- 1Beijing Earthquake Agency, Beijing, China
- 2Beijing Institute of Geo-Engineering, Beijing, China
- 3Northwest Institute of Eco-Environment and Resources Chinese Academy of Sciences, Lanzhou, China
The Beijing and Zhangjiakou-Bohai Fault Zone is a group of NW-W orderly active fault zones with high seismic activity and abundant geothermal resources since the Cenozoic. Many violent earthquakes occurred here, where it was an important area for earthquake monitoring and research. In order to explore the temporal and spatial variation characteristics of gas geochemistry in the Zhangjiakou-Bohai Fault Zone of the capital circle, this study cited the previous two-stage survey data of 23 geothermal hot springs in the west and east of the Zhangjiakou-Bohai Fault Zone of the capital circle in 2013 and 2018. In order to fill the gap in hot spring gas geochemistry in Beijing (the middle of the Zhangjiakou-Bohai Fault Zone), 21 emergent gas samples from hot springs were collected after many field surveys from October 2020 to November 2021. The test results of 44 gas samples with chemical compositions and isotope changes of helium, neon, and carbon showed that: (1) The helium isotope ratio (3He/4He (Rc/Ra)) of hot spring gases in the Zhangjiakou-Bohai Fault Zone ranged between 0.03 and 2.86Ra (Ra = air, 3He/4He = 1.39 × 10−6), and the calculated maximum proportion of mantle-derived helium was up to 35.4%. It was revealed that although the geological fluid in the fault zone mainly came from crustal source, the mantle-derived helium was still considerable. The hot spring gases in Beijing (the middle of the Zhangjiakou-Bohai Fault Zone) were mainly composed of nitrogen, whose concentration was more than 69%, featuring a low CO2 concentration of 0–6.1% and a δ13CCO2 value ranging from −19‰ to −9.6‰ (vs.PDB) and showing the mixing characteristics of organic sediments and mantle sources. (2) The upwelling release of mantle-derived materials in Zhangjiakou-Bohai Fault Zone shared a good corresponding relationship with regional seismicity, which could promote the inoculation and occurrence of regional earthquakes. In the peak area, the transition zone from the western mountainous area of the Zhangjiakou-Bohai Fault Zone to the plain showed that more mantle-derived materials upwelled, and more deep fluid upwelled. The comparative analysis of regional seismicity showed that deep fluid played an important role in controlling regional seismicity in the area with relatively strong upwelling of deep fluid in the Zhangjiakou-Bohai Fault Zone.
1 Introduction
The subduction of the ancient Pacific Plate is the geodynamic mechanism which leads to the thinning and destruction of the lithospheric mantle in the North China Craton. The destruction area of the North China Craton mainly occurs in the area to the east of Taihang Mountain in North China, including the integral destruction of lithospheric mantle and the strong transformation and thinning of crust as well as the obvious changes in physicochemical properties of the lithospheric mantle (Zhu et al., 2011; Feng et al., 2020).
The Zhangjiakou-Bohai Fault Zone is located at the junction of three tectonic units: the North China Basin, Yanshan Uplift, and Taihang Mountain Uplift (Xu et al., 1998), where faults are intersected and cut to form a complex seismogenic structural pattern. The Zhangjiakou-Bohai Tectonic Zone starts from the northern margin of Taihang Mountain in the west, is distributed along the junction area of Yanshan Mountain and the North China Basin, and enters the Bohai Sea in the east. It is an important NW seismic activity zone in Eastern China. In this zone, 26 earthquakes above M6 occurred (Zhao et al., 2011), and it is an active zone of typical structural faults and earthquakes.
According to the research result of the relationship between the temporal and spatial changes of helium isotope and carbon isotope in hot spring gases and fault activity in active areas such as San Andreas fault and related structures in the United States (Kulongoski et al., 2013), North Anatolia fault in Turkey (Italiano et al., 2009) and a large number of volcanic fault areas in Japan (Umeda and Ninomiya, 2009), strong fault activity areas were good channels for deep material migration. In the area with a high helium isotope ratio of hot spring gases in an active fault zone, there was usually an obvious high conductivity and low velocity area in the deep part. According to the investigation result of the temporal and spatial variations of helium isotope and carbon isotope in hot spring gases in an active fault zone, the temporal and spatial variation characteristics of mantle-derived helium and carbon dioxide in different proportions could be obtained quantitatively (Zhou et al., 2020; Zhou et al., 2021), and then the relationship between the activity of the active fault zone and mantle-derived helium and carbon dioxide in hot spring gases was further studied.
The Zhangjiakou-Bohai Fault Zone is also rich in geothermal water resources. Due to the combination of its regional geological conditions and geothermal background, it is very favorable for the storage and formation of geothermal water, and it is also one of the areas with the richest low-temperature hot water resources in China (Chen, 1988; Pang et al., 2014). Zhangjiakou-Bohai Fault Zone covers a large area, and there is no systematic and comprehensive studies and investigations on geothermal water in the whole area. Previous scholars did some intermittent research on geothermal hydrology and helium isotope distribution characteristics in the western basin-ridge structure area and the eastern basin of the Zhangjiakou-Bohai Fault Zone. Lu (2016) However, the lack of research on Beijing, which is located in the middle of the Zhangjiakou-Bohai Fault Zone, leads to the incomplete exploration of the whole Zhangjiakou-Bohai Fault Zone. In order to more comprehensively and systematically discuss whether the deep fluid upwelling and its possible temporal and spatial changes also exist in the Zhangjiakou-Bohai Fault Zone, a systematic geochemical study on hot spring gases has been conducted in the central Beijing in recent years, and basic data have been provided for future geochemical seismic monitoring of hot spring gas, mineral resources evaluation and environmental research in Beijing and the Zhangjiakou-Bohai Fault Zone.
Two groups of conjugate movement faults (NE-NEE and NW-NWW) are mainly distributed in the Zhangjiakou-Bohai Fault Zone, which together form the NW-W Zhangjiakou-Bohai Fault Zone (Chen et al., 2016) and have a certain active period (Ma et al., 2004). Strong earthquakes occurred in these fault zones where they were the areas with the most frequent strong earthquakes. The formation of the present geological structure and geomorphic pattern in the Zhangjiakou-Bohai Fault Zone originated from tectonic activity since the Archean. The Mesozoic Yanshan movement not only caused the previous strata to fold and fracture with magmatic activity but also developed a series of NE, NWW, and near EW faults (Chen et al., 2016). As the North China fault block began to disintegrate, Taihang Mountain, Yanshan Mountain, and Luxi Mountain rose, and their interior settled. This helped cultivate the early form of the structure of the North China Basin. In the Paleogene period, large-scale uneven fault depression activities occurred in the North China Basin, forming a series of staggered patterns of fault depression uplifts and depressions. The thick Cenozoic deposits in the North China Rift Basin were in contrast with the ancient bedrock outcrops in Yanshan Mountain in the north and Taihang Mountain in the southwest. The piedmont fault difference could reach several kilometers. Even in the rift basin, there were many kinds of depressions, uplifts, and secondary uplift structural units, which constituted the complex structure of the upper crust of the Zhangjiakou-Bohai Fault Zone (Zhang et al., 2007).
Since the 1970s, the accuracy of earthquake location has become higher and higher. It was found that the earthquake distribution in the capital circle crossed the Yanshan Mountains, forming a very important seismic zone in the north of the North China-Zhangjiakou-Bohai Seismic Zone. Moreover, there was seismic activity in the vicinity of NE faults such as Hexiwu Fault, Liulingshan Northern Fault, Weiguang Basin Southern Margin Fault, Huangzhuang-Gaoliying Fault, and Xiadian New Fault, which formed a network distribution with the NWW Zhangjiakou-Bohai Seismic Zone (Ma et al., 2004).
