- 1State Key Laboratory of Isotope Geochemistry, Guangzhou Institute of Geochemistry, Chinese Academy of Sciences, Guangzhou, China
- 2CAS Center for Excellence in Deep Earth Science, Guangzhou, China
- 3Southern Marine Science and Engineering Guangdong Laboratory (Guangzhou), Guangzhou, China
- 4College of Earth and Planetary Sciences, University of Chinese Academy of Sciences, Beijing, China
Well-preserved paleo-shoreline systems suggest lake shrinkage occurred in a vast region of the Tibetan Plateau, indicating dramatic paleo-environmental changes that linked closely to variations in Asian monsoon precipitation, glacial meltwater, and evaporation. The elevation and chronology of these shoreline features can be used to reconstruct lake level fluctuation history and regional paleo-climate. Here, we report optically stimulated luminescence (OSL) dating of 14 sediment samples from three of seven paleo-shorelines at south of Dagze Co, central Tibetan Plateau, using coarse-grained quartz and K-feldspar. Two fine grain silt samples were also measured. The results suggest the following: 1) The highest paleo-shoreline for Dagze Co has an elevation around 4,525 m (+55 m above modern lake). Its age is estimated to be 9.1 ± 0.5 to 9.6 ± 0.6 ka, suggesting a relative humid condition in the early Holocene. 2) After that, the lake level dropped ∼20 m and remained relatively stable (+35 m) from ∼8 to 5 ka, implying a dry condition with reduced runoff. Lake level further declined for ∼40 m since about 5 ka. 3) The general trend of shrinkage for Dagze Co broadly matches studies from adjacent lakes, suggesting a common pattern of lake’s evolution on the south/central plateau in the late Quaternary. The long-term decreasing trend of Asian monsoon precipitation should be the main reason.
Introduction
The Tibetan Plateau (TP) has a profound impact on global atmospheric circulations and the Asian monsoon system (e.g., An, 2000; Chen et al., 2020). Known as “the water tower of Asia,” it is the origin of Asia’s many great rivers, supplying runoffs for over 1 billion people (Krause et al., 2010; Song et al., 2013; Jonell et al., 2020; Wei et al., 2020). With a total area of more than 40,000 km2, more than 1,000 lakes (>1 km2 area) are widely distributed across the plateau, comprising an important component of water resources in the TP (Ma et al., 2011; Zhang et al., 2013; Liu et al., 2018). The evolution of lakes is closely linked to variations in climatic controls. Many studies have been carried out using lake core sediment with proxy analysis to reconstruct paleo-environment change (e.g., Zhu et al., 2008; Wang et al., 2009; Hou et al., 2017). Lake level and water volume are among the most important features to study the evolution of lakes. Paleo-shorelines are geomorphic features formed in the interaction of lake water, aerodynamics, and land, representing a temporary stationary state of the lake level due to precipitation–evaporation balance (e.g., Otvos, 2000), i.e., paleo-shorelines can provide ideal records for studying lake level fluctuation history.
The presence of a large number of paleo-shorelines 10–200 m above the modern lake in TP indicates that lake shrinkage occurred in a vast region (Li et al., 2009; Hudson and Quade, 2013). The timing for lake highstands and their relationship with climatic controls have been discussed in several recent studies (Chen et al., 2013; Liu et al., 2013b; Lai et al., 2014; Yan and Wünnemann, 2014; Zhou et al., 2020; Hou et al., 2021; Feng et al., 2022). Some researchers have pointed out that the spatiotemporal complexity for the timing of the recent highest lake levels in TP mainly follows such a pattern: many lakes in northwestern TP reached their maximum extents during the latest Pleistocene (Kong et al., 2007; Liu et al., 2016; Zhang et al., 2022), while many lakes in the southern/southwestern TP reach their highest levels in the early Holocene (Chen et al., 2013; Rades et al., 2013; Ahlborn et al., 2015; Hudson et al., 2015; Huth et al., 2015; Rades et al., 2015). Lake highstands in the MIS 5 mainly occur for lakes in the northeastern TP (see reviews and references in Zhang et al., 2020), while mid-Holocene expansions were also reported (e.g., Liu et al., 2015). The controlling climatic mechanism behind this pattern needs further investigation. However, there is still a lack of research for a comprehensive understanding of the history of lake level changes in TP, especially for small/medium-sized lake basins.
Dagze Co is a small lake in a closed basin in central TP (Figure 1A). It is surrounded by a well-preserved paleo-shoreline system. In this work, a detailed field survey was conducted using a differential GPS and a drone to identify and measure the relative elevations of paleo-shorelines. These shorelines were optically dated using quartz/K-feldspar grains to establish a detailed chronology for lake level fluctuations.
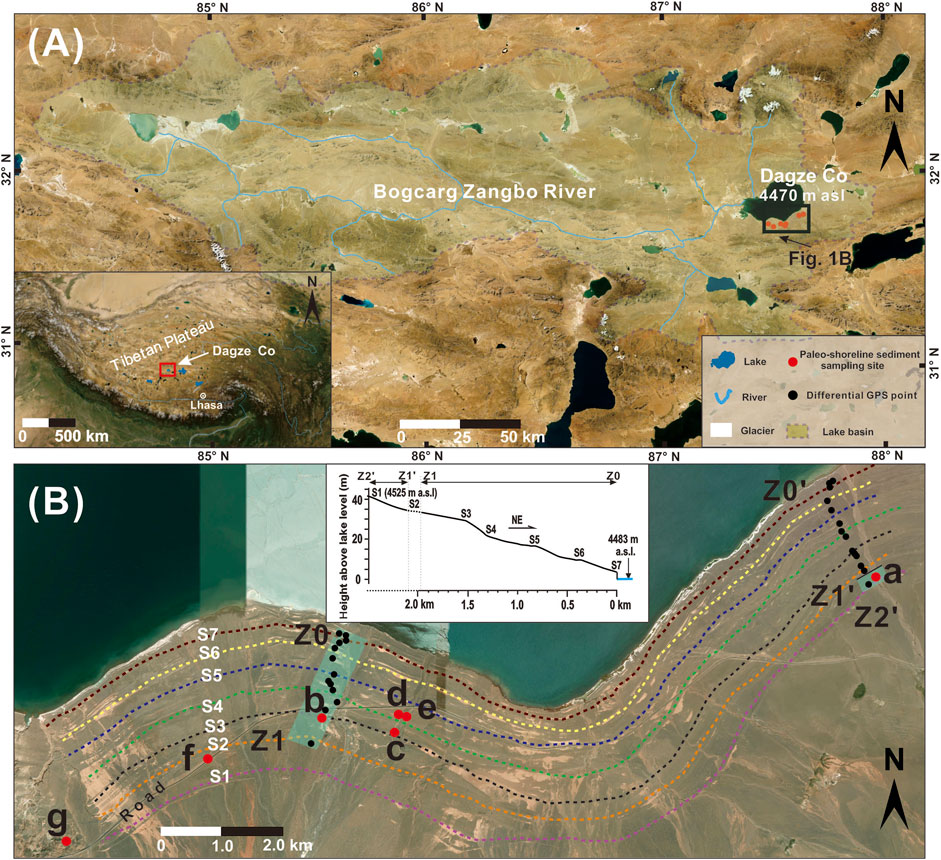
FIGURE 1. Study region of Dagze Co. (A) The catchment area and main rivers of Dagze Co; (B) satellite image of the paleo-shorelines of Dagze Co. Red full circles in (A,B) represent sample locations, and black spots represent sites for differential GPS measurement. Satellite images are from the Google Earth.
