- 1Department of Civil, Structural and Environmental Engineering, University of Dublin Trinity College, Dublin, Ireland
- 2Scientific Unit, National Parks and Wildlife Service, Dublin, Ireland
- 3Department of Geology and Trinity Centre for the Environment, University of Dublin Trinity College, Dublin, Ireland
Calcareous fens are peat-wetlands fed mainly by groundwater, located in topographic hollows and served by springs or seepages of water derived by contact with base-rich mineral ground. In the European Union they are protected habitats requiring special conservation measures. To better understand the environmental supporting conditions of alkaline fen habitat found in Ireland, a 2-years hydrological and hydrochemical monitoring programme was carried out on four contrasting fen sites that covered an ecohydrological gradient representing intact to highly degraded conditions. This paper presents a methodology (and its limitations) of how the evaluation of the temporal and spatial variability of the quality and hydrological dynamics associated with different representative vegetation communities within the four fens can be used to develop ecohydrological metrics for fen habitat. The conditions supporting fen habitat in “good” ecological condition, indicative of a functioning alkaline habitat, were determined and contrasted with conditions found in habitats deemed to be in “poor” ecological condition. Results indicate that an annual water level always above the ground surface within a threshold depth envelope of between 0.030 and 0.28 m is required for at least 60% of the year for healthy fen vegetation. In terms of hydrochemistry, higher concentrations of nutrients were found in the sediments at depth and the groundwater feed compared to the phreatic water table. This suggests the fens may be incorporating the incoming nutrients into their internal biogeochemical cycles and that there is a net accumulation of nutrients within the wetlands. Although envelopes of nutrient concentrations in the surface water of the fen associated with habitat in good ecological condition can be defined (e.g., dissolved reactive phosphorus concentrations of between 6 and 37 µg-P/l for PF1 habitat), these levels should be regarded as the water quality after the fen vegetation has effectively interacted with the higher incoming nutrient levels in the groundwater. Moreover, the local hydrogeological conditions that define groundwater pathways (and associated water quality impacts) in such calcareous fens, allied to the localized nature of these measurements in these field studies, makes it difficult to define groundwater water quality threshold values with any confidence.
Introduction
Wetlands are among the most productive ecosystems in the world (de Groot et al., 2012; USEPA, 2018) and provide many ecosystem services such as climate regulation, hotspots of biodiversity, water purification, flood protection, recreation, and ecotourism (Millennium Ecosystem Assessment, 2005; Barbier, 2011). However, despite efforts to restore degraded wetlands, it is estimated that over two-thirds of European wetlands have been lost in the past 100 years due to regulation and drainage (European Commission, 1995; Zedler and Kercher, 2005). Accurate methods of assessing the ecological health of such wetlands, therefore, need to be developed which can then support appropriate management decisions for their conservation and protection with respect to past, current, and future anthropogenic impacts, including the potential impacts of a changing global climate.
Fens are peat forming wetlands that are fed by groundwater as well as surface water and have a water table near or at the surface throughout the whole year (Kellner, 2003; McBride et al., 2011; Aggenbach et al., 2013). They are located in topographic depressions and are fed by springs or seepages of water that has been in contact with mineral ground (Proctor, 2010). Unlike bog habitats which are usually dominated by Sphagnum species, fens are generally more alkaline depending on the groundwater source and tend to be dominated by sedges and bryophytes (with the exception of base-poor fens). However, like bogs, fens accumulate peat. The composition of the fen vegetation reflects the chemical composition of the dominant water supply and the duration of a mean water level. The combination of these factors dictates what type of fen develops and its hydrological regime (McBride et al., 2011). A “poor” fen is defined by more acidic conditions with very low concentrations of nutrients with floristic similarities to a bog, while a “rich” fen has relatively higher mineral content, is usually more alkaline and hosts a more diverse plant and animal community. Poor fen is typically found within blanket bog environments and is also referred to as flushes or transitional laggs, but can lose contact with its groundwater feed due to peat accumulation, thereby transitioning into bog. Calcareous fens are listed in Annex 1 of the European Union (EU) Habitats Directive (Council Directive 92/43/EEC, habitat codes 7230, 7210, and 7140) as habitats requiring special conservation measures, including the designation of suitable sites as Special Areas of Conservation (SACs). Their principal source of nutrients is from surface or base-rich groundwater and the substrate is an alkaline to slightly acidic peat soil. The dramatic decline in the area of natural fens all over the world, and in particular the species-rich calcareous fens, has resulted in the loss of highly valuable habitats and related species. Fens are therefore now considered to be among the most threatened habitat types in Europe (Joosten and Clarke 2002; Klimkowska et al., 2010; Lamers et al., 2014).
Conceptual models of calcareous fens have been developed (Whiteman et al., 2009; Mitch and Gosselink, 2015) whereby climate and geology determine the landscape position and regulation of the hydrological regime, which in turn then controls the development of the physicochemical soil environment and its own microclimate. The water supply mechanisms and biogeochemistry determine the distribution and composition of plant communities that are capable of thriving in the habitat. Precipitation, evapotranspiration, surface and groundwater inflows and outflows create a hydroperiod which controls the fen water balance (Mitch and Gosselink, 2015). The groundwater discharge zone in calcareous fens results in a relatively stable annual water table, that is, continuously at or near the land surface, but never inundated for significant lengths of time (Duval et al., 2011; Sampath et al., 2015). Groundwater (which is higher in alkalinity than rainwater) can enter fens via different pathways. When surface soil and drift deposits (i.e., Quaternary sediments) are permeable, groundwater will flow into the overlying fen peat via diffuse upward discharge. In cases where the underlying bedrock is not permeable, groundwater will most likely discharge at the margins of soil or drift deposits through discrete springs or seepages. In reality, most fens receive groundwater through a combination of these two mechanisms, (McBride et al., 2011). Even though fens should generally be situated in geological settings that prevent them from dying out, thus helping their resilience, many are still especially sensitive to relatively small changes in the hydrological cycle due to the often low capacity of groundwater storage in their supporting catchments (van Diggelen et al., 2006; Wheeler et al., 2009). The depth and variants in peat stratum within fens have a significant effect on mean vertical permeability. Different values of hydraulic conductivity by orders of magnitude may be found depending on the degree of decomposition of the peat and its thickness (Stofberg et al., 2016).
Fen biological diversity is not only controlled by groundwater flows and its chemistry (Stofberg et al., 2016) but it also relies on some sort of isolation from other surface waters (Amon et al., 2002) which implies fens are usually saturated by groundwater but rarely flooded by adjacent surface waters (Bedford and Godwin, 2003). Furthermore, the hydrological regime is controlled by different hydraulic gradients which vary between recharge and discharge zones in both groundwater and surface waters. These gradients may occur naturally or may have been created by anthropogenic interference at some point, such as dewatering associated with industrial activities (quarrying, mining etc.) land drainage or groundwater pumping for water supply. Nutrients can be derived from both natural and anthropogenic sources and transmitted via terrestrial and atmospheric pathways. More complex processes in fens that control fen vegetation make it a challenge to predict biotic reactions to fluctuations of the water table. For example, it has been found that the redox reactions involving nitrate, sulphate and phosphate in groundwater fed wetlands may support a chemical reaction where sulphate ions may also cause phosphorus release (Lucassen et al., 2004).
