- 1Faculty of Geosciences and Environmental Engineering, Southwest Jiaotong University, Chengdu, China
- 2Bureau of Qinghai Environmental Geological Prospecting, Xi’ning, China
- 3Key Lab of Geo-environment of Qinghai Province, Xi’ning, China
- 4Institute of Hydrogeology and Environmental Geology, Chinese Academy of Geological Science, Shijiazhuang, China
- 5Center for Hydrogeology and Environmental Geology, China Geological Survey, Baoding, China
- 6School of Water Resources and Environment, China University of Geosciences (Beijing), Beijing, China
- 7School of Water Resources and Hydropower Engineering, North China Electric Power University, Beijing, China
Climate warming is the greatest future challenge to the hydrosphere and the human community, especially in arid and semiarid regions. This study took the Golmud river watershed on the Tibetan Plateau as an example to numerically identify the development of groundwater flow systems in a large arid sedimentary basin and explore what would the dramatic climate warming pose on groundwater flow system. The numerical results show that the Golmud river watershed has developed three hierarchical groundwater flow systems. River seepage is the predominant recharge for the groundwater systems inside the basin. The local groundwater flow system discharges some 82.69% of all groundwater in the basin, followed by the intermediate system with 14.26% and the regional system with 3.05%. The local system is mainly distributed in the shallow area of the alluvial-pluvial fan at the piedmont and provides the dominant water resource for human exploitation and oasis ecological usages. Climate warming would increase about 30.78% of the quantity of the recharge water to the groundwater system inside the basin via river seepage due to the increasing precipitation and increased glacier melt in the headwater region of the watershed. These waters would pose disturbances to all groundwater flow systems but to different degrees. The local flow system exhibits the largest response to the climate warming with more than 90% of increased water cycled in and discharged through it. The significant groundwater level rising leads to the trailing edge of the overflow belt at the piedmont moving ∼5 km towards to the mountain pass, which would potentially pose a water disaster to the local region. The influences of climate warming on the intermediate and regional flow system are relatively limited. This study provides a preliminary understanding of the influences of climate warming on the groundwater flow systems in arid endorheic basins and is essential for tackling future climate change challenges faced by arid and semiarid regions.
Introduction
Groundwater is a crucial constitutive part of the hydrosphere of our planet and accounts for approximately 97% of continental liquid freshwater resources (Hatch-Kuri and Carrillo-Rivera, 2021). It is essential for maintaining the eco-environment, irrigating agriculture, and for supporting the development of industry and human communities (Gu et al., 2018; Xiao et al., 2018; Adimalla, 2019; Li et al., 2019; Zhang et al., 2021a). It is estimated that 50% of agricultural production, 40% of industrial consumption, and 40% of domestic water consumption of the global population rely on groundwater resources (Dash et al., 2019; Xiao et al., 2021). In fact, groundwater is the only water supply for the daily consumption of about 2.5 billion people around the world (Díaz-Alcaide and Martínez-Santos, 2019; Xiao et al., 2022b). The intensive exploitation of groundwater resource has resulted in groundwater resource depletion, ecological degradation, and geohazards in many regions worldwide (Xu et al., 2015; Gu et al., 2017b; Antonellini et al., 2019; Li et al., 2019; Huang et al., 2020; Zhang et al., 2021b). It is a more significant factor in arid and semiarid regions where surface water is scarce (Moharir et al., 2019; Hao et al., 2020; Mookiah et al., 2021; Qu et al., 2021; Xiao et al., 2022c; Liu et al., 2022). Groundwater and its behaviors are a fundamental part of the scientific management of groundwater resource for implementing the sustainable development of human society and eco-environment systems.
In addition to anthropogenic disturbances, dramatic climate change is another factor that has posed and will continue to pose unprecedented challenges and threats to water safety and sustainability globally (Brolsma et al., 2010; Ghimire et al., 2021). The planet is suffering from rapid climate warming, and this is expected to continue for the foreseeable decades or centuries (Hemmerle and Bayer, 2020; David Raj et al., 2022; IPCC, 2022). Climate warming not only results in increased temperatures but also poses a series of related influences on earth system (Bussi et al., 2022; Gan et al., 2022). The hydrosphere is expected to be the main sphere that is directly and significantly affected by the global climate change (Kim et al., 2022; Rafiei-Sardooi et al., 2022). The warming climate will significantly change the status of the balance of water reservoirs on earth. It is widely known that the polar icecaps and glaciers on high plateaus and mountains are melting under the climate warming (Straneo and Heimbach, 2013; Kraaijenbrink et al., 2017). This is releasing a giant quantity of liquid water, increasing the amount of liquid water in water circulation and leading to geohazards like global sea level rising (Minea et al., 2022). Compared to the effects on sea level, the influence on continental water and its circulation are more significant and will present more complex challenges, especially for arid and semiarid regions that have relatively fragile eco-environments (Sarzaeim et al., 2017). Due to the scarcity of surface water, groundwater is the critical water that participates in water circulation and supports the natural ecosystems and human communities in arid and semiarid regions (Goderniaux et al., 2011; Xiao et al., 2022a). Thus, groundwater and its behaviors in arid and semiarid regions under climate change should be given special attentions.
The Tibetan plateau has been called the third pole of the earth, and it features the largest amount of water in glaciers anywhere on earth apart from the north and south poles (Qu et al., 2019). Although the Tibetan plateau is known as the water tower of Asia (Hao et al., 2021), the northern part of it is typically arid and semiarid (Gu et al., 2017a; Hao et al., 2018; Luo et al., 2021; Wang et al., 2022). The potential giant quantity of melted water from glaciers for the arid and semiarid regions can amplify the effects of climate warming for the continental hydrosphere (Ma Y. et al., 2017; Chen et al., 2021). Thus, a typical endorheic watershed in the arid northern Tibetan plateau was selected as the study area to obtain insights into the likely outcomes of climate warming for the hydrosphere, groundwater circulation, and behavior of the groundwater system.
The specific aims of this study are 1) modeling the status of groundwater at present climate conditions, 2) revealing the development of the hierarchical groundwater flow systems in an arid and semiarid large sedimentary basin, and 3) developing a preliminary understanding of groundwater behaviors and the evolution of groundwater flow systems in arid sedimentary basins due to climate warming. This study can provide scientific support for managing groundwater resources, coping with the water crisis and related geohazards posed by climate warming, and implementing the sustainable development of arid and semiarid regions in the future challengeable environment.
