- 1School of Earth Sciences and Engineering, Xi’an Shiyou University, Xi’an, China
- 2Shaanxi Key Laboratory of Petroleum Accumulation Geology, Xi’an Shiyou University, Xi’an, China
- 3PetroChina Zhejiang Oilfield Company, Hangzhou, China
Natural gas is a clean and efficient energy source. Shale gas, one of the unconventional natural gases, is becoming an indispensable part of natural gas. Compared with marine facies shale gas, which has large-scale exploitation, marine and continental transitional facies shale gas have greater development potential. It could be the new direction of development in the future. In this article, the basic geological characteristics, sedimentary environment, and reservoir characteristics of organic-rich transitional facies shale in the southern Sichuan basin are analyzed by lithologic characteristics, chemical element analysis, reservoir space, gas bearing characteristics, and so on and are compared with domestic and abroad transitional shale gas. The results show that the sedimentary period of transitional shale of Upper Permian Leping Formation has a high paleoproductivity level and deposition rate favorable for the accumulation of organic matter in southern Sichuan basin. It was warm and humid paleoclimatic by Sr, V, Cr, Ni, Co, and other trace elements analysis. According to the experimental test analysis of reservoir characteristics, the organic matter is mainly type Ⅲ kerogen, and the thermal evolution degree is mainly overmature in the reservoir. The reservoir space is mainly of microfractures and micropores. The main types of micropore development are intergranular pore, intragranular pore, and organic pore. Compared with other shale gas regions that form industrial airflow, the study region with large thickness, highly brittle mineral content, high level of organic carbon content, and excellent gas-bearing characteristics, it has favorable conditions for shale gas accumulation. The southern Sichuan basin could be the key area for breakthroughs in the exploration and development of transitional shale gas in the next step.
1 Introduction
According to the sedimentary background, shale can be divided into three types: marine facies; transitional facies; and continental facies (Montgomery et al., 2006; Liang et al., 2008). In China, marine facies shale is mainly developed in Paleozoic strata, transitional facies shale in Carboniferous–Permian strata, and continental facies shale in Cenozoic strata (Zou et al., 2015; Dong et al., 2016; Zou et al., 2016; Li., 2022). At present, China has achieved great success in shallow, middle, and deep marine shale gas (Liang et al., 2016; Yang et al., 2019; Zhang et al., 2020a; Liang et al., 2020). The exploration and research of transitional facies shale gas are at the initial stage. Previous studies have shown that China’s transitional facies shale gas resource distribute widely and has great resource potential, such as Ordos basin, Qinshui basin, and southern of north China basin. Transitional facies shale gas resource of 19.8 × 1012 m3, accounted for 25% of the total shale gas resource in China. Although the study of transitional facies shale gas started late, it will have great exploration and developmental prospects (Zou et al., 2010; Dong et al., 2016; Yang et al., 2017; Guo et al., 2018; Kuang et al., 2020).
It is currently believed that the Ordos basin and Sichuan basin are the regions most likely to take the lead in the commercial development of transitional facies shale gas. The favorable areas of the two basins can reach 13.3 × 104 km2, accounting for 72% of the whole country. The total geological resources of the two basins can reach 13.5 × 1012 m3, accounting for 68% of the whole country (Chen et al., 2012; Ding et al., 2013; Shan et al., 2015; Li et al., 2019). Zhaotong National Shale Gas Demonstration Zone, which is located at the junction of Yunnan, Guizhou, and Sichuan, has realized the commercial development of coal bed methane of the upper Permian Leping formation and the production reached 1.1 × 108 m3 in 2019. At an early stage, Shan Chang’an and other researchers have done a lot of basic research on coalbed methane reservoir and gas accumulation mechanism of Leping formation in Zhaotong Demonstration Zone (Chen et al., 1999; Shan et al., 2018; Kang et al., 2019; Shan et al., 2019; Shan et al., 2020). Petro China Zhejiang Oilfield Company had found good carbonaceous shale gas in the process of drilling and evaluating the coalbed methane resources of Leping formation, the highest gas measured value up to 67%. This article systematically combs the geological characteristics of the transitional facies shale gas of the upper Permian Leping formation in the southern Sichuan basin (Figure 1), making clear the geological characteristics and resource potential of transitional facies shale gas in the Leping Formation of the southern Sichuan basin. The obtained knowledge will be of great significance to the exploration and development of transitional shale gas in other areas.