The presence or absence of a plant species can be used as a bioindicator and can provide information on the environmental conditions in the habitat in which it is found. One commonly used method in Europe of quantifying the relationship between indicator plant species and various environmental variables, for example, is the Ellenberg index (Ellenberg, 1988; Hill et al.,1999). The type of vegetation in wetlands and its location is intimately entwined with the hydrological conditions, and hence, these ecohydrological relationships need to be properly understood and evaluated, from which healthy envelopes/thresholds can then be defined for the different key wetland habitats (Eamus et al., 2006; Bertassello et al., 2019; Bhatnagar et al., 2021a). Such metrics can be used in order to assess the ecohydrological status of wetlands and monitor and manage their current and future existence. Regan et al. (2020), for example, have recently evaluated the ecohydrological envelope of different ecotopes on raised bogs in Ireland, using water table duration curves to show that active areas of Sphagnum growth occur where water tables are within 0.1 m of the ground surface for approximately 90% of a given year. Although fens are protected habitats requiring special conservation measures, and the literature highlights how land management can have a significant control on the hydrology in fens and the consequential quality of fen vegetation (van Diggelen et al., 2006; Wheeler et al., 2009; McBride et al., 2020), there appear to be very few studies of such wetland systems from which the environmental supporting conditions can be determined. The objective of this study, therefore, is to develop a methodology which can be used to investigate and better assess the hydrological and hydrochemical controls that support healthy Irish calcareous fen habitats. These insights can then be used to help to develop more rigorous conceptual models of the hydrological and hydrochemical functioning of these complex wetland systems.
Materials and methods
Study sites
The most recent estimate for the extent of Annex I fen habitat in Ireland is 27,257 ha (NPWS et al., 2019), of which 46% is alkaline fen, 26% is Cladium fen, 28% is transition mire and 0.05% is petrifying spring. Four calcareous fens in Ireland were selected, representing a range of different conditions with at least one fen wetland considered to be under water quantity pressure (i.e., either damaged or under pressure from drainage or abstraction), one site considered to be under water quality pressure (i.e., under pressure from nutrient pollution) and at least one site, that is, considered to still be in a relatively pristine (intact) state. The selection was achieved following an extensive review of fens in Ireland with information gathered from the Irish Peatland Conservation Council peatland sites database (IPCC, 2009), the SAC database from the NPWS (NPWS et al., 2019) and wetland survey reports for counties Kildare, Louth, Monaghan and Wicklow prepared by Foss (2007), in which fens were ranked against a scoring system of a range of different criteria, including designation status [Special Areas of Conservation (SAC), Natural Heritage Area (NHA) or none], Annex I Habitat type (7210—calcareous fens with Cladium mariscus and species of the Caricion davallianae and 7230—alkaline fens), underlying geology, geomorphology, water chemistry (base-rich/poor), damage, previous research data, threats, and pressures (water quality and/or quantity). Details about the four fens chosen for the field study are listed in Table 1, with their location shown in Supplementary Figure S1 (Supplementary Information). Note that because of its size, Pollardstown fen was spilt into two separate fieldwork areas for monitoring—sites A and D—as shown on Supplementary Figure S2. These were selected due to a previous research project on the fen that had been set up to assess the effect of a motorway construction on groundwater levels (Kuczynska, 2008; Misstear et al., 2009). Similarly, Tory Hill and Ballymore fens were selected due to previous investigations on the impact (or not) of marginal drainage activities on fen ecohydrology.
Vegetation surveys and community mapping
The fens were surveyed by different ecologists in order to find out what habitats occurred within the wetlands systems. The habitats of Scragh Bog and Pollardstown fens were surveyed by ecologists from BEC Consultants in 2019 (BEC, 2019). Tory Hill and Ballymore were surveyed by John Conaghan in 2015 and 2017 respectively (Regan and Conaghan, 2016; Regan and Conaghan, 2017). At each water level monitoring/sampling location (see Section 2.3), 2 m × 2 m relevés with plant species percentages were recorded next to the piezometer nests which were used to classify the vegetation communities present. The habitat maps for each site were used to assign the broad habitat type present at each relevé site based on the Fossitt habitat code (Fossitt, 2000), developed for Ireland which include PF1 (rich fen and flush), PF2 (poor fen and flush) and PF3 (transition mire and quaking bog) and NF referring to non-fen areas of vegetation. These were then mapped using ArcGIS® software, as shown in Figure 1. In parallel, a remote sensing, satellite-based study was carried out on the fens to develop a methodology to categorise fen communities using this ground truth field survey data (details in Bhatnagar et al., 2020; Bhatnagar et al., 2021b). Habitat quality was then determined using the draft condition assessment criteria and methodology set out in the Pilot Fen Survey 2019–20 (Perrin, 2021). Full details of the assessment criteria and fen quality assessments can be found in Supplementary Appendix B. This provides tables indicating how each relevé was assessed for ecological condition using the criteria with the result deemed to denote good or poor quality fen habitat condition respectively.
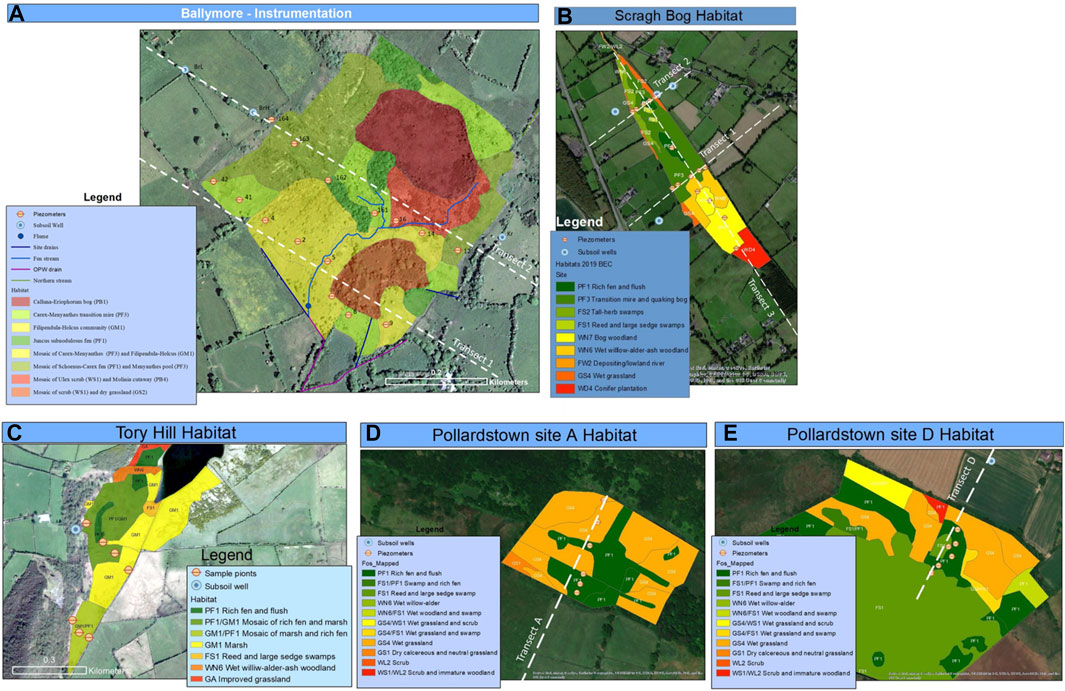
FIGURE 1. Habitat maps fens also showing the location of instrumentation transects (A) Ballymore (Regan and Connaghan, 2017) (B) Scragh Bog (BEC, 2019) (C) Tory Hill (Regan and Conaghan, 2016) (D) Pollardstown Site A (BEC, 2019) (E) Pollardstown Site D (BEC, 2019).
