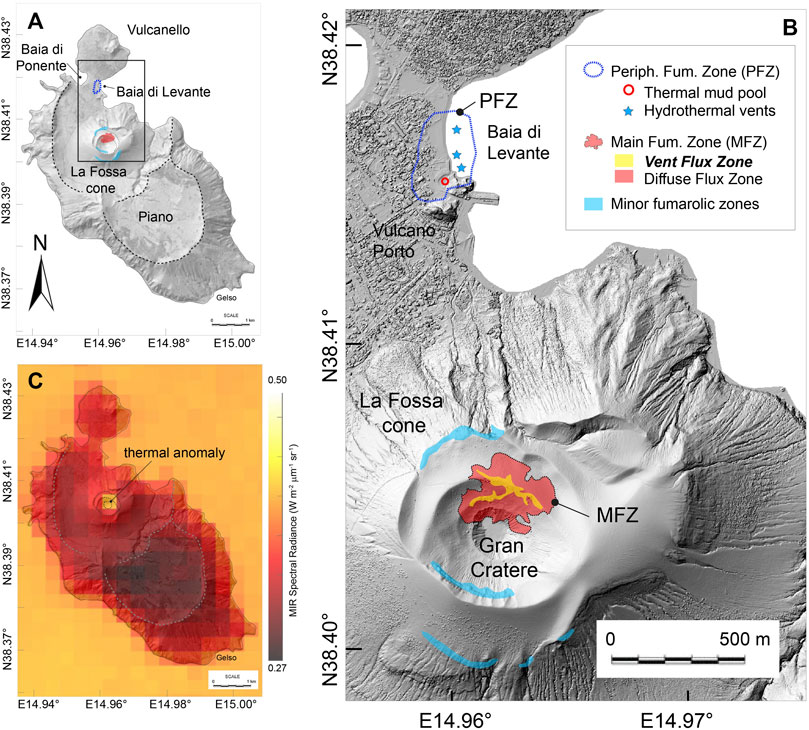
FIGURE 1. (A) Sketch map of Vulcano Island, the southernmost island of Aeolian Archipelago, showing the location of La Fossa cone and the Baia di Levante. (B) Detail of the Gran Cratere and the north flank of La Fossa cone, showing the approximate outlines of the Main Fumarolic Zone (MFZ) and the Peripheral Fumarolic Zone (PFZ) (modified from Diliberto 2011, 2017, 2021, Madonia 2020; Müller et al., 2021; Mannini et al., 2019). Following Mannini et al., 2019, the MFZ (inside the Grand Cratere) is subdivided into two distinct zones: (1) the vent flux zone (in yellow) and (2) the diffuse flux zone (in red). Minor fumarolic zones surrounding the Gran Cratere are shown in light blue; The PFZ is located in the Vulcano Porto area. Hydrothermal manifestations in this area consist of a thermal mud pool (red circle) and several hydrothermal vents (blue stars) in the Baia di Levante; (C) Nightime VIIRS image (375 m resolution) acquired on 1st September 2021, showing one anomalous pixel located over the MFZ of La Fossa crater (color map scaled to MIR radiance).
A new major episode of unrest began in September 2021 (INGV weekly reports, 2021) and was followed on 1 October 2021 by the raising of the alert level from green to yellow (Dipartimento della Protezione Civile, 2021). The unrest (still ongoing at the time of this writing) is characterized by an increase in degassing, by variations in the temperature and chemical composition of the fumaroles (Inguaggiato et al., 2022), as well as by the appearance of micro-seismicity associated with movement of fluids (very long period events) accompanied by a moderate deformation of La Fossa cone (INGV weekly reports, 2021, 2022a, b).
Heat flux at La Fossa volcano
Heat release at La Fossa is monitored since 1984 by the Istituto Nazionale di Geofisica e Vulcanologia (INGV) through a network of permanent ground stations located in the Main Fumarolic Zone (MFZ), in the Peripheral Fumarolic Zone (PFZ), and in minor steam-heated soil zones around the cone (Diliberto 2011, 2017, 2021; Madonia 2020; Figure 1B).
The MFZ is an area of persistent degassing located on the northern edge of the Gran Cratere (Figure 1B). According to Mannini et al., 2019, the MFZ is composed of two distinct thermal regions (Figure 1B) (I) a broad zone of diffuse surface degassing, named the diffuse flux zone, and characterized by ground temperatures a few degrees above the background and (II) a zone of focused fumarolic venting, named vent flux zone, where a large number of open vents and fractures (fumaroles) are distributed across the field. The fumaroles have temperature ranging from less than 100°C to more than 500°C and result from the mixing between magmatic and hydrothermal fluids (Chiodini et al., 1995; Harris and Maciejewski 2000; Diliberto 2011; Madonia 2020).
A series of minor fumarolic areas are also present on the external rims of the La Fossa cone (Figure 1B). These exhalating zones are composed of low-temperature fumaroles (typically <100°C) that have a geochemical signature compatible with a variable mixing between hydrothermal and shallow meteoric components (Madonia 2020).
Finally, the peripheral fumarolic zone (PFZ) is located ∼1.4 km north of La Fossa crater, in an area that includes the village of Vulcano Porto, the Baia di Levante beach, and the shallow sea areas in front of it (Figure 1B). In this zone, famous for the thermal mud pool, the presence of an extensive hydrothermal aquifer is testified by diffuse degassing of CO2 together with numerous carbon-rich thermal wells (up to 80°C) and several bubbling hydrothermal vents (<100°C) (Federico et al., 2010; Diliberto et al., 2021).
