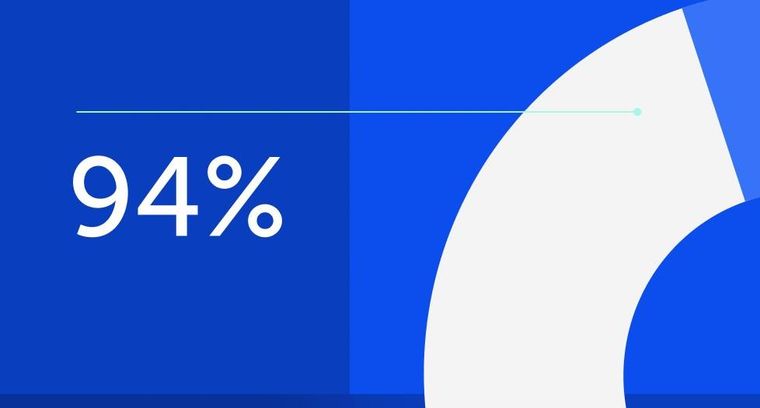
94% of researchers rate our articles as excellent or good
Learn more about the work of our research integrity team to safeguard the quality of each article we publish.
Find out more
ORIGINAL RESEARCH article
Front. Earth Sci., 16 May 2022
Sec. Structural Geology and Tectonics
Volume 10 - 2022 | https://doi.org/10.3389/feart.2022.915747
This article is part of the Research TopicEffects of Deep Fluids in Hydrocarbon Accumulations in Sedimentary BasinsView all 26 articles
Multi-stage hydrocarbon generation and accumulation are very common in petroliferous basins. Also, determination of hydrocarbon accumulation stages and each related diagenetic feature is important for understanding the distribution of current oil and gas resources in one individual basin. However, in the case of conventional sandstone reservoirs, few studies have focused on these resulting reservoir features (e.g., physical property and wettability) that are caused by the first accumulation of hydrocarbons and their geological implications. In this study, taking the reservoir from the Eocene Dongying Depression as an example, we investigated the features of the early hydrocarbon accumulation and its effects on the late accumulation, by analyzing the early accumulation-related paragenesis, fluid inclusions, clay minerals content, reservoir physical property, and wettability in sandstones. Our results show that the early stage accumulation of the overpressured hydrocarbon fluid could support and offset some loads of overburden, weaken the normal compaction, and preserve some original pores. Also, the early hydrocarbon accumulation resulted in an alternated, diagenetic fluid environment from the initial acidic to subsequent alkalic conditions, with the overall dissolution intensity stronger than the cementation, and formed a large number of secondary pores. As a result, the early accumulation was beneficial to reservoir space development and provided more space for the late hydrocarbons. In addition, the early hydrocarbon accumulation could cause the alteration of rock wettability and gradually formed a lipophilic migration pathway from the source rock to the reservoir. Also, the late stage of hydrocarbons would preferentially choose this dominant pathway for their migration (i.e., inheritance of migration pathway).
The study of hydrocarbon accumulation in the sandstone reservoir is a core content of oil and gas exploration in petroliferous basins. Generally, due to the complexity of hydrocarbon source rock distributions and their burial and heating history that is related to tectonic evolution, many petroliferous basins involved multiple hydrocarbon generation and accumulation. Also, the determination of hydrocarbon accumulation stages and the comparative study of the features in each stage have important guiding significance for understanding the distribution of current oil and gas resources.
The Dongying Depression located in the Bohai Bay Basin is an important oil-rich depression in east China, including the two early (i.e., before the tectonic uplift at the end of Paleogene) and the late (i.e., after tectonic uplift to present) stages of hydrocarbon generation and accumulation since the Paleogene (Jiang et al., 2003; Guo et al., 2012). According to the traditional exploration guiding direction in this area, the hydrocarbon resources accumulated during the late stage are the main source for the current reserves. Also, this guiding direction played an important role in the previous hydrocarbon exploration, and had an impact on the previous studies on hydrocarbon accumulation that were mainly focused on the late stage. Because the salt-water lacustrine source rocks have been shown to be more abundant in resources during the early generation and expulsion stage, these source rocks show good prospects for the exploration of the early accumulation in the Dongying Depression. Therefore, it is of great theoretical and practical significance to correctly understand the features of the early hydrocarbon accumulation and their enrichment laws. At present, there are relatively few relevant studies on the early hydrocarbon accumulation and its effects on the late stage. Here, we took the conventional sandstone reservoir that was considered the main source of hydrocarbon production in the study area as the research object, and discussed the features of early hydrocarbon accumulation and its geological implications in the Dongying Depression, by analyzing micro-petrography, fluid inclusions, clay minerals contents, reservoir physical property, and wettability. Our results show that the early accumulation was beneficial to reservoir space development, and provided more space and a preferential migration pathway for the late stage of hydrocarbons.