Under the action of a single NEE principal compressive stress field, NWW tandem blocks moved easily, forming a NWW seismic activity intensive zone on the block boundary, while the conjugate NE or NEE faults had the structural conditions for earthquakes of moderate or strong magnitude or above. The Zhangjiakou-Bohai Fault Zone has become a structural zone closely related to earthquakes (Chen et al., 2016).
2 Measurement and experimental methods
From October 2020 to November 2021, after investigations on geothermal wells in Beijing, 21 hot spring gas samples from geothermal well were collected along the north margin of Yanji Basin, Huangzhuang-Gaoliying, Xiadian, and Nankou-Sunhe, NW faults in the Zhangjiakou-Bohai Fault Zone and related areas (Figure 1 and Supplementary Table S1).
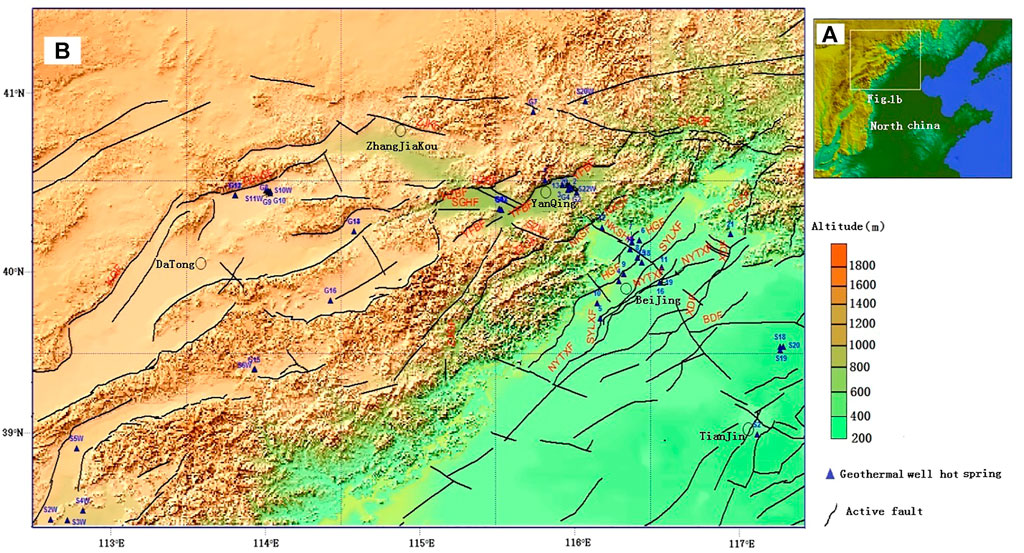
FIGURE 1. Distribution of gas sampling sites of hot springs in geothermal wells of the Zhangjiakou-Bohai Fault Zone. (A) Topographic map of North China. (B) Topographic map of the Zhangjiakou-Bohai Fault Zone and location map of sampling sites, active faults according to Xu et al. (2016).
In addition, using the previous survey work, from September 2011 to November 2012, the China Earthquake Administration predicted that it had conducted three field geological surveys in the western part of the Zhangjiakou-Bohai Fault Zone in the capital circle, investigated hot spring sites and took 18 gas samples; From July to August 2016, 24 samples of hot springs and geothermal water gas were taken from the east and west of the Zhangjiakou-Bohai Fault Zone in the capital circle (Figure 1; Supplementary Table S1).
The hot spring gas sampling container was a 500 ml glass bottle, and it was collected in the field by the drainage and gas taking method. The components of N2, H2, CO2, O2 and CH4 in hot spring samples were measured by the Agilent Macro 490 portable gas chromatograph laboratory, and the measurement accuracy was estimated as follows: the relative standard deviation was <0.5% when the content was 1% ∼ 100%, the relative standard deviation was <1% when the content was 0.01% ∼ 1%, and the relative standard deviation was <0.001% ∼ 0.01% (Zhou et al., 2015).
The He, Ne, and C isotopes in hot spring gas samples were analyzed by the Northwest Institute of Ecological Environment Resources, Chinese Academy of Sciences. The concentrations and isotopes of He and Ne in hot spring gases were analyzed by a Noblesse noble gas isotope mass spectrometer. When the R value in helium isotope measurement was above 1 × 10−7, the test error was ±10%, and the measurement data error at 1 × 10−8∼1 × 10−7 were ±15% (Cao et al., 2018). The carbon isotope ratio was analyzed by the Delta Plus XL mass spectrometer, which was manufactured by Thermo Finnigan, USA and consisted of HP6890 gas chromatography, combustion/conversion furnace, interface and the DeltaPlusXP mass spectrometer. Stable carbon isotope composition was expressed by δ13C; the accuracy of 13C/12C was 0.6‰ (Li et al., 2014).
3 Measurement results
He in mantle source, crust source and air had their own characteristic 3He/4He ratios, which were (1.1 × 10−5∼1.4 × 10−5, 2 × 10−8 and 1.39 × 10−6 (Mamyrin et al., 1970; Ozima and Podosek, 1983). After atmospheric correction (Rc/Ra) of the 3He/4He value, the percentage of He in the mantle source could be calculated (Sano and Wakita, 1985).
The average proportion of helium from geothermal hot springs in Beijing’s main fault zone in the Zhangjiakou-Bohai Fault Zone in this survey ranges from 0.03% to 35.4% (Supplementary Table S2).
Geothermal hot springs can be divided into two types according to the main components of the gases. In the first type, CO2 concentration is the main component, and the average CO2 concentration is more than 50%. In the second type, N2 is the main component of hot springs. It can be seen from the concentration data of geothermal hot springs surveyed (Supplementary Table S2) that the main components of the gases are N2, which is a main component of hot springs. There were a total of 21 hot springs, and the average concentration of N2 in hot springs ranged from 63.89% to 94.74%. The average concentration range of He in all hot spring gases was 48 × 10−6∼1502 × 10−6, that of H2 was 265.1 × 10−6, and that of CH4 was 0.070%∼38.41%. The average distribution range of 3He/4He (Rc/Ra) was 0.24∼2.86, while that of δ13CCO2 (PDB) was -23.6‰∼−2.9‰, and that of δ13CCH4 (PDB) was -63.7‰ ∼−14.1‰ (Supplementary Table S2).
Based on the previous measurement results of geothermal hot spring gases in the Zhangjiakou-Bohai Fault Zone, the average ratio of helium in mantle source of geothermal hot spring gases with helium isotope measured in 44 areas ranged from 0.03% to 35.4%, and the helium in hot spring gases mainly came from crust sources (Supplementary Table S2). However, 40 of them had obvious mantle-derived helium (>2%) (Figure 2).
