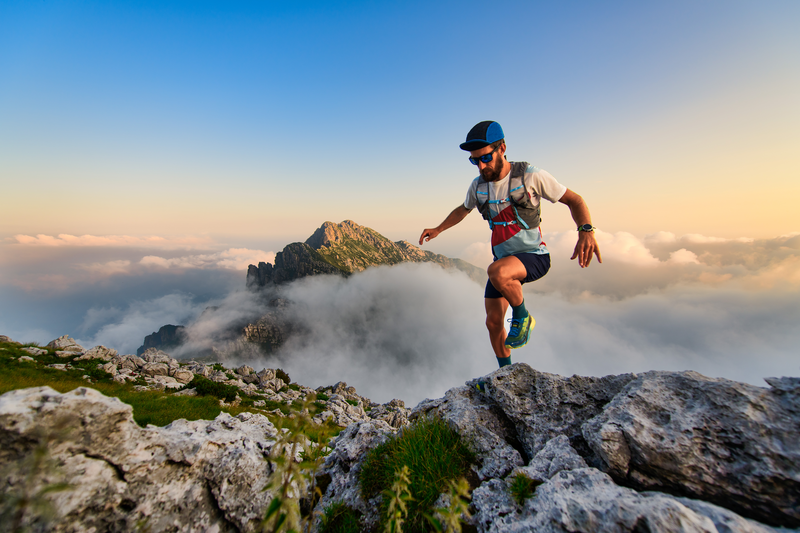
95% of researchers rate our articles as excellent or good
Learn more about the work of our research integrity team to safeguard the quality of each article we publish.
Find out more
ORIGINAL RESEARCH article
Front. Earth Sci. , 07 July 2022
Sec. Structural Geology and Tectonics
Volume 10 - 2022 | https://doi.org/10.3389/feart.2022.918244
This article is part of the Research Topic Effects of Deep Fluids in Hydrocarbon Accumulations in Sedimentary Basins View all 26 articles
In addition to tectonic fractures, the ubiquitous and important bedding fractures are often overlooked in the imbibition processes. Based on the identification of different fracture types in tight sandstone from the middle Permian Lucaogou Formation in the Jimusar Sag, NW China, spontaneous imbibition of water experiments was carried out, aiming to investigate the effect of tectonic and bedding fractures on hydrocarbon recovery efficiency in tight reservoir rocks. The results show that the difference in connectivity between the vertical (tectonic fractures) and horizontal (bedding fractures) directions of pores and pore throats is the main reason for the distinction in microscale permeability and, hence, fluid imbibition. The difference in the time required for imbibition between bedding and tectonic fractures is subtle, but the former is slightly lower than the latter in terms of imbibition depth and efficiency. Higher temperatures can shorten the reaction time of imbibition. On the basis that the tectonic fractures are opened, opening the same scale of bedding can increase the imbibition effectiveness by 8.7% at reservoir conditions (27 MPa, 80°C), and the closer to the fracture, the higher the imbibition efficiency in the matrix. The samples with higher porosity and permeability per unit volume have high imbibition oil recovery. However, the imbibition efficiency of the samples with lower porosity and permeability is higher, i.e., the final imbibition efficiency is inversely proportional to porosity and permeability.
Spontaneous imbibition refers to the process in which porous media imbibe wetting liquid spontaneously by means of capillary forces (Morrow and Mason, 2001; Hasan et al., 2006; Mason et al., 2009; Zhou et al., 2018). Spontaneous imbibition can be seen in two ways. On the one hand, the change curve of imbibition over time can be used to describe its process (Li et al., 2018). Gu et al. (2017) found that with the increase in the rate of fluid imbibition (volume of liquid/unit of time), the spontaneous imbibition recovery efficiency which is defined as the amount of oil that is recovered after the spontaneous imbibition occurred compared to original reservoir reserves is improved. However, Peng et al. (2010) showed that when the spontaneous imbibition rate is greater than a certain value, the improvement of spontaneous imbibition recovery will slow down or stabilize. Li et al. (2018) proposed that with the increase in interfacial tension, the thickness of spontaneous imbibition film first increases and then decreases. On the other hand, the control factors of spontaneous imbibition were explored. Previous studies have shown that reservoir and fluid properties have an impact on spontaneous imbibition, including temperature (Babadagli, 2001), wetting index and interfacial tension (Wei et al., 2016), clay mineral content (Cai et al., 2020), porosity (Liu and Dai, 2008), permeability (Yang et al., 2019), pore size and pore throat properties (Lai et al., 2019), water content and crude viscosity in rocks (Fischer et al., 2008), geometry and boundary conditions of samples (Standnes and Chun, 2004; Hasan et al., 2006; Mason et al., 2009), contact area of rocks with wetting phase fluids, and initial water saturation (Wang et al., 2019a). These studies show that results from different imbibition experiments are impacted by the properties of the samples both solid and liquid.
Imbibition studies are used to help understand fluid recovery efficiencies. At present, studies on imbibition aiming at improving oil recovery from low permeability rocks have been mainly emphasized the fractured carbonate reservoirs or low-permeability and ultra-low-permeability unfractured tight shale reservoirs, and they have mainly focused on the influence of reservoir physical properties such as pore and throat characteristics on spontaneous imbibition (Standnes and Chun, 2004; Hasan et al., 2006; Mason et al., 2009; Saberhosseini et al., 2019; Junira et al., 2020). On the one hand, capillary forces make the positive spontaneous imbibition effect of crude oil significant to the production of crude oil at the early stage. On the other hand, due to the strong heterogeneity between the matrix and fracture network, the fractures are likely to become flow channels for water and oil (Kathel et al., 2013). Therefore, the high permeability channel formed by fractures leads to poor water flooding efficiency in low-permeability reservoirs (Zhao et al., 2018). The permeability of a tight reservoir matrix is low, generally less than 0.1 mD in the subsurface, and conventional water flooding is difficult for improved oil production, and spontaneous imbibition is an alternative method for tight oil reservoir development (Schechter et al., 1994). Oil recovery can be improved by displacement using imbibition (Al-Attar, 2010). Spontaneous imbibition has attracted much attention due to its simple operation, low cost, and high efficiency (Mirzaei and Dicarlo, 2013). At the fracture surface and near the fracture matrix, spontaneous imbibition of water into the small pores takes place, while oil is displaced into the large pores (Wang et al., 2019b; Dai et al., 2019). The displacement of oil in the matrix can only be realized by capillary spontaneous imbibition, while the displacement of oil in fractures can be called forced imbibition because of the viscous pressure gradient (Babadagli, 2000) applied in the system. In the past, intact or single linear fracture rock samples were used in the study of fracture spontaneous imbibition, but in tight oil reservoirs, the fractures are considered as fracture networks. There have been limited studies on the spontaneous imbibition into tectonic and bedding fractures in tight reservoirs. For tight reservoirs, the developed bedding and tectonic fractures are very different in genesis and structure. As an important flow channel in tight reservoirs, attention should be paid to bedding fractures (Zhang et al., 2017). During simple burial and diagenesis of sediments, fractures will be near-horizontal and parallel to the original bedding (texture) (Zeng et al., 2008). These are mainly developed in argillaceous rocks, clastic rocks, and turbidite. Tectonic fractures are formed under the interaction of different tectonic stress fields and usually have high dip angles (Zeng and Li, 2009). At present, the horizontal well and multi-stage hydraulic fracturing method are used to artificially create more fractures to open up tight oil reservoirs and produce oil (Langford et al., 2013; Kim et al., 2015). However, for tight reservoirs with undeveloped tectonic fractures, it is challenging for hydraulic fracturing to re-open the pre-existing limited fractures and hydraulic fractures to form a large volume of fracture network. The bedding fracture is easy to open and has significant lateral extension (Zeng et al., 2008), making it an important channel for oil and gas flow (Wang et al., 2016). Therefore, for the tight reservoirs with developed bedding fractures but no tectonic fractures, it is important to understand the mechanism of imbibition and replacement of the fluid in both the bedding fracture and in the matrix porosity on either side of the bedding fracture and to explore and confirm the imbibition capacity and efficiency of the rock.