The Bohai Bay Basin is located in east China (Figure 1A) and represents a faulted-depressed basin developed in the Paleozoic cratonic basement, filled by Mesozoic–Cenozoic strata. It is a lacustrine basin developed during the tectonic movement in the background of Paleozoic bedrock (Zhang et al., 2009). The Dongying Depression is located in the southeastern Bohai Bay Basin. Under the division effect of the Central Fault Belt and the basal fluctuation structure, the Dongying Depression can be divided into seven secondary tectonic units from north to south: Northern Steep-slope Belt, Central Uplift Belt, Lijin Sag, Minfeng Sag, Niuzhuang Sag, Boxing Sag, and Southern Gentle-slope Belt (Figure 1B).
FIGURE 1. Regional geological setting of the study area. (A) Location of the Dongying Depression, Bohai Bay Basin, east China. (B) Tectonic framework of the Dongying Depression.
The Cenozoic strata of the Dongying Depression comprise the Eocene Kongdian Formation (Ek), Eocene Shahejie Formation (Es), Eocene Dongying Formation (Ed), Neogene Guantao Formation (Ng), Neogene Minghuazhen Formation (Nm), and the Quaternary Pingyuan Formation (Qp), respectively, from bottom to top (Figure 2). The sandstone reservoirs within the Eocene Shahejie Formation host the most valuable hydrocarbon resources. The aforementioned four sags are not only the sedimentary center of the depression, but also the host areas of the main source rocks. Two main units of source rocks for the petroleum system, including the upper 4th member of the Shahejie Formation (Es4 upper) and the lower 3rd member of the Shahejie Formation (Es3 lower) were developed (Zhang et al., 2009; Chen et al., 2016).
FIGURE 2. Generalized Cenozoic stratigraphic column and tectonic setting of the study area. Quat., Quaternary; Paleoce., Paleocene; st., stage; conglom., conglomerate; sandst., sandstone; mudst., mudstone; unconf., unconformity.
The tectonic evolution of the basin consists of the syn-rifting stage (65.0–24.6 Ma, during the deposition stage of the Kongdian, Shahejie, and Dongying formations) and post-rifting stage (24.6 Ma to the present, during the deposition stage of Guantao, Minghuazhen, and Pingyuan formations; Qiu et al., 2014). The syn-rifting stage occurred from the Palaeocene to the Oligocene and can be subdivided into 4 episodes: an initial episode (I), an expansion episode (II), an expansion and rapid subsiding episode (III), and a contraction episode (IV) (Chang, 1991; Xie et al., 2001). A subsequent period of uplift and erosion followed the deposition of the Dongying Formation from 24.6 to 14 Ma, and the contact with the overlying Guantao Formation is a regional unconformity in the study area (Guo et al., 2012).
Under controls of the abovementioned tectonic evolution background, there is little difference in hydrocarbon generation history of the two main source rocks from Es4 upper to Es3 lower in 4 sags of the Dongying Depression. In particular, due to burial and reburial that were separated by the uplift and erosion from 24.6 to 14 Ma, the hydrocarbon generation history could be divided into the early and two late stages (Guo et al., 2012). The contribution of the source rocks from the Es4 upper to the early hydrocarbon resource is significantly greater than that of the Es3 lower, because of its deeper burial depth and higher temperature. In the central area of each sag, the early stage of hydrocarbon generation and expulsion of the source rocks from the Es4 upper mainly occurred from 38.2 to 24.6 Ma, and the late stage generally occurred from the Miocene to the present.
All the thin sections for fluid inclusion observation were polished on both sides with the thickness of about 90 μM. The petrographic analysis of inclusions was performed using the criteria described by Goldstein and Reynolds (1994). Fluid inclusion petrographic analysis was carried out by using a Leica DM-2700P microscope with transmitted light (TL) and ultra-violet (UV) illumination (365 nm peak of Hg). The wavelength of the excitation filter used for the fluorescence is from 340 to 380 nm, and of the suppression filter is 425 nm. The microthermometric studies of fluid inclusions were carried out using a Linkam THMSG600 heating–cooling stage with temperature calibrated according to the triple point of H2O (0.0°C), the critical point of H2O (374.1°C), and the triple point of CO2 (−56.6°C) using synthetic fluid inclusions The homogenization temperatures of fluid inclusions in each FIA were obtained by thermal cycling using temperature steps of 1°C as described by Goldstein and Reynolds (1994).
The relative abundances of clay minerals were estimated by XRD analysis using a Panalytical X'Pert PRO Diffractometer with Cu–Ka radiation (40 kV, 30 mA). Before analysis, each sample was oven-dried at 40°C for 2 days and ground to powder (80–100 mesh) using an agate mortar and pestle. Subsequently, the powder was smear mounted on glass slides for the XRD analysis of clay minerals. Identities and semiquantitative relative abundances (wt%) of the various mineral phases were deduced by computer diffractogram analysis.
