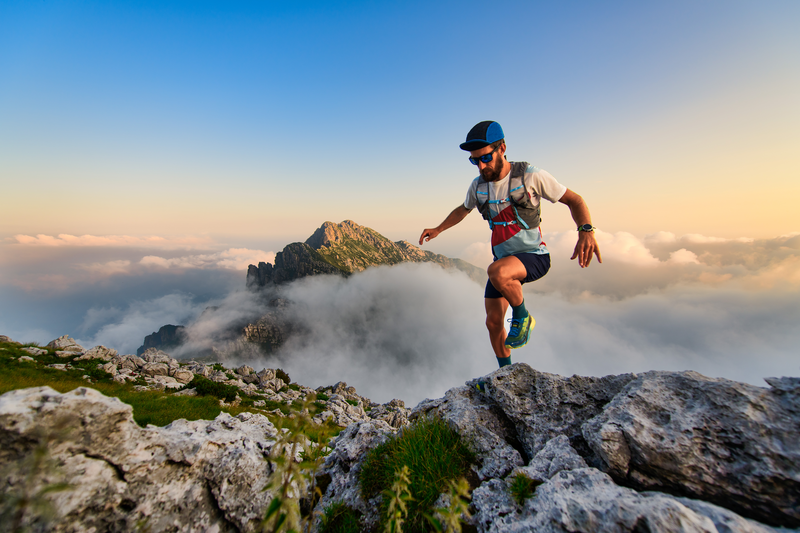
94% of researchers rate our articles as excellent or good
Learn more about the work of our research integrity team to safeguard the quality of each article we publish.
Find out more
ORIGINAL RESEARCH article
Front. Earth Sci. , 11 October 2022
Sec. Geohazards and Georisks
Volume 10 - 2022 | https://doi.org/10.3389/feart.2022.932110
This article is part of the Research Topic New Challenges for Seismic Risk Mitigation in Urban Areas View all 11 articles
Seismic monitoring networks are increasingly being used in urban areas to record and locate earthquakes. Recordings in the proximity of buildings also allow assessing, as a first approximation, the expected building damage. The DARR (Damage Assessment for Rapid Response) method provides local-scale information on expected damage patterns. The potential of this approach is discussed here for the August 24 M6 event of the Central Italy seismic sequence (2016–2017). We focus only on the first event of the sequence because cumulative damage is outside the scope of this study. The earthquake recordings are available from two Italian monitoring networks: the Italian Accelerometric Archive (ITACA) and the OSS (Osservatorio Sismico delle Strutture), which collects data from monitored buildings and bridges in Italy. We selected four target areas (Amatrice, Norcia, Visso and Sulmona) characterized by different epicentral distances and building typologies, that suffered different levels of damage during the M6 event on 24 August 2016. Using recordings either in the free field or in the basement of buildings, the expected relative displacement of building typologies common in the studied areas is calculated with the DARR method. Using predefined damage thresholds from literature, the obtained results allow quantifying the expected damage for dominant building typologies in the surroundings of the recording sites. We investigate and discuss the potential use and applicability of the DARR method in different areas depending on the epicentral distance and building characteristics. The results indicate that the DARR approach is useful for supporting and improving rapid response activities after a seismic event.
Most casualties caused directly by earthquakes are due to damage in residential buildings (So and Spence, 2013). For this reason, the rapid assessment of expected damage can support effective response actions and prioritize interventions, thereby reducing human losses. Assessing damage to buildings depends on multiple factors, such as the characteristics of the ground shaking, the building’s vulnerability (and its response to shaking) and the occurrence of local amplification (site effects).
Ground motion recordings allow extracting the ground shaking characteristics and, in particular, the peak parameters (e.g., peak ground acceleration, PGA). The engineering community has devoted considerable effort to identifying the critical values of ground motion that lead to building damage and collapse. This was done based on both empirical (Rota et al., 2008; Masi et al., 2019) and analytical (Borzi et al., 2008; Donà et al., 2020) approaches. Fragility and vulnerability curves have been defined for different building types and are currently used to estimate expected damage in case of seismic events (e.g., Borzi et al., 2008; Poggi et al., 2020).
Measuring and analyzing the ground motion is therefore of paramount importance for estimating expected damage to buildings. However, using peak ground motion parameters to assess expected damage does not account for the frequency content of the recorded signal. For this reason, the coverage of seismic monitoring networks is increasing worldwide and includes seismic stations installed both in the field and in buildings or infrastructure (Mori et al., 1998; Trifunac et al., 2001; Okada et al., 2004; Espinosa-Aranda et al., 2009; Gorini et al., 2010; Satriano et al., 2011; Wu et al., 2013; Parolai et al., 2017; Bragato et al., 2021). In Italy, ground motion recordings are collected in the Italian Accelerometric Archive (ITACA, Russo et al., 2022). In addition, the Italian monitoring network, OSS (Osservatorio Sismico delle Strutture, Dolce et al., 2017) comprises more than 120 public buildings, and some bridges and dams continuously monitored by low-cost seismic sensors.
The recorded signal in buildings allows assessing their response to earthquakes and monitoring changes to their structural health (e.g., Rahmani et al., 2015; Rahmani and Todorovska, 2021). Past studies have demonstrated the relevance of assessing the building’s fundamental period (Goel and Chopra, 1997), which is a key parameter for estimating the expected performance of buildings during earthquakes (Michel et al., 2010). Some authors (e.g., Crowley and Pinho, 2010; Michel et al., 2010) pointed out discrepancies between the simplified period-height relationships used in most building codes (e.g., Eurocode, CEN 2004) and the fundamental period estimated experimentally using ambient noise measurements (e.g., Gallipoli et al., 2009). Thus, several authors proposed period-height relationships based on experimentally estimated fundamental periods (e.g., Gallipoli et al., 2009; Michel et al., 2010; Gallipoli et al., 2022). It is also relevant to compare the fundamental frequency range of buildings and soils (e.g., Gallipoli et al., 2020) to assess the possible occurrence of soil-building resonance (e.g., Bard et al., 1996; Mucciarelli et al., 2004).
Since 2009, the OSS network has provided significant information on the dynamic response of single buildings during the main Italian earthquakes (Spina et al., 2010). The occurrence of damage is estimated by comparing the observed interstory drift values with thresholds defined in literature (e.g., Rossetto and Elnashai, 2003 for reinforced concrete, RC, buildings). However, the procedure requires at least two recordings (one at the top and another at the bottom of the building) in order to estimate the interstory drift ratio.
Based solely on recordings at the bottom of buildings (or in the free field nearby), it is possible to rapidly estimate the occurrence of damage by taking the simplified buildings linear dynamic response into account (Scaini et al., 2021; Petrovic et al., 2022). In fact, the DARR (Damage Assessment for Rapid Response) method uses the entire recording and simulates the maximum relative displacement (drift) for a specific building type (defined by fundamental frequency and damping ratio) based on simplified oscillators (single or multi-degree-of-freedom) using the Z transform (Lee, 1990; Jin et al., 2004; Parolai et al., 2015). The method has produced successful results for selected building types, including unreinforced masonry (URM) buildings (Petrovic et al., 2022), a common building typology in Italy and in particular in Central Italy (Sorrentino et al., 2019).