In this study, we first systematically identify bedding and tectonic fractures of the sandstones from the Jimusar Sag in NW China, and then we study the imbibition properties of imbibition efficiency, imbibition curve, and imbibition rate, etc. of these fracture types at different scales and conditions. The differences in imbibition effects between bedding and tectonic fractures are documented, and the types of imbibition and main controlling factors of bedding fractures are further discussed. The purpose of this article, therefore, is to explore whether fracturing fluid in the ubiquitous bedding fractures can be imbibed and replace crude oil in the reservoir and to compare the difference in imbibition efficiency between bedding and tectonic fractures, so as to provide a theoretical basis to aid in the development and production of tight oil.
Spontaneous imbibition was first reported by Moore and Slobod (1956). For hydrophilic rocks containing oil and water, the wetting phase is water, and the non-wetting phase is oil. Under constant external displacement pressures, a capillary force is generated at the boundary of the two phases due to wettability difference, inducing spontaneous imbibition, which makes aqueous fluids (either formation water or fracturing fluid) replace oil (Jadhunandan and Morrow, 1991). The capillary force can be described by the Young–Laplace equation:
where Pc is the capillary pressure; σ is the interfacial tension between oil and water phases; θ is the contact angle; r is the pore radius. θ is related to reservoir wettability.
According to formula (1), only when the reservoir is water wet, can water be imbibed by the matrix and oil be displaced from the pores. This is because when the wettability changes from lipophilic to hydrophilic, the capillary pressure changes from negative to positive. In addition, if the reservoir is hydrophilic, the capillary pressure is inversely proportional to the capillary radius. Therefore, a low permeability reservoir has a larger spontaneous imbibition tendency when the throat radius decreases. Wettability is therefore a key factor.
Based on the different directions of the water phase entering the rock matrix and oil and gas discharging, imbibition can be divided into two types of consequent and reverse modes (Schechter et al., 1994). Consequent imbibition is dominated by gravity, and the imbibition direction of wetting phase water is the same as that of oil. Reverse imbibition is dominated by capillary force, and the direction of water entering the matrix is opposite to that of oil (Figure 1). In a tight reservoir with small pore throats (permeability <0.1 × 10−3μm2), large capillary force, and a high ratio of capillary force to gravity, the rock matrix is surrounded by fracturing fluid, and its imbibition process is reverse imbibition controlled by capillary force (Schechter et al., 1994).
FIGURE 1. Model diagram of imbibition mechanism. (A) consequence imbibition and (B) reverse imbibition.
Jimusar Sag is a secondary tectonic unit located on the eastern uplift of the Junggar Basin in NW China, and it occupies an area of 1,278 km2. Deposited on basement rocks consisting of Carboniferous folded strata, the sag is dustpan-shaped and features a high in the east and a low in the west without obvious boundary traits. It is adjacent to the Beisantai uplift in the northwest and southwest, the Shaqi uplift in the northeast, with the Fukang fault zone to the south, and a transitional slope rising to the Guxi uplift in the east (Cao et al., 2016, Figure 2).
FIGURE 2. Location (A), Structural setting (B) and stratigraphic column (C) of Jimusar Sag. The middle Permian Lucaogou Formation (P2l) is the tight reservoir of this study.
Jimusar Sag is a typical multi-cycle lacustrine sedimentary basin. Since the Permian, it has experienced four stages of tectonic movement (Gao et al., 2016). The late Hercynian tectonic movement and Yanshan tectonic movement played an important role in the formation of large-scale shale oil and gas reservoirs in the study area. During the late-middle Permian period, a lacustrine facies was deposited known as the Lucaogou Formation, which is now the main tight and shale reservoirs of oil production focus in the study area. In 2010, the flow of oil from tight reservoirs in the Lucaogou Formation was obtained for the first time in well J23 (Zou et al., 2015). In 2017, high oil flow (flow rate >100 t/d) was achieved in JHW025 and JHW023 (Yang and Zhang, 2019). The tight reservoir in the Lucaogou Formation is thicker in the southwest and thinner in the northeast, with a thickness of 200–350 m.
The lithology of reservoirs in the study area is between sandstone and shale. There are two sweet spots of tight reservoir development in the Lucaogou Formation (Figure 3). Sweet spot interval 1 (upper sweet spot) is located in the second member (P2l22), and its lithology is mainly composed of gray doloarenite, feldspathic siltstone, lithic siltstone, and dolomitic sandstone, intercalated with gray mudstone and dolomitic mudstone. This sweet spot is mainly developed on the eastern slope of the sag, with a thickness range of 13–43 m and an average of about 33 m. Sweet spot interval 2 (lower sweet spot) is located in the first member (P2l12), and its lithology is mainly composed of gray dolomitic siltstone, intercalated with gray mudstone or gray dolomitic siltstone, and light gray mudstone interbedded with argillaceous siltstone. The thickness of the lower sweet spot developed in the whole sag is 17–68 m with an average of 43 m.
The Lucaogou Formation has a large unconventional resource potential, and oil reserves are estimated to be 11.12 × 108t (Du et al., 2019). Lucaogou Formation has a tight reservoir with a low-pressure gradient of about 1.05–1.20 MPa/km (Wu et al., 2014). The permeability of the two sweet spot sections in the study area is generally less than 0.1mD (Figure 4A). According to the influence curve of permeability on imbibition and displacement (Figure 4B), the oil recovery mode of the sweet spot section in the study area should be mainly imbibition oil recovery. The Lucaogou Formation developed abundant bedding fractures with a linear density of more than 3.4 lines/m, which seriously restricts the extension of tectonic fractures opened via fracturing.
FIGURE 4. (A) permeability frequency distribution plot of Lucaogou Formation in Jimusar Sag and (B) effect of permeability on imbibition and displacement (modified after Wang (2019)).