Porosity, permeability, and rock wettability data were collected from the Research Institute of Petroleum Exploration and Development, Shengli Oilfield Company, SINOPEC. Helium porosity and nitrogen permeability experiments were performed on 25 mm × 25 mm columnar core plugs using ULTRAPORE-200, a porosimeter, and an ULTRA-PER-MTM200 permeameter. The wettability was conducted using the measurement of the contact angle between the liquid (i.e., oil or water) and the rock surface.
The secondary overgrowth edge of quartz is common in the sandstone reservoir (Figure 3). Most quartz grains generally develop only one overgrowth generation. In rare cases, the quartz particles exhibit two generations, and the degree of the early overgrowth was significantly stronger than that of the late overgrowth (Figure 3B). The acidic alteration of detrital feldspars is a common diagenetic reaction in sandstones, which would generate clay minerals (e.g., authigenic kaolinite and illite) and SiO2 as products (Yuan et al., 2015). This dissolution of feldspar grains is widespread in our study area and usually shows significant dissolution pores within the grains (Figure 3). The quartz overgrowth often coexists with the feldspar dissolution in petrography, showing that the dissolution often occurs on the same side and adjacent to the overgrowth. In some cases, kaolinite dominates the authigenic clay minerals, and generally occurs as vermicular aggregates with many intercrystalline micropores, filling the primary pores and the feldspar dissolution pores. These petrographic observations suggest that the feldspar dissolution was coeval with the quartz and kaolinite precipitation, and provided the silica source of the authigenic quartz overgrowth. Thus, there are two feldspar dissolution stages and associated quartz cementation generations in the study area.
FIGURE 3. Petrographic features of diagenetic phenomena and sequence in the sandstones in the Dongying Depression. (A) Feldspar dissolution was coeval with the quartz and kaolinite precipitation. (B) two generations of quartz overgrowth. (C) Fe-calcite replaced the primary quartz particle and first overgrowth generation. Qz, quartz; Fs, feldspar; Kao, kaolinite; Carb, carbonate; Qzo, quartz overgrowth; FDP, feldspar dissolution pore; KMP, intercrystalline micropores of kaolinite.
Carbonate precipitation occurred later than the early feldspar dissolution and quartz precipitation, showing the features of Fe–calcite that replaced the primary quartz particle and first overgrowth generation, and filled the dissolution pores of feldspar (Figure 3). The Fe–calcite was replaced by the ankerite, which indicates that the Fe–calcite precipitation was earlier than the ankerite (Wang Y. Z. et al., 2020). Thus, according to these petrographic features, the main paragenetic sequence includes the early feldspar acidic alteration and quartz overgrowth, subsequent Fe–calcite and ankerite precipitation, and the late feldspar dissolution and quartz precipitation.
Fluid inclusion assemblages (FIAs) in two quartz overgrowth generations and carbonate cements are all comprise primary, two-phase, oil + gas hydrocarbon inclusions, and coexisting aqueous inclusions (Figure 4). The presence of primary hydrocarbon inclusions attests to the two precipitation stages of the quartz, and the middle carbonate cementation was all coeval with the hydrocarbon-bearing fluids accumulation process. In addition, within the overgrowths and cements, the FIAs are also commonly located along the healed microfractures in quartz. Thus, based on the aforementioned sequence, FIAs occurred as healed microfracture trapped during the first quartz generation, would not cut across the carbonate cements and the second quartz overgrowth in petrography. However, the late healed microfracture of FIAs could cut across the early healed microfracture within the same quartz particles, the two overgrowth edges, and the carbonate cements. Based on the microthermometry, the homogenization temperatures (Th) of the coexisting aqueous inclusions formed during the early quartz precipitation stage range from 95 to 125°C, lower than those trapped during the late quartz precipitation stage with the range of 110∼140°C. Combined with the burial and heating history of source rocks, the first stage of the feldspar dissolution and quartz precipitation occurred during the early hydrocarbon accumulation, and the second stage occurred due to the late hydrocarbon accumulation. During the early accumulation, the hydrocarbon-bearing fluids migrated into the pores of the reservoir, and the oil would not adhere to the outer surface of quartz particles due to their hydrophilicity (Chen et al., 2011). Due to the relatively high water saturation and low oil saturation in such silica supplied internally water-wet reservoirs at this time, the oil emplacement would not prevent the quartz cementation completely in sandstones (Worden et al., 1998). Also, the presence of the oil in pores could not inhibit the direct contact between the solution and minerals, and resulted in the preferential entrapment of the oil droplet as the overgrowth of the first quartz generation. Thus, between the primary quartz particle and the first overgrowth edge, the dust lines are mainly composed of hydrocarbon inclusions, which show the same fluorescence color as those on both sides (i.e., hydrocarbon inclusions within the overgrowth and healed microfracture in the particle; Figures 4B,C). The primary, coexisting aqueous inclusions within the Fe–calcite show the Th values range from 123 to 135°C, indicating the deposition of Fe–calcite during the late period of the early accumulation stage (i.e., before tectonic uplift), while the subsequent precipitation of ankerite occurred during the tectonic uplift stage (Wang Y. Z. et al., 2020).
