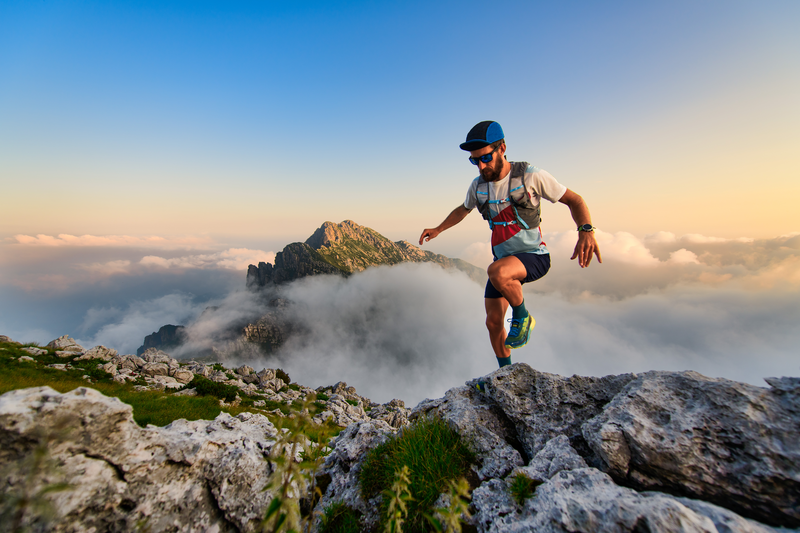
94% of researchers rate our articles as excellent or good
Learn more about the work of our research integrity team to safeguard the quality of each article we publish.
Find out more
EDITORIAL article
Front. Earth Sci. , 08 August 2022
Sec. Structural Geology and Tectonics
Volume 10 - 2022 | https://doi.org/10.3389/feart.2022.957558
This article is part of the Research Topic Continental Basin and Orogenic Processes: Tectonic Deformation and Associated Landscape and Environmental Evolution View all 15 articles
Editorial on the Research Topic
Continental Basin and Orogenic Processes: Tectonic Deformation and Associated Landscape and Environmental Evolution
Plate tectonics drives the development of large basins and orogens in the Earth’s continents, such as the Tibetan Plateau, the Zagros, the Andes, the Alps and their adjacent basins, and the Basin and Range province in the western United States. Among them, the Circum-Tibetan Plateau basin and orogen system is a typical example (Jia et al., 2013). Tectonic processes in orogenic belts may interact with environmental/climatic changes to affect landscape evolution, through the processes of rock uplift/exhumation, surface erosion and weathering, and associated isostatic deformation (Molnar and England, 1990; Raymo and Ruddiman, 1992; Shi et al., 1999; Ge, 2006; Zheng and Yao, 2006; Bonnet, 2009; Whipple, 2009). These processes can further influence the sediment transportation and deposition in the range-bounding basins, whose changes in space and mass will feedback to deep tectonic processes, leading to basin-orogen coupling processes (Armitage et al., 2011; Leeder, 2011; Li et al., 2003). Despite major progress in recent decades, how these processes interact with each other on different spatiotemporal scales remains a leading scientific issue (Burbank and Pinter, 1999; Willett et al., 2006; Bishop, 2007; National Research Council, 2010). The 14 papers in this Research Topic utilize interdisciplinary approaches to study and improve our understanding of the basin and orogenic processes and their effects on landscape and changes in the paleoenvironment around the Tibetan Plateau, Northeast China, and East China Sea Basin region.
The spatiotemporal evolution of orogenic tectonic deformation constitutes the foundation for studying the basin-orogen processes. Chen Q. et al. attempted to determine the initial deformation of the Atushi anticline within the Kashi foreland thrust-and-fold belt of southwestern Tian Shan. By integrating remote-sensing mapping, field investigation, and 26Al/10Be cosmogenic burial dating of the growth strata near the boundary of the Pliocene-Pleistocene Atushi and Xiyu Formations, the authors interpreted that the Atushi anticline started to develop around ∼1.8 Ma. Zhao and Pan applied the technique of GPS velocity decomposition and cluster analysis to constrain the strain partition and accommodation in the Qilian fold-and-thrust belt (QFTB) and its tectonic relationship with adjacent blocks. Their work revealed that the East and West QFTB are featured by lateral extrusion and range-normal crustal shortening-lateral extension, respectively. Such deformation patterns may be attributed to regional simple shear and pure shear, respectively. Jia et al. reconstructed three episodes of compressive tectonic activity of the northeastern Qilian Shan during the Mesozoic and Cenozoic by detailed field mapping of the Sunan and Huangcheng basins bounding the range front. They argue that such a tectonic process may be affected both by the far-field effects of the India-Asia collision and intraplate orogenic processes relating to the collision between the Alashan Block and the Qilian Shan. Tian et al. used detrital apatite fission track thermochronological data to constrain the Meso-Cenozoic tectono-thermal evolution of the Micang Shan-Daba Shan tectonic belt in central China. The authors found that differential across-range exhumation of the Micang Shan and Daba Shan, and a relatively rapid cooling since ∼160 Ma in response to the Qinling orogenic uplift, and the occurrence of differential uplift between sedimentary strata and basement may relate to variations in deep structure or intensities of thrust and nappe in different stages.