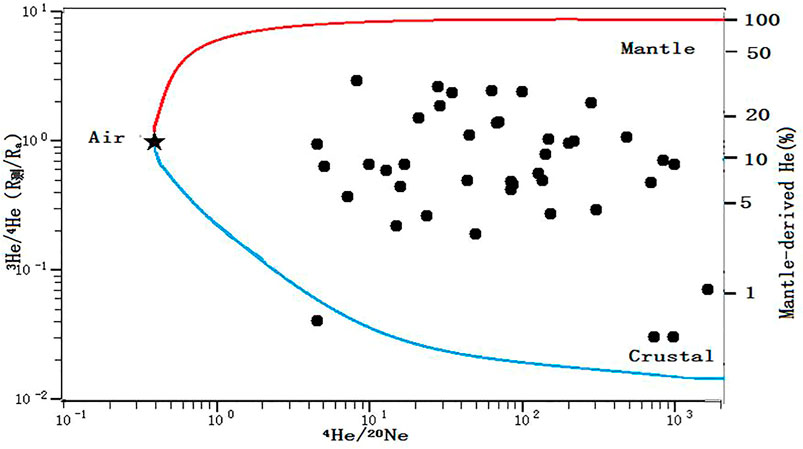
FIGURE 2. Plot of 3He/4He vs. 4He/20Ne average ratios in 44 samples of hot spring gas. Mixing lines between the atmosphere and upper mantle and the atmosphere and crust were calculated using the end members: air (3He/4He = 1.4 × 10–6, 4He/20Ne4 = 0.318), upper mantle (3He/4He = 12 × 10–6, 4He/20Ne = 100, 000), old continental crust (3He/4He = 0.02 × 10–6, 4He/20Ne = 100,000) (after Ozima and Podosek, 1983; Ballentine et al., 2005).
4 Discussion and conclusion
4.1 Source of hot spring gases
4.1.1 He
The 3He/4He values of hot spring gas samples from Beijing and the Zhangjiakou-Bohai Fault Zone were less than 3.0Ra, showing the characteristics of typical mixed source helium. The 3He/4He ratios in mantle source, crust source and air were (1.1 × 10−5, 2 × 10−8 and 1.39 × 10−6 (Mamyrin et al., 1970; Ozima and Podosek, 1983; Wei et al., 2015; Mar Alonso, et al., 2021; Domokos, et al., 2021). After atmospheric correction (Rc/Ra) of the 3He/4He value, the percentage of He in the mantle source could be calculated (Sano and Wakita, 1985). The average ratio of mantle-derived helium in hot springs in Beijing and the Zhangjiakou-Bohai Fault Zone ranged from 0.03% to 35.40%. Therefore, helium in hot springs was mainly from the crust source (64.6%–99.67%) (Figure 2 and Supplementary Table S2). 40 of all 44 geothermal hot springs had obvious mantle-derived helium (>2%) (Supplementary Table S2).
It was easy to observe a large amount of mantle-derived helium in volcanic areas such as Tengchong Volcano (Zhao et al., 2012) and Wudalianchi Volcano (Xu et al., 2013). However, there were no Cenozoic active volcanoes in Beijing, so it was impossible for mantle-derived helium to be released in volcanic areas. Mantle-derived helium was diluted by helium from the crust during its upward migration along the deep fault, which was also observed in the southwest fault zone of China (Zhou et al., 2015; Zhou et al., 2020). In addition, the mantle-derived helium in the Zhangjiakou-Bohai Fault Zone could not be 3He produced from tritium decay during nuclear bomb explosions (Yokoyama et al., 1999).
In general, Zhangjiakou-Bohai Fault Zone geothermal hot springs had obvious mantle-derived helium (>2%); this is related to the destruction of the North China Craton The subduction of the Pacific Plate led to the destruction of the North China Craton and the up welling of mantle magma, enhanced fluid metasomatism, melting and magmatism (Zhu et al., 2011), and the Zhangjiakou-Bohai Fault Zone provided a good channel for upward migration of mantle-derived helium.
The relatively high helium isotope 3He/4He value is located in the Yanfan basin-Changping area, which belongs to the transition zone from Yanshan uplift mountainous area to plain (Figure 8).
4.1.2 CO2
The carbon isotope composition of carbon-containing compounds in hot springs contains a lot of important information (Hilton, 1996; Kulongoski et al., 2013; Babuška et al., 2016). A large number of research results showed that δ13CCO2 of organic origin CO2 was generally less than −10‰ and mainly distributed between −30‰ and −10‰; δ13CCO2 of inorganic origin CO2 was generally greater than−8‰ and mainly distributed between −8‰ and +3‰. In inorganic origin CO2, the δ13CCO2 value of CO2 transformed from carbonate rock was close to that of carbonate rock, which was about 0 ± 3‰. The δ13CCO2 value of CO2 of volcanic magma origin and mantle origin was −6‰ ± 2‰ (Domokos et al., 2021).
Among the gases of 44 hot springs, only the central Beijing tectonic zone had complete CO2 concentration data and carbon isotope data. There were some CO2 concentration data in the west. The concentration of CO2 in the hot springs of Beijing and part of the western Zhangjiakou-Bohai Fault Zone was low, ranging between 0–6.1%, and the concentration of N2 was high, ranging between 69.18–99.42%. Carbon isotopes of CO2 from various sources in the hot spring gases of Beijing overlapped with each other.
It can be seen from the relationship of δ13CCH4−−δ13CCO2 that the organic origin CO2 in the gas of well points 11, 13, 15, 10, 12, 1, 14, 16, and 21 was mainly formed by methane oxidation (Figure 4).
In Beijing, δ13CCO2 values vary from −19‰ to −9.6‰, which shows the characteristics of organic sediments mixed with mantle origin (mainly organic). Figure 3 shows the analytical results of Beijing hot spring gas. It is then clear that all Beijing hot spring gases are plotted within the two mixing lines. This feature strongly suggests that CO2 in Beijing hot spring gases is released from three different sources: crustal metamorphic, mantle and organic components (Figure 3).
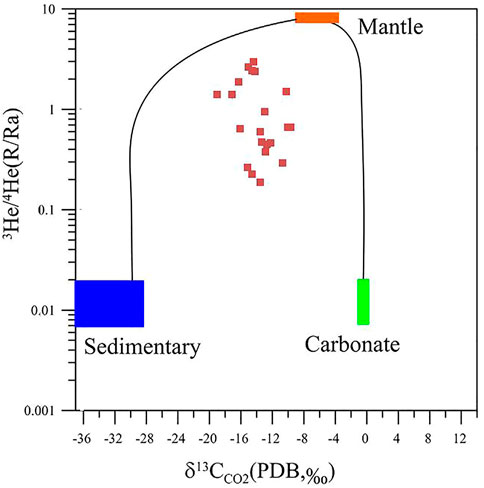
FIGURE 3. Diagram of 3He/4He (R/Ra) vs.δ13CCO2 (PDB,‰) ratios (Mixed line according to Xu et al., 1984).
The δ13CCO2 value is mainly organic, but the relatively high δ13CCO2 value in the Yanfan basin-Nankoushanqian fault area shows that mantle-sourced CO2 is also relatively high (Figure 4).
4.1.3 CH4
The concentration of CH4 in hot spring gases of the main tectonic zone of Beijing mostly varied from 0% to 6.1%. However, the XJ point of Xiji, which was located at the intersection of Zhangjiakou-Bohai Fault Zone and Xiadian Fault, was up to 27.06%. This is worthy of further study. The relationship diagram of carbon isotopeδ13CCH4−δ13CCO2 (Figure 5) showed that the methane of hot spring sites of geothermal well No.2 and No.20 was of inorganic origin. The other hot springs were of organic origin, among which the gas CH4 of the hot spring sites No.5, No.7, No.4, and No.19 was formed by methane-producing bacteria under strict anaerobic conditions (Domokos et al., 2021). The gas CH4 in hot spring wells No. 3, 6, and 18 was mainly formed by carbon dioxide reduction.