The DARR method can be extended to estimate expected damage to specific building typologies in the surrounding area of a recording (Scaini et al., 2021). In addition to interstory drift limits (e.g., Borzi et al., 2008; Rossetto et al., 2016), relative displacement limits (e.g., Lagomarsino and Giovinazzi, 2006) are available for different building types. These include both RC and URM typologies associated with different characteristics (height, age of construction, seismic design level). The occurrence of damage is assessed by comparing the estimated relative displacement or interstory drift (ratio) for each building type with the limits available in literature. However, DARR is based on a number of assumptions, in particular on an average building height (when considering building types instead of specific buildings) and the simplified dynamic behavior of building typologies (dominated by the fundamental mode/modes, obtained from period-height relationships from literature), the choice of thresholds for damage occurrence, and the homogeneous soil conditions in the target area.
In this work, we present an application of the DARR method to the first shock of the Central Italy seismic sequence, which occurred on 24 August 2016 (Rossi et al., 2018). Recordings are available at several locations (both in free field and in structures) and at different distances from the epicenter, from both the ITACA and OSS databases. We focus our study on four target areas (Amatrice, Norcia, Visso and Sulmona) with different epicentral distances for which different damage levels have been observed. The analysis of the available recordings of the event in the four locations shows that, based on the earthquake recordings and the building characteristics, it is possible to quickly estimate whether structural damage is expected for previously characterized building typologies dominant in the study area. Outcomes are compared with damage evidence collected during field surveys (visual inspection and subsequent damage assessment) performed in these areas after the 24 August 2016 event (e.g., Fiorentino et al., 2018; Sorrentino et al., 2019; D’Ayala et al., 2019).
For the damage assessment, we use DARR, a method proposed by Scaini et al. (2021) and Petrovic et al. (2022). The linear dynamic behavior of buildings is simulated as simple single-degree-of-freedom (SDOF) oscillators, describing the buildings in a first order approximation by their fundamental frequency and the damping ratio. The fundamental frequencies are estimated from building-soil specific period-height relationships from Gallipoli et al. (2022). An average story height of 3.5 m is assumed for the ground floor (e.g., Chieffo et al., 2019), for the upper storys 3 m are considered (e.g., Sorrentino et al., 2019). The latter is compatible with the minimum required height of 2.7 m in Italy and some other European countries (Appolloni and D’Alessandro, 2021) and accounts for the floor thickness. Additionally, 1.5 m are added for the roof. For the damping ratio, a standard value of 5% (e.g., Eurocode 8, CEN 2004) is used. The relative displacement between top and bottom (drift) is calculated with the Z transform (e.g., Lee, 1990; Jin et al., 2004; Parolai et al., 2015). The total displacement at the top can be obtained as the sum of the displacement at the bottom and the relative displacement. The interstory drift ratio is estimated by dividing the relative displacement (drift) by the building height.
The occurrence of damage can be estimated using either interstory drift (e.g., Rossetto et al., 2016) or relative displacement limits from literature (e.g., Lagomarsino and Giovinazzi, 2006), based on the characteristics (construction materials) and height of the studied buildings. In this study, we consider the relative displacement damage limits for low to mid-rise URM (simple stone and regular) and RC buildings, representative of the Italian building stock and dominant in the study area. The relative displacement thresholds for different damage states are adopted from Lagomarsino and Giovinazzi (2006, Table 1) for different building typologies. The limits for low, moderate and high-code RC frames are adopted for the zone of higher seismicity in the Italian official seismic zonation (Italian Seismic Hazard map, Meletti et al., 2006; Stucchi et al., 2011 and following modifications). The description of damage levels for both masonry and reinforced concrete buildings is based on the EMS-98 (Grünthal, 1998) macroseismic scale. The method focuses on the expected occurrence of structural damage (extensive or complete). Extensive damage corresponds to level-3 damage of EMS-98 (moderate structural damage, heavy non-structural damage). Complete damage includes both level 4 and 5 of the EMS-98 scale (heavy and very heavy structural damage and/or collapse). The absence of damage is associated with a relative displacement lower than 70% of the yielding displacement (Table 1), which corresponds to level-1 damage of the EMS-98 following Lagomarsino and Giovinazzi (2006). In all other cases, non-structural damage is assumed.
TABLE 1. Relative displacement limits (in centimeters) for selected building typologies according to Lagomarsino and Giovinazzi (2006).
Our study makes use of the recordings collected by the OSS network (Osservatorio Sismico delle Strutture, Dolce et al., 2017) and the ITACA database (Russo et al., 2022), in order to estimate the expected damage for selected locations for the 24 August 2016 M6 event. We focus on the first event of the Central Italy 2016 sequence, since the applied DARR method (Scaini et al., 2021; Petrovic et al., 2022) does not account for cumulative damage.
Based on the analysis of the damage patterns after the first event of the Central Italy seismic sequence (24 August 2016, Figure 1), four areas (Figures 2A–D) are selected for the application of DARR: Amatrice, Norcia, Visso and Sulmona. Two of the selected areas were very close to the epicenter (Amatrice and Norcia, respectively 9 and 15 km away). Nonetheless, the observed damage patterns (Stewart and Lanzo, 2018; D’Ayala et al., 2019) are very different due to the characteristics of the buildings (e.g., presence or absence of retrofitting) and the occurrence of site effects. Moreover, the different damage patterns could be due to the near-source effect (Luzi et al., 2016; Tinti et al., 2016; Chiaraluce et al., 2017). The third area (Visso) is located at a medium distance of 28 km from the epicenter. Damage was mostly observed in the central-western part of the town and hardly in the historical center located in the southeastern part (Gaudiosi et al., 2016). The fourth area (Sulmona) is located at a greater distance from the epicenter (approximately 90 km): here, the shaking was perceived by inhabitants but did not cause significant damage to the buildings.
FIGURE 1. Map showing the locations of the four test sites Amatrice, Norcia, Visso and Sulmona (red rhombi) and the epicenter of the M6 event (yellow star).
Since for Amatrice no recordings were available from the OSS network, a free field recording from the ITACA database located close to the historical center was used to estimate the expected damage for selected building typologies. For Norcia, Visso and Sulmona, recordings of sensors of the OSS, installed in the basement of a building located in the historical center (Norcia: 3 story RC school building, Visso: 2 story simple stone URM school building, Sulmona: 4 story RC hospital building) were used for the damage assessment in the target areas which correspond to the historical centers of the three towns. In the case of Visso, the target area also includes an urbanized area located in the central-western part of the town.
The building stock of the historical centers of the four considered towns is/was composed mainly of low- and mid-rise simple stone buildings mostly constructed before 1919 (Munari et al., 2010; Sorrentino et al., 2019). In addition, low-to mid-rise regular masonry and RC frame buildings were considered due to their presence in the studied areas. These buildings were constructed typically between 1950 and 1980.
The ground motion recordings in Amatrice are available from the ITACA database. The considered station AMT (Figure 2A, red square) is located on sandy-silty lithofacies (Todrani and Cultrera, 2021), which can be classified as soft soils. The minimum and maximum distances between the recording and the buildings in the old town are of 200 and 600 m, respectively (Figure 2A). Unfortunately, no recording in the historical center of Amatrice is available.
FIGURE 2. (A–D): Location of the earthquake recordings and the selected buildings for the four target areas (from left to right, Amatrice, Norcia, Visso and Sulmona). (E–H): Results of DARR for 3-story simple stone URM buildings in the target areas of Amatrice, Norcia, Visso and Sulmona. (I–K): Documented damage collected from post-event surveys after the 24 August 2016 event in Amatrice, Norcia and Visso. The damage patterns are extracted from OpenStreetMap (OpenStreetMap contributors, 2015) and are in good agreement with the documented post-event damage (e.g., Gaudiosi et al., 2016; Stewart and Lanzo, 2018; D’Ayala et al.,. 2019). In Sulmona no damage was observed in Sulmona.