Hydrology
Fen piezometers were installed from 3 m up to 8 m depths across different transects across the fens to measure the groundwater pressure head at specific depths and to retrieve water quality samples. The piezometers (Stuart Well Services Ltd.) were constructed from PVC with a 45 cm long filter tip at the end. The filter tip was made of HDPE and had an engaged filter with an average pore diameter of approximately 60 µm and a permeability of approximately 3 × 10−4 m/sec. Holes were drilled to the depth just before the transition from peat and post glacial lacustrine sediments to glacial deposits, while recording the soil logs of the different layers. Phreatic tubes were also installed at the same locations to measure the free water table (and retrieve water quality samples). The phreatic tubes were made of 40 mm diameter PVC pipe and had horizontal slots of 3 mm every 2.5 cm on the section that was installed in the fen, encased in geosock material to ensure no particulate fines would enter the tube. The tops of each piezometer and phreatic tube were accurately levelled using a differential global positioning system (GPS) (Trimble 4700) with horizontal/vertical accuracy of 0.01 m and water levels were monitored manually using a Van Walt V025 dip meter (referenced against GPS points) at every field visit (approximately 6 weeks intervals). At selected locations continuous water level data were collected using pressure transducers (Van Essen Divers®) with in-built dataloggers to give a full time series of the water level fluctuations. The combination of piezometers and phreatic tubes enabled information to be gathered on downward or upward hydraulic gradients within the peat, which will help to give subsequent insights concerning the source of typical chemical compositions of the fen pore water.
Instrumentation was also installed in the wider catchment area of the research sites to gain information about the groundwater quality (and levels) feeding the fens. Piezometers were installed using a rotary drilling rig from Geological Survey Ireland (GSI) as deep as possible down to the interface between Quaternary sediments and bedrock. In addition, a number of pre-existing boreholes that reached into the bedrock (which typically served as current or previous private domestic water supplies) were also used for monitoring. At Pollardstown fen and Tory Hill, data for meteorological parameters were collected by weather stations (Campbell Scientific) installed at each wetland site. Hourly measurements of air temperature and humidity, rainfall, barometric pressure and net radiation were recorded by data loggers. From these data, potential evapotranspiration was calculated according to the Penman–Monteith method (FAO, 1998). Actual evapotranspiration was calculated on a daily basis using a cumulative soil moisture balancing approach for the catchments. For Ballymore and Scragh Bog fens with no weather station, meteorological data were collected from the nearest Met Éireann weather station. Topographical data were derived from a combination of LiDAR mapping as well as surveying in the field using a Trimble 4700 differential GPS system.
Water quality
Water samples were taken from the well, piezometer and phreatic tube at approximately 6 weeks intervals (simultaneously with water level measurements), as a representative sample of the underlying groundwater in that area. Each well was purged prior to sampling to remove standing water from the sample points thus making sure that the collected sample was fresh groundwater instead of stagnant water that may have been remained within the sampler for a long period. Water samples collected were first tested in the field for electrical conductivity, dissolved oxygen, pH and temperature using a HANNA® HI98195 and YSI Pro2030 multimeters. Samples to be used for parameters except total phosphorus were filtered with a 0.45 μm Minisart filter into a 30 ml sterile plastic sample bottles. Samples to be used for dissolved reactive phosphorus analysis were filtered 15 min after collection (USGS, 2005). The unfiltered sample collected for total phosphorus were stored into sterilised 100 ml amber glass bottles Samples were stored in a coolbox keeping the samples in the dark and cool (4°C) during the transfer to the laboratory. Replicate (duplicate) samples and transport/trip blanks were used as quality control (QC) measures to ensure reliability of the sampling and laboratory protocols.
Eighteen water quality parameters were analysed from the water samples collected. Every parameter except for total phosphorus was measured in its dissolved form and all analyses were carried out in triplicate. Dissolved reactive phosphorus (DRP) and total phosphorus (TP) was analysed using a Hach spectrophotometer with a method detection limit (MDL) calculated to be approximately 1 μg/L. Note, DRP refers to the measurement of reactive phosphorus in samples that were filtered through 0.45 µm filters in the field. Total ammonia, nitrite and total oxidised nitrogen were measured using a Lachat 3-channel flow injection analyser with MLDs calculated to be approximately 1 μg/L. Total dissolved nitrogen (TDN) and dissolved organic carbon (DOC) were measured using the Shimadzhu TOC-L with a MDL of 4 μg/L.
Data analysis
The statistical analysis of water level and water quality data were performed using statistical packages in R-Studio and presented as box and whisker plots. All data were first tested for normality by graphical means and Shapiro-Wilk tests. In a limited number of cases where data were not normally distributed, log transformations were utilised to induce normality. A Welch t-test was used to calculate significant differences between the different means of hydrochemistry and hydrology data groups. A p value < 0.05 was used to indicate a significant difference between groups.
Results
Habitat assessment
The vegetation habitat maps derived for the fens are shown in Figure 1, which also show the position of the instrumentation transects. These show the spatial distribution of the different vegetation communities. Note, non-fen habitat areas (according to the Fossitt categorization) were also mapped and included in statistical plots.
The results of the assessment criteria classification of the areas where the monitoring points were located into good and poor ecological condition is given in detail in Supplementary Information (Supplementary Appendix B) and summarized in Table 2. This shows the distribution of different habitats between the fen sites and the fact that all of the monitoring points in Tory Hill (surveyed as PF1 habitat) were deemed to be in poor ecological condition. It should be noted that Tory Hill fen had already previously been determined to be of poor status due to an adjacent drainage channel which has been clearly quantified in this study (as seen later in Section 3.3).
Hydrological conditions supporting fen vegetation
Boxplots are presented of the phreatic water levels using the fen quality groupings good ecological condition or poor ecological condition in Figure 2. A clear difference in the behaviour of the phreatic water level can be seen between the poor and good ecological condition locations of the PF1 (rich, fen, and flush) habitat. The PF1 habitat in poor ecological condition were all located in Tory Hill fen and had the lowest median water level of all displayed habitats of −0.175 m below ground level (interquartile range from −0.003 to −0.331 m). The PF1 sites deemed to be in good ecological condition had much higher phreatic water levels with a median water level of +0.231 m above ground level (interquartile range +0.110 to +0.341 m). For PF2 and PF3, the good-quality habitats reveal slightly higher median levels than their poor counterparts, 0.016 and 0.089 m above ground level for PF2 and PF3, respectively. All PF2 habitats (good and poor) were recorded on Pollardstown fen, while the PF3 habitats were found on both Ballymore and Scragh bog.
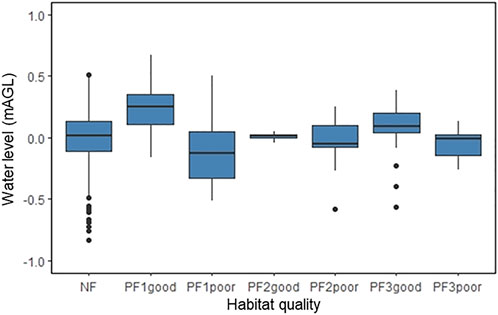
FIGURE 2. Phreatic water level in metres above ground level for Fossitt habitat defined to be in good or poor ecological conditions. Non-fen habitats denoted as NA.
The fluctuations in the piezometric water levels are less reflective of the ecological conditions between the different fen habitat types (Figure 3). This is to be expected as it is the phreatic layer that has a direct impact on the fen vegetation, whereas the link between the piezometric pressure and the vegetation in the phreatic layer is more indirect. It is notable that the median piezometric levels for all good fen habitats were slightly lower, which implies that downward gradients are more common in the areas of good fen vegetation. This may suggest an hypothesis that such fens have a nutrient cycling mechanism incorporating this downwards gradient, into the substrate below. This may also suggest that the groundwater source of base-rich water (required to keep the vegetation as calcareous fen vegetation) is entering the fen more laterally rather than from the base of the fen.