The region of greatest heat loss is certainly the MFZ, which in 1998 produced about ∼90% of the total heat flux of La Fossa volcano (Chiodini et al., 2005). However, it is well known that the number and extent of the fumaroles vary with time in response to fumarole migration, self-sealing, or because of variation in the deep heat source (Harris and Maciejewski 2000; Harris et al., 2009; Harris et al., 2012; Mannini et al., 2019). For example, between 1994 and 1997 Harris and Maciejewski 2000, measured an enlargement of the exhalation area which brought the heat flux released by the MFZ to increase from 45 to 67 MW. On the other hand, using satellite and field data acquired between 2000 and 2019, Mannini and coauthors (2019), calculated a total heat flux from the MFZ ranging from 5 to 14 MW (much lower than in the 90s), compatible with a general reduction of the number, distribution, and extension of the fumarolic areas.
For the same period Mannini et al. (2019) estimated that most of the heat released from the MFZ is lost through convection (∼85%), while the remaining 15% (i.e., from 0.68 to 1.92 MW) represents the radiative component. The latter is in turn composed of the contribution of the two thermal zones described above; that is, 0.58–1.75 MW are radiated from the diffuse flux zone and only 0.1–0.26 MW are radiated from the vent flux zone. These partitionings suggest that the heat flux radiated by the vent flux zone alone, although composed of the highest temperature fumaroles, represents only 1–2% of the total heat flux. On the other hand, the same authors found a strong coupling between the heat flux radiated by the two zones of MFZ, so that the perturbations of the hydrothermal system caused by deep sources manifest themselves through variations of heat flux from both regions of MFZ.
Methods
Visible Infrared Imaging Radiometer Suite is a whiskbroom multispectral sensor that acquires data in 22 spectral bands (from 0.4 to 12.5 μm). Among these two Imaging Bands (named I04 and I05), at 375 m resolution, acquire in the Middle Infrared (MIR) and Thermal Infrared (TIR) regions (at 3.74 and 11.45 μm, respectively). Currently, two VIIRS sensors are mounted onboard the NASA and NOAA’s Suomi-NPP (in orbit from October 2011) and JPSS-1 (or NOAA-20, in orbit from November 2017) satellites (Cao et al., 2017). In this work, we have elaborated 7,723 nighttime images acquired above La Fossa volcano between 19 January 2012 and 15 July 2022 (Figure 2). The data have been elaborated by applying the MIROVA algorithm to the VIIRS Imaging Bands, following the procedure already used for MODIS (Coppola et al., 2015) and VIIRS (Campus et al., 2022) bands, at 1 km and 750 m resolution, respectively. Thanks to the improved spatial resolution of the Imaging Bands, the algorithm allowed to detect thermal anomalies over La Fossa volcano (as the one shown in Figure 1C), otherwise undetected using MODIS (1 km) and VIIRS (750 m). Hence, for each hot-spot contaminated pixel we calculated the Volcanic Radiative Power (VRP) using the MIR-method (Coppola et al., 2013):
where
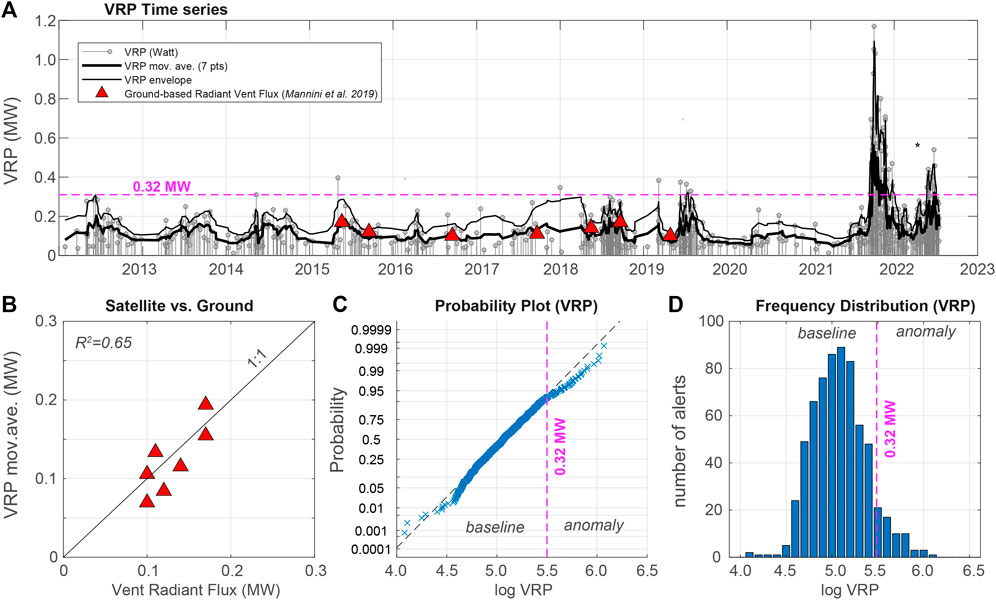
FIGURE 2. (A) Timeseries of Volcanic Radiative Power (VRP) recorded over La Fossa volcano by VIIRS imaging bands (375 m resolution) between 2012 and 2022. Gray stems correspond to single VRP detection from VIIRS data. The thick black curve represents the moving average (7 points) of the VRP. The thin black curve represents the envelope of the VRP calculated using Hilbert filter. Red triangles correspond to the radiant vent flux measured during field campaigns by Mannini et al., 2019. (B) The relationship between satellite-derived VRP and field-derived vent radiant flux indicates an excellent correlation (R2 = 0.65). (C) Probability plot and (D) frequency distribution of the VRP dataset (log-transformed) outline the presence of two thermal regimes corresponding to the baseline activity (VRP <0.32 MW) and anomaly (>0.32 MW), respectively. The automatic detection of VRP >0.32 MW occurred at a rate (on a daily scale) of about two orders of magnitude higher since September 2021.
