- 1State Key Laboratory of Petroleum Resources and Prospecting, China University of Petroleum, Beijing, China
- 2College of Geosciences, China University of Petroleum, Beijing, China
- 3Southwest Oil and Gas Field Company PetroChina, Chengdu, China
The evolution mechanism of hydrocarbons in ultra-deeply-buried and ultra-old strata is the core issue of petroleum geology moving towards Deep Earth. Reconstructing the evolutionary history of ultra-deeply-buried hydrocarbons is the key to shedding light on deep hydrocarbon accumulation and evolution mechanisms. Furthermore, it can help point out directions for oil and gas exploration in Deep Earth. Anyue gas field in the central Sichuan Basin is the Frontier of deep natural gas exploration in China. This study selected the natural gas reservoirs of the Upper Sinian Dengying Formation in the central Sichuan Basin as the research object. By integrating analysis of natural gas geochemical characteristics, source rock evaluation, reservoir bitumen-source correlation, inclusion analysis, one-dimensional and two-dimensional hydrocarbon accumulation simulations, the generation and evolution of hydrocarbons in different structural regions, namely the inherited paleo-uplift and slope area in central Sichuan Basin, have been dynamically restored and compared. The results show that: 1) The natural gas of the ultra-deeply-buried Sinian Dengying formation in central Sichuan is typical oil-cracking gas from the paleo-oil reservoir. The Sinian gas is mainly sourced from the Qiongzhusi/Maidiping Formation. 2) The formation of Sinian gas reservoirs includes three stages: the formation of paleo-oil-reservoirs; the cracking of paleo-oil-reservoirs into paleo-gas-reservoirs; the adjustment of the paleo-gas-reservoirs. 3) Source rock and reservoir evaluation, quantitative solid bitumen analysis, and hydrocarbon accumulation simulation show that the natural gas accumulation conditions in the slope area are better than in the inherited uplift area. The scale of the paleo-oil-reservoirs in the slope area may be greater than that in the inherited uplift area.
Introduction
According to China Drilling Regulations, the deeply-buried strata refers to strata with a burial depth of 4,500–6,000 m, while the ultra-deeply-buried refers to a depth greater than 6,000 m (Zhao et al., 2014; Cao et al., 2022). China has made significant breakthroughs in deep and ultra-deep hydrocarbon exploration in recent years. By the end of 2019, PetroChina’s proven deep and ultra-deep natural gas geological reserves had reached 2.86 trillion cubic meters; however, the proven resource rate only reached 13%, indicating an overall low exploration degree (Li et al., 2020). Deep and ultra-deep marine oil and gas exploration has become the Frontier of China’s oil and gas exploration.
The classical kerogen thermal-degraded theory proposed by (Tissot and Welte, 1978) postulates that oil generation from sedimentary organic matter mainly occurs in the so-called “oil window” with a formation temperature of no more than 160°C. Thus, it is generally accepted that oil can not stably exist in deep strata. However, in recent years, China has found many industrial oil reservoirs in ultra-deeply-buried strata (Zhu G. et al., 2017b; Zhu et al., 2021), dramatically challenging Tissot’s theory. Therefore, the phase evolution of hydrocarbon in deeply-buried and ultra-deeply-buried strata has become the research hotspots of petroleum geology.
China’s deep, ultra-deep oil and gas exploration mainly concentrates on the Tarim and Sichuan Basins. As typical superimposed basins, the ultra-deeply-buried strata of the Tarim and Sichuan Basins have a complex thermal and tectonic evolutionary history, and the research on the hydrocarbon phase evolution and accumulation process is still not in-depth (Ren et al., 2020). In sharp contrast to the Tarim Basin, natural gas reservoirs dominate deeply-buried and ultra-deeply-buried strata of the central Sichuan Basin. The Sinian Dengying and Cambrian Longwangmiao Formations are the main exploration targets of the Anyue gas field in the central Sichuan Basin. By the end of 2020, the proven geological reserves of natural gas in the Anyue gas field (the Gaoshiti-Moxi area) have exceeded 1 × 1012 m3, with an annual production capacity of about 170 × 108 m3 (Wei et al., 2022). Gas reservoirs of the Dengying and Longwangmiao Formations experienced multi-stage tectonic movements during their long evolutionary history and are characterized by considerable burial depth (more than 4,500 m), high evolutionary degree (typical dry gas) (Dai et al., 2018), oil-cracking origin (Wei et al., 2015a; Wu et al., 2016a). A typical evolutionary mode of hydrocarbon in the deep and ultra-deeply-buried strata of the central Sichuan Basin is: paleo-oil-reservoir → paleo-gas-reservoir → today’s gas reservoir (Zhu et al., 2015). However, it is worth noting that this mode was established primarily based on fluid inclusion analysis (Yang et al., 2022). The detailed evolution process of the hydrocarbon phase and its main controlling factors remain unclear (Li et al., 2021). Therefore, in recent years, the deep gas research in the Sichuan Basin has been greatly concentrated on reconstructing the hydrocarbon accumulation history and clarifying its phase evolution mechanism.
From the exploration’s perspective, the PetroChina Southwest Oil and Gas Field Company has made significant exploration breakthroughs on the North Slope of the Anyue Gas Field during the past several years. The exploration wells PT1 and JT1 obtained industrial gas flow in the second and the fourth members of the Dengying Formation, respectively (Xu et al., 2020). The exploration breakthroughs of the North Slope have aroused some scientific questions. 1) Tectonic simulation shows that after the oil generation peak, the Anyue gas area was inherited in the high-part of paleo-uplift, while the North Slope gradually evolved into a structural slope area (Ma et al., 2020). For the slope area, the formation of “traps” is crucial to the large-scale hydrocarbon accumulation. Have some structural traps favorable for oil and gas accumulation ever been formed in the slope area during the process of hydrocarbon accumulation and evolution? 2) (Xie et al., 2021a) found that the gas isotopic characteristics in the slope area are different from that in the uplift area, and he speculated that it was partly due to different thermal maturity. However, it lacks evidence from the thermal simulation of source rocks in the slope and inherited uplift areas. 3) How the matching between tectonic evolution and thermal evolution of source rocks controls the hydrocarbon accumulation in the slope area and in the uplift area? 4) Can the hydrocarbon evolution and accumulation processes in the slope and uplift regions be compared under an entirely consistent thermal background? The key to solving these questions is simultaneously reconstructing the two regions’ hydrocarbon generation, accumulation, and evolution history.
In this study, based on the geochemical analysis of natural gas, source rocks and reservoir solid bitumen, gas/bitumen-source correlation, reservoir characterization, one-dimensional and two-dimensional simulations of hydrocarbon accumulation, we completed the restoration of hydrocarbon generation, accumulation, and evolution simulation on the North Slope and the Gaoshiti-Moxi area simultaneously, so as to dynamically compare the evolution process of hydrocarbon in different structural positions for ultra-deep marine carbonate reservoirs.
Geological background
Sichuan Basin contains six first-ordered tectonic units (Figure 1A), and the research area (the North Slope of central Sichuan Basin) is located in the Central Gentle Tectonic Belt. The Gaoshiti-Moxi area is a large-scaled uplift shaped in the Caledonian movement at the end of Silurian and preserved to date (Zou et al., 2014; Wei et al., 2015c). The North Slope mainly refers to the large-scaled monoclinic structure adjacent to the north of the Gaoshiti-Moxi area. The north side of the North Slope is bounded by Jiulong Mountain (longti1 well location), and its west side is close to the Deyang-Anyue rift trough (Figure 1A). Like the Gaoshiti-Moxi area, the North Slope used to be part of the paleo-central-Sichuan-uplift formed in the Caledonian movement and preserved by the Indosinian movement. Subsidence of the North Slope began in the Indosinian movement and gradually transformed into a sloped shape (Ma et al., 2019). Therefore, the Gaoshiti-Moxi area is also called the inherited uplift, while the North Slope is the non-inherited structural area (Yang et al., 2020).
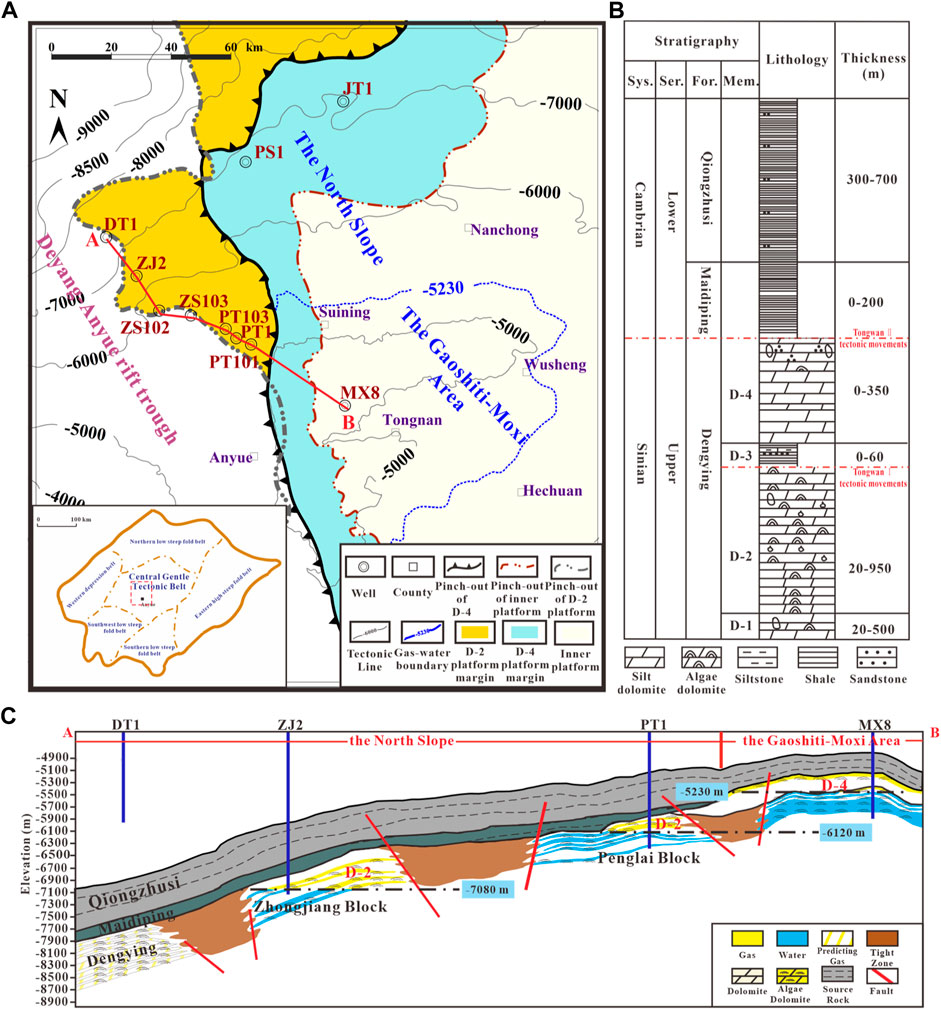
FIGURE 1. An overview of the geologic background of the research area. (A) The sedimentary facies of the Dengying Formation overlaying on the structural map of the Dengying top surface. (B) Stratigraphic column of the Dengying Formation and its source rocks. (C) Typical gas reservoir profiles showing the gas-water relationships in the Gaoshiti-Moxi area (D-4), the North Slope (the Zhongjiang (D-2), and the Penglai Blocks (D-2)).