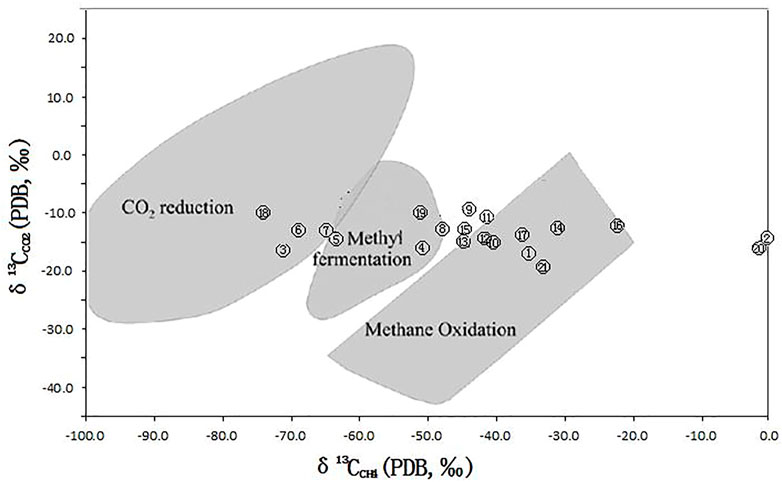
FIGURE 5. Relationship diagram of 13CCH4−δ13CCO2 (base figure according to Woltemate et al. (1984)).
4.1.4 H2
The hydrogen in the fault zone may have the following sources: (1) As a deep source of gas, a large amount of H2 was stored on the Earth when the Earth was formed. H2 would escape into the atmosphere along the weak areas of the Earth (Neal and Stanger, 1983); (2) When the fracture broke, water-rock reaction occurred on the fresh silicate rock surface to produce hydrogen (Kameda et al., 2003). The more active the active fault zone was, the more developed the fresh silicate rock fracture surface in the fault zone was, and the more H2 was produced (Kita et al., 1982); (3) U and Th elements in rocks produced high concentrations of hydrogen with water during radioactive decay (Lin et al., 2005; Donze et al., 2020); (4) A large amount of hydrogen was produced in the serpentinization process of olivine (Katayama et al., 2010; Donze et al., 2020); (5) Soil organic matters produced hydrogen during anaerobic bacterial fermentation (Libert et al., 2011). H2 is the lowest density gas known in the world, featuring strong diffusivity and penetrability. It is difficult to dissolve in water, slightly polluted by dissolving hydrogen in atmospheric precipitation during the upward migration of deep crust, and it is a very good tracer gas (Neal and Stanger, 1983). The survey results showed that the normal change of hydrogen concentration in Beijing was basically between 0 and 100ppm, which was a micro-concentration change (Supplementary Table S2).
4.2 Relationship between spatial change of the gases of hot spring in geothermal well and fault activity and seismic activity
Since the 1980s, a group of scholars have studied the upper mantle structure of the crust in North China by tomography. The present strong seismic activity and obviously low velocity of the lower crust in North China are different from other stable cratons in the world. The lithospheric structure is highly heterogeneous, which is important evidence of the destruction of the North China Craton.
The subduction of the Pacific Plate has a profound influence on the velocity structure of the North China Craton. It can be clearly seen from the topography and the distribution of gravity gradient zones that their boundary lines are parallel to the Japanese Trench and the Ryukyu Trench. The underthrust of the Pacific Plate made the upper mantle of the North China Craton form a mantle wedge-lead, and the geochemical action above the mantle wedge made the asthenosphere material rise and result in the thinning and fracture of the lithosphere (Wu et al., 2008; Zhu et al., 2011). However, the impact of the subduction of the Pacific Plate is far more than that. Geological activities such as magma intrusion, earthquakes, volcanic activity, and mineral formation caused by the destruction of the North China Craton are closely related to it. The subduction of the Pacific Plate can provide evidence for the geodynamics of the Zhangjiakou-Bohai Fault Zone (Zhu and Xu, 2019) (Figure 6).
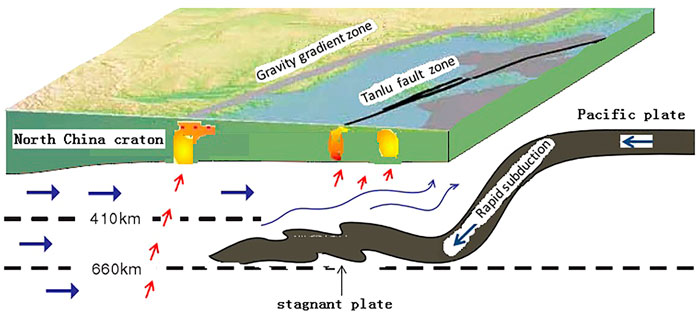
FIGURE 6. Mantle convection mechanism of destruction and transformation of the North China Craton (base map after Zhu et al., 2011).
The intrusion of mantle-derived materials in the middle and upper crusts and the horizontal change of hot rock mass in the Zhangjiakou-Bohai Seismic Zone may be due to the increase in the supply of deep crustal fluids caused by the intrusion of mantle-derived materials. The long-standing fluids under the seismogenic layer of the crust will affect the structure of the fault zone, reduce the strength of the fault zone and change the regional stress field, which will lead to the concentration of stress on the fault zone and then easily cause earthquakes (Yang et al., 2018) and generate the Zhangjiakou-Bohai Fault Zone.
4.2.1 Spatial differences of helium release from deep source
The existing observation data showed that the unsteady mantle flow caused by the continuous subduction of the Pacific Plate to the East Asian continent since the Mesozoic played an important role in the overall destruction of the eastern part of the North China Craton [13, 24, 36, and 61]. The mantle magma caused by the destruction of the North China Craton invaded the crust of North China and caused the release of deep-source helium in the mantle, including the Zhangjiakou-Bohai Fault Zone. In terms of space, the trend of two large tectonic zones in Eastern China (the north-south gravity gradient zone and the Tanlu Fault Zone) also showed a correlation with the subduction of the Pacific Plate (Figure 6).
The helium isotope correction value of geothermal hot spring water in the Zhangjiakou-Bohai Tectonic Zone showed an obvious peak area from west to east (Figures 7, 8), which indicated that the Rc/Ra value in the Zhangjiakou-Bohai Fault Zone showed obvious spatial distribution differences from west to east:
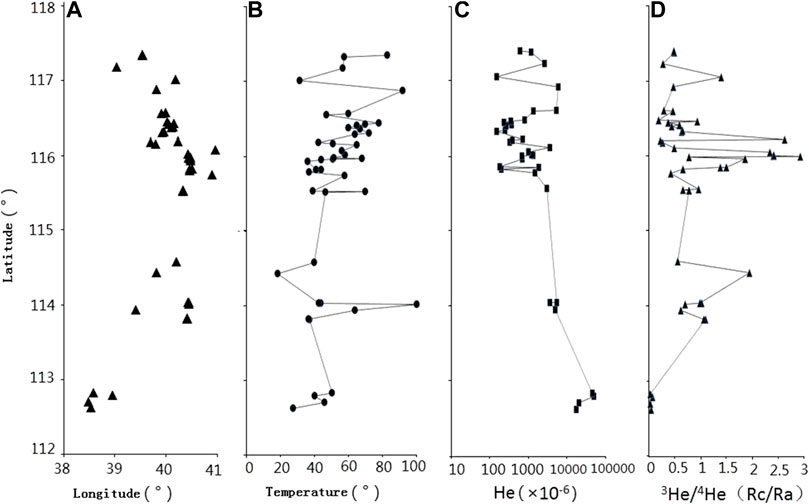
FIGURE 7. Spatial variation of hot spring water temperature; He concentration in hot spring gases and helium isotope correction value (A) distribution of geothermal hot spring sampling sites; (B) temperature; (C) He concentration; (D) helium isotope correction value.