Sample Collection and Method
Study Area
Dagze Co (31.82°–31.98°N, 87.42°–87.65°E) is situated in a fractured basin in central TP. Well-preserved paleo-shorelines can be observed at the east and south sides of the lake (Figure 1A). The lake has a surface area of 245 km2 and a catchment area of 10,885 km2 (Wang and Dou, 1998; Hou et al., 2017). As a carbonate-type saltwater lake, Dagze Co has an annual evaporation of about 2,302 mm, which is much higher than the average annual precipitation of ∼ 316 mm (Table 1). Bogcarg Zangbo is the major infilling river.
Sampling and Altitude Measurement
According to satellite image and fieldwork, seven paleo-shorelines were identified, and they were named as S1–S7 (Figure 1B). We selected three shorelines to take samples at the south of the lake (S1, S3, and S4). This region mainly consists of an alluvial fan with a gentle slope, and the slope gradually flattened near the lakeshore. Seasonal rivers/gullies flow northward and cut through several paleo-shorelines, exposing sediment profiles. S1 seems the highest one (+55 m) with an apparent ridge feature (∼2 m in height), although there may be a higher one, but owing to a flat surface topography from thick alluvium aggradation, we were not able to locate it in the field. S3 is the most developed one and extends several kilometers (Figure 1B). One river cuts through S1, and a good profile was exposed. Under a coverage of alluvial gravels and surface soil (0.5 m), a thick and well-sorted silty–sandy layer was found (2 m). Beneath the sandy layer were massive poorly sorted pebbles and coarse sands, probably related to fluvial conditions in a high-energy sedimentary environment. Two samples were collected from the thick layer of silty sands (Figure 2A). Because these samples were taken from the location of S1, and they are typical near-shore sediment, we believe their optically stimulated luminescence (OSL) ages can be used to represent the age of S1. Likewise, four samples were taken from two rivers where they cut through S3 (Figures 2B, C). For S4, four samples were collected from one river at its both sides where it cuts through S4 (Figures 2D, E). In addition, two surface samples near the road between S1 and S2 (Figure 1B, Figure 2F) were collected, and two others were collected from a silt, loess-like profile (Figure 1B, Figure 2G). We think these samples should be later sediment after the formation of paleo-shorelines, so their ages may provide a conservative estimation. A stainless tube (5 cm in diameter and 25 cm in length) was inserted into the sand layer with the open side of the tube being sealed. After the tube was removed from sediment, the other end was also sealed from sunlight.
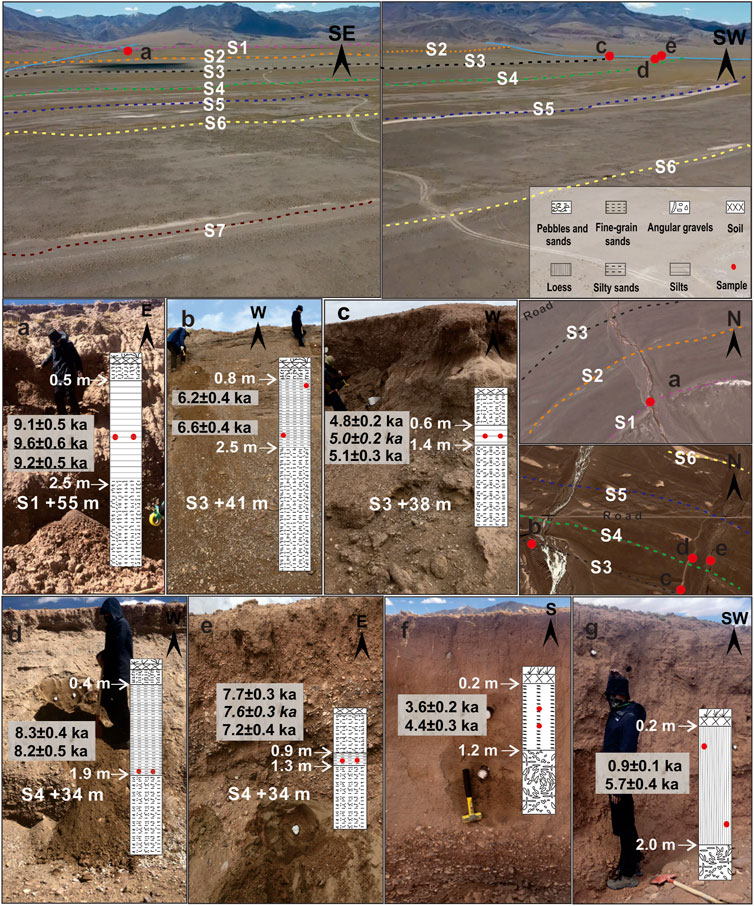
FIGURE 2. Field photos, sampling profiles, and OSL ages. The fieldwork was carried out in 2019 and 2021. Note that ages in italics refer to pIRIR 225°C results from K-feldspar, while underlined ages refer to fine-grained quartz (4–11 μm) results.
A differential GPS was used to determine the relative elevation of paleo-shorelines, with an error of centimeter scale. We set up the base station at the modern lake shore and took the portable station for shoreline elevation measurement (Z1–Z0 and Z2′–Z0′ sections in Figure 1B). In particular, we were not able to yield absolute elevations because of the lack of geodetic surveying dataset in this region. The relative height of shorelines is shown in Figure 1B, and the results suggest a total gap of 44.8 m above the modern lake level. The elevation of 4,470 m from Google Earth (GE) was used as the modern lake level, and further calculations (area and volume) were all based on this elevation. In particular, S1 in GE has an elevation of 4,525 m, i.e., 55 m above 4,470 m; on the contrary, our differential GPS suggests that S1 is 44.8 m above the modern lake level. Given that the differential GPS can yield accurate relative elevations (centimeter scale error), the possible reason should be that the lake level has raised ∼10 m, implying a recent lake expansion for Dagze Co (e.g., Zhang et al., 2019).
In summary, 10 samples from three shorelines were collected, while four other surface deposits were also collected.
