- 1Department of Earth and Environmental Sciences, University of Milano-Bicocca, Milano, Italy
- 2Conisma Local Research Unit, University of Milano-Bicocca, Milano, Italy
- 3Department of Materials Science, University of Milano-Bicocca, Milano, Italy
- 4DiSTAV, Università di Genova, Genova, Italy
The most important reef of the Mediterranean is the Coralligène (Coralligenous = C), including several types of calcareous algal-invertebrate build-ups growing in normal open marine conditions. We analyzed and compared two C samples from the Ligurian Sea developed in different environmental settings: 1) off Portofino on a rocky cliff, at a depth of about 40 m and 2) in front of Bogliasco, on a sub-horizontal substrate at a depth of 10 m. The maximum AMS radiocarbon dating provided an older age for Bogliasco (about 5 ka BP) than for Portofino (about 3.6 ka BP), and the mean accumulation rate of the Portofino build-up (about 80 µm y−1) was found to be higher than the one in Bogliasco (about 65 µm y−1). The different sides of each build-up showed a remarkable heterogeneity in the dominant cover by living organisms, and the comparison between the two build-ups highlighted an evident diversity in their taxonomic composition and structure, although crustose coralline algae (CCA) are the dominant framework builder and major autogenic ecosystem engineers at both localities, in the present as in the past millennia. Other major components of the structure are bryozoans and serpulids, and an important role is played by sediment filling. In Bogliasco, extreme climate events and major peaks of fine matrix and terrigenous grains are observed, lithologically related to the drainage basin of the Poggio creek and associated with charophyte occurrence and reduced CCA abundance. The occurrence of the rare Sporolithon ptychoides was observed both in Portofino at about 750 BCE and in Bogliasco. These Sporolithon phases are likely related to warm and humid spells punctuating the Holocene climate fluctuations in the Ligurian Sea. Because coralline algae are confirmed to be the most important habitat engineer of the Mediterranean reefs, they deserve more attention in the framework of any monitoring initiative aimed at C management and conservation.
1 Introduction
Coralline algae are autogenic habitat engineers responsible for the formation of intertidal algal rims, subtidal rhodoliths, and algal reefs known in the Mediterranean as Coralligène (Coralligenous = C). Coralligenous is known in the Mediterranean as a hard biogenic substrate mainly produced by the superposition of several generations of calcareous red algae, living in dim light conditions. In the framework of marine benthic bionomics, C is identified as the ecological climax stage for the Mediterranean circalittoral zone (Pérès, 1982), although further studies recognized also C in dim-light infralittoral settings (Ballesteros, 2006; Bracchi et al., 2016).
The C bio-constructions grew at a water depth of 20–140 m (Laborel 1961, 1987) by in situ superposition of carbonate skeletal material, produced during the last Holocene sea level rise (Sartoretto et al., 1996). Coralligenous is a heterogeneous habitat (Sartoretto, 2017) and a prominent biogeological system of the Mediterranean seascape (Ballesteros, 2006; Casas-Guell et al., 2016; Bracchi et al., 2017; Pierdomenico et al., 2021), a unique hot spot of marine biodiversity (Laubier, 1966; Ballesteros, 2006; Di Iorio et al., 2021; Piazzi et al., 2021), a major carbonate factory and storage (Canals and Ballesteros, 1997; Cebrian et al., 2000; Marchese et al., 2020), and a target of EU environmental protection, due to the ecosystem services that it provides and its sensitivity to direct and indirect human impacts, including the ongoing climate change (among others: Balata et al., 2005; Martin and Gattuso, 2009; Piazzi et al., 2011; Gatti et al., 2015; Montefalcone et al., 2017; Costanzo et al., 2021).
C includes several types of open marine algal-invertebrate reefs, a few centimeters to several meters in thickness, which can be described in terms of benthic zonation, build-up morphology, type of basal substrate, style of development, and components (Pérès 1982; Laborel 1987; Bracchi et al., 2017; Bracchi et al., 2019). Several species of calcareous red algae Corallinophycidae (crustose coralline algae, CCA) and some calcareous Peyssonneliales are characteristic habitat-engineers (Laubier 1966; Pérès 1982; Ballesteros, 2006; Rosso and Sanfilippo 2009; Ponti et al., 2011; Boudouresque et al., 2016; De Jode et al., 2019) and the volumetrically most important fossil framework builders in C (Basso et al., 2007; Titschack et al., 2008; Bracchi et al., 2014, 2016, 2022). Other important builders are bryozoans, polychaetes, and cnidarians (Ballesteros 2006; Bellan-Santini et al., 2007; Corriero et al., 2019), and bryozoans producing large, long-lived, habitat-forming colonies may be primary builders (Cocito, 2004; Di Geronimo et al., 2002; Novosel et al., 2004; Rosso and Sanfilippo 2009). The coralligenous habitat has been defined as an eco-ethological crossroads and an assemblage of several communities rather than a unique community (Sartoretto, 2017), and several different associations and facies have been described under the term “coralligenous” (Ballesteros, 2006; Casas-Güell et al., 2016; Sartoretto, 2017; Corriero et al., 2019). The most obvious and coarse distinction is between C developing on rocky walls and C developing from subhorizontal substrates (sedimentary or rocky) (Pérès 1982; Bracchi et al., 2017; Sartoretto, 2017; Piazzi et al., 2009; Piazzi et al., 2022). The interplay of biotic and abiotic factors (mainly light exposure, availability of trophic resources, substrate exposure, sedimentation rate, freshwater influence, and biotic interactions) controls the several facies of C, unevenly distributed along geographical and depth gradients (among others: Gili and Ros 1985; Sartoretto, 1994; Garrabou et al., 2002; Di Geronimo et al., 2001; Virgilio et al., 2006; Casellato and Stefanon, 2008; Ponti et al., 2011; Falace et al., 2015; Çinar et al., 2020).
Our knowledge about C accumulation rate and age, although very fragmentary (Sartoretto et al., 1996; Bertolino et al., 2017a; Sartoretto, 2017), suggests that present-day conspicuous algal build-ups required thousands of years to form, depending on favorable combinations of biologically mediated carbonate precipitation by algal engineers, persistence of compatible oceanographic conditions, and sedimentation rate, in turn controlled by the overarching geological setting. Radiocarbon dating has revealed that the accumulation rate of the C build-ups is very low (0.006–0.83 mm y−1). It has been suggested that after 6,000 years BP, the accretion rate of deep C, below a depth of 60 m, could have been very low to nil, possibly because of the increasingly unfavorable environmental conditions during Holocene sea level rise (Sartoretto et al., 1996) or the human pressures which strongly affected the species living on the surface of the biogenic build-ups (Montefalcone et al., 2017; Zunino et al., 2019; Gómez-Gras et al., 2021). For example, the increase in turbidity, burial, and sediment deposition represents threats to coralligenous assemblages, leading to a shift in the community structure and influencing its spatial and temporal variability (Bourcier, 1986; Balata et al., 2005).
Increasing atmospheric CO2 concentration is leading to global warming, marine pH decrease, and lower carbonate saturation state (Sarmiento et al., 1998; IPCC 2022). High-Mg calcite CCA and aragonite skeletal remains of invertebrates are the most soluble carbonates to respond to ocean acidification (Hall-Spencer et al., 2008; Kuffner et al., 2008; Rodolfo-Metalpa et al., 2010; Basso, 2012), and in the meantime, both chemical and biogenic erosion of marine carbonates will be enhanced by marine acidification (Wisshak et al., 2014). Predictions of future habitat evolution require knowledge of former climates and can be modeled on the basis of long time-series and information recorded in the recent geological past (Alverson and Kull 2003; Luterbacher et al., 2012). The coralline-engineered habitats identified as C are structured by a range of physical forces and geobiological processes over geological times and are complex paleoenvironmental archives (Nalin et al., 2006; Titschack et al., 2008; Bracchi et al., 2014, 2016). The aim of this study is to assess the contribution of calcareous autogenic engineers to the present-day Ligurian C growing on rocky wall vs. sub-horizontal substrate, to define its age and mean accumulation rate, and to explore the response of C structural composition and major calcareous bio-engineers to climate and oceanographic Holocene changes potentially recorded in the build-ups.
2 Materials and methods
Coralligenous build-ups were sampled by SCUBA diving from two localities along the Ligurian coast (NW Italy) (Figure 1; Bertolino et al., 2014). One was collected using a hammer and chisel from the base of a submerged vertical cliff in Portofino (P), at a water depth (wd) of 40 m in front of Punta del Faro (Figures 1, 2A; Cánovas Molina et al., 2016, Figure 4). The other one was recovered 150 m off Bogliasco (B) at a water depth (wd) of 10 m (Figures 1, 2B), where it lay on a sub-horizontal seafloor among the Posidonia meadow (Bertolino et al., 2017b, Figure 1).
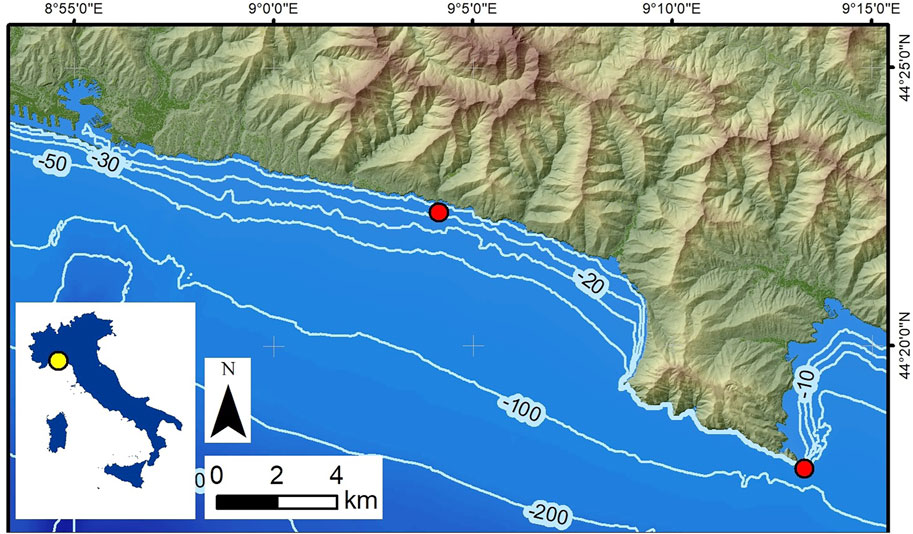
FIGURE 1. Locations of the two sampled sites (red circles). Portofino is bottom right, and Bogliasco is in the centre.
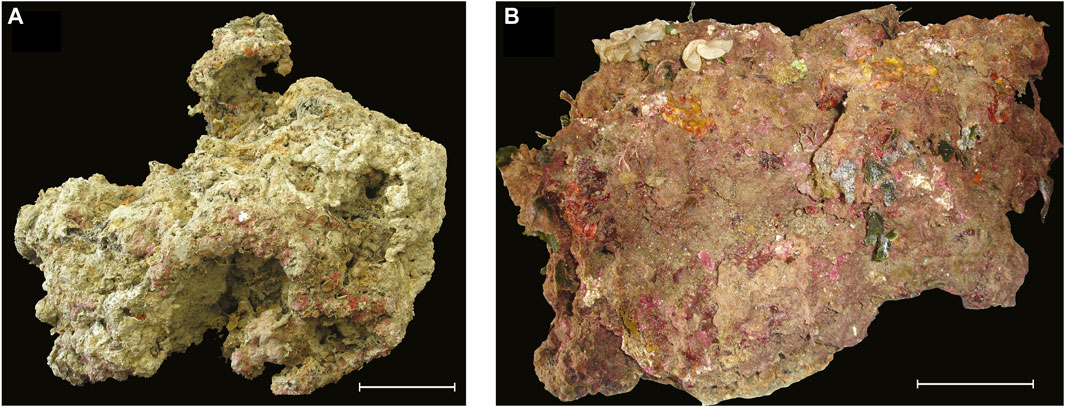
FIGURE 2. Two build-ups before slicing/coring. (A) Portofino side A. (B) Bogliasco side E. Scale is 10 cm.
2.1 Build-up surface cover
The external surface of each build-up was studied in terms of cover and coverage of 12 major categories, based on their visual distinctivity and potential role in bioconstruction: FilF_A, filamentous algae and algal turf <2 cm high (Connell et al., 2014); ErF_A, erect fleshy macroalgae, including Halimeda, Flabellia, and Padina sp.; Pey, other weakly calcified or fleshy laminar algae, mainly Peyssonnelia spp.; CCA_CCPey, non-geniculate Corallinophycidae and completely calcified Peyssonnelia spp; FORAM, encrusting foraminifera; SPNG, sponges; Encr_ ANTH, encrusting corals; Er_ANTH, erect corals; MOL, mollusks; ANN, tube-dwelling annelids; Encr_BRY, encrusting bryozoans; and Er_BRY, erect bryozoans. The acronym CCA to indicate crustose coralline algae is widely used in the scientific literature, although the term crustose is not correct within the accepted nomenclature for coralline growth forms (Woelkerling et al., 1993). For the sake of simplicity, we use the term CCA to indicate the non-geniculate Corallinophycidae, with no implication about their growth-form.