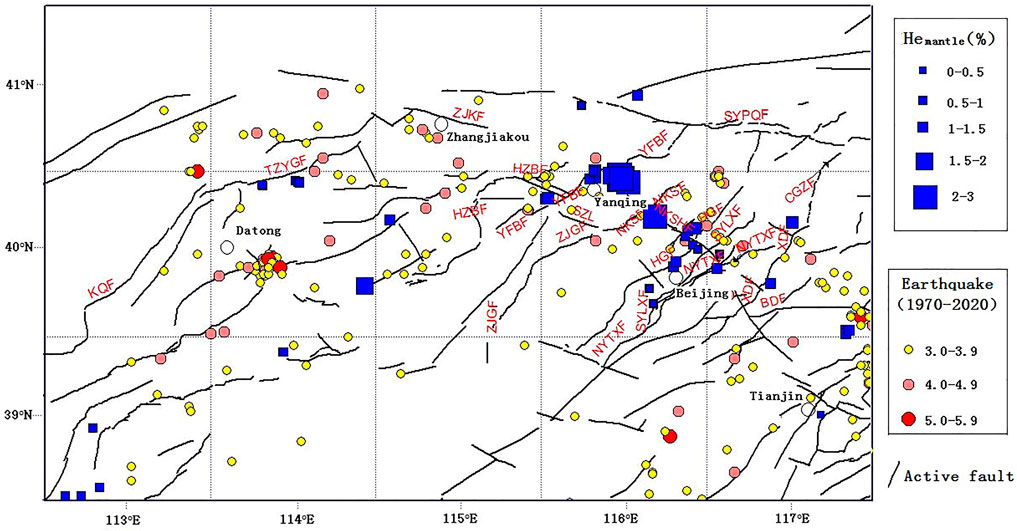
FIGURE 8. Relationship between the ratio of mantle-derived helium in geothermal wells and hot spring wells in the Zhangjiakou-Bohai Fault Zone and seismicity.
This peak area (about E116° in longitude) included the Yanfan Basin-Changping Area, which was located in the transition zone from the mountainous area of the Yanshan Uplift to the plain. It was the intersection transition zone of two tectonic units with complex fault structures and active seismic activity (Li, 2021), and its highest mantle source He was 35.4%. Other areas did not have such remarkable characteristics of mantle-derived helium release. From the differences in spatial changes (Wei et al., 2015) this peak area (about E116° in longitude) was just in a transition zone. That was from the central and western parts of the North China Craton where the lithosphere was partially reformed or thinned to the eastern part of the craton (Figure 6). This transition zone was closely related to the significant changes of crust and lithosphere thickness near the boundary between the eastern part of the Craton and the central and western parts of the Craton. The gravity gradient zone between the north and the south was closely related to the sudden change in topography (Figure 6), which also indicated the particularity of this transitional zone.
From the source of helium, the mantle magma caused by the destruction of the North China Craton invaded the crust of North China, and it also caused the release of deep-source helium in the mantle, including the Zhangjiakou-Bohai Fault Zone and the emergence of peak areas. Specifically, partial melting (or initial partial melting) of lithosphere or asthenosphere mantle rocks is the most effective way to release mantle-derived He (Ballentine and Burnard, 2002), and the most direct evidence is the active volcano system (or Quaternary or Cenozoic volcanic activity area) and the existence of deep melt revealed by geophysical observation. In the volcanic activity areas, mantle-derived melt can transport mantle He to the Earth’s surface and atmosphere through magma eruption or intrusion. This is common in volcanic activity areas represented by mid-ocean ridge and island arc volcanic areas. This area is a non-volcanic activity area, and there is an obvious low velocity anomaly in the lower mantle 70 km (Figure 9) (Santosh et al., 2010), which indicates that the deep lithospheric mantle in this area may be partially melted, which provides a material basis for the crustal displaying of mantle-derived He.
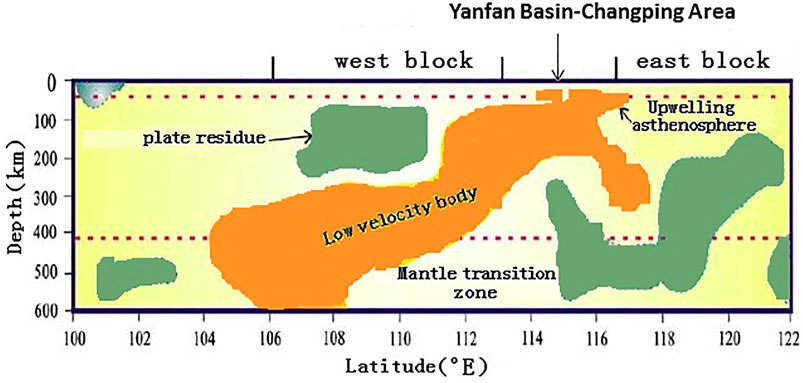
FIGURE 9. Mantle structure model of the North China Craton at 40°N latitude (base map after Santosh et al., 2010).
Generally, the rapid and short-range migration and other factors will cause the mantle He to be contaminated with radioactive He to a relatively low degree. However, with the distance from the Yanqing-Changping Area (the highest proportion of mantle-derived He is 35.4%) along the trend of the fault zone or the distance from the fault zone in the vertical direction, the amount of radioactive He in the crust will increase gradually, which will lead to the difference in the spatial distribution of mantle-derived materials inside and outside the fault zone.
4.2.2 The nature, scale, and attitude of the fault zone play a decisive role in the migration of mantle-derived components to the earth’s surface.
According to the research, the peak area of the mantle source located at (about E116° n longitude) included the northern margin fault of the Yanji Basin, and the low-velocity bodies in the crust were found below the Machikou Depression (Gao et al., 2010). The active faults in the shallow crust that controlled these depression structures included the NEE fault in the northern margin of the Yanji Basin, the Nankou piedmont fault, and the Huangzhuang-Gaoliying fault. These faults have been active since the late Pleistocene, and they are very active. The deep faults in the Earth’s crust and active faults in the shallow part of the crust that controlled the development of basins might be in a state of “convergence without intersection” (Gao et al., 2010), and these deep and shallow faults intersected with the Sunhe fault in the northwest to the south, which led to the increase in fragmentation and permeability of the fault zone and was conducive to the rapid migration of mantle-derived materials to the Earth’s surface.
Therefore, the deep fault zone with high permeability and a high dip angle provided favorable structural conditions for the upwelling of mantle-derived materials in the region. This phenomenon was also observed at the intersection of Xianshuihe Fault, Longmenshan Fault and Moxi Fault in China (Zhou et al., 2017; Zhu et al., 2017; Zhou et al., 2020; Li et al., 2022; Xu et al., 2022) and in the western USA (Hu et al., 2018). The area with low P wave velocity corresponded to a higher helium isotope. In the intersection area of faults, faults developed, which provided a good channel for the upward migration of deep fluids. A large amount of mantle-derived helium migrated to the Earth’s surface.
The contribution rate of mantle-derived helium in the Zhangjiakou-Bohai Fault Zone was at a medium level. Although the seismic activity was not higher than that in Sichuan-Yunnan, it still had strong activity. Especially, the Yanji Basin, which was located in the Zhangjiakou-Bohai Fault Zone with strong seismicity and high frequency, had a high value background of mantle-derived helium (Zhou, 2011; Zhang, 2013) and an abnormal high value background of soil gas concentrations of He and H2, CO2, and Rn (Li et al., 2009). It was worthy of further research on earthquake prediction as a key monitoring area.