Sample Preparation and Measurement
OSL dating was conducted in the Luminescence Dating Laboratory of Guangzhou Institute of Geochemistry, Chinese Academy of Sciences (GIG-CAS). Sediment at two ends of the tube was separated for uranium (U), U), thorium (Th), and potassium (K) concentration analysis. Under a subdued red-light condition, a series of physical/chemical treatments were applied to obtain coarse grains of quartz and K-feldspar (Aitken, 1998). Samples were treated with 10% hydrochloric acid (HCl) and 10% hydrogen peroxide (H2O2) to remove carbonates and organic materials, respectively. Then grains went through dry sieving to obtain the fraction between 90 and 125 μm, from which K-feldspar and quartz grains were separated successively by sodium polytungstate heavy liquid with densities of 2.58 and 2.75 g/cm3, respectively. The K-feldspar grains were etched with 10% hydrofluoric (HF) acid for more than 10 min, and the quartz grains were etched with 40% HF acid for more than 45 min to remove the outer part and possible feldspar contaminant. Moreover, 10% HCl and distilled water were used to wash quartz and K-feldspar grains, before being dried for De measurement. For samples of DZ-S1-1 and DZ-S1-2, the 4- to 11-μm polymineral grains were separated according to Stokes’ law. Then these grains were immersed in 30% hydrofluorosilicic (H2SiF6) for 3–4 days in an ultrasonic bath to extract the fine-grained quartz component.
Equivalent dose was determined using the SAR protocol (Murray and Wintle, 2000; Wintle and Murray, 2006). OSL and IRSL measurements were carried out using an automated Risø TL-DA-20 reader equipped with a90Sr/90Y beta source (0.10 Gy/s on aluminum discs). Quartz and K-feldspar signals were stimulated by blue LED (470 ± 30 nm) and infrared light (870 ± 40 nm) and detected through two 3-mm Hoya U-340 glass filter and a package of Schott BG-39 with Corning 7–59 filters, respectively. Small aliquots were used all through De measurement (Duller, 2008), and 20–40 discs were tested for each sample. To perform cross-checking from the multi-dating method (e.g., Long et al., 2015), two samples from S3 and S4 were also dated using coarse-grained K-feldspar, based on the protocol of pIRIR225 (Buylaert et al., 2009). For quartz, OSL stimulation was carried out for 40 s at 110°C, and the initial 0.4 s of signal was integrated for growth curve construction after subtracting the last 10-s signals. IRSL stimulation was carried out for 110 s at 50°C, while the second IRSL was measured at 225°C for 110 s.
Dose rate was determined based on the calculation of radiation from U, Th, K, and cosmic rays. K content was determined using an X-ray fluorescence spectrometer in GIG-CAS. The concentration of U and Th was measured by inductively coupled plasma–mass spectrometry (ICP-MS). Cosmic ray contributions were estimated for each sample as a function of burial depth, altitude, and geomagnetic latitude (Prescott and Hutton, 1994). Water content was estimated to be 5 ± 5% (e.g., Chen et al., 2016).
Luminescence Behaviors
The preheat plateau test was carried out on the sample of DZ-S4-3, with preheating temperature varying from 180 to 280°C with a 20°C increment (Figure 3D). Under each preheating temperatures, six aliquots were measured. A plateau was observed between 200 and 260°C (Figure 3D), and we selected a preheat condition of 240°C (10 s) for natural/regenerative dose measurement and a cut heat of 200°C for test dose measurement. Dose recovery tests were also performed on the same sample, and the results indicated that the ratio of measured to given dose was in the range of 1.0 ± 0.1 (Figure 3D). Aliquots with recycling ratios outside 1.0 ± 0.1 and recuperations higher than 5% were excluded from the De calculation. Quartz grains in this study mainly show good luminescence features such as bright signal, fast component dominance (Figures 3A, B), normal distribution, and concentrated single peak for De values (Figures 3E, F), indicating that these samples were well reset and the SAR protocol was reliable for our samples.
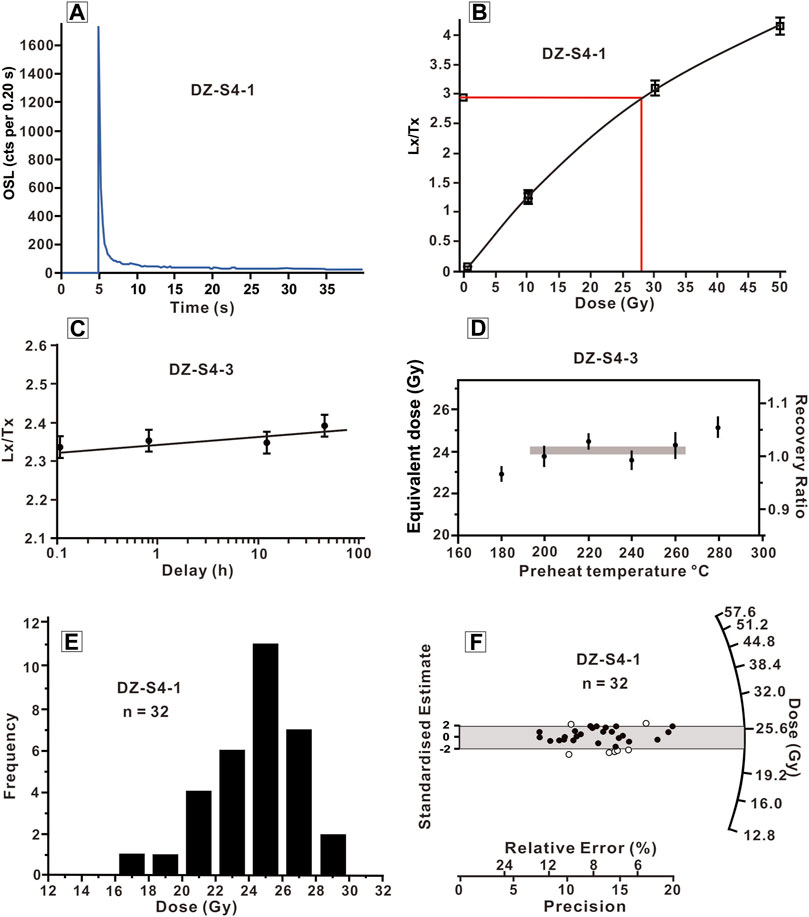
FIGURE 3. OSL decay curve and dose–response curve for the sample of DZ-S4-1 (A,B), fading test of quartz from sample DZ-S4-3 (C), preheat plateau tests for DZ-S4-3, (D) and De distributions in histograms and radial plots for sample DZ-S4-1 (E,F).
Some recent studies reported very weak natural quartz signals from Tibetan lakes or even anomalously fading (e.g., Tsukamoto and Rades, 2016; Hou et al., 2021). To compare with the quartz results, two samples from S3 and S4 were dated using the pIRIR225 procedure (Buylaert et al., 2009). Their K-feldspar results agree well with quartz ages (Table 2), suggesting that the OSL chronology for the samples in the study is reliable. The fading test was also conducted on the quartz grains of a sample DZ-S4-3, and the results suggest that no evident fading was observed (Figure 3C).
The results of OSL ages are summarized in Table 2.