The study samples are from the middle Permian Lucaogou Formation (P2l) sweet spot section of well JHW043 (about 2,920 m in depth). The lithology is gray-white argillaceous silt with well-developed lamina. The porosity of the two core samples of group A and group B is 14.5 and 5.9%, respectively. The core samples were drilled vertically and horizontally. The cores were drilled vertically to the bedding for group V and parallel to the bedding for group H. The upper and lower bottom surfaces of group V cylinders are the simulated bedding fractures, and the upper and lower bottom surfaces of group H are the simulated tectonic fractures (Supplementary Figure S1). The relevant physical parameters are shown in Table 1. The plunger sample is wrapped by a Φ25 mm heat-shrinkable tube to separate the core side from the environment. Only the upper and lower bottom surfaces (simulating the surfaces of the bedding and tectonic fractures) contact the fracturing fluid during the experiment.
TABLE 1. Porosity and permeability data table of the outcrop samples of the Lucaogou Formation in the study area.
Two thin sections of R-A and R-B were made by using the aforementioned two plunger sample scraps for wettability testing (Figure 5). The test results show that the wetting angle of group A sample is 76°, showing weak hydrophilicity, and that of group B sample is 42°, showing hydrophilicity.
The small-scale cuboid sample is made by using the gray-white argillaceous silt samples of the core in the same well section (Supplementary Figure S1). Four samples are taken from group A with well-developed texture and permeability of 0.5–3.5 μD, including one bedding fracture sample (fracture area/volume is 2 cm2/1 cm3), two tectonic fracture samples (fracture area/volume are 2 cm2/1 cm3 and 4 cm2/1 cm3, respectively), and one fully exposed core sample (bedding fracture area is 2 cm2, tectonic fracture area is 4 cm2, and volume is 1 cm3). The mass of the sample was continuously weighed by the mass method, indicating the imbibition reaction process in a dynamic manner. This group of samples has a high degree of saturated oil, and the difference of imbibition effect for bedding and tectonic fractures is indicated by the comparison of multiple groups of experiments. However, because the exact porosity of the sample is unknown, an accurate imbibition efficiency cannot be obtained.
The large-scale test sample is from the gray-white tight sandstone outcrop in Baoming Mining Industries. The measured porosity is about 3.6%, the permeability of parallel bedding fracture is 1.3 μD, and that of vertical bedding fracture is 0.7 μD. The sample was cut into four cuboid cores of 10 × 8 × 2 cm. First, the core was vacuumized for 72 h and then saturated with simulated oil (the ratio of crude oil to kerosene is 1:4) at 27 MPa and 80°C under high pressure for 72 h, and the mass basically does not change further. By weighing the mass before and after the reaction, the calculated saturation degree is about 91%. Four cores were spliced into two groups of samples. Holes were drilled, electrodes were buried, water inlet and outlet holes were drilled at the designated positions to make a waterproof layer, and epoxy resin was used for cementing. After 48 h, the epoxy resin was completely solidified, and then the sample was connected with the instrument with a soldering gun. The waterproof layer was made, and the sample was ready. The schematic diagram of the sample is shown in Supplementary Figure S1.
Currently, there are three kinds of physical laboratory simulation methods for imbibition experiments: volume, mass, and micro-experiment methods. Among them, the volume method and the mass method can be used to study the impact of different parameters on the overall imbibition of the core, which is mainly the qualitative description of the experimental results. Micro-experiment methods, including computed tomography (CT) and magnetic resonance images (MRI), can be used to explore the impact of the internal microstructure of rock matrix on the imbibition effect, as well as the characteristics such as the change law of oil-water flow pattern and spatial distribution in the process of imbibition, which is a qualitative description of the experimental process. With the further development of unconventional oil and gas resources, it is difficult to solve new problems by using the traditional experimental means and methods. Therefore, based on the conventional methods of imbibition experiment, it is necessary to further improve and innovate appropriate experimental methods.
The principle of the mass method is that the oil-bearing core is suspended in the wetting phase fluid container. In the vertical direction, gravity is downward, the buoyancy and added tension are upward, and the three forces are balanced. As a result of imbibition, the wetting phase fluid (deionized water) with higher density replaces the oil phase with lower density in the core, which makes the core density increase, while the core volume remains unchanged. Therefore, the buoyancy remains unchanged. The increased value of added tension force ΔF is equal to the increment of density ΔG. The change in tension force can be expressed by the change in electronic scale reading. This method is very accurate, and data are obtained at any time point in the imbibition process.
In this study, a high-precision scale (model: Shimadzu AUW120D, accuracy: 0.000001 g) was used. Measurements of the mass were recorded every minute. Due to the density difference between water and oil, the rock sample imbibes water and discharges oil, so the mass gradually increases. The calculation formula of imbibition recovery efficiency R (imbibition efficiency) is as follows:
where Δm is the incremental mass of the rock sample at time t, g; ρw is the density of the wetting phase, g/cm3; ρo is the density of oil, g/cm3; Vo is the volume of saturated oil of rock sample, cm3.
The experimental process is as follows: oil drainage, drying, measuring porosity and permeability, weighing dry weight, oil saturation, weighing wet weight, wrapping the sides of plunger sample tightly with heat-shrinkable tube (to control fracture type), putting into HT and HP reaction vessel to simulate oil-water imbibition displacement process, the sample is taken out and weighed at certain intervals, and the mass data are recorded and tabulated. The experimental conditions were as follows: the pressure was 30 MPa and the temperature was 80–100°C. This experiment can accurately measure the sample porosity, calculate the pore space (i.e., the buoyancy method; finding detail in Qiao et al. (2020)), and then calculate the imbibition efficiency by weight gain. However, the core weighing error of this method is large, the imbibition rate is fast in the initial stage, and the mass of the core changes rapidly. Some data may be lost by manual weighing.
In order to better simulate subsurface conditions, a set of HT and HP imbibition experiments were designed to carry out oil-water imbibition displacement. The change of resistivity between 15 pairs of electrodes reflects the change in oil saturation, which is the result of oil displacement at formation temperature and pressure. The inner diameter of the device is Ф470 mm × 120 mm, the maximum working pressure is 30 MPa, and the maximum working temperature is 150°C.
The sample is placed in a pressure vessel which is then heated and pressurized to subsurface formation conditions of 27 MPa and 80°C. The fracturing fluid in the fracture interacts with the oil in the matrix by imbibition and displacement. As the imbibition proceeds, the weight of the sample increases gradually as the oil is replaced by water, and the resistance measured between the electrodes decreases gradually as the oil saturation of the sample declines. When the resistance vs. time curve flattens off, the imbibition is considered to have stopped. The imbibition efficiency is calculated from the change of mass before and after the imbibition.