For volcanic thermal features with temperatures comprised in the range where the approximation holds, the MIR-method allows estimating the VRP with an error of 30% (Coppola et al., 2013). On the other hand, for features composed of thermal components even lower than 600 K, the VRP underestimates the total flux thus representing only the fraction radiated by a smaller and hotter portion of the feature (Coppola et al., 2013). As it will be shown below, in the specific case of the La Fossa volcano, the VRP proved to be very efficient in measuring the heat radiated exclusively by the vent flux zone of the MFZ.
More specific approaches can be applied to estimate the total heat flux of the MFZ (this including both the vent and diffuse flux zones), by assuming for example the size/temperature of each region, their emissivities, as well as the transmissivity of the atmosphere (Harris and Stevenson, 1997a). However, in this work, we preferred to use the VRP “as is”, since it is immediately applicable in near-real-time, without any atmospheric correction nor the need for any assumptions (which could be nontrivial during ongoing eruptive crises). Moreover, measuring the VRP of La Fossa’s fumaroles allows a direct comparison with the radiant power continuously measured on other volcanic systems (for example Stromboli) and by automatic systems such as MIROVA, MODVOLC, and FIRMS.
Results
The timeseries of VRP recorded between 2012 and 2022 is shown in Figure 2A, where is combined with the periodic measurements of the heat radiated by the vent flux zone, as derived from ground thermal images (Mannini et al., 2019). As a whole between 28 January 2012 and 15 July 2022, VIIRS-375 detected 696 anomalies with VRP ranging from ∼0.01 to ∼1.17 MW.
The comparison between the satellite data (moving average on seven points) and punctual ground measurements (Figure 2B) reveals an fair correlation (R2 = 0.65), with points dispersed around the 1:1 ratio. This relationship, although purely empirical, suggests that in the case of La Fossa, the VRP retrieved using the MIR-method (Eq. (1)) can be considered a reliable proxy of the heat radiated by the vent flux zone.
During typical fumarolic activity (e.g between January 2012 and August 2021) the VRP fluctuates well below 1 MW (Figure 2D), with a mean value of ∼0.12 MW and a standard deviation (σ) of ∼0.06 MW. In this period, VIIRS 375 detected 426 anomalies with an average interval between detections of ∼9 days. This low detection rate and the low VRP explains the lack of thermal anomalies at La Fossa volcano detected by the most common automatic systems such as MIROVA (https://www.mirovaweb.it/), MODVOLC (http://modis.higp.hawaii.edu/) or FIRMS (https://firms.modaps.eosdis.nasa.gov/active_fire/), whose detection limit is about 1 MW (Coppola et al., 2020).
On the other hand, the frequency distribution (log-transformed) and the probability plot of the whole VRP dataset (Figures 1C,D) show a pattern composed of two thermal regimes (hereby called baseline and anomaly) separated at VRP equal to ∼0.32 MW (logVRP = 5.5). Numerous detections above the 0.32 MW threshold were recorded after September 2021, coinciding with the unrest phase.
Discussion
The decadal trend of VRP delineates overall stability in VRP (steady-state activity in Harris and Stevenson, 1997b) which fluctuated mostly between 0.1 and 0.2 MW (Figure 2A). Two periods of increased thermal activity, occurred in the summer of 2018 and 2019 when frequent alerts (every 1–2 days), were punctuated by some measurements above 0.3 MW. These anomalous heat releases were accompanied by increased in-soil CO2 flux measured at different sites, including the PFZ (Di Martino et al., 2020). Based on δ13C-CO2 and CO2 flux data, Di Martino et al., 2020, suggest that such increases reflected an increase of degassing from a deep magma body, therefore tracked also by the increase in VRP.
On the contrary, from 2019 to the first half of 2021, the venting area was characterized by a prolonged period of lower than normal VRP (∼0.1 MW), as evidenced by the cumulative curve of thermal energy release (Figure 3). Indeed, in that period there is a deviation from the long-term steady-state thermal trend (∼0.17 MW), which reaches a minimum in June 2021 clearly outside the normal fluctuations (±2σ) recorded since 2012 (Figure 3B). Changes in heat flux associated to fumarole migration and sealing were already observed between 1994 and 1999 (Harris and Maciejewski 2000). According to Harris and Maciejewski 2000, a decrease in the heat flux of MVZ could be ascribed to 1) a decrease in system permeability (including shallow or deep sealing) and/or 2) a decrease in degassing of the deep, magmatic source. We do not have sufficient data to be able to distinguish between these two mechanisms but it is interesting to note that precursory decreases in the gas/heat flux before explosive eruptions are reported elsewhere (with a variable duration of decreased periods spanning from years to minutes) and commonly attributed to the sealing and pressurization of the hydrothermal system (Christenson et al., 2010; Yokoo et al., 2013; Kazahaya et al., 2016, 2019; Mori et al., 2017).
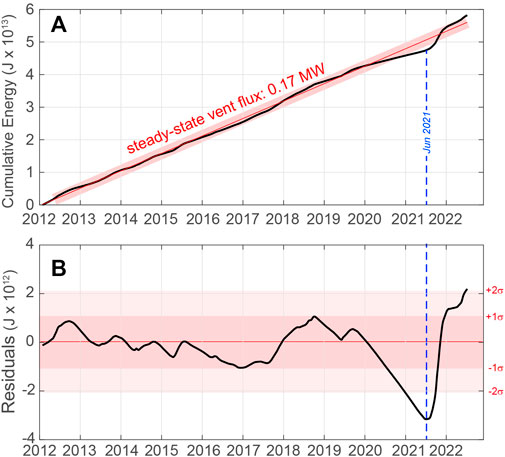
FIGURE 3. (A) Cumulative radiant energy released by the vent flux area of La Fossa volcano between 2012 and 2022 (only Suomi-NPP has been considered). The steady-state thermal emission model (red line) indicates a long-term radiant flux of ∼0.17 MW. (B) Residuals of thermal energy (observed—model) show the departure from the steady-state model (red line with ± 2σ variations). A clear decrease in the thermal emission was recorded between July 2020 and June 2021 resulting from particularly low thermal emissions. In the following months, La Fossa enters a state of major unrest (still ongoing on 15 July 2022).