Study area
General setting of the Golmud river watershed
The Golmud river watershed is a typical endorheic watershed on the Tibetan Plateau. It is located at the south margin of the Qaidam basin, which is the largest basin on the Tibetan Plateau. This watershed is bounded by the Kunlun Mountains in the south and Lake Dabusun in the north (Figure 1), and it extends between 36°59′43.60″ and 35°50′12.34″ latitude and from 94°16′13.86″ to 95°20′19.56″ longitude with a covering area of ∼12180 km2. The Golmud river, the second largest river in the basin, runs through this watershed and flows from the Kunlun Mountains to Lake Dabusun. This watershed is characterized by the dry continental climate of the plateau. The precipitation and evaporation are extremely variable in time and space. Annual precipitation can reach 200 mm in the mountainous area, but it is below 50 mm in the basin. Most of precipitation occurs in the rainy season (June to August), and accounts for more than 60% of the precipitation over the course of the year. Conversely, evaporation presents an increasing trend from south to the north and even exceeds 2600 mm per year in the basin. The high annual evaporation and low precipitation in the basin result in nearly no effective production of water resources for surface and ground water from local precipitation. The water resources of the watershed are mainly produced in the south and source from precipitation and snow/ice melt water in the mountainous area. The water resources in the basin are dominantly derived from river water and a part of underground lateral flow at mountain passes. River water flows into the basin, and almost all water seepages into the aquifers in the middle-upper part of the alluvial fan. Only in the flooding season, some river waters reach the lower part of the alluvial fan. As a result, the ecology in the basin is groundwater dependent. Due to the scarcity of precipitation and surface water, groundwater is also the main supplying water source for the various purposes of the human community. Thus, understanding groundwater and its behavior is significant for the sustainable development of this hyper arid region.
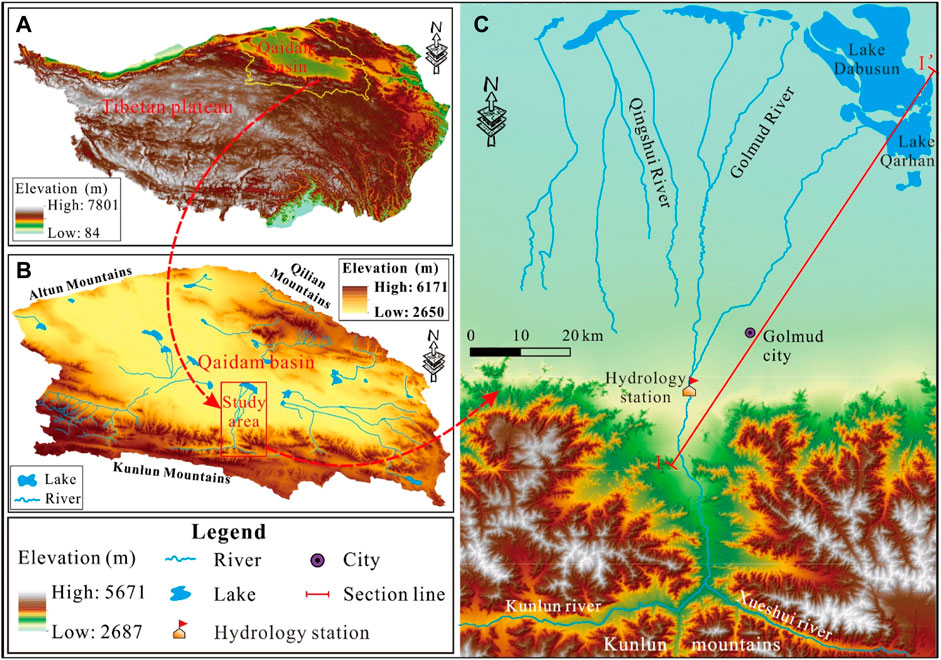
FIGURE 1. Location of (A) the Tibetan Plateau, (B) the Qaidam Basin, and (C) the Golmud river watershed.
Geology and hydrogeology
The Qaidam basin is a closed basin, formed during the uplift of the Tibetan Plateau. The Golmud watershed is one of numerous endorheic watersheds in the basin (Figure 1). This watershed has an outcropping stratigraphy at an age from Proterozoic to Quaternary. The pre-Quaternary strata are dominantly located in the mountainous areas. Inside the basin, the strata are dominated by hundreds and thousands of meters of Quaternary deposits. There are four types of aquifer systems in the watershed, including the permafrost aquifer system, carbonate karst aquifer system, fractured aquifer system, and porous aquifer system (Hu and Jiao, 2015). With the exception of the porous aquifer system, the other three aquifer systems are only distributed in the mountainous area. A porous aquifer system occurs along the river valleys in the mountainous area and inside the basin. The lithologies of Quaternary deposits inside the basin are spatially variable, varying from pebble and gravel in the upper parts of the alluvial fan to coarse sand in the middle-lower alluvial fan and then to fine sand and clay in the middle-lower lying depression of the watershed. Regionally, there are three continuous clay layers distributed at the depths of 60, 290, and 450 m in the basin (Figure 2). As a result, the aquifer system in the basin has a variable structure and changes from a single thick phreatic structure to a multilayer one.
The Quaternary porous aquifer system in the basin mainly receives its recharge water from river seepage in the middle-upper areas of the alluvial fan. The lateral inflow at the mountain pass also contributes recharge water to the Quaternary aquifer systems in the basin to some degree. Groundwater flows from the upper area of the Golmud alluvial fan towards the basin center and experiences several transformations between groundwater and surface water. The groundwater level has great depths in the middle-upper areas of the alluvial fan, which can reach to hundreds of meters, but it gradually evolves to a much shallower depth along the groundwater flow path towards the basin center. Most of the groundwater level in the areas from the front of alluvial fan to the basin center has depths of less than 3 m, so significant evaporation occurs. All groundwater and surface water finally discharge into the salt Lake Dabusun, the terminal of the Golmud watershed, and is lost to evaporation. Due to the shortage of surface water in human-settled areas, the groundwater has been greatly exploited for supporting the development of human community in the watershed. Additionally, the ecology in the oasis and the lower areas of the watershed is dependent on the groundwater. Overall, the groundwater inside the basin is mainly recharged by river seepage in the middle-upper area of the alluvial fan and lateral inflow at the mountain pass and discharges through evaporation, human pumping, and plant usage.