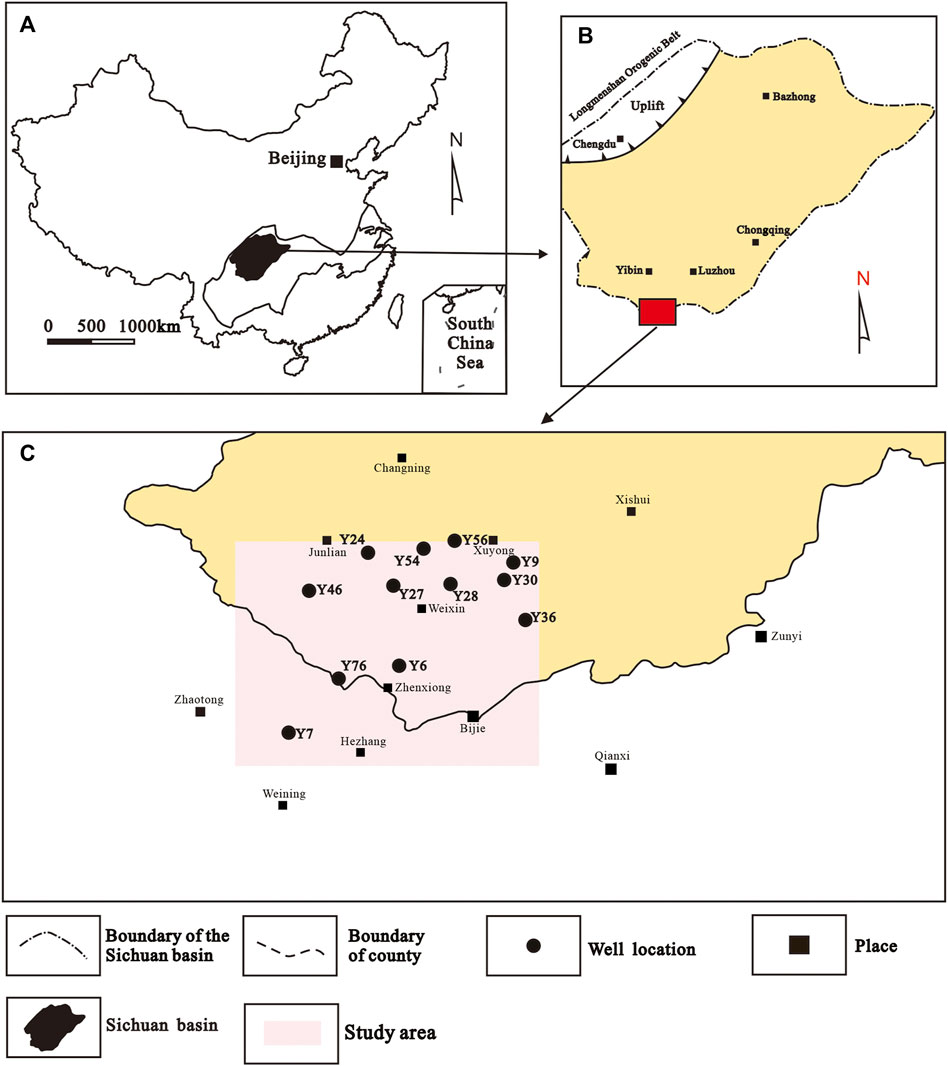
FIGURE 1. Schematic diagram of the study area. (A) Tectonic location of the Sichuan Basin within China. (B) Location of the study area in the Sichuan Basin. (C) Distribution of the study wells.
2 Geological Setting
Most areas of Yunnan, Guizhou, and Sichuan were uplifted to the earth’s crust at the end of the Middle Permian, and the Middle Permian of Maokou formation suffered from weathering and denudation in different degrees (Chen et al., 1999; Ma et al., 2009; Shen et al., 2019; Wang et al., 2020; Gao., 2021). The sedimentation under the bottom coal seam of the Leping formation in the study area is the product of the swamp developed on the erosive surface (Shao et al., 1998; Zheng and Hu, 2010; Shao et al., 2013; Xiao et al., 2020). Subsequently, a large-scale basalt eruption occurred in the western region, and the basalt wedge-shaped layers were accumulated on the planation surface of the Maokou formation limestone. After that, the tectonic movement gradually changed from ascending to descending, which started the deposition of the main strata in the Late Permian (Chen et al., 1999; Ma et al., 2009; Zhang et al., 2020b; Yu et al., 2022).