4.3 Time change of helium isotope in geothermal hot spring gases
From October 2011 to November 2020, there were more than two gas helium isotope analysis data in 9 hot spring spots in the Zhangjiakou-Bohai Fault Zone, among which 8 spots showed a synchronous increase and still maintained a high value until April 2020. During this period, the earthquakes with a magnitude of 3 or above in the capital circle showed a trend of increasing activity from 2015 (Figure 10). This might be related to the increase in upwelling of deep fluid in the Zhangjiakou-Bohai Fault Zone. The rising helium isotope value of hot spring gases in this area indicated that there was an obvious upwelling of deep fluid, which might weaken the fault to some extent (Cappa and Rutqvist, 2012; Klemperer et al., 2013; X Zhang et al., 2019) and increase the pore pressure inside the fault (Giammanco et al., 2008; Terakawa et al., 2013; Berryman, 2016). This played an important role in promoting the preparation and occurrence of earthquakes (Fulton and Saffer, 2009; Brauer et al., 2011). At present, the monitoring time interval of helium isotope in the Zhangjiakou-Bohai Fault Zone is long, and the resolution of earthquake prediction is not sufficient. It is necessary to conduct high-density long-term observations in the future and further observe the correlation between corresponding changes and earthquakes with the observation of gas concentration.
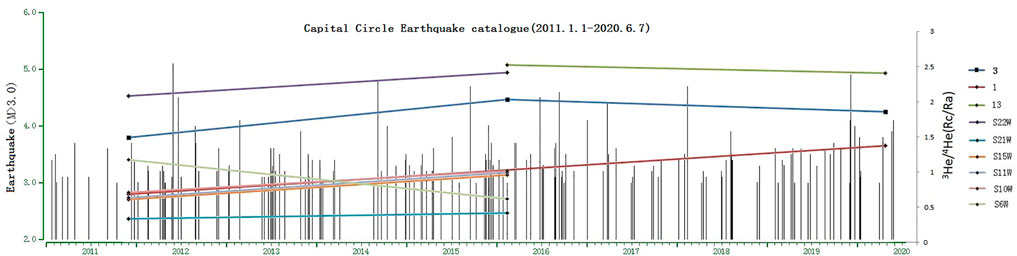
FIGURE 10. The relationship between seismic activities with percentage of mantle-derived He in hot spring gases along the Zhangjiakou-Bohai Fault Zone.
5 Conclusion
1) According to the gas composition characteristics of hot springs, the geothermal hot springs in the Zhangjiakou-Bohai Fault Zone are mainly composed of N2. Helium in hot spring gases mainly comes from the crust source, and N2 mainly comes from the atmosphere.
2) The existing observation data showed that Zhangjiakou-Bohai Fault Zone geothermal hot springs had obvious mantle-derived helium (>2%). This is related to the destruction of the North China Craton, the subduction of the Pacific Plate led to the destruction of the North China Craton and the up welling of mantle magma, enhanced fluid metasomatism, melting, and magmatism and Zhangjiakou-Bohai Fault Zone provided a good channel for upward migration of mantle-derived helium, the mantle magma caused by the destruction of the North China Craton invaded the crust of North China, and caused the release of deep-source helium in the mantle, including the Zhangjiakou-Bohai Fault Zone.
3) The upwelling release of mantle-derived materials in Zhangjiakou-Bohai Fault Zone has a good corresponding relationship with regional seismicity, and it may promote the preparation and occurrence of regional earthquakes. The deep faults in the Earth’s crust have the deep tectonic background of seismic development.
4) There is an obvious peak in the helium isotope of geothermal hot springs of the Zhangjiakou-Bohai Fault Zone, and it is located in the transition zone from the mountainous area to the plain in the Zhangjiakou-Bohai Fault Zone- It also happens to be the North-South gravity gradient belt across North China (about E116° in longitude). From there more mantle-derived materials upwelled.
Data availability statement
The original contributions presented in the study are included in the article/Supplementary Material; further inquiries can be directed to the corresponding author.
Author contributions
MY: task management, field investigation, paper writing; GL: document collection and sorting, paper compilation; ZL, field investigation, data collection; MY: data collection and field investigation; GL: operation of tasks; PH: field survey, sampling; XS: data sorting; KH: preparation and commissioning of instruments; BC: instrument measurement; XW, preparation and coordination of field work; LL and LX participated in the analysis of gas isotope sample.
Acknowledgments
We thank the editor and two reviewers for their constructive comments and suggestions.
Conflict of interest
The authors declare that the research was conducted in the absence of any commercial or financial relationships that could be construed as a potential conflict of interest.
Publisher’s note
All claims expressed in this article are solely those of the authors and do not necessarily represent those of their affiliated organizations, or those of the publisher, the editors, and the reviewers. Any product that may be evaluated in this article, or claim that may be made by its manufacturer, is not guaranteed or endorsed by the publisher.
Supplementary material
The Supplementary Material for this article can be found online at: https://www.frontiersin.org/articles/10.3389/feart.2022.933066/full#supplementary-material
Reference
Alonso, M., Pérez, N. M., Padrón, E., Hernández, P. A., Melián, G. V., Sumino, H., et al. (2021). Changes in the thermal energy and the diffuse 3He and 4He degassing prior to the 2014-2015 eruption of Pico do Fogo volcano, Cape Verde. J. Volcanol. Geotherm. Res. 416, 107271. ISSN 0377-0273. doi:10.1016/j.jvolgeores.2021.107271
Babuška, V., Růžek, B., and Dolejš, D. (2016). Origin of earthquake swarms in the Western Bohemian Massif: Is the mantle CO2 degassing, followed by the Cheb Basin subsidence, an essential driving force? Tectonophysics 668-669, 42–51. doi:10.1016/j.tecto.2015.12.008
Ballentine, C. J., and Burnard, P. G. (2002). 12. Production, release and transport of noble gases in the continental crust. Rev. Mineral. Geochem. 47, 481–538. doi:10.2138/rmg.2002.47.1210.1515/9781501509056-014
Berryman, J. G. (2016). Role of fluid injection in the evolution of fractured reservoirs. Int. J. Eng. Sci. 103, 45–58. doi:10.1016/j.ijengsci.2016.02.004
Bräuer, K., Kämpf, H., Koch, U., and Strauch, G. (2011). Monthly monitoring of gas and isotope compositions in the free gas phase at degassing locations close to the Nový Kostel focal zone in the Western Eger Rift¸ Czech Republic. Chem. Geol. 290 (3), 163–176. doi:10.1016/j.chemgeo.2011.09.012
Cao, C., Zhang, M., Tang, Q., Yang, Y., Lv, Z., Zhang, T., et al. (2018). Noble gas isotopic variations and geological implication of Longmaxi shale gas in Sichuan Basin, China. Mar. Petroleum Geol. 89, 38–46. doi:10.1016/j.marpetgeo.2017.01.022
Cappa, F., and Rutqvist, J. (2012). Seismic rupture and ground accelerations induced by CO2 injection in the shallow crust. Geophys. J. International 190 (3), 1784–1789. doi:10.1111/j.1365-246x.2012.05606.x
Chen, C., Jianming, H. E., Layue, L. I., and Long, D. U. (2016). Analysis of the deformation characteristics of Zhangjiakou-Bohai Fault zone and its adjacent regions based on crossfault observation data and GPS data[J]. South china J. Seismol. 36 (3), 17–28. doi:10.13512/j.hndz.2016.03.003
Domokos, G., Pujol, M., Gilfillan, M., Stuart, V., and Stuart, F. M. (2021). Noble gases constrain the origin, age and fate of CO2 in the vaca muerta shale in the neuquén basin (Argentina). Chem. Geol. 577, 120294. ISSN 0009-2541. doi:10.1016/j.chemgeo.2021.120294
Donze, F., Truche, L., Namin, P., Lefeuvre, N., and Bazarkina, E. (2020). Migration of natural hydrogen from deep-seated sources in the são francisco basin, Brazil. Geosciences 10, 346. doi:10.3390/geosciences10090346
Feng, Y., Yang, J., Sun, J., and Zhang, J. (2020). Material records of North China Craton destruction induced by Mesozoic paleo Pacific plate subduction. Sci. Sin. -Terrae. 50, 651–662. doi:10.1360/SSTe-2019-0210
Fulton, P. M., and Saffer, D. M. (2009). Potential role of mantle-derived fluids in weakening the San Andreas Fault. J. Geophys. Res. 114 (B7), B07408. doi:10.1029/2008jb006087
Gao, Z., Chen, Q., Huang, J., Cheng, Q., and Li, L. (2010). Velocity structure beneath the active faults in beijing area and their seismo-tectonic characteristics. Technol. Earthq. Disaster Prev., 5. 271. 280.