Sedimentary archives such as sediment provenance signals encode critical records of tectonic and landscape changes and have thus been utilized to study geologic events relating to basin-orogen coupling and surface processes. Pan et al. exploited detrital apatite fission track age distributions obtained from the Jiudong basin in the North Qilian Shan, where a continuous late Cenozoic sediment sequence was deposited with a good post-7 Ma chronologic framework, to examine the expansion of the Tibetan Plateau. Their results of obvious provenance change suggest two phases of significant expansion events of the North Qilian Shan since the Pliocene. Zhuang et al. examined two boreholes in the Taiyuan basin in the Shanxi Rift System bounding the eastern Ordos Block. The borehole stratigraphic analysis demonstrated two mega-transgressions that formed basin-wide paleolakes during ca. 5.8–4.4 and ca. 2.2–1.6 Ma. After a comprehensive analysis of the potential mechanisms for the transgressions, the authors suggested two periods of rifting-induced events of intensive subsidence during the Late Miocene-Quaternary. Liu et al. obtained detrital zircon chronology of sediments from the Pliocene-Pleistocene Sanmen Formation in the Weihe and Sanmenxia basins, and found that the two basins were integrated since the late Pliocene; and the Sanmen Gorge was finally established at ∼1 Ma, leading to the formation of the modern Yellow River. Fu et al. analyzed a large number of detrital zircon U-Pb ages of Miocene sediments from the East China Sea Basin (ECSB) and Taiwan to decipher the sediment sources. Their results indicate that the early-middle Miocene sediments in the ECSB and Taiwan share similar sources from the North China and Korean Peninsula, and the sediments in Taiwan were mostly supplied by the ECSB. However, during the late Miocene-Quaternary, the Yangtze River system became the major source of sediments in the ECSB. Such an abrupt provenance change suggests a distinct drainage reorganization and the late Miocene formation of the modern Yangtze River system.
Progress in recent decades on quantitative geomorphology, chronology, and topographic surveys are significantly improving understanding of how landscapes evolve in response to tectonic processes. In the northeastern margin of the Tibetan Plateau, Chen G. et al. integrated techniques of remote-sensing, field mapping, and cosmogenic 10Be chronology, topographic and quantitative geomorphic analysis, to argue that the development of the multi-segmented Dongbatu Shan thrust fault system may control the regional drainage evolution. Dong et al. explored the fluvial response to the regional tectonic uplift, from the perspective of longitudinal variations in grain sizes, lithology, and the roundness of the riverbed gravels of three large rivers flowing through the northern Qilian Shan. They found that the grain-size distribution in these arid/semi-arid areas may be a useful tool for evaluating the fluvial response to active tectonic uplift. Li Y. et al. made use of several geomorphic indices (e.g., channel steepness and χ-elevation data) and fluvial knickpoint celerity to analyze the response time of drainage in the Helan Shan, to the east of the northeastern margin of the Tibetan Plateau. Their results with previous thermochronologic data indicate the tectonic transformation of the Helan Shan from southwest to northwest during the early Quaternary, responding to the northeastward growth of the Tibetan Plateau. In the eastern Tibetan Plateau, field investigation and analyses of river incision and isostasy led Li H. et al. to propose both deep tectonics and the numerous earthquake-induced dams on the surface contribute to developing the regional high-elevation and high-relief landscape. Yu et al. applied the regional channel steepness index to quantify the regional rock uplift rate and its spatial variation in the central part of the grading topography area in the southeastern margin of the Tibetan Plateau. Their results show a southward decrease in channel steepness, hence a decrease in inferred rock uplift rate; and the region’s high rock uplift rate may relate to the NE-trending thrust fault system in the region. Collectively, they suggest limited extrusion by thrusting in the region. Outside the Tibetan Plateau, Lin et al. integrated analyses of cosmogenic 10Be-derived basin erosion rates, quantitative geomorphic indices of hypsometric curves, χ values, and channel steepness across the Great Khingan Mountains in NE China to infer the range’s asymmetry and disequilibrium state. They further argued that both the inherited Cenozoic tectonics and climate gradient may affect the evolution of the drainage basins capping the Great Khingan Mountains.
In summary, the 14 papers demonstrate the state-of-the-art advances in the research of basin and orogenic processes, from the perspective of interactions between tectonic deformation and associated surface processes and environmental changes. We believe that the results from these papers will contribute to the development of geoscience communities, such as continental tectonics, tectonic geomorphology, and paleoclimate changes.
All the authors listed have made a substantial, direct and intellectual contribution to the work, and approved it for publication.