The Upper Sinian Dengying Formation is one of the main targets of the Anyue Gas Field. It is deeply buried, with the deepest elevation reaching -8,300 m. Four members make up the Dengying Formation, the second and fourth members of which are high-quality reservoirs (Figure 1B). While well maintained in the Gaoshiti-Moxi area, the Dengying Formation is severely eroded in the North Slope. Only the Dengying Formation’s first and second members are unconformably in contact with overlying strata (Figure 1B). Thus, the Dengying Formation is distributed in steps from the North Slope to Gaoshiti-Moxi area. The platform margin reef-flat facies dominate the high-quality reservoir (Feng et al., 2021). The sedimentary facies change gradually from west to east to inner platform facies. The reservoir rock is primarily microbial dolomite.
The natural gas in Dengying Formation is mainly sourced from the overlying black shale of the Cambrian Qiongzhusi and Maidiping Formations, with a small contribution from the third member of the Dengying Formation (Wei et al., 2022). The thickness of the Qiongzhusi and Maidiping Formations is thinner from the North Slope to the Gaoshiti-Moxi area, and the Maidiping Formations are barely deposited in the Gaoshiti-Moxi area.
A typical gas reservoir profile (Figure 1C) shows that the Gaoshiti-Moxi area is dominated by anticlinal structural gas reservoirs with a uniform gas-water contact (−5230 m). In contrast, the gas-water relationship in the North Slope is more complicated, dominated by stratigraphic-lithologic gas reservoirs (Zhao et al., 2020). As shown in Figure 1, the gas-water contact in the Zhongjiang Block (ZJ2) and the Penglai Block (PT1) are different, showing that they are different gas reservoirs.
Sampling and experiments
In this study, to dynamically reconstruct the hydrocarbon generation, accumulation, and evolution history in ultra-deeply-buried strata, natural gas analysis, source rock analysis, and fluid inclusion analysis were performed in the Analysis Experiment Center of the Exploration and Development Research Institute of PetroChina Southwest Oil and Gas Field Company. The gas samples are obtained from the main exploration wells. Also, we calculated the content of solid bitumen of the Dengying reservoir rocks with the help of the casting thin section images.
Gas analysis
First, the gas components were analyzed utilizing Agilent 6890 gas chromatography (GC–IRMS). After that, the carbon isotope experiment on methane and ethane was carried out following the standard SY/T 5238-2019 by using Isochrom II Stable isotope ratio mass spectrometer at 23°C and 60% RH. The carbon isotope (δ13C) is reported as per mil (‰) deviations from the carbon isotope composition of the VPDB (Vienna Pee Dee Belemnite) standard. Similarly, the deuterium isotope was also analyzed for the methane and ethane according to standard SY/T 5238-2019.
Geochemical analysis of source rock
The pyrolysis experiment and TOC analysis were conducted on the Qiongzhusi source rocks (Є1q) formations utilizing Rock-Eval and C/S analyzer to clarify the quality of the primary source rocks.
Fluid inclusion
The fluid inclusion homogeneous temperature test was conducted in the Analytical Laboratory of Beijing Research Institute of Uranium Geology via LINKAM THMS600 (7035) heating and cooling stage, following standard EJ/T1105-1999 (Determination of temperature for fluid inclusion in minerals). Also, the features of fluid inclusions, like distribution, size, and liquid-to-vapor ratio, were described.
The volumetric content of solid bitumen
Core samples of the D-2 and D-4 members were taken from exploration wells to calculate the volumetric solid bitumen content in the reservoir rock. According to standard SY/T 5368–2016, casting thin sections specimens were made, and the pore structure was observed using a Transmission reflection polarizing microscope. After that, the volumetric content of reservoir solid bitumen was calculated by image processing through the following steps:
a. For every depth with casting thin section specimen, we selected five images for quantitative analysis of solid bitumen. b. We accurately identified and extracted the solid bitumen through color mode conversion (from RGB to YCbCr), K-means clustering, and binarization. c. The plane porosity of the solid bitumen was calculated on the binarized image, and the average plane porosity of the five selected images was taken as the volumetric content of solid bitumen in this depth.
Results and discussion
Natural gas characterization
The natural gas component analysis on 61 gas sample from key exploration wells shows that: ① the CH4 content of gas reservoirs in the Penglai block (D-2) is mainly distributed in the range of 94%–96%, and that in the Zhongjiang block (D-2) is less than 90%, while that in the Gaoshiti-Moxi area (D-4) is between the two (Figure 2A); ② The C2H6 content of gas reservoirs in the three regions is very low (less than 0.1%) (Figure 2B), but the humidity coefficient (C2+/C1+) slightly varies—the humidity coefficient of gas reservoirs in the Penglai block is mainly distributed between 0.05% and 0.1%; that in the Zhongjiang block is mainly less than 0.05%; while that in the Gaoshiti-Moxi area (D-4) is a bit higher, mainly 0.025%–0.075% (Figure 2C); however, they are all typical dry gas (humidity coefficient is less than 5%); ③ Gas reservoirs in the three regions are all Medium-CO2-Bearing-gas-reservoirs–the CO2 content in Gaoshiti-Moxi area, Penglai and Zhongjiang blocks are 4%–8%, 2%–4% and >8%, respectively (Figure 2D); ④ Gas reservoirs in the three regions all belong to Slightly-N2-Bearing-gas-reservoirs (Figure 2E); ⑤ The H2S content in the Gaoshiti-Moxi area and the North Slope differs significantly—greater than 30 g/m3 in the Penglai and Zhongjiang blocks, belonging to High-sulfur-bearing-gas-reservoir; while less than 30 g/m3 in the Gaoshiti-Moxi area, belonging to Medium-sulfur-bearing-gas-reservoir (Figure 2F).
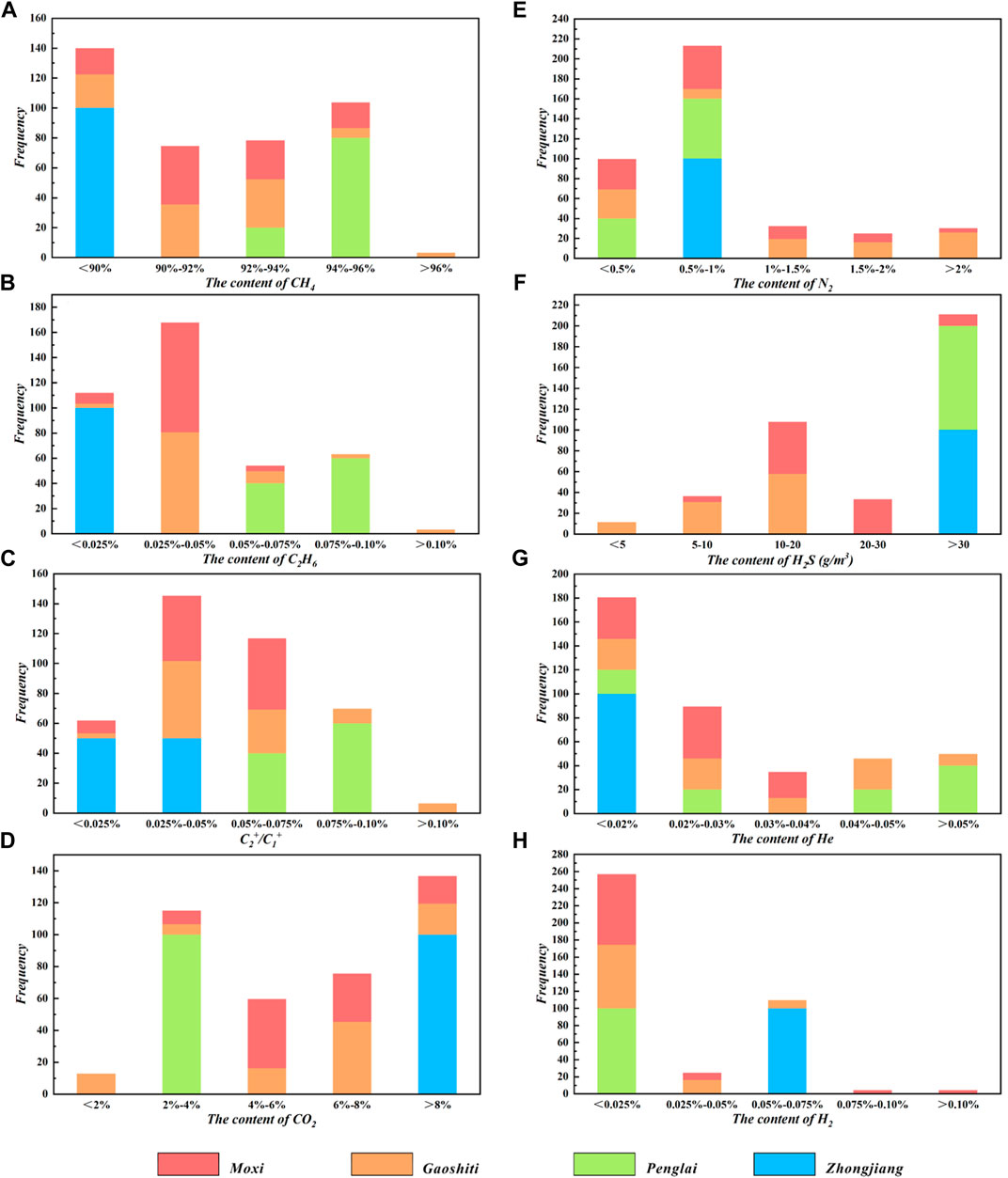
FIGURE 2. Histograms show the comparison of natural gas composition from the Gaoshiti-Moxi area (the Moxi and Gaoshiti areas) and the North Slope (the Penglai and Zhongjiang Blocks). The frequency histograms showing the distribution of the CH4 content (A), C2H6 content (B), C2+/C1+ (C), CO2 content (D), N2 content (E), H2S content (F), He content (G) and H2 content (H) in different blocks in the central Uplift and the North Slope of the Sichuan Basin.