Materials and methods
Development of the groundwater flow model
Conceptual model and numerical model methodology
Aquifers inside the basin can be generally divided into hydrogeological layers using three regional aquitards (Figure 2). The upper layer is distributed from the mountain pass to the low-lying depression (Lake Dabusun) of the basin, with lithologies, varying from high hydraulic permeability to relatively low hydraulic permeability. This layer is regarded as the upper aquifer, which is phreatic in the alluvial fan area and semi-confined in parts of the middle to lower areas of the watershed. It has great thickness in the alluvial fan but smaller thickness from the front of the alluvial fan northwards. The second layer is distributed from the front of the alluvial fan to the lower parts of the fine-soil plain. This layer is constrained by the regional continuous aquitards at the depths of 60 and 290 m. It is semiconfined at the front of the alluvial fan and confined in the lower stream area. Below the continuous clay layer, at a depth of 290 m, there are still two further regional aquifers, which are divided by a regional aquitard at a depth of 450 m and all confined. The third aquifer has a relatively good hydraulic connectivity and great thickness. The fourth aquifer is intercalated with many layers of differing relative permeability in the fine soil plain and salt marsh plain, and as a result, the groundwater there flows very slowly.
To investigate the groundwater flow system in the basin and its behaviors due to climate change, a cross section parallel to the main groundwater flow direction in the watershed (I-I′ presented in Figure 1) is chosen as the geometry for the simulation of two-dimensional steady flow. This geometry ranges from the mountain pass to the terminal lake with a length of 100 km (Figure 2). To illustrate the strong evaporation effects on the groundwater level fluctuation in the fine soil plain and salt marsh plain, the geometry of the simulation is discretized vertically as irregular cells with the aid of the gradient mesh size method. The smallest cells have a thickness of 0.1 m and are located near the surface (Figure 3). The thickness of the cells gradually increases with increasing depth, reaching ∼80 m at the deepest point. Horizontally, the geometry is discretized using the equal discretization method. The viscosity and density of the groundwater are assumed to have negligible spatial variatio. Thus, groundwater flows inside the basin can be considered to be driven by gravity, as described by the following equation:
where σ (h) is the specific storage, h represents the pressure head, z is the elevation, t signifies time, K(h) denotes the unsaturated hydraulic conductivity, and q represents the source or sink. The two-dimensional groundwater flow simulations are established and solved using the TOUGH 2 program (Transport Of Unsaturated Groundwater and Heat).
Parametrization and boundary conditions
In the model domain, five predominant lithologies are found inside the basin, including sandy gravel/pebble, sand, silty soil, silty clay, and clay. The hydrogeological parameters of these lithologies are initially given in the model according to the borehole drilling records, pumping tests, and empirical values from similar studies (Gu et al., 2017a; Hao et al., 2018). The parameters are adjusted in the calibration and validation process, with the aid of hydraulic head observation data. There are two types of recharge for the groundwater, including river infiltration (seepage) and lateral inflow. The river infiltration mainly occurs at the piedmont (i.e., the alluvial fan area). The lateral inflow only exists at the boundary between the bedrock at the mountain pass and the sediments of the alluvial fan plain. These two groundwater recharges are set as the specified flow boundary and introduced by the WATE module of TOUGH2 in the model. The groundwater in the study area is mainly discharged via human pumping, evaporation, and springs. Human pumping is dominantly located in the alluvial fan plain, which is also set as a specified flow boundary and modelled using the WATE module. The evaporation and springs are defined as mixed boundary and simulated using the EVAP and DELV module of TOUGH2, respectively. The EVAP module is a new development module for evaporation calculation, which is based on the content of soil water (Hao et al., 2016). The lake boundary at the low-lying depression of the watershed is defined as the specific head boundary. The DELV module is similar to the Drain module of MODFLOW. The bottom boundary and the northern lateral boundary of the model geometry are generalized as impermeable boundaries (Figure 2), i.e., a zero flux boundary. The parameters of the aforementioned boundaries are given according to the field survey and measurement.
Scenario analysis
Scenario 1: Present status
Scenario 1 illustrates groundwater flow patterns under present climatic and pumping condition. The sources and sinks of the model are assigned according to the annual average seen in 2016. Potential evaporation is defined as 2600 mm, drawing on local meteorological data (Table 1). The seepage/infiltration of the river water is given based on hydrological station data and is set as 135.65 m3·d−1 along the profile. The lateral inflow of the bedrock from the mountainous area is estimated using Darcy’s section method and is 0.04 m3·d−1 for the model geometry (Table 1). The pumping quantity is obtained according to the results of the regional hydrogeological survey and assigned as 20.65 m3·d−1 for the cross section domain.
Scenario 2: Climate warming
Scenario 2 presents the evolution of the groundwater flow system under the influence of global climate warming. Previous research indicates that the Qaidam basin has experienced significant warming over the past few decades, and it is estimated that this trend will continue (Kuang and Jiao, 2016). This warming will significantly influence the climatic and hydrological features of the watershed. The quantity of melting snow and ice water would increase. The same trend would occur for the annual precipitation in the watershed. As a result, the quantity of recharge water into the basin from the mountainous area would increase. However, evaporation would decrease along with the climate warming due to increased humidity. Overall, the influence of climate warming on water resources inside the basin would be via the recharge water quantity at the piedmont and through evaporation inside the basin.
It is estimated that about 40% of the total water at the mountain pass originates from the precipitation in the mountainous area, and the remainder is from the snow and ice melt water (Chen et al., 2014). The temperature of the Qaidam basin is expected to increase at a rate of 0.26°C/10 a (Table 1) (Nan et al., 2004). As a result, the precipitation and melt water will increase to approximately 110.35% (Hu et al., 2015) and 160% (Shi, 2001), respectively, by 2100, and the evaporation rate in 2100 would be 52% of the present rate (Table 1) (Ma R. et al., 2017). According a previous survey, the quantity of water seepage from the riverbed has no significant relationship to the river water runoff flux from the mountain pass to the hydrology station (Figure 1C) but relates to the one from the hydrology station north. The seepage water quantity of different parts of the Golmud river can be estimated using the empirical equation below:
where QS and QN represent the river seepage water quantity from the mountain pass to the hydrology station (Figure 1C) and from the hydrology station northward, respectively. Qms and Qhs are the river water runoff flux at the mountain pass and the hydrology station, respectively.
