- 1State Key Laboratory of Mining Response and Disaster Prevention and Control in Deep Coal Mines, Anhui University of Science and Technology, Huainan, China
- 2Institute of Energy, Hefei Comprehensive National Science Center, Hefei, China
- 3Center for Rock Instability and Seismicity Research, School of Resources and Civil Engineering, Northeastern University, Shenyang, China
Many coal mines are being abandoned for economic and environmental reasons in China. The repurposing of abandoned open-pit coal mines into pumped storage hydropower (PSH) can help with the storage of renewable energy, improve mine environments, and provide added economic value. Construction of PSH plant will change the water level of the abandoned pit, which is envisaged as the lower reservoir, thus influencing the slope stability. In this study, the Fushun West Open-Pit coal mine was taken as an example. Borehole investigation and tilt photogrammetry were used to obtain the rock mass quality and volume of the abandoned pit. A natural lake, dumps, and another open-pit mine are envisaged as the upper reservoir, which could bring 40, 3,200, and 2,000 MW electricity installed capacity, and −295 m, −200 m, and −150 m water levels in the lower reservoir. The numerical analysis software GeoStudio was used for slope stability analysis considering the hydraulic effect under different water levels. A potential landslide risk may happen in several zones under specific water levels. Further imperviousness and reinforcement work is needed to guarantee slope stability for PSH plant construction in the Fushun West Open-Pit coal mine.
Introduction
In China in 2021, coal-power plant created 59.67% of electricity generation, which is the largest source of CO2 emissions. Coal is the most carbon-intensive fissile fuel, therefore phasing it out is critical to limit climate change. As one of the major providers of public finance for coal phase-out projects, China has pledged that carbon emissions will peak by 2030, and neutrality by 2060; that is, it has “dual carbon goals.” In recent years, renewable energy including sources such as solar and wind has taken a more and more important part of the electricity market. During this period, many coal mines have been abandoned. According to statistical data from the Chinese Academy of Engineering (Yuan et al., 2018; Yuan and Yang, 2021), there will 12,000 abandoned coal mines in China by 2020, and this number will increase to 15,000 in 2030. Intermittent renewable energy sources are not feasible due to their fluctuating nature, which may be negative for electrical systems or may waste energy. Energy storage allows energy from intermittent sources to be saved for periods of higher demand, which could reduce imbalances between energy demand and energy production. The efficiency of PSH is typically between 70 and 85%, making it one of the more efficient methods for storing energy (Chen et al., 2009; Pérez-Díaz et al., 2015; Fan et al., 2020). The United States Department of Energy Global Energy Storage Database reports that PSH accounts for around 95% of all energy storage installations worldwide, with a total installed throughput capacity of over 181 GW (Uría-Martínez et al., 2021).
Starting from public data of the National Energy Administration of China, and National Development and Reform Commission of China, there were 6,571 abandoned coal mines during 2014–2018, and their spatial distribution is shown in Figure 1. The mines are mainly distributed in northeastern and middle China. They may lead to continuous ground subsidence, blackdamp accumulation, and hidden cracks, which pose a hazard to safety and the environment. In fact, abandoned coal mines, especially underground coal mines with a long history, are hard to reclaim. The difficulties include missing geological and mining data, and also stakeholders. The ownership of abandoned coal mines is usually returned to the Chinese government, and the reuse of these mines needs additional approval. Coal mines in production but ready to be abandoned gain more interest. As shown in Figure 2, China’s production capacity of coal is mainly distributed in northern and eastern China, which may see a substantial decline in the next decades under the “dual carbon goals” (Dong et al., 2016). These coal mines that are to be abandoned have a good spatial correlation with China’s main wind and solar farms (He and Kusiak, 2017; Li et al., 2019; Li et al., 2021), and it is of great engineering significance to use these coal mines for energy storage.
PSH plant operates with at least two water reservoirs, including an upper and a lower reservoir, located at different elevation levels. Pumps driven by the excess renewable energy can transport the water into the upper reservoir. There it is stored in the form of potential energy, which can be converted back into electrical energy by releasing the water into the lower reservoir. In this case, the available volume and the height difference of the two reservoirs are key to the installed capacity of the PSH plant. The main disadvantage of PSH is the special nature of the site required, needing both geographical height and water availability. Suitable sites are therefore likely to be in hilly or mountainous regions, and potentially in areas of natural beauty, making PSH susceptible to social and environmental issues. As shown in Figure 3, abandoned coal mines, especially open-pit coal mines, can provide abundant space and water resources for PSH, which have a lower environmental and societal impact. Besides, the reuse of abandoned coal mines can contribute to adding value to local communities after the cessation of mining activities. Compared with abandoned underground coal mines, open-pit mines can provide more reliable and viewable space, and the installation of PSH will not be limited for the sizes of shafts or tunnels. Open-pit space is more concentrated, which means less support and imperviousness costs.