Via the observation of rock thin sections of more than 30 wells and core scanning photos of more than 20 wells in the target formation located in the Jimusar Sag, the bedding and bedding fractures of Lucaogou Formation in Jimusar Sag are widely developed, and tectonic fractures are locally developed (Figure 6). Bedding fractures are generally located at the boundary between different lithologies and often run through the full diameter of the core. They are generally developed at a low angle close to horizontal, with narrow fracture widths, rough surfaces, and a relatively continuous nature. They are often not filled or only partially filled with mudstone, calcite, or bitumen. The density of bedding fractures is more than 10 lines/m. Most of the oil-saturated and oil-immersed sections are closely related to bedding fractures (Supplementary Figure S1A). The fractures in oil-bearing reservoirs are mostly characterized by dissolution, such as uneven bedding fractures, corrosion grooves, and even string bead phenomenon in the study area. Tectonic fractures are mainly of high angle, and a few are of medium and low angles. The length is beyond the centimeter level, and the opening is mostly less than 5 mm. Most of them are filled or semi-filled by calcite (Supplementary Figure S1B). The oil-bearing property is good, and most of the tectonic fractures are limited by bedding fractures or developed along with the bedding fractures (Supplementary Figure S1C). The linear density is generally less than 3–5 lines/m. From the perspective of oil accumulation, the contribution of tectonic fractures is less than that of bedding fractures because tectonic fractures are much less abundant than bedding fractures.
FIGURE 6. Relationship between dual lateral logging parameter r and fracture dip angle of Lucaogou Formation.
Two groups of cores A and B were made into thin sections along the vertical bedding direction (tectonic fracture section) and horizontal bedding direction (bedding fracture section). The thin section of rock shows that the lamina is thin, about 0.1–3 mm. The bedding is linear and parallel to each other, being horizontal bedding, reflecting the characteristics of weak hydrodynamics (Supplementary Figure S2). The opening of the bedding fracture is small, its extension range is not large with a bending shape and pinch-out. Generally, the length of the bedding fracture is between 1 and 1,000 μm and the width is between 1 and 5 μm. A minority of bedding fractures are filled with mud, calcite, and asphalt with a filling degree of about 20%. Dissolution pores are highly developed, accounting for about 65% of the total. However, the pores are not connected to microfractures, resulting in poor connectivity (Figure 7A). Consequently, the vertical permeability is lower than the horizontal permeability. Observation shows that the oil content in the horizontal section is less, and oil may be more easily lost along this direction, and the residual oil is distributed irregularly in the porosity in the form of being scattered (Figures 7B, C).
FIGURE 7. Characteristics of pore connectivity in vertical thin sections (A) and horizontal thin section (B) of tight sandstone and fluorescence characteristics of vertical section (C,E) and horizontal section (D,F) of tight sandstone.
In addition to bedding fractures, there are other types of fractures, such as tectonic and dissolution fractures, whose dimensions are greater than those of pore throat diameters and are reasonable oil migration channels (Su et al., 1991). The microfractures in the vertical section (tectonic fracture) are developed parallel to the bedding. The opening of the microfracture is about 10–20μm, and the length is about 200–400 μm. The porosity of the vertical thin section is basically consistent with that of the horizontal section, and a small number of residual intergranular pores can be observed under the microscope. The dissolution pores are mainly developed in shale rocks, indicating that they were formed by organic acid dissolution of calcite and feldspar. Neighboring dissolved pores form fractures and further form open bedding fractures visible to the naked eye. Some microfractures connect to form longer bedding fractures (Figure 7D). In the vertical section, the oil in the banded structure is distributed along with the lamina and bedding fractures. There is less oil in the fractures that are well-connected with the adjacent fractures, but more oil is found in the isolated pores and fractures (Figures 7E, F).
Based on the observation of the previous thin section, there is obvious anisotropy in the horizontal (bedding fracture section) and vertical (tectonic fracture section) directions. From the vertical section, the pores are long and narrow. The adjacent pores can be connected to form microfractures, even further to form bedding fractures, and oil is enriched in the pores and microfractures with good connectivity. From the direction of bedding fractures, the connectivity of pores is poor, and oil is distributed in the pores irregularly in a scatted manner. Therefore, it is easier for oil and gas to migrate into the tectonic fractures along with the horizontal narrow pores and microfractures, which indicates that there are obvious differences in oil migration and imbibition characteristics between bedding and tectonic fractures.
The control pressure of the imbibition experiment of this tight reservoir fracture was 30 MPa (wellhead pressure), and different formation conditions were simulated by setting different temperatures (80–100°C). The experimental results are consistent with those of other researchers (Gu et al., 2017; Li et al., 2018), i.e., under the condition of constant temperature, the imbibition reaction can be divided into three stages. The first stage consists of very rapid imbibition, the second stage is rapid imbibition, and the third stage is slow to no imbibition (Figures 8A, B). The shape of the imbibition curve in general mainly depends on the mineral composition, permeability, pore radius distribution, and pore space connectivity. In the initial stage of very rapid reaction, the imbibition rate of all samples is proportional to the square root of time, which is consistent with previous research results (Washburn, 1921; Handy, 1960), and the maximum imbibition rate is achieved at about 2 h, indicating that imbibition is mainly dominated by capillary forces in a low permeability core, and the influence of gravity can be ignored (Li and Horne 2000; Li et al., 2015). After that stage, the imbibition rate decreases rapidly. This stage is not significantly impacted by temperature. However, the straight line of imbibition rate vs time does not pass through the origin, mainly because the oil droplets ejected in the initial stage are distributed on the core surface. Therefore, the spontaneous imbibition rate was zero for the first hour in the beginning, and the oil volume measured is less than the actual spontaneous imbibition volume. Spontaneous imbibition recovery efficiency (R—Eq. 2) is suitable to describe the general influence of different factors on spontaneous imbibition. In the rapid reaction stage, the imbibition rate decreases slowly and then remains stable. With the increase in temperature, the rapid imbibition stage becomes shorter. In the slow to no imbibition stage, the imbibition rate decreases further. At high temperatures, this stage is significantly longer. At the same time, there is no obvious change law in different sample types in the experiment. At the same time, generally speaking, the imbibition rate of group B is significantly higher than that of group A, which may be due to the stronger hydrophilicity of group B. However, spontaneous imbibition is actually a complex process, involving quite a few factors such as porosity, absolute permeability, interfacial tension, pore size, fluid viscosity, and so on, and different curve shapes and different spontaneous imbibition recovery rates can be obtained even for cores with the same physical properties (Hu et al., 2020).