From the natural gas component’s perspective, though natural gases in different Dengying reservoirs are typical dry gas, they differ in the gas component. Remarkably, the gas component of gas reservoirs in the North Slope differs significantly from that of the Gaoshiti-Moxi area—abundant in the H2S content, which may result from a more complicated TSR affection (Zhu et al., 2021).
Moreover, the natural gases of Dengying reservoirs in the North Slope and the Gaoshiti-Moxi area fall within the range of oil-cracking gas (Figure 3), indicating that the natural gases of Dengying reservoirs have the same genetic type. Noteworthy, they differ in maturity—attributing to the increase in burial depth, the maturity of natural gas gradually increases from south to north. Generally, the equivalent maturity of natural gas of the Dengying reservoirs in the North Slope is located in an interval greater than 2%.
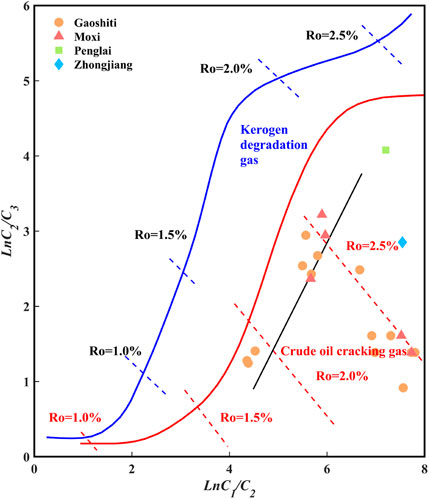
FIGURE 3. Discrimination plot demonstrating the natural gas genesis and maturity based on the identification chart created by (Xie et al., 2021a).
Source rock evaluation
The source rocks of the Lower Cambrian (the Qiongzhusi and the Maidiping Formations) and the Upper Sinian (the third member of the Dengying Formation) in central Sichuan are mainly marine black shale, containing a small amount of carbonaceous shale and riching in algae. The thickness of the third member of the Dengying Formation varies from 0 to 60 m, mainly distributed in the Gaoshiti-Moxi area and almost eroded in the platform margin on the North Slope. In contrast, the Maidiping Formation is mainly distributed in the North Slope. The lower Cambrian Qiongzhusi Formation is the thickest and most widely distributed source rock in the North Slope and the Gaoshiti-Moxi area, ranging in thickness from 300 to 700 m.
In the Gaoshiti-Moxi area, the TOC of the Qiongzhusi Formation is concentrated in the range of 0.51%–11.34%, with 1.41% and 1.86% on average and median. On the North Slope, the TOC of the Qiongzhusi Formation ranges from 0.50% to 5.94%, with medians and averages of 1.49% and 1.59%, respectively. From TOC’s perspective, the quality of the Qiongzhusi source rock in the North Slope does not differ significantly from that in the Gaoshiti-Moxi area. Moreover, we can see from Figure 4 that the quality of the Maidiping Formation matches the Qiongzhusi in terms of TOC (median:1.64%; aver.:1.86%). However, unlike TOC, there is a distinct difference in pyrolysis. The S1+S2 (hydrocarbon generation potential) on the North Slope is significantly lower than that in the Gaoshiti-Moxi area, which may attribute to a greater maturity led by a more substantial burial depth.
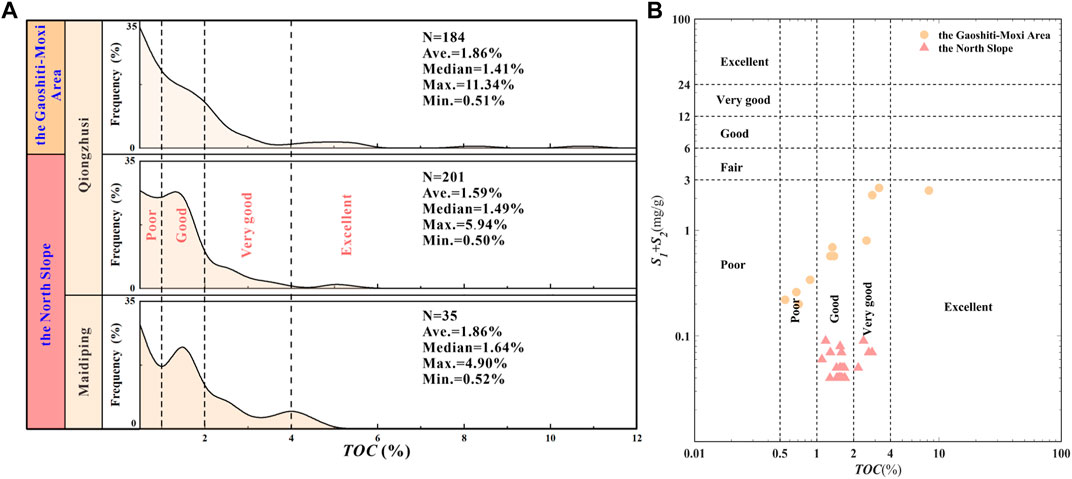
FIGURE 4. A comprehensive comparison of Lower Cambrian source rocks on the North Slope and in Gaoshiti-Moxi. (A)Comparing the qualities of Lower Cambrian source rocks (the Qiongzhusi and Maidiping Formations) on the North Slope and in the Gaoshiti-Moxi area. (B) Source rocks in Taihe and Gaoshiti-Moxi are primarily good-excellent, but Taihe’s average quality is better than those in Gaoshiti-Moxi, according to TOC and S1+S2.
Gas-source correlation
The natural gas of the Dengying reservoirs is at a high-mature stage and belongs to typical dry gas; therefore, it carries very little geochemical information. Only limited parameters such as the composition of light hydrocarbon and their carbon isotope may still inherit the characteristics of the source rock and can be used for the correlation to the source (Dai et al., 2018).
In well PT1, the carbon isotopes of methane (Dengying Formation) range between−33.95‰ and−33.90‰, with an average value of—33.93‰. Ethane’s carbon isotope in PT1 varies from−29.31‰ to−29.19‰, averaging−29.24‰. Furthermore, CO2 in well PT1 has an average carbon isotope of −0.48‰ but a range of −0.98‰ to −0.14‰. In contrast, a much smaller tendency can be seen in well ZJ2, where the average carbon isotope values for methane, ethane, and CO2 are−34.88‰,−33.18‰, and−17.88‰, respectively (Table 1). The isotopic sequence of methane and ethane in Dengying reservoirs on the North Slope shows a normal pattern (δ13C1 < δ13C2), but we observe differences in different reservoirs. The difference could be caused by different thermal maturities (Xie et al., 2021a) and TSR alteration (Xie et al., 2021a; Zhu et al., 2022).
Generally, the carbon isotope of methane (δ13C1) becomes heavier with increasing thermal maturity. In contrast, the carbon isotope of C2+, such as ethane (δ13C2), varies little with increasing thermal maturity but primarily varies with the type of parent material (kerogen) (Xie et al., 1999). Thus, the carbon isotope of C2+ is a better indicator for gas-source correlation than that of methane (Yanhua et al., 2006; Wei et al., 2015c; Wu et al., 2016b). However, as shown in Figure 5, neither the carbon isotopes of methane nor ethane from Dengying reservoirs can be correlated effectively with those from Qiongzhusi. As shown in Figure 5, the mean value of δ13C1 and δ13C2 of Dengying reservoirs are −34.4‰ and −29.0‰, respectively. It differs from the carbon isotope in Qiongzhusi shale (-35.3‰). The δ13C2 of natural gas in the Dengying reservoirs is relatively heavy—heavier than source rock (Qiongzhusi Formation and D-3 Member) (Zhang et al., 2019). It may be contributed by a mixed source (Wei et al., 2015b) or apparent alterations by TSR. To conclude, using the carbon isotope of methane or ethane for gas-source correlation is insufficient to identify the sources of natural gas.
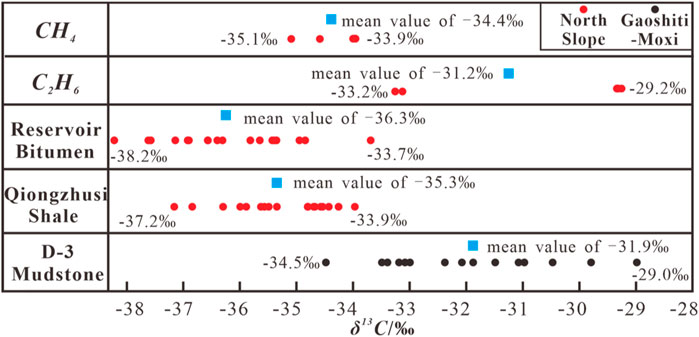
FIGURE 5. The gas/bitumen-source correlation on the North Slope. * Methane, ethane, and solid bitumen are from the Dengying reservoirs of ZJ2 and PT1 wells.
Compared with natural gas, another product of oil-cracking, the reservoir solid bitumen, carries more geochemical information and shows apparent advantages in correlation with the source for highly mature to over-mature natural gas reservoirs (Cai et al., 2017). The latest research even carried out the bitumen-source correlation with the help of trace and rare elements (Zhu et al., 2022). We can see from Figure 5 that the carbon isotope of the bitumen in the Dengying reservoirs in the North Slope ranges from −38.2‰ to −33.7‰, showing similar characteristics to the Qiongzhusi shale (from −37.2‰ to −33.9‰). Furthermore, the carbon isotope of reservoir solid bitumen is distinct from that of the D-3 mudstone (Figure 5), demonstrating that the Qiongzhusi shale is the primary source of the Dengying reservoirs in the North Slope area.
Geological features of sinian gas reservoirs
On the North Slope, typical cores from the main exploration wells (Figures 6A–E) show that: 1) Algal dolomite (microbial dolomite) dominates the Dengying reservoir rocks (D-2 and D-4 members), which can be categorized into three types–algal sandy dolomite, algal stromatolitic dolomite, and microcrystalline dolomite. 2) In algal stromatolitic dolomite and algal sandy dolomite, pores and caves are more developed than in microcrystalline dolomite. 3) Bitumen partially fills the porous spaces of almost all reservoir rocks.