According to Vignaroli et al. (2019) and Milana et al. (2018), the old town of Amatrice was built on sands or conglomerates. Amplification effects affect the ground shaking on the Amatrice terrace (Milana et al., 2018). We assume that the recording from the AMT station can be used to assess the expected damage in the old town, but the ground acceleration on the Amatrice terrace might be larger than at the recording site due to amplification effects (Gaudiosi et al., 2021).
Following the DARR method, the relative displacement (drift) for different building typologies representative for the building stock of Amatrice (2 and 3 story URM buildings in the historical center and 3–6 story RC buildings in the town) are estimated (Table 2). The maximum absolute value of both components (aligned with the main perpendicular directions of the town) is reported in Table 2.
TABLE 2. Damage assessment for Amatrice for the most common building typologies (2-3 story URM and 3–6 story RC buildings).
The studied building typologies were prevalent in the town of Amatrice. The building stock in the historical center was predominantly simple stone URM buildings (95%), most of them with 2 or 3 stories (Sorrentino et al., 2019). The fundamental periods T of the different building typologies are calculated using the period-height relationships proposed by Gallipoli et al. (2022) for URM and RC buildings on soft soils (URM: T = 0.0170H, RC-MRF: T = 0.0164H). The recordings of station AMT were first rotated to be aligned with the main perpendicular directions of the historical center, to take the orientation of the studied buildings (as a first order approximation, see Figure 2) into account. Based on the relative displacement limits in Table 1 (low-rise simple stone URM, extensive damage: 0.85 cm, complete damage: 1.40 cm; low-rise regular URM, extensive damage: 1.38 cm, complete damage: 2.36 cm), non-structural damage should be expected for 2 story simple stone and regular URM buildings (Table 2, relative displacement: 0.63 cm). Following the damage limits in Table 1, extensive damage should be expected for 3 story simple stone URM buildings (Table 2, relative displacement: 1.57 cm) and non-structural damage for 3 story regular URM buildings.
Our results for the expected extensive damage of 3 story simple stone URM buildings (Table 2; Figure 2E) are in accordance with the information on observed damage in the Amatrice historical center (e.g., Figure 2I; Stewart and Lanzo, 2018; D’Ayala et al., 2019). Following these reports, most of the low and mid-rise URM buildings were either highly damaged or totally destroyed during the 24 August 2016 event (see Figures 3A,B for a comparison of the main road before and after the considered M6 event). According to Sorrentino et al. (2019) most damage occurred to buildings with more than 2 stories. A video of the damage undergone by the historical center is available at: https://www.youtube.com/watch?v = 3-UDfhIH70M. Outside the historical center, in Amatrice a number of low (1–3 story) and mid-rise (4–7 story) RC buildings (of which 37 surveyed by Masi et al., 2019) were constructed in different time periods, based on different building codes (low and moderate). For these buildings, different damage levels were observed after the analyzed M6 event (Stewart and Lanzo, 2018; Masi et al., 2019; D’Ayala et al., 2019). In our study, we considered the damage thresholds for both low and moderate code RC frame buildings. Results are presented for 3–6 story RC buildings which are the most frequent RC buildings in the study area. Following both the low and moderate code thresholds, non-structural damage should be expected for 4–6 story RC frame buildings. Here, we present two examples of RC buildings for which information on the damage state is available from literature (D’Ayala et al., 2019). The first one is a 6 story RC building constructed in the late 1980s, following a moderate building code (Figure 2A, yellow triangle), only non-structural damage was inspected after the event. The second is a 5 story RC building constructed in the late 1970s or early 1980s following a low building code (Figures 3C, 2A, red triangle). This building was extensively damaged during the M6 event (Figure 3C), suffering structural damage (partial collapse of the masonry infill panels and shear failure of some columns). Additional pictures of the damage (non-structural and structural) undergone by the two RC buildings are available in D’Ayala et al. (2019). Considering the damage thresholds for the two selected mid-rise RC frame buildings, from our results we expect non-structural damage for both buildings. This is in accordance with the observed damage for the moderate-code, but not for the low-code building.
FIGURE 3. Photos of Amatrice. (A) View of the historical center of Amatrice on 26 February 2012 (Wikimedia Commons, Silvio Sorcini, licensed under CC-BY 4.0 and is available at: https://commons.wikimedia.org/wiki/File:2012-02-26_Corso_Amatrice.jpg); (B) Historical center on 29 August 2016, damaged by the M6 event of 24 August 2016 (Wikimedia Common, Diego Bianchi, licensed under CC-BY 2.0 and is available at: https://commons.wikimedia.org/wiki/File:Terremoto_centro_Italia_2016_-_Amatrice_-_corso_Umberto_I_(29242968591).jpg); (C) 5 story RC building, constructed following a low code, showing extensive damage photographed on August 31, 2017 (Mapillary, https://www.mapillary.com/, valentina_p, licensed under CC BY SA 4.0).
For Norcia, the M6 earthquake recordings of the sensors (OSS, Figure 2B) installed in the basement of the San Benedetto school building (e.g., Comodini et al., 2018; Falcone et al., 2021) were used to estimate the expected relative displacements (Table 3, maximum absolute values of the two horizontal components are reported) using the DARR method. The target area corresponds to the historical center, which according to Sorrentino et al. (2019) was mostly constituted by low-rise URM buildings (in total 95%): 74% of them had 2 stories, 15% 1 story and 11% 3 stories. Hence, the building typologies of 1–3 story URM buildings were analyzed in this study. Moreover, low and mid-rise RC buildings were studied.
TABLE 3. Damage assessment for different building typologies for Norcia (1-3 story URM and 3-5 story RC buildings).
The historical center is located in an intermountain sedimentary basin (e.g., Bindi et al., 2011; Luzi et al., 2019; Pagliaroli et al., 2020), i.e., on soft soils with potential occurrence of site effects. Thus, the fundamental periods T of the building typologies are calculated using the period-height relationships for URM and RC buildings on soft soil from Gallipoli et al. (2022). Since the school building was aligned the same way as most of the buildings in the historical center, the traces were not rotated.
Based on our results (Table 3, Figure 2F), non-structural, extensive and complete damage should be expected for 1, 2, and 3 story simple stone URM buildings, respectively. For 1–3 story regular URM buildings, non-structural damage should be expected. During the first event of the sequence, only a few buildings were damaged, most of them not structurally (Figure 2J and D’Ayala et al., 2019), but some of them also suffered structural damage. After the 1979 (Val Nerina) and 1997 (Umbria-Marche) events, most of the buildings in Norcia have been strengthened and retrofitted (Sisti et al., 2019; Sorrentino et al., 2019). Structural damage was only observed for a few masonry buildings with poor or no retrofitting. The URM buildings in the historical center of Norcia are assumed to behave as regular URM buildings to account for the retrofitting. In that case, only non-structural damage would be observed, in accordance with the few damage observed in the historical center of Norcia after the M6 event.