Results and Discussion
OSL Chronology
Two samples were collected from the well-sorted silty–sandy layer of S1, DZ-S1-1, and S1-2. For sample S1-1, its coarse-grained quartz age (9.1 ± 0.5 ka) agrees well with its fine grain result (9.6 ± 0.6 ka). Since not enough coarse grains could be separated from the sample S1-2, we measured its fine-grained quartz, and an age of 9.2 ± 0.5 ka was obtained. These three results agree well with each other and suggest that S1 has an age of ∼9.3 ka, indicating that Dagze Co had a lake highstand of +55 m in the early Holocene. Climate records from proxy studies of Dagze Co also suggest a relatively moist climate with intense runoff during 19–12 ka (Hou et al., 2017). Massive pebbles beneath the sand layer in S1 also confirm a strong river runoff before 9.3 ka. The age gap between 9.3 to 12 ka might because of the following: 1) uncertainties arising from different dating method (e.g., reservoir effect of C-14) and, 2) after water infill reached its peak, the lake might have maintained at this level for a period and began to decline with a time lag. It is noted that a similar paleo-shoreline to S1 was dated as the middle Holocene (Liu et al., 2020; Liu et al., 2021). The difference may because our samples of S1 were taken from the 2-m-thick well-sorted sand layer, which was typical near-shore sediment. In particular, the overlying and underlying massive gravels/pebbles are more related to diluvial/fluvial conditions, and quartz/K-feldspar grains within these pebble layers are also harder to be fully bleached prior to burial.
Four samples from S3 have an age of 4.8 ± 0.2, 5.1 ± 0.3, 6.2 ± 0.4, and 6.6 ± 0.4 ka. These four samples were taken from two sites where two seasonal rivers cut through S3, and their elevations vary slightly from 4,511 to 4,508 m. One K-feldspar sample from DZ-S3-3 yields an age of 5.0 ± 0.2 ka, which is consistent with its quartz age of 4.8 ± 0.2 ka. The results of these four samples suggest a high lake level of +40 m in the middle Holocene. Compared with those of S1, the results clearly show that lake level rapidly dropped about 20 m since 9.2 to 5–6 ka. A proxy study from Dagze Co also suggests relatively high lake water salinity, reduced runoff, and a dry environment during the early to middle Holocene (Hou et al., 2017).
For S4, four samples yield ages of 7.2 ± 0.4, 7.7 ± 0.3, 8.2 ± 0.5, and 8.3 ± 0.4 ka. Combining with one K-feldspar result of 7.6 ± 0.3 ka of DZ-S4-3, these results agree well with each other and suggest that S4 has an age of 7.2–8.3 ka. Notably, this lake level is slightly higher than that of S3, suggesting a wetter condition. A slightly increased runoff was also found in proxy signals from Dagze Co, implying a relatively humid climate during the middle to late Holocene (Hou et al., 2017). In addition, our results also suggest that the lake level of Dagze Co probably remained stable for a considerable duration between 8 ka and about 5 ka, with some slight fluctuations.
The DZ-TR profile (Figure 2F) was collected from the road near surface between S1 and S2. Their ages are 3.6 ± 0.2 and 4.4 ± 0.3 ka. Since these sediments are deposits near the alluvium, their ages imply the aggradation of sediments atop of S2 was still active until about 4 ka. The DZ-TL profile was loess-like silt atop of S2, and two samples yield ages of 0.9 ± 0.1 and 5.7 ± 0.4 ka, from top down. In the field, this profile occurred as massive loess-like materials with occasional appearance of inter-bedded gravels. We tend to attribute it to a combination of aeolian activity with occasional alluviums.
Lake Level and Water Volume Reconstruction
Based on the elevation of S1, S3, and S4, the past lake area was reconstructed using DEM data in Figure 4 (source: Shuttle Radar Topography Mission data, ∼90-m resolution). There is a watershed with a height of 4,527 m to the west. If Dagze Co can reach that level, it might have connected to a dry basin to its west (the red areas in Figure 4). If so, at the highest lake level of 4,525 m (+55 m), the extent of Dagze Co was estimated as 1,639 km2, which was more than six times its present size.
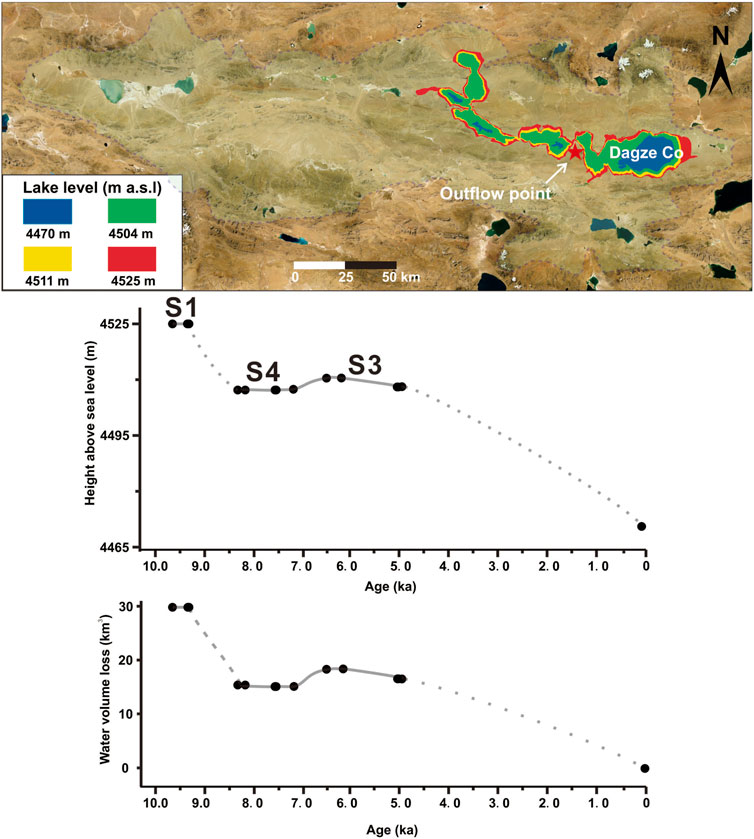
FIGURE 4. Reconstruction of coverage areas for paleo-Dagze Co, and lake level fluctuation history and water volume loss of Dagze Co since the early Holocene.
From S1 to about S4, the lake area has shrunk from 1,639 to 1,141 km2, and the volume of water decreased from 29.93 to 14.29 km3. The lake probably remained at a stable level of 4,504–4,511 m at 8–5 ka with slight fluctuations. A further decline of ∼ 40 m since about 5 ka was observed. In summary, the lake level of Dagze Co dropped by 55 m, with a total water loss of about 29.93 km3.