After the end of the Dongwu movement, a large area of Yunnan, Guizhou, and Sichuan began to subside and sea water intruded into the eastern Sichuan from southeast through Southeast Guizhou (Zhao et al., 2012; Luo et al., 2014; Su et al., 2020; Sun, 2020). In the Late Permian, northwest Guizhou and southeast Sichuan often alternate between a shallow beach land and coastal plain, and the typical coal bearing formation of land–sea interaction developed (China General Administration of Coalfield Geology, 1996; China General Administration of Coalfield Geology, 1999). The typical shallow marine limestone in the coal seam is missing, and the environment is mainly coastal plain and transitional facies due to the influence of Luzhou and Central Guizhou Uplift in the eastern part of southern the Sichuan basin. The overall topography of the study area slopes from southwest to northeast. It is an offshore piedmont plain in the western part of the study area. The transgression zone, which is in the eastern part of the study area, forms a large area of tidal flat swamp facies (Figure 2A). At this time, the lacustrine facies and fluvial facies, dominated by continental sand and mudstone deposits, were formed in the western side of the study area (China General Administration of Coalfield Geology, 1996; China General Administration of Coalfield Geology, 1999), and occlusion water swamp facies developed locally. However, stable swamp was difficult to form because of the rapid crustal vein-like subsidence, large terrain differentiation, and uneven basement. In addition, the cycle development was poor because of complex surface water flow, strong hydrodynamic force, and the thickness and phase transition of sediments, especially clastic rocks that changed frequently. With the continuous advance of sea water from the east to west, the coal measure strata deposited in tidal flat gradually migrated from east to west, so as to develop the tidal flat swamp facies widely in the centre of study area in the late deposition of Leping formation (Figure 2B).
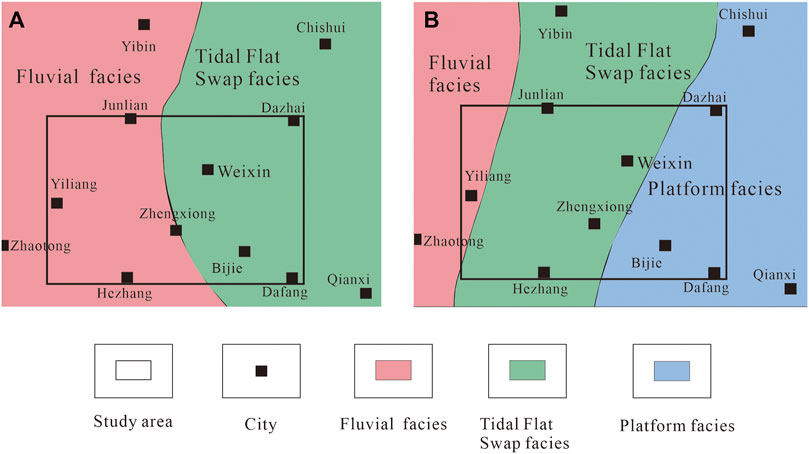
FIGURE 2. Sedimentary stage of Leping Formation. (A) Early sedimentary stage of Leping Formation. (B) Late sedimentary stage of Leping Formation.
3 Reservoir Characteristics
3.1 Lithologic Association and Distribution Characteristics
The overlying strata of Leping formation is green silty mudstone of Feixianguan formation, and the underlying strata of Leping formation is basalt of Emeishan formation or limestone of Maokou formation. The top and bottom boundary of the study area is clearly marked with good contrast. The coal measure strata of Leping formation is generally in the transitional facies sedimentary environment, with frequent interaction of sandstone, mudstone, and coal seam which reflects the frequent turbulence of sea water. The sea water continuously advanced from the west to east, and the coal measure strata are gradually distributed upward from the east to west during the whole sedimentation period of Leping formation. The lithology of Leping formation can be divided into three lithologic associations from east to west in the study area (Figure 3): A type (upper coal and lower sand type) in the west; B type (top ash, middle coal, and lower sand type) in the middle; and C type (upper ash and lower coal type) in the east.