Despite the benefits, there are few PSH plant using abandoned open-pit coal mines being constructed, and related researches remain in initial stages. Nevertheless, Wessel et al. (2015) analyzed the economic evaluation of a PSH plant erected in an abandoned open-pit mine, and they found that PSH in an abandoned open-pit mine can be constructed at reasonably low investment costs and operated at low specific production costs. Menéndez and Loredo (2019) analyzed the potential usage of abandoned coal mines for PSH and productive restoration for biomass production in northern Spain. Guo et al. (2019) analyzed the economic, environmental, and social indicators of PSH using abandoned open-pit mines. Pujades et al. (2016; 2017) studied the influence of groundwater exchanges on the efficiency of PSH plant using open-pit mines. Madlener and Specht (2020) proved that PSH plant using abandoned coal mines is both technically feasible and economically reasonable in Germany. Poulain et al. (2021) investigated the hydrodynamic and hydrochemical impacts of PSH activities on water inside quarries and in the surrounding rock media.
Current research has indicated the economic and technical feasibility of PSH plant using abandoned open-pit mines. In this study, the Fushun West Open-Pit coal mine is considered as a PSH plant to be reformed. The engineering background, advantages, and benefits of this motivation are introduced in detail. Tile photogrammetry was then used to obtain the volume and area of the open pit, considered the lower reservoir. We designed a three-phase construction scheme using the natural lake, dumps, and another open pit as the upper reservoir. The installed capacity was estimated, as well as the water level of lower reservoir. Finally, numerical simulation software GeoStudio was used to analyze the slope stability considering the hydraulic effect under different water levels.
Engineering background of the Fushun West Open-Pit coal mine
Project overview
The Fushun West Open-Pit coal mine, located in Fushun City, Liaoning Providence, P.R. China, is a state-owned company. Over 7,000 workers are employed here. Fushun is one of the resource-depleted cities in China, and economic development is a challenge. In the past hundred years, over 190 million tons of coal and 480 million tons of oil shale have been mined, forming the largest open pit in Asia. The pit is located in downtown Fushun City, and many important buildings are distributed around the pit, the closest distance being 200 m. Because of resource depletion, the Fushun West Open-Pit coal mine will be abandoned in the next few years. Repurposing of the abandoned open pit as a PSH plant for excess renewable energy will contribute to the Chinese “dual carbon goals,” while increasing its economic value and stabilizing the regional job market.
As shown in Figure 4, the mine has a length of 6.6 km from east to west, a width of 2.2 km from south to north, and a maximum height of the slope reaching 420 m in 2021. The average altitude of the surface is +80 m in the north, and +120 m in the south, while the bottom of the pit is −350 m. Another open-pit coal mine with the shortest linear distance of 500 m is located in the east, called Fushun East Open-Pit coal mine. The Hun River is 1.7 km north of the pit, and the annual runoff of the Hun River ranges from 5 billion m3 to 7 billion m3 (Luo et al., 2022). A tributary of the Hun River, namely Guchengzi River, is located in the west at a distance of 1.6 km. As shown in Figure 4, Nanhuayuan Lake is there, marked in pink, which could be reformed as the upper reservoir in the PSH plant. Besides, three dumps (Dongshechang, Xishechang, and Wangliang), marked in red in Figure 4, are distributed to the southeast and southwest of the pit.
Engineering geology
The engineering geology is rather complicated in the Fushun West Open-Pit coal mine. A typical profile (exploration line E1600) is shown in Figure 5 and its location is marked by the dashed red line in Figure 4. A syncline controls the orientation of the strata. The strike of the syncline axis is roughly east–west, and the two wings are nearly symmetrical. There is interbedding of mudstone and shale distributed in the center of the syncline. The thickness of the coal seam ranges from 30 to 145 m, with an average value of 55.5 m. The roof and floor of the coal seam are oil shale and tuff, respectively. Two reverse faults are distributed in the north slope, namely F1 and F1A. The strike of F1 is NE 80° and the dip angle varies from 47° to 52°, while the strike of F1A varies from NE 80° to NE 85° and the dip angle varies from 70° to 75°. The intersection of the two faults forms creates an inverted triangle rock mass composed of Cretaceous sandstone. The surface is relatively flat, and Fushun city downtown sits at the crest of the northern slope.
Over 100 historical landslides of different sizes have occurred in the Fushun West Open-Pit coal mine, and many researchers have done studies on the stability analyses and deformation monitoring of its high and steep rock slopes (Yang et al., 2014; He et al., 2015; Zhang et al., 2019). In this study, an engineering geological borehole was drilled in the north slope, and the location of this borehole is marked in Figure 5. Core taken from the borehole is shown in Figure 6. As we can see, shallow rock masses are heavily weathered, while deep rock masses remain in a slightly weathered state. The RQD and joint spacing of the cores were measured. A point load test was used to find the strength of the intact rock, and then transformed using uniaxial compression strength (UCS), according to the suggested method of the International Society for Rock Mechanics (ISRM). There are several water outlet points in the slope, and rock masses are generally “damp.” Finally, the RMR method was employed to evaluate the rock mass quality. As shown in Figure 6, the rock masses are mainly “very poor, RMR <20,” “poor, 20 < RMR <41,” and “fair 40 < RMR <61.” We believe mechanical analyses of the abandoned open pit should focus on the impervious design and slope stability while the PSH plant is installed.