FIGURE 4. Petrographic features of fluid inclusion assemblages (FIAs) within quartz and carbonate in sandstone reservoirs in the Dongying Depression. (A) FIA occurred as healed microfracture within quartz, details (zoom) of the area shown in the yellow box in Figure 3A. (B–C) Hydrocarbon inclusions within the overgrowth and healed microfracture within the quartz particle show the same fluorescence color, details of the areas shown in the yellow boxes in Figure 3B. (D) Two-phase, oil + gas hydrocarbon inclusions within Fe-calcite, details of the area shown in the yellow box in Figure 3C. V, vapor; L, liquid.
The hydrocarbon accumulation stages and the related diagenetic sequences of the sandstone reservoir in the Dongying Depression are shown in Figure 5. The acidic fluids due to the generation of organic acids as the byproducts of hydrocarbon generation (Heydari and Wade, 2002) in the initial period of the early hydrocarbon accumulation, caused the dissolution of the original feldspar and carbonate minerals (e.g., calcite and dolomite; Wang Y. Z. et al., 2020) in the reservoir. Also, this process was accompanied by the formation of authentic kaolinite and the first overgrowth of quartz. With the continuous consumption of organic acids, Fe–calcite began to precipitate in the late period of early accumulation. After that, the whole region entered the tectonic uplift stage, and the diagenesis was dominated by the cementation of ankerite. During the subsequent late hydrocarbon accumulation, the coeval acidic fluids led to the dissolution of feldspar and carbonate minerals again. Due to the lesser amount of organic acids generated in this stage, the scale of dissolution and quartz overgrowth is lower than that in the early hydrocarbon accumulation stage.
The results of clay mineral contents of the reservoir show that, in most cases, the average content of illite is higher than that of kaolinite (Figure 6). For the sandstone reservoirs that have experienced the both stages (i.e., the early and late) of hydrocarbon accumulation, kaolinite contents are usually quite lower than those of illite. The average contents of the illite and kaolinite in the 4 sags including the Minfeng, Lijin, Niuzhuang, and Boxing are 60.3% vs. 10.3%, 42.4% vs. 9.1%, 41.2% vs. 17.3% and 44.0% vs. 8.5%, respectively. However, for the reservoirs with the only one late stage of hydrocarbon accumulation, except for the Minfeng Sag showing the average lower content of illite than kaolinite (15.3% vs. 55.4%), there is no significant difference in the average contents of the two clay minerals in the Lijin, Niuzhuang, and Boxing sags (i.e., 37.5% vs. 24.3%, 31.2% vs. 23.5%, and 27.7% vs. 22.2%, respectively).
FIGURE 6. Comparison of clay minerals contents between reservoir with the two hydrocarbon accumulation stages and the reservoir with the one late stage in the Dongying Depression.
The early hydrocarbon accumulation mainly affects reservoir physical properties by changing the diagenesis process in sandstones, including the compaction, cementation, and dissolution. Compaction reduces porosity by decreasing the intergranular volume due to the elevation of overburdens, and cementation fills the pores by the precipitation of authigenic minerals. However, dissolution results in the increase of pores through the dissolution of minerals by fluid–rock interaction, which is beneficial to improve the pore structure and reservoir properties. Under constraints by the abovementioned fluid inclusion studies on the hydrocarbon accumulation stages, we conducted the statistical analysis of the physical properties of the sandstone reservoirs with the same lithology and similar burial depth. The results show that (Figure 7), the porosity and permeability of reservoirs that have experienced the early and late two stages of hydrocarbon accumulation are generally superior to those with only one late stage. This indicates, in response to the early hydrocarbon accumulation, the overall porosity increasing due to the production of the secondary pores caused by dissolution, is significantly higher than the porosity reduction caused by compaction and cementation.
FIGURE 7. Comparison of physical properties between the reservoir with the two hydrocarbon accumulation stages and the reservoir with the one late stage in the Dongying Dipression.