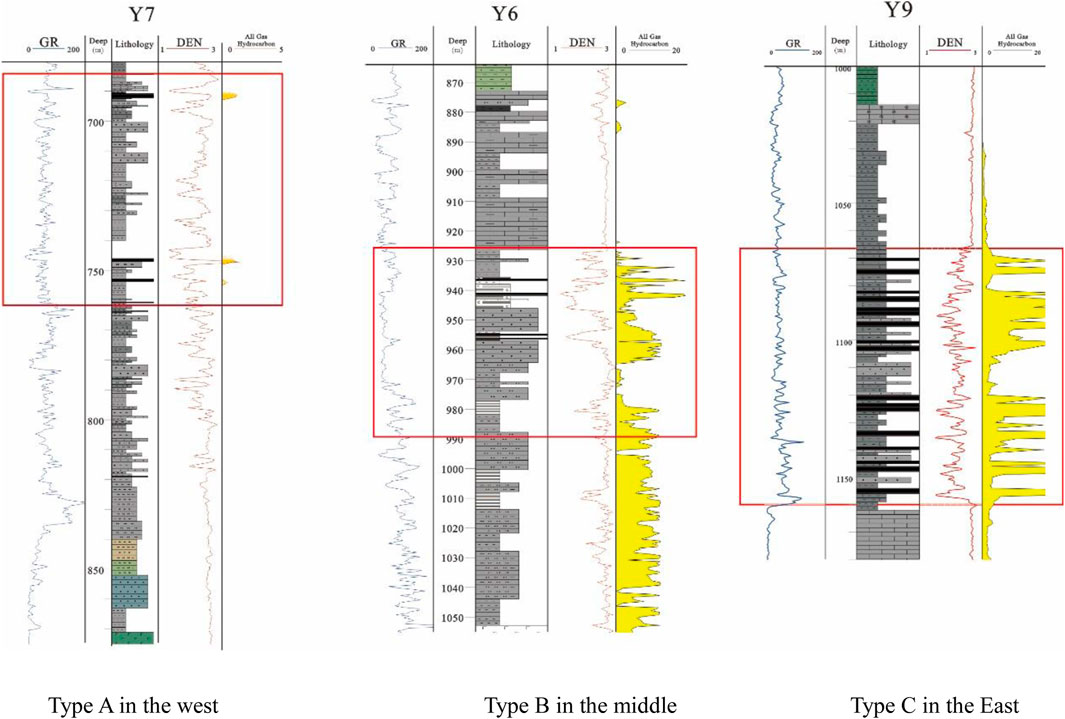
FIGURE 3. Lithological association and distribution characteristics of the study area. The red box represents the Leping Formation. Type A in the west. Type B in the middle. Type C in the east.
Type A (upper coal and lower sand): in the upper section, sand, coal, mud, carbonaceous mudstone interbedded; in the lower section, sand and mud interbedded, mainly in Junlian, Hezhang, and other areas in the west of the study area. Type B (top ash, medium coal, and lower sand): a small amount of limestone at the top, sand, coal, and mud interbedded in the middle section, sand and mud interbedded in the lower section, mainly in Gongxian and Zhenxiong areas in the middle of the study area. Type C (upper ash and lower coal): interbedded limestone, mud, and coal in the upper section, interbedded sand, coal, mud, and carbonaceous mudstone in the lower section, mainly in the eastern Xuyong area of the study area.
The mudstone series in the coal measure strata of Leping formation can be further divided into two types: mudstone and carbonaceous mudstone (carbonaceous shale). Thus, the main lithology in Leping formation includes four types: carbonaceous mudstone (carbonaceous shale), coal, mudstone, and sandstone (Table 1). Coal, mudstone, and sandstone are generally easier to distinguish by the field profile or naked eye core observation. Coal and carbonaceous mudstone (carbonaceous shale) are difficult to distinguish macroscopically. Thus, they are usually distinguished by the amount of organic carbon in the laboratory. In general, carbonaceous mudstone (carbonaceous shale), coal, sandstone, and mudstone have obvious differences in conventional logging (gamma, neutron, resistivity, and density) and electric imaging logging. In addition, the four types of lithology are different in mineral composition and organic carbon content. The organic carbon content is often used as the criterion for distinguishing the four types of lithology: the organic carbon content of carbonaceous mudstone is between 6 and 40%, the organic carbon content of coal is more than 40%, and that of mudstone and sandstone is less than 6% (Wang et al., 2005). The specific differences are shown in Table 1.