Reservoir design of the PSH plant in the Fushun West Open-Pit coal mine
Lower reservoir
Tilt photogrammetry was used to obtain the three-dimensional (3D) surface of the Fushun West Open-Pit coal mine. The tilt photogrammetry system includes a DJ M300 RTK drone and a SHARE 102S five-lens camera. The five-lens camera helped to obtain images from different directions. DJL Terra software was used for geometric correction, regional network joint adjustment, and tilt image matching. Finally, a 3D surface containing point cloud and texture was established, shown in Figure 7. The 3D surface was transferred into digital orthophoto map (DOM) and digital elevation model (DEM). The DEM was inputted into geographic information system (GIS) software to carry out the inundation analysis, as well as emulate the volume of the pit at different altitudes. As shown in Figure 8, the volume of the pit increases with the water level. The cumulative volume of the pit is 91 million m3 when the water level reaches −150 m, and 1019 million m3 when the water level is +70 m, which is the maximum altitude of the pit. The result indicated that there is a very huge space in the Fushun West Open-Pit coal mine. Carrying out PSH in the abandoned open pit would be greatly convenient and cost-effective.
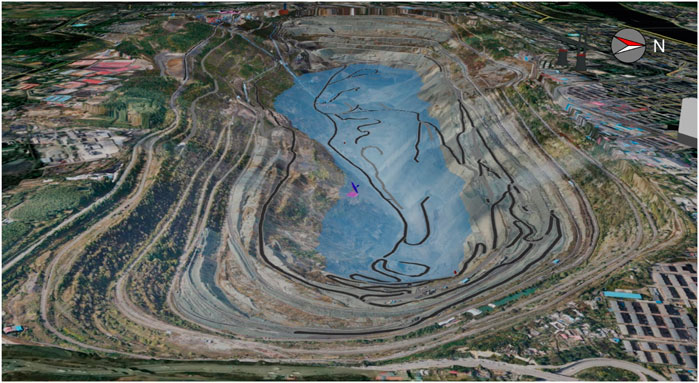
FIGURE 7. Inundation analysis using the 3D surface obtained by tilt photogrammetry. The gray line represents the transportation road, and the light blue represents water.
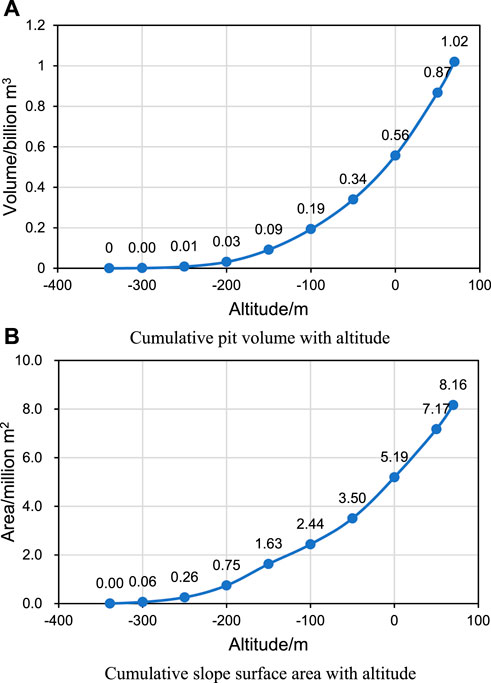
FIGURE 8. Relationship between altitude, volume, and area of the Fushun West Open-Pit coal mine. (A) Cumulative pit volume with altitude. (B) Cumulative slope surface area with altitude.
The average water inflow in the Fushun West Open-Pit coal mine is 64,000 m3/d. Here we assumed that groundwater recharge is stable and the water is stored in a natural way; 3.9 and 43.6 years are needed if the water level reaches −150 m and +70 m, respectively. Thus, we need additional water sources to form the lower reservoir of the PSH plant faster. The Hun River can share its 1/30 runoff, about 200 million m3 of water into the Fushun West Open-Pit coal mine. In all, 5.5 months and 5.1 years are needed for the water levels to reach −150 m and +70 m with artificial water storage. Besides, water used in the PSH plant should be kept clean to avoid damage to the hydraulic turbine. The water stored in the reservoir could discharge into the Guchengzi River, and then inflow into the Hun River periodically. A tunnel/canal is needed to connect the Hunhe River and the open pit, and another tunnel/canal connecting Guchengzi River and the open pit, as shown in Figure 4.
Abandoned open pits cannot be considered to be impervious reservoirs, and they are connected to the surrounding rock masses which are a porous medium. Water exchange will influence the PSH efficiency and will bring environmental impacts (Poulain et al., 2021). Figure 8 shows the relationship between the cumulative area of slope surface and its altitude. The cumulative slope surface is 5.19 million m2 and 8.16 million m2 when the water level reaches 0 m and +70 m. A preliminary scheme for imperviousness includes the following steps: 1) The bottom and slope surfaces of the open pit is clean and flat. 2) A certain thickness of homogeneous clay is laid evenly at the bottom of the open pit, and a geomembrane with high breaking strength, tensile strength, and elongation is selected to cover the clay. 3) Concrete is used to reinforce the jointed or highly weathered rock masses on the slope surface.