Comparison With Other Lakes and Climate Records
In Figure 5, the lake fluctuation history of Dagze Co was compared to those of several adjacent Tibetan lakes such as Selin Co (Shi et al., 2017; Hou et al., 2021), Nam Co (Zhou et al., 2020; Huang et al., 2022), Baqan Tso (Huth et al., 2015), Zhari Namco (Chen et al., 2013), and Ngangla Ring Tso (Hudson et al., 2015). In general, lakes from the southern TP have their highest levels mainly occurred in the early Holocene, which follows well with the enhanced Indian summer monsoon precipitation in the early Holocene (Figure 6, Berger and Loutre, 1991; Hong et al., 2003; Ji et al., 2005; Wang et al., 2005), while the highest levels of lakes from the northwestern TP mainly occur in the latest Pleistocene, probably related to the strong glacier meltwater by then (e.g., Liu et al., 2016; Zhang et al., 2022). From lake sediments and ice-core records on the plateau, an early Holocene wet/warm phase with substantial precipitation has been widely described (Thompson et al., 1989; Thompson et al., 1997; Wang et al., 2002). δ18O values from lacustrine carbonate in five lakes in western China show a rapid intensification of monsoon rainfall at about 12–11 ka (Wei and Gasse, 1999). A rainy episode at about 8 ka (Van Campo and Gasse, 1993) and a high lake level around 9 ka (Gasse et al., 1991) shown in the pollen record from Sumxi Co also suggest a long-term trend toward aridity since about 7 ka.
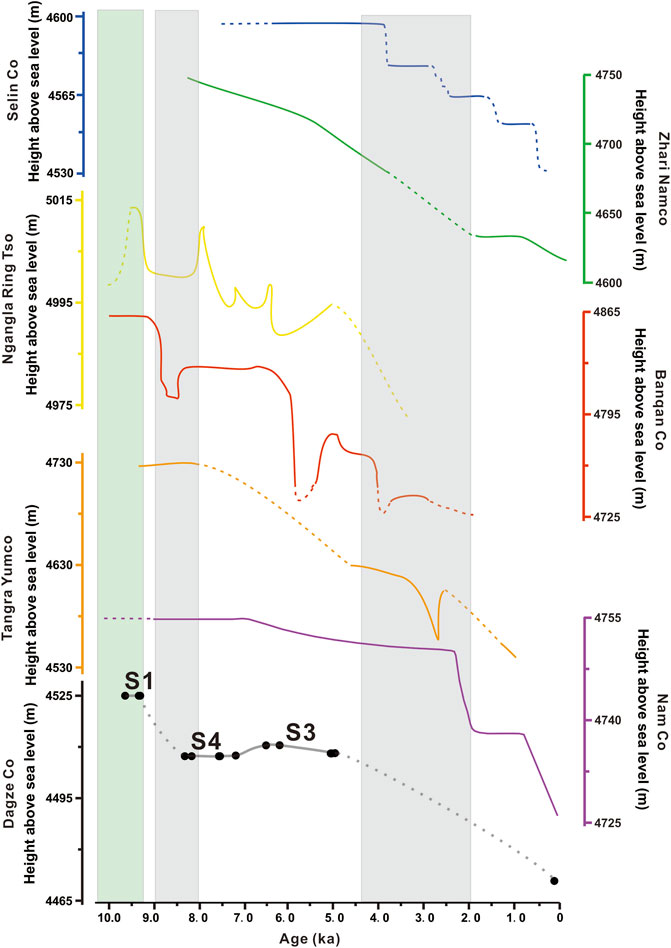
FIGURE 5. Comparison of lake level changes in Dagze Co with those of other lakes in southern plateau in the Holocene: Selin Co (Shi et al., 2017; Hou et al., 2021), Zhari Namco (Chen et al., 2013), Ngangla Ring Tso (Huth et al., 2015), Banqan Co (Hudson et al., 2015), Tangra Yumco (Ahlborn et al., 2015), and Nam Co (Zhou et al., 2020; Huang et al., 2022).
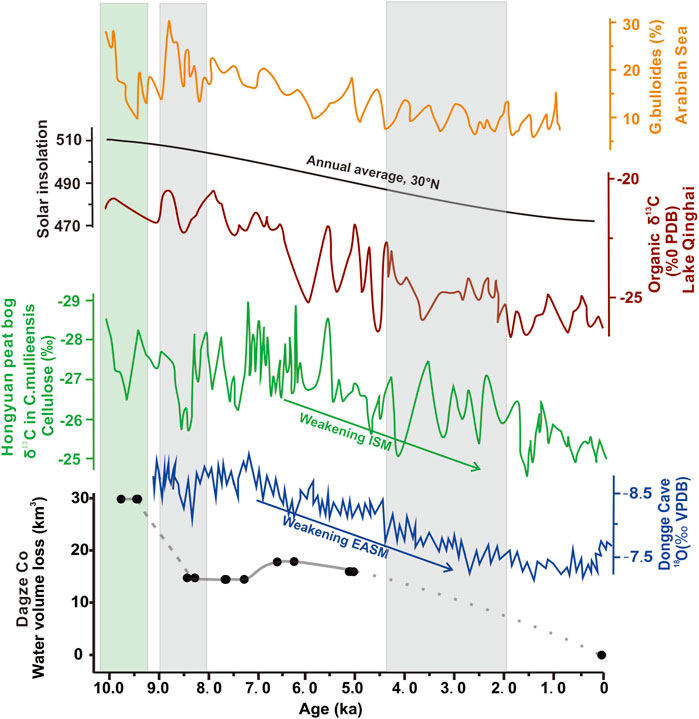
FIGURE 6. Changes in lake volume of Dagze Co since the early Holocene and other related climatic proxies: G. bulloides proxy record from Arabian Sea (Gupta et al., 2003), summer solar insolation at 30°N (Berger and Loutre, 1991), proxy records from Qinghai Lake (Ji et al., 2005), δ13C records from Hongyuan peat bog (Hong et al., 2003), and oxygen isotope records in the stalagmite from Dongge Cave (Wang et al., 2005).
Given the lack of evidence of glacial activity in Dagze Co, we attribute that the loss of water volume is mainly related to the net balance between Asian monsoon precipitation and evaporation. According to DEM analysis, Dagze Co has lost a total of 29.93 km3 of water and coincided with the general weakening trend of Asian monsoon since the early Holocene (Figure 6). Our findings from Dagze Co generally follow a similar pattern to those climatic records from Qinghai Lake (Ji et al., 2005), Dongge Cave (Wang et al., 2005), and the Arabian Sea (Gupta et al., 2003) (Figure 6). It is also noted that the strongest Indian monsoon precipitation occurred in the early Holocene, whereas the east Asian monsoon reached its peak in the early/middle Holocene; accordingly, lakes in southern TP have their highest levels occurred in the early Holocene, while lakes in northeastern TP reached their recent maximum in the middle Holocene (e.g., Chen et al., 2020; Zhang et al., 2020). Dagze Co lies within the region of Asian monsoon; its input of lake water should be under a joint control of ISM and EASM. This study shows that monsoonal precipitation has penetrated the basin of Dagze Co in the interior of the plateau during the early Holocene. Compared with records of the westerlies (see reviews and references in Chen et al., 2020), our result from Dagze Co implies that impact from westerlies in this region was not apparent during the early/middle Holocene. Notably, it is also possible that a lowered evaporation caused by low temperature could also lead to lake level rise, and such possibility should also be considered (e.g., Hou et al., 2021).