Giammanco, S., Palano, M., Scaltrito, A., Scarfì, L., and Sortino, F. (2008). Possible role of fluid overpressure in the generation of earthquake swarms in active tectonic areas: The case of the Peloritani Mts. (Sicily, Italy). J. Volcanol. Geotherm. Res. 178 (4), 795–806. doi:10.1016/j.jvolgeores.2008.09.005
Hilton, D. R. (1996). The helium and carbon isotope systematics of a continental geothermal system: results from monitoring studies at Long Valley caldera (California, U.S.A.). Chem. Geol. 127 (4), 269–295. doi:10.1016/0009-2541(95)00134-4
Hu, J., Badal, J., Yang, H., Li, G., and Peng, H. (2018). Comprehensive crustal structure and seismological evidence for lower crustal flow in the southeastern margin of Tibet revealed by receiver functions. Gondwana Res. 55, 42–59. doi:10.1016/j.gr.2017.11.007
Italiano, F., Martinelli, G., Bonfanti, P., and Caracausi, A. (2009). Long-term (1997-2007) geochemical monitoring of gases from the Umbria-Marche region. Tectonophysics 476, 282–296. doi:10.1016/j.tecto.2009.02.040
Kameda, J., Saruwatari, K., and Tanaka, H. (2003). H2 eneration in wet grinding of granite and single-crystal powders and implications for H2 oncentration on active faults. Geophys. Res. Lett. 30, 2063. doi:10.1029/2003gl018252
Katayama, I., Kurosaki, I., and Hirauchi, K. (2010). Low silica activity for hydrogen generation during serpentinization: An example of natural serpentinites in the Mineoka ophiolite complex, central Japan. Earth Planet. Sci. Lett. 298, 199–204. doi:10.1016/j.epsl.2010.07.045
Kita, I., Matsuo, S., and Wakita, H. (1982). H2generation by reaction between H2O and crushed rock: An experimental study on H2degassing from the active fault zone. J. Geophys. Res. 87 (B13), 10789–10795. doi:10.1029/jb087ib13p10789
Klemperer, S. L., Kennedy, B. M., Sastry, S. R., Makovsky, Y., Harinarayana, T., and Leech, M. L. (2013). Mantle fluids in the Karakoram fault: Helium isotope evidence. Earth Planet. Sci. Lett. 366, 59–70. doi:10.1016/j.epsl.2013.01.013
Kulongoski, J. T., Hilton, D. R., Barry, P. H., Esser, B. K., Hillegonds, D., and Belitz, K. (2013). Volatile fluxes through the Big Bend section of the San ndreas Fault, California: Helium and carbon-dioxide systematics. Chem. Geol. 339, 2–102. doi:10.1016/j.chemgeo.2012.09.007
Li, H. (2021). Earthquake mechanism analysis of cross-faults system of Zhangjiakou-bohai fault belt in North China[J]. IOP Conf. Ser. Earth Environ. Sci. 658 (1), 012–013. doi:10.1088/1755-1315/658/1/012013
Li, Y., Du, J., Wang, F., Zhou, X., Pan, X., and Wei, R. (2009). Geochemical characteristics of soil gas in Yanqing--Huailai basin , North China. Act. a Seismol. Sin. 31 (1), 82–91.
Li, Z., Wang, X., Li, L., Zhang, M., Tao, M., Xing, L., et al. (2014). Development of new method of δ13C measurement for trace hydrocarbons in natural gas using solid phase micro-extraction coupled to gas chromatography isotope ratio mass spectrometry. J. Chromatogr. A 1372, 228–235. doi:10.1016/j.chroma.2014.10.089
Li, Q., Zhao, C., Wang, Y., Zhou, Y., and Ran, H. (2022). Spring gas geochemistry in the weixi-qiaohou fault zone: understanding the fluid characteristics of the Western boundary of the sichuan-yunnan rhombic block. Geochem. Int. 60 (1), 109–121. doi:10.1134/s0016702921150027
Libert, M., Bildstein, O., Esnault, L., Jullien, M., and Sellier, R. (2011). Molecular hydrogen: An abundant energy source for bacterial activity in nuclear waste repositories. Phys. Chem. Earth, Parts A/B/C 36, 1616–1623. doi:10.1016/j.pce.2011.10.010
Lin, L. H., Hall, J., Lippmann-Pipke, J., Ward, J. A., Lollar, B. S., DeFlaun, M., et al. (2005). Radiolytic H2 in continental crust: Nuclear power for deep subsurface microbial communities. Geochem. Geophys. Geosystems 6, Q07003. doi:10.1029/2004gc000907
Lu, C. (2016). Geochemical characteristics of fluid and its Genesis in the capital area of China. Beijing: Institute of earthquake forecasting, CEA.
Ma, W., Xu, X., Yu, G., and Zhang, L. (2004). The relationship between seismic activity and fault activity in Beijing region. Seismol. Geol. 26 (2), 293–304. doi:10.1007/BF02873097
Mamyrin, B. A., Anufrier, G. S., Kamensky, I. L., and Tolstikhin, I. N. (1970). Determination of the isotopic composition of helium in the atmosphere. Geochem. Int. 7, 498–505.
Neal, C., and Stanger, G. (1983). Hydrogen generation from mantle source rocks in Oman. Earth Planet. Sci. Lett. 66, 315–320. doi:10.1016/0012-821x(83)90144-9
Ozima, M., and Podosek, F. A. (1983). Noble gas geochemistry. Cambridge: Cambridge University Press, 367.
Pang, Z., Huang, S., and Hu, S. (2014). Progress and Prospect of geothermal research in China(1995-2014). Geol. Sci. 2014 (3), 719–727.