Starting from June-July 2021, a reversal trend is observed in the VRP timeseries, with a thermal flux that gradually returns to increase (Figure 4A). This change was roughly concomitant with an increase in CO2 flux in the crater area followed, in August, by an increase in the temperature of the fumaroles, and by a slight increase in temperature and conductivity in some wells around cone (INGV report 2021). The unrest becomes evident in mid-September when a net increase in magmatic gas flux was recorded in the crater area and in the thermal aquifer at the base of the cone (INGV report 2021). Since 13 September 2021 the clinometric network of INGV has recorded a slight uplift of the cone, accompanied by an increase in the frequency of occurrence of seismic events, which include (for the first time at this volcano) Very Long Period (VLP) events (INGV report 2021). This acceleration has been recorded by VIIRS (Figure 4A), which, starting from 17 September, begins to detect frequent thermal anomalies with VRP values higher than the statistical threshold (0.32 MW).
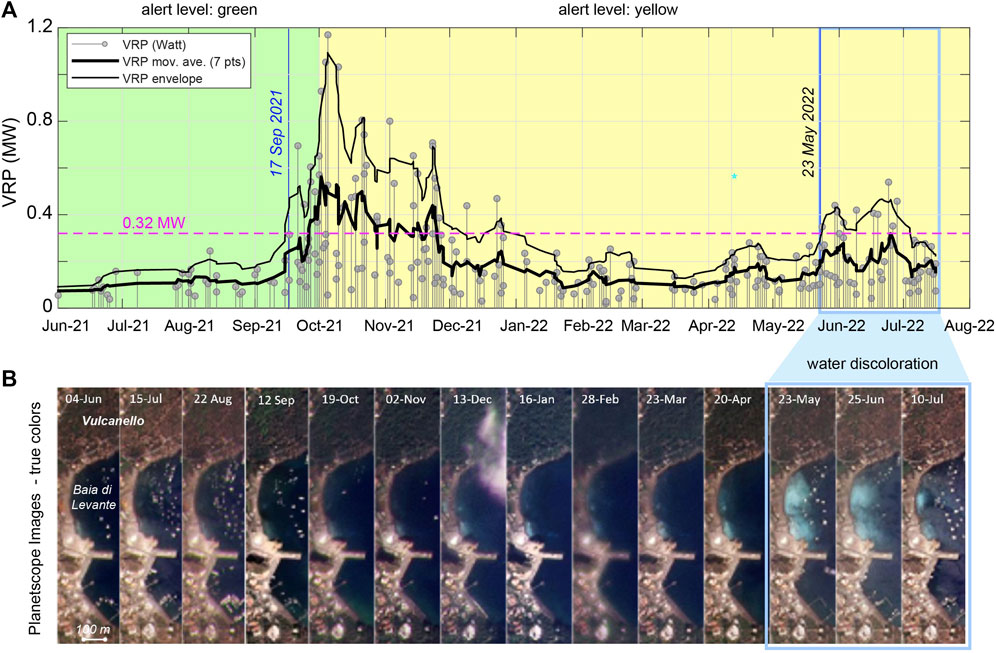
FIGURE 4. Timeseries of (A) VRP (gray stems) recorded between June 2021 and July 2022. The horizontal dashed line defines the statistical threshold of VRP (∼0.3 MW) separating the background from anomaly values. This threshold was overpassed continuously between 17 September and 20 December 2021, in concomitance with the main escalation phase of thermal and degassing unrest. Renewed increase of VRP occurred in May 2022 leading to overpass the threshold on 24 May. (B) One year-long selection of natural color images acquired by the PlanetScope satellites over the Baia di Levante (Image credits: Planet Team, 2017). During May-July 2022, in concomitance with the second thermal pulse recorded in the crater area, the Baia di Levante experienced a period of water discoloration (turned milky white) associated to underwater degassing (INGV weekly Report 2022a).
The escalating signs of volcanic unrest led the Civil Protection Department (DPC) to switch from the green alert level (the volcano is in a state of quiescence) to the yellow one (state of minor superficial hydrothermal crisis of the volcano) on October 1st (Dipartimento della Protezione Civile, 2021).
A few days later, on 5 October, the VRP reached a peak of ∼1.17 MW (Figure 4A), after which a slight decrease started to be observed, in agreement with a gradual reduction of the seismicity, deformation, and CO2 fluxes in soils (INGV report 2021). Between February and April 2022 the VRP decreased to values just above the baseline, albeit with a frequency of ∼0.7 alerts/day, which is about 7 times higher than the typical detection rate during the baseline activity.
A new increase in VRP begins to be recorded in April 2022 (Figure 4A) and was followed, on 22–23 May, by an anomalous degassing event from underwater hydrothermal vents in the Baia di Levante (INGV report 2022a; Figure 4B). This event caused the discoloration of the seawater, clearly visible on the PlanetScope images (Planet Team, 2017) acquired on 23 May (Figure 4B). This second thermal pulse reached the peak on 24 June (∼0.54 MW) and then decreased in the first weeks of July. At the time of this writing (15 July) frequent thermal anomalies are still detected with VRP decreasing below 0.3 MW; the discoloration of the water in the Baia di Levante is still present albeit in a smaller area (Figure 4B). Notably, this latter monthly-long thermal pulse and the peripheral submarine degassing event were also accompanied by the resumption of local seismic activity, likely linked to the dynamics of hydrothermal fluids (INGV weekly Report 2022b).