One example of the retrofitting of the buildings in Norcia is the 3 story RC school building, from which the recordings in the basement were used for the damage assessment. The building was designed in the 1960s and retrofitted in 2003 and 2011 (Comodini et al., 2018). The second retrofitting included the installation of dissipative braces on the 1st to 3rd floors. Figure 4 shows the simulated and observed relative displacements for this building for an M3.9 event occurring on 30 November 2013 at approximately 20 km distance and for the M6 event of 24 August 2016. The observed accelerations at the top and bottom of the building and the corresponding Fourier Spectra are presented in Supplementary Figure S1, S2. The building is simulated as an SDOF oscillator in both directions, with fundamental frequencies of 4.3 Hz (longitudinal direction X) and 4.6 Hz (transverse direction Y) and a damping ratio of 2% for small magnitude events. The values characterizing the SDOF oscillator (frequency and damping) have been estimated using a small magnitude event and tested for several small magnitude events, giving satisfactory results in all cases. As presented in Figure 4A, the simulated and observed maximum absolute relative displacements are similar for the M3.9 event for both directions. For the y direction, the relative displacement was almost precisely reconstructed. When considering the M6 event, probably due to the activation of the dissipators, the damping increases in the y direction. Therefore, a 10% damping ratio (Ferraioli and Lavino, 2019; Foti et al., 2020) is assumed for this direction. Although we do not precisely reconstruct the observed relative displacement at the top of the building, the simulated and observed maximum absolute relative displacements at the top of the building are similar. The simulated maximum absolute relative displacement was estimated as 1.7 cm. When considering the damage limits for a low-rise RC frame building constructed after a low or moderate code, non-structural or no damage should be expected, respectively. This is in accordance with the fact that only non-structural damage was observed.
FIGURE 4. Observed (blue) and simulated relative displacements (orange) at the top of the building for an M3.9 event of 30-11-2013 (A) and the M6 event of 24-08-2016 (B) for the two main directions of the building (X and Y).
For the Visso target area, the recordings in the basement of the Pietro Capuzi school building (OSS, Figure 2C) have been used to estimate the expected relative displacements (Table 4, maximum absolute values of the two horizontal components are reported) and the corresponding expected damage. The main axes of the school building were approximately oriented as the dominant building directions of the Visso town. The case study of the Visso school building has been studied in Petrovic et al. (2022) and, following the limits for 1–2 story simple stone URM buildings in Table 1, complete damage should be expected for the August 24 M6 event. After the event, moderate to severe damage has been observed (Brunelli et al., 2021). The Visso target area includes both the historical part (located in the southeast) and the central-western part of the town. The town of Visso is/was mainly composed of 2–4 story simple stone URM and 2–3 story regular URM buildings (Gaudiosi et al., 2016) constructed on soft soils (Brunelli et al., 2021). Thus, the T-h relationships for soft soils (Gallipoli et al., 2022) were used. Many buildings had been partially reconstructed or retrofitted after the 1997 Umbria-Marche sequence (Gaudiosi et al., 2016).
Following the considered damage limits (Table 1), non-structural damage should be expected for 2–4 story simple stone URM buildings (Table 4, Figure 2G). For 2–3 and 4 story regular URM buildings, no damage and non-structural damage should be expected, respectively. Our results are partially in accordance with the fact that damage has been observed only for a few buildings in the historical center (Gaudiosi et al., 2016).
However, the surveys performed after the 24 August event (Gaudiosi et al., 2016) show that both non-structural and structural damage occurred for many simple stone buildings outside the historical center (Figure 2K), including some partially reconstructed after the 1997 Umbria-Marche sequence (Gaudiosi et al., 2016). These differences might be due to a combination of factors including the variability of the dynamic behavior of buildings within the same typology and the occurrence of site effects, also suggested by Gaudiosi et al. (2016).
The recordings in the basement of the Orthopedic Surgery Pavilion of the Sulmona hospital (part of OSS, Figure 2D) have been used to calculate the expected relative displacements (Table 5, maximum absolute values of the two horizontal components are reported) in the target area of Sulmona (epicentral distance: approximately 90 km). The buildings in the historical center are mostly URM (simple stone) buildings constructed before 1800 (Munari et al., 2010). Thus, we considered here 2 and 3 story URM buildings for the historical city center, as well as low and mid-rise RC buildings for the recently constructed buildings. Sulmona was constructed on terraced fluvial and alluvial deposits (Di Giulio et al., 2015), therefore, the T-h relationships for soft soils (Gallipoli et al., 2022) were considered. The hospital building’s main directions are aligned with the dominant building directions in the town of Sulmona.
TABLE 5. Damage assessment for different building typologies for Sulmona (2-3 story URM and 3-5 story RC buildings).
No damage should be expected Figure 2H based on the obtained relative displacement (Table 5, Figure 2H) using the limits for different damage states (Table 1) for all building typologies. Our results are in accordance with the fact that no damage was reported in Sulmona after the M6 event.
In this study, we tested and verified the potential of the DARR method for providing local-scale information on structural damage for selected building typologies dominant in the studied areas. The estimation is based on a single recording in or close to be selected target area when the site conditions can be assumed homogeneous. Since only the recordings of one sensor are needed for each target area, it is a cost-effective and quick method for a rapid estimation of the expected damage both in single monitored buildings and target areas, and can support rapid response actions of the civil protection. If more than one recording is present for a considered target area, the choice should be made depending on the proximity of the recording and the similarity of geological conditions.
The DARR method relies on several assumptions discussed in Scaini et al. (2021) and Petrovic et al. (2022). There is a wide number of studies on the dynamic response of specific buildings to earthquakes (e.g., Rahmani and Todorovska, 2021) that allow identifying damage patterns in a precise way. The DARR method only accounts for the linear dynamic response of buildings, assuming that it can support the identification of structural damage. With this approximation, the precise reconstruction of the traces during the non-linear behavior is not possible, but the overstepping of the thresholds and the subsequent damage is successfully assessed (Scaini et al., 2021; Petrovic et al., 2022). For the purposes of rapid damage assessment, this approximation is satisfactory. DARR can be used for a large number of buildings with prior information (dominant building typologies, average building heights, period-height relationships, soil conditions) supporting rapid post-event damage assessment. Nonetheless, to correctly define the damage thresholds and to assess the expected damage, precise information on the building typologies (including construction age for building code identification) is needed. In particular, the use of period-height relationships requires information on the average building or story height, which might vary among the typologies, leading to different values of the fundamental frequency and thus, different relative displacement and expected damage. In case of scarce information on the building typologies, the identification of the damage thresholds and thus, the assessment of expected damage might be erroneous. In our study, we estimated the frequencies representative for the considered building typologies from period-height relationships from literature (Gallipoli et al., 2022). These relationships were developed from ambient vibration measurements in residential buildings in southern and northeastern Italy. There might be a variation in the frequencies of the building typologies of the studied area due to variations in the construction (e.g., materials, story heights etc.). Peak relative displacements increase drastically for mid to high-rise buildings with frequencies lower than 2 Hz (Norcia) and 5 Hz (Amatrice), as shown by the response spectra in Figure 5. Following the damage limits (Table 1), these buildings would suffer extensive or complete damage according to DARR.
FIGURE 5. Displacement Response Spectra for (A) Amatrice (B) Norcia (C) Visso and (D) Sulmona for the two main directions of the buildings (gray and black lines) in the considered towns.
The DARR method relies heavily on the choice of the relative displacement or interstory drift limits used for the damage assessment. Currently, the literature provides both interstory drift (e.g., Borzi et al., 2008; Shahzada et al., 2011; Chourasia et al., 2016; Minas and Galasso, 2019) and relative displacement limits (e.g., Lagomarsino and Giovinazzi, 2006; Frankie et al., 2013; Lestuzzi et al., 2016). Each set of limit values is derived based on different assumptions and for specific building typologies and study areas, and their use and validity depends on the typology dynamic behavior. DARR assumes that the relative displacement and interstory drift limits derived with different methods (e.g., numerical methods) are comparable with those found with the Z-transform for the purpose of a simplified damage assessment. However, further work is needed to validate the usability of the relative displacement and interstory drift limits derived with numerical methods. This would be strongly supported by the availability of more recordings both at the bottom and at the top of damaged buildings in correspondence of structural damage.