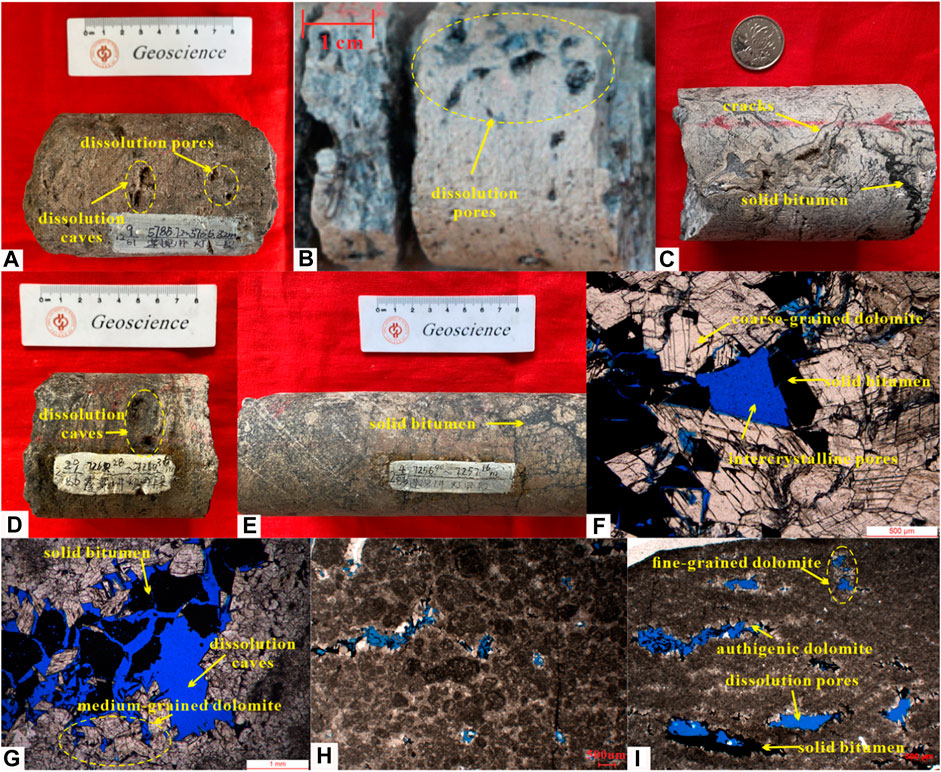
FIGURE 6. Typical cores and casting thin section photomicrographs illustrating the main lithologic types of the Dengying Reservoir on the North Slope and the occurrence of solid bitumen in cores and pore space. (A) PT1, 5785.72-5785.82 m, D-2, algal sandy dolomite, with solid bitumen filling in the dissolution pores and caves; (B) PT1, 5729 m, D-2, algal sandy dolomite, with developed cracksa and dissolution pores filled with solid bitumen; (C) ZJ2, 6546.27 m, D-2, algal dolomite, with solid bitumen filling in the dissolved pores and cracks; (D) PS1, 7260.28-7260.36 m, D-4, algal stromatolitic dolomite; (E) PS1, 7256.90-7257.16 m, D-4, microcrystalline dolomite, with solid bitumen filling in the dissolved cracks; (F) PT1, 5729.44 m, D-2, algal sandy dolomite, coarse-grained dolomite, bitumen partialy filling in the inter-crystalline pores of coarse-crystalline-dolomite; (G) ZJ2, 6553.56 m, D-2, algal dolomite, medium-grained dolomite, bitumen partialy filling in the inter-crystalline dissolved caves; (H) PS1, 7260.28 m, D-4, algal stromatolitic dolomite, inter-crystalline and intragranular pores partially filled by the solid bitumen after fine-grained authigenic dolomite formed; (I) PS1, 7262.41-7262.54 m, D-4, microcrystalline dolomite, bitumen filling in the intragranular pores after fine-grained authigenic dolomite formed.
Images of casting thin sections (Figures 6F–I) show: 1) The pore space of the algal dolomite is composed of intergranular dissolution pores, intragranular dissolution pores, and inter-crystalline pores, with developed dissolution caves and few micro-fractures. 2) The solid bitumen is widely distributed in the pore network and primarily distributed in the inter-crystalline and dissolution pores. 3) The wide distribution of the solid bitumen indicates that most gas reservoirs in the Dengying Formation resulted from the cracking of paleo-oil-accumulation.
With the help of core routine physical properties analysis of several typical wells (DB1, PT1, and ZJ2 wells in the North Slope; GS2 and MX10 wells in the Gaoshiti-Moxi area) (Figure 7), this study compared the Dengying reservoir’s physical properties in the uplift and the slope areas. The result shows that: 1) The Dengying reservoirs in the North Slope and the Gaoshiti-Moxi area are typical tight carbonate with low porosity and low permeability (air permeability <1 mD). 2) On the North Slope, the maximum and minimum porosity of the Dengying reservoirs is around 5% and 2%, respectively, with a median value of about 3.00%. In contrast, the median porosity of the Dengying reservoirs in the Gaoshiti-Moxi area is merely 2.34%, demonstrating a more dense nature of the reservoir. Noteworthy, the gas permeability of the Dengying reservoirs in the North Slope is also better than that of the Gaoshiti-Moxi area (Figure 7A). Therefore, though the burial depth of the Dengying reservoirs on the North Slope is greater than that of the Gaoshiti-Moxi area, the physical properties of the Dengying reservoirs seem better. 3) The permeability of the Dengying reservoirs in the North Slope is more concentrated, mainly in the range of 0.01–1 mD, while that in the Gaoshiti-Moxi area is more scattered. A better correlation relationship between porosity and the logarithmic permeability (Figure 7B) indicates that the heterogeneity of the Dengying reservoirs on the North Slope is lower than that in the Gaoshiti-Moxi area.
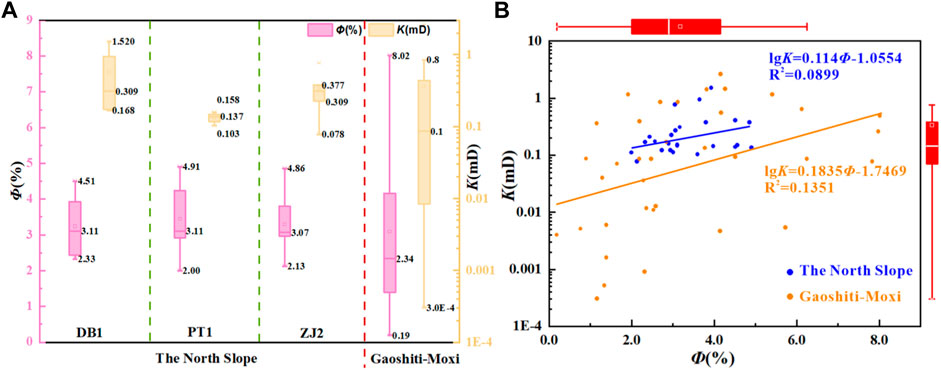
FIGURE 7. (A)Routine physical properties analysis for core samples from the North Slope and the Gaoshiti-Moxi area; (B) A scatter plot showing the correlation between porosity and permeability. A comparison of the physical properties of Dengying reservoir rocks from the North Slope and Gaoshiti-Moxi.
In addition, as shown in Figure 8, a thin section image processing procedure was used to determine the amount of solid bitumen in the reservoir pore network (Table. 2) of Dengying reservoirs. The results show that: 1) The solid bitumen content (plane porosity of solid bitumen) of the Dengying reservoirs on the North Slope (12.75%) is greater than that in the Gaoshiti-Moxi area (2.52%). 2) On the North Slope, the solid bitumen fills the pore space more severely than in the Gaoshiti-Moxi area. The filling degree of solid bitumen in the pore space varies from 16.97% to 87.88%, with an average value of 63.40%, demonstrating an average half-filling to full-filling status. In contrast, the filling degree of solid bitumen in the Gaoshiti-Moxi area ranges from 1.92% to 67.11%, averaging 36.08%, indicating a half-filling status. 3) The effective residual porosity of the Dengying reservoirs on the North Slope is distributed in the range of 1.75% to 14.19% with an average value of 4.41%. It is slightly smaller than that in the Gaoshiti-Moxi area (0.98%–12.34%, averaging:5.38%). However, it is worth noting that the Gross Plane Porosity of the Dengying reservoirs on the North Slope (averaging: 17.60%) is greater than that in the Gaoshiti-Moxi area (7.9%), indicating that during burial history, the pore space on the North Slope was ever more developed than that in the Gaoshiti-Moxi area. However, the later oil accumulation and oil-cracking resulted in a more severe solid bitumen filing status, which led to a lower residual porosity. On the other hand, it also illustrates that more oil was cracked to generate gas on the North Slope than in the Gaoshiti-Moxi area during the evolution history.
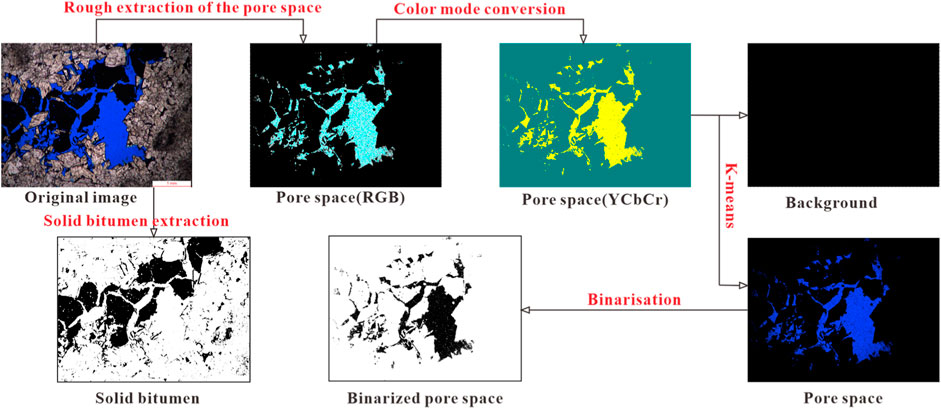
FIGURE 8. Workflow, explaining how casting thin section images can be used to determine the solid bitumen’s volumetric content.