Results and discussion
Model calibration and validation
The model was calibrated to minimize the difference between the model simulation results and real hydrogeological conditions to an acceptable threshold. Hydraulic heads from 63 boreholes from the mountain pass to the terminal lake were observed in this process. Trial and error correction was employed to adjust the hydraulic parameters to reflect the real hydrogeological conditions of the watershed.
The comparison of the simulated hydraulic heads after parameter calibration and observed hydraulic heads is given in Figure 4. The deviation between the simulated and observed hydraulic heads varied in spatial terms, from a small one in the fine soil plain and salt marsh plain to a relatively large one in the alluvial fan (the piedmont area). The maximum deviation between the simulated hydraulic head and the observed hydraulic head was within 0.8 m in the fine soil plain and salt marsh plain and reached 4.8 m in the alluvial fan. Large seasonal hydraulic head fluctuation and a steep hydraulic gradient were the main causes of relatively large deviations for model results (Islam et al., 2017). The deviation for the simulated hydraulic heads in the middle- to lower-stream area (the fine soil plain and the salt marsh plain) is attributed to the lithological heterogeneity of the aquifers (Gu et al., 2017a). For the whole model cross section, the root mean square error (RMSE) of the simulated hydraulic head and the observed hydraulic head was only 1.57 m. Overall, the simulated results reflect the real regional hydrogeological conditions.
To further validate the calibrated model, the isotopic ages of the groundwater were introduced to compare the hydraulic age of the groundwater. Groundwater samples along the cross section were collected for radioactive isotope analysis (Figure 5). A total of 13 groundwater samples were obtained, including 4 from the phreatic aquifers in the alluvial fan and 9 from the deep aquifers of the fine soil plain and salt marsh plain. Isotopic ages of the groundwater were estimated using the tritium approach if the water had a tritium content greater than 1 TU (tritium unit) and obtained by the radiocarbon method if the tritium content was below 1 TU. Particle tracking was employed for calculating groundwater hydraulic age, contoured by linear interpolation (Gu et al., 2017a). As shown in Figure 5, the simulated hydraulic ages of groundwater are largely consistent with the isotopic ages of the groundwater. Thus, the calibrated model has superior performance in simulating the groundwater flow in the study area and can be used for exploring the flow pattern and behaviors of groundwater.
The calibrated hydrological parameters of the two-dimensional groundwater model are presented in Table 2. The horizontal permeabilities (Kh) of sand and sandy gravel/pebble are in the range of 13.7–56.3 m·d−1, with an anisotropy ratio (Kh/Kv) of 10. For the silty soil and silty clay, the horizontal permeabilities (Kh) were 0.62 m·d−1 and 0.13 m·d−1, respectively, with an anisotropy ratio (Kh/Kv) of 5. The clay had the horizontal permeability (Kh) of 0.001 and an anisotropy ratio (Kh/Kv) of 5. The porosity of sandy gravel/pebble, sand, and silty soil ranged from 0.35 to 0.50, and those of silty clay and clay were in the range of 0.60–0.65.
The present groundwater flow system
The groundwater flow pattern in the present climate and pumping condition is presented in Figure 6. The present study area is a typical Tóthian basin and features several hierarchical groundwater flow systems. Three hierarchical groundwater flow systems, including the local, intermediate, and regional system, have developed inside the basin. The flow patterns of groundwater are strictly governed by the distribution of lithology distribution along the profile. The groundwater flow lines present an upwardly convex shape when meeting poor permeable strata. This is significant at the front of the alluvial fan and in the middle area of the fine soil plain, where a lot of continuous clay exists.
The local groundwater flow system is distributed in the alluvial fan plain. The groundwater in this flow system obtains recharge water from the seepage of the Golmud river in the middle and upper parts of the alluvial fan. It generally discharges at the front of the alluvial fan, where the overflow area of the watershed is found. The cycled groundwaters in this flow system provide the predominant water source for domestic usage and industrial purposes. The shallow buried features of the groundwater level and the largely natural overflow quantity of this system at the lower area make the front of the alluvial fan plain being the oasis of the watershed and suitable for perch.
The intermediate groundwater flow system occurs below the local groundwater flow system and ranges from the upper part of the alluvial fan plain to the lower parts of the fine soil plain. This system receives recharge water from the seepage of the Golmud river in the upper part of the alluvial fan plain. The groundwater in this flow system moves northward and sinks into the aquifer in the upper and middle areas of the alluvial fan. However, due to the influence of continuous clay layers in the lower parts of the alluvial fan, groundwater presents an upward-flowing patterns there, and the flow paths are constrained by the two continuous clay layers. The largest cycle depth of the groundwater in this system reaches approximately 600 m at the middle to lower parts of the alluvial fan and adjacent to the continuous clay layers. All groundwaters in this system are discharged in the middle to lower parts of the fine soil plain through springs and evaporation. The groundwater in this system supports the ecology of the fine soil plain and is significantly important for the environment maintenance of the watershed.
The regional groundwater flow system ranges from the mountain pass to the low-lying depression (Lake Dabusun) of the watershed and is distributed below the local groundwater flow system and the intermediate groundwater flow system. This groundwater flow system is mainly controlled by a continuous clay layer at a depth of 290 m regionally and is developed below it. The groundwater in this system is dominantly recharged by river seepage at the top of the alluvial fan and discharged as evaporation and salt lake water in the salt marsh plain. The flow paths of groundwater in this system are disorganized in middle to lower area of the watershed (i.e., the fine soil plain and the salt marsh plain). The distribution of clay layers has a great influence on the shape of groundwater flow paths.