Conclusion
1) A lake highstand of Dagze Co (+55 m) occurred at 9.1 ± 0.5 to 9.6 ± 0.6 ka, suggesting a relatively humid period in the early Holocene. Intensified Asian monsoon precipitation in the early Holocene should be the main reason for the high lake level.
2) Lake level subsequently dropped about 20 m since 9.2 ka and probably remained at this level (+35 m) from about 8 to 5 ka, with slight fluctuations. A dry condition and reduced runoff should be the main reason for lake shrinkage. Lake level further dropped another 40 m in the late Holocene.
3) The shrinkage of Dagze Co generally follows a similar pattern to the evolution process of adjacent Tibetan lakes and the trend of Asian monsoon intensity, suggesting a long-term control of monsoon precipitation.
Data Availability Statement
The original contributions presented in the study are included in the article/Supplementary Material, and further inquiries can be directed to the corresponding author.
Author Contributions
YW: conducted the experiment and field trip, performed data analysis, and wrote the draft; YC: conducted the field trip, performed data analysis, and organized/revised the draft; LH: helped with the experiment and sample collection; PD: helped with sample collection and discussion; HC: helped with sample collection and discussion; and GW: organized the scientific framework.
Funding
This work was financially supported by the Second Tibetan Plateau Scientific Expedition and Research Program (STEP) (2019QZKK0202), the National Natural Science Foundation of China (41772176 and 41402150), the Key Special Project for Introduced Talents Team of Southern Marine Science and Engineering Guangdong Laboratory (Guangzhou) (GML2019ZD0308) and the Youth Innovation Promotion Association of the Chinese Academy of Sciences (2017403).
Conflict of Interest
The authors declare that the research was conducted in the absence of any commercial or financial relationships that could be construed as a potential conflict of interest.
Publisher’s Note
All claims expressed in this article are solely those of the authors and do not necessarily represent those of their affiliated organizations or those of the publisher, the editors, and the reviewers. Any product that may be evaluated in this article, or claim that may be made by its manufacturer, is not guaranteed or endorsed by the publisher.
Acknowledgments
Kai Kong and Xijie Wang are acknowledged for their assistance in the fieldwork.
References
Ahlborn, M., Haberzettl, T., Wang, J., Fürstenberg, S., Mäusbacher, R., Mazzocco, J., et al. (2015). Holocene Lake Level History of the Tangra Yumco Lake System, Southern-Central Tibetan Plateau. Holocene 26 (2), 176–187. doi:10.1177/0959683615596840
Aitken, M. J. (1998). Introduction to Optical Dating: The Dating of Quaternary Sediments by the Use of Photon-Stimulated Luminescence. Oxford: Oxford University Press.
An, Z. S. (2000). The History and Variability of the East Asian Paleomonsoon Climate. Quat. Sci. Rev. 19 (1-5), 171–187. doi:10.1016/S0277-3791(99)00060-8
Berger, A., and Loutre, M. F. (1991). Insolation Values for the Climate of the Last 10 Million Years. Quat. Sci. Rev. 10 (4), 297–317. doi:10.1016/0277-3791(91)90033-Q
Buylaert, J. P., Murray, A. S., Thomsen, K. J., and Jain, M. (2009). Testing the Potential of an Elevated Temperature IRSL Signal from K-Feldspar. Radiat. Meas. 44 (5), 560–565. doi:10.1016/j.radmeas.2009.02.007
Chen, F., Zhang, J., Liu, J., Cao, X., Hou, J., Zhu, L., et al. (2020). Climate Change, Vegetation History, and Landscape Responses on the Tibetan Plateau during the Holocene: A Comprehensive Review. Quat. Sci. Rev. 243, 106444. doi:10.1016/j.quascirev.2020.106444
Chen, Y., Aitchison, J. C., Zong, Y., and Li, S.-H. (2016). OSL Dating of Past Lake Levels for a Large Dammed Lake in Southern Tibet and Determination of Possible Controls on Lake Evolution. Earth Surf. Process. Landforms 41 (11), 1467–1476. doi:10.1002/esp.3907
Chen, Y., Zong, Y., Li, B., Li, S., and Aitchison, J. C. (2013). Shrinking Lakes in Tibet Linked to the Weakening Asian Monsoon in the Past 8.2 ka. Quat. Res. 80 (2), 189–198. doi:10.1016/j.yqres.2013.06.008
Duller, G. A. T. (2008). Single-Grain Optical Dating of Quaternary Sediments: Why Aliquot Size Matters in Luminescence Dating. Boreas 37 (4), 589–612. doi:10.1111/j.1502-3885.2008.00051.x
Feng, Y., Hou, Y., Zhang, J., Yang, N., Cai, Y., Yang, F., et al. (2022). Timing of Holocene Lake Highstands Around Dawa Co in Inner Tibetan Plateau: Comparison of Quartz and Feldspar Luminescence Dating with Radiocarbon Age. Quat. Geochronol. 69, 101267. doi:10.1016/j.quageo.2022.101267
Gasse, F., Arnold, M., Fontes, J. C., Fort, M., Gibert, E., Huc, A., et al. (1991). A 13,000-Year Climate Record from Western Tibet. Nature 353 (6346), 742–745. doi:10.1038/353742a0
Gupta, A. K., Anderson, D. M., and Overpeck, J. T. (2003). Abrupt Changes in the Asian Southwest Monsoon during the Holocene and Their Links to the North Atlantic Ocean. Nature 421 (6921), 354–357. doi:10.1038/nature01340
Hong, Y. T., Hong, B., Lin, Q. H., Zhu, Y. X., Shibata, Y., Hirota, M., et al. (2003). Correlation between Indian Ocean Summer Monsoon and North Atlantic Climate during the Holocene. Earth Planet. Sci. Lett. 211 (3), 371–380. doi:10.1016/S0012-821X(03)00207-3
Hou, J., Tian, Q., Liang, J., Wang, M., and He, Y. (2017). Climatic Implications of Hydrologic Changes in Two Lake Catchments on the Central Tibetan Plateau Since the Last Glacial. J. Paleolimnol. 58 (2), 257–273. doi:10.1007/s10933-017-9976-9