Sano, Y., and Wakita, H. (1985). Geographical distribution of3He/4He ratios in Japan: Implications for arc tectonics and incipient magmatism. J. Geophys. Res. 90, 8729–8741. doi:10.1029/jb090ib10p08729
Santosh, M., Zhao, D., and Kusky, T. (2010). Mantle dynamics of the Paleoproterozoic North China Craton: A perspective based on seismic tomography. J. Geodyn. 49 (1), 39–53. doi:10.1016/j.jog.2009.09.043
Terakawa, T., Hashimoto, C., and Matsu'ura, M. (2013). Changes in seismic activity following the 2011 Tohoku-oki earthquake: Effects of pore fluid pressure. Earth Planet. Sci. Lett. 365, 17–24. doi:10.1016/j.epsl.2013.01.017
Umeda, K., and Ninomiya, A. (2009). Helium isotopes as a tool for detecting concealed active faults. Geochem. Geophys. Geosystems 10 (8), Q08010. doi:10.1029/2009gc002501
Wei, W., Aeschbach-Hertig, W., and Chen, Z. (2015). Identification of He sources and estimation of He ages in groundwater of the North China Plain. Appl. Geochem. 63, 182–189. ISSN 0883-2927. doi:10.1016/j.apgeochem.2015.08.010
Woltemate, I., Whiticar, M. J., and Schoell, M. (1984). Carbon and hydrogen isotopic composition of bacterial methane in a shallow freshwater lake. Limnol. Oceanogr. 29 (5), 985–992.
Wu, F., Xu, Y., Gao, S., and Zheng, J. (2008). Lithospheric thinning and destruction of the North China Craton. Acta Petrol. Sin. 24 (6), 1145–1174. doi:10.1016/j.sedgeo.2008.03.008
Xu, J., Song, C., and Chu, Q. (1998). Preliminary study on the seismotectonic characteristics of the Zhangjiakou Penglai fault zone. Seismol. Geol. 20 (2), 146–154. doi:10.1088/0256-307X/16/9/027
Xu, S., Zheng, G., Nakai, S., Wakita, H., Wang, X., and Guo, Z. (2013). Hydrothermal He and CO2 at Wudalianchi intra-plate volcano, NE China. J. Asian Earth Sci. 62, 526–530. doi:10.1016/j.jseaes.2012.11.001
Xu, X., Han, Z., Yang, X., Zhang, S., Yu, G., Zhou, B., et al. 2016, Seismotectonic map of China and adjacent areas, Beijing: Seismological Press. Data DB
Xu, S., Guan, L. F., Zhang, M. L., Zhong, J., Liu, W., and Xie, X. G (2022). Deep source gas release from Xianshui River Anning River fault zone in the eastern margin of Qinghai Tibet Plateau Chinese Science. Chin. Sci. Earth Sci. 52 (2), 291–308. doi:10.1360/SSTe-2021-0062
Yang, Q., Wu, Q., Sheng, Y., Gao, J., Song, J., and Di, L. (2018). Body wave imaging and seismogenic environment analysis of zhangbo seismic belt and its adjacent areas. Chin. J.Geophys. (in Chinese) 61 (8), 3251–3262. doi:10.6038/cjg.2018L0628
Yokoyama, T., Nakai, S., and Wakita, H. (1999). Helium and carbon isotopic compositions of hot spring gases in the Tibetan Plateau. J. Volcanol. Geotherm. Res. 88, 99–107. doi:10.1016/s0377-0273(98)00108-5
Zhang, W. (2013). Geochemistry of hot spring fluid in the basin ridge tectonic area of northwest Beijing. Beijing: Institute of earthquake forecasting,CEA.
Zhang, H., Xie, F., Jiao, Q., and Li, R. (2007). Cross-fault Deformation Observation and Crustalstress Field in Capital Circle Region. Seismol. Geol. 29 (4), 706–715. doi:10.3969/j.issn.0253-4967.2007.04.002
Zhang, X., Jeffrey, R. G., and He, C. (2019). A Numerical Model for the Effect of Permeability Change on Faulting During Fluid Injection[J]. J. Geophys. Res. Solid Earth 124, 2080.
Zhao, B., Gao, Y., Shi, Y., and Jin, H. (2011). Crustal shear wave splitting in the intersection area of yanzhangjiakou Bohai seismic belt and Shanxi seismic belt. Chin. J.Geophys. 54 (6), 1517–1527. (in Chinese). doi:10.3969/j.issn.0001-5733.2011.06.Oil
Zhao, C., Ran, H., and Wang, Y. (2012). Present-daymantle-derivedheliumreleaseintheTengchongvolcanicfield, Southwest China: Implicationsfortectonicsandmagmatism. Acta Petrol. Sin. 28 (4), 1189–1204. doi:10.1007/s11783-011-0280-z
Zhou, X. (2011). Gas geochemistry in Western sichuan after wenchuan ms 8.0 earthquake. Hefei: China University of science and Technology Press.
Zhou, X., Wang, W., Chen, Z., Yi, Y., Liu, L., Xie, C., et al. (2015). Hot Spring Gas Geochemistry in Western Sichuan Province, China After the Wenchuan Ms 8.0 Earthquake. Terr. Atmos. Ocean. Sci. 26 (4), 361–373. doi:10.3319/tao.2015.01.05.01(tt)
Zhou, X., Liu, L., Chen, Z., Cui, Y., and Du, J. (2017). Gas geochemistry of the hot spring in the Litang fault zone, Southeast Tibetan Plateau. Appl. Geochem. 79, 17–26. doi:10.1016/j.apgeochem.2017.01.022
Zhou, X., Wang, W., Li, L., Hou, J., Xing, L., Li, Z., et al. (2020). Geochemical features of hot spring gases in the Jinshajiang-Red River fault zone, Southeast Tibetan Plateau. Acta Petrol. Sin., 36(7): 2197–2214. doi:10.18654/1000-0569/2020.07.18
Zhou, X., Yan, Y., Fang, W., Wang, W., Shi, H., Li, P., et al. (2021). Short-Term Seismic Precursor Anomalies of Hydrogen Concentration in Luojishan Hot Spring Bubbling Gas, Eastern Tibetan Plateau. Front. Earth Sci. 8, 586279. doi:10.3389/feart.2020.586279
Zhu, R., and Xu, Y. (2019). The subduction of the west Pacific plate and the destruction of the North China Craton. Sci. China Earth Sci. 62, 1340–1350. doi:10.1007/s11430-018-9356-y
Zhu, R., Chen, L., Wu, f., and liu, J. (2011). The time, scope and mechanism of the destruction of the North China Craton. Chin. Sci. Earth Sci. 41 (5), 583–592. doi:10.1007/s11430-011-4203-4
Keywords: helium isotope, carbon isotope, gas geochemistry, Zhangjiakou-Bohai fault zone, hot spring in geothermal well
Citation: Yang M, Liu G, Liu Z, Ma J, Li L, Wang Z, Hua P, Xing L, Sun X, Han K, Cui B and Wu X (2022) Geochemical characteristics of geothermal and hot spring gases in Beijing and Zhangjiakou Bohai fault zone. Front. Earth Sci. 10:933066. doi: 10.3389/feart.2022.933066
Received: 30 April 2022; Accepted: 04 July 2022;
Published: 05 September 2022.
Edited by:
Ying Li, China Earthquake Administration, ChinaReviewed by:
Zhi Chen, China Earthquake Administration, ChinaXiaocheng Zhou, China Earthquake Administration, China
Copyright © 2022 Yang, Liu, Liu, Ma, Li, Wang, Hua, Xing, Sun, Han, Cui and Wu. This is an open-access article distributed under the terms of the Creative Commons Attribution License (CC BY). The use, distribution or reproduction in other forums is permitted, provided the original author(s) and the copyright owner(s) are credited and that the original publication in this journal is cited, in accordance with accepted academic practice. No use, distribution or reproduction is permitted which does not comply with these terms.
*Correspondence: Guiping Liu, bGl1Z3BAYmpzZWlzLmNu