The discharge water quantity of various groundwater flow systems at present were identified. The three hierarchical flow systems perform quite differently in terms of cycling and discharging water quantity in the basin (Table 3). Most groundwater in the basin is cycled and discharged through the local flow system and flows in the shallow parts of the watershed. This part of the groundwater accounts for more than 80% of the cycling groundwater in the watershed. The intermediate groundwater flow system accounts for the second-most amount of the cycling groundwater in the watershed but far less than the local groundwater flow system. In its cycling and discharging quantity, it accounts for approximately 14.26% of the water of the entire watershed. Although the regional groundwater flow system has the largest distribution area in the watershed, it features the lowest cycling and discharging water quantity, only 3% of the entire basin. This is ascribed to the relatively poor permeability of the aquifer regionally. Thus, the surface water in salt lakes in low-lying depression (Lake Qarhan and Lake Dabusun) is not dominantly recharged by the water cycle beneath the ground. It is supported and maintained by the flowing water of various spring-fed rivers of the watershed. It is also suggested that the rich salt minerals in the low-lying depression of the watershed are not simply brought and formed by the regional groundwater flow. The water of the ancient salt lakes migrating from the west to the center of the Qaidam basin over the course of geological history may form the predominant contribution of these rich salts of the low-lying depression of the Golmud watershed.
The evolution of groundwater flow system under climate warming
Climate warming has a significant influence on the groundwater system in the study area. The water balances of the modeled profile under the present climate scenario and the climate warming scenario are presented in Table 4. Compared to the present climate, climate warming would greatly increase the recharge water quantity from the mountainous area. River seepage, which is the dominant recharge water for aquifers inside the basin, has increased from 135.61 m3·d−1 to 177.35 m3·d−1 for this profile. Climate warming would cause the river seepage water quantity to increase 30.78% over its present quantity. Due to the limited water quantity from bedrock lateral inflow, climate warming would not significantly change the percentage of each recharge in the total recharged water. The increase in river seepage water quantity has had a significant influence on the discharges of groundwater system inside the basin, and the quantities of all natural discharges would increase under the climate warming scenario. The discharge quantity of the springs has the largest variation and would increase from 78.59 m3·d−1 to 120 m3·d−1 for the whole profile. The quantities of discharge water due to evaporation and outflow into the lake would also increase under the climate warming, but their changes are not significant.
The percentage of each discharge shows that the climate warming would not change the order of the recharge and discharge quantities inside the basin (Table 4). River seepage would still be the predominant means of recharge for groundwater systems in the basin and would account for 99.98% of the total recharge water quantity under climate warming scenario. The bedrock water quantity in lateral inflow is limited and only accounts for about 0.02%. For the discharge in the groundwater system, although the order of water quantities would not be changed by climate warming, the percentage of each discharge in the total discharge of the profile would be significantly changed. The percentage of spring discharge on the total discharge water quantity would greatly increase, from 57.94 to 67.65%. On the other hand, other natural discharge and human pumping would decrease in the percentage of the total discharge quantity. Evaporation discharge would decrease from 25.86 to 19.79% in percentage, and the human pumping would decrease from 15.22 to 11.64%. The percentage of outflow into the lake would have a small influence, only decreasing from 0.98 to 0.93%.
Climate warming would also significantly change the flow characteristics of groundwater along the profile (Figure 7). The groundwater level in the alluvial-pluvial fan would greatly increase as a result, and the trailing edge of the overflow belt would move about 5 km toward to the mountain pass. This would make most of the urban area of the city of Golmud into the overflow area of the groundwater, increasing the susceptibility of the region to water disasters. The hierarchical groundwater flow systems developed in the basin would also be disturbed by climate warming. Overall, the influences on the three flow systems would differ. Significant influences would only be observed in the local groundwater flow system. The largest cycle depth of groundwater in this system would increase from the 280 m of present climate (Scenario 1) to 360 m in the climate warming condition (Scenario 2). Climate warming would not have a significant influence on the intermediate groundwater flow system or the regional groundwater flow system, and the discharge water ranges of these two systems would experience nearly no change under climate warming.
The cycling water of all hierarchical groundwater flow systems would increase in quantity due to climate warming, but the degree of increase varies among the systems. The increase in cycling water in local groundwater is especially significant. The cycling water quantity of this system would increase from 112.16 m3·d−1 to 152.40 m3·d−1. Although the quantities of cycling water of the intermediate groundwater flow system and the regional groundwater flow system would increase due to climate warming, the increase would not be significant for these two systems. The cycling water quantities of these two systems would only increase from 19.34 m3·d−1 to 20.05 m3·d−1 and from 4.14 m3·d−1 to 4.95 m3·d−1, respectively. On a percentage basis, the quantity of cycling water in the local system would increase from 82.69 to 85.91% of the total cycling water of the profile, but in the intermediate and regional systems, it would decrease from 14.26 to 11.30% and from 3.05 to 2.79%.
Overall, the climate warming would increase groundwater recharge in the basin predominantly through the river seepage at the piedmont, and most of the additional water would discharge out of the groundwater system via springs. It should be noted that the discharge outflow into the lake would be relatively stable and not significantly disturbed by climate change. All hierarchical groundwater flow systems would respond to climate warming. Local groundwater shows the strongest response to the climate warming, ranked by the intermediate system. The regional groundwater flow system has the weakest response to climate change and may be negligible. The local groundwater flow system cycles and discharges most of the water added by climate warming. Thus, the local groundwater system developed at the piedmont should be examined with a view to reducing and eliminating potential water disasters posed by climate warming in this arid region.
Conclusion
Climate change is an unprecedented challenge facing the human community and will significantly influence the earth’s hydrosphere. The present study examines a large sedimentary watershed in the arid northern Tibetan Plateau as an example to numerically investigate the development of groundwater flow systems in an arid basin and their behaviors under climate warming. The main findings are as follows:
The study area is a typical Tóthian basin and has developed three hierarchical groundwater flow systems, i.e. the local, intermediate, and regional systems, under present climate condition. The river seepage that occurs at the middle to upper part of the alluvial-pluvial plain is the predominant recharge water for all systems in the basin, and it accounts for more than 99% of the total water quantity. The distribution of groundwater flow systems is controlled by the sedimentary lithology inside the basin. The local system is distributed in the alluvial-pluvial plain with the shallowest buried features and largest quantity of cycling water. Approximately 82.69% of the cycled groundwater inside the basin is discharged through the local flow system and provides the dominant water resource for human exploitation and the oasis in the watershed. The intermediate system distributing below the local system has the largest cycled depth of ∼600 m. The cycled water in this system accounts about 14.26% of the total groundwater quantity in the basin and is significantly important for the eco-environmental maintenance in the middle and lower watershed. The regional system is located below all the other flow systems and ranges from the mountain pass to the low-lying depression of the basin. The cycled water quantity in this system only accounts for ∼3% and is not the dominant contribution of water and salt minerals for the basin center.