Hou, Y., Long, H., Shen, J., and Gao, L. (2021). Holocene Lake-Level Fluctuations of Selin Co on the Central Tibetan Plateau: Regulated by Monsoonal Precipitation or Meltwater? Quat. Sci. Rev. 261, 106919. doi:10.1016/j.quascirev.2021.106919
Huang, L., Chen, Y., Wu, Y., Zeng, T., and Wei, G. (2022). Lake Level Changes of Nam Co since 25 ka as Revealed by OSL Dating of Paleo-Shorelines. Quat. Geochronol. 70, 101274. doi:10.1016/j.quageo.2022.101274
Hudson, A. M., Quade, J., Huth, T. E., Lei, G., Cheng, H., Edwards, L. R., et al. (2015). Lake Level Reconstruction for 12.8-2.3 ka of the Ngangla Ring Tso Closed-Basin Lake System, Southwest Tibetan Plateau. Quat. Res. 83 (1), 66–79. doi:10.1016/j.yqres.2014.07.012
Hudson, A. M., and Quade, J. (2013). Long-Term East-West Asymmetry in Monsoon Rainfall on the Tibetan Plateau. Geology 41 (3), 351–354. doi:10.1130/G34548C.110.1130/g33837.1
Huth, T., Hudson, A. M., Quade, J., Guoliang, L., and Hucai, Z. (2015). Constraints on Paleoclimate from 11.5 to 5.0 ka from Shoreline Dating and Hydrologic Budget Modeling of Baqan Tso, Southwestern Tibetan Plateau. Quat. Res. 83 (1), 80–93. doi:10.1016/j.yqres.2014.07.011
Ji, S., Xingqi, L., Sumin, W., and Matsumoto, R. (2005). Palaeoclimatic Changes in the Qinghai Lake Area during the Last 18,000 Years. Quat. Int. 136 (1), 131–140. doi:10.1016/j.quaint.2004.11.014
Jonell, T. N., Aitchison, J. C., Li, G., Shulmeister, J., Zhou, R., and Zhang, H. (2020). Revisiting Growth and Decline of Late Quaternary Mega-Lakes across the South-Central Tibetan Plateau. Quat. Sci. Rev. 248 (15), 106475. doi:10.1016/j.quascirev.2020.106475
Kong, P., Na, C., Fink, D., Huang, F., and Ding, L. (2007). Cosmogenic 10Be Inferred Lake-Level Changes in Sumxi Co Basin, Western Tibet. J. Asian Earth Sci. 29 (5), 698–703. doi:10.1016/j.jseaes.2006.04.010
Krause, P., Biskop, S., Helmschrot, J., Flügel, W.-A., Kang, S., and Gao, T. (2010). Hydrological System Analysis and Modelling of the Nam Co Basin in Tibet. Adv. Geosci. 27, 29–36. doi:10.5194/adgeo-27-29-2010
Lai, Z., Mischke, S., and Madsen, D. (2014). Paleoenvironmental Implications of New OSL Dates on the Formation of the "Shell Bar" in the Qaidam Basin, Northeastern Qinghai-Tibetan Plateau. J. Paleolimnol. 51 (2), 197–210. doi:10.1007/s10933-013-9710-1
Li, D., Li, Y., Ma, B., Dong, G., Wang, L., and Zhao, J. (2009). Lake-Level Fluctuations since the Last Glaciation in Selin Co (Lake), Central Tibet, Investigated Using Optically Stimulated Luminescence Dating of Beach Ridges. Environ. Res. Lett. 4 (4), 045204. doi:10.1088/1748-9326/4/4/045204
Li, X., Hou, J., Wang, M., and Xu, L. (2021). Ecolosystem Changes and Possible Mechanisms of Dagze Co in the Tibetan Plateau During the Past 1000 Years. J. Lake Sci. 33 (4), 1276–1288. doi:10.18307/2021.0427
Liu, S., Jia, Q., Liu, X., Zheng, M., and Liu, L. (2013a). The Depositional Environment and Organic Sediment Component of Dagze Co, a Saline Lake in Tibet, China. Acta Ecol. Sin.(Engl. Ed.) 33 (18), 5785–5793.
Liu, W., Sun, F., Li, Y., Zhang, G., Sang, Y.-F., Lim, W. H., et al. (2018). Investigating Water Budget Dynamics in 18 River Basins across the Tibetan Plateau through Multiple Datasets. Hydrol. Earth Syst. Sci. 22 (1), 351–371. doi:10.5194/hess-22-351-2018
Liu, X.-J., Lai, Z.-P., Zeng, F.-M., Madsen, D. B., and E, C.-Y. (2013b). Holocene Lake Level Variations on the Qinghai-Tibetan Plateau. Int. J. Earth Sci. 102 (7), 2007–2016. doi:10.1007/s00531-013-0896-2
Liu, X.-J., Lai, Z., Madsen, D., and Zeng, F. (2015). Last Deglacial and Holocene Lake Level Variations of Qinghai Lake, North-Eastern Qinghai-Tibetan Plateau. J. Quat. Sci. 30 (3), 245–257. doi:10.1002/jqs.2777
Liu, X.-J., Madsen, D. B., Liu, R., Sun, Y., and Wang, Y. (2016). Holocene Lake Level Variations of Longmu Co, Western Qinghai-Tibetan Plateau. Environ. Earth Sci. 75 (4), 301. doi:10.1007/s12665-015-5188-7
Liu, X., Cong, L., Li, X., Madsen, D., Wang, Y., Liu, Y., et al. (2020). Climate Conditions on the Tibetan Plateau during the Last Glacial Maximum and Implications for the Survival of Paleolithic Foragers. Front. Earth Sci. 8, 549. doi:10.3389/feart.2020.606051
Liu, X., Madsen, D., and Zhang, X. (2021). The Driving Forces Underlying Spatiotemporal Lake Extent Changes in the Inner Tibetan Plateau during the Holocene. Front. Earth Sci. 9, 1–17. doi:10.3389/feart.2021.685928
Long, H., Shen, J., Wang, Y., Gao, L., and Frechen, M. (2015). High-Resolution OSL Dating of a Late Quaternary Sequence from Xingkai Lake (NE Asia): Chronological Challenge of the "MIS 3a Mega-Paleolake" Hypothesis in China. Earth Planet. Sci. Lett. 428, 281–292. doi:10.1016/j.epsl.2015.07.003
Ma, R., Yang, G., Duan, H., Jiang, J., Wang, S., Feng, X., et al. (2011). China's Lakes at Present: Number, Area and Spatial Distribution. Sci. China Earth Sci. 54 (2), 283–289. doi:10.1007/s11430-010-4052-6
Murray, A. S., and Wintle, A. G. (2000). Luminescence Dating of Quartz Using an Improved Single-Aliquot Regenerative-Dose Protocol. Radiat. Meas. 32 (1), 57–73. doi:10.1016/S1350-4487(99)00253-X
Otvos, E. G. (2000). Beach Ridges — Definitions and Significance. Geomorphology 32 (1), 83–108. doi:10.1016/S0169-555X(99)00075-6
Prescott, J. R., and Hutton, J. T. (1994). Cosmic Ray Contributions to Dose Rates for Luminescence and ESR Dating: Large Depths and Long-Term Time Variations. Radiat. Meas. 23 (2), 497–500. doi:10.1016/1350-4487(94)90086-8
Rades, E. F., Hetzel, R., Xu, Q., and Ding, L. (2013). Constraining Holocene Lake-Level Highstands on the Tibetan Plateau by 10Be Exposure Dating: A Case Study at Tangra Yumco, Southern Tibet. Quat. Sci. Rev. 82, 68–77. doi:10.1016/j.quascirev.2013.09.016