Wettability is a tendency of a fluid to stick to the rock surface when other types of fluid are present, which is a physical phenomenon of fluid flowing or expanding on a solid surface under the action of surface molecular forces (Jerauld and Rathmell, 1997). For the statistical analysis of the reservoir wettability (Figure 8), in the samples that have experienced only one late stage of hydrocarbon accumulation, the hydrophilic samples are dominant (87.5%), and the proportion of lipophilic samples is only 2.1%. For the samples that have experienced the early and two late stages of hydrocarbon accumulation, the hydrophilic samples are still dominant, whereas the proportion of lipophilic and neutral-state samples increased to 33.9%. Thus, the inherent wettability (i.e., original hydrophilicity) of reservoir rocks is hard to be altered by the late hydrocarbon accumulation, even though the resource contribution in our study area is considered mainly from the late stage. Alternatively, the early hydrocarbon accumulation is conducive to altering the reservoir wettability. As the increasing of the contact between the early hydrocarbon–bearing fluids and the rock surface, the polar molecules in the non-hydrocarbon and asphaltene would gradually adsorb to the surface, leading to the progressive alteration of reservoir from hydrophilic to the neutral stage, or even lipophilic.
FIGURE 8. Comparison of rock wettability between reservoir with the two hydrocarbon accumulation stages (A) and the reservoir with the one late stage (B) in the Dongying Depression.
The effects of the early hydrocarbon generation and migration into the reservoir mainly include the diageneses (i.e., compaction, cementation, and dissolution) and the resultant alteration of reservoir pore structure and physical properties (porosity and permeability) in sandstones, and the alteration of rock wettability and the resultant formation of dominant pathways during the process of hydrocarbon primary migration. The two effects worked together, and influenced the speed and scale of the late stage of hydrocarbon accumulation.
In general, the increase in burial depth and overburden would cause the damage to the primary pore structure and reduction of physical properties in sandstones. The early stage of hydrocarbon generation and expulsion from source rocks in the Dongying Depression was accompanied by fluid overpressure, with the values ranging from only modestly in excess of hydrostatic, to approaching and perhaps exceeding lithostatic sporadically (Wang M. et al., 2020). Thus, we consider that the early hydrocarbon accumulation could buffer the compaction of the overlying strata, and the buffer capacity depended on the fluid overpressure during accumulation in the reservoir. Moreover, when the early overpressured fluid replaced the original formation water and emplaced the intergranular space (Figure 9), it could support or offset the part of the overburdens, reduce the effective stress of the formation, and greatly preserve the original pores in the reservoir, which are beneficial for the late accumulation.
FIGURE 9. (A–B) Emplacement of the early hydrocarbon (bitumen) in the sandstone in the Dongying Depression.
Acidic and alkalic fluid conditions usually occur at different stages of the diagenetic process (Qiu et al., 2007). Different dissolution and precipitation characteristics of minerals in the reservoir within different fluid conditions will result in a different effect on the reservoir physical properties. In particular, in the alkalic fluids, carbonate and feldspar will precipitate, while dissolution occurs for quartz and its solubility elevates rapidly with the increase of the pH value. This alkalic fluid condition matches the original sedimentary environment (i.e., alkalic lacustrine) of our studied reservoir, which is favorable for the formation of early carbonates and feldspars (Figure 5; Song, 2005). During the subsequent organic matter maturation to yield hydrocarbons, large quantities of CO2 and organic acids could be generated (Surdam et al., 1989). Because the solubility of most minerals is strongly dependent on pore fluid pH, weakly acidic CO2 and organic acids have been implicated in many studies as causative agents for their dissolution, such as carbonate and feldspar (Surdam et al., 1989; Heydari and Wade, 2002). The organic acid anions typically dominate the fluid pH (i.e., organic acid maxima) generally from 80 to 120°C. Thus, during the initial period of early hydrocarbon generation and expulsion from source rocks, a large number of organic acids generated and migrated into the reservoir along with the hydrocarbons, and were partially consumed to neutralize the alkalic formation water. As a result, the fluid condition gradually altered from alkalic to acidic, leading to the dissolution of former carbonate and feldspar, and precipitation of quartz (Figure 5). At temperature greater than 100°C, the organic acids begin to decarboxylate and generate hydrocarbon and CO2, and the rate of this reaction presumably increases with rising temperature. At some point from 120 to 160°C, the organic acid anions will greatly diminish (Surdam et al., 1989). Thus, in the late period of early accumulation, since the fluid altered from acidic to alkalic, deposition of Fe–calcite and ankerite occurred, accompanied by the quartz alkalic dissolution (Figure 5). Therefore, under the influence of the early accumulation, the fluid exhibited an alternated, diagenetic environment from the initial alkalic, subsequent acidic to the final alkalic conditions, which caused the changes of dissolution and precipitation of different minerals. Based on our statistical results (Figure 7), the overall dissolution intensity of the sandstone reservoir was stronger than the cementation during the early stage of hydrocarbon accumulation, resulting in the formation of large numbers of secondary pores, improving the physical properties, and providing more space for the late accumulation.