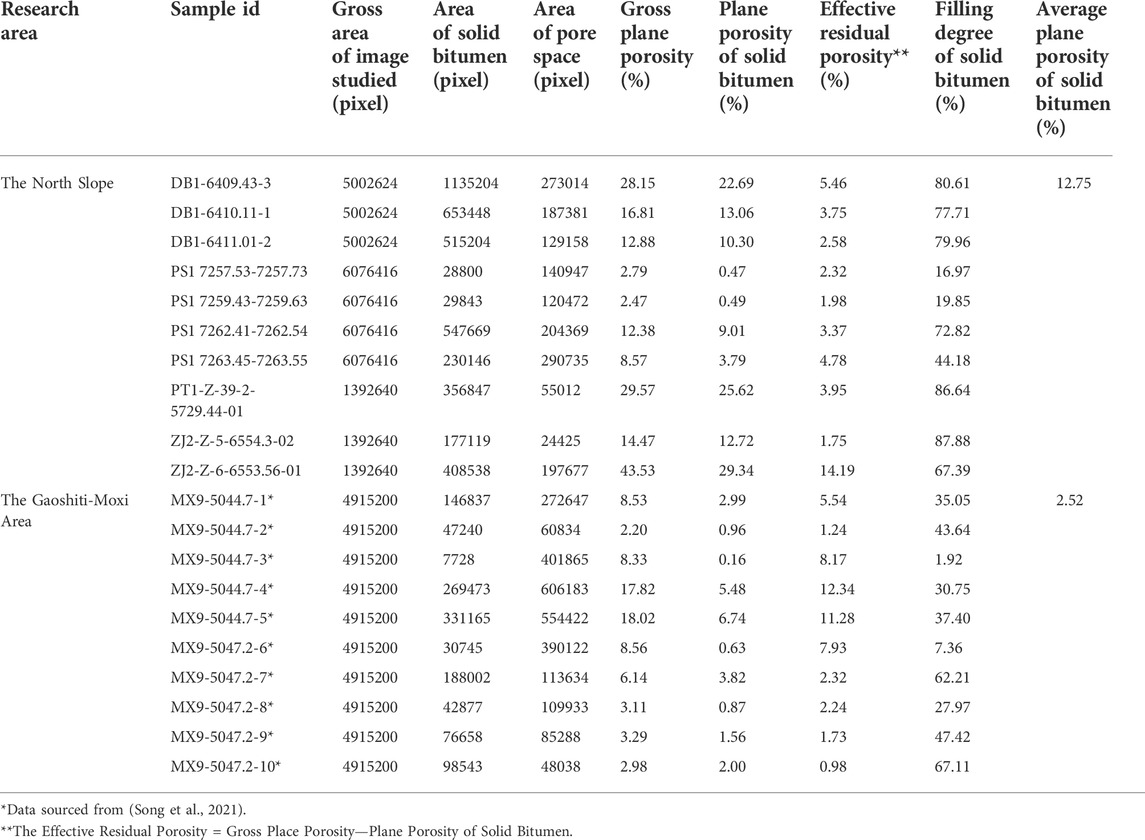
TABLE 2. The content of the solid bitumen in the pore space was obtained from casting thin section micrographs using the image processing method.
Fluid inclusion analysis
We found three types of hydrocarbon inclusions in the Dengying reservoirs (Figure 9), distributed in groups in dolomites and quartz. Type I hydrocarbon inclusions (Figure 9A) are mainly one-phase (all liquid, oil) inclusions. Type II hydrocarbon inclusions (Figure 9B) are primarily two-phase gas-liquid aqueous fluid inclusions. These two types of inclusions are all distributed in dolomite. In contrast, type III hydrocarbon inclusions (Figure 9C) are found in quartz and are one-phase gas inclusions. Moreover, we investigated the homogenization temperatures of two-phase gas-liquid brine inclusions accompanied by these three types of hydrocarbon inclusions. The homogenization temperatures show an increasing trend from brine inclusions accompanied by the type I hydrocarbon inclusions (homogenization temperatures: 130–140°C) to type II (homogenization temperatures: 170–190°C) and type III (homogenization temperatures: 210–220°C) (Figure 10). Fluid inclusion investigation indicates three hydrocarbon accumulation stages during the evolution of the Dengying reservoirs of the Penglai block. The first two stages were dominated by liquid hydrocarbons, whereas the third stage was dominated by dry gas.
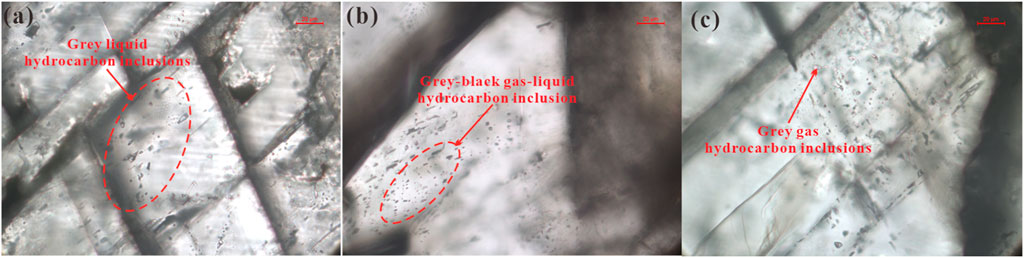
FIGURE 9. Photomicrographs demonstrating the fluid inclusions’ occurrence in the Dengying Formation (Well PT1) on the North Slope. (A) Grey hydrocarbon inclusions. Mono-phase (all-liquid) oil inclusions, triangular, elliptical, or elongated in shape, with a size of 2–6 μm, are distributed in groups in dolomite; polarized light. (B) Two-phase gas-liquid aqueous fluid inclusions with a gas-liquid ratio of less than 5%. Fluid inclusions are distributed primarily in groups in dolomite; polarized light. (C) Grey gas inclusions, mono-phase all-gas, with a size of 2–10 μm, mainly distributed in groups in quartz; polarized light.
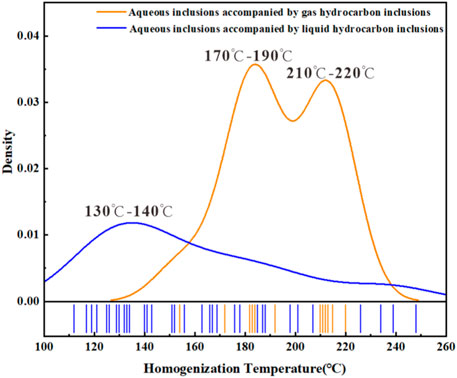
FIGURE 10. An illustration of the homogenization temperature of two-phase gas-liquid brine inclusions found in the Dengying reservoirs, Well PT1, and the Penglai Block of the North Slope.
1D and 2D simulation on hydrocarbon generation, accumulation, and evolution
In this study, the dynamic reconstruction of the hydrocarbon generation, accumulation, and evolution history in ultra-deeply-buried strata was carried out with the help of the Schlumberger PetroMod software. After deposition, the Sinian Dengying Formation experienced a long evolutionary history, including five crucial tectonic movements: the Tongwan movement, the Caledonian movement of Early Paleozoic, the Indosinian movement of Triassic, the Yanshan movement, and the Himalayan movement (Wang et al., 2021). Based on previous investigations, we obtained the plain distribution of the erosion thickness in every key tectonic movement (Zhang et al., 2018). Also, the heat flow is a crucial parameter for reconstructing the thermal evolution history (Xu et al., 2018). This study referred to the previous investigations on the thermal history of the Sichuan Basin (Liu et al., 2014; Zhu et al., 2017a).
For 1D thermal simulation, this study utilized the Vandenbroucke (1999)-TII-(NorthSea) model (Vandenbroucke et al., 1999) to investigate the maturity evolution history of the source rocks on the North Slope (the Qiongzhusi shale for ZJ2 and PT1 wells) and in Gaoshiti-Moxi area (the Qiongzhusi shale and D-3 mudstone for MX8 well) (Figure 11). One-dimensional simulation shows that: 1) After the deposition of the second member of the Dengying Formation, the third and fourth members of the Dengying Formation were severely eroded on the North Slope during the Tongwan movement. Therefore, the D-3 source rock only exists on the North Slope (MX8 well). 2) After a rapid deposition in the early Cambrian, the strata were severely uplifted (2000–2,500 m) during the Caledonian movement. The Qiongzhusi shale in ZJ2 and PT1 on the North Slope started to generate oil in the late Silurian, similar to the Qiongzhusi and D-3 source rocks in the Gaoshiti-Moxi area (MX8 well). Before the Late Carboniferous, due to the ongoing uplift process, the maturity of the source rocks remained in a low stage (the equivalent Ro was about 0.5%). 3) Since the late Carboniferous, the source rocks continued to subside, and the thermal evolution continued. Although there were slight uplifts during the Hercynian movement (uplift occurred in the Middle-Late Permian, 130–160 m) and the Indosinian movement (uplift occurred in the Early Triassic, 10–120 m), the maturation of the source rock continued. 4) Generally, the source rocks of the North Slope and the Gaoshiti-Moxi area reached the oil generation peak (equivalent Ro: 0.7%–1.0%) during the Indosinian movement, and the paleo-oil-accumulation started to crack from the late Indosinian to early Yanshan movement. It is worth noting that from north to south (from the slope area to the uplift), the degree of the thermal evolution of source rocks tends to decrease gradually. Thus, the paleo-oil-accumulation started to crack at the end of the Triassic on the North Slope while remaining almost uncracked at the early Jurassic in the Gaoshiti-Moxi area. Also, the dry gas reservoirs formed in the early Jurassic on the North Slope while in the late Jurassic in the Gaoshiti-Moxi area.
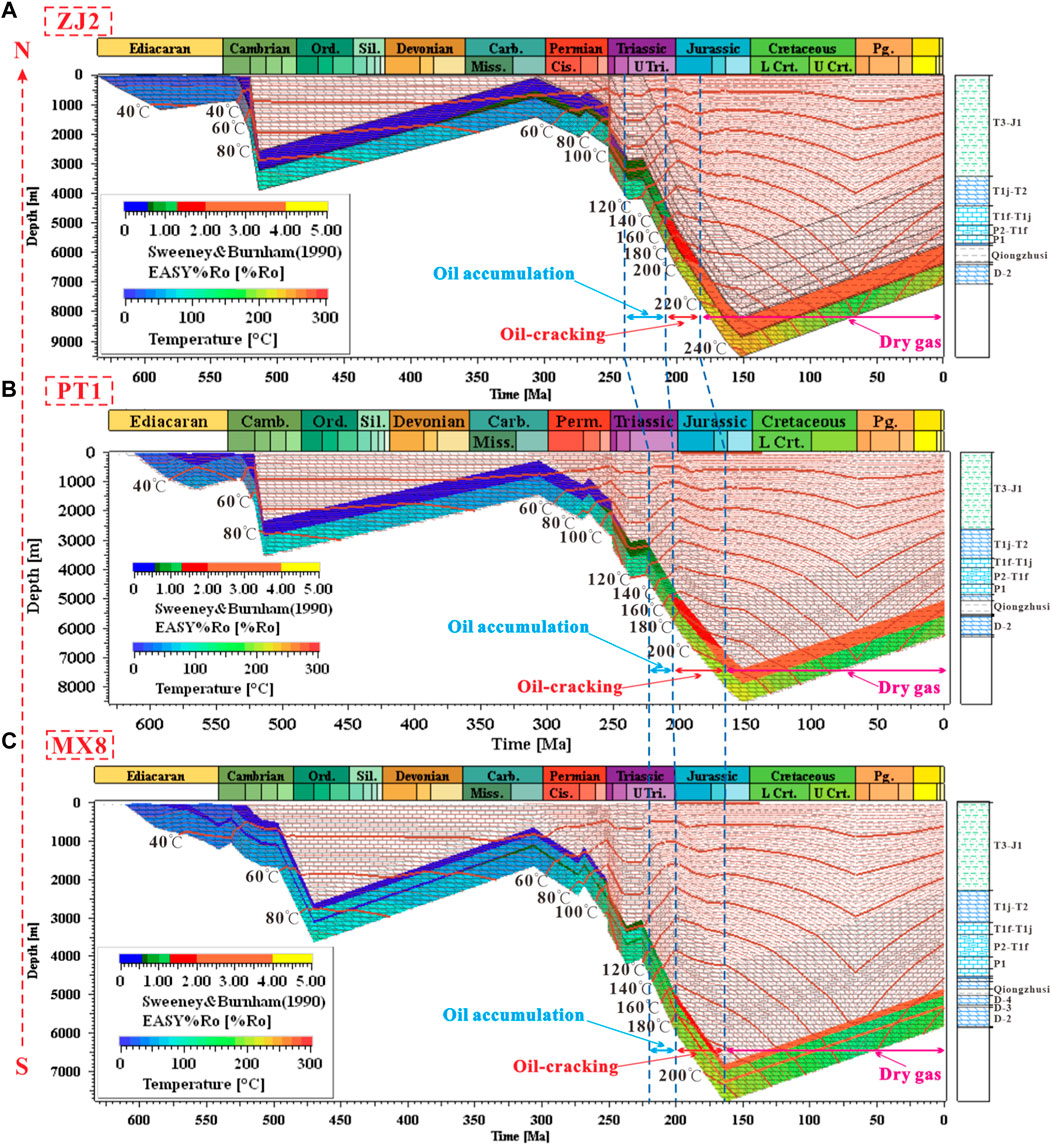
FIGURE 11. Burial and thermal evolution of the Upper Ediacaran Dengying reservoir and Lower Cambrian Qiongzhusi/Maidiping source rocks in the three blocks (the Moxi, Penglai, and Zhongjiang Blocks) of the central Sichuan Basin. (A) One-dimensional simulation for well ZJ2 of the Zhongjiang Block on the North Slope shows the burial history, the temperature evolution of Dengying reservoir, and the maturity of Qiongzhusi source rock. (B) One-dimensional simulation for well PT1 of the Penglai Block on the North Slope. (C) One-dimensional simulation for well MX8 of Gaoshiti-Moxi area.