Climate warming would have a significant influence on groundwater systems in the basin. More water would be yielded in the headwater area, and the recharge water of the groundwater system in the basin would be greatly increased. By 2100, climate warming would increase the river seepage water quantity 130.78% of its present level. This giant recharge water quantity would cause a significant disturbance to the groundwater system. Most of the increased water would discharge out of the groundwater system through springs. Influences on other means of discharge would not be significant. Groundwater flow systems produce different responses to climate warming. The local system has the strongest response, followed by the intermediate system. The influence on the regional system is limited and negligible. Most of the increased water due to climate warming would cycle and discharge through the local system and result in a significant increase in groundwater level. This leads to the trailing edge of the overflow belt at the piedmont moving about 5 km toward the mountain pass, threatening a potential water disaster to the local regions. Climate warming would result in a slight increase in the cycled water quantity in the intermediate system but nearly no change for its discharge range. This study can provide scientific insights into the development of groundwater flow systems in a large arid sedimentary basin and their behaviors under climate warming, and is essential to address the water challenges induced by future climate warming in arid and semiarid regions.
Data availability statement
The original contributions presented in the study are included in the article/Supplementary material, and further inquiries can be directed to the corresponding author.
Author contributions
Conceptualization: YX, SW, and YL; methodology: YX, QH, KL, and YZ; formal analysis: YX, HY, SY, and LW; investigation: SW, ZQ, KL, and YL; data curation: SW, KL, ZQ, HY, and LW; software: YX, KL, YZ, and QH; writing—original draft: YX; writing—review and editing: QH; supervision: SW and SY.
Funding
This research was funded by the National Natural Science Foundation of China (grant number 42007183), the Natural Science Foundation of Sichuan Province (grant number 2022NSFSC1084), the Fundamental Research Funds for the Central Universities (grant number 2682021ZTPY063), the Key Lab of Geo-environment of Qinghai Province (grant number 2021KJ011, 2021KJ00102), and the Student Research Training Program of Southwest Jiaotong University (grant number 2022174).
Acknowledgments
The authors appreciate the editors and reviewers for their critical comments and suggestions which greatly helped us to improve the present manuscript.
Conflict of interest
The authors declare that the research was conducted in the absence of any commercial or financial relationships that could be construed as a potential conflict of interest.
Publisher’s note
All claims expressed in this article are solely those of the authors and do not necessarily represent those of their affiliated organizations, or those of the publisher, the editors and the reviewers. Any product that may be evaluated in this article, or claim that may be made by its manufacturer, is not guaranteed or endorsed by the publisher.
References
Adimalla, N. (2019). Groundwater quality for drinking and irrigation purposes and potential health risks assessment: A case study from semi-arid region of South India. Expo. Health 11 (2), 109–123. doi:10.1007/s12403-018-0288-8
Antonellini, M., Giambastiani, B. M. S., Greggio, N., Bonzi, L., Calabrese, L., Luciani, P., et al. (2019). Processes governing natural land subsidence in the shallow coastal aquifer of the Ravenna coast, Italy. CATENA 172, 76–86. doi:10.1016/j.catena.2018.08.019
Brolsma, R. J., van Vliet, M. T. H., and Bierkens, M. F. P. (2010). Climate change impact on a groundwater-influenced hillslope ecosystem. Water Resour. Res. 46 (11), 8782. doi:10.1029/2009WR008782
Bussi, G., Whitehead, P. G., Nelson, R., Bryden, J., Jackson, C. R., Hughes, A. G., et al. (2022). Green infrastructure and climate change impacts on the flows and water quality of urban catchments: Salmons Brook and Pymmes Brook in north-east London. Hydrology Res. 53 (4), 638–656. doi:10.2166/nh.2022.013
Chen, J., Kuang, X., Lancia, M., Yao, Y., and Zheng, C. (2021). Analysis of the groundwater flow system in a high-altitude headwater region under rapid climate warming: Lhasa River Basin, Tibetan Plateau. J. Hydrology Regional Stud. 36, 100871. doi:10.1016/j.ejrh.2021.100871
Chen, Y., Zhi, L., Fan, Y., Wang, H., and Fang, G. (2014). Research progress on the impact of climate change on water resources in the arid region of Northwest China. Acta Geogr. Sin. 69 (9), 1295–1304. doi:10.11821/dlxb201409005
Dash, C. J., Sarangi, A., Singh, D. K., and Adhikary, P. P. (2019). Numerical simulation to assess potential groundwater recharge and net groundwater use in a semi-arid region. Environ. Monit. Assess. 191 (6), 371. doi:10.1007/s10661-019-7508-y
David Raj, A., Kumar, S., and Sooryamol, K. R. (2022). Modelling climate change impact on soil loss and erosion vulnerability in a watershed of Shiwalik Himalayas. Catena 214, 106279. doi:10.1016/j.catena.2022.106279
Díaz-Alcaide, S., and Martínez-Santos, P. (2019). Review: Advances in groundwater potential mapping. Hydrogeol. J. 27 (7), 2307–2324. doi:10.1007/s10040-019-02001-3