In this study, we considered different building typologies that are representative for the studied areas. The selection of damage thresholds for the analyzed building typologies is critical and further work is needed in order to estimate the expected behavior of specific building types (e.g., retrofitted URM buildings) and the influence of different soil conditions (e.g., Gallipoli et al., 2020). In addition, different damage state definitions are available from literature, based either on empirical damage identification (e.g., EMS-98, Grunthal, 1998) or limit states identified by numerical modeling (e.g., Borzi et al., 2008). In order to compare the outcomes of different damage assessment, a strong uniformation effort is required, as discussed by e.g., Rossetto and Elnashai (2003), Faravelli et al. (2019).
Amatrice and Norcia had a similar epicentral distance (9 and 15 km, respectively) and the ground motion recordings of the August event in Norcia and Amatrice are comparable in terms of PGA and PGD. However, during the 24 August 2016 M6 event, masonry buildings in Norcia successfully resisted a ground motion that might have led to a structural damage state (D4-D5) as documented in Amatrice (Figure 2I). The different damage patterns might be due to several factors including site effects, building typologies (e.g., retrofitting) and orientation, and the main direction of seismic wave propagation. In particular, DARR assumes that the ground motion in the target areas is homogeneous and can be represented by the recording. However, Figure 5 shows the different response spectra in Amatrice and Norcia, with large amplitudes at lower frequency related to local site effects. All these factors should be pondered when interpreting the recording from a single station and concur to identify the target area for applying DARR. For the Amatrice test site, the location of the recordings was outside the historical center (target area). Due to site effects, the relative displacement and thus, the expected damage might be slightly underestimated.
Apart from a number of studies on specific buildings (e.g., Ferraioli and Lavino, 2019; Gandelli et al., 2019; Foti et al., 2020), to our knowledge there are no studies that estimate the expected increase in relative displacement or interstory drift capacity for specific retrofitted building typologies. Additional studies on relative displacement or interstory drift limits for retrofitted building typologies might improve the results on the expected damage from the DARR method. In addition, no specific period-height relationships exist for retrofitted buildings which modify the stiffness of the buildings (e.g., Michel et al., 2018). Finally, retrofitting can influence the building’s damping and can be different in the two main building directions, as shown for the case of San Benedetto school in Norcia. Further research on the effect of retrofitting on the dynamic response of the considered building typologies would be desirable.
For the case study of Visso, non-structural damage should be expected for the August 24 event for 2–4 story simple stone URM buildings. The school building in Visso was a 2 story simple stone URM building and was damaged during this event. However, the school building has to be considered as a different building typology, with much higher floor heights and a more complex T shape. Following the T-h relationships from Gallipoli et al. (2022), for a 2 story building of 8 m height, we assume an average fundamental frequency of 7.35 Hz. In contrast, the school building was 13.5 m high and had a fundamental frequency of 3.18 Hz (Ferrero et al., 2020). For this reason, the expected relative displacement is much higher (3.5 cm) than the one estimated for a standard 2 story URM building, resulting in complete damage. The response spectra in Figure 5 shows that for Visso the expected relative displacement increases for fundamental frequencies below 4 Hz. In the study of the school building in Visso (Petrovic et al., 2022), the damping ratio has been estimated as 15%. In this study, the standard value of 5% (EC8, CEN 2004) was used, resulting in a slightly higher relative displacement.
DARR supports the combined use of both relative displacement and interstory drift limits which might be appropriate for different case-studies (e.g., high-rise flexible buildings). Further work is needed to explore how these limits work and their performance for different building types. The validation of DARR is, at the time, limited by the difficulties of having simultaneous availability of the required data which comprise earthquake recordings, buildings characteristics (type, material, age, height, fundamental frequencies and damping) and knowledge of the soil conditions. Future efforts will be devoted to testing the method in other areas where the information is available.
There are several studies on the effect of different ground motion parameters on the expected structural building damage (e.g., Ghimire et al., 2021 for RC buildings). The optimum criterion depends on the expected collapse mechanism (which varies between building typologies), and can be defined based on multiple indicators, including duration (Hancock and Bommer, 2006). This criterion would help the rapid identification of areas where structural damage is expected and prioritize interventions.
In this work, we present an application of the DARR method to the August 24 M6 event of the Central Italy seismic sequence (2016–2017). Expected damage is estimated in four selected towns in Central Italy (Amatrice, Norcia, Visso and Sulmona) where earthquake recordings were available. Results of the damage assessment, performed for dominant building typologies (URM and RC frames), were validated with post-event surveys. DARR successfully estimated expected damage for some building types as mid-rise URM (e.g. in the historical center of Amatrice) but failed to identify the damage occurred for low-rise URM buildings (e.g. in the town of Amatrice). The reasons for this are discussed in the article pointing out the aspects to be improved in future. Extensive and complete damage is obtained in accordance with the observed damage for 2 and 3 story simple stone URM buildings in Norcia and non-structural damage for regular URM buildings. It also correctly estimates the absence of structural damage in a target area located at a larger epicentral distance (Sulmona). Our results suggest that relative displacement limits are suitable for the damage assessment of low and mid rise building typologies considered in this work. DARR has the potential to provide a timely and cost-effective estimation of the expected damage, both for selected buildings and target areas, to support rapid response in the aftermath of a potentially destructive earthquake.
The datasets presented in the study are publicly available. All datasets presented/accessed in this study are cited in the article/Supplementary Material. Earthquake recordings were extracted from ITACA (https://itaca.mi.ingv.it/) and OSS (https://oss.protezionecivile.it/osspublic/#/).
BP: Conceptualization, data analysis and interpretation, Writing-original draft preparation; CS: Conceptualization, data collection and interpretation, Writing-original draft preparation; SP: Conceptualization, Writing-reviewing and editing.
Bojana Petrovic is supported by a research fellowship from the German Research Foundation (Deutsche Forschungsgemeinschaft, DFG, PE 2891/1-1, Projektnummer 428372009). This research was partially funded by the Autonomous Region Friuli Venezia Giulia, Veneto Region and the ARMONIA project (real-time acceleration network for monitoring sites and buildings in Italy and Austria, 2014–2020 INTERREG V-A Italy–Austria).
The DARR method was developed for the Friuli Venezia-Giulia region within the research activities supported by the Autonomous Region Friuli Venezia Giulia, the Veneto Region and the ARMONIA project (real-time acceleration network for monitoring sites and buildings in Italy and Austria, 2014–2020 INTERREG V-A Italy–Austria).
The authors declare that the research was conducted in the absence of any commercial or financial relationships that could be construed as a potential conflict of interest.
All claims expressed in this article are solely those of the authors and do not necessarily represent those of their affiliated organizations, or those of the publisher, the editors and the reviewers. Any product that may be evaluated in this article, or claim that may be made by its manufacturer, is not guaranteed or endorsed by the publisher.
The Supplementary Material for this article can be found online at: https://www.frontiersin.org/articles/10.3389/feart.2022.932110/full#supplementary-material
Appolloni, L., and D’Alessandro, D. (2021). Housing spaces in nine European countries: A comparison of dimensional requirements. Int. J. Environ. Res. Public Health 18, 4278. doi:10.3390/Ijerph18084278
Bard, P. Y., Gueguen, G., and Wirgin, A. (1996). “A note on the seismic wavefield radiated from large building structures into soft soils,” in Proceedings of 11th World Conference on Earthquake Engineering.