The imbibition efficiency is related to the imbibition rate and can be divided into three stages. However, the imbibition efficiency is more closely related to the properties of the sample itself. Both groups of samples show that the imbibition effect of tectonic fractures is about 6.7% higher than that of the bedding fracture sample (46.2% for the tectonic fracture sample and 43.2% for the bedding fracture sample) (Figure 8C), which may represent the difference in imbibition efficiency of different pore structures, i.e., high porosity and permeability are more conducive to imbibition efficiency, which is also consistent with previous research results (Sun et al., 2020). During the stage of the imbibition rate increase, the difference in imbibition efficiency of various samples is very small (Figure 8D). With the continuous infiltration of the osmotic medium, because of the weaker hydrophilicity of group A samples, the flow resistance of the liquid is greater than that of the samples of group B. The spontaneous imbibition of the samples of group A slows down, and the spontaneous imbibition of the samples of group B continues, which leads to the difference in imbibition efficiency in different samples. Finally, when the recovery efficiency is stable, the final imbibition effect of group B cores with lower porosity but stronger hydrophilicity is slightly higher than that of group A cores with higher porosity. The average imbibition effect increases by about 5.7% (Figures 8C, D). However, the total amount of oil imbibed is lower than that of group A. Therefore, it is considered that the amount of imbibition in cores with good porosity and permeability is large, but the imbibition reaction is not as thorough as that of cores with high hydrophilicity, i.e., the final imbibition efficiency is inversely proportional to porosity and permeability, and is directly proportional to hydrophilicity. Based on the previous research results, it is concluded that the hydrophilicity is more important than its porosity and permeability in our study samples.
Figures 8C, D show that the imbibition efficiency increases as the temperature rises. The reasons may be as follows: 1. The increase in temperature can reduce the adhesion work/droplets of oil film to the hole wall in porous media. With the increase in temperature, the interfacial tension and wettability decrease (Sun et al., 2020), which leads to a decrease in adhesion. The latter makes more oil driven by imbibition (Li, 2006). 2. An increase in temperature will also reduce the viscosity of the oil. This makes the flow resistance decrease when oil is discharged from porous media, which is beneficial to the oil drainage process (Sola et al., 2007). 3. The movable liquid increases at high temperatures. Under high temperatures, water and oil layers become thinner due to the more active molecular movement of water and oil (Wang et al., 2015). The effective diameter of pores increases, and the flow resistance decreases. This accelerates oil recovery by imbibition. Although the effect of increasing the temperature on improving imbibition is not obvious, it can greatly shorten the imbibition time (from nearly 50 to 30 h).
Figure 9A shows the variation in imbibition efficiency with time at room temperature and pressure for bedding fractures and tectonic fractures with different specific surface areas. The imbibition process can also be divided into three stages, i.e., very rapid imbibition, rapid imbibition, and slow to no imbibition. The poor imbibition quality of the unit volume core sample is about 6.5 × 10−3–8.0 × 10−3 g/cm3, i.e., the volume of oil imbibition and displacement per cubic centimeter core is about 0.0325–0.0400 ml, and the imbibition efficiency is about 20.1–26.2%. The cuboid samples also show the difference between tectonic and bedding fractures for imbibition. The imbibition termination time of tectonic fractures (20 h) is less than that of bedding fractures (40 h). The imbibition time can be greatly reduced (by about 15 h) by opening bedding and tectonic fractures (full exposure core imbibition) simultaneously. The imbibition efficiency of tectonic fractures (average imbibition efficiency is about 26.2%) is significantly higher than that of bedding fractures (20.1%), and the imbibition efficiency of bedding fractures is 77% of that of tectonic fractures. This difference is mainly due to the poor connectivity and poor capillary force of bedding fractures. The imbibition of the fully exposed core is between bedding and tectonic fractures, which indicates that it is the coupling of bedding and tectonic fractures. However, the increase in bedding fracture opening has no positive effect on the imbibition efficiency.
FIGURE 9. Variation of imbibition efficiency at normal temperature and pressure (A), resistance change in the fractures (B) and in rock (C), and changes of oil saturation at various measurement points in rocks (D).
In most experiments, the samples are usually immersed in a spontaneous imbibition fluid without confining pressure. Therefore, compared with the in situ stress state under formation conditions, the recovery efficiency of effective permeability resulting from the initiation and extension of microfractures may be overestimated. Moreover, the retained fluid still has high pressure, which provides a driving force for entering the matrix during immersion. In addition, the recovery of hydrocarbons is usually obtained by spontaneous imbibition experiments at ambient temperature. However, at formation temperature, the viscosity of the oil is lower and, as a result, the ability to flow is improved. Therefore, it is necessary to devise experiments that can combine in situ stress and temperature to simulate real reservoir conditions. The resistivity of oil-bearing rocks is determined by oil saturation (So), formation water resistivity, and rock physical parameters (Archie, 1950). By continuously measuring the resistance values at different positions of the two groups of samples, the resistivity variation curves (Figures 9B, C) can be converted into oil saturation (Table 2) with the Archie formula (Eq. 3). Based on the dynamic oil saturation curves (Figure 9D) dynamically measured by oil-water migration model software, the difference in imbibition oil recovery in bedding and tectonic fractures is studied.
where Rt is the resistivity of oil-bearing rock, Ω/m; R0 is the resistivity of water-bearing rock, Ω/m; SO is the oil saturation, %; rock-electro parameters b = 1, n = 2.
Imbibition at reservoir conditions was conducted for tight reservoir with a porosity of 3.6%, Kh = 1.3 μD, and Kv = 0.7 μD (27 MPa, 80°C). Physical simulation experiment of oil-water imbibition displacement efficiency of bedding fracture and bedding + tectonic fractures shows that the backflow fluid in fracture (including tectonic and bedding fractures) can imbibe and replace oil in the matrix. The closer to the fracture, the higher the displacement efficiency of the flow back fluid in the fracture to the oil in the matrix will be. The average efficiency of the single tectonic fracture model is 50.3%, and that of the bedding fracture opening increased model is 54.7%, showing the imbibition effect can be improved by 8.7% after the bedding fracture is opened based on the same scale of unit area tectonic fractures have been opened (Table 2). Under the tight condition, the imbibition rate of samples with low porosity (and low permeability) is higher than that of samples with high porosity (high permeability), but the total imbibition capacity is lower. The imbibition rate and efficiency of bedding fractures are slightly lower than those of tectonic fractures. Due to the difference between vertical and horizontal permeability, vertical permeability is much lower than horizontal permeability.
A tight reservoir is characterized by small pore throat diameters, high capillary forces, and a high ratio of capillary force to gravitational force. Tight reservoirs can be considered closed environments, and crude oil cannot diffuse outward. Therefore, its imbibition process is reverse imbibition controlled by capillary force.
Thin section data show that the reservoir of the Lucaogou Formation in the study area is well developed, and the horizontal pore connectivity is good (Figure 7). The framework of the imbibition model is constructed to simulate the imbibition effect of different fracture types for crude oil in the rock matrix. The imbibition pattern of bedding fractures shows that the water phase enters the reservoir matrix along the vertical pore throat and displaces the crude oil therein (Figure 10A). Based on the pattern, it can be seen that the pore throat of the channel of bedding fracture imbibition is narrow, and the path of water phase entry and oil phase discharge is long and tortuous. Due to the influence of compaction and cementation, the upper part of the throat in the longitudinal direction is flattened, and the pore throat decreases or even disappears, forming a closure, resulting in the existence of stagnant pores in the bedding fracture imbibition.
FIGURE 10. Imbibition pattern of bedding fractures (A) and of tectonic fractures (B) and gravity (buoyancy) effect on imbibition response (C) and reverse imbibition pressure in the lower part of rock matrix (D).