During the 1988–90 crisis anomalous temperature and degassing occurred also outside the crater area, including the Vulcano Porto area (Capasso et al., 1999). A link between central (MFZ) and peripheral (PFZ) heat flux has been also proposed by Chiodini et al. (1991) who found a positive correlation between increased inputs of high-temperature fluids at the crater and increases in temperature and pressure estimated for Baia di Levante fluids. If this model also applies to the current unrest phase, the pulsation observed in May-July 2022 could effectively represent a second pressurization phase with deep fluids affecting both the MFZ and PFZ.
Conclusive remarks and perspective
The unrest of La Fossa continues as this work is elaborated and further, more in-depth analysis will be possible by integrating satellite data with multiparametric measurements carried out on the ground (e.g., Diliberto et al., 2022). These preliminary results demonstrate the effectiveness of VIIRS 375 m data in tracking and quantifying the baseline thermal activity of the La Fossa volcano and promptly detecting any variation from the steady-state conditions, associated with volcanic unrest. These findings are of particular value, especially for remote and poorly-monitored volcanoes, where the satellite data may be the only source of information capable of detecting pre-eruptive signals (Furtney et al., 2018). The current satellite thermal monitoring systems (e.g., MIROVA, MODVOLC) are based on MODIS data whose detection limit (∼1 MW) allows monitoring active volcanoes and tracking ongoing eruptions (Coppola et al., 2020). The use of VIIRS imaging bands, at 375 m resolution, offers new opportunities for monitoring of quiescent volcanoes, as it lowers the detection limit by more than an order of magnitude and enables the detection of smaller and colder anomalies associated with fumarolic fields. We thus suggest that the systematic use of these data on a global scale may contribute to the timely detection and characterization of further unrest worldwide.
Data availability statement
The raw data supporting the conclusions of this article will be made available by the authors, without undue reservation.
Author contributions
CD conceived and wrote the work. CA adapted the MIROVA algorithm to VIIRS data. CD, LM, CA, and MF contributed to develop, maintain and analyzed the MIROVA data in NRT. All authors listed have made a substantial, direct and intellectual contribution to the work, and approved it for publication.
Funding
This research has benefited from funding provided by a collaborative project between the earth Sciences departments of the University of Florence and the University of Turin. This research was partially supported by the Italian Ministry for Universities and Research (MIUR).
Acknowledgments
We thank R. Kazahaya, and an reviewer for the constructive comments on the first version of the manuscript and for the valuable advices that improved the final work. We thank the Associate Editor and the Chief Editor for some fundamental suggestions in the structuring of the work. We acknowledge the LANCE system (http://lancemodis.eosdis.nasa.gov/) for providing Level 1B VIIRS data in near-real time.
Conflict of interest
The authors declare that the research was conducted in the absence of any commercial or financial relationships that could be construed as a potential conflict of interest.
Publisher’s note
All claims expressed in this article are solely those of the authors and do not necessarily represent those of their affiliated organizations, or those of the publisher, the editors and the reviewers. Any product that may be evaluated in this article, or claim that may be made by its manufacturer, is not guaranteed or endorsed by the publisher.
References
Aiuppa, S., Federico, C., Giudice, G., and Gurrieri, S. (2005). Chemical mapping of a fumarolic field: La Fossa crater, Vulcano island (aeolian islands, Italy). Geophys. Res. Lett. 32 (13), L13309. doi:10.1029/2005GL023207
Alparone, S., Cannata, A., Gambino, S., Gresta, S., Milluzzo, V., and Montalto, P. (2010). Timespace variation of volcano-seismic events at La Fossa (Vulcano, aeolian islands, Italy): New insights into seismic sources in a hydrothermal system. Bull. Volcanol. 72, 803–816. doi:10.1007/s00445-010-0367-6
Barberi, F., Neri, G., Valenza, M., and Villari, L. (1991). 1987–1990 unrest at Vulcano. Acta Vulcanol. 1, 95–106.
Braddock, M., Biggs, J., Watson, I. M., Hutchison, W., Pyle, D. M., and Mather, T. A. (2017). Satellite observations of fumarole activity at aluto volcano, Ethiopia: Implications for geothermal monitoring and volcanic hazard. J. Volcanol. Geotherm. Res. 341, 70–83. doi:10.1016/j.jvolgeores.2017.05.006
Campus, A., Laiolo, M., Massimetti, F., and Coppola, D. (2022). The transition from MODIS to VIIRS for global volcano thermal monitoring. Sensors 22 (5), 1713. doi:10.3390/s22051713
Cao, C., Xiong, X., Wolfe, R., DeLuccia, F., Liu, Q., Blonski, S., et al. (2017). Visible infrared imaging radiometer suite (VIIRS) sensor data record (SDR) user’s guide, version 1.3. NOAA Technical Report NESDIS. College Park, MD, USA: NESDIS.