Bindi, D., Luzi, L., Parolai, S., Di Giacomo, D., and Monachesi, G. (2011). Site effects observed in alluvial basins: The case of Norcia (central Italy). Bull. Earthq. Eng. 9, 1941–1959. doi:10.1007/s10518-011-9273-3
Borzi, B., Crowley, H., and Pinho, R. (2008). Simplified pushover-based earthquake loss assessment ( SP- BELA ) method for masonry buildings. Int. J. Archit. Herit. 2, 353–376. doi:10.1080/15583050701828178
Bragato, P. L., Comelli, P., Saraò, A., Zuliani, D., Moratto, L., Poggi, V., et al. (2021). The OGS-Northeastern Italy seismic and deformation network: Current status and outlook. Seismol. Res. Lett. 92 (3), 1704–1716. doi:10.1785/0220200372
Brunelli, A., de Silva, F., Piro, A., Parisi, F., Sica, S., Silvestri, F., et al. (2021). Numerical simulation of the seismic response and soil–structure interaction for a monitored masonry school building damaged by the 2016 Central Italy earthquake. Bull Earthquake Eng. 19, 1181–1211. doi:10.1007/s10518-020-00980-3
CEN (2004). European standard EN 1998-1: 2004—eurocode 8: Design of structures for earthquake resistance. Part 1: General rules, seismic action and rules for buildings. Brussels, Belgium: European Committee for Standardization.
Chiaraluce, L., Di Stefano, R., Tinti, E., Scognamiglio, L., Michele, M., Casarotti, E., et al. (2017). The 2016 central Italy seismic sequence: A first look at the mainshocks, aftershocks, and source models. Seismol. Res. Lett. 88 (3), 757–771. doi:10.1785/0220160221
Chieffo, N., Formisano, A., and Ferreira, T. M. (2019). Damage scenario-based approach and retrofitting strategies for seismic risk mitigation: An application to the historical centre of Sant’Antimo (Italy). Eur. J. Environ. Civ. Eng. 25, 1929–1948. doi:10.1080/19648189.2019.1596164
Chourasia, A., Bhattacharyya, S. K., Bhandari, N. M., and Bhargava, P. (2016). Seismic performance of different masonry buildings: Full-scale experimental study. J. Perform. Constr. Facil. 30–5. doi:10.1061/(ASCE)CF.1943-5509.0000850
Comodini, F., Fulco, A., and Mezzi, M. (2018). Performance of A building with dissipative bracing system under strong earthquakes. Thessaloniki: 16th European conference on earthquake engineering, 18–21 June 2018.
Crowley, H., and Pinho, R. (2010). Revisiting Eurocode 8 formulae for periods of vibration and their employment in linear seismic analysis. Earthq. Eng. Struct. Dyn. 39 (2), 223–235. doi:10.1002/eqe.949
D’Ayala, D., Faure-Walker, J., Mildon, Z., Lombardi, D., Galasso, C., Pedicone, D., et al. (2019). The M W 6.2 Amatrice. Italy Earthquake Of 24th August 2016 - A Field Report By Eefit, ©EEFIT. London: EEFIT.
Di Giulio, G., De Nardis, R., Boncio, P., Milana, G., Rosatelli, G., Stoppa, F., et al. (2015). Seismic response of a deep continental basin including velocity inversion: The Sulmona intramontane basin (central apennines, Italy). Geophys. J. Int. 204, 418–439. doi:10.1093/gji/ggv444
Dolce, M., Nicoletti, M., De Sortis, A., Marchesini, S., Spina, D., and Talanas, F. (2017). Osservatorio sismico delle strutture: The Italian structural seismic monitoring network. Bull. Earthq. Eng. 15, 621–641. doi:10.1007/s10518-015-9738-x
Donà, M., Carpanese, P., Follador, V., Sbrogiò, L., and Da Porto, F. (2020). Mechanics-based fragility curves for Italian residential URM buildings. Bull. Earthq. Eng. 19, 3099–3127. doi:10.1007/s10518-020-00928-7
Espinosa-Aranda, J. M., Cuellar, A., Garcia, A., Ibarrola, G., Islas, R., Maldonado, S., et al. (2009). Evolution of the Mexican seismic alert system (SASMEX), seismol. Res. Lett. 80 (5), 694–706. doi:10.1785/gssrl.80.5.694
Falcone, G., Vacca, V., Mori, F., Naso, G., and Spina, D. (2021). Evaluation of building seismic retrofitting costs founded on experimental data. The case study of “San Benedetto” School (Norcia, Italy). Italian J. Geosciences 140 (3), 365–381. doi:10.3301/ijg.2021.09
Faravelli, M., Borzi, B., Polli, D., and Pagano, M. (2019). Calibration of a mechanics-based method for large-scale vulnerability assessment. Bull. Earthq. Eng. 17, 2485–2508. doi:10.1007/s10518-019-00560-0
Ferraioli, M., and Lavino, A. (2019). A displacement-based design method for seismic retrofit of RC buildings using dissipative braces. Math. Probl. Eng., 1–28. doi:10.1155/2018/5364564
Ferrero, C., Lourenco, P. B., and Calderini, C. (2020). Nonlinear modeling of unreinforced masonry structures under seismic actions: Validation using a building hit by the 2016 central Italy earthquake. Bull. Earthq. Eng. 14 (51), 92–114. doi:10.3221/IGF-ESIS.51.08
Fiorentino, G., Forte, A., Pagano, E., Sabetta, F., Baggio, C., Lavorato, D., et al. (2018). Damage patterns in the town of Amatrice after August 24th 2016 central Italy earthquakes. Bull. Earthq. Eng. 16, 1399–1423. doi:10.1007/s10518-017-0254-z
Foti, D., Ruggiero, F., Sabbà, M. F., and Lerna, M. (2020). A dissipating frames for seismic retrofitting and building energy-efficiency. Infrastructures 5 (9), 74. doi:10.3390/infrastructures5090074
Frankie, T. M., Gencturk, B., and Elnashai, A. S. (2013). Simulation-based fragility relationships for unreinforced masonry buildings. J. Struct. Eng. (U. S.) 139, 400–410. doi:10.1061/(ASCE)ST.1943-541X.0000648
Gallipoli, M. R., Calamita, G., Tragni, N., Pisapia, D., Lupo, M., Mucciarelli, M., et al. (2020). Evaluation of soil-building resonance effect in the urban area of the city of Matera (Italy). Eng. Geol. 272, 105645. doi:10.1016/j.eng-geo.2020.105645
Gallipoli, M. R., Mucciarelli, M., and Vona, M. (2009). Empirical estimate of fundamental frequencies and damping for Italian buildings. Earthq. Eng. Struct. Dyn. 38, 973–988. doi:10.1002/eqe.878
Gallipoli, M. R., Petrovic, B., Scaini, C., Calamita, G., Tragni, N., Barnaba, C., et al. (2022). FRIBAS database for better characterization of RC and URM buildings: Towards specific T-H relationships. (submitted to Bulletin of Earthquake Engineering, under review).