Both the carbonate acidic dissolution and quartz alkalic dissolution show the high efficiency in producing secondary pores and have great improvement to the reservoir properties. The acidic dissolution of feldspar (i.e., K-feldspar and plagioclase) is considered the main source of the authentic kaolinite and illite in our studied reservoir (Shao et al., 2015; Yuan et al., 2015). Thus, the situation of adding porosity might be different for the feldspar dissolution in a closed fluid system (Yuan et al., 2015). The deposition and stable existence of different clay minerals require different fluid conditions. Acidic fluid is a necessary condition for the stable existence of kaolinite, while illite and chlorite require an alkalic fluid environment that is rich in K+ and Mg2+, respectively. In particular, amid the K+ enriched fluid with high temperature higher than 125°C, illitization of kaolinite could occur (Franks and Zwingmann, 2010). Also, this reaction would cause the consumption of K+ in fluid, further leading to the dissolution of K-feldspars. Based on this, the geological processes that may lead to the current results of clay mineral contents (Figure 6) in the reservoir are as follows. At the initial period of the early hydrocarbon accumulation, acidic fluids dominated by organic acids entered the reservoir and began to preferentially dissolve plagioclases (Huang et al., 2009) in the reservoir, forming the first-stage authigenic kaolinite and releasing the Na+ and Ca2+ into fluids. To the subsequent alkalic fluids in the late period of the early accumulation, due to the low K+ content in the fluid, illitization of kaolinite was inhibited. At this time, the main source of illite is formed by the dehydration of smectite. The dissolution of the K-feldspar and formation of the second-stage authigenic kaolinite occurred, due to the acidic fluids introduction that accompanied the late stage of hydrocarbon accumulation. With the consumption of acidic fluid and increase of pH, kaolinite began to transform into illite in large quantities, thus leading to the reservoirs that have experienced the early and two late stages of hydrocarbon accumulation, showing the characteristics of high illite and low kaolinite content. While for the reservoirs with only one late stage of hydrocarbon accumulation, the acidic fluid was preferred to dissolve in plagioclase rather than K-feldspar to form the kaolinite. The dehydration of smectite occurred to form illite, and the illitization of kaolinite was suppressed due to the lack of K+ in the fluid. Thus, the contents of kaolinite and illite in the current reservoir show little difference, and in some areas, the content of kaolinite is possibly higher than that of illite.
Without any external force, fluid flow in the sandstone reservoir is determined by the capillary force under the influence of pore structure. The wetting fluid could stick to the inner wall of pores and surface of particles, while the non-wetting fluid is generally present as an isolated liquid droplet and hard to be adsorbed on the mineral surface, and the capillary force acts as resistance for its movement. In other words, the wettability of rock affects the fluid flow in pores, and the capillary force is an important driving force for the movement of wetting phase, but a strong resistance for the movement of non-wetting phase.
Before the early hydrocarbon generation and migration from the source rocks to the reservoir, both of these two types of sedimentary rocks were formed in water, and were water-saturated and hydrophilic. Thus, at this time, the capillary force in pores is equal to the resistance of hydrocarbon fluid, which is not conducive to its migration. Only when the external force is greater than the displacement pressure of the wetting phase fluid (i.e., the original sedimentary water in pores), the non-wetting phase (i.e., hydrocarbon fluid) could migrate within the source rocks or reservoir and displaced the original pore water. The early hydrocarbon accumulation could lead to the progressive alteration of reservoir wettability from hydrophilic to the neutral stage, or even the lipophilic stage (Figure 8). Therefore, in these wettability-altered reservoir samples, the early hydrocarbon accumulation would alter the capillary force from the initial resistance to the driving force for hydrocarbon fluid migration in pores. Also, these alterations would further induce preferential migration of the late hydrocarbons in these lipophilic pathway systems. As a result, during the early hydrocarbon fluid generation and expulsion from source rocks to the reservoir, a preferential, lipophilic migration pathway from the source to the reservoir was gradually formed. In addition, the migration of the hydrocarbons generated in the late stage would preferentially inherit this dominant migration pathway.
The overpressured hydrocarbon fluid during the early stage could support and offset some loads of overburden, weaken the normal compaction of the overburden, and preserve some original pores. Also, the early hydrocarbon accumulation resulted in an alternated, diagenetic environment from the initial acidic to subsequent alkalic fluid, with the overall dissolution intensity stronger than the cementation, and formed a large number of secondary pores. Therefore, the early accumulation was beneficial to reservoir space development, and provided more space for the late stage.