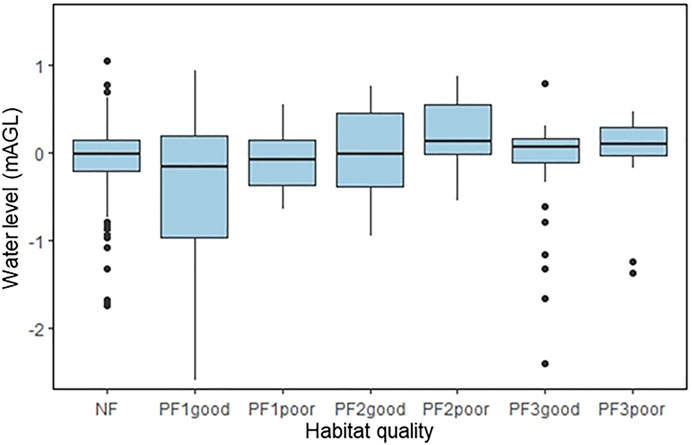
FIGURE 3. Piezometric water level in metres above ground level in good, poor or non-fen (Other) habitats.
To further investigate the influence of soil saturation on the quality of the vegetation, phreatic tube water levels were plotted against duration for the different quality fen habitats (Figure 4). Most of the fen habitats deemed to be in good ecological condition show minimal change in water levels. In most habitats, water levels were above ground level for >90% of the hydrological year, with some exceptions where the level was found 0.1 m below ground level for short periods of time. Highest levels were recorded in habitat PF1, with some especially high elevations, up to 0.73 m, for <16% of the year. This habitat seems to require a tighter envelope of water levels always above the ground surface from approximately 0.11–0.34 m depth of flooding all year round (based on 25th to 75th percentile levels). These “flooding” levels were also seen in the poor-quality PF1 habitat sites; however, here the levels change drastically and are below ground elevation for most of the year (70%), with levels down to 0.43 m below ground level. From this, it can be concluded that the overall hydrological controls for fen vegetation in good condition seem to be reflected in phreatic water levels above ground level with minimal level changes for most (over 90%) of the hydrological year. The only exception to this is at Tory Hill fen—which was chosen for the study because it is known to be affected by drainage (as indicated by the red line in Figure 4)—which exhibits much lower water levels during summer periods. Evidence from Ordnance Survey maps shows that this drain has been in existence since at least the late 1830’s, at the time with a much more meandering course; at some stage between the 1830’s and the 1900’s the outflow stream/drain was straightened and substantially deepened. Some areas of rich fen and flush (PF1) at Tory Hill do seem to be resilient to that lower water level regime; however, the vegetation has been shown to be in poor ecological condition with less species richness, which matches previous findings from the United Kingdom (Wheeler et al., 2009).
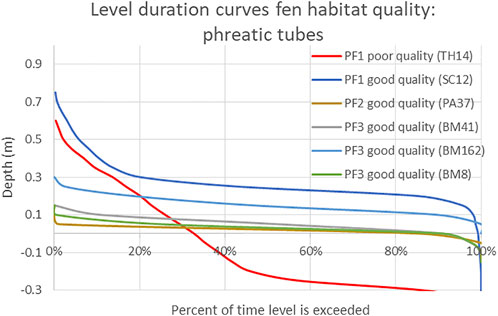
FIGURE 4. Phreatic level duration curves recorded in good and poor fen habitat. The negative numbers are water levels below ground level.
In terms of water level variations, the phreatic water level variations in Ballymore, Pollardstown, Scragh bog, and Tory Hill fens show minor short-term fluctuations across the year, with levels increasing by up to 20 cm during periods of extreme rainfall and hydrographs controlled by their main surface water outlets (see Supplementary Figure S3). Many of the piezometric levels across the fens show evidence of more seasonal fluctuations, presumably more linked to water seasonal recharge dynamics and groundwater levels in the supporting aquifers, which is particularly noticeable in the very dry summer of 2018 with some levels dropping by over a meter (see Supplementary Figure S4).
In general, to support fen vegetation in good ecological condition the overall surface water level needs to be within a range of 0.03–0.28 m above ground level, and these levels should be sustained for at least 60% of the year. These envelope values were calculated by taking the first and third quartiles of the “good” quality fen habitats.
Hydrochemical conditions supporting fen vegetation
In a similar manner to the water level analysis, the water chemistry data from surface water samples taken from the phreatic tubes, piezometers at the base of the peat layer and groundwater-feed monitoring points have been analysed statistically and presented as boxplots according to vegetation habitat types for DRP (Figure 5), TP (Figure 6), total dissolved nitrogen (Figure 7) and ammonia (Figure 8). This shows statistically significant differences in nutrient concentrations between the phreatic wells (low concentrations) and in both the piezometers sampling deeper substrate and groundwater feed from the surrounding catchment (high concentrations) for almost all habitat types—see Supplementary Table S7. The median values of nutrients found in the near-surface phreatic zone of good-quality PF1 fen habitat were found to be 9 μg-P/l for DRP, 92 μg-P/l for TP, 0.55 mg-N/l for ammonia and 1.26 mg-N/l for total dissolved nitrogen; for good-quality PF2 fen habitat were 12 μg-P/l for DRP, 231 μg-P/l for TP, 0.21 mg-N/l for ammonia and 1.86 mg-N/l for total dissolved nitrogen; and for good-quality PF3 fen habitat were 13 μg-P/l for DRP, 82 μg-P/l for TP, 0.55 mg-N/l for ammonia, 0.01 mg-N/l for total oxidised nitrogen and 1.26 mg-N/l for total dissolved nitrogen. However, this does not mean that levels found above these medians should necessarily be regarded as nutrient pollution. There will be hydrochemical controls associated with an internal cycling system in which the fen recycles nutrients (released from the breakdown of vegetation) from the phreatic water levels into the sediments below, although this an area that needs more targeted research.
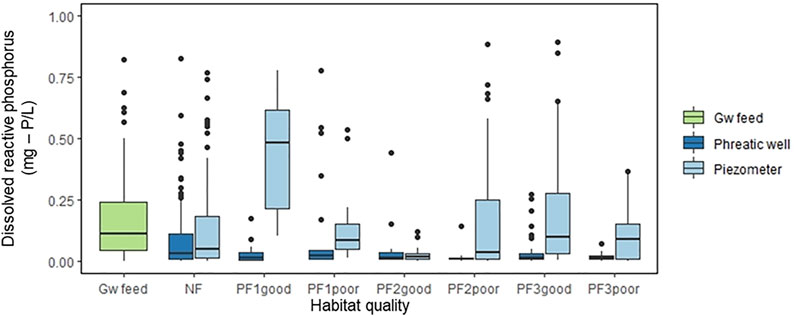
FIGURE 5. Total dissolved reactive phosphorus (mg-P/l) for calcareous fen vegetation Fossitt habitat types PF1, PF2, and PF3 in the fen phreatic tubes and piezometers of good, poor or non-fen (other) habitats, and groundwater (Gw) feed from the wider catchment.
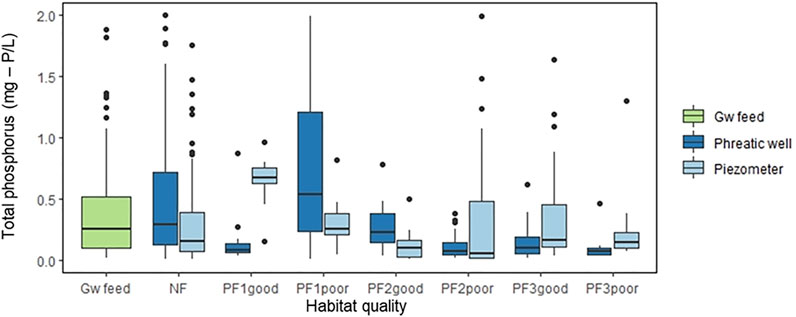
FIGURE 6. Total phosphorus (mg-P/l) for calcareous fen vegetation Fossitt habitat types PF1, PF2, and PF3 in the fen phreatic tubes and piezometers of good, poor or non-fen (other) habitats, and groundwater (Gw) feed from the wider catchment.