Gandelli, E., Taras, A., Distl, J., and Quaglini, V. (2019). Seismic retrofit of hospitals by means of hysteretic braces: Influence on acceleration-sensitive non-structural components. Front. Built Environ. 5 (100), 1–13. doi:10.3389/fbuil.2019.00100
Gaudiosi, I., Vignaroli, G., Sirianni, P., Giallini, S., Mori, F., Polpetta, F., et al. (2016). Local seismic response studies in the north-western portion of the August 24th, 2016 Mw 6.0 earthquake affected area. The case of Visso village (central Apennines). Ann. Geophys. 59. doi:10.4401/ag-7258
Gaudiosi, I., Simionato, M., Mancini, M., Cavinato, G. P., Coltella, M., Razzano, R., et al. (2021). Evaluation of site effects at Amatrice (central Italy) after the August 24th, 2016, mw 6.0 earthquake. Soil Dyn. Earthq. Eng. 144, 106699. doi:10.1016/j.soildyn.2021.106699
Ghimire, S., Guéguen, P., and Astorga, A. (2021). Analysis of the efficiency of intensity measures from real earthquake data recorded in buildings. Soil Dyn. Earthq. Eng. 147, 106751. doi:10.1016/j.soildyn.2021.106751
Goel, R. K., and Chopra, A. K. (1997). Period formulas for moment-resisting frame buildings. J. Struct. Eng. (N. Y. N. Y). 123, 1454–1461. doi:10.1061/(asce)0733-9445(1997)123:11(1454)
Gorini, A., Nicoletti, M., Marsan, P., Bianconi, R., De Nardis, R., Filippi, L., et al. (2010). The Italian strong motion network. Bull. Earthq. Eng. 8, 1075–1090. doi:10.1007/s10518-009-9141-6
Grünthal., G. (1998). “European macroseismic scale 1998 (EMS-98),” in European seismological commission, subcommission on engineering seismology, working group macroseismic scales (Luxembourg: Conseil de l'Europe, Cahiers du Centre Européen de Géodynamique et de Séismologie), 15.
Hancock, J., and Bommer, J. J. (2006). A state-of-knowledge review of the influence of strong-motion duration on structural damage. Earthq. Spectra 22 (3), 827–845. doi:10.1193/1.2220576
Jin, X., Ma, Q., and Li, S. (2004). Comparison of four numerical methods for calculating seismic dynamic response of SDOF systems, 13th World Conf. on Earthquake Engineering. Canada: Vancouver. 1–6 August 2004Paper Number 2889.
Lagomarsino, S., and Giovinazzi, S. (2006). Macroseismic and mechanical models for the vulnerability and damage assessment of current buildings. Bull. Earthq. Eng. 4, 415–443. doi:10.1007/s10518-006-9024-z
Lee, V. W. (1990). Efficient algorithm for computing displacement, velocity and acceleration responses of an oscillator to arbitrary ground motion. Soil Dyn. Earthq. Eng. l9 (6), 288–300. doi:10.1016/s0267-7261(05)80015-6
Lestuzzi, P., Podestà, S., Luchini, C., Garofano, A., Kazantzidou-Firtinidou, D., Bozzano, C., et al. (2016). Seismic vulnerability assessment at urban scale for two typical Swiss cities using Risk-UE methodology. Nat. Hazards (Dordr). 84, 249–269. doi:10.1007/s11069-016-2420-z
Luzi, L., D’Amico, M., Massa, M., and Puglia, R. (2019). Site effects observed in the Norcia intermountain basin (Central Italy) exploiting a 20-year monitoring. Bull. Earthq. Eng. 17, 97–118. doi:10.1007/s10518-018-0444-3
Luzi, L., Pacor, F., Puglia, R., Lanzano, G., Felicetta, C., D’Amico, M., et al. (2016). The central Italy seismic sequence between August and december 2016: Analysis of strong‐motion observations. Seismol. Res. Lett. 88 (5), 1219–1231. doi:10.1785/0220170037
Masi, A., Chiauzzi, L., Santarsiero, G., Manfredi, V., Biondi, S., Spacone, E., et al. (2019). Seismic response of RC buildings during the Mw 6.0 August 24, 2016 Central Italy earthquake: The Amatrice case study. Bull. Earthq. Eng. 17, 5631–5654. doi:10.1007/s10518-017-0277-5
Meletti, C., Montaldo, V., Stucchi, M., and Martinelli, F. (2006). Database della pericolosità sismica MPS04. Rome: Istituto Nazionale di Geofisica e Vulcanologia (INGV). doi:10.13127/sh/mps04/db
Michel, C., Gueguen, P., Lestuzzi, P., and Bard, P.-Y. (2010). Comparison between seismic vulnerability models and experimental dynamic properties of existing buildings in France. Bull. Earthq. Eng. 8, 1295–1307. doi:10.1007/s10518-010-9185-7
Michel, C., Karbassi, A., and Lestuzzi, P. (2018). Evaluation of the seismic retrofitting of an unreinforced masonry building using numerical modeling and ambient vibration measurements. Eng. Struct. 158, 124–135. doi:10.1016/j.engstruct.2017.12.016
Milana, G., Cultrera, G., Bordoni, P., Bucci, A., Cara, F., Cogliano, R., et al. (2018). Local site effects estimation at Amatrice (Central Italy) through seismological methods. Bull. Earthq. Eng. 18, 5713–5739. doi:10.1007/s10518-019-00587-3
Minas, S., and Galasso, C. (2019). Accounting for spectral shape in simplified fragility analysis of case-study reinforced concrete frames. Soil Dyn. Earthq. Eng. 119, 91–103. doi:10.1016/j.soildyn.2018.12.025
Mori, J., Kanamori, H., Davis, J., Hauksson, E., Clayton, E., Heaton, T., et al. (1998). Major improvements in progress for Southern California earthquake monitoring. Eos Trans. AGU. 79, 217–221. doi:10.1029/98eo00157
Mucciarelli, M., Masi, A., Gallipoli, M. R., Harabaglia, P., Vona, M., Ponzo, F., et al. (2004). Analysis of RC building dynamic response and soil-building resonance based on data recorded during a damaging earthquake (Molise, Italy, 2002). Bull. Seismol. Soc. Am. 94, 1943–1953. doi:10.1785/012003186
Munari, M., Valluzzi, M. R., Cardani, G., Anzani, A., Binda, L., and Modena, C. (2010). “Seismic vulnerability analyses of masonry aggregate buildings in the historical centre of Sulmona (Italy),” in Proceeding 13th International Conference on Structural Faults and Repair (Edinburgh), 1–10.
Okada, Y., Kasahara, K., Hori, S., Obara, K., Sekiguchi, S., Fujiwara, H., et al. (2004). Recent progress of seismic observation networks in Japan hi-net, F-net, K-net and KiK-net. Earth Planets Space 56, xv. doi:10.1186/BF03353076
OpenStreetMap contributors (2015). OpenStreetMap contributors. Available at: https://planet.openstreetmap.org.
Pagliaroli, A., Pergalani, F., Ciancimino, A., Chiaradonna, A., Compagnoni, M., de Silva, F., et al. (2020). Site response analyses for complex geological and morphological conditions: Relevant case-histories from 3rd level seismic microzonation in central Italy. Bull. Earthq. Eng. 18, 5741–5777. doi:10.1007/s10518-019-00610-7
Parolai, S., Bindi, D., Boxberger, T., Milkereit, C., Fleming, K., and Pittore, M. (2015). On-site early warning and rapid damage forecasting using single stations: Outcomes from the REAKT project. Seismol. Res. Lett. 86 (5), 1393–1404. doi:10.1785/0220140205
Parolai, S., Boxberger, T., Pilz, M., Fleming, K., Haas, M., Pittore, M., et al. (2017). Assessing earthquake early warning using sparse networks in developing countries: Case study of the Kyrgyz Republic. Front. Earth Sci. 5. doi:10.3389/feart.2017.00074
Petrovic, B., Scaini, C., and Parolai, S. (2022). Damage assessment for rapid response (DARR): Validation for different ground motion levels and building types. (submitted to SRL, under review).