Gan, R., Li, D., Chen, C., Yang, F., and Ma, X. (2022). Impacts of climate change on extreme precipitation in the upstream of Chushandian Reservoir, China. Hydrology Res. 53 (3), 504–518. doi:10.2166/nh.2022.135
Ghimire, U., Shrestha, S., Neupane, S., Mohanasundaram, S., and Lorphensri, O. (2021). Climate and land-use change impacts on spatiotemporal variations in groundwater recharge: A case study of the bangkok area, Thailand. Sci. Total Environ. 792, 148370. doi:10.1016/j.scitotenv.2021.148370
Goderniaux, P., Brouyère, S., Blenkinsop, S., Burton, A., Fowler, H. J., Orban, P., et al. (2011). Modeling climate change impacts on groundwater resources using transient stochastic climatic scenarios. Water Resour. Res. 47 (12), 12516. doi:10.1029/2010WR010082
Gu, X., Shao, J., Cui, Y., and Hao, Q. (2017a). Calibration of two-dimensional variably saturated numerical model for groundwater flow in arid inland basin, China. Curr. Sci. 113 (3), 403–412. doi:10.18520/cs/v113/i03/403-412
Gu, X., Xiao, Y., Yin, S., Hao, Q., Liu, H., Hao, Z., et al. (2018). Hydrogeochemical characterization and quality assessment of groundwater in a long-term reclaimed water irrigation area, north China plain. Water 10 (9), 1209. doi:10.3390/w10091209
Gu, X., Xiao, Y., Yin, S., Shao, J., Pan, X., Niu, Y., et al. (2017b). Groundwater level response to hydrogeological factors in a semi-arid basin of Beijing, China. J. Water Supply. Res. Tec. 66 (4), 266–278. doi:10.2166/aqua.2017.093
Hao, Q., Lu, C., Zhu, Y., Xiao, Y., and Gu, X. (2018). Numerical investigation into the evolution of groundwater flow and solute transport in the eastern Qaidam basin since the last glacial period. Geofluids 2018 (1), 1–12. doi:10.1155/2018/9260604
Hao, Q., Shao, J., Cui, Y., and Zhang, Q. (2016). Development of a new method for efficiently calculating of evaporation from the phreatic aquifer in variably saturated flow modeling. J. Groundw. Sci. Eng. 4 (1), 26. doi:10.19637/j.cnki.2305-7068.2016.01.004
Hao, Q., Xiao, Y., Chen, K., Zhu, Y., and Li, J. (2020). Comprehensive understanding of groundwater geochemistry and suitability for sustainable drinking purposes in confined aquifers of the wuyi region, central north China plain. Water 12 (11), 3052. doi:10.3390/w12113052
Hao, S., Zhu, F., and Cui, Y. (2021). Land use and land cover change detection and spatial distribution on the Tibetan Plateau. Sci. Rep. 11 (1), 7531. doi:10.1038/s41598-021-87215-w
Hatch-Kuri, G., and Carrillo-Rivera, J. J. (2021). “Groundwater flow systems and their importance in the assessment of transboundary groundwater: The Mexico–U.S.A. Case,” in Intensified land and water use: A holistic perspective of local to regional integration. Editor M. M. Alconada-Magliano (Cham: Springer International Publishing), 141.
Hemmerle, H., and Bayer, P. (2020). Climate change yields groundwater warming in bavaria, Germany. Front. Earth Sci. (Lausanne). 8, 575894. doi:10.3389/feart.2020.575894
Hu, L., and Jiao, J. J. (2015). Calibration of a large-scale groundwater flow model using GRACE data: A case study in the Qaidam basin, China. Hydrogeol. J. 23 (7), 1305–1317. doi:10.1007/s10040-015-1278-6
Hu, Q., Jiang, D., and Fan, G. (2015). Climate change projection on the Tibetan Plateau: Results of CMIP5 models. Chin. J. Atmos. Sci. 39 (2), 260.
Huang, F., Chunyu, X., Zhang, D., Chen, X., and Ochoa, C. G. (2020). A framework to assess the impact of ecological water conveyance on groundwater-dependent terrestrial ecosystems in arid inland river basins. Sci. Total Environ. 709, 136155. doi:10.1016/j.scitotenv.2019.136155
Islam, M. B., Firoz, A. B. M., Foglia, L., Marandi, A., Khan, A. R., Schüth, C., et al. (2017). A regional groundwater-flow model for sustainable groundwater-resource management in the south Asian megacity of Dhaka, Bangladesh. Hydrogeol. J. 25 (3), 617–637. doi:10.1007/s10040-016-1526-4
Kim, S. J., Asadzadeh, M., and Stadnyk, T. A. (2022). Climate change impact on water supply and hydropower generation potential in Northern Manitoba. J. Hydrology Regional Stud. 41, 101077. doi:10.1016/j.ejrh.2022.101077
Kraaijenbrink, P. D. A., Bierkens, M. F. P., Lutz, A. F., and Immerzeel, W. W. (2017). Impact of a global temperature rise of 1.5 degrees Celsius on Asia’s glaciers. Nature 549 (7671), 257–260. doi:10.1038/nature23878
Kuang, X., and Jiao, J. J. (2016). Review on climate change on the Tibetan Plateau during the last half century. J. Geophys. Res. Atmos. 121 (8), 3979–4007. doi:10.1002/2015jd024728
Li, P., He, X., Li, Y., and Xiang, G. (2019). Occurrence and health implication of fluoride in groundwater of loess aquifer in the Chinese loess plateau: A case study of tongchuan, northwest China. Expo. Health 11 (2), 95–107. doi:10.1007/s12403-018-0278-x
Liu, J., Ma, Y., Gao, Z., Zhang, Y., Sun, Z., Sun, T., et al. (2022). Fluoride contamination, spatial variation, and health risk assessment of groundwater using GIS: A high-density survey sampling in weifang city, north China. Environ. Sci. Pollut. Res. 29 (23), 34302–34313. doi:10.1007/s11356-021-18443-w
Luo, Y., Xiao, Y., Hao, Q., Zhang, Y., Zhao, Z., Wang, S., et al. (2021). Groundwater geochemical signatures and implication for sustainable development in a typical endorheic watershed on Tibetan plateau. Environ. Sci. Pollut. Res. 28 (35), 48312–48329. doi:10.1007/s11356-021-14018-x
Ma, R., Huang, J., Tian, H., Cui, X., and Wang, D. (2017a). Characteristics of precipitation,evaporation and temperature at the Golmud River Catchment in recent 60 years. Arid. Land Geogr. 40 (5), 1005.