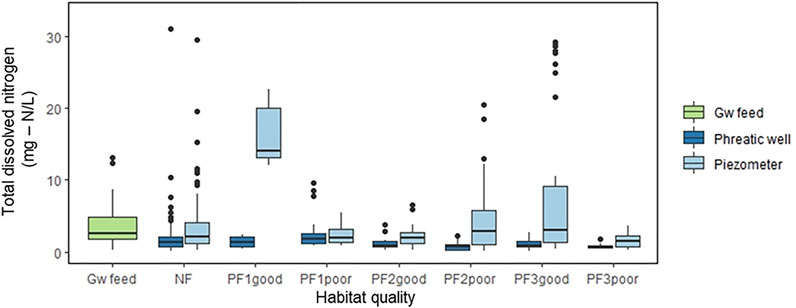
FIGURE 7. Total dissolved nitrogen (mg-N/l) for calcareous fen vegetation Fossitt habitat types PF1, PF2, and PF3 in the fen phreatic tubes and piezometers of good, poor or non-fen (Other) habitats, and groundwater (Gw) feed from the wider catchment.
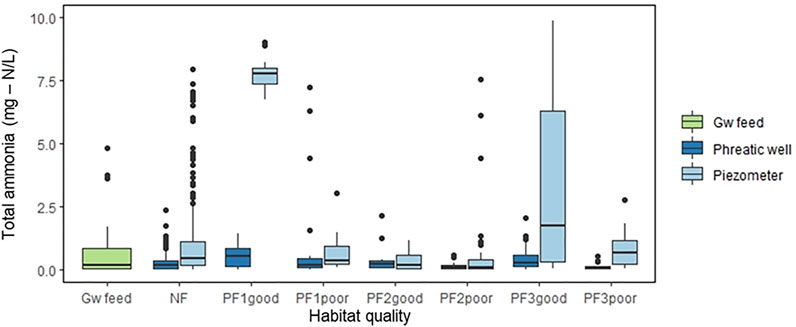
FIGURE 8. Total ammonia concentrations (mg-N/l) for calcareous fen vegetation Fossitt habitat types PF1, PF2, and PF3 in the fen phreatic tubes and piezometers of good, poor or non-fen (other) habitats, and groundwater (Gw) feed from the wider catchment.
Whilst the groundwater monitoring points across the fen sites showed higher concentrations of nutrients in general compared to the concentrations in the phreatic level in the fens, the nutrient concentrations in the sampling points that were deemed to be reflective of groundwater input into the fens reveal a very wide range, making it difficult to draw conclusions about the relevance of these results as to whether they are truly reflective of the groundwater feed. The groundwater nutrients results are shown according to the different fen sites in Figures 9, 10. This wide range of results is revealed, for example, at Pollardstown fen. At Pollardstown A (PA), the monitoring borehole upgradient of this site has a high median DRP concentration of 607 μg/L, while data from another nearby EPA monitoring borehole over the same period reported DRP concentrations consistently less than 10 μg/L. On the other side of the fen the groundwater monitoring point used for Pollardstown D (PD) in this study had a much lower median DRP concentration of 69 μg/L, and another study carried out on the springs feeding the fen from a large gravel aquifer showed them to have a median DRP concentration of just 8 μg/L (Grogan, 2020). Local hydrogeological conditions will have a strong influence on the water quality actually arriving at the fen vegetation root systems.
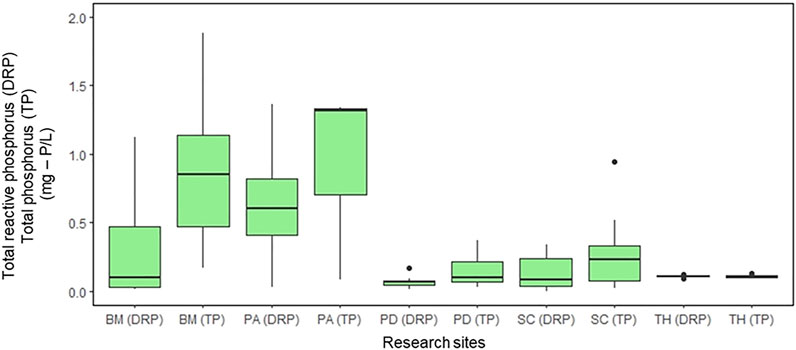
FIGURE 9. Groundwater quality results for total phosphorus and dissolved reactive phosphorus at the different fen sites (SC, Scragh bog; PD, Pollardstown fen; BM, Ballymore; TH, Tory Hill).
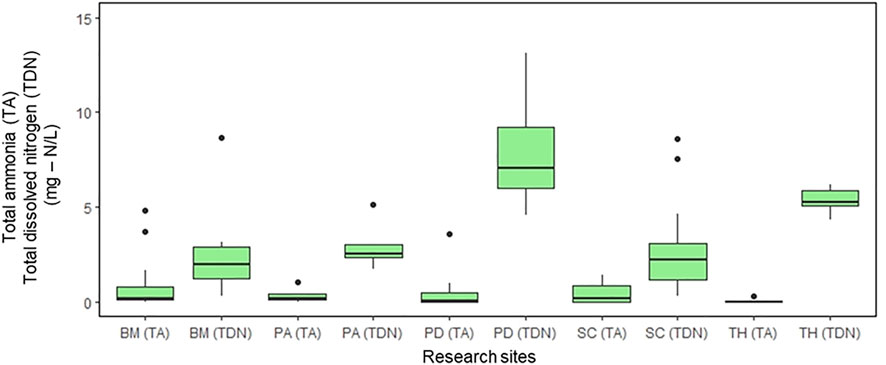
FIGURE 10. Groundwater quality results for total ammonia and total dissolved nitrogen at the different fen sites (SC, Scragh bog; PD, Pollardstown fen; BM, Ballymore; TH, Tory Hill).
In terms of seasonal variations, the data by habitat classification has been divided into two times of the year to reflect broadly the growing season of the vegetation (Spring/Summer) compared to its more dormant season (Autumn/Winter). These plots for nutrient water quality aspects are all presented in the Supplementary Information (Supplementary Figures S5–S9). In terms of seasonal variations, none of the main nutrients showed significant differences across the seasons across any of the fens in the phreatic water quality monitoring points (Supplementary Table S8), although in general the phosphorus (both DRP and Total P) concentrations were slightly higher in the main growing season on Ballymore, Pollardstown, and Scragh bog fens, possibly attributed to the higher level of microbial activity in the organic sediment in the warmer temperatures. The piezometric monitoring points also showed no significant differences in nutrient concentrations between seasons although with the exception of DRP and Total P in Tory Hill, being higher in the spring/summer (Supplementary Table S9). Examples of time series for representative phosphorus concentrations for the phreatic and piezometric sampling points in the different habitats are given in Figures 11, 12.
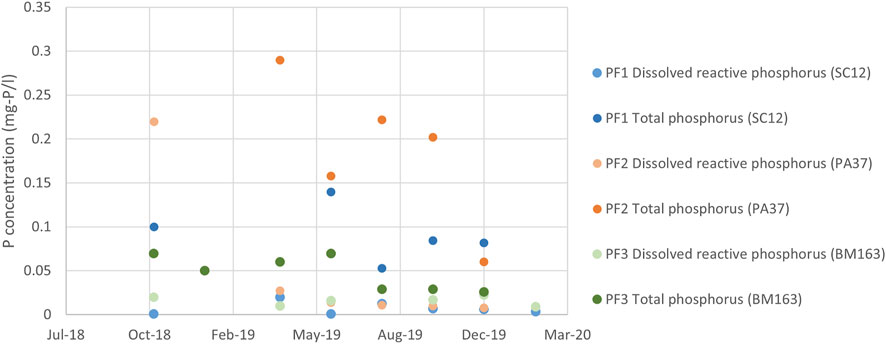
FIGURE 11. Time series of phreatic water quality for total phosphorus and dissolved reactive phosphorus selected monitoring points representative of good quality fen habitat.