Capasso, G., Favara, R., Francofonte, S., and Inguaggiato, S. (1999). Chemical and isotopic variations in fumarolic discharge and thermal waters at Vulcano island (aeolian islands, Italy) during 1996: Evidence of resumed volcanic activity. J. Volcanol. Geotherm. Res. 88, 167–175. doi:10.1016/S0377-0273(98)00111-5
Caputo, T., Bellucci Sessa, E., Silvestri, M., Buongiorno, M. F., Musacchio, M., Sansivero, F., et al. (2019). Surface temperature multiscale monitoring by thermal infrared satellite and ground images at Campi Flegrei volcanic area (Italy). Remote Sens. (Basel). 11 (9), 1007. doi:10.3390/rs11091007
Chiodini, G., Cioni, R., Guidi, M., and Marini, L. (1991). Geochemical variations at Fossa grande crater fumaroles (Vulcano island, Italy) in summer 1988. Acta Vulcanol. 1, 179–192.
Chiodini, G., Cioni, R., Marini, L., and Panichi, C. (1995). Origin of the fumarolic fluids of Vulcano Island, Italy and implications for volcanic surveillance. Bull. Volcanol. 57, 99–110. doi:10.1007/BF00301400
Chiodini, G., Frondini, F., and Raco, B. (1996). Diffuse emission of CO2 from the Fossa crater, Vulcano island (Italy). Bull. Volcanol. 58, 41–50. doi:10.1007/s004450050124
Chiodini, G., Granieri, D., Avino, R., Caliro, S., Costa, A., and Werner, C. (2005). Carbon dioxide diffuse degassing and estimation of heat release from volcanic and hydrothermal systems. J. Geophys. Res. 110, B08204. doi:10.1029/2004JB003542
Christenson, B. W., Reyes, A. G., Young, R., Moebis, A., Sherburne, S., Cole-Baker, J., et al. (2010). Cyclic processes and factors leading to phreatic eruption events: Insights from the 25 September 2007 eruption through Ruapehu Crater Lake, New Zealand. J. Volcanol. Geotherm. Res. 191, 15–32. doi:10.1016/j.jvolgeores.2010.01.008
Coppola, D., Laiolo, M., Cigolini, C., Delle Donne, D., and Ripepe, M. (2015). Enhanced volcanic hot-spot detection using MODIS IR data results from the Mirova system. Geol. Soc. Lond. Sp. Public. 426, 181–205. doi:10.1144/sp426.5
Coppola, D., Laiolo, M., Cigolini, C., Massimetti, F., Delle Donne, D., Ripepe, M., et al. (2020). Thermal remote sensing for global volcano monitoring experiences from the Mirova system. Front. Earth Sci. 7, 362. doi:10.3389/feart.2019.00362
Coppola, D., Laiolo, M., Piscopo, D., and Cigolini, C. (2013). Rheological control on the radiant density of active lava flows and domes. J. Volcanol. Geotherm. Res. 249, 39–48. doi:10.1016/j.jvolgeores.2012.09.005
De Astis, G., Lucchi, F., Dellino, P., La Volpe, L., Tranne, C. A., Frezzotti, M. L., et al. (2013). Chapter 11 Geology, volcanic history and petrology of Vulcano (central Aeolian archipelago). Memoirs 37, 281–349. Chapter 11. doi:10.1144/m37.11
Di Traglia, F., Pistolesi, M., Rosi, M., Bonadonna, C., Fusillo, R., and Roverato, M. (2013). Growth and erosion: The volcanic geology and morphological evolution of La Fossa (Island of Vulcano, Southern Italy) in the last 1000years. Geomorphology 194, 94–107. doi:10.1016/j.geomorph.2013.04.018
Diliberto, I. S., Cangemi, M., Gagliano, A. L., Inguaggiato, S., Jacome Paz, M. P., Madonia, P., et al. (2021). Volcanic gas hazard assessment in the Baia di Levante area (Vulcano Island, Italy) inferred by geochemical investigation of passive fluid degassing. Geosciences 11 (11), 478. doi:10.3390/geosciences11110478
Diliberto, I. S. (2017). Long-term monitoring on a closed-conduit volcano: A 25 year long time-series of temperatures recorded at La Fossa cone (Vulcano island, Italy), ranging from 250 °C to 520 °C. J. Volcanol. Geotherm. Res. 346, 151–160. doi:10.1016/j.jvolgeores.2017.03.005
Diliberto, I. S. (2011). Long-term variations of fumaroles temperatures on Vulcano Island (Italy). Ann. Geophys. 54, 175–185. doi:10.4401/ag-5183
Di Martino, R. M. R., Capasso, G., Camarda, M., De Gregorio, S., and Prano, V. (2020). Deep CO2 release revealed by stable isotope and diffuse degassing surveys at Vulcano (Aeolian Islands) in 2015–2018. J. Volcanol. Geotherm. Res 401, 106972. doi:10.1016/j.jvolgeores.2020.106972
Diliberto, I. S., Pailot Bonnètat, S., Harris, A. J. L., Bani, P., Rafflin, V., Boudoire, G., et al. (2022). “The 2021 unrest at Vulcano: Insights from ground-based and satellites observations,” in EGU General Assembly 2022, Vienna, Austria, 23–27 May 2022. EGU22-11576. doi:10.5194/egusphere-egu22-11576
Dipartimento della Protezione Civile, (2021). Press release from the Italian Civil protection department, volcano risk section. Available at: https://www.protezionecivile.gov.it/it/comunicato-stampa/01ott21-allerta-gialla-vulcano-0 [Accessed November 14, 2021].