Combining the 1D simulation results of PT1 with the fluid inclusion analysis, we found that: Type I hydrocarbon inclusions were formed when oil charged into the reservoir to form a paleo-oil-reservoir during the Indosinian movement. Thus, these fluid inclusions are all liquid inclusions. Type II hydrocarbon inclusions were formed during the oil-cracking stage while the paleo-oil-reservoir still remained, resulting in the two-phase hydrocarbon inclusions. Type III hydrocarbon inclusions were formed after the paleo-oil-reservoirs were entirely cracked, leaving only gas in the reservoirs.
For comparison’s sake, we selected a typical seismic profile, crossing typical blocks of the North Slope and the uplift area (Zhongjiang-Penglai-Goshiti-Moxi section) for 2D simulation. We obtained the 2D simulation results after several critical tectonic movements to simultaneously compare the hydrocarbon generation, accumulation, and evolution status in different structural positions (the slope and uplift areas).
1) In the late Caledonian (306 Ma), the Qiongzhusi/Maidiping shale on the North Slope began to generate oil intensively. The expulsed oil directly charged into the second member of the Dengying Formation from the top and the side. At this time, we can see several paleo-oil-reservoirs formed in low-amplitude uplifts on the North Slope. In contrast, there was barely any oil accumulation in the D-4 and the D-2 members in the Gaoshiti-Moxi area. 2) In the late Indosinian (200 Ma), though paleo-oil-reservoirs still dominated in the Dengying Formation on the North Slope and in the Gaoshiti-Moxi area (Feng et al., 2016), the paleo-oil-reservoirs on the North Slope started to crack. Noteworthy, paleo-oil-reservoirs also formed in the Canglangpu Formation on the North Slope and the Longwangmiao Formation in the Gaoshiti-Moxi area. 3) In the middle to late Yanshan movement (163 Ma), the burial depth of the Dengying Formation reached the maximum. As a result, the paleo-oil-reservoirs of the Dengying Formation on the North Slope and in the Gaoshiti-Moxi area entirely cracked, and paleo-gas-reservoirs widely distributed in these two regions. Also, abundant reservoir solid bitumen was retained as another typical product of oil-cracking (Gao et al., 2018; Liu et al., 2021). 4) During the Himalayan Movement, since the vertical tectonic movement dominated the central Sichuan Basin, the North Slope, and Gaoshiti-Moxi area were relatively structurally stable. After dynamic adjustment, paleo-gas-reservoirs were kept in situ and gradually transformed into today’s gas reservoirs (Li et al., 2021).
Above all, the widely distributed hydrocarbon accumulation of the Sinian Dengying Formation in the slope and the uplift areas of the central Sichuan Basin is favored by the following factors (Figure 12): 1) High-quality source rocks: The widely distributed high-quality Qiongzhusi/Maidiping shale is the primary source of the Sinian gas reservoirs. Moreover, the thickness of the source rocks on the North Slope is larger than in the Gaoshiti-Moxi area. (2)High-quality reservoirs: Favored by the two episodes of the Tongwan movement, the Sinian Dengying reservoirs (D-2 and D-4) are well developed. Greater original porosity and solid bitumen content of the reservoirs on the North Slope than in the Gaoshiti-Moxi area indicates a greater paleo-oil accumulation on the North Slope. 3) Good source-reservoir assemblage: The Dengying reservoirs are directly covered by the Qiongzhusi/Maidiping source rocks, forming an excellent source-reservoir assemblage, enabling hydrocarbon to charge into the reservoirs efficiently. It is worth mentioning that the source-reservoir assemblage in the slope area is better than that in the uplift area, enabling hydrocarbon to charge into the reservoirs from the top and the side. Besides, these thick shales on the top of the reservoirs also serve as good caprocks, which is also a controlling factor in the preservation of the ultra-deeply-buried gas reservoirs after multi-stage tectonic evolution.
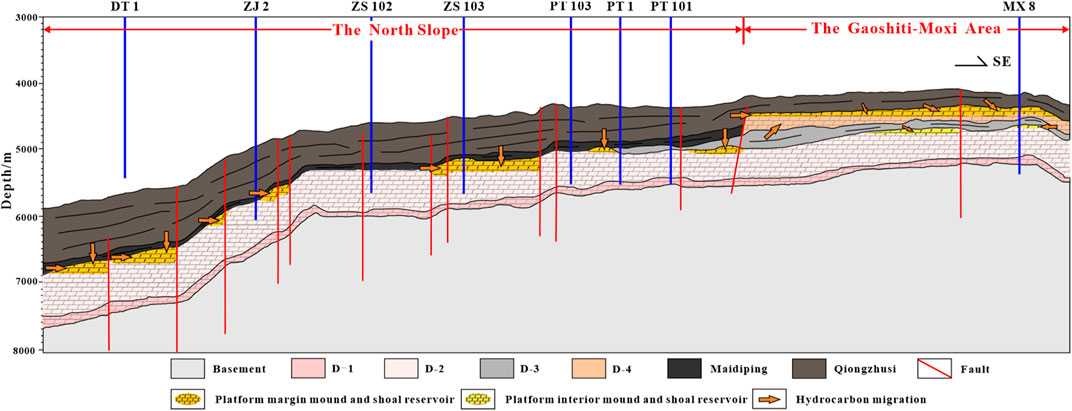
FIGURE 12. The hydrocarbon accumulation mode of the Sinian Dengying gas reservoirs in Central Sichuan Basin.
Favored by these factors, the typical evolution mode of the ultra-deeply-buried Dengying gas reservoirs is: paleo-oil-reservoir, paleo-gas-reservoir to the present gas reservoir (Figure 13). Special attention should be paid to two issues: 1) The thermal evolution of source rocks on the North Slope is earlier than that in the uplift area. Therefore, the formation of paleo-oil reservoirs and paleo-gas-reservoirs on the North Slope is earlier than that in the uplift area. Combined with the tectonic evolution, it is possible that a large amount of oil/gas ever migrated from the slope area to the uplift area. This lateral migration of hydrocarbon may also result in the complexity of gas isotope and the mixture of gases of different thermal maturity mentioned by (Wei et al., 2015a; Wei et al., 2015b; Xie et al., 2021a; Xie et al., 2021b). 2) A more significant amount of solid bitumen content and a better reservoir quality on the North Slope may indicate that the scale of paleo-oil-reservoirs on the North Slope may be greater than that in the inherited uplift area. However, it still requires further verification.