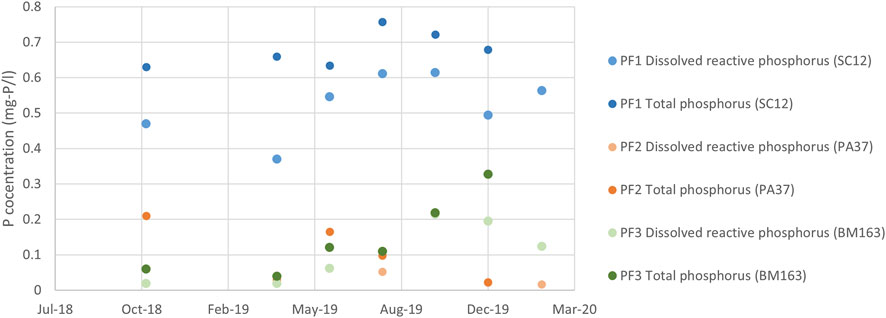
FIGURE 12. Time series of piezometric water quality for total phosphorus and dissolved reactive phosphorus selected monitoring points representative of good quality fen habitat.
Overall, these results suggest that the fen vegetation needs, or at least can tolerate, a certain level of nutrients. Envelope recommendations for each Fossitt habitat are based on the first and third quartiles in boxplots of phreatic water table concentrations recorded in good fen habitats. However, it is important to note that these values should be viewed as being representative of “remnant” nutrients not taken up by the vegetation or cycled into lower sediments rather than the nutrient feed needed to sustain the fen. These levels are also a reflection of the dilution by rainwater evident from the mixed surface/groundwater at phreatic levels. The reported values in Table 3, however, can be viewed as representative of typical conditions found in the shallow phreatic zone of good-quality fen habitat.

TABLE 3. Nutrient concentration envelopes for habitats in good ecological condition within Irish calcareous fens.
Discussion
Four fens were instrumented and monitored over a 2-year period, to investigate both their hydrology and hydrochemistry in relation to their different vegetation types. The water level-duration analyses revealed clear differences between fen habitat considered to be in good versus poor ecological condition. The field investigations suggest that a threshold water level envelope of between 3 and 28 cm above ground level, which should be sustained for at least 60% of the year, is required to maintain fen vegetation in a good ecological condition with the mean annual water level always above the surface. The PF1 (rich fen and flush) habitat seems to require a higher envelope of water levels always above the ground surface from approximately 100–400 mm depth of flooding all year round, based on the first and third quartiles of the healthy fen habitats. If these threshold ecohydrological envelopes for a “healthy” fen are met, the system would appear to have a natural resilience against short-term natural environmental stressors such as drought or flooding.
In terms of the hydrochemistry, the results are more complex to interpret. Although envelopes of nutrients in the surface water of the fen associated with fen habitat in good ecological condition can be defined (e.g., dissolved reactive phosphorus concentrations of between 6 and 37 µg-P/l for PF1 habitat) these levels should be regarded as the water quality after the fen vegetation has interacted with the higher incoming nutrient levels in the groundwater. Viewing the fen as a whole, it could be seen to perform as a self-cleansing system for nutrients flowing in from groundwater and surface water, whereby they get caught in the wetland’s internal biogeochemical cycles, thus leaving water with lower nutrient concentrations to discharge from the wetland. Ultimately, the annual build-up of organic material (including nutrients) causes peat formation. However, the exact location of the groundwater feed to much of the fens has not been ascertained and so it is not clear whether high inlet nutrient concentrations may be causing a problem in very localized areas. In general though, the groundwater feed appears to be supplying nutrients at higher concentrations (than what is found in the phreatic layer of the fens) which help to support, or at least are tolerated by, the fen vegetation. The maximum values in Table 3 should not therefore be used as Threshold Values above which damage will be inflicted on the fen, as no direct link was found between high nutrient concentrations and “poor” ecological condition in the fen habitats. These phreatic water concentrations can be considered to be residual concentrations after vegetation uptake, surface water dilution and dispersion rather than being directly linked to the nutrients entering the fen from the catchment. The vegetation was also found to be relatively resilient against short- to medium-term changes in nutrient supply from groundwater or surface water, again hypothesized to be due to nutrient uptake and internal recycling by the vegetation. Any threshold hydrochemical metrics should therefore include a time dimension. The median nutrient concentrations in the individual groundwater monitoring points in the catchment surrounding the different fens, ranged from, 51 to 630 μg-P/l for DRP, 101 to 1361 μg-P/l for TP, 0.07 to 0.93 mg-N/l for ammonia, 0.04 to 2.08 mg-N/l for total oxidized nitrogen and 1.95 to 7.08 mg-N/l for total dissolved nitrogen. However, as discussed, it is debatable as to what these values represent and there was a wide range between different fen sites (as well as within each site) and this is an area that needs a lot more study, particularly in such fractured/fissured type aquifer systems that typically feed calcareous fens. Also, more rigorous water balances need to be ascertained for each fen to be able to define the relative contributions of groundwater versus surface water flows into the fen across the year from which the actual mass of nutrients entering (and existing) the fen via the different pathways can be quantified, before any firm conclusions about this element of a conceptual model can be drawn. In any case, these monitored nutrient levels around the fens did not appear to be causing any evident ecological stress to the fen ecosystems (as far as can be determined from the relatively short duration of the research study, at least). While all the sites had multiple entry points for groundwater, the localized nature of the monitoring measurements (in terms of depth as well as spatially) makes it difficult to define a groundwater threshold values with any confidence from the results of these field studies. More targeted research is needed in terms of understanding the fate and transport of nutrients coming into the fens in groundwater compared with the levels found in the surface water. This will require more extensive analysis of the hydrogeology and groundwater chemistry in the wider catchments feeding the wetlands.
In summary, these field studies have shown that the natural resilience of Irish calcareous fens can be decreased by significant changes to the soil saturation/water table in the fen catchment, as was found in Tory Hill fen where all sites were shown to be in poor ecological condition. Even though the research did not find evident damage by nutrient pollution, very high levels of pollution input into such wetlands can cause the nutrient cycling system to become overloaded. This may result in levels harmful to fen vegetation and the likelihood that species diversity will diminish as the smaller herbs are outcompeted by species typical of other habitats, such as swamps, scrub or woodland. Hence, more targeted research is needed in terms of understanding the complex water quality dynamics within such wetlands, involving accumulation and internal cycling of nutrients, in order to establish pragmatic nutrient TVs. This study has also revealed the challenges on how best to best monitor and represent the groundwater quality feeding such systems. Clearly defined springs do represent an integrated groundwater input from a wider catchment but are often very difficult to locate and may well be below the surface of the wetland. Boreholes may only represent very localized sources of pollution and are not necessarily reflective of the average feed to the wetland. Whilst legislation calls for groundwater threshold values to be set to protect associated aquatic ecosystems and human health (Hinsby et al., 2008), it is not clear in the case of these calcareous fen groundwater dependent terrestrial ecosystems (GWDTEs) whether the relatively high nutrients in the groundwater feed (compared to the water quality in the fen) are actually supporting the healthy fen vegetation and to what level they would need to rise to become damaging. Furthermore, the nature of the aquifers that support such fens are all different and complex, ranging from fractured bedrock to sand and gravel, and their impacts can be slow due to long residence times. There has been a general lack of long-term monitoring programmes on the interaction between aquifers and GDEs (Klove et al., 2011a; Klove et al., 2011b) and this relatively short-term study has revealed the difficulty of defining generic “representative” water quality values for conservation/management purposes. Local hydrogeological conditions define groundwater pathways (and associated water quality impacts) in such calcareous fens and so any thresholds set for groundwater quality should depend on local conditions.