In addition, the early hydrocarbon accumulation could cause the alteration of rock wettability. During the process of the early accumulation, a lipophilic migration pathway from the source rock to the reservoir formed gradually. Also, the late stage of hydrocarbon fluid would preferentially choose this dominant pathway for migration (i.e., inheritance of the migration pathway).
The original contributions presented in the study are included in the article/Supplementary Material, further inquiries can be directed to the corresponding author.
MW conducted the analyses, interpreted the data, and wrote the manuscript. YC, GS, HL, YSW, and PZ conceived and designed the study, interpreted the data, and wrote the manuscript. YQ, TC, JL, PL, YF, HZ, QH, and YLW interpreted the data and wrote the manuscript.
This work was supported by the National Natural Science Foundation of China (Nos. 42173042, 42102152, and U1762108), the fellowship of China Postdoctoral Science Foundation (Nos. BX20200385 and 2021M703581), and the Natural Science Foundation of Shandong Province (No. ZR2021QD012).
Authors GS, HL, YSW, PZ, YQ, TC, JL, XL, PL, and YF were employed by the company Shengli Oilfield Company.
The remaining authors declare that the research was conducted in the absence of any commercial or financial relationships that could be construed as a potential conflict of interest.
All claims expressed in this article are solely those of the authors and do not necessarily represent those of their affiliated organizations, or those of the publisher, the editors and the reviewers. Any product that may be evaluated in this article, or claim that may be made by its manufacturer, is not guaranteed or endorsed by the publisher.
Chang, C. Y. (1991). Geological Characteristics and Distribution Patterns of Hydrocarbon Deposits in the Bohai Bay Basin, East China. Mar. Petroleum Geol. 8, 98–106. doi:10.1016/0264-8172(91)90048-6
Chen, Y., Ge, Y. J., Zhou, Z. Z., and Mao, C. (2011). Experimental Study of the Constraint of Minerals Wettability on Hydrocarbon-Bearing Inclusion Forming in Reservoir. Acta Geol. Sin. 85 (04), 569–575. (in Chinese with English abstract).
Chen, Z., Huang, W., Liu, Q., Zhang, L., and Zhang, S. (2016). Geochemical Characteristics of the Paleogene Shales in the Dongying Depression, Eastern China. Mar. Petroleum Geol. 73, 249–270. doi:10.1016/j.marpetgeo.2016.02.022
Franks, S. G., and Zwingmann, H. (2010). Origin and Timing of Late Diagenetic Illite in the Permian-Carboniferous Unayzah Sandstone Reservoirs of Saudi Arabia. Bulletin 94 (8), 1133–1159. doi:10.1306/04211009142
Goldstein, R. H., and Reynolds, T. J. (1994). “Systematics of FLuid Inclusions in Diagenetic Minerals,” in SEPM Society for Sedimentary Geology (Tulsa, OK: SEPM), 31. doi:10.2110/scn.94.31
Guo, X., Liu, K., He, S., Song, G., Wang, Y., Hao, X., et al. (2012). Petroleum Generation and Charge History of the Northern Dongying Depression, Bohai Bay Basin, China: Insight from Integrated Fluid Inclusion Analysis and Basin Modelling. Mar. Petroleum Geol. 32, 21–35. doi:10.1016/j.marpetgeo.2011.12.007
Heydari, E., and Wade, W. J. (2002). Massive Recrystallization of Low-Mg Calcite at High Temperatures in Hydrocarbon Source Rocks: Implications for Organic Acids as Factors in Diagenesis. Aapg Bull. 86, 1285–1303. doi:10.1306/61eedc7e-173e-11d7-8645000102c1865d
Huang, K. K., Huang, S. J., Tong, H. P., and Liu, L. H. (2009). Thermodynamic Calculation of Feldspar Dissolution and its Significance on Research of Clastic Reservoir. Geol. Bull. China. 28 (4), 474–482. (in Chinese with English abstract). doi:10.3969/j.issn.1671-2552.2009.04.008
Jerauld, G. R., and Rathmell, J. J. (1997). Wettability and Relative Permeability of Prudhoe Bay: A Case Study in Mixed-Wet Reservoirs. SPE Reserv. Eng. 12 (01), 58–65. doi:10.2118/28576-pa
Jiang, Y. L., Liu, H., Zhang, L., Tan, L. J., and Wang, N. (2003). Analysis of Petroleum Accumulation Phase in Dongying Sag. Oil Gas. Geo 24 (03), 215–218. (in Chinese with English abstract). doi:10.3321/j.issn:0253-9985.2003.03.005