We indicate as cover the percentage of surface occupied by live organisms vs. dead skeletal remains (based on preservation of soft tissues and delicate structures, original sheen, and color). Because the build-up is biogenic, the live + dead covers give 100%. We indicate as coverage the sum of the percentage of surface covered by each category. Because of possible superposition of different organisms, the sum of coverages may exceed 100%. We approximated each build-up to a hexahedron and named the six sides from A to F. Each side was sampled with 15 × 15 cm frames, in order to map the surface and to calculate the percentage cover and the total coverage for each category. Manual mapping of each component has been performed using ImageJ Image Analysis Software (Rasband, 1997). Voucher specimens were detached from the build-up surface for identification at the lowest possible taxonomic level, under an optical microscope and/or a scanning electron microscope (SEM) (soft-bodied and calcareous algae, mollusks, bryozoans, corals, and annelids). Live crustose coralline algae were identified under an SEM following the morphoanatomical keys provided by Irvine and Chamberlain, (1994), Bressan and Babbini (2003) and more recent updates (among others: Basso and Rodondi, 2006; Athanasiadis and Ballantine, 2014; Pezzolesi et al., 2019). Lithophyllum stictiforme is a common species of C encompassing a group of molecularly defined cryptic species (Pezzolesi et al., 2019). For this reason, in absence of molecular data and with the aim to keep a uniform taxonomic framework both for the living and the dead/fossil corallines, this species is indicated here as Lithophyllum group stictiforme. Algal taxonomy follows AlgaeBase (Guiry and Guiry, 2022), and invertebrate taxonomy follows WoRMS (WoRMS Editorial Board, 2019).
2.2 Multivariate statistics
The percentage values of the categories identified in the external cover of the two build-ups were considered separately for each of the 12 surfaces of the build-ups (6 for Portofino and 6 for Bogliasco) and statistically treated using the software Past (Hammer et al., 2001). A Q-mode clustering of the surfaces based on percentage data was performed by Euclidean distance with no preliminary transformation. The same similarity data were used for the ordination of the surfaces by 2D Multidimensional Scaling and Correspondence Analysis.
2.3 Carbonate framework builders
In order to study the internal structure of build-ups, the Portofino sample has been sliced along the apparent growth direction, normal to side A. Nine panoramic thin sections (60 × 45 mm) were prepared from the slice (Figure 3).
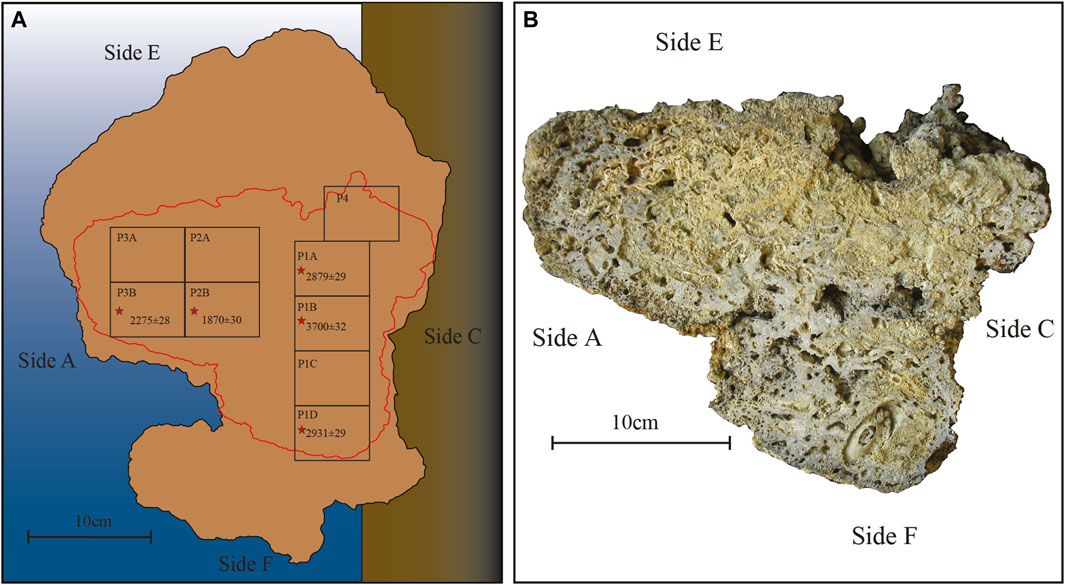
FIGURE 3. Portofino build-up. (A) Reconstruction of the original position with the indication of the different sides indicated by letters. The red line indicates the position of the slice from which the panoramic thin sections (= numbered rectangles) have been obtained. The asterisks indicate the dated thin sections along with their tRC age (BP) (Table 4). (B) The slice before cutting for thin sections, oriented on the base of the build-up sides. Note the large embedded Lithophaga shell close to side F.
The sample of Bogliasco has been cored (Figure 4). Three cores with a diameter of 50 mm and different lengths have been obtained. Core 1 (cm 20) was sampled from side E toward side F, and core 3 (cm 12) joined core 1 from side F. Cores 1 and 3 were more or less perpendicular to core 2. Core 2 had a recovery of 23 cm, from side C toward side A, stopping at 9 cm below the surface of side A. Thus, a total sample thickness of 32 cm (23 cm cored +9 cm not cored) was measured from side C to side A. Cores have been hardened by embedding in resin, in order to avoid fragmentation, and sliced in half. Twenty-one standard petrographic thin sections (48 × 28 mm) have been obtained from the Bogliasco cores (Figure 4).
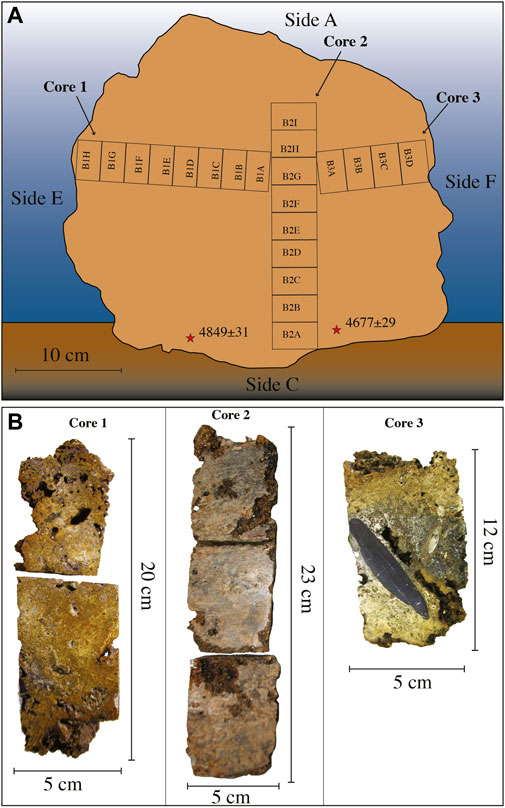
FIGURE 4. Bogliasco build-up. (A) Reconstruction of the original position with the indication of the different sides indicated by letters. The numbered rectangles indicate the position of the thin sections and the asterisks indicate the position of the dated fragments. (B) The Bogliasco cores. In core 3, note the dark layer rich in matrix and terrigenous grains, with a large cobble.
Observation and identification of the build-up components in petrographic thin sections were performed under transmitted-light optical microscopes (an Olympus BH-2 and a Leica Leitz-Laborlux-S) in the facilities of the Milano-Bicocca University. The biological literature has been integrated with paleontological contributions for the identification of fossil algae (Braga and Bassi, 2007; Basso et al., 2009; Hrabovský et al., 2016).
Point counting was performed on a total of 30 thin sections to quantify the contribution of the major coralligenous builders (Flügel, 2010). We analyzed a grid of 100 points for each of the 21 standard petrographic thin sections obtained from the Bogliasco cores and 200 points each for the 9 panoramic thin sections from the Portofino slice. Identified categories in the thin section were as follows: porosity, CCA, geniculate coralline algae, Peyssonneliales, unidentified encrusting algae; Charophyceae; bryozoans; annelids; mollusks; foraminifera; muddy matrix; terrigenous grains. Points corresponding to voids within the buildup framework, such as spaces between different skeletal particles, have been considered and counted as indicative of structural porosity of the build-up, whilst microcavities inside individual skeletal elements, like the interior of conceptacles or annelid tubes, were not considered. We counted as terrigenous grains those extrabasinal elements corresponding to sand grains or larger (>63 µm), while the finer fraction (<63 µm) was attributed to the matrix category, with no distinction about its composition.
2.4 Radiocarbon dating
Radiocarbon dating was performed by the Milano-Bicocca University Centre for Dating and Archaeometry (CUDAM) on selected fragments of CCA from both build-ups, after ascertaining the absence of diagenetic overprints and recrystallization. Five samples for radiocarbon dating were collected from the Portofino slice, numbered RC300, 301, 302, 303, and 304 moving from side F to side E (Figure 3), and 2 samples from the Bogliasco build-up, just internally to the C surface, named RC305 and 306 (Figure 4). The net accumulation rate was assumed as constant and calculated upon the vertical distance between the dated samples (calibrated ages) and the top living surface. We use the term accumulation rate to indicate the net accretion of the build-up that results from the interplay of the original growth rate of the builders, the accumulation of sediment, and the erosion. For the Bogliasco build-up, assuming a more or less constant accumulation rate along the vertical direction, we associated an approximate age to each thin section of the vertical core 2.
3 Results
3.1 Build-up surface cover
3.1.1 Portofino
The build-up sample was a 45 × 37 × 41 cm block, irregularly shaped, with a protuberance toward side F (Figure 2A).
Side C was partially attached to the sub-vertical substrate and had only 32% of living cover. Sides D and F showed a low percentage of living cover, 42% and 43% respectively (Figure 3), whereas sides A, B, and E had the most abundant cover of living organisms, up to 92% (Figure 3; Table 1).
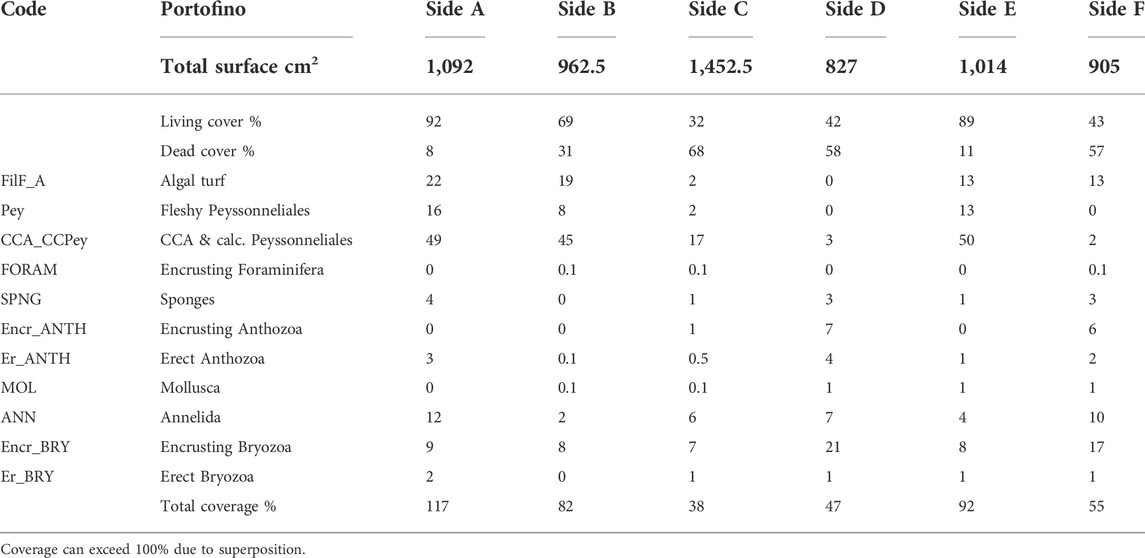
TABLE 1. Percentage values of total superficial cover (live vs. dead) and categories’ coverage on the surface of the Portofino build-up.
Coverage showed the highest percentages on sides A, B, and E (up to 117%, Table 1).
The most abundant organisms were calcareous algae (up to 50%), with the exception of sides D and F where encrusting bryozoan colonies dominated. Filamentous algae were also abundant (up to 22%), while erect fleshy algae were not observed. Bryozoans and, among them, encrusting colonies were abundant. Tube-dwelling annelids reached considerable percentage cover on sides A and F (13 and 10%, respectively) (Supplementary Table S2).