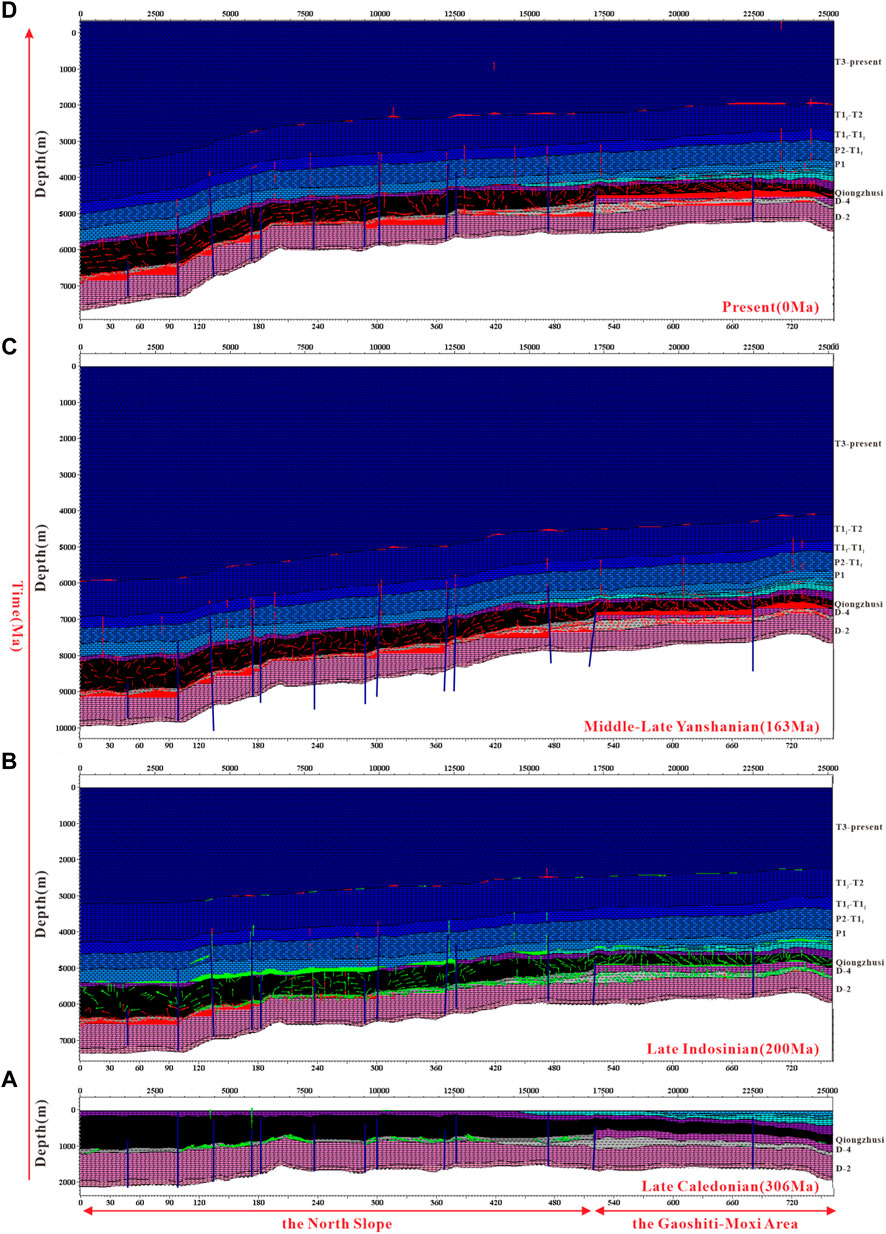
FIGURE 13. Two-dimensional simulation for the comparison of the formation and evolution of Sinian gas reservoirs in different blocks of the central Sichuan Basin. (A) In late Caledonian, oil started to accumulate on the North Slope. The Dengying strata in all regions were relatively gentle. (B) In late Indosinian, a large amount of oil was generated and migrated laterally and horizontally to reservoirs. Oil of the Dengying reservoirs in Zhongjiang block cracked into gas due to a deeper burial depth. The Dengying strata in all regions remained relatively gentle. (C) In middle-late Yanshanian, due to the rapid subsidence of the North Slope, the strata then dipped north. The Gaoshiti-Moxi area gradually became an uplift area. Almost all the oil reservoirs cracked, and gas migrated upwards and laterally. (D) The present gas reservoir profile shows the gas reservoirs’ potential distribution.
Remained challenges and improvements
Generally, when the PetroMod is utilized to simulate one-dimensional and two-dimensional hydrocarbon accumulation, the results reflect more on the source rocks’ thermal evolution and hydrocarbon’s phase evolution in reservoirs. The specific distribution of paleo oil and gas reservoirs also depends on the distribution of reservoirs and traps. Therefore, it is worth noting that there is a certain difference between the specific location of paleo oil and gas accumulation reflected in the two-dimensional hydrocarbon accumulation simulation and the actual location, which needs further verification. It will be a good improvement to understand the formation of lithologic traps under the control of widely developed strike-slip faults in Central Sichuan (Zhang et al., 2022) and apply them to two-dimensional simulation. Other than this deficiency, an improvement in this study is that we selected a more suitable hydrocarbon evolution model for the research of ultra-deeply-buried strata—a typical oil-cracking model in the North Sea (Vandenbroucke et al., 1999). Thus, the results seem satisfying in the aspects of the hydrocarbon evolution. Also, the 2D simulation illustrates that before the late Yanshan movement, the structural difference between the North Slope and Gaoshiti-Moxi area was not as significant as today, and there were also many low-amplitude uplifts on the North Slope, which are favorable for paleo-oil/gas accumulation. However, after the Yanshan movement, the structural pattern of the North Slope changed significantly and gradually evolved into the present slope area, which is consistent with previous investigations (Ma et al., 2020).
Conclusion
In summary, this study presented an integrated investigation of the hydrocarbon generation, accumulation, and evolution in the ultra-deeply-buried strata in the central Sichuan Basin based on geochemical analysis of source rocks, gas and solid bitumen, lithologic and physical analysis of reservoirs, gas/bitumen-source correlation, 1D and 2D hydrocarbon accumulation simulation. The following conclusions have been achieved:
(1) The geological conditions for hydrocarbon generation and accumulation in the slope area are better than in the uplift area.
(2) The gas in ultra-deeply-buried Sinian Dengying reservoirs in central Sichuan is typical oil-cracking gas soured mainly from the Qiongzhusi/Maidiping shale. The formation of Sinian gas reservoirs includes three stages: the formation of paleo-oil-reservoirs; the cracking of paleo-oil-reservoirs into paleo-gas-reservoirs; the adjustment of the paleo-gas-reservoirs.
(3) Source rock and reservoir evaluation, quantitative solid bitumen analysis, and hydrocarbon accumulation simulation indicate that the scale of paleo-oil-reservoirs on the North Slope may be greater than that in the inherited uplift area, which requires further verification.
Data availability statement
The raw data supporting the conclusions of this article will be made available by the authors, without undue reservation.
Author contributions
Methodology and writing, original draft preparation, ZS; supervision, BZ and ZS; investigation, XD; visualization and formal analysis, BG; writing—review and editing, ZS and XD; project administration, BZ, XT, YW, DY; sampling and experiments, YW and DY.
Funding
The present research was funded by the National Natural Science Foundation of China, grant number 41802148; the State Key Laboratory of Petroleum Resources and Prospecting, grant number PRP/indep-04-1611 and 2462017YJRC025 and the PetroChina Southwest Oilfield Company, grant number JS 2021-096.
Acknowledgments
We thank the PetroChina Southwest Oilfield Company for providing core samples and necessary data.
Conflict of interest
Authors BZ, XT, XC, KM, HP, YW, and DY were employed by Southwest Oil and Gas Field Company PetroChina.
The remaining authors declare that the research was conducted in the absence of any commercial or financial relationships that could be construed as a potential conflict of interest.
Publisher’s note
All claims expressed in this article are solely those of the authors and do not necessarily represent those of their affiliated organizations, or those of the publisher, the editors and the reviewers. Any product that may be evaluated in this article, or claim that may be made by its manufacturer, is not guaranteed or endorsed by the publisher.
References
Cai, C., Xiang, L., Yuan, Y., Xu, C., He, W., Tang, Y., et al. (2017). Sulfur and carbon isotopic compositions of the Permian to Triassic TSR and non-TSR altered solid bitumen and its parent source rock in NE Sichuan Basin. Org. Geochem. 105, 1–12. doi:10.1016/j.orggeochem.2016.12.004
Cao, Y., Yuan, G., Yang, H., Wang, Y., Liu, K., Zan, N., et al. (2022). Current situation of oil and gas exploration and research progress of the origin of high-quality reservoirs in deep-ultra-deep clastic reservoirs of petroliferous basins. Acta Pet. Sin. 43 (1), 112–140. doi:10.7623/syxb202201010
Dai, J., Ni, Y., Qin, S., Huang, S., Peng, W., and Han, W. (2018). Geochemical characteristics of ultra-deep natural gas in the Sichuan Basin, SW China. Pet. Explor. Dev. 45 (4), 619–628. doi:10.1016/s1876-3804(18)30067-3
Feng, M., Qiang, Z., Shen, P., Zhang, J., Tao, Y., and Xia, M. (2016). Evidences for hydrothermal dolomite of sinian Dengying formation in gaoshiti-moxi area, Sichuan Basin. Acta Pet. Sin. 37 (5), 587–598. doi:10.7623/syxb201605003
Feng, Q. F., Xiao, Y. X., Hou, X. L., Chen, H. K., Wang, Z. C., Feng, Z., et al. (2021). Logging identification method of depositional facies in sinian Dengying formation of the Sichuan Basin. Pet. Sci. 18 (4), 1086–1096. doi:10.1016/j.petsci.2020.10.002
Gao, P., Liu, G. D., Lash, G. G., Li, B. Y., Yan, D. T., and Chen, C. (2018). Occurrences and origin of reservoir solid bitumen in sinian Dengying formation dolomites of the Sichuan Basin, SW China. Int. J. Coal Geol. 200, 135–152. doi:10.1016/j.coal.2018.11.001
Li, J. Z., Tao, X. W., Bai, B., Huang, S. P., Jiang, Q. C., Zhao, Z. Y., et al. (2021). Geological conditions, reservoir evolution and favorable exploration directions of marine ultra-deep oil and gas in China. Pet. Explor. Dev. 48 (1), 60–79. doi:10.1016/s1876-3804(21)60005-8
Li, X., Guo, Z., Hu, Y., Liu, X., Wan, Y., Luo, R., et al. (2020). High-quality development of ultra-deep large gas fields in China: Challenges, strategies and proposals. Nat. Gas. Ind. 40 (2), 75–82. doi:10.3787/j.issn.1000-0976.2020.02.008
Liu, S., Li, Z., Deng, B., Sun, W., Li, Z., Ding, Y., et al. (2021). Occurrence morphology of bitumen in Dengying Formation deep and ultra-deep carbonate reservoirs of the Sichuan Basin and its indicating significance to oil and gas reservoirs. Nat. Gas. Ind. 41 (8), 102–112. doi:10.3787/j.issn.1000-0976.2021.08.010
Liu, Y., Qiu, N., Xie, Z., Yao, Q., and Wu, B. (2014). Characteristics and effects on gas accumulation of the sinian-lower cambrian temperature-pressure field in the central paleo-uplift, Sichuan Basin. Acta Sedimentol. Sin. 32 (3), 601–610. doi:10.14027/j.cnki.cjxb.2014.03.023
Ma, K., Shen, P., Wang, Z., Tian, X., Peng, H., Wang, H., et al. (2019). Natural gas exploration potential of Longwangmiao Formation on the north slope of Chuanzhong paleo-uplift from the characteristics ofFour Paleo. Nat. Gas. Geosci. 30 (10), 1451–1464. doi:10.11764/j.issn.1672-1926.2019.07.016
Ma, K., Zhang, X., Peng, H., Sun, Y., Yang, D., Zhong, J., et al. (2020). Tectonic evolution of Moxi north slope in Sichuan Basin and its effect on forming Sinian oil and gas reservoirs. Nat. Gas Explor. Dev. 43 (1), 8–15. doi:10.12055/gaskk.issn.1673-3177.2020.01.002
Ren, Z., Cui, J., Qi, K., Yang, G., Chen, Z., Yang, P., et al. (2020). Control effects of temperature and thermal evolution history of deep and ultra-deep layers on hydrocarbon phase state and hydrocarbon generation history. Nat. Gas. Ind. 40 (2), 22–30. doi:10.3787/j.issn.1000-0976.2020.02.003
Song, Z., Liu, G., Luo, B., Zeng, Q., Tian, X., Dai, X., et al. (2021). Logging evaluation of solid bitumen in tight carbonate in deepburied and ultra-deep-buried strata of the central Sichuan Basin. Acta Sedimentol. Sin. 39 (1), 197–211. doi:10.14027/j.issn.1000‐0550.2020.127
Tissot, B. P., and Welte, D. H. (1978). “formation of petroleum in relation to geological processes. Timing of oil and gas generation,” in Petroleum formation and occurrence: A new approach to oil and gas exploration. Editors B. P. Tissot, and D. H. Welte (Berlin, Heidelberg: Springer Berlin Heidelberg), 185–201.