Ma, Y., Ma, W., Zhong, L., Hu, Z., Li, M., Zhu, Z., et al. (2017b). Monitoring and modeling the Tibetan plateau’s climate system and its impact on east asia. Sci. Rep. 7 (1), 44574. doi:10.1038/srep44574
Minea, I., Boicu, D., Amihăiesei, V., and Iosub, M. (2022). Identification of seasonal and annual groundwater level trends in temperate climatic conditions. Front. Environ. Sci. 10, 852695. doi:10.3389/fenvs.2022.852695
Moharir, K., Pande, C., Singh, S. K., Choudhari, P., Kishan, R., and Jeyakumar, L. (2019). Spatial interpolation approach-based appraisal of groundwater quality of arid regions. J. Water Supply Res. Technology-Aqua 68 (6), 431–447. doi:10.2166/aqua.2019.026
Mookiah, M., Jha, S. K., and Biswas, A. (2021). Assessment of groundwater mass balance and zone budget in the semi-arid region: A case study of palar sub-basin, Tamil nadu, India. J. Earth Syst. Sci. 130 (4), 187. doi:10.1007/s12040-021-01691-2
Nan, Z., Li, S., and Cheng, G. (2004). Scenario prediction of permafrost change on the Qinghai-Tibet Plateau in the next 50 and 100 years. Sci. China Ser. D Earth Sci. 34 (6), 528–534. doi:10.1360/zd2004-34-6-528
Qu, B., Zhang, Y., Kang, S., and Sillanpää, M. (2019). Water quality in the Tibetan plateau: Major ions and trace elements in rivers of the “water tower of asia”. Sci. Total Environ. 649, 571–581. doi:10.1016/j.scitotenv.2018.08.316
Qu, S., Shi, Z., Liang, X., Wang, G., and Jin, X. (2021). Origin and controlling factors of groundwater chemistry and quality in the Zhiluo aquifer system of northern Ordos Basin, China. Environ. Earth Sci. 80 (12), 439. doi:10.1007/s12665-021-09735-y
Rafiei-Sardooi, E., Azareh, A., Joorabian Shooshtari, S., and Parteli, E. J. R. (2022). Long-term assessment of land-use and climate change on water scarcity in an arid basin in Iran. Ecol. Model. 467, 109934. doi:10.1016/j.ecolmodel.2022.109934
Sarzaeim, P., Bozorg-Haddad, O., Fallah-Mehdipour, E., and Loáiciga, H. A. (2017). Climate change outlook for water resources management in a semiarid river basin: The effect of the environmental water demand. Environ. Earth Sci. 76 (14), 498. doi:10.1007/s12665-017-6834-z
Shi, Y. (2001). Estimation of the water resources affected by climatic warming and glacier shrinkage before 2050 in west China. J. Glaciol. Geocryol. 23 (4), 333.
IPCC (2022). “Climate change 2022: Mitigation of climate change”, (Eds) P. R. Shukla, R. S. J. Skea, A. A. Khourdajie, R. v. Diemen, D. McCollum, M. Pathaket al. (Cambridge, UK and New York, NY, USA: IPCC.
Straneo, F., and Heimbach, P. (2013). North Atlantic warming and the retreat of Greenland's outlet glaciers. Nature 504 (7478), 36–43. doi:10.1038/nature12854
Wang, S., Xie, Z., Wang, F., Zhang, Y., Wang, W., Liu, K., et al. (2022). Geochemical characteristics and quality appraisal of groundwater from huatugou of the Qaidam basin on the Tibetan plateau. Front. Earth Sci. (Lausanne). 10. doi:10.3389/feart.2022.874881
Xiao, Y., Hao, Q., Zhang, Y., Zhu, Y., Yin, S., Qin, L., et al. (2022a). Investigating sources, driving forces and potential health risks of nitrate and fluoride in groundwater of a typical alluvial fan plain. Sci. Total Environ. 802, 149909. doi:10.1016/j.scitotenv.2021.149909
Xiao, Y., Liu, K., Hao, Q., Li, Y., Xiao, D., and Zhang, Y. (2022b). Occurrence, controlling factors and health hazards of fluoride-enriched groundwater in the lower flood plain of Yellow River, Northern China. Expo. Health 14, 345–358. doi:10.1007/s12403-021-00452-2
Xiao, Y., Liu, K., Hao, Q., Xiao, D., Zhu, Y., Yin, S., et al. (2022c). Hydrogeochemical insights into the signatures, genesis and sustainable perspective of nitrate enriched groundwater in the piedmont of Hutuo watershed, China. Catena 212, 106020. doi:10.1016/j.catena.2022.106020
Xiao, Y., Liu, K., Yan, H., Zhou, B., Huang, X., Hao, Q., et al. (2021). Hydrogeochemical constraints on groundwater resource sustainable development in the arid Golmud alluvial fan plain on Tibetan plateau. Environ. Earth Sci. 80 (22), 750. doi:10.1007/s12665-021-10076-z
Xiao, Y., Shao, J., Frape, S., Cui, Y., Dang, X., Wang, S., et al. (2018). Groundwater origin, flow regime and geochemical evolution in arid endorheic watersheds: A case study from the Qaidam basin, northwestern China. Hydrol. Earth Syst. Sci. 22 (8), 4381–4400. doi:10.5194/hess-22-4381-2018
Xu, Y.-S., Yuan, Y., Shen, S.-L., Yin, Z.-Y., Wu, H.-N., and Ma, L. (2015). Investigation into subsidence hazards due to groundwater pumping from Aquifer II in Changzhou, China. Nat. Hazards (Dordr). 78 (1), 281–296. doi:10.1007/s11069-015-1714-x
Zhang, Y., Dai, Y., Wang, Y., Huang, X., Xiao, Y., and Pei, Q. (2021a). Hydrochemistry, quality and potential health risk appraisal of nitrate enriched groundwater in the Nanchong area, southwestern China. Sci. Total Environ. 784, 147186. doi:10.1016/j.scitotenv.2021.147186
Keywords: groundwater flow system, climate change, numerical modelling, arid watershed, Tibetan plateau
Citation: Xiao Y, Liu K, Zhang Y, Yang H, Wang S, Qi Z, Hao Q, Wang L, Luo Y and Yin S (2022) Numerical investigation of groundwater flow systems and their evolution due to climate change in the arid Golmud river watershed on the Tibetan Plateau. Front. Earth Sci. 10:943075. doi: 10.3389/feart.2022.943075
Received: 13 May 2022; Accepted: 28 July 2022;
Published: 14 September 2022.
Edited by:
Filippo Catani, University of Florence, ItalyReviewed by:
Jiajun Peng, Kyoto University, JapanBo Zhao, Institute of Mountain Hazards and Environment (CAS), China
Copyright © 2022 Xiao, Liu, Zhang, Yang, Wang, Qi, Hao, Wang, Luo and Yin. This is an open-access article distributed under the terms of the Creative Commons Attribution License (CC BY). The use, distribution or reproduction in other forums is permitted, provided the original author(s) and the copyright owner(s) are credited and that the original publication in this journal is cited, in accordance with accepted academic practice. No use, distribution or reproduction is permitted which does not comply with these terms.
*Correspondence: Shengbin Wang, d3NiMTM4OTc2NTEwOTZAMTI2LmNvbQ==