3.1.2 Bogliasco
The build-up sample was a 50 × 40 × 40 cm block, showing a porous surface with cm- to mm-sized irregular cavities (Figure 2B). The block clearly showed a more densely populated portion with a high living cover, corresponding to the sides originally exposed to light (sides A, B, and E, Figure 4), contrasting with the very low living cover of side C, which was partially in contact with the seafloor, and almost devoid of algae. Sides A, B, and E were dominated by filamentous (up to 69%) and subordinate calcareous algae (up to 22%, Table 2). Erect fleshy algae are reported for all sides but were most abundant on sides A (10%) and F (12%). Bryozoans were abundant on side D (14%), whereas annelids are dominant on side C (11%) (Table 2). Anthozoa were not observed (Supplementary Table S2).
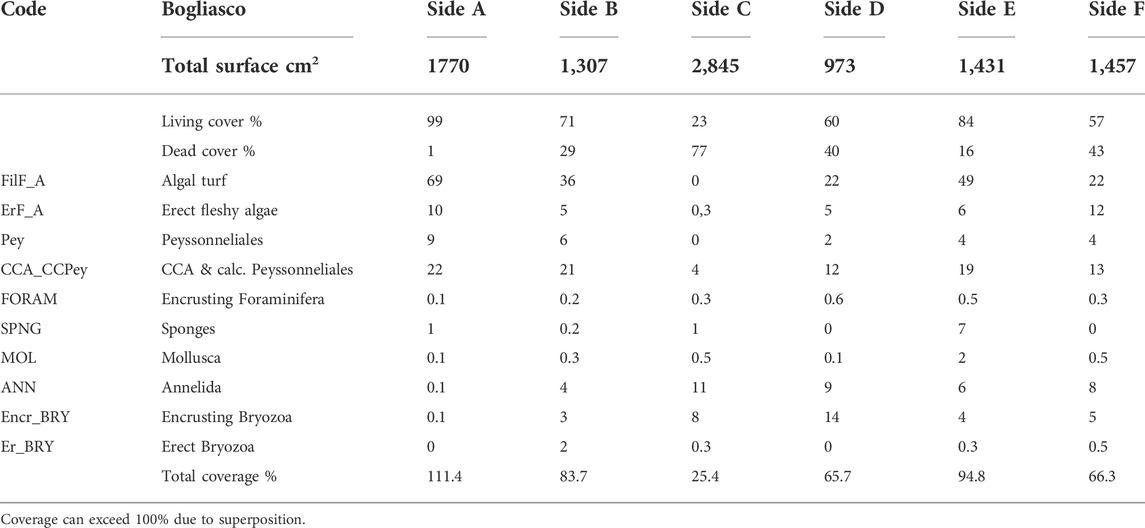
TABLE 2. Percentage values of total superficial cover (live vs. dead) and categories’ coverage on the surface of the Bogliasco build-up.
3.2 Multivariate statistics
The percentages of the identified categories for each side of the two build-ups (Tables 1, 2) were plotted in the dendrogram (Figure 5A). At the Euclidean distance of 35%, three clusters of similar sides are identified (1–3), based on their cover components. Cluster 1 exclusively contains the well-lit sides of Bogliasco, while cluster 3 is composed of the Portofino sides with the highest cover of calcareous algae. The same clusters were recognized in the 2D MDS (Euclidean distance, no transformation, Figure 5B). Cluster 2 contains the remaining sides, highlighting a similarity (only 15% distance) between the “dark” sides C of both build-ups.
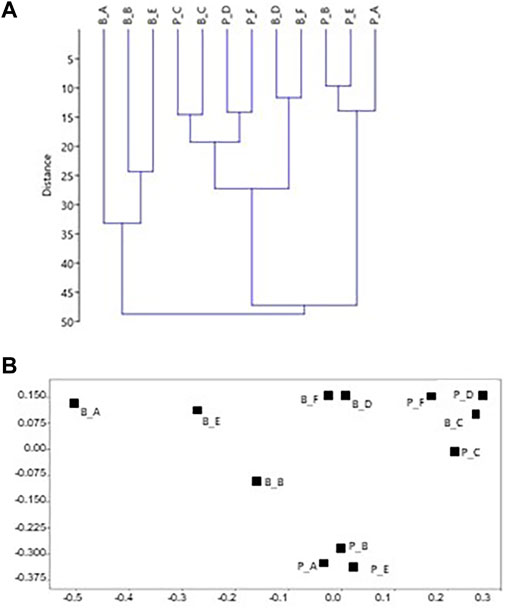
FIGURE 5. Plots of the statistical treatment based on surface coverage data from the two build-ups. Each side is labeled with a first letter indicating the build-up (P=Portofino and B=Bogliasco) with another letter indicating the side. For example, P_A is side A of Portofino build-up. Euclidean distance, no transformation. (A) Dendrogram (B) 2D plot of the ordination by multidimensional scaling. In (B) the bottom and darker sides are plotted together on the top right. Top left is the shallow well-lit side, while the well-lit deep sides are plotted at the bottom center.
The correspondence analysis (CA) of the same data showed the plot of the build-up sides in a space with 3 opposite vertices (Figure 6). The upper left corresponds to erect fleshy algae, calcareous algae are plotted below, and encrusting corals on the upper right, respectively. All of the fleshy algae (turf and filamentous, erect, and laminar Peyssonnelia) fell in the upper left quadrant, while the upper right quadrant is animal-dominated. In the lower half of the CA plot, calcareous algae drive the position of the Portofino sides belonging to cluster 3 (Figures 5B, 6).
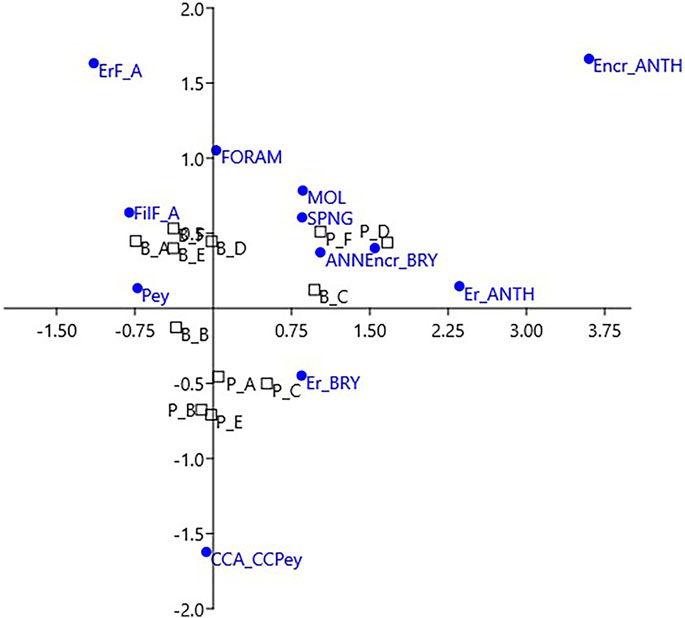
FIGURE 6. Correspondence analysis for the coverage data of the two build-ups. Labels of sides as in Figure 5. Labels of categories as in Tables 1, 2.
3.3 The build-up framework builders
3.3.1 Radiocarbon dating and accumulation rates
Radiocarbon dating of the Portofino build-up provided a calibrated age ranging between 1720 and 1620 BCE (3700 ± 32 years BP, RC301) in the proximity of side F and between 485 and 585 CE (1870 ± 30 years BP, RC303) in the proximity of side E (Supplementary Table S1). The oldest material was recovered from the side of the build-up that was in close proximity of the substrate from which the build-up developed, whereas the youngest portion was located near the photophilous side of the coralligenous block. The mean calculated accumulation rate is 80 μm y−1.
Two separate CCA fragments at the base of the Bogliasco sample (side C, bottom) were dated at 3290–3130 BCE (4849 ± 31 years BP, RC305) and 2985–2895 BCE (4677 ± 29 years BP, RC306). The mean calculated accumulation rate corresponds to 65 μm y−1.
3.3.2 Portofino
Dating the internal structure of the Portofino build-up revealed a complex pattern of growth direction. For this reason, thin sections have been reorganized on the basis of the results of radiocarbon dating, from the oldest one (P1B) to the most recent (P4), the latter obtained next to side E (Figure 3). The inner structure was macroscopically porous but hard and lithified, with cm-thick compact areas. Crustose coralline algae were the dominant component in most sections, ranging from 28% (P3B) to 56% (P2B) (Table 3, Figures 7, 8). In sections P1D and P3B, the most abundant component was matrix (Figure 8), formed by microgranular mud, mainly composed of biogenic fragments. Matrix typically filled the intraskeletal porosity or the interskeletal porosity between adjacent algal crusts.
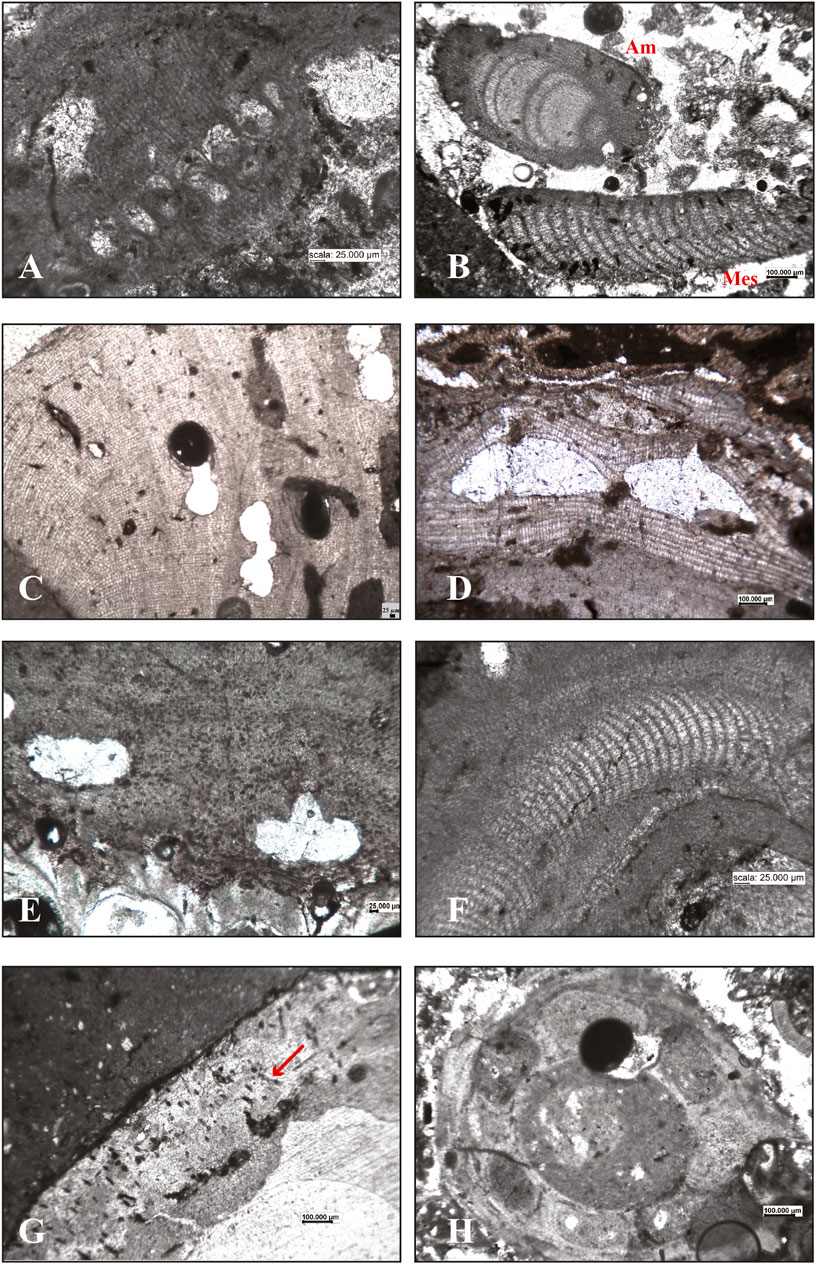
FIGURE 7. Calcareous algae in the internal structure of the studied build-ups. Corallinophycidae: (A) oblique section of solitary sporangia of Sporolithon ptychoides; (B) longitudinal section of Amphiroa intergeniculum (Am) and a fragment of a Mesophyllum sp. hypothallus (Mes); (C) longitudinal section of Lithophyllum gr. stictiforme; (D) longitudinal section of Titanoderma pustulatum showing the distinctive palisade cells and two uniporate conceptacles; (E) Spongites fruticulosus growing on a bryozoan colony; (F) the hypothallus of a Mesophyllum sp. Peyssonneliales: (G) Peyssonnelia sp, hypobasal aragonite calcification showing traces of rhizoids (arrow). Charophyta: (H) unidentified charophyte remains.