Vandenbroucke, M., Behar, F., and Rudkiewicz, J. L. (1999). Kinetic modelling of petroleum formation and cracking: implications from the high pressure/high temperature elgin field (UK, North Sea). Org. Geochem. 30 (9), 1105–1125. doi:10.1016/S0146-6380(99)00089-3
Wang, X., Tian, H., Xiao, X. M., Liu, D. H., Min, Y. S., Li, T. F., et al. (2021). Methane-dominated gaseous inclusions in the Sinian carbonate reservoirs in central Sichuan Basin and their implications for natural gas accumulation. Mar. Pet. Geol. 125, 104871. doi:10.1016/j.marpetgeo.2020.104871
Wei, G., Du, J., Xu, C., Zou, C., Yang, W., Shen, P., et al. (2015a). Characteristics and accumulation modes of large gas reservoirs in Sinian-Cambrian of Gaoshiti-Moxi region, Sichuan Basin. Acta Pet. Sin. 36 (1), 1–12. doi:10.7623/syxb201501001
Wei, G., Xie, Z., Song, J., Yang, W., Wang, Z., Li, J., et al. (2015b). Features and origin of natural gas in the Sinian-Cambrian of central Sichuan paleo-uplift, Sichuan Basin, SW China. Pet. Explor. Dev. 42 (6), 768–777. doi:10.1016/s1876-3804(15)30073-2
Wei, G., Yang, W., Xie, W., Su, N., Xie, Z., Zeng, F., et al. (2022). Formation mechanisms, potentials and exploration practices of large lithologic gas reservoirs in and around an intracratonic rift: Taking the sinian—Cambrian of Sichuan Basin as an example. Pet. Explor. Dev. 49 (2), 530–545. doi:10.1016/s1876-3804(22)60044-2
Wei, G., Yang, W., Xie, W., Xie, Z., Zeng, F., Mo, W., et al. (2015c). Formation conditions, accumulation models and exploration direction of large gas fields in sinian-cambrian, Sichuan Basin. Nat. Gas. Geosci. 26 (5), 785–795. doi:10.11764/j.issn.1672-1926.2015.05.0785
Wu, W., Luo, B., Luo, W., and Wang, W. (2016a). Further discussion about the origin of natural gas in the Sinian of central Sichuan paleo-uplift, Sichuan Basin, China. Nat. Gas. Geosci. 27 (8), 1447–1453. doi:10.11764/j.issn.1672-1926.2016.08.1447
Wu, W., Luo, C., Zhang, J., and Liu, W. (2016b). Evolution law and Genesis of ethane carbon isotope of oil type gas. Acta Pet. Sin. 37 (12), 1463–1471. doi:10.7623/syxb201612002
Xie, Z., Li, J., and Lu, X. (1999). An approach to the changing origin and classification of ethane carbon isotope in marine gas of Tarim basin. Pet. Explor. Dev. 26 (6), 27–29.
Xie, Z., Li, J., Yang, C., Tian, X., Zhang, L., Li, J., et al. (2021a). Geochemical characteristics of Sinian-Cambrian natural gas in central Sichuan paleo-uplift and exploration potential of Taihe gas area. Nat. Gas. Ind. 41 (7), 1–14. doi:10.3787/j.issn.1000-0976.2021.07.001
Xie, Z., Wei, G., Li, J., Xu, L., Zhang, L., Li, J., et al. (2021b). Geochemical characteristics and accumulation pattern of gas reservoirs of the Sinian-Permian in central Sichuan uplift zone, Sichuan Basin. China Pet. Explor. 26 (6), 50–67. doi:10.3969/j.issn.1672-7703.2021.06.004
Xu, C., Shen, P., Yang, Y., Zhao, L., Luo, B., Wen, L., et al. (2020). New understandings and potential of Sinian-Lower Paleozoic natural gas exploration in the central Sichuan paleo-uplift of the Sichuan Basin. Nat. Gas. Ind. 40 (7), 1–9. doi:10.14027/j.issn.1000‐0550.2020.127
Xu, Q., Qiu, N., Liu, W., Shen, A., and Wang, X. (2018). Thermal evolution and maturation of sinian and cambrian source rocks in the central Sichuan Basin, southwest China. J. Asian Earth Sci. 164, 143–158. doi:10.1016/j.jseaes.2018.06.015
Yang, S., Wu, G., Zhu, Y., Zhang, Y., Zhao, X., Lu, Z., et al. (2022). Key oil accumulation periods of ultra-deep fault-controlled oil reservoir in northern Tarim Basin, NW China. Pet. Explor. Dev. 49 (2), 285–299. doi:10.1016/S1876-3804(22)60024-7
Yang, Y., Wen, L., Xie, J., Luo, B., Huang, P., Ran, Q., et al. (2020). Progress and direction of marine carbonate gas exploration in Sichuan Basin. China Pet. Explor. 25 (3), 44–55. doi:10.3969/j.issn.1672-7703.2020.03.005
Yanhua, S., Yanrong, Z. O. U., Pingan, P., Shuichang, Z., Yongqiang, X., and Jinzhong, L. I. U. (2006). Kinetic modeling of stable carbon isotope ratios of ethane from coal in confined system and its significance in geological application. Geochimica 35 (2), 151–156. doi:10.19700/j.0379-1726.2006.02.005
Zhang, H., Hu, W., Li, W., Chen, Z., Hao, M., Guo, X., et al. (2018). Establishment of paleo-topography evolutionary section of key strata boundary and its geological implications. Pet. Geol. Recovery Effic. 25 (2), 8–14. doi:10.13673/j.cnki.cn37-1359/te.2018.02.002
Zhang, P., Liu, G., Cai, C., Li, M., Chen, R., Gao, P., et al. (2019). Alteration of solid bitumen by hydrothermal heating and thermochemical sulfate reduction in the Ediacaran and Cambrian dolomite reservoirs in the Central Sichuan Basin, SW China. Precambr. Res. 321, 277–302. doi:10.1016/j.precamres.2018.12.014
Zhang, X., Ran, Q., Chen, K., Zhang, B., Zhang, C., Ma, B., et al. (2022). The control effect of strike-slip fault on Dengying Formation reservoir and gas enrichment in Anyue gas field in central Sichuan Basin. Nat. Gas. Geosci. 33 (6), 1–14. doi:10.11764/j.issn.1672-1926.2021.11.009
Zhao, W., Hu, S., Liu, W., Wang, T., and Li, Y. (2014). Petroleum geological features and exploration prospect in deep marine carbonate strata onshore China: A further discussion. Nat. Gas. Ind. 34 (4), 1–9. doi:10.3787/j.issn.1000-0976.2014.04.001
Zhao, W., Wang, Z., Jiang, H., Fu, X., Xie, W., Xu, A., et al. (2020). Exploration status of the deep Sinian strata in the Sichuan Basin: Formation conditions of old giant carbonate oil/gas fields. Nat. Gas. Ind. 40 (2), 1–10. doi:10.3787/j.issn.1000-0976.2020.02.001
Zhu, G., Wang, T., Xie, Z., Xie, B., and Liu, K. (2015). Giant gas discovery in the Precambrian deeply buried reservoirs in the Sichuan Basin, China: Implications for gas exploration in old cratonic basins. Precambr. Res. 262, 45–66. doi:10.1016/j.precamres.2015.02.023
Zhu, C., Qiu, N., Cao, H., Liu, Y., and Jiang, Q. (2017a). Tectono-thermal evolution of the eastern Sichuan Basin: constraints from the vitrinite reflectance and apatite fission track data. Earth Sci. Front. 24 (3), 94–104. doi:10.13745/j.esf.2017.03.008
Zhu, G., Liu, X., Yang, H., Su, J., Zhu, Y., Wang, Y., et al. (2017b). Genesis and distribution of hydrogen sulfide in deep heavy oil of the Halahatang area in the Tarim Basin, China. J. Nat. Gas Geosci. 2 (1), 57–71. doi:10.1016/j.jnggs.2017.03.004
Zhu, G., Milkov, A. V., Li, J., Xue, N., Chen, Y., Hu, J., et al. (2021). Deepest oil in Asia: Characteristics of petroleum system in the Tarim basin, China. J. Pet. Sci. Eng. 199, 108246. doi:10.1016/j.petrol.2020.108246
Zhu, L., Liu, G., Song, Z., Zhao, W., Tian, X., Dai, X., et al. (2021). The differences in natural gas from the Dengying Formation in different areas of the north slope of the central Sichuan Paleo-uplift and its controlling factors. Pet. Sci. Bull. 03, 344–355. doi:10.3969/j.issn.2096-1693.2021.03.028
Zhu, L., Liu, G., Song, Z., Zhao, W., Li, Q., Tian, X., et al. (2022). Reservoir solid bitumen-source rock correlation using the trace and rare Earth elements–implications for identifying the natural gas source of the Ediacaran-Lower Cambrian reservoirs, central Sichuan Basin. Mar. Pet. Geol. 137, 105499. doi:10.1016/j.marpetgeo.2021.105499
Keywords: hydrocarbon generation, accumulation, evolution history, dengying formation, central sichuan basin
Citation: Song Z, Ding X, Zhang B, Ge B, Tian X, Chen X, Ma K, Peng H, Wang Y and Yang D (2022) Dynamic reconstruction of the hydrocarbon generation, accumulation, and evolution history in ultra-deeply-buried strata. Front. Earth Sci. 10:927903. doi: 10.3389/feart.2022.927903
Received: 25 April 2022; Accepted: 18 August 2022;
Published: 27 September 2022.
Edited by:
Chen Zhang, Chengdu University of Technology, ChinaReviewed by:
Zuozhen Han, Shandong University of Science and Technology, ChinaKeji Yang, Hebei GEO University, China
Copyright © 2022 Song, Ding, Zhang, Ge, Tian, Chen, Ma, Peng, Wang and Yang. This is an open-access article distributed under the terms of the Creative Commons Attribution License (CC BY). The use, distribution or reproduction in other forums is permitted, provided the original author(s) and the copyright owner(s) are credited and that the original publication in this journal is cited, in accordance with accepted academic practice. No use, distribution or reproduction is permitted which does not comply with these terms.
*Correspondence: Zezhang Song, songzz@cup.edu.cn; Benjian Zhang, zbjian@petrochina.com.cn