Upper reservoir
The altitude difference between the upper and lower reservoirs should remain relatively high, which could provide the water head for the hydraulic turbine. Besides, the effective storage capacity (volume of the reservoir between maximum and minimum water levels) of the two reservoirs should be equal. This is the reason why commercially and socially acceptable site selection for PSH plants is a critical issue (Rehman et al., 2015). A reversible pump turbine is used to pump the water into the upper reservoir, where excess electric power is stored in the form of potential energy. During times of high electric power demand, the reversible pump turbine can generate electric power by releasing the water into the lower reservoir, shown in Figure 3. According to Liaoning Province’s “14th five-year plan,” the overall installed capacity of wind and solar energy will increase to 30,000 MW by 2025. In the Fushun West Open-Pit coal mine, the huge volume of the pit proves the potentiality of installed capacity. The site selection of the upper reservoir is key for the PSH plant. Reference is made to the experience of other large PSH plant in China including Fengning PSH plant, Hongping PSH plant, and Guangzhou PSH plant (Zhang et al., 2015). Phased construction is suitable for the Fushun West Open-Pit coal mine, since it can provide experience, recapture underutilized space, and economize the overall occupancy costs.
We designed three phases for the upper reservoir construction of the PSH plant in the Fushun West Open-Pit coal mine. In phase I, the available Nanhuayuan Lake can be considered as an upper reservoir, and the average altitude of Nanhuayuan Lake is +95 m. In phase II, three dumps are transformed into upper reservoirs, with the altitude of three dumps being +185 m, +180 m, and +150 m for Xishechang, Dongshechang, and Wangliang, respectively. The Fushun East Open-Pit coal mine will be abandoned by 2035, and the altitude of the pit bottom will be −270 m. In phase III, the abandoned Fushun East Open-Pit coal mine can be repurposed as an upper reservoir. Dumps can provide a higher water head, compared with the Fushun East Open-Pit mine for the construction of the PSH plant. Unfortunately, the dumps are composed of crushed stone, so more imperviousness and reinforcement work is needed.
The installed capacity N of the PSH plant is calculated with Eq. 1:
where
Slope stability analysis under different water levels
According to the experience of the hydropower station, water level fluctuation is noted as the main factor resulting in instability and failure of the reservoir slope. Accurate slope stability analysis under water level fluctuation is key for ensuring the safety of the reservoir projects (Zhang et al., 2012; Xiong et al., 2019; Cui et al., 2021). In particular, the seepage and stress coupling effects have a significant influence on the slope stability. As we mentioned in the ‘Upper Reservoir’ section, the water level of the lower reservoir is −295 m, −200 m, and −150 m under the three construction phases. In this study, the modular of SEEP/W and SLOPE/W of the numerical analysis tool GeoStudio are used to analyze the slope stability of the Fushun West Open-Pit coal mine under different water levels. In this process, the hydraulic analysis result from SEEP/W is used as the starting point for SLOPE/W, and the safety factor of rock slope is calculated.
In the Fushun West Open-Pit coal mine, 2D hydraulic analyses and slope stability analyses were performed in the representative profile E1600. Referring to the earlier researches (Yang et al., 2005; Yang et al., 2014), the mechanical and hydraulic parameters of the rock masses are listed in Table 1. Models built by SEEP/W were used for 2D hydraulic analysis using Darcy’s law, and the rock masses were considered as both a saturated and unsaturated medium. The average element size of the numerical model is 10 m with triangle-quad hybrid mesh. A total of 24,441 nodes and 24,419 elements were meshed. Borehole investigation revealed the underground water level of the slope which is approximately −60 m in the south. Besides, the Hun River is located in the north with a water level of 25 m. The corresponding water heads are set as the boundary conditions on the left and right, shown as the red line in Figure 9. The total water heads are −295 m, −200 m, and −150 m in three phases, respectively, shown as the blue line in Figure 9.
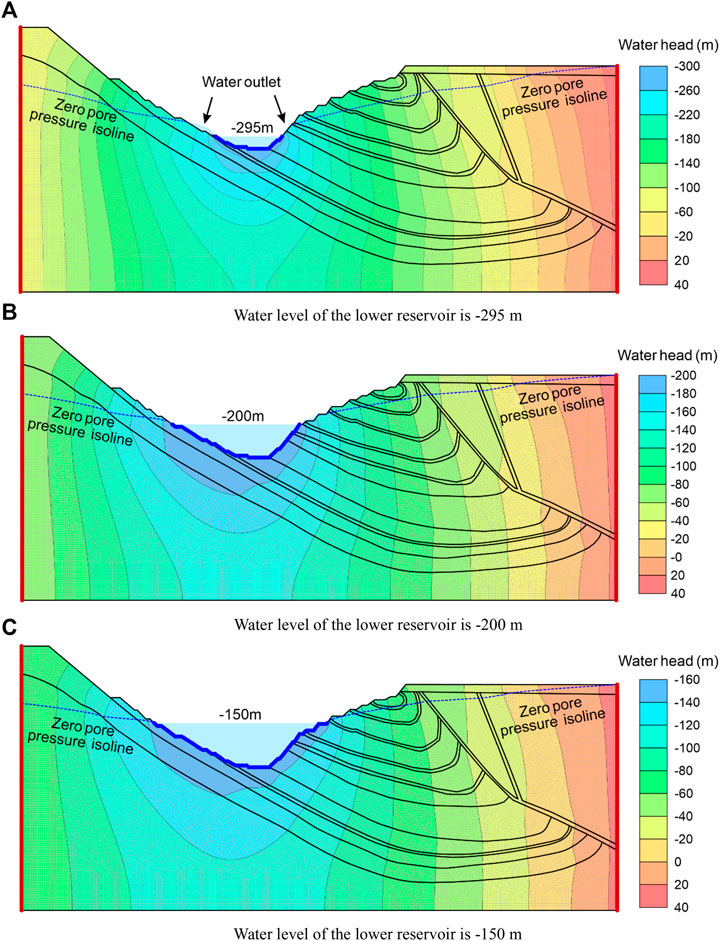
FIGURE 9. Hydraulic analyses under different water levels. (A) Water level of the lower reservoir is −295 m. (B) Water level of the lower reservoir is −200 m. (C) Water level of the lower reservoir is −150 m.