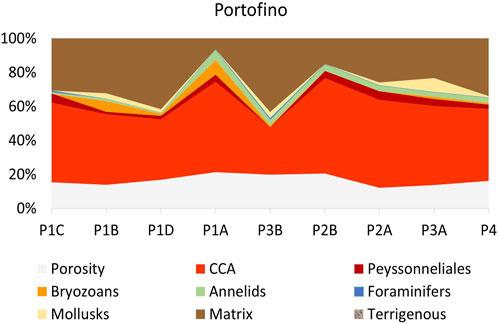
FIGURE 8. Portofino C: internal dominance from thin section point counting of the categories as in Table 3. The label of the studied thin sections is reported on the horizontal axis.
Among the other biogenic components, bryozoans were abundant in sections P1B (6.15%) and P1A (8.51%), annelids had a significant cover in section P1A (6.38%), and mollusks were relatively abundant in section P3A (8.22%) (Table 3, Figure 8). Peyssonneliales and encrusting foraminifera, although present, were never abundant. Porosity was more or less constant, ranging from 13.70% to 21.28% (Table 3, Figure 8).
The most abundant species of coralline algae were Spongites fruticulosus Kützing (up to 16.92% in P1B), Titanoderma pustulatum (J.V.Lamouroux) Nägeli (up to 12.71% in P4), and Mesophyllum cf. philippi (Foslie) Adey (7.68% in P1B) (Table 3). Lithophyllum group stictiforme (12.71%) was identified only in thin section P4, whereas Phymatolithon cf. lenormandii (Areschoug) W.H.Adey (2.74%) was identified only in thin section P2B. Thin section P1D also contained Sporolithon ptychoides Heydrich (11.41%) (Table 3).
3.3.3 Bogliasco
Core 2 was sampled from side C (bottom) to side A (top), almost crossing the whole build-up along its mainly vertical growth direction (Figure 4). The 9 thin sections, named from B2A (bottom) upward to B2I, showed a poor preservation of the calcareous skeletal components, hindering the identification of calcareous algae, which nevertheless remained the dominant biogenic component with up to 57% in thin section B2E (Table 4, Figure 9). Among the identified species, the most common were T. pustulatum and L. gr. stictiforme. S. fruticulosus was identified in thin section B2G, whereas the uncommon S. ptychoides was detected in B2H, associated with T. pustulatum and a significant contribution by bryozoans, annelids, and foraminifera. Uncommon intergenicula of articulated coralline algae occurred randomly (B2E and B2G) (Table 4).
Bryozoans were co-dominant with CCA in thin sections B2F (35.7%) and common elsewhere, with the exception of the CCA-dominated section B2E, where bryozoans were missing. Annelids were abundant in thin section B2G (18.7%), whereas foraminifera were abundant on thin sections B2F (14.3%) and B2H (14.7%) and mollusks on thin sections B2D (39.39%) and B2F (14.3%).
Matrix and terrigenous were variable through the core, absent from section B2F and up to 42.5% in B2B. Section B2G records about 34% of the sedimentary infill associated with the maximum abundance of annelids and the occurrence of charophytes. Porosity varied cyclically along the core (Table 4, Figure 9).
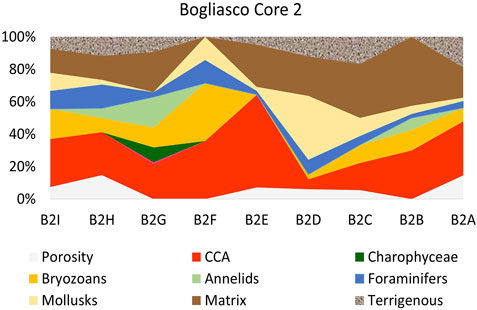
FIGURE 9. Bogliasco C, core 2: internal dominance from thin section point counting of the categories as in Table 4. The label of the studied thin sections is reported on the horizontal axis.
Cores 1 and 3, perpendicular to core 2, have been considered as representative of the presumed lateral development of the build-up (Figure 4). The inner structure was found to be lithified and locally highly compact, although pluricentimetric irregular cavities were observed.
In the thin sections obtained from Core 1, from B1A (internal) to B1H (toward side E) (Figure 4), the most abundant biogenic component was CCA (up to 61.11% in B1A) (Table 5). Among them L. gr. stictiforme, which was always present, and T. pustulatum were the most abundant species (Table 5). The species S. fruticulosus was uncommon, identified only in thin section B1G (Table 5).
Bryozoans were ubiquitous and particularly abundant in thin sections B1D (20%) and B1F, where they overcome CCA abundance (Table 5). Annelids were also common and abundant in thin section B1E, where they equaled CCA (18.2%) and where foraminifers also reached an important percentage. Mollusks were remarkably common in thin section B1C (14.3%), associated with the occurrence of charophytes and abundant sedimentary infill (matrix + terrigenous = 42.8%), while both bryozoans and annelids were missing (Table 5).
Matrix, as microgranular mud mostly of biogenic origin, was very abundant in thin sections B1D (50%), B1C (37.1%), and B1F (35.3%) (Table 5). Terrigenous grains were dominant in thin sections B1G (41.2%) where, adding to matrix, the sum of these two categories reached the maximum value, exceeding 55% (Table 5).
The thin sections of Core 3 (B3A internal, to B3H, close to side F, Figure 4) showed CCA as the most abundant biogenic component, although percentages did not exceed 31.8% (B3A) (Table 5). Bryozoans, annelids, and mollusks remarkably overtook where CCA were absent (B3D). The identified CCA species were L. gr. stictiforme and T. pustulatum.
Matrix was ubiquitous and abundant, and together with terrigenous grains, these two abiogenic components became dominant in thin sections B3A and B3B (Table 5), coarsely corresponding to a gray layer where terrigenous grains up to 6 cm long occurred (Figure 4). The largest fragments showed an elongated shape with well-rounded margins and were composed of calcareous shale.
4 Discussion
4.1 External components
The quantitative analysis of surface cover and coverage of both coralligenous build-ups easily allows us to identify the photophilic and sciaphilic sides, highlighting an important variability in C surface associations, depending on substrate orientation. The Portofino C, overhanging on a rocky wall at a depth of about 40 m, shows the most abundant living cover and coverage on its best-lit sides A and E, with calcareous algae as the dominant category. Filamentous algae, although present, are never dominant, as expected on the basis of the water depth of the sample close to the lower limit of the infralittoral zone in the Mediterranean. An important reduction of the live cover is observed on the other sides, with Bryozoa and tube-dwelling Annelida becoming dominant on the westward side D and on the lower and darkest side F. Both octocorals and Scleractinia are also more common on the dim-lit sides, although with low total dominance.
The Bogliasco C is presently growing within the infralittoral Posidonia meadow, with its upper, best-lit side dominated by filamentous and fleshy algae, with subordinate Peyssonneliales and CCA. Bryozoans are also important contributors, especially on sides D and F, together with annelids, confirming their preference for the shadowed and protected substrates (Rosso and Sanfilippo, 2009; Sanfilippo et al., 2011), including the lower surface of calcareous laminar algae.
The comparison between the overhanging and deeper Portofino C and the shallower Bogliasco build-up highlights the abundant fleshy algal cover and the absence of corals as the most distinctive traits of Bogliasco C, likely due to its shallow position. The distinctive green algae Halimeda tuna and Flabellia petiolata are scattered over the Bogliasco C surface, as commonly reported in other shallow coralligenous algal assemblages (Ballesteros, 2006; Piazzi et al., 2022), while they were not observed in the Portofino sample. Calcareous algae in Bogliasco exhibit about half of the cover in Portofino, confirming their basically sciaphilic nature and the negative effect of space competition with the faster-growing fleshy algae.
The surface of C pillars in Sicily (Di Geronimo et al., 2001) presents a similar pattern of algal dominance, confirming the early reports of shallow-water corallines becoming hidden under a canopy of H. tuna and F. petiolata (Laborel, 1987).
Coralligenous was indicated as the climax biocenosis of the circalittoral zone (Pérès, 1982; Ballesteros, 2006; Cánovas Molina et al., 2016), although C habitats are considered as heterogeneous systems, showing high spatial, morphological, and biological variability (Ferdeghini et al., 2000; Casas-Güell et al., 2015; Cánovas Molina et al., 2016; Sartoretto, 2017; Piazzi et al., 2022). Light exerts a major control on the depth extent of the infralittoral and circalittoral zones and certainly also on the depth distribution of the various coralligenous facies (Pérès, 1982; Ballesteros, 2006; Cánovas Molina et al., 2016). C is reported to develop between 0.05% and 3% of the surface irradiance (Ballesteros, 2006). Irradiance values >3% correspond to the shallowest coralligenous, once defined as “precoralligenous” (Pérès, 1982) because they typically hosted a higher diversity of fleshy algae, among which were Flabellia petiolata and Halimeda tuna.
Coralligenous is known in the circalittoral zone mainly 1) on rocky cliffs, 2) on sedimentary seafloors, and 3) as an enclave of circalittoral, in the shadow of the canopy of leaves at the base of the infralittoral Posidonia meadows (Sartoretto 2017). Portofino C clearly corresponds to the first type, while Bogliasco C is not completely included in category 2) nor 3) above, being developed among Posidonia plants and reaching a considerable height in the middle of the meadow that, by definition, characterizes the infralittoral zone. A similar infralittoral coralligenous, apparently a paradox of benthic bionomics, was reconstructed in the Quaternary marine terrace of Le Castella (MIS 3, Bracchi et al., 2016; Nalin et al., 2020).
4.2 Framework builders and Holocene oceanographic fluctuations
Crustose coralline algae showed a roughly antithetical pattern with matrix, alternating as the dominant components of the C internal structure. Although present and locally abundant, the other biogenic components played a secondary role as framework builders (Figures 8, 9).
4.2.1 Portofino
The interior structure of the Portofino build-up was very compact, with a rather constant percent porosity, never exceeding 21.28%. Among CCA, the most common species belong to the genus Mesophyllum. Contrarily to previous reports indicating M. alternans (junior synonym of M. philippii (Athanasiadis and Ballantine, 2014) as a shallow component (Sartoretto et al., 1996; Ballesteros, 2006), this coralline species was remarkably abundant in the relatively deep Portofino C (40 m).
T. pustulatum was ubiquitous. This species is widespread in the Mediterranean (Babbini and Bressan, 1997) and has already been indicated as an important framework builder in C (Sartoretto et al., 1996). This suggestion was confirmed in the Pleistocene C examples outcropping in the Cutro terraces (Nalin et al., 2006; Basso et al., 2007).
S. fruticulosus is also widespread in the Mediterranean Sea (Babbini and Bressan, 1997; Basso and Rodondi, 2006), where it occurs as rhodoliths on soft bottoms (Basso and Rodondi, 2006; Barberá et al., 2012; Joher et al., 2015), and was rarely reported as a component of the coralligenous build-ups, down to a water depth of 75 m (Basso and Rodondi, 2006; Catra et al., 2007). S. fruticulosus occurred cyclically in the Portofino build-up, around 3700 BP (P1B), 2879 BP (P1A), and 1870 BP (P2B), always together with low matrix abundance. Therefore, it can be associated with periods with low sedimentation rate and consequently interpreted as a species with a low tolerance for disturbance by sediment deposition, and likely associated turbidity.
In the Portofino inner structure, up to 40.43% CCA did not show or preserve sufficient diagnostic features for taxonomic identification. Nevertheless, merging the two components (identified + non-identified coralline algae) highlights CCA as the most important framework builder in late Holocene coralligenous build-up, as already reported for the Ligurian Sea (Sartoretto et al., 1996; Cerrano et al., 2001; Bertolino et al., 2017a; Bertolino et al., 2017b). All the other calcareous organisms showed negligible contributions and rather invariant quantitative distribution in the inner structure. Across the build-up, high CCA abundance was accompanied by a minor matrix, filling the sub-millimeter cavities between crusts. On the contrary, local dominant matrix was observed filling not only the cavities between crusts but also cm-size voids, apparently corresponding to a phase of slow growth of the primary builders. In correspondence of high-matrix content, the most abundant CCA species was T. pustulatum for thin sections P1D and P3B and S. ptychoides for thin section P1D.