Federico, C., Capasso, G., Paonita, A., and Favara, R. (2010). Effects of steam-heating processes on a stratified volcanic aquifer: Stable isotopes and dissolved gases in thermal waters of Vulcano Island (Aeolian archipelago). J. Volcanol. Geotherm. Res. 192, 178–190. doi:10.1016/j.jvolgeores.2010.02.020
Frazzetta, G., La Volpe, L., and Sheridan, M. F. (1983). Evolution of the Fossa cone, Vulcano. J. Volcanol. Geotherm. Res. 17, 329–360. doi:10.1016/0377-0273(83)90075-6
Furtney, M. A., Pritchard, M. E., Ebmeier, S. K., Jay, J. A., Carn, S. A., McCormick Kilbride, B. T., et al. (2018). Synthesizing multi-sensor, multi-satellite, multi-decadal data sets for global volcano monitoring. J. Volcanol. Geotherm. Res. 365, 38–56. doi:10.1016/j.jvolgeores.2018.10.002
Granieri, D., Avino, R., Carapezza, M. L., Chiodini, G., Ranaldi, M., Ricci, T., et al. (2006). Correlated increase in CO2 fumarolic content and diffuse emission from La Fossa crater (Vulcano, Italy): Evidence of volcanic unrest or increasing gas release from a stationary deep magma body? Geophys. Res. Lett. 33, L13316. doi:10.1029/2006GL026460
Harris, A. J. L., Alparone, S., Bonforte, A., Dehn, J., Gambino, S., Lodato, L., et al. (2012). Vent temperature trends at the Vulcano Fossa fumarole field: The role of permeability. Bull. Volcanol. 74, 1293–1311. doi:10.1007/s00445-012-0593-1
Harris, A. J. L., Lodato, L., Dehn, J., and Spampinato, L. (2009). Thermal characterization of the Vulcano fumarole field. Bull. Volcanol. 71, 441–458. doi:10.1007/s00445-008-0236-8
Harris, A. J. L., and Maciejewski, A. J. H. (2000). Thermal surveys of the Vulcano Fossa fumarole field 1994–1999: Evidence for fumarole migration and sealing. J. Volcanol. Geotherm. Res. 102, 119–147. doi:10.1016/S0377-0273(00)00184-0
Harris, A. J. L., and Stevenson, D. S. (1997b). Magma budgets and steady-state activity of Vulcano and Stromboli. Geophys. Res. Lett. 24 (9), 1043–1046. doi:10.1029/97GL00861
Harris, A. J. L., and Stevenson, D. S. (1997a). Thermal observations of degassing open conduits and fumaroles at Stromboli and Vulcano using remotely sensed data. J. Volcanol. Geotherm. Res. 76, 175–198. doi:10.1016/S0377-0273(96)00097-2
Harris, A. J. L. (2013). Thermal remote sensing of active volcanoes: A user's manual. Cambridge: Cambridge University Press.
Inguaggiato, S., Vita, F., Diliberto, I. S., Mazot, A., Calderone, L., Mastrolia, A., et al. (2022). The extensive parameters as a tool to monitoring the volcanic activity: The case study of Vulcano island (Italy). Remote Sens. (Basel). 14, 1283. doi:10.3390/rs14051283
INGV weekly Reports (2021). Bollettini settimanali INGV Vulcano (12102021). Available at: https://cme.ingv.it/bollettini-e-comunicati/bollettini-settimanali-vulcano [Accessed July 2022].
INGV weekly Reports (2022a). Bollettino settimanale Vulcano (20220531). Available at: https://cme.ingv.it/bollettini-e-comunicati/bollettini-settimanali-vulcano [Accessed July 2022].
INGV weekly Reports (2022b). Bollettino settimanale Vulcano (20220712). Available at: https://cme.ingv.it/bollettini-e-comunicati/bollettini-settimanali-vulcano [Accessed 2022].
Kazahaya, R., Shinohara, H., Mori, T., Iguchi, M., and Yokoo, A. (2016). Pre-eruptive inflation caused by gas accumulation: Insight from detailed gas flux variation at sakurajima volcano, Japan. Geophys. Res. Lett. 43, 11219–11225. doi:10.1002/2016GL070727
Kazahaya, R., Shinohara, H., Ohminato, T., and Kaneko, T. (2019). Airborne measurements of volcanic gas composition during unrest at Kuchinoerabujima volcano, Japan. Bull. Volcanol. 81, 7. doi:10.1007/s00445-018-1262-9
Madonia, P. (2020). Variations of low temperature fumaroles as a tool for detecting changes in volcanic activity state: A brief overview. Adv. Geosci. 52, 97–103. doi:10.5194/adgeo-52-97-2020
Mannini, S., Harris, A. J. L., Jessop, D. E., Chevrel, M. O., and Ramsey, M. S. (2019). Combining ground-and ASTER-based thermal measurements to constrain fumarole field heat budgets: The case of Vulcano Fossa 2000-2019. Geophys. Res. Lett. 46, 11868–11877. doi:10.1029/2019GL084013
Mercalli, G. (1907). I vulcani attivi della terra: Morfologia-Dinamismo-Prodotti-Distribuzione geografica-cause. Nature 76, 291. Con 82 Incisioni e 26 Tavle. U. Hoepli.
Montalto, A. (1996). Signs of potential renewal of eruptive activity at La Fossa (Vulcano, Aeolian Islands). Bull. Volcanol. 57, 483–492. doi:10.1007/BF00304434
Mori, T., Morita, M., and Iguchi, M. (2017). Sulfur dioxide flux monitoring using a regular service ferry after the 2014 eruption of Kuchinoerabujima Volcano, Japan. J. Nat. Disaster Sci. 38 (1), 105–118. doi:10.2328/jnds.38.105
Mothes, P. A., Ruiz, M. C., Viracucha, E. G., Ramón, P. A., Hernández, S., Hidalgo, S., et al. (2017). “Geophysical footprints of cotopaxi’s unrest and minor eruptions in 2015: An opportunity to test scientific and community preparedness,” in Volcanic unrest. Advances in Volcanology. Editors J. Gottsmann, J. Neuberg, and B. Scheu (Cham: Springer). doi:10.1007/11157_2017_10
Müller, D., Bredemeyer, S., Zorn, E., De Paolo, E., and Walter, T. R. (2021). Surveying fumarole sites and hydrothermal alteration by unoccupied aircraft systems (UAS) at the La Fossa cone, Vulcano Island (Italy). J. Volcanol. Geotherm. Res. 413, 107208. doi:10.1016/j.jvolgeores.2021.107208
Paonita, A., Federico, C., Bonfanti, P., Capasso, G., Inguaggiato, S., Italiano, F., et al. (2013). The episodic and abrupt geochemical changes at La Fossa fumaroles (Vulcano Island, Italy) and related constraints on the dynamics, structure, and compositions of the magmatic system. Geochim. Cosmochim. Acta 120, 158–178. doi:10.1016/j.gca.2013.06.015
Planet Team (2017). Planet application program interface: In space for life on Earth. San Francisco, CA. https://api.planet.com.