Rades, E. F., Tsukamoto, S., Frechen, M., Xu, Q., and Ding, L. (2015). A Lake-Level Chronology Based on Feldspar Luminescence Dating of Beach Ridges at Tangra Yum Co (Southern Tibet). Quat. Res. 83 (3), 469–478. doi:10.1016/j.yqres.2015.03.002
Shi, X., Kirby, E., Furlong, K. P., Meng, K., Robinson, R., Lu, H., et al. (2017). Rapid and Punctuated Late Holocene Recession of Siling Co, Central Tibet. Quat. Sci. Rev. 172, 15–31. doi:10.1016/j.quascirev.2017.07.017
Song, C., Huang, B., and Ke, L. (2013). Modeling and Analysis of Lake Water Storage Changes on the Tibetan Plateau Using Multi-Mission Satellite Data. Remote. Sens. Environ. 135, 25–35. doi:10.1016/j.rse.2013.03.013
Thompson, L. G., Mosley-Thompson, E., Davis, M. E., Bolzan, J. F., Dai, J., Klein, L., et al. (1989). Holocene-Late Pleistocene Climatic Ice Core Records from Qinghai-Tibetan Plateau. Science 246 (4929), 474–477. doi:10.1126/science.246.4929.474
Thompson, L. G., Yao, T., Davis, M. E., Henderson, K. A., Mosley-Thompson, E., Lin, P.-N., et al. (1997). Tropical Climate Instability: The Last Glacial Cycle from a Qinghai-Tibetan Ice Core. Science 276 (5320), 1821–1825. doi:10.1126/science.276.5320.1821
Tsukamoto, S., and Rades, E. F. (2016). Performance of Pulsed OSL Stimulation for Minimising the Feldspar Signal Contamination in Quartz Samples. Radiat. Meas. 84, 26–33. doi:10.1016/j.radmeas.2015.11.007
Van Campo, E., and Gasse, F. (1993). Pollen- and Diatom-Inferred Climatic and Hydrological Changes in Sumxi Co Basin (Western Tibet) since 13,000 Yr B.P. Quat. Res. 39 (3), 300–313. doi:10.1006/qres.1993.1037
Wang, J., Zhu, L., Nishimura, M., Nakamura, T., Ju, J., Xie, M., et al. (2009). Spatial Variability and Correlation of Environmental Proxies during the Past 18,000 Years Among Multiple Cores from Lake Pumoyum Co, Tibet, China. J. Paleolimnol. 42 (3), 303–315. doi:10.1007/s10933-008-9277-4
Wang, N., Yao, T., Thompson, L. G., Henderson, K. A., and Davis, M. E. (2002). Evidence for Cold Events in the Early Holocene from the Guliya Ice Core, Tibetan Plateau, China. Sci. Bull. 47 (17), 1422–1427. doi:10.1360/02tb9313
Wang, Y., Cheng, H., Edwards, R. L., He, Y., Kong, X., An, Z., et al. (2005). The Holocene Asian Monsoon: Links to Solar Changes and North Atlantic Climate. Science 308 (5723), 854–857. doi:10.1126/science.1106296
Wei, K., and Gasse, F. (1999). Oxygen Isotopes in Lacustrine Carbonates of West China Revisited: Implications for Post Glacial Changes in Summer Monsoon Circulation. Quat. Sci. Rev. 18 (12), 1315–1334. doi:10.1016/S0277-3791(98)00115-2
Wei, K., Ouyang, C., Duan, H., Li, Y., Chen, M., Ma, J., et al. (2020). Reflections on the Catastrophic 2020 Yangtze River Basin Flooding in Southern China. Innovation 1 (2), 100038. doi:10.1016/j.xinn.2020.100038
Wintle, A. G., and Murray, A. S. (2006). A Review of Quartz Optically Stimulated Luminescence Characteristics and Their Relevance in Single-Aliquot Regeneration Dating Protocols. Radiat. Meas. 41 (4), 369–391. doi:10.1016/j.radmeas.2005.11.001
Yan, D., and Wünnemann, B. (2014). Late Quaternary Water Depth Changes in Hala Lake, Northeastern Tibetan Plateau, Derived from Ostracod Assemblages and Sediment Properties in Multiple Sediment Records. Quat. Sci. Rev. 95, 95–114. doi:10.1016/j.quascirev.2014.04.030
Zhang, G., Yao, T., Chen, W., Zheng, G., Shum, C. K., Yang, K., et al. (2019). Regional Differences of Lake Evolution across China during 1960s-2015 and its Natural and Anthropogenic Causes. Remote Sens. Environ. 221, 386–404. doi:10.1016/j.rse.2018.11.038
Zhang, L., Su, F., Yang, D., Hao, Z., and Tong, K. (2013). Discharge Regime and Simulation for the Upstream of Major Rivers over Tibetan Plateau. J. Geophys. Res. Atmos. 118 (15), 8500–8518. doi:10.1002/jgrd.50665
Zhang, S., Zhang, J., Zhao, H., Liu, X., and Chen, F. (2020). Spatiotemporal Complexity of the "Greatest Lake Period" in the Tibetan Plateau. Sci. Bull. 65 (16), 1317–1319. doi:10.1016/j.scib.2020.05.004
Zhang, S., Zhao, H., Sheng, Y., Zhang, J., Zhang, J., Sun, A., et al. (2022). Mega-Lakes in the Northwestern Tibetan Plateau Formed by Melting Glaciers during the Last Deglacial. Quat. Sci. Rev. 285, 107528. doi:10.1016/j.quascirev.2022.107528
Zhou, J., Zhou, W., Dong, G., Hou, Y., Xian, F., Zhang, L., et al. (2020). Cosmogenic 10Be and 26Al Exposure Dating of Nam Co Lake Terraces since MIS 5, Southern Tibetan Plateau. Quat. Sci. Rev. 231, 106175. doi:10.1016/j.quascirev.2020.106175
Keywords: paleo-shoreline, OSL dating, Dagze Co, lake level change, Tibetan Plateau
Citation: Wu Y, Chen Y, Huang L, Ding P, Cui H and Wei G (2022) OSL Dating of Paleo-Shorelines at Dagze Co, Central Tibetan Plateau. Front. Earth Sci. 10:940013. doi: 10.3389/feart.2022.940013
Received: 09 May 2022; Accepted: 01 June 2022;
Published: 11 July 2022.
Edited by:
Bo Li, University of Wollongong, AustraliaReviewed by:
Xiangjun Liu, Northwest Normal University, ChinaJifeng Zhang, Institute of Tibetan Plateau Research (CAS), China
Copyright © 2022 Wu, Chen, Huang, Ding, Cui and Wei. This is an open-access article distributed under the terms of the Creative Commons Attribution License (CC BY). The use, distribution or reproduction in other forums is permitted, provided the original author(s) and the copyright owner(s) are credited and that the original publication in this journal is cited, in accordance with accepted academic practice. No use, distribution or reproduction is permitted which does not comply with these terms.
*Correspondence: Yiwei Chen, Y2hlbnl3QGdpZy5hYy5jbg==