Thin section P1D corresponded to a calibrated age of 790–745 BCE which is known as a phase of increasing temperature in the Alboran Sea (Martrat et al., 2014; Bazzicalupo et al., 2020) and increased humidity and terrestrial runoff in the Southern Alps and in the Gulf of Lyon (Wirth et al., 2013; Jalali et al., 2016), associated with negative North Atlantic Oscillation (NAO) index (Faust et al., 2016) (Figures 8, 10). During this time interval, warm summers and dry winters characterized the south-western Mediterranean (Zielhofer et al., 2019). The genus Sporolithon is considered a warm-water taxon (Bressan and Babbini, 2003; Braga and Bassi, 2007; Basso et al., 2009). In particular, the species S. ptychoides is common at tropical latitudes (Adey and Macintyre, 1973; Johansen, 1981), overgrowing coral on shallow fringing reefs and reef flats 1–1.5 m deep (Richards et al., 2017), or in relatively deep water and cryptic sites (Adey, 1979, 1986). S. ptychoides is rarely reported in the Mediterranean; it is mainly reported in the central and southern Tyrrhenian Sea and in the Ionian Sea (Alongi et al., 1996; Babbini and Bressan, 1997; Di Geronimo et al., 2002). The occurrence of S. ptychoides in the Portofino build-up is therefore a possible index of a milder climate than today. The matrix-dominated thin section P3B corresponded to a calibrated age of 85–175 CE, which falls within the early Roman Climate Optimum. Also, this period is reported in literature as warm and humid, supporting terrestrial run off and sediment deposition at coastal sites (Bini et al., 2020).
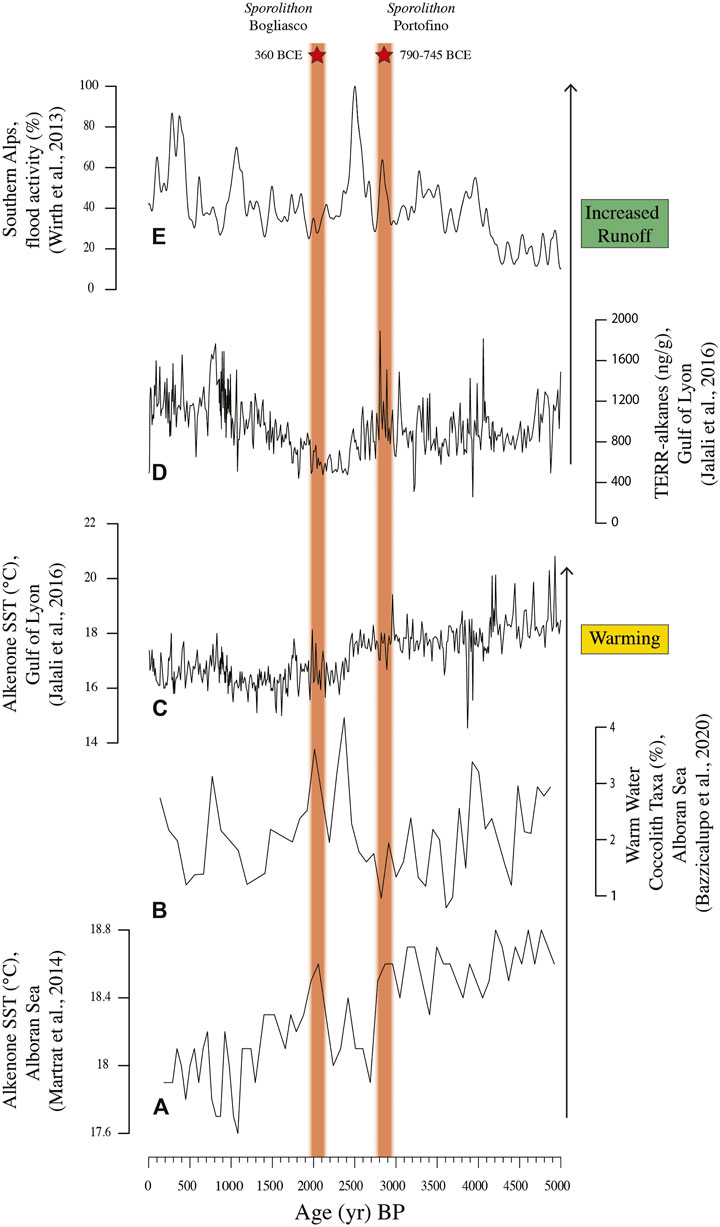
FIGURE 10. Late Holocene climate fluctuations and the stratigraphic position of the Sporolithon occurrences recorded in the Portofino and Bogliasco build-ups.
The calculated accumulation rate of 0.08 mm y−1 for the Portofino C is compatible with previous results from the north-western Mediterranean (Sartoretto et al., 1996).
4.2.2 Bogliasco
The inner structure of the Bogliasco build-up appeared compact, with porosity never exceeding 22.73%. A major contribution of both matrix and terrigenous clasts was reported (up to 42.50% in thin section B2B, Figure 9), as already observed in other shallow coralligenous build-up on horizontal substrate (Cocito et al., 2002). Among CCA, L. gr. stictiforme was the commonest species. The basal side C was consistently dated at 3,290–3130 BCE (RC305) and 2,985–2895 BCE (RC306); thus, the inception of the Bogliasco build-up occurred during the warm Copper Age, about 5 ka BP. At this time, the sea-level was at least 3 m below the present one along the Ligurian coast (Lambeck et al., 2004); therefore, the build-up started to grow at a water depth of about 7 m, in a very shallow infralittoral environment. Although the abundant matrix and terrigenous grains recorded at the base of the build-up are not surprising in this shallow coastal setting (Figure 9), it should be considered that a coincident phase of wet winters has been reported for the southwestern Mediterranean (Zielhofer et al., 2019), probably favoring the development of sedentary heterotrophs such as annelids and boring mollusks, through the increased food supply from continental weathering. Interestingly, the terrigenous and matrix components showed a decrease toward the core top, although the trend is broken in correspondence to thin section B2F, when these sedimentary components abruptly disappear. This seems to correspond to the end of the Late Bronze Age, ca. 1000 BCE. B2F shows the equal contribution by CCA and bryozoans, accompanied by mollusks and foraminifera. This association might have developed during an arid period, recorded in the western Mediterranean by dry winters and warm summers (Jalut et al., 2000; Bond et al., 2001; Zielhofer et al., 2019; Figure 10).
Successively, a wetter climate fostered the freshwater input from the continent and sedimentation in shallow settings. This was testified in the Bogliasco build-up by an increase in annelids and the occurrence of charophyte remains in thin section B2G, at about 400 BCE (Figure 9). Freshwater and sedimentation are not favorable to CCA, which reduced in abundance. The Roman Period (about 250 BCE-400 CE) brought a warmer phase, probably recorded in the overlying thin section B2H, as testified by the occurrence of the warm-water taxon S. ptychoides (Figures 7, 9, 10).
Cores 1 and 3 have been collected more or less normal to core 2. Core 1 crossed core 2 approximately at the level of thin section B2F, thus covering approximately the last 3,000 years, which is compatible with the calculated mean accumulation rate for the Bogliasco build-up.
As already observed in core 2, core 1 also recorded the passage from the drier Late Bronze Age, when CCA were abundant (thin section B1A) to the warm and wetter Roman Period, when sedimentation was favored. This is supported by the abundance of matrix and terrigenous grains in thin sections B1B to B1F, accompanied by the occurrence of charophyte remains (B1C). CCA increased toward the surface of side E in thin sections B1G and B1H.
Core 3 was shorter and recorded a striking and extreme event of sedimentation, likely induced by a flood, with the accumulation of coarse terrigenous clasts up to cobble size (thin sections B3A and B3B). The largest clasts had a rounded shape, supporting the hypothesis of a long transport from the provenance site. The calcareous shale recognized in the largest clasts is compatible with the lithologies of the drainage basin of the Poggio creek, where the Monte Antola Formation outcrops. This Formation is a turbiditic limestone with levels of shales, Upper Cretaceous-Medium Eocene in age (Abbate and Sagri, 1967; Scholle, 1971). The calculated ages of these thin sections were related to the Roman Period.
4.2.3 Build-ups comparison
The inner structure of the build-ups revealed that matrix or biogenic/terrigenous sediments have filled the originally vacuolar framework, resulting in an inner compact structure in both Portofino and Bogliasco build-ups. The overhanging Portofino build-up revealed a complex pattern of growth direction, while the Bogliasco build-up had a more regular vertical accretion. Both build-ups revealed that the most important contributors to the structural development of the rigid framework are CCA. The conservation of calcareous algae was generally poor, despite their very young geological age, and they did not often show the diagnostic features that are required for species identification. This observation opens further questions about the relationship between age and taphonomic signatures in calcareous frameworks. In both build-ups, the genus Titanoderma plays an important role among CCA, although its highly distinctive vegetative anatomy may result in an overestimation of its abundance, in comparison to other corallines. The most common species in the shallow Bogliasco C was L. gr. stictiforme, while the genus Mesophyllum was found to be more common in the deeper Portofino C. A very shallow distribution of L. gr. stictiforme is in agreement with previous observations for the Apulian C (unpublished); however, the observed depth distribution of the two genera show an opposite pattern with respect to other published records for the NW Mediterranean (Sartoretto et al., 1996). In the C from Banyuls (France), distributed from 20 to 40 m in depth, Mesophyllum philippi (as Mesophyllum lichenoides) was reported as the most abundant framework builder, with Lithophyllum stictiforme (as Pseudolithophyllum expansum) also being abundant (Bosence, 1985). However, the paucity of quantitative data suggests caution, and further investigations are required to clarify the matter.
Di Geronimo et al. (2002) reported the occurrence of S. ptychoides inside the Sicilian bio-constructed pillars, although they did not provide any temporal frame for the growth of the bioconstruction. In our samples, S. ptychoides is reported for thin section P1D in Portofino, dated at 790–745 BCE, and for thin section B2H in Bogliasco, indirectly attributed to the Roman Period. Based on literature data, both intervals correspond to phases of warm and rather humid climate in the western Mediterranean. However, the two occurrences of Sporolithon are separated by a few centuries (Figure 10), and the B2H date was calculated on the basis of a presumed constant accumulation rate. Therefore, we cannot exclude the possibility that only one warm “Sporolithon event” occurred in the Ligurian build-ups at about 2.7 ka BP. This age corresponds to a negative NAO Index (Faust et al., 2016), but it should be considered that the Portofino and Bogliasco build-ups grew on the Ligurian continental shelf during the Late Holocene, when the teleconnection between the Western Mediterranean winter rains and the NAO index show an opposite pattern (Jalali et al., 2018; Zielhofer et al., 2019; Figure 10).
The main factors influencing structure, biomass, and metabolism of marine benthic organisms such as CCA are sedimentation-related mechanisms, namely, burial or changes in the quantity/quality of suspended matter and water transparency (Balata et al., 2005), which in turn influence light penetration and may alternatively favor the photoautotrophs or the suspension feeders (Laborel, 1961).
Sedimentation in littoral systems is linked to terrestrial sources and can be affected by natural processes and human activities that have historically altered the coastal erosion/deposition profiles and the transport of suspended particles (Owens, 2020).
Marine sedimentation affects the horizontal rather than the vertical surfaces more easily (Cocito et al., 2002), and shallow-water settings are expected to be more frequently affected by terrigenous input than the deeper settings. In the case of the studied build-ups, differences in the sedimentary regime can be traced between the build-up on the vertical cliff in Portofino and the one from the sub-horizontal substrate in Bogliasco. Most of the Portofino build-up had a contribution higher than 40% by calcareous algae, both on its surfaces and in the inner structure, and minor phases of dominance by annelids and bryozoans, as already reported for build-ups on vertical substrate (Balata et al., 2005).
Portofino build-up contained matrix, as microcrystalline mud with abundant biogenic grains, but no larger terrigenous grains were observed, indicating a minor influence of transport from land.
On the contrary, as expected, the sediment contribution was more abundant in the Bogliasco build-up, both as matrix and as terrigenous fragments, partially inhibiting the surficial coralline algal development, which never exceeds 30.67%. The vicinity of the Bogliasco build-up to the coast also allowed the reconstruction of a past extreme event of flood, recorded inside the bioconstruction as an interval of abundant and large terrigenous clasts lithologically related to the drainage basin of the Poggio creek.
In both build-ups, the identification of levels with abundant calcareous skeletal components alternating with levels of matrix/terrigenous suggests the occurrence of a cyclicity in the formation of the framework.
As already indicated, the reconstruction of C build-up development is not trivial. This is related to the alternation of favorable phases of deposition of carbonate and, in particular, calcareous algae as a major framework builder, and disruption linked to high sedimentation transport that results in shifts of abundance among major components, as already observed for sponge diversity (Bertolino et al., 2017a).
According to many authors (Antonioli et al., 2001; Antonioli et al., 2002; Lambeck et al., 2004; Taricco et al., 2009), the Mediterranean sea level rose from −10 m to −4 m about 6.5 ka BP. The studied area is considered as tectonically stable and the estimated sea-level rise is 0.3 mm y−1 with slight deceleration during the last 1,500 years and stabilization near the present datum from about 500 years BP (Laborel et al., 1994; Pirazzoli, 2005). Therefore, the Portofino build-up did not undergo any significant depth variation, while the Bogliasco one developed at a depth changing from about 7 m, 5 ka BP, to the present water depth of 10 m.