Nevertheless, classifying the habitats, on the basis of the Irish Fossitt scheme, along transects in a variety of fens, revealed a clear distinction in responses to the water level regime between poor and good quality fen habitats. Rich fen habitat conditions correlated with higher maintained water levels compared to poor fen conditions. The difference in the apparent response to phreatic water quality conditions was much more equivocal. However, the hydrochemical regime at all the habitats appeared resilient to much more variable regional groundwater quality upgradient of the fens, for both good quality and poor quality fen. While these results point to the development of appropriate metrics for defining “good” fen habitat, however defined, there is a clear need for further research before pragmatic measures can be established. The mechanisms through which hydrochemical concentrations, particularly of nutrients, are attenuated along the pathways between regional groundwater inputs and the phreatic zone of the vegetation needs to be understood. Is local fen hydrogeology or hydro-biological recycling, or both, critical? More focused research is needed to develop the conceptual models in terms of better understanding the internal biochemical cycling that occurs in these systems. This needs to be complemented by further analysis of hydraulic gradients and water balances across these ecosystems at the groundwater/surface water interface to define rigorous conceptual models. Consequently, an important conclusion with respect to the establishment of metrics is the need to incorporate the concept of scale, both spatial and in temporal, in the relevant monitoring observations. Fens are typically a mosaic of habitats, evolving in time, as well as being fed by a corresponding pattern in hydrogeological inputs. The target for fen condition assessment must be clear—for example, the whole fen or a particularly sensitive habitat within the fen? Such focus should then relate to the relevant hydrogeology (ies) in order to establish appropriate monitoring points for groundwater inputs. This study of four different fen wetlands across the island of Ireland is a positive step in the direction of a practical protocol for establishing threshold metrics.
Data availability statement
The raw data supporting the conclusions of this article will be made available by the authors, without undue reservation.
Author contributions
LG and SR conceived the study and gained the required funding. EB, SR, PJ, CC, and LG. planned the experiments. EB performed the experiments and analysed data. EB and LG wrote the manuscript. All authors interpreted the results, wrote and reviewed the manuscript.
Funding
The funding for the study was initially provided by the Environmental Protection Agency under grant number 2016-LS-13 as well as a grant from the Irish National Parks and Wildlife Service.
Acknowledgments
The authors wish to thank members of the EPA Steering Committee, particularly Hans Schutten (Wetlands International), Mark Whiteman (Environment Agency, United Kingdom), Maurice Eakin (National Parks and Wildlife Service) and Matt Craig (Environmental Protection Agency). The authors would also like to thank Fernando Fernandez (National Parks and Wildlife Service).
Conflict of interest
The authors declare that the research was conducted in the absence of any commercial or financial relationships that could be construed as a potential conflict of interest.
Publisher’s note
All claims expressed in this article are solely those of the authors and do not necessarily represent those of their affiliated organizations, or those of the publisher, the editors and the reviewers. Any product that may be evaluated in this article, or claim that may be made by its manufacturer, is not guaranteed or endorsed by the publisher.
Supplementary material
The Supplementary Material for this article can be found online at: https://www.frontiersin.org/articles/10.3389/feart.2022.917233/full#supplementary-material.
References
Aggenbach, C. J. S., Backx, H., Emsens, W. J., Grootjans, A. P., Lamers, L. P. M., Smolders, A. J. P., et al. (2013). Do high iron concentrations in rewetted rich fens hamper restoration? Preslia 85 (3), 405–420.
Amon, J. P., Thompson, C. A., Carpenter, Q. J., and Miner, J. (2002). Temperate zone fens of the glaciated midwestern USA. Wetlands 22 (2), 301–317. doi:10.1672/0277-5212(2002)022[0301:TZFOTG]2.0.CO;2
Barbier, E. B. (2011). Wetlands as natural assets. Hydrological Sci. J. 56 (8), 1360–1373. doi:10.1080/02626667.2011.629787
BEC (2019). Habitat surveys of Scragh bog and Pollardstown fen. Chennai: BEC Consultants. Unpublished report.
Bedford, B. L., and Godwin, K. S. (2003). Fens of the United States: Distribution, characteristics, and scientific connection versus legal isolation. Wetlands 23 (3), 608–629. doi:10.1672/0277-5212(2003)023[0608:FOTUSD]2.0.CO;2
Bertassello, L. E., Jawitz, J. W., Aubeneau, A. F., Botter, G., and Rao, P. S. C. (2019). Stochastic dynamics of wetlandscapes: Ecohydrological implications of shifts in hydro-climatic forcing and landscape configuration. Sci. Total Environ. 694, 133765. doi:10.1016/j.scitotenv.2019.133765
Bhatnagar, S., Gill, L. W., Regan, S., Naughton, O., Johnston, P. M., Waldren, S., et al. (2020). Mapping vegetation communities inside wetlands using Sentinel-2 imagery in Ireland. Int. J. Appl. Earth Obs. Geoinf. 88, 102083. doi:10.1016/j.jag.2020.102083
Bhatnagar, S., Gill, L. W., Regan, S., Waldren, S., and Ghosh, B. (2021b). A nested drone-satellite approach to monitoring the ecological conditions of wetlands. ISPRS J. Photogrammetry Remote Sens. 174, 151–165. doi:10.1016/j.isprsjprs.2021.01.012
Bhatnagar, S., Gill, L. W., Waldren, S., Sharkey, N., Naughton, O., Johnston, P., et al. (2021a). Ecohydrological metrics for vegetation communities in turloughs (ephemeral karstic wetlands). Ecohydrology 14, e2316. doi:10.1002/eco.2316
De Groot, R., Brander, L., Van Der Ploeg, S., Costanza, R., Bernard, F., Braat, L., et al. (2012). Global estimates of the value of ecosystems and their services in monetary units. Ecosyst. Serv. 1 (1), 50–61. doi:10.1016/j.ecoser.2012.07.005
Duval, T. P., Waddington, J. M., and Branfireun, B. A. (2011). Hydrological and biogeochemical controls on plant species distribution within calcareous fens. Ecohydrology 5 (1), 73–89. doi:10.1002/eco.202
Eamus, D., Froend, R., Loomes, R., Hose, G., and Murray, B. (2006). A functional methodology for determining the groundwater regime needed to maintain the health of groundwater-dependent vegetation. Aust. J. Bot. 54, 97–114. doi:10.1071/BT05031
European Commission (1995). Commission’s communication to the Council and the parliament: Wise use and conservation of wetlands. Brussels, Belgium: European Commission.
FAO (Food and Agriculture Organization of the United Nations) (1998). Crop evapotranspiration – guidelines for computing crop water requirements. FAO irrigation and drainage paper 56. Rome: FAO.
Foss, P. J. (2007). National Parks & Wildlife service study of the extent and conservation status of springs, fens and flushes in Ireland 2007. Ireland: Unpublished Report for the National Parks and Wildlife Service, Department of the Environment, Heritage and Local Government.
Grogan, C. (2020). An investigation into the hydrochemistry and nutrient loading from groundwater pathways to Pollardstown Fen. Trinity College Dublin: Co. Kildare. Unpublished B.A.(Mod.) Environmental Science dissertation.