As shown in Figure 9, the isoline of the water head is approximately vertical in the side boundary, and tends to be parallel to the slope surface while near the pit. Besides, the water head is a steady decline from the side boundary to the pit. The zero pore pressure isolines of all three phases are relatively smooth, marked as the blue dotted line in Figure 9. The zero pore pressure isoline starts from the zero water head point in the boundary and extends to the water level of the pit (lower reservoir). Especially, the zero pore pressure isoline is higher than the water level in the southern slope, while the water level of the pit is −295 m, which means there are several potential water outlet points in working condition.
The hydraulic analysis result from SEEP/W was then imported into SLOPE/W to carry out the slope stability analysis. We adopted the Mohr-Column constitutive model for the limit equilibrium method using the rock mass mechanical parameters listed in Table 1. Since the upper and lower slopes have different slope angles, both in the south and north of the pit, four kinds of slope stability analysis schemes were taken into consideration, shown in Figure 10. In the northern pit, the upper slope ranges from −43 m to −183 m, and the slope ranges from −199 m to −350 m were divided into two independent zones for slope stability analysis. In the southern pit, two independent zones including the slope ranges from +200 m to −210 m, and slope ranges from -210 m to −323 m were divided. As shown in Figure 10, the safety factor and potential slide body (the green region in Figure 10) of those slope stability analysis schemes were obtained.
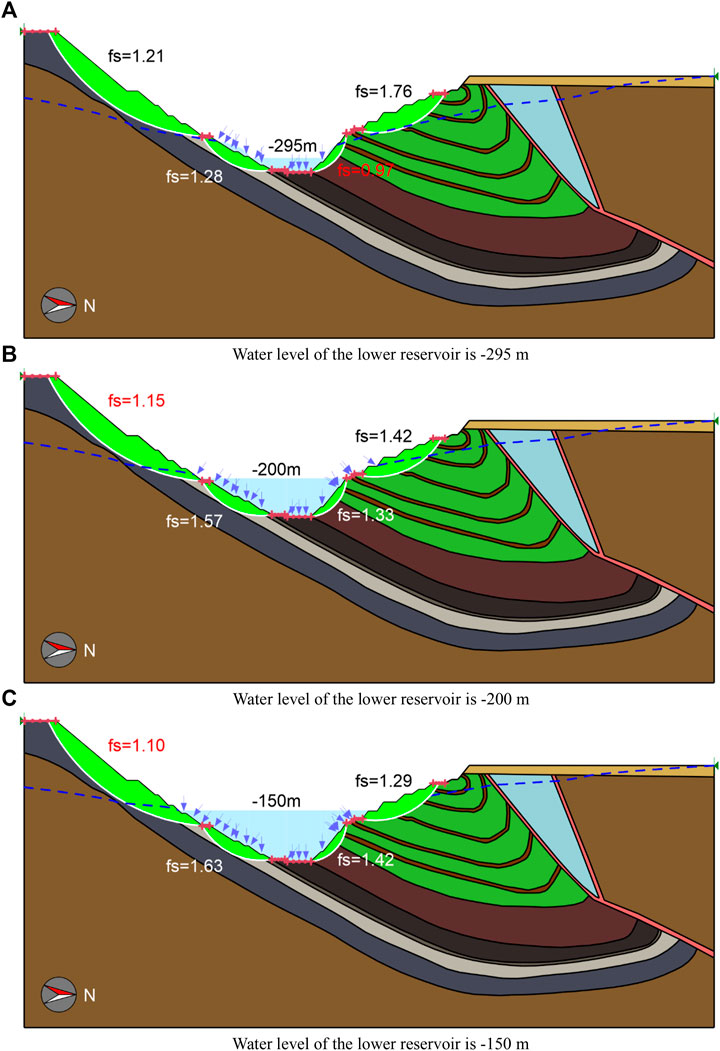
FIGURE 10. Slope stability analyses under different water levels. (A) Water level of the lower reservoir is −295 m. (B) Water level of the lower reservoir is −200 m. (C) Water level of the lower reservoir is −150 m.
The lower slopes are mainly distributed below the zero pore pressure isoline. A higher water level could bring additional hydrostatic pressure on the slope surface, thus delivering a higher safety factor. The zero pore pressure isoline is preferred, to pass through the upper slope in both the southern and northern pits; a higher water level would decrease the effective stress which would be negative to the slope stability. The safety factor of the upper slope decreases with the increase of the water level. In particular, a safety factor of less than 1.20 means that the slope remains in a critical state. These working conditions are marked in red in Figure 10. Poor rock mass quality and high zero pore pressure isoline are the main reasons for rock landslide in the Fushun West Open-Pit mine. Further imperviousness and reinforcement work is needed to improve the slope stability.