Sartoretto et al. (1996) reported that after 5 ka BP, the accumulation rate of C should be insignificant below 60 m wd. We did not deal with the C growth below 60 m; however, both the studied Ligurian build-ups are younger than 5 ka BP and are slowly but actively growing, as demonstrated by the occurrence of live habitat formers at their surfaces and by their growth rates, which are compatible with published data for other relatively shallow C build-ups (Sartoretto et al., 1996; Bertolino et al., 2017b).
5 Conclusion
Coralligenous build-ups are among the most important biogenic frameworks of the Mediterranean shelf (Ingrosso et al., 2018), characterized by slow but continuous growth on both vertical and horizontal substrates.
The quantitative analyses of the major components at the surface of the two studied samples of C, collected in the Ligurian Sea, show an important variability depending on orientation and exposure (Figures 5, 6). The abundant fleshy algal cover and the absence of corals are the most distinctive traits of Bogliasco C when compared with Portofino (Figures 5, 6). Calcareous algae in Bogliasco exhibit about half of the cover in Portofino, confirming their basically sciaphilic nature and the negative effect of higher sedimentation and space competition with the faster-growing fleshy algae, resulting in a lower accumulation rate of the Bogliasco build-up. Surface and internal components indicate that coralline algae and completely calcified Peyssonneliales represent the most important framework builders, with the genus Lithophyllum apparently more common in the shallow Bogliasco C, while Mesophyllum plays a major role in the deeper Portofino C. Bryozoans are the second most significant contributor to the C framework, locally showing an opposite trend of abundance with respect to coralline algae.
The studied build-ups developed during the Late Holocene, recording its numerous climate fluctuations. Matrix and terrigenous fragments are negatively correlated with calcareous algae, due to the detrimental effect of a high sedimentation rate on the growth of these photosynthetic organisms. Therefore, we recognize a cyclicity in the inner structure of both build-ups, with the framework composition shifting from calcareous algae-dominated to matrix-dominated. These shifts correspond to Late Holocene climate fluctuations, and in particular, matrix-dominated intervals are correlated with wetter periods, under which sediment transport from the continent is favored, whereas calcareous algae-dominated intervals correspond to drier spells. Coralligenous build-ups are special recorders, able to catch extreme events of flooding and the occurrence of warm “Sporolithon events.”
While the internal structure of the Bogliasco build-up is completely coherent with the definition of Coralligenous, its present-day shallow distribution, growing amid Posidonia, documents an important exception to the rule. In fact, this supports the concept that C is not only the climax of the circalittoral zone, also occurring in the enclave at the base of Posidonia leaves, but it may also develop and gain significant vertical accretion within the infralittoral zone (Ballesteros, 2006; Bracchi et al., 2016). A mosaic of benthic biocoenoses is expected to occur across ecological boundaries, and further investigations are required to clarify the controls of this spatial pattern.
The occurrence of live calcifiers at the surface of the studied build-ups and their accumulation rates of 0.080 mm y−1 for Portofino and 0.065 mm y−1 for Bogliasco demonstrate that the Ligurian C is slowly but actively growing.
Physical forces and geological and biological processes controlling the build-ups act at variable temporal and spatial scales, which explains the disparate disciplinary and technical approaches displayed for their exploration. The consequence, however, is an insufficient interdisciplinary communication leading to a fragmented and incomplete understanding of the phenomenon of bioconstruction, an inadequate short-term vision, and poor management of these important habitats. Brief, local investigations, and those monitoring methods based exclusively on visual census of the mega-epibenthos presently dwelling on the algal substrate, appear largely insufficient for describing the diverse expressions of the coralline-engineered habitats as historical structures, and should be integrated in the framework of a geobiological approach. Adding the correct temporal and spatial frame has important implications for our understanding of the history and fate of marine temperate/cold biogenic habitats under the ongoing human impacts, ocean warming, and acidification and for improving their management.
Data availability statement
The original contributions presented in the study are included in the article/Supplementary Material; further inquiries can be directed to the corresponding author.
Author contributions
Conceptualization, DB; methodology, DB, VB, and FM; formal analysis, DB and VB; investigation, DB, VB, FM, and GB; data curation, VB, DB, FM, and PB; writing—original draft preparation, DB and VB; writing—review and editing, DB, VB, PB, and GB; supervision, DB; project administration, DB; funding acquisition, DB. All authors have read and agreed to the published version of the manuscript.
Funding
Project “CRESCIBLUREEF—Grown in the blue: new technologies for knowledge and conservation of Mediterranean reefs,” funded by the Italian Ministry of Research and University—Fondo Integrativo Speciale per la Ricerca (FISR), project number: FISR_04543.
Acknowledgments
The authors wish to thank Riccardo Broggini, Erika Cantoro, and Elena Grimoldi for their contributions to material preparation and data collection in the framework of their theses in Marine Geology.
Conflict of interest
The authors declare that the research was conducted in the absence of any commercial or financial relationships that could be construed as a potential conflict of interest.
Publisher’s note
All claims expressed in this article are solely those of the authors and do not necessarily represent those of their affiliated organizations, or those of the publisher, the editors, and the reviewers. Any product that may be evaluated in this article, or claim that may be made by its manufacturer, is not guaranteed or endorsed by the publisher.
Supplementary material
The Supplementary Material for this article can be found online at: https://www.frontiersin.org/articles/10.3389/feart.2022.961632/full#supplementary-material.
References
Abbate, E., and Sagri, M. (1967). Suddivisioni litostratigrafiche nei calcare ad elmintoidi Auctt, della placca dell' Ebro-Antola e correlazioni con terreni simili affioranti tra Voghera e Castelnovo de' Monti (Appennino Settentrionale). Soc. Geol. Ital. Mem. 6, 23–65.
Adey, W. H. (1986). “Coralline algae as indicators of sea-level”, in sea-level research. Editor O. van de Plassche (Dordrecht: Springer), 229–280. doi:10.1007/978-94-009-4215-8_9
Adey, W. H. (1979). “Crustose coralline algae as microenvironmental indicators for the Tertiary”, in Historical biogeography, plate tectonics, and the changing environment. Editors J. Gray, and A. J. Boucot (Oregon State University Press), 459–464.
Adey, W. H., and Macintyre, I. G. (1973). Crustose coralline algae: A re-evaluation in the geological sciences. Geol. Soc. Am. Bull. 84, 883–904. doi:10.1130/0016-7606(1973)84<883:ccaari>2.0.co;2
Alongi, G., Cormaci, M., and Furnari, G. (1996). On the occurrence of Sporolithon ptychoides Heydrich (corallinales, sporolithaceae, rhodophyta) in the Mediterranean sea. Cryptogam. Algol. 17, 131–137.
Alverson, K., and Kull, C. (2003). Understanding future climate change using paleorecords, in Global Climate. Editors X. Rodó, and F. A. Comín (Berlin, Heidelberg: Springer), 153–185. doi:10.1007/978-3-662-05285-3_9
Antonioli, F., Silenzi, S., and Frisia, S. (2001). Tyrrhenian Holocene palaeoclimate trends from spelean serpulids. Quaternary Science Reviews 20 (15), 1661–1670. doi:10.1016/S0277-3791(01)00012-9
Antonioli, F., Cremona, G., Immordino, F., Puglisi, C., Romagnoli, C., Silenzi, S., Valpreda, E., and Verrubbi, V. (2002). New data on the Holocenic sea-level rise in NW Sicily (Central Mediterranean Sea). Global and Planetary Change 34, 1-2, 121–140, doi:10.1016/s0921-8181(02)00109-1
Athanasiadis, A., and Ballantine, D. L. (2014). The genera Melyvonnea gen. nov. and Mesophyllum s.s. (Melobesioideae, Corallinales, Rhodophyta) particularly from the central Atlantic Ocean. Nordic J. Bot. 32, 385–436. doi:10.1111/njb.00265
Babbini, L., and Bressan, G. (1997). Recensement de Corallinacées de la Mer Méditerranée et considérations phytogéographiques. Bibl. Phycol. 103, 1–421.
Balata, D., Piazzi, L., Cecchi, E., and Cinelli, F. (2005). Variability of Mediterranean coralligenous assemblages subject to local variation in sediment deposition. Mar. Environ. Res. 60, 403–421. doi:10.1016/j.marenvres.2004.12.005
Ballesteros, E. (2006). Mediterranean coralligenous assemblages. Oceanogr. Mar. Biol. 44, 123–195. doi:10.1201/9781420006391.ch4
Barberá, C., Moranta, J., Ordines, F., Ramón, M., De Mesa, A., Díaz-Valdés, M., et al. (2012). Biodiversity and habitat mapping of Menorca channel (Western Mediterranean): Implications for conservation. Biodivers. Conserv. 21, 701–728. doi:10.1007/s10531-011-0210-1
Basso, D. (2012). Carbonate production by calcareous red algae and global change. Geodiversitas 34(1), 13–33. doi:10.5252/g2012n1a2
Basso, D., Nalin, R., and Massari, F. (2007). Genesis and composition of the Pleistocene Coralligène de plateau of the Cutro Terrace (Calabria, Southern Italy). N. Jb. Geol.Paläont. 244/2, 73–182. doi:10.1127/0077-7749/2007/0244-0173
Basso, D., Nalin, R., and Nelson, C. S. (2009). Shallow-water Sporolithon rhodoliths from North Island (New Zealand). Palaios 24, 92–103. doi:10.2110/palo.2008.p08-048r
Basso, D., and Rodondi, G. (2006). A Mediterranean population of Spongites fruticulosus (Rhodophyta, Corallinales), the type species of Spongites, and the taxonomic status of S. stalactitica and S. racemosa. Phycologia 45, 403–416. doi:10.2216/04-93.1
Bazzicalupo, P., Maiorano, P., Girone, A., Marino, M., Combourieu-Nebout, N., Pelosi, N., et al. (2020). Holocene climate variability of the Western Mediterranean: Surface water dynamics inferred from calcareous plankton assemblages. Holocene 30, 691–708. doi:10.1177/0959683619895580
Bellan-Santini, D., Bellan, G., Bitar, G., Harmelin, J.-G., and Pergent, G. (2007). Handbook for interpreting types of marine habitat for the selection of sites to be included in the national inventories of natural sites of conservation interest. Tunis: UNEP-MAP-RAC/SPA.
Bertolino, M., Calcinai, B., Cattaneo-Vietti, R., Cerrano, C., Lafratta, A., Pansini, M., et al. (2014). Stability of the sponge assemblage of Mediterranean coralligenous concretions along a millennial time span. Mar. Ecol. 35, 149–158. doi:10.1111/maec.12063
Bertolino, M., Cattaneo-Vietti, R., Costa, G., Pansini, M., Fraschetti, S., and Bavestrello, G. (2017a). Have climate changes driven the diversity of a Mediterranean coralligenous sponge assemblage on a millennial timescale? Palaeogeogr. Palaeoclimatol. Palaeoecol. 487, 355–363. doi:10.1016/j.palaeo.2017.09.020
Bertolino, M., Costa, G., Carella, M., Cattaneo-Vietti, R., Cerrano, C., Pansini, M., et al. (2017b). The dynamics of a Mediterranean coralligenous sponge assemblage at decennial and millennial temporal scales. PLoS ONE 12, e0177945. doi:10.1371/journal.pone.0177945
Bini, M., Zanchetta, G., Regattieri, E., Isola, I., Drysdale, R. N., Fabiani, F., et al. (2020). Hydrological changes during the Roman Climatic Optimum in northern Tuscany (Central Italy) as evidenced by speleothem records and archaeological data. J. Quat. Sci. 35, 791–802. doi:10.1002/jqs.3224
Bond, G., Kromer, B., Beer, J., Muscheler, R., Evans, M. N., Showers, W., et al. (2001). Persistent solar influence on North Atlantic climate during the Holocene. Science 294, 2130–2136. doi:10.1126/science.1065680
Bosence, D. W. J. (1985). “The "coralligène" of the mediterranean - a recent analog for Tertiary Coralline Algal Limestones”, in Paleoalgology: Contemporary research and applications. Editors D. F. Toomey, and M. H. Nitecki (Berlin, Heidelberg: Springer), 216–225. doi:10.1007/978-3-642-70355-3_16
Boudouresque, C. F., Blanfuné, A., Harmelin-Vivien, M., Personnic, S., Ruitton, S., Thibaut, T., et al. (2017). “Where seaweed forests meet animal forests: The examples of macroalgae in coral reefs and the mediterranean coralligenous ecosystem”, in Marine animal forests. Editors S. Rossi, L. Bramanti, A. Gori, and C. Orejas (Cham: Springer), 369–396. doi:10.1007/978-3-319-21012-4_48
Bourcier, M. (1986). “Évolution, en cinq années, des herbiers à Posidonia oceanica et du macrobenthos circalittoral action conjuguée des activités humaines et des modifications climatiques”, in Change, over fîve years, of a Posidonia oceanica bed and circalittoral macrobenthos the combined effect of human activity and climatic conditions (Banyuls s/mer, France: Vie et Milieu), 1–8. Avaliable at: https://hal.sorbonne-universite.fr/hal-03023741.