The imbibition pattern of tectonic fractures shows that the water phase enters the reservoir along the bedding direction to replace the oil phase therein (Figure 10B). The result indicates that the imbibition reaction mainly occurs in the horizontal pores with good connectivity, the reaction channels are straight, and the main channels of imbibition are pores or microfractures in the bedding direction. The stagnant pores which cannot participate in the imbibition reaction of bedding fractures can still participate in the imbibition reaction of tectonic fractures. For imbibition in tectonic fractures, if there are fairly wide microfractures or bedding fractures (Figure 10C), the water phase in the left tectonic fractures cannot overcome the gravity effect under the condition of the small capillary force, therefore, the imbibition reaction will not occur; while the water phase in the tectonic fractures on the right can replace the crude oil in the microfractures or bedding fractures because the capillary force and gravity are in the same direction. As shown in Figure 10D, due to the imbibition from the lower part of the matrix into the deep core, the replaced oil droplets are difficult to discharge due to the combined action of capillary force and gravity, resulting in the imbibition reaction being unable to further enter the deep matrix.
Comparing the imbibition patterns of bedding and tectonic fractures, it can be found that the imbibition channel of bedding fracture is more tortuous, but there is no significant difference in imbibition time between bedding and tectonic fractures due to its narrow throat and large capillary force. Compared with the tectonic fractures, there are some stagnant pores in the bedding fractures, which leads to the final imbibition efficiency of the bedding fractures being lower than that of the tectonic fractures. The higher the linear density of bedding fractures is, the higher the imbibition reaction efficiency is and the more thorough the reaction will be.
It shall be emphasized that under the setting of a tight reservoir, the influence of permeability on imbibition needs to be weakened, i.e., it shall not be over-emphasized that the smaller the permeability, the smaller the pore throat, the stronger the imbibition. Critical factors such as curvature of pore throat and reservoir wettability, etc., shall also be considered. Taking the tight oil reservoir of the Lucaogou Formation in Jimusar Sag as an example, due to the overall lipophilicity of the reservoir, the pore throat radius of the reservoir is small, the capillary force is large, and the effect of imbibition is good.
In this study, the difference in imbibition between bedding and tectonic fractures only lies in the difference of pore throat structure in horizontal and vertical directions and the gravity effect caused by the oil-water density difference. The time required for imbibition of bedding fracture is not significantly different from that of tectonic fractures, and the depth and efficiency of imbibition are slightly lower than those of tectonic fractures.
(1) The tectonic fractures of the Lucaogou Formation in Jimusar Sag are locally developed while the bedding fractures are widely developed. The linear density of bedding fractures can be 2.0–4.5 lines/m, which is 4–5 times the linear density of tectonic fractures.
(2) Under the condition of normal temperature and pressure, the imbibition efficiency of bedding fracture is 20.1%, and the imbibition depth is about 1.0 cm. The imbibition efficiency of tectonic fractures is about 26.2%, and the imbibition depth is about 1.7 cm. The imbibition efficiency of bedding fracture is about 76.7% of that of tectonic fractures; under the condition of simulated formation temperature and pressure, the imbibition efficiency of the bedding fracture sample is about 43.2%, and that of tectonic fractures is about 46.2%. The imbibition efficiency of bedding fractures is about 93.4% of that of tectonic fractures. The reaction time can be shortened if the temperature is higher.
(3) Under the simulated formation temperature and pressure (80°C, 100 MPa), the opening of bedding fractures can increase the imbibition efficiency of the reservoir by 8.7% based on the same scale of opened tectonic fractures, and the closer to the fracture, the higher the imbibition efficiency.
(4) The per unit volume amount of imbibition in cores with good porosity and permeability is large, but the imbibition reaction is not as thorough as that of cores with high hydrophilicity, i.e., the final imbibition efficiency is inversely proportional to the porosity and permeability and is directly proportional to hydrophilicity.
The original contributions presented in the study are included in the article/Supplementary Material; further inquiries can be directed to the corresponding author.
CL and AL presented the idea and design of the research. CL and AL wrote the article and led the data analysis and interpreted the results with QL, JL, DL, and PL.
This work is financially supported by the grants from the National Natural Science Foundation of China (No. 41502209), the Natural Science Foundation of Hebei Province (D2019403041), National first-class undergraduate course “sedimentary rock petrology” (2020 No. 8, 2020 No. 85), Provincial educational reform projects (2020GJJG589, 2020J03), and Provincial postgraduate demonstration course “principles of sedimentology” (kcjsx2021092).
Author CL was employed by Jiqing Oilfield Operation Area of Xinjiang Oilfield Company.
The remaining authors declare that the research was conducted in the absence of any commercial or financial relationships that could be construed as a potential conflict of interest.
All claims expressed in this article are solely those of the authors and do not necessarily represent those of their affiliated organizations, or those of the publisher, the editors, and the reviewers. Any product that may be evaluated in this article, or claim that may be made by its manufacturer, is not guaranteed or endorsed by the publisher.
The Supplementary Material for this article can be found online at: https://www.frontiersin.org/articles/10.3389/feart.2022.918244/full#supplementary-material.