Hill, M. O., Mountford, J. O., Roy, D. B., and Bunce, R. G. H. (1999). Technical Annex -ellenberg's indicator values for British plants. Wallingford, Oxfordshire: Centre for Ecology and Hydrology, Natural Environment Research Council.
Hinsby, K., de Melo, M. T. C., and Dahl, M. (2008). European case studies supporting the derivation of natural background levels and groundwater threshold values for the protection of dependent ecosystems and human health. Sci. Total Environ. 401, 1–20. doi:10.1016/j.scitotenv.2008.03.018
IPCC (2009). Ireland's peatland conservation action plan 2020: Halting the loss of peatland biodiversity. Dublin, Ireland: Irish Peatland Conservation Council.
Joosten, H., and Clarke, D. (2002). Wise use of peatlands. Jyvaskyla: International Mire Conservation Group and International Peat Society.
Kellner, E. (2003). Wetlands—different types, their properties and functions. Technical report TR-04-08. Dept of Earth Sciences/Hydrology, Uppsala University (2003).
Klimkowska, A., Grootjans, A. P., Kotowski, W., and Van Diggelen, R. (2010). Prospects for fen meadow restoration on severely degraded fens. Perspect. Plant Ecol. Evol. Syst. 12, 245–255. doi:10.1016/j.ppees.2010.02.004
Kløve, B., Ala-aho, P., Bertrand, G., Boukalova, Z., Erturk, A., Goldscheider, N., et al. (2011a). Groundwater dependent ecosystems. Part I: Hydroecological status and trends. Environ. Sci. Policy 14 (7), 782–793. doi:10.1016/j.envsci.2011.04.005
Kløve, B., Allan, A., Bertrand, G., Druzynska, E., Ertürk, A., Goldscheider, N., et al. (2011b). Groundwater dependent ecosystems. Part II. Ecosystem services and management in Europe under risk of climate change and land use intensification. Environ. Sci. Policy 14 (7), 782–793. doi:10.1016/j.envsci.2011.04.005
Kuczynska, A. (2008). Eco-hydrology of Pollardstown fen, Co. Kildare, Ireland. Dublin, Ireland. PhD thesis. Trinity College Dublin: Department of Civil, Structural and Environmental Engineering.
Lamers, L. P. M., Vile, M. A., Grootjans, A. B., Acreman, M. C., van Diggelen, R., Evans, M. G., et al. (2014). Ecological restoration of rich fens in Europe and north America: From trial and error to an evidence-based approach. Biol. Rev. 90 (1), 182–203. doi:10.1111/brv.12102
Lucassen, E. C. H. E. T., Smolders, A. J. P., Van Der Salm, A. L., and Roelofs, J. G. M. (2004). High groundwater nitrate concentrations inhibit eutrophication of sulphate-rich freshwater wetlands. Biogeochemistry 67 (2), 249–267. doi:10.1023/B:BIOG.0000015342.40992.cb
McBride, A., Diack, I., Droy, N., Hamill, H., Jones, P., Schutten, J., et al. (2011). The fen management handbook. Perth, UK: Scottish Natural Heritage.
Millennium Ecosystem Assessment (2005). Ecosystems and human well-being: Synthesis. Washington, DC: Island Press.
Misstear, B., Brown, L., and Johnston, P. (2009). Estimation of groundwater recharge in a major sand and gravel aquifer in Ireland using multiple approaches. Hydrogeol. J. 17 (3), 693–706. doi:10.1007/s10040-008-0376-0
NPWS (National Parks and Wildlife Service) (2019). “The status of protected EU habitats and species in Ireland,”. Editors Habitat Assessments, D. Lynn, and F. O’Neill (Dublin: National Parks and Wildlife Services, Department of Culture, Heritage and the Gaeltacht), Vol. 2. Available at: https://www.npws.ie/sites/default/files/publications/pdf/NPWS_2019_Vol2_Habitats_Article17.pdf.
Perrin, P. M. (2021). Pilot fen survey 2019-20, unpublished report for the. Dublin: National Parks and Wildlife Service.
Proctor, M. C. F. (2010). Environmental and vegetational relationships of lakes, fens and turloughs in the Burren. Biol. Environ. 110B, 17–34. doi:10.1353/bae.2010.0026
Regan, S., and Connaghan, J. (2017). Eco-hydrological investigation of Ballymore fen SAC. Ireland: Co. Westmeath, Prepared for the Office of Public Works.
Regan, S., and Connaghan, J. (2016). Eco-hydrological investigation of Tory Hill fen SAC, Co. Limerick. Dublin: Office of Public Works.
Regan, S., Swenson, M., O’Connor, M., and Gill, L. (2020). “Ecohydrology, greenhouse gas dynamics and restoration guidelines for degraded raised bogs,”. STRIVE Report Series No. 342 (Wexford, Ireland: Environmental Protection Agency), 40pp.
Sampath, P. V., Liao, H. S., Curtis, Z. K., Doran, P. J., Herbert, M. E., May, C. A., et al. (2015). Understanding the groundwater hydrology of a geographically-isolated prairie fen: Implications for conservation. PLoS ONE 10 (10), e0140430. doi:10.1371/journal.pone.0140430
Stofberg, S. F., van Engelen, J., Witte, J. P. M., and van der Zee, S. E. A. T. M. (2016). Effects of root mat buoyancy and heterogeneity on floating fen hydrology. Ecohydrology 9 (7), 1222–1234. doi:10.1002/eco.1720
USEPA (2018,). Why are wetlands important? Retrieved from United States Environmental Protection Agency: https://www.epa.gov/wetlands/why-are-wetlands-important.
van Diggelen, R., Middleton, B., Bakker, J., Grootjans, A., and Wassen, M. (2006). Fens and floodplains of the temperate zone: Present status, threats, conservation and restoration. Appl. Veg. Sci. 9 (2), 157–162. Available at: http://www.jstor.org/stable/4620474. doi:10.1111/j.1654-109x.2006.tb00664.x
Wheeler, B. D., Shaw, S., and Tanner, K. (2009). “A wetland framework for impact assessment at statutory sites in England and Wales,”. Integrated Catchment science programme, Science report: SC030232 (UK: Environment Agency).
Whiteman, M. I., Wheeler, B. D., Shaw, S. C., Lewis, T., Grout, M., and Tanner, K. (2009). “Use of WETMECS typology to aid understanding of groundwater-dependent terrestrial ecosystems in England and Wales,” in Groundwater quality assessment and monitoring. Editors P. Quevauviller, A. M. Fouillac, J. Grath, and R. Ward (New Jersey, United States: John Wiley & Sons), 259–272.
Keywords: calcareous fen, wetland, ecohydrology, water level duration, nutrient threshold
Citation: Bijkerk E, Regan S, Johnston PM, Coxon C and Gill LW (2022) The challenge of developing ecohydrological metrics for vegetation communities in calcareous fen wetland systems. Front. Earth Sci. 10:917233. doi: 10.3389/feart.2022.917233
Received: 10 April 2022; Accepted: 27 July 2022;
Published: 06 September 2022.
Edited by:
Inga Retike, University of Latvia, LatviaReviewed by:
Konstantina Katsanou, IHE Delft Institute for Water Education, NetherlandsFrançois Colin, Montpellier SupAgro, France
Copyright © 2022 Bijkerk, Regan, Johnston, Coxon and Gill. This is an open-access article distributed under the terms of the Creative Commons Attribution License (CC BY). The use, distribution or reproduction in other forums is permitted, provided the original author(s) and the copyright owner(s) are credited and that the original publication in this journal is cited, in accordance with accepted academic practice. No use, distribution or reproduction is permitted which does not comply with these terms.
*Correspondence: Laurence W. Gill, bGF1cmVuY2UuZ2lsbEB0Y2QuaWU=