Discussion
Many researches have indicated the technically and economically feasibility of PSH plant using abandoned mines. Until now, no such PSH plant is operated or has been constructed in China. The three most possible abandoned open-pit mines that could be reformed as PSH plant are Fushun West Open-Pit coal mine presented in this article, Haizhou Open-Pit coal mine located in Liaoning Province (Maihemuti et al., 2016), and Luanping Open-Pit iron mine located in Hebei Province. According to the published information from the State Grid and the China Southern Grid which are the main suppliers of PSH plant in China, the average construction cost of regular PSH projects in the State Grid was 5.32 Chinese yuan/W and 5.46 Chinese yuan/W in the China Southern Power Grid. The construction cost of PSH plant using abandoned open-pit mines is unknown in China. Due to the imbalance between production and consumption of electricity, a PSH plant could buy electricity at a low price and sell at a high price on the electricity market. The Chinese government will give financial support including subsidies and tax preference to PSH plant. Besides, the reuse of dumps and abandoned open pits could solve a series of pollution problems in the surrounding environment. The construction of PSH plant could also bring environmental restoration and geological disaster control. In this article, we give a primitive estimation of possible installed capacity, and detailed economic analyses are still needed in further studies.
The main rock mechanical risk for the reservoirs of PSH plant includes slope instability (Song et al., 2017) and groundwater exchange (Wang et al., 2014; Bodeux et al., 2017). The cyclic pumping and injection result in a rapid fluctuation of water levels in the reservoirs of the PSH plant, and the water injection from the upper reservoir to the lower reservoir will lead to a cyclic dynamic load on the slope rock masses. These impacts have a negative influence on the properties of slope rock masses, thus slope stability. Groundwater exchange between reservoirs and surrounding rock masses will influence the efficiency of the pumps and turbines, because of affecting the head difference between the reservoirs. Impervious design and slope stability analyses should be the focus of mechanical analysis in further studies. Besides, monitoring and an early warning method for rock landslides are a cost-effective way to guarantee the safety of the reservoir slope (Liu et al., 2021; Zhou et al., 2021; Li et al., 2022).
Conclusion
Based on the engineering background of the Fushun West Open-Pit coal mine, we designed a PSH plant which reformed the abandoned pit as the lower reservoir, and the natural lake, dumps, and another open pit were considered the upper reservoir. PSH plant using abandoned open-pit mines can bring economic, social, and environmental benefits. The other conclusions of this study can be summarized as follows:
1) The tilt photogrammetry result revealed that the cumulative volume and area of the pit is 1019 million m3, which has an enormous potential usage for PSH plant construction. Borehole investigation showed the rock masses quality RMR of the pit is generally “very poor,” “poor,” and “fair,” so imperviousness design and slope stability should be the focus of mechanical analysis.
2) Nanhuayuan Lake, three dumps, and Fushun East Open-Pit coal mine are designed as the upper reservoir of the phased PSH plant construction. A primitive estimation shows that the installed capacity of the PSH plant in three phases could reach 40, 3,200, and 2,000 MW, and the water levels of the lower reservoir are -295 m, -200 m, and -150 m, respectively.
3) Numerical simulation using GeoStudio software in the Fushun West Open-Pit coal mine indicated that the water level does have a significant influence on the slope stability. The increase of the water level has a positive effect on slope stability while the slope is under the zero pore pressure isoline, and negative while the zero pore pressure isoline crosses the potential slide body. While using the abandoned pit as lower reservoir for the PSH plant construction, slope stability analysis revealed that the pit has a potential landslide risk which needs further imperviousness and reinforcement work.
Data availability statement
The original contributions presented in the study are included in the article/supplementary material, and further inquiries can be directed to the corresponding author.
Author contributions
FL contributed the conception and design of the study. KY and TY are the funder and supervisor of this manuscript, and they also contributed the data collection. YG and JL completed the engineering geology investigation and wrote the “Engineering background of the Fushun West Open-Pit coal mine.” QL and QF performed the statistical analysis.
Funding
This work is supported by the University Natural Science Research Project of Anhui Province (KJ2021A0458), the Institute of Energy, Hefei Comprehensive National Science Center (21KZS215), the National Key Research and Development Program of China (2017YFC1503101), the Collaborative Innovation Project of Anhui Universities (GXXT-2019-029), and the National Science Foundation of China (U1903216).
Conflict of interest
The authors declare that the research was conducted in the absence of any commercial or financial relationships that could be construed as a potential conflict of interest.
Publisher’s note
All claims expressed in this article are solely those of the authors and do not necessarily represent those of their affiliated organizations, or those of the publisher, the editors, and the reviewers. Any product that may be evaluated in this article, or claim that may be made by its manufacturer, is not guaranteed or endorsed by the publisher.