Bracchi, V. A., Basso, D., Marchese, F., Corselli, C., and Savini, A. (2017). Coralligenous morphotypes on subhorizontal substrate: A new categorization. Cont. Shelf Res. 144, 10–20. doi:10.1016/j.csr.2017.06.005
Bracchi, V. A., Basso, D., Savini, A., and Corselli, C. (2019). Algal reefs (Coralligenous) from glacial stages: Origin and nature of a submerged tabular relief (Hyblean Plateau, Italy). Mar. Geol. 411, 119–132. doi:10.1016/j.margeo.2019.02.008
Bracchi, V.A., Bazzicalupo, P., Fallati, L., Varzi, A.G., Savini, A., Negri, M.P., et al. (2022). The main builders of Mediterranean Coralligenous: 2D and 3D quantitative approaches for its identification. Front. Earth Sci. (Lausanne). 10. doi:10.3389/feart.2022.910522
Bracchi, V. A., Nalin, R., and Basso, D. (2016). Morpho-structural heterogeneity of shallow-water coralligenous in a Pleistocene marine terrace (Le Castella, Italy). Palaeogeogr. Palaeoclimatol. Palaeoecol. 454, 101–112. doi:10.1016/j.palaeo.2016.04.014
Bracchi, V. A., Nalin, R., and Basso, D. (2014). Paleoecology and dynamics of coralline dominated facies during a Pleistocene transgressive-regressive cycle (Capo Colonna marine terrace, Southern Italy). Palaeogeogr. Palaeoclimatol. Palaeoecol. 414, 296–309. doi:10.1016/j.palaeo.2014.09.016
Braga, J. C., and Bassi, D. (2007). Neogene history of Sporolithon Heydrich (Corallinales, Rhodophyta) in the Mediterranean region. Palaeogeogr. Palaeoclimatol. Palaeoecol. 243, 189–203. doi:10.1016/j.palaeo.2006.07.014
Bressan, G., and Babbini, L. (2003). Biodiversità marina delle coste italiane: Corallinales del Mar Mediterraneo: Guida alla determinazione. Giulio Relini 10, 1123–4245.
Canals, M., and Ballesteros, E. (1997). Production of carbonate particles by phytobenthic communities on the Mallorca-Menorca shelf, northwestern Mediterranean Sea. Deep Sea Res. Part II Top. Stud. Oceanogr. 44, 611–629. doi:10.1016/s0967-0645(96)00095-1
Cánovas Molina, A., Montefalcone, M., Vassallo, P., Morri, C., Nike Bianchi, C., and Bavestrello, G. (2016). Combining literature review, acoustic mapping and in situ observations: An overview of coralligenous assemblages in Liguria (NW Mediterranean sea). Sci. Mar. 80, 7–16. doi:10.3989/scimar.04235.23A
Casas-Güell, E., Cebrian, E., Garrabou, J., Ledoux, J.-B., Linares, C., and Teixidó, N. (2016). Structure and biodiversity of coralligenous assemblages dominated by the precious red coral Corallium rubrum over broad spatial scales. Sci. Rep. 6, 36535. doi:10.1038/srep36535
Casas-Güell, E., Teixidó, N., Garrabou, J., and Cebrian, E. (2015). Structure and biodiversity of coralligenous assemblages over broad spatial and temporal scales. Mar. Biol. 162, 901–912. doi:10.1007/s00227-015-2635-7
Casellato, S., and Stefanon, A. (2008). Coralligenous habitat in the northern Adriatic Sea: An overview. Mar. Ecol. 29, 321–341. doi:10.1111/j.1439-0485.2008.00236.x
Catra, M., Giardina, S., Giaccone, T., Basso, D., and Giaccone, G. (2007). Coralligenous assemblages of Sicchitello (Ustica - Palermo). Biol. Mar. Mediterr. 14, 174–175.
Cebrián, E., Ballesteros, E., and Canals, M. (2000). Shallow rocky bottom benthic assemblages as calcium carbonate producers in the Alboran Sea (Southwestern Mediterranean). Oceanol. Acta 23, 311–322. doi:10.1016/s0399-1784(00)00131-6
Cerrano, C., Bavestrello, G., Bianchi, C. N., Calcinai, B., Cattaneo-Vietti, R., Morri, C., et al. (2001). “The role of sponge bioerosion in Mediterranean coralligenous accretion,” in The role of sponge bioerosion in the Mediterranean coralligenous accretion” in Mediterranean ecosystems: Structure and processes. Editors F. M. Faranda, L. Guglielmo, and G. Spezie (Verlag, Italy: Springer), 235–240. doi:10.1007/978-88-470-2105-1_30
Çinar, M. E., Féral, J. P., Arvanitidis, C., David, R., Taşkin, E., Sini, M., et al. (2020). Coralligenous assemblages along their geographical distribution: Testing of concepts and implications for management. Aquat. Conserv. Mar. Freshw. Ecosyst. 30, 1578–1594. doi:10.1002/aqc.3365
Cocito, S., Bedulli, D., and Sgorbini, S. (2002). Distribution patterns of the sublittoral epibenthic assemblages on a rocky shoal in the Ligurian Sea (NW Mediterranean). Sci. Mar. 66, 175–181. doi:10.3989/scimar.2002.66n2175
Cocito, S. (2004). Bioconstruction and biodiversity: Their mutual influence. Sci. Mar. 68, 137–144. doi:10.3989/scimar.2004.68s1137
Connell, S. D., Foster, M. S., and Airoldi, L. (2014). What are algal turfs? Towards a better description of turfs. Mar. Ecol. Prog. Ser. 495, 299–307.
Corriero, G., Pierri, C., Mercurio, M., Nonnis Marzano, C., Onen Tarantini, S. O., Gravina, M. F., et al. (2019). A Mediterranean mesophotic coral reef built by non-symbiotic scleractinians. Sci. Rep. 9, 1–17. doi:10.1038/s41598-019-40284-4
Costanzo, L. G., Marletta, G., and Alongi, G. (2021). Non-indigenous macroalgal species in coralligenous habitats of the marine protected area Isole Ciclopi (Sicily, Italy). Ital. Bot. 11, 31–44. doi:10.3897/italianbotanist.11.60474
De Jode, A., David, R., Haguenauer, A., Cahill, A. E., Erga, Z., Guillemain, D., et al. (2019). From seascape ecology to population genomics and back. Spatial and ecological differentiation among cryptic species of the red algae Lithophyllum stictiforme/L. cabiochiae, main bioconstructors of coralligenous habitats. Mol. Phylogenetics Evol. 137, 104–113. doi:10.1016/j.ympev.2019.04.005
Di Geronimo, I., Di Geronimo, R., Improta, S., Rosso, A., and Sanfilippo, R. (2001). Preliminary observations on a columnar coralline build-up from off SE Sicily. Biol. Mar. Medit. 8, 229–237.
Di Geronimo, I., Di Geronimo, R., Rosso, A., and Sanfilippo, R. (2002). Structural and taphonomic analysis of a columnar coralline algal build-up from SE Sicily. Geobios 35 (35), 86–95. doi:10.1016/s0016-6995(02)00050-5
Di Iorio, L., Audax, M., Deter, J., Holon, F., Lossent, J., Gervaise, C., et al. (2021). Biogeography of acoustic biodiversity of NW Mediterranean coralligenous reefs. Sci. Rep. 11, 16991 doi:10.1038/s41598-021-96378-5
Falace, A., Kaleb, S., Curiel, D., Miotti, C., Galli, G., Querin, S., et al. (2015). Calcareous bio-concretions in the northern Adriatic Sea: Habitat types, environmental factors that influence habitat distributions, and predictive modeling. PLoS ONE 10, e0140931. doi:10.1371/journal.pone.0140931
Faust, J. C., Fabian, K., Milzer, G., Giraudeau, J., and Knies, J. (2016). Norwegian fjord sediments reveal NAO related winter temperature and precipitation changes of the past 2800 years. Earth Planet. Sci. Lett. 435, 84–93. doi:10.1016/j.epsl.2015.12.003
Ferdeghini, F., Acunto, S., Cocito, S., and Cinelli, F. (2000). Variability at different spatial scales of a coralligenous assemblage at Giannutri Island (Tuscan Archipelago, northwest Mediterranean). Hydrobiologia 440, 27–36. doi:10.1007/978-94-017-1982-7_3
Flügel, E. (2010). Microfacies of carbonate rocks: Analysis, interpretation and application. Berlin: Springer-Verlag.
Garrabou, J., Ballesteros, E., and Zabala, M. (2002). Structure and dynamics of north-western Mediterranean rocky benthic communities along a depth gradient. Estuar. Coast. Shelf Sci. 55, 493–508. doi:10.1006/ecss.2001.0920
Gatti, G., Bianchi, C. N., Morri, C., Montefalcone, M., and Sartoretto, S. (2015). Coralligenous reefs state along anthropized coasts: Application and validation of the COARSE index, based on a rapid visual assessment (RVA) approach. Ecol. Indic. 52, 567–576. doi:10.1016/j.ecolind.2014.12.026
Gili, J. M., and Ros, J. D. (1985). Study and cartography of the benthic communities of Medes Islands (NE Spain). Mar. Ecol. 6, 219–238. doi:10.1111/j.1439-0485.1985.tb00323.x
Gómez-Gras, D., Linares, C., Dornelas, M., Madin, J. S., Brambilla, V., Ledoux, J. B., et al. (2021). Climate change transforms the functional identity of Mediterranean coralligenous assemblages. Ecol. Lett. 24, 1038–1051. doi:10.1111/ele.13718
Guiry, M. D., and Guiry, G. M. (2022). “AlgaeBase”, in World-wide electronic publication (Galway: National University of Ireland). AvaliableAt: https://www.algaebase.org.
Hall-Spencer, J. M., Rodolfo-Metalpa, R., Martin, S., Ransome, E., Fine, M., Turner, S. M., et al. (2008). Volcanic carbon dioxide vents show ecosystem effects of ocean acidification. Nature 454(7200), 96–99. doi:10.1038/nature07051
Hammer, Ø., Harper, D. A. T., and Ryan, P. D. (2001). Past: Paleontological statistics software package for education and data analysis. Palaeontol. Electron. 4, 9.
Hrabovský, J., Basso, D., and Doláková, N. (2016). Diagnostic characters in fossil coralline algae (Corallinophycidae: Rhodophyta) from the miocene of southern moravia (carpathian foredeep, Czech republic). J. Syst. Palaeontol. 14, 499–525. doi:10.1080/14772019.2015.1071501
Ingrosso, G., Abbiati, M., Badalamenti, F., Bavestrello, G., Belmonte, G., Cannas, R., et al. (2018). Mediterranean bioconstructions along the Italian coast. Adv. Mar. Biol. 79, 61–136. doi:10.1016/bs.amb.2018.05.001
IPCC (2022). Climate change 2022: Impacts, adaptation, and vulnerability. Contribution of working group II to the sixth assessment report of the intergovernmental panel on climate change. Editors H. O Pörtner, D. C. Roberts, M. Tignor, E. S. Poloczanska, K. Mintenbeck, A. Alegriaet al. (Cambridge, United Kingdom and New York, NY, USA: Cambridge University Press).
Irvine, L. M., and Chamberlain, Y. M. (1994). “Seaweeds of the British isles,” in Rhodophyta. Part 2B. Corallinales, hildenbrandiales (London: HMSO).
Jalali, B., Sicre, M. A., Bassetti, M. A., and Kallel, N. (2016). Holocene climate variability in the north-western Mediterranean sea (Gulf of Lyon). Clim. Past. 12, 91–101. doi:10.5194/cp-12-91-2016
Jalali, B., Sicre, M. A., Klein, V., Schmidt, S., Maselli, V., Lirer, F., et al. (2018). Deltaic and coastal sediments as recorders of Mediterranean regional climate and human impact over the past three millennia. Paleoceanogr. Paleoclimatology 33, 579–593. doi:10.1029/2017pa003298
Jalut, G., Esteban Amat, A. E., Bonnet, L., Gauquelin, T., and Fontugne, M. (2000). Holocene climatic changes in the Western Mediterranean, from south-east France to south-east Spain. Palaeogeogr. Palaeoclimatol. Palaeoecol. 160, 255–290. doi:10.1016/s0031-0182(00)00075-4
Joher, S., Ballesteros, E., and Rodrigues-prieto, C. (2015). Contribution to the study of deep coastal detritic bottoms: The algal communities of the continental shelf off the Balearic Islands, western Mediterranean. Medit. Mar. Sci. 16, 573–590. doi:10.12681/mms.1249
Kuffner, I. B., Andersson, A. J., Jokiel, P. L., Rodgers, K. U. S., and Mackenzie, F. T. (2008). Decreased abundance of crustose coralline algae due to ocean acidification. Nat. Geosci.. 1(2), 114–117. doi:10.1038/ngeo100
Laborel, J. (1961). Le concrétionnement algal ‘coralligène’ et son importance géomorphologique en Méditerranée. Rec. Trav. St. Mar. Endoume 23, 37–60.