Al-Attar, H. H. (2010). Experimental Study of Spontaneous Capillary Imbibition in Selected Carbonate Core Samples. J. Petroleum Sci. Eng. 70 (3), 320–326. doi:10.1016/j.petrol.2009.12.003
Babadagli, T. (2000). Efficiency of Capillary Imbibition Dominated Displacement of Nonwetting Phase by Wetting Phase in Fractured Porous Media. Transp. porous media 40 (3), 323–344. doi:10.1023/A:1006777703905
Babadagli, T. (2000). Scaling of Co-current and Counter-current Capillary Imbibition for Surfactant and Polymer Injection in Naturally Fractured Reservoirs. SPE J. 6 (4), 465–478. doi:10.2118/62848-MS
Cai, J., Li, C., Song, K., Zou, S., Yang, Z., Shen, Y., et al. (2020). The Influence of Salinity and Mineral Components on Spontaneous Imbibition in Tight Sandstone. Fuel 269, 117087. doi:10.1016/j.fuel.2020.117087
Cao, Z., Liu, G., Kong, Y., Wang, C., Niu, Z., Zhang, J., et al. (2016). Lacustrine Tight Oil Accumulation Characteristics: Permian Lucaogou Formation in Jimusaer Sag, Junggar Basin. Int. J. Coal Geol. 153, 37–51. doi:10.1016/j.coal.2015.11.004
Dai, C., Cheng, R., Sun, X., Liu, Y., Zhou, H., Wu, Y., et al. (2019). Oil Migration in Nanometer to Micrometer Sized Pores of Tight Oil Sandstone during Dynamic Surfactant Imbibition with Online NMR. Fuel 245, 544–553. doi:10.1016/j.fuel.2019.01.021
Du, J., Hu, S., Pang, Z., Ling, S., Hou, L., and Zhu, R. (2019). The Types, Potentials and Prospects of Continental Shale Oil in China. China Pet. Explor. 24 (5), 560–568. doi:10.3969/j.issn.1672-7703.2019.05.003
Gao, G., Zhang, W., Xiang, B., Liu, G., and Ren, J. (2016). Geochemistry Characteristics and Hydrocarbon-Generating Potential of Lacustrine Source Rock in Lucaogou Formation of the Jimusaer Sag, Junggar Basin. J. Petroleum Sci. Eng. 145, 168–182. doi:10.1016/j.petrol.2016.03.023
G. E. Archie, G. E. (1950). Introduction to Petrophysics of Reservoir Rocks. Bulletin 34 (5), 943–961. doi:10.1306/3D933F62-16B1-11D7-8645000102C1865D
Gu, X., Pu, C., Huang, H., Huang, F., Li, Y., Liu, Y., et al. (2017). Micro-influencing Mechanism of Permeability on Spontaneous Imbibition Recovery for Tight Sandstone Reservoirs. Petroleum Explor. Dev. 44 (6), 1003–1009. doi:10.1016/S1876-3804(17)30112-X
Handy, L. L. (1960). Determination of Effective Capillary Pressures for Porous Media from Imbibition Data. SPE J. 219, 75–80. doi:10.1080/1478643600823834510.2118/1361-g
Hu, Y., Zhao, C., Zhao, J., Wang, Q., Zhao, J., Gao, D., et al. (2020). Mechanisms of Fracturing Fluid Spontaneous Imbibition Behavior in Shale Reservoir: A Review. J. Nat. Gas Sci. Eng. 82, 103498. doi:10.1016/j.jngse.2020.103498
Jadhunandan, P. P., and Morrow, N. R. (1991). Spontaneous Imbibition of Water by Crude Oil Brine Rock Systems. Situ 15 (4), 319–345. doi:10.1016/0016-2361(91)90109-N
Jing, W., Huiqing, L., Genbao, Q., Yongcan, P., and Yang, G. (2019a). Investigations on Spontaneous Imbibition and the Influencing Factors in Tight Oil Reservoirs. Fuel 236, 755–768. doi:10.1016/j.fuel.2018.09.053
Junira, A., Sepehrnoori, K., Biancardi, S., Ambrose, R., Yu, W., and Ganjdanesh, R. (2020). Optimization of Huff-N-Puff Field Gas Enhanced Oil Recovery through a Vertical Well with Multiple Fractures in a Low-Permeability Shale-Sand-Carbonate Reservoir. Energy fuels. 34 (11), 13822–13836. doi:10.1021/acs.energyfuels.0c02606
Kathel, P., and Mohanty, K. K. (2013). Wettability Alteration in a Tight Oil Reservoir. Energy fuels. 27, 6460–6468. doi:10.1021/ef4012752
Kim, J., Chun, M., Jung, W., Park, H., and Sung, W. (2015). Optimum Design of Multi-Stage Hydraulically Fractured Multi-Horizontal Shale Gas Well Using Flow Regime Analysis. Geosci. J. 19 (3), 481–487. doi:10.1007/s12303-014-0058-y
Lai, F., Li, Z., Zhang, T., Zhou, A., and Gong, B. (2019). Characteristics of Microscopic Pore Structure and its Influence on Spontaneous Imbibition of Tight Gas Reservoir in the Ordos Basin, China. J. Petroleum Sci. Eng. 172, 23–31. doi:10.1016/j.petrol.2018.09.020
Langford, M., Holland, B., Green, C. A., Bocaneala, B., and Norris, M. (2013). “Offshore Horizontal Well Fracturing: Operational Optimisation in the Southern North Sea,” in SPE Offshore Europe Oil and Gas Conference and Exhibition (Aberdeen, United Kingdom: Society of Petroleum Engineers). doi:10.2118/166550-MS
Li, J. (2006). The Influence of Surfactant System on the Process of Imbibition. Beijing: Graduate School of Chinese Academy of Sciences.
Li, K., and Horne, R. N. (2000). “Characterization of Spontaneous Water Imbibition into Gas-Saturated Rocks,” in SPE/AAPG Western Regional Meeting, 19-22 June, Long Beach, California 6, 375–384. (SPE-62552-MS). doi:10.2118/74703-PA
Li, M., Yang, H. e., Lu, H., Wu, T., Zhou, D., and Liu, Y. (2018). Investigation into the Classification of Tight Sandstone Reservoirs via Imbibition Characteristics. Energies 11 (10), 2619. doi:10.3390/en11102619
Lianbo, Z., and Xiang-Yang, L. (2009). Fractures in Sandstone Reservoirs with Ultra-low Permeability: A Case Study of the Upper Triassic Yanchang Formation in the Ordos Basin, China. Bulletin 93 (4), 461–477. doi:10.1306/09240808047
Liu, X., and Dai, C. (2008). Experimental Study of Imbibition Law in Low Permeability Sandstone Oilfield. Drill. Prod. Technol. 31 (6), 110–112.
Mason, G., Fischer, H., Morrow, N. R., Ruth, D. W., and Wo, S. (2009). Effect of Sample Shape on Counter-current Spontaneous Imbibition Production vs Time Curves. J. Petroleum Sci. Eng. 66, 83–97. doi:10.1016/j.petrol.2008.12.035
Mirzaei, M., and Dicarlo, D. (2013). Imbibition of Anionic Surfactant Solution into Oil-Wet Capillary Tubes. Transp. Porous Med. 99 (1), 37–54. doi:10.1007/s11242-013-0172-8
Moore, T. F., and Slobod, R. L. (1956). The Effect of Viscosity and Capillarity on the Displacementof Oil by Water. Prod. Mon. 20, 20–30. doi:10.1086/266630
Morrow, N. R., and Mason, G. (2001). Recovery of Oil by Spontaneous Imbibition. Curr. Opin. Colloid & Interface Sci. 6 (4), 321–337. doi:10.1016/s1359-0294(01)00100-5
Peng, Y., He, S., He, S., and Han, D. (2010). Effect of Permeability of Porous Medium on Spontaneous Imbibition in Water-Wet Sandstone. J. Daqing Pet. Inst. 34 (4), 51–56.
Saberhosseini, S. E., Ahangari, K., and Mohammadrezaei, H. (2019). Optimization of the Horizontal-Well Multiple Hydraulic Fracturing Operation in a Low-Permeability Carbonate Reservoir Using Fully Coupled XFEM Model. Int. J. Rock Mech. Min. Sci. 114, 33–45. doi:10.1016/j.ijrmms.2018.09.007
Schechter, D. S., Zhou, D., and Orr, F. M. (1994). Low IFT Drainage and Imbibition. J. Petroleum Sci. Eng. 11 (4), 283–300. doi:10.1016/0920-4105(94)90047-7
Sedaee Sola, B., Rashidi, F., and Babadagli, T. (2007). Temperature Effects on the Heavy Oil/water Relative Permeabilities of Carbonate Rocks. J. Petroleum Sci. Eng. 59 (1), 27–42. doi:10.1016/j.petrol.2007.02.005
Standnes, D. C., and Chun, D. (2004). Experimental Study of the Impact of Boundary Conditions on Oil Recovery by Co-current and Counter-current Spontaneous Imbibition. Energy fuels. 18 (1), 271–282. doi:10.1021/ef030142p
Su, A., Cheng, K., and Jin, W. (1991). Application of Fluorescent Flake Analysis in the Study of Primary Oil and Gas Migration. Petroleum Explor. Dev. 6, 19–24.