Qiu, L. W., Zhao, W., and Liu, K. Y. (2007). Alkali Diagenesis and its Application in Jiyang Depression. Pet. Geol. Recy. 14 (2), 10–15. (in Chinese with English abstract). doi:10.3969/j.issn.1009-9603.2007.02.003
Qiu, N. S., Zuo, Y. H., Chang, J., and Li, W. Z. (2014). Geothermal Evidence of Meso-Cenozoic Lithosphere Thinning in the Jiyang Sub-basin, Bohai Bay Basin, Eastern North China Craton. Gondwana Res. 26 (3-4), 1079–1092. doi:10.1016/j.gr.2013.08.011
Shao, S. X., Zeng, J. H., Zhang, S. W., Song, G. Q., Meng, W., Wang, X. J., et al. (2015). Types, Characteristics and Origin of Kaolinite in Sandstone Reservoir of Shahejie Formation, Dongying Sag. Acta Sedimentol. Sin. 33 (06), 1204–1216. (in Chinese with English abstract). doi:10.14027/j.cnki.cjxb.2015.06.013
Song, M. S. (2005). Sedimentary Environment Geochemistry in the Shasi Section of Southern Ramp, Dongying Depression. J. Mineral. Petrol 25 (1), 67–73. (in Chinese with English abstract). doi:10.19719/j.cnki.1001-6872.2005.01.013
Surdam, R. C., Crossey, L. J., Hagen, E. S., and Heasler, H. P. (1989). Organic-inorganic Interactions and Sandstone Diagenesis. Aapg Bull. 73, 1–23. doi:10.1306/703c9ad7-1707-11d7-8645000102c1865d
Wang, M., Chen, Y., Bain, W. M., Song, G., Liu, K., Zhou, Z., et al. (2020a). Direct Evidence for Fluid Overpressure during Hydrocarbon Generation and Expulsion from Organic-Rich Shales. Geology 48, 374–378. doi:10.1130/g46650.1
Wang, Y. Z., Xie, Q. W., Cao, Y. C., Lin, M. R., Wang, Y. S., Li, Y. Z., et al. (2020b). Influence of Multistage Oil Emplacement on Carbonate Cementation in the Eocene Shahejie Formation, Dongying Sag, Bohai Bay Basin, China. Mar. Pet. Geol. 112, 104063.
Worden, R. H., Oxtoby, N. H., and Smalley, P. C. (1998). Can Oil Emplacement Prevent Quartz Cementation in Sandstones? Pet. Geosci. 4 (2), 129–137. doi:10.1144/petgeo.4.2.129
Xie, X., Bethke, C. M., Li, S., Liu, X., and Zheng, H. (2001). Overpressure and Petroleum Generation and Accumulation in the Dongying Depression of the Bohaiwan Basin, China. Geofluids 1, 257–271. doi:10.1046/j.1468-8123.2001.00017.x
Yuan, G., Cao, Y., Gluyas, J., Li, X., Xi, K., Wang, Y., et al. (2015). Feldspar Dissolution, Authigenic Clays, and Quartz Cements in Open and Closed Sandstone Geochemical Systems during Diagenesis: Typical Examples from Two Sags in Bohai Bay Basin, East China. Bulletin 99 (11), 2121–2154. doi:10.1306/07101514004
Keywords: hydrocarbon accumulation, sandstone reservoir, diagenesis, wettability, fluid inclusion
Citation: Wang M, Chen Y, Song G, Liu H, Wang Y, Zhang P, Qiu Y, Chen T, Li J, Liu X, Liu P, Feng Y, Zhang H, Huang Q and Wang Y (2022) Features of the Early Hydrocarbon Accumulation and Its Effects on the Late Accumulation in Sandstone Reservoir: A Case Study of the Eocene Dongying Depression, Bohai Bay Basin. Front. Earth Sci. 10:915747. doi: 10.3389/feart.2022.915747
Received: 08 April 2022; Accepted: 14 April 2022;
Published: 16 May 2022.
Edited by:
Chen Zhang, Chengdu University of Technology, ChinaReviewed by:
Qiqiang Ren, Chengdu University of Technology, ChinaCopyright © 2022 Wang, Chen, Song, Liu, Wang, Zhang, Qiu, Chen, Li, Liu, Liu, Feng, Zhang, Huang and Wang. This is an open-access article distributed under the terms of the Creative Commons Attribution License (CC BY). The use, distribution or reproduction in other forums is permitted, provided the original author(s) and the copyright owner(s) are credited and that the original publication in this journal is cited, in accordance with accepted academic practice. No use, distribution or reproduction is permitted which does not comply with these terms.
*Correspondence: Yong Chen, WW9uZ2NoZW56eUB1cGMuZWR1LmNu
Disclaimer: All claims expressed in this article are solely those of the authors and do not necessarily represent those of their affiliated organizations, or those of the publisher, the editors and the reviewers. Any product that may be evaluated in this article or claim that may be made by its manufacturer is not guaranteed or endorsed by the publisher.
Research integrity at Frontiers
Learn more about the work of our research integrity team to safeguard the quality of each article we publish.