References
Bodeux, S., Pujades, E., Orban, P., Brouyère, S., and Dassargues, A. (2017). Interactions between groundwater and the cavity of an old slate mine used as lower reservoir of an UPSH (underground pumped storage hydroelectricity): A modelling approach. Eng. Geol. 217, 71–80. doi:10.1016/j.enggeo.2016.12.007
Chen, H. S., Cong, T. N., Yang, W., Tan, C. Q., Li, Y. L., and Ding, Y. L. (2009). Progress in electrical energy storage system: A critical review. Prog. Nat. Sci. 19 (3), 291–312. doi:10.1016/j.pnsc.2008.07.014
Cui, S. H., Pei, X. J., Jiang, Y., Wang, G. H., Fan, X. M., Yang, Q. W., et al. (2021). Liquefaction within a bedding fault: Understanding the initiation and movement of the Daguangbao landslide triggered by the 2008 Wenchuan Earthquake (Ms=8.0). Eng. Geol. 295, 106455. doi:10.1016/j.enggeo.2021.106455
Dong, J., Feng, T. T., Sun, H. X., Cai, H. X., Li, R., and Yang, Y. (2016). Clean distributed generation in China: Policy options and international experience. Renew. Sustain. Energy Rev. 57, 753–764. doi:10.1016/j.rser.2015.12.171
Fan, J. Y., Xie, H. P., Chen, J., Jiang, D. Y., Li, C. B., Ngaha, T. W., et al. (2020). Preliminary feasibility analysis of a hybrid pumped-hydro energy storage system using abandoned coal mine goafs. Appl. Energy 258, 114007. doi:10.1016/j.apenergy.2019.114007
Guo, Z., Ge, S. S., Yao, X. L., Li, H., and Li, X. Y. (2019). Life cycle sustainability assessment of pumped hydro energy storage. Int. J. Energy Res. 44 (2), 192–204. doi:10.1002/er.4890
He, L. M., Wu, L. X., Liu, S. J., Wang, Z., Su, C., and Liu, S. N. (2015). Mapping two-dimensional deformation field time-series of large slope by coupling DInSAR-SBAS with MAI-SBAS. Remote Sens. 7, 12440–12458. doi:10.3390/rs70912440
He, Y. S., and Kusiak, A. (2017). Performance assessment of wind turbines: Data-derived quantitative metrics. IEEE Trans. Sustain. Energy 9 (1), 65–73. doi:10.1109/tste.2017.2715061
Li, G. D., Li, G. Y., and Zhou, M. (2019). Model and application of renewable energy accommodation capacity calculation considering utilization level of inter-provincial tie-line. Prot. Control Mod. Power Syst. 4, 1–12. doi:10.1186/s41601-019-0115-7
Li, H. J., Deng, J. H., Yuan, S., Feng, P., and Arachchige, D. (2021). Monitoring and identifying wind turbine generator bearing faults using deep belief network and EWMA control charts. Front. Energy Res. 9, 799039. doi:10.3389/fenrg.2021.799039
Li, H. J., He, Y. S., Xu, Q., Deng, J. H., Li, W. L., and Wei, Y. (2022). Detection and segmentation of loess landslides via satellite images: A two-phase framework. Landslides 19 (3), 673–686. doi:10.1007/s10346-021-01789-0
Liu, F. Y., Yang, Z. Q., Deng, W. X., Yang, T. H., Zhou, J. R., Yu, Q. L., et al. (2021). Rock landslide early warning system combining slope stability analysis, two-stage monitoring, and case-based reasoning: A case study. Bull. Eng. Geol. Environ. 80 (11), 8433–8451. doi:10.1007/s10064-021-02461-6
Luo, P. J., Han, X. Q., and Chen, N. (2022). Study on complexity planning model of pumped storage in abandoned open-pit mine: A case study of Fushun open-pit mine. Front. Earth Sci. (Lausanne). 10. doi:10.3389/feart.2022.808371
Madlener, R., and Specht, J. M. (2020). An exploratory economic analysis of underground pumped-storage hydro power plants in abandoned deep coal mines. Energies 13 (21), 5634. doi:10.3390/en13215634
Maihemuti, B., Wang, E. Z., Hudan, T., and Xu, Q. J. (2016). Numerical simulation analysis of reservoir bank fractured rock-slope deformation and failure processes. Int. J. Geomech. 16 (2), 04015053. doi:10.1061/(asce)gm.1943-5622.0000533
Menéndez, J., and Loredo, J. (2019). Use of closured open pit and underground coal mines for energy generation: Application to the Asturias Central Coal Basin (Spain). E3S Web Conf. 80, 01005. doi:10.1051/e3sconf/20198001005
Pérez-Díaz, J. I., Chazarra, M., García-González, J., Cavazzini, G., and Stoppato, A. (2015). Trends and challenges in the operation of pumped-storage hydropower plants. Renew. Sustain. Energy Rev. 44, 767–784. doi:10.1016/j.rser.2015.01.029
Poulain, A., Pujades, E., and Goderniaux, P. (2021). Hydrodynamical and hydrochemical assessment of pumped-storage hydropower (PSH) using an open pit: The case of obourg chalk quarry in Belgium. Appl. Sci. (Basel). 11 (11), 4913. doi:10.3390/app11114913
Pujades, E., Orban, P., Bodeux, S., Archambeau, P., Erpicum, S., and Dassargues, A. (2017). Underground pumped storage hydropower plants using open pit mines: How do groundwater exchanges influence the efficiency? Appl. Energy 190, 135–146. doi:10.1016/j.apenergy.2016.12.093
Pujades, E., Willems, T., Bodeux, S., Orban, P., and Dassargues, A. (2016). Underground pumped storage hydroelectricity using abandoned works (deep mines or open pits) and the impact on groundwater flow. Hydrogeol. J. 24 (6), 1531–1546. doi:10.1007/s10040-016-1413-z
Rehman, S., Al-Hadhrami, L. M., and Akam, M. M. (2015). Pumped hydro energy storage system: A technological review. Renew. Sustain. Energy Rev. 44, 586–598. doi:10.1016/j.rser.2014.12.040
Song, J., Dong, M., Koltuk, S., Hu, H., Zhang, L. Q., and Azzam, R. (2017). Hydro-mechanically coupled finite-element analysis of the stability of a fractured-rock slope using the equivalent continuum approach: A case study of planned reservoir banks in blaubeuren, Germany. Hydrogeol. J. 26 (3), 803–817. doi:10.1007/s10040-017-1694-x
Uría-Martínez, R., Johnson, M. M., and Shan, R. (2021). U.S. Hydropower market report (January, 2021). Springfield: U.S. Department of Commerce.