Poggi, V., Scaini, C., Moratto, L., Peressi, G., Comelli, P., Bragato, P. L., et al. (2020). Rapid damage scenario assessment for earthquake emergency management. Seismol. Res. Lett. 92, 2513–2530. doi:10.1785/0220200245
Rahmani, M., Ebrahimian, M., and Todorovska, M. I. (2015). Time-wave velocity analysis for early earthquake damage detection in buildings: Application to a damaged full-scale RC building. Earthq. Eng. Struct. Dyn. 44 (4), 619–636. doi:10.1002/eqe.2539
Rahmani, M., and Todorovska, M. I., (2021). Structural health monitoring of a 32-storey steel-frame building using 50 years of seismic monitoring data, Earthq. Eng. Struct. Dyn. 50, 1777–1800. doi:10.1002/eqe.3422
Rossetto, T., and Elnashai, A. (2003). Derivation of vulnerability functions for European-type RC structures based on observational data. Eng. Struct. 25, 1241–1263. doi:10.1016/s0141-0296(03)00060-9
Rossetto, T., Gehl, P., Minas, S., Galasso, C., Duffour, P., Douglas, J., et al. (2016). Fracas: A capacity spectrum approach for seismic fragility assessment including record-to-record variability. Eng. Struct. 125, 337–348. doi:10.1016/j.engstruct.2016.06.043
Rossi, A., Tertulliani, A., Azzaro, R., Graziani, L., Rovida, A., Maramai, A., et al. (2018). The 2016–2017 earthquake sequence in central Italy: Macroseismic survey and damage scenario through the EMS-98 intensity assessment. Bull. Earthq. Eng. 17, 2407–2431. doi:10.1007/s10518-019-00556-w
Rota, M., Penna, A., and Magenes, G. (2008). “A procedure for deriving analytical fragility curves for masonry buildings,” in Proceeding 14th World Conference on Earthquake Engineering, Beijing, China, October 12–17, 2008.
Russo, E., Felicetta, C., D’Amico, M. C., Sgobba, S., Lanzano, G., Mascandola, C., et al. (2022). Italian Accelerometric Archive v3.2 - Istituto Nazionale di Geofisica e Vulcanologia, Dipartimento della Protezione Civile Nazionale, Roma. doi:10.13127/itaca.3.2
Satriano, C., Elia, L., Martino, C., Lancieri, M., Zollo, A., and Iannaccone, G. (2011). PRESTo, the earthquake early warning system for Southern Italy: Concepts, capabilities and future perspectives. Soil Dyn. Earthq. Eng. 31, 137–153. doi:10.1016/j.soildyn.2010.06.008
Scaini, C., Petrovic, B., Tamaro, A., Moratto, L., and Parolai, S. (2021). Near-real-time damage estimation for buildings based on strong-motion recordings: An application to target areas in northeastern Italy. Seismol. Res. Lett. 92 (6), 3785–3800. doi:10.1785/0220200430
Shahzada, K., Gencturk, B., Khan, A. N., Naseer, A., Javed, M., and Fahad, M. (2011). Vulnerability assessment of typical buildings in Pakistan. Int. J. Earth Sci-ences Eng. 4 (6), 208–211.
Sisti, R., Di Ludovico, M., Borri, A., and Prota, A. (2019). Damage assessment and the effectiveness of prevention: The response of ordinary unreinforced masonry buildings in Norcia during the central Italy 2016–2017 seismic sequence. Bull. Earthq. Eng. 17, 5609–5629. doi:10.1007/s10518-018-0448-z
So, E., and Spence, R. (2013). Estimating shaking-induced casualties and building damage for global earthquake events: A proposed modelling approach. Bull. Earthq. Eng. 11, 347–363. doi:10.1007/s10518-012-9373-8
Sorrentino, L., Cattari, S., Da Porto, F., Magenes, G., and Penna, A. (2019). Seismic behaviour of ordinary masonry buildings during the 2016 central Italy earthquakes. Bull. Earthq. Eng. 17, 5583–5607. doi:10.1007/s10518-018-0370-4
Spina, D., Acunzo, G., Fiorini, N., Mori, F., and Dolce, M. (2010). A probabilistic simplified seismic model of masonry buildings based on ambient vibrations. Bull. Earthq. Eng. 17, 985–1007. doi:10.1007/s10518-018-0481-y
Stewart, J. P., and Lanzo, G. (2018). “Engineering reconnaissance following the october 2016 central Italy earthquakes, GEER (geotechnical extreme events reconnaissance association), report No. GEER-0500,”Editors P. Zimmaro, and J. P. Stewart. doi:10.18118/G6HS39
Stucchi, M., Meletti, C., and Montaldo, V. (2011). Seismic hazard assessment (2003-2009) for the Italian building code. Bull. Seismol. Soc. Am. 101 (4), 1885–1911. doi:10.1785/0120100130
Tinti, E., Scognamiglio, L., Michelini, A., and Cocco, M. (2016). Slip heterogeneity and directivity of theML6.0, 2016, Amatrice earthquake estimated with rapid finite-fault inversion. Geophys. Res. Lett. 43, 10745–10752. doi:10.1002/2016gl071263
Todrani, A., and Cultrera, G. (2021). Near-source simulation of strong ground motion in Amatrice downtown including site effects. Geosciences 11, 186. doi:10.3390/geosciences11050186
Trifunac, M. D., Hao, T. Y., and Todorovska, M. I. (2001). Response of A 14-story reinforced concrete structure to nine earthquakes: 61 Years of observation in the hollywood storage building. Available at: www.usc.edu/dept/civil_eng/Earthquake_eng/.
Vignaroli, G., Mancini, M., Bucci, F., Cardinali, M., Cavinato, G. P., Moscatelli, M., et al. (2019). Geology of the central part of the Amatrice basin (central apennines, Italy). J. Maps 15, 193–202. doi:10.1080/17445647.2019.1570877
Keywords: seismic damage assessment, rapid response, DARR method, seismic building monitoring, Central Italy 2016 earthquake sequence, simplified building models, dynamic behavior of buildings, seismic retrofitting
Citation: Petrovic B, Scaini C and Parolai S (2022) Applying the damage assessment for rapid response approach to the august 24 M6 event of the seismic sequence in central Italy (2016). Front. Earth Sci. 10:932110. doi: 10.3389/feart.2022.932110
Received: 29 April 2022; Accepted: 01 August 2022;
Published: 11 October 2022.
Edited by:
Matteo Picozzi, University of Naples Federico II, ItalyReviewed by:
Stefania Tarantino, Istituto Nazionale di Geofisica e Vulcanologia, ItalyCopyright © 2022 Petrovic, Scaini and Parolai. This is an open-access article distributed under the terms of the Creative Commons Attribution License (CC BY). The use, distribution or reproduction in other forums is permitted, provided the original author(s) and the copyright owner(s) are credited and that the original publication in this journal is cited, in accordance with accepted academic practice. No use, distribution or reproduction is permitted which does not comply with these terms.
*Correspondence: Bojana Petrovic, YnBldHJvdmljQG9ncy5pdA==
Disclaimer: All claims expressed in this article are solely those of the authors and do not necessarily represent those of their affiliated organizations, or those of the publisher, the editors and the reviewers. Any product that may be evaluated in this article or claim that may be made by its manufacturer is not guaranteed or endorsed by the publisher.
Research integrity at Frontiers
Learn more about the work of our research integrity team to safeguard the quality of each article we publish.