Laborel, J. (1987). Marine biogenic constructions in the Mediterranean, a review. Sci. Rep. Port. Cros Natl. Park 13, 97–126.
Laborel, J., Morhange, C., Lafont, R., Le Campion, J., Laborel-Deguen, F., and Sartoretto, S. (1994). Biological evidence of sea-level rise during the last 4500 years on the rocky coasts of continental southwestern France and Corsica. Mar. Geol. 120, 203–223. doi:10.1016/0025-3227(94)90059-0
Lambeck, K., Antonioli, F., Purcell, A., and Silenzi, S. (2004). Sea-level change along the Italian coast for the past 10,000yr. Quat. Sci. Rev. 23, 1567–1598. doi:10.1016/j.quascirev.2004.02.009
Laubier, L. (1966). Le coralligène des albères : Monographie biocénotique. Ann. l’Institut Océanogr. 43, 139–316.
Marchese, F., Bracchi, V. A., Lisi, G., Basso, D., Corselli, C., and Savini, A. (2020). Assessing fine-scale distribution and volume of Mediterranean algal reefs through terrain analysis of multibeam bathymetric data. A case study in the southern Adriatic continental shelf. Water 12, 157. doi:10.3390/w12010157
Luterbacher, J., García-Herrera, R., Akcer-On, S., Allan, R., Alvarez-Castro, M. C., Benito, G., et al. (2012). A review of 2000 years of paleoclimatic evidence in the Mediterranean in The climate of the Mediterranean region: from the past to the future. Editor P. Lionello (Elsevier), 87–185. doi:10.1016/B978-0-12-416042-2.00002-1
Martin, S., and Gattuso, J.-P. (2009). Response of Mediterranean coralline algae to ocean acidification and elevated temperature. Glob. Change Biol. 15, 2089–2100. doi:10.1111/j.1365-2486.2009.01874.x
Martrat, B., Jimenez-Amat, P., Zahn, R., and Grimalt, J. O. (2014). Similarities and dissimilarities between the last two deglaciations and interglaciations in the North Atlantic region. Quat. Sci. Rev. 99, 122–134. doi:10.1016/j.quascirev.2014.06.016
Montefalcone, M., Morri, C., Bianchi, C. N., Bavestrello, G., and Piazzi, L. (2017). The two facets of species sensitivity: Stress and disturbance on coralligenous assemblages in space and time. Mar. Pollut. Bull. 117, 229–238. doi:10.1016/j.marpolbul.2017.01.072
Nalin, R., Basso, D., and Massari, F. (2006). “Pleistocene coralline algal build-ups (coralligène de plateau) and associated bioclastic deposits in the sedimentary cover of Cutro marine terrace (Calabria, southern Italy)”, in Cool–water carbonates: Depositional systems and palaeoenvironmental controls. Editors H. M. Pedley, and G. Carannante (London, UK: Geol. Soc. Spec. Publ.), 255, 11–22. doi:10.1144/GSL.SP.2006.255.01.02
Nalin, R., Lamothe, M., Auclair, M., and Massari, F. (2020). Chronology of the marine terraces of the Crotone Peninsula (Calabria, southern Italy) by means of infrared-stimulated luminescence (IRSL). Mar. Petroleum Geol. 122, 104645. doi:10.1016/j.marpetgeo.2020.104645
Novosel, M., Pozar-Domac, A., and Pasaric, M. (2004). Diversity and distribution of the Bryozoa along underwater cliffs in the Adriatic Sea with special reference to thermal regime. Mar. Ecol. 25, 155–170. doi:10.1111/j.1439-0485.2004.00022.x
Owens, P. N. (2020). Soil erosion and sediment dynamics in the Anthropocene: A review of human impacts during a period of rapid global environmental change. J. Soils Sediments 20, 4115–4143. doi:10.1007/s11368-020-02815-9
Pérès, J. M. (1982). “Major benthic assemblages”, in Marine ecology. Editor O. Kinne (Hoboken, NJ: Wiley and Sons), 373–521.
Pezzolesi, L., Peña, V., Le Gall, L., Gabrielson, P., Kaleb, S., Hughey, J., et al. (2019). Mediterranean Lithophyllum stictiforme (Corallinales, Rhodophyta) is a genetically diverse species complex: implications for species circumscription, biogeography and conservation of coralligenous habitats. J. Phycol. 55, 473–492. doi:10.1111/jpy.12837
Piazzi, L., Balata, D., Cecchi, E., Cinelli, F., and Sartoni, G. (2009). Species composition and patterns of diversity of macroalgal coralligenous assemblages in the north-western Mediterranean Sea. J. Nat. Hist. 44, 1–22. doi:10.1080/00222930903377547
Piazzi, L., Cinti, M. F., Guala, I., Grech, D., La Manna, G., Pansini, A., et al. (2021). Variations in coralligenous assemblages from local to biogeographic spatial scale. Mar. Environ. Res. 169, 105375. doi:10.1016/j.marenvres.2021.105375
Piazzi, L., Ferrigno, F., Guala, I., Cinti, M. F., Conforti, A., De Falco, G., et al. (2022). Inconsistency in community structure and ecological quality between platform and cliff coralligenous assemblages. Ecol. Indic. 136, 108657. doi:10.1016/j.ecolind.2022.108657
Piazzi, L., Gennaro, P., and Balata, D. (2011). Effects of nutrient enrichment on macroalgal coralligenous assemblages. Mar. Pollut. Bull. 62, 1830–1835. doi:10.1016/j.marpolbul.2011.05.004
Pierdomenico, M., Bonifazi, A., Argenti, L., Ingrassia, M., Casalbore, D., Aguzzi, L., Viaggiu, E., Le Foche, M., and Chiocci, F.L. (2021). Geomorphological characterization, spatial distribution and environmental status assessment of coralligenous reefs along the Latium continental shelf. Ecol. Indic. 131. 108219, doi:10.1016/j.ecolind.2021.108219
Pirazzoli, P. A. (2005). A review of possible eustatic, isostatic and tectonic contributions in eight late-Holocene relative sea-level histories from the Mediterranean area. Quat. Sci. Rev. 24, 1989–2001. doi:10.1016/j.quascirev.2004.06.026
Ponti, M., Fava, F., and Abbiati, M. (2011). Spatial-temporal variability of epibenthic assemblages on subtidal biogenic reefs in the northern Adriatic Sea. Mar. Biol. 158, 1447–1459. doi:10.1007/s00227-011-1661-3
Rasband, W. S. (1997). ImageJ. Bethesda, Maryland, USA: U. S. National Institutes of Health. AvaliableAt: https://imagej.nih.gov/ij/.
Richards, J. L., Sauvage, T., Schmidt, W. E., Fredericq, S., Hughey, J. R., and Gabrielson, P. W. (2017). The coralline genera Sporolithon and Heydrichia (Sporolithales, Rhodophyta) clarified by sequencing type material of their generitypes and other species. J. Phycol. 53, 1044–1059. doi:10.1111/jpy.12562
Rodolfo-Metalpa, R., Martin, S., Ferrier-Pagès, C., and Gattuso, J.-P. (2010). Response of the temperate coral Cladocora caespitosa to mid- and long-term exposure to pCO2 and temperature levels projected for the year 2100 AD, Biogeosciences, 7, 289–300, doi:10.5194/bg-7-289-2010
Rosso, A., and Sanfilippo, R. (2009). The contribution of bryozoans and serpuloideans to coralligenous concretions from SE Sicily in UNEP-MAP-RAC/SPA, Proceedings of the First Symposium on the Coralligenous and Other Calcareous Bio-Concretions of the Mediterranean Sea, Tabarka, January 15–16, 123–128.
Sanfilippo, R., Rosso, A., Basso, D., Violanti, D., Di Geronimo, I., Di Geronimo, R., Benzoni, F., and Robba, E. (2011). Cobbles colonization pattern from a tsunami-affected coastal area (SW Thailand, Andaman Sea). Facies 57, 1–13. doi:10.1007/s10347-010-0226-0
Sarmiento, J. L., Hughes, T., Stouffer, R. J., and Manabe, S. (1998). Simulated response of the ocean carbon cycle to anthropogenic climate warming. Nature 393(6682), 245–249. doi:10.1038/30455
Sartoretto, S. (2017). “IV.3.1 Biocénose coralligène,” in Fiches descriptives des biocénoses benthiques de Méditerranée. Editor L. Riviere (Paris, France: Paris UMS PatriNat OFB-CNRS-MNHN), 497–502.
Sartoretto, S. (1994). Structure et dynamique d'un nouveau type de bioconstruction à Mesophyllum lichenoides (Ellis) Lemoine (Corallinales, Rhodophyta). Acad. Sci., Série 3, Sci. vie 317, 156–160.
Sartoretto, S., Verlaque, M., and Laborel, J. (1996). Age of settlement and accumulation rate of submarine "coralligène" (−10 to −60 m) of the northwestern Mediterranean Sea; relation to Holocene rise in sea level. Mar. Geol. 130, 317–331. doi:10.1016/0025-3227(95)00175-1
Scholle, P. A. (1971). Diagenesis of deep-water carbonate turbidites, upper cretaceous Monte Antola flysch, northern apennines, Italy. J. Sediment. Pet. 4, 233–250.
Taricco, C., Ghil, M., Alessio, S., and Vivaldo, G. (2009). Two millennia of climate variability in the Central Mediterranean. Clim. Past. 5, 171–181. doi:10.5194/cp-5-171-2009
Titschack, J., Nelson, C. S., Beck, T., Freiwald, A., and Radtke, U. (2008). Sedimentary evolution of a late Pleistocene temperate red algal reef (coralligène) on rhodes, Greece: Correlation with global sea-level fluctuations. Sedimentology 55, 1747–1776. doi:10.1111/j.1365-3091.2008.00966.x
Virgilio, M., Airoldi, L., and Abbiati, M. (2006). Spatial and temporal variations of assemblages in a Mediterranean coralligenous reef and relationships with surface orientation. Coral reefs. 25, 265–272. doi:10.1007/s00338-006-0100-2
Wirth, S. B., Glur, L., Gilli, A., and Anselmetti, F. S. (2013). Holocene flood frequency across the Central Alps - solar forcing and evidence for variations in North Atlantic atmospheric circulation. Quat. Sci. Rev. 80, 112–128. doi:10.1016/j.quascirev.2013.09.002
Wisshak, M., Schönberg, C. H., Form, A., and Freiwald, A. (2014). Sponge bioerosion accelerated by ocean acidification across species and latitudes? Helgol. Mar. Res. 68, 253–262. doi:10.1007/s10152-014-0385-4
Woelkerling, W. J., Irvine, L. M., and Harvey, A. S. (1993). Growth-forms in non-geniculate coralline red algae (Coralliinales, Rhodophyta). Aust. Syst. Bot. 6, 277–293. doi:10.1071/sb9930277
WoRMS Editorial Board (2019). World register of marine species. Avaliable at: http://www.marinespecies.org.
Zielhofer, C., Köhler, A., Mischke, S., Benkaddour, A., Mikdad, A., and Fletcher, W. J. (2019). Western Mediterranean hydro-climatic consequences of Holocene ice-rafted debris (Bond) events. Clim. Past. 15, 463–475. doi:10.5194/cp-15-463-2019
Keywords: coralligenous, algal reef, coralline algae, Sporolithon, Ligurian Sea, extreme climate events, Holocene
Citation: Basso D, Bracchi VA, Bazzicalupo P, Martini M, Maspero F and Bavestrello G (2022) Living coralligenous as geo-historical structure built by coralline algae. Front. Earth Sci. 10:961632. doi: 10.3389/feart.2022.961632
Received: 05 June 2022; Accepted: 22 July 2022;
Published: 30 August 2022.
Edited by:
Olev Vinn, University of Tartu, EstoniaReviewed by:
Ergun Taskin, Celal Bayar University, TurkeyRodrigo Carvalho, Rio de Janeiro Botanical Garden, Brazil
Copyright © 2022 Basso, Bracchi, Bazzicalupo, Martini, Maspero and Bavestrello. This is an open-access article distributed under the terms of the Creative Commons Attribution License (CC BY). The use, distribution or reproduction in other forums is permitted, provided the original author(s) and the copyright owner(s) are credited and that the original publication in this journal is cited, in accordance with accepted academic practice. No use, distribution or reproduction is permitted which does not comply with these terms.
*Correspondence: Daniela Basso, daniela.basso@unimib.it