Sun, Y., Gao, K., Yu, Z., Fang, Y., Chang, Y., Chen, A., et al. (202010739). Dynamic Imbibition with Aid of Surfactant in Tight Oil Fracture Network Model. J. Petroleum Sci. Eng. 193, 107393. doi:10.1016/j.petrol.2020.107393
Wang, F., Yang, K., You, J., and Lei, X. (2019b). Analysis of Pore Size Distribution and Fractal Dimension in Tight Sandstone with Mercury Intrusion Porosimetry. Results Phys. 13, 102283. doi:10.1016/j.rinp.2019.102283
Wang, H., Rezaee, R., and Saeedi, A. (2015). Evaporation Process and Pore Size Distribution in Tight Sandstones: a Study Using NMR and MICP. Procedia Earth Planet. Sci. 15, 767–773. doi:10.1016/j.proeps.2015.08.124
Wang, R., Ding, W., Zhang, Y., Wang, Z., Wang, X., He, J., et al. (2016). Analysis of Developmental Characteristics and Dominant Factors of Fractures in Lower Cambrian Marine Shale Reservoirs: A Case Study of Niutitang Formation in Cen'gong Block, Southern China. J. Petroleum Sci. Eng. 138, 31–49. doi:10.1016/j.petrol.2015.12.004
Wang, X. (2019). Upstream Development Strategy and Recent Exploration Progress of Yanchang Group. Beijing: The 8th Annual China Petroleum Geology Conference.
Washburn, E. W. (1921). The Dynamics of Capillary Flow. Phys. Rev. 17, 273–283. doi:10.1103/PhysRev.17.273
Wei, Q., Li, Z., Wang, X., Bai, R., Cai, W., and Hong, L. (2016). Mechanism and Influence Factors of Imbibition in Fractured Tight Sandstone Reservoir: An Example from Chang 8 Reservoir of Wuqi Area in Ordos Basin. Pet. Geol. Recovery Eff. 23 (4), 102–107. doi:10.13673/j.cnki.cn37-1359/te.2016.04.016
Wu, C., Guo, Z., and Tang, F. (2014). Early Exploitation Characteristics of Lucaogou Tight Oil of Permian in Jimusaer Sag, Junggar Basin. Xinjiang Pet. Geol. 35 (5), 570–573.
Yang, R., and Zhang, T. (2019). Horizontal Well Drilling Technology for Shale Oil in Lusaogou Formation, Jimusar Depression. Xinjiang Petroleum Nat. Gas 15 (3), 36–40.
Yang, Z., Liu, X., Li, H., Lei, Q., Luo, Y., and Wang, X. (2019). Analysis on the Influencing Factors of Imbibition and the Effect Evaluation of Imbibition in Tight Reservoirs. Petroleum Explor. Dev. 46 (4), 779–785. doi:10.1016/S1876-3804(19)60235-1
Yildiz, H. O., Gokmen, M., and Cesur, Y. (2006). Effect of Shape Factor, Characteristic Length, and Boundary Conditions on Spontaneous Imbibition. J. Petroleum Sci. Eng. 53 (3), 158–170. doi:10.1016/j.petrol.2006.06.002
Zeng, L., Gao, C., Qi, J., Wang, Y., Li, L., and Qu, X. (2008). The Distribution Rule and Seepage Effect of the Fractures in the Ultra-low Permeability Sandstone Reservoir in East Gansu Province, Ordos Basin. Sci. China Ser. D-Earth Sci. 51 (2), 44–52. doi:10.1007/s11430-008-6015-8
Zhang, C., Zhu, D., Luo, Q., Liu, L., Liu, D., Yan, L., et al. (2017). Major Factors Controlling Fracture Development in the Middle Permian Lucaogou Formation Tight Oil Reservoir, Junggar Basin, NW China. J. Asian Earth Sci. 146, 279–295. doi:10.1016/j.jseaes.2017.04.032
Zhao, M., He, H., Dai, C., Sun, Y., Fang, Y., Liu, Y., You, Q., Zhao, G., and Wu, Y. (2018). Enhanced Oil Recovery Study of a New Mobility Control System on the Dynamic Imbibition in a Tight Oil Fracture Network Model. Energy fuels. 32 (3), 2908–2915. doi:10.1021/acs.energyfuels.7b03283
Zhou, X., Morrow, N. R., and Ma, S. (2000). Interrelationship of Wettability, Initial Water Saturation, Aging Time, and Oil Recovery by Spontaneous Imbibition and Waterflooding, Aging Time, and Oil Recovery by Spontaneous Imbibition and Waterflooding. SPE J. 5, 199–207. doi:10.2118/35436-MS10.2118/62507-pa
Zhou, Z., Teklu, T., Li, X., and Hazim, A. (2018). Experimental Study of the Osmotic Effect on Shale Matrix Imbibition Process in Gas Reservoirs. J. Nat. Gas Sci. Eng. 49, 1–7. doi:10.1016/j.jngse.2017.10.005
Keywords: spontaneous imbibition, capillary force, bedding fracture, tectonic fracture, middle Permian Lucaogou Formation
Citation: Liang C, Liu A, Luo Q, Luo J, Li D and Li P (2022) Differences in Imbibition Efficiency Between Bedding and Tectonic Fractures in the Lucaogou Formation in the Jimusar Sag: Evidence From Simulation Experiments. Front. Earth Sci. 10:918244. doi: 10.3389/feart.2022.918244
Received: 12 April 2022; Accepted: 25 May 2022;
Published: 07 July 2022.
Edited by:
Chen Zhang, Chengdu University of Technology, ChinaReviewed by:
Liangwei Xu, Peking University, ChinaCopyright © 2022 Liang, Liu, Luo, Luo, Li and Li. This is an open-access article distributed under the terms of the Creative Commons Attribution License (CC BY). The use, distribution or reproduction in other forums is permitted, provided the original author(s) and the copyright owner(s) are credited and that the original publication in this journal is cited, in accordance with accepted academic practice. No use, distribution or reproduction is permitted which does not comply with these terms.
*Correspondence: Aoran Liu, YW9yYW5saXVAY3VnLmVkdS5jbg==
Disclaimer: All claims expressed in this article are solely those of the authors and do not necessarily represent those of their affiliated organizations, or those of the publisher, the editors and the reviewers. Any product that may be evaluated in this article or claim that may be made by its manufacturer is not guaranteed or endorsed by the publisher.
Research integrity at Frontiers
Learn more about the work of our research integrity team to safeguard the quality of each article we publish.