Wang, E. Z., Zhong, J. W., Zhao, Y. T., and Mao, W. R. (2014). Analysis of seepage and seepage control measures in the rock masses of the Huilong pumped-storage power station. Bull. Eng. Geol. Environ. 74 (4), 1453–1462. doi:10.1007/s10064-014-0702-9
Wessel, M., Madlener, R., and Hilgers, C. (2015). Economic feasibility of semi-underground pumped storage hydro power plants in open pit mines. Energies 13 (16), 4178. doi:10.3390/en13164178
Xiong, X., Zhi, Z., Xiong, Y., Peng, M., and Zhang, F. (2019). Unsaturated slope stability around the Three Gorges Reservoir under various combinations of rainfall and water level fluctuation. Eng. Geol. 261, 105231. doi:10.1016/j.enggeo.2019.105231
Yang, T. H., Xie, L. K., Wang, S. Y., Zheng, H., Rui, Y. Q., and Tang, C. A. (2005). Effect evaluation of distortion for north project of side slope in Fushun west open cast. Chin. J. Rock Mech. Eng. 24 (11), 1841–1846. doi:10.3321/j.issn:1000-6915.2005.11.004
Yang, T. H., Xu, T., Liu, H. Y., Zhang, C. M., Wang, S. Y., Rui, Y. Q., et al. (2014). Rheological characteristics of weak rock mass and effects on the long-term stability of slopes. Rock Mech. Rock Eng. 47, 2253–2263. doi:10.1007/s00603-013-0527-z
Yuan, L., Jiang, Y. D., Wang, K., Zhao, Y. X., Hao, X. J., and Xu, C. (2018). Precision exploitation and utilization of closed/abandoned mine resources in China. J. China Coal Soc. 43 (1), 14–20. doi:10.13225/j.cnki.jccs.2017.1803
Yuan, L., and Yang, K. (2021). Further discussion on the scientific problems and countermeasures in the utilization of abandoned mines. J. China Coal Soc. 46 (1), 16–24. doi:10.13225/j.cnki.jccs.yg20.1966
Zhang, F., Yang, T. H., Li, L. C., Wang, Z., and Xiao, P. (2019). Cooperative monitoring and numerical investigation on the stability of the south slope of the Fushun west open-pit mine. Bull. Eng. Geol. Environ. 78, 2409–2429. doi:10.1007/s10064-018-1248-z
Zhang, M. S., Dong, Y., and Sun, P. P. (2012). Impact of reservoir impoundment-caused groundwater level changes on regional slope stability: A case study in the loess plateau of western China. Environ. Earth Sci. 66 (6), 1715–1725. doi:10.1007/s12665-012-1728-6
Zhang, S. F., Andrews-Speed, P., and Perera, P. (2015). The evolving policy regime for pumped storage hydroelectricity in China: A key support for low-carbon energy. Appl. Energy 150, 15–24. doi:10.1016/j.apenergy.2015.03.103
Keywords: abandoned open-pit coal mine, pumped storage hydropower (PSH), reservoir design, slope stability analysis, water level
Citation: Liu F, Yang K, Yang T, Gao Y, Li J, Liu Q and Fu Q (2022) Pumped storage hydropower in an abandoned open-pit coal mine: Slope stability analysis under different water levels. Front. Earth Sci. 10:941119. doi: 10.3389/feart.2022.941119
Received: 11 May 2022; Accepted: 15 August 2022;
Published: 12 September 2022.
Edited by:
Shenghua Cui, Chengdu University of Technology, ChinaReviewed by:
Sandeep Kumar Duran, Lovely Professional University, IndiaRitu Raj Kaur, Guru Nanak Dev University, India
Peng Tang, Jiangxi University of Science and Technology, China
Jaspreet Singh, I. K. Gujral Punjab Technical University, India
Copyright © 2022 Liu, Yang, Yang, Gao, Li, Liu and Fu. This is an open-access article distributed under the terms of the Creative Commons Attribution License (CC BY). The use, distribution or reproduction in other forums is permitted, provided the original author(s) and the copyright owner(s) are credited and that the original publication in this journal is cited, in accordance with accepted academic practice. No use, distribution or reproduction is permitted which does not comply with these terms.
*Correspondence: Ke Yang, a2V5YW5nQGF1c3QuZWR1LmNu