This work was supported by NSFC grants (41720104003, 51988101, 41941016, 41972227), the China Second Tibetan Plateau Scientific Expedition and Research (2019QZKK0708) project, Fundamental Research Funds for the Central Universities (2021XZZX005), the Qianjiang Talent Program (QJD190202), and the 100 Talents Program of Zhejiang University.
The authors declare that the research was conducted in the absence of any commercial or financial relationships that could be construed as a potential conflict of interest.
All claims expressed in this article are solely those of the authors and do not necessarily represent those of their affiliated organizations, or those of the publisher, the editors and the reviewers. Any product that may be evaluated in this article, or claim that may be made by its manufacturer, is not guaranteed or endorsed by the publisher.
We thank all authors for their contributions, all reviewers for their constructive comments and suggestions, and the Frontiers editorial team for their support.
Armitage, J. J., Duller, R. A., Whittaker, A. C., and Allen, P. A. (2011). Transformation of tectonic and climatic signals from source to sedimentary archive. Nat. Geosci. 4, 231–235. doi:10.1038/ngeo1087
Bishop, P. (2007). Long-term landscape evolution: Linking tectonics and surface processes. Earth Surf. Process. Landf. 32, 329–365. doi:10.1002/esp.1493
Bonnet, S. (2009). Shrinking and splitting of drainage basins in orogenic landscapes from the migration of the main drainage divide. Nat. Geosci. 2, 766–771. doi:10.1038/ngeo666
Burbank, D., and Pinter, N. (1999). Landscape evolution: The interactions of tectonics and surface processes. Basin Res. 11, 1–6. doi:10.1046/j.1365-2117.1999.00089.x
Ge, X. (2006). Multi-stage uplifts of the Qinghai-Tibet plateau and their environmental effects. Earth Sci. Front. 13, 118–130. (in Chinese with English abstract).
Jia, C., Li, B., Lei, Y., and Chen, Z. (2013). The structure of Circum-Tibetan Plateau Basin-Range System and the large gas provinces. Sci. China Earth Sci. 56, 1853–1863. doi:10.1007/s11430-013-4649-7
Leeder, M. R. (2011). Tectonic sedimentology: Sediment systems deciphering global to local tectonics. Sedimentology 58, 2–56. doi:10.1111/j.1365-3091.2010.01207.x
Li, J.-l., Xiao, W.-J., and Yan, Z. (2003). Basin-range coupling and its sedimentation. Acta Sedimentol. Sin. 21, 52–60. (in Chinese with English abstract).
Molnar, P., and England, P. (1990). Late cenozoic uplift of mountain ranges and global climate change: Chicken or egg? Nature 346, 29–34. doi:10.1038/346029a0
National Research Council (2010). Landscapes on the edge: New horizons for research on Earth's surface. Washington, D.C: National Academies Press.
Raymo, M., and Ruddiman, W. F. (1992). Tectonic forcing of late Cenozoic climate. Nature 359, 117–122. doi:10.1038/359117a0
Shi, Y., Li, J., and Li, B. (1999). Uplift of the Qinghai-Xizang (Tibetan) plateau and East Asia environmental change during late Cenozoic. Acta Geogr. Sin. 54, 20–28. (in Chinese with English abstract).
Whipple, K. X. (2009). The influence of climate on the tectonic evolution of mountain belts. Nat. Geosci. 2, 97–104. doi:10.1038/ngeo413
Willett, S., Hovius, N., Brandon, M., and Fisher, D. (2006). Tectonics, climate, and landscape evolution. Boulder, Colorado, United States: Geological Society of America, 449.
Keywords: basin and orogen, coupling, tectonic deformation, landscape evolution, environmental change
Citation: Shi X, Chen H, Yang R, Zhang H and Yuan X (2022) Editorial: Continental Basin and Orogenic Processes: Tectonic Deformation and Associated Landscape and Environmental Evolution. Front. Earth Sci. 10:957558. doi: 10.3389/feart.2022.957558
Received: 31 May 2022; Accepted: 03 June 2022;
Published: 08 August 2022.
Edited and reviewed by:
Derek Keir, University of Southampton, United KingdomCopyright © 2022 Shi, Chen, Yang, Zhang and Yuan. This is an open-access article distributed under the terms of the Creative Commons Attribution License (CC BY). The use, distribution or reproduction in other forums is permitted, provided the original author(s) and the copyright owner(s) are credited and that the original publication in this journal is cited, in accordance with accepted academic practice. No use, distribution or reproduction is permitted which does not comply with these terms.
*Correspondence: Xuhua Shi, c2hpeHVodWFAemp1LmVkdS5jbg==; Hanlin Chen, aGxjaGVuQHpqdS5lZHUuY24=
Disclaimer: All claims expressed in this article are solely those of the authors and do not necessarily represent those of their affiliated organizations, or those of the publisher, the editors and the reviewers. Any product that may be evaluated in this article or claim that may be made by its manufacturer is not guaranteed or endorsed by the publisher.
Research integrity at Frontiers
Learn more about the work of our research integrity team to safeguard the quality of each article we publish.