Ramsey, M. S., and Flynn, I. T. W. (2020). The spatial and spectral resolution of ASTER infrared image data: A paradigm shift in volcanological remote sensing. Remote Sens. (Basel). 12, 738. doi:10.3390/rs12040738
Ramsey, M. S., Harris, A. J., and Watson, I. M. (2021). Volcanology 2030: Will an orbital volcano observatory finally become a reality? Bull. Volcanol. 84, 6. doi:10.1007/s00445-021-01501-z
Reath, K. A., Pritchard, M. E., Moruzzi, S., Alcott, A., Coppola, D., and Pieri, D. (2019). The AVTOD (ASTER volcanic thermal output database) Latin America archive. J. Volcanol. Geotherm. Res. 376, 62–74. doi:10.1016/j.jvolgeores.2019.03.019
Reath, K. A., Ramsey, M. S., Dehn, J., and Webley, P. W. (2016). Predicting eruptions from precursory activity using remote sensing data hybridization. J. Volcanol. Geotherm. Res. 321, 18–30. doi:10.1016/j.jvolgeores.2016.04.027
Ricci, T., Finizola, A., Barde-Cabusson, S., Delcher, E., Alparone, S., Gambino, S., et al. (2015). Hydrothermal fluid flow disruptions evidenced by subsurface changes in heat transfer modality: The La Fossa cone of Vulcano (Italy) case study. Geology 43 (11), 959–962. doi:10.1130/G37015.1
Selva, J., Bonadonna, C., Branca, S., De Astis, G., Gambino, S., Paonita, A., et al. (2020). Multiple hazards and paths to eruptions: A review of the volcanic system of Vulcano (aeolian islands, Italy). Earth. Sci. Rev. 207, 103186. doi:10.1016/j.earscirev.2020.103186
Silvestri, M., Rabuffi, F., Pisciotta, A., Musacchio, M., Diliberto, I. S., Spinetti, C., et al. (2019). Analysis of thermal anomalies in volcanic areas using multiscale and multitemporal monitoring: Vulcano island test case. Remote Sens. (Basel). 11, 134. doi:10.3390/rs11020134
Vaughan, R. G., Lowenstern, J. B., Keszthelyi, L. P., Jaworowski, C., and Heasler, H. (2012). Mapping temperature and radiant geothermal heat flux anomalies in the Yellowstone geothermal system using ASTER thermal infrared data. Trans. Geotherm. Resour. Counc. 36 (2), 1403–1409.
Wadge, G., and Aspinall, W. P. (2014). Chapter 24 A review of volcanic hazard and risk-assessment praxis at the Soufrière Hills Volcano, Montserrat from 1997 to 2011. Memoirs 39, 439–456. doi:10.1144/M39.24
Wadge, G., Voight, B., Sparks, R. S. J., Cole, P. D., Loughlin, S. C., and Robertson, R. E. A. (2014). Chapter 1 an overview of the eruption of soufrière hills volcano, Montserrat from 2000 to 2010. Memoirs 39, 1–40. doi:10.1144/M39.1
Way, L., Pritchard, M. E., Wike, L., Reath, K., Gunawan, H., Prambda, O., et al. (2022). Detection of thermal features from space at Indonesian volcanoes from 2000 to 2020 using ASTER. J. Volcanol. Geotherm. Res. 430, 107627. doi:10.1016/j.jvolgeores.2022.107627
Wooster, M. (2003). Fire radiative energy for quantitative study of biomass burning derivation from the bird experimental satellite and comparison to modis fire products. Remote Sens. Environ. 86, 83–107. doi:10.1016/s0034-4257(03)00070-1
Keywords: volcanic unrest, la Fossa crater (vulcano island), satellite, thermal monitoring, VIIRS
Citation: Coppola D, Laiolo M, Campus A and Massimetti F (2022) Thermal unrest of a fumarolic field tracked using VIIRS imaging bands: The case of La fossa crater (Vulcano Island, Italy). Front. Earth Sci. 10:964372. doi: 10.3389/feart.2022.964372
Received: 08 June 2022; Accepted: 02 August 2022;
Published: 25 August 2022.
Edited by:
Yosuke Aoki, The University of Tokyo, JapanReviewed by:
Ryunosuke Kazahaya, Geological Survey of Japan (AIST), JapanGaetana Ganci, Section of Catania, Italy
Copyright © 2022 Coppola, Laiolo, Campus and Massimetti. This is an open-access article distributed under the terms of the Creative Commons Attribution License (CC BY). The use, distribution or reproduction in other forums is permitted, provided the original author(s) and the copyright owner(s) are credited and that the original publication in this journal is cited, in accordance with accepted academic practice. No use, distribution or reproduction is permitted which does not comply with these terms.
*Correspondence: D. Coppola, diego.coppola@unito.it