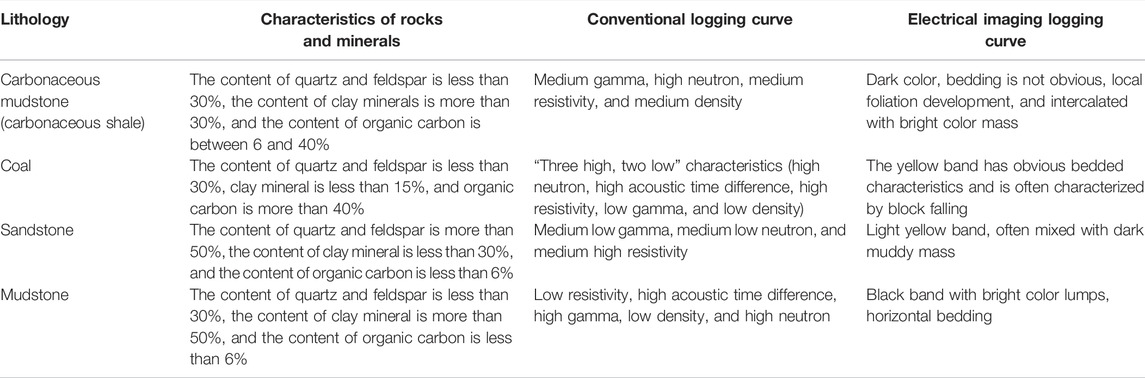
TABLE 1. Statistics of lithology and logging characteristics of carbonaceous mudstone, coal, sandstone, and mudstone.
According to the laboratory test results (Figure 4), the mineral composition of the transitional facies shale reservoir is composed of quartz, feldspar, pyrite, and clay minerals. The average content of brittle minerals is 66.9%, and the average content of clay minerals is 33.1%. There is a small amount of anatase in the stratum which is speculated to be Emeishan basalt formation. There are three types of clay minerals in Leping Formation. Chlorite content is the highest in clay mineral, ranging from 28 to 61%, with an average of 41.5%. The second clay mineral is iron-montages, accounting for 20–52%, with an average of 35.7%. The remaining clay mineral is kaolinite.
3.2 Geochemical Characteristics
3.2.1 Elemental Analysis
Sedimentary rock is formed by the redistribution of elements in the earth’s crust. In the deposition process, the physical and chemical changes of various elements follow sediments as carriers, and the phenomena of migration, transformation, combination, and dispersion had taken place. Because of the difference of sedimentary environment, the accumulation rule of elements is also different. Therefore, the sedimentary environment can be identified by the feature of element variation. An X-ray fluorescence spectrometer was used to test the element content of the samples. The depositional environment of Leping Formation is analyzed according to the geochemical indexes from test results. According to the previous research results, Chemical Index of Alteration (CIA) can be used to judge the paleoclimate of the study area (Nesbitt and Young, 1982; McLennan et al., 1993). In general, the high CIA values represent warm and wet paleoclimate and the low CIA values reflect dry and cold paleoclimate. The CIA between 50 and 65 reflects the dry and cold climate under the background of low degree chemical weathering; the CIA between 65 and 85 reflects the warm and humid climate under the background of moderate chemical weathering; and the CIA between 85 and 100 reflects the thermal and humid climate under the background of strong chemical weathering. In addition, Sr/Cu ratio is also a common indicator to distinguish the climatic environment. The Sr/Cu ratio of 1.3–5.0 indicates a warm and humid climate; the Sr/Cu ratio greater than 5.0 indicates a dry and thermal climate (Lerman and Baccini, 1978). According to the measured results, the CIA of the rock samples from the Leping Formation in the study area is between 81.09 and 95.66, with an average of 86.10 (Table 2); the Sr/Cu ratio is between 0.66 and 51.44, with an average of 5.23 (Table 2). However, it was found that the maximum value of Sr/Cu was 51.44, which was abnormally high. Thus, after removing the abnormal value, the Sr/Cu ratio is between 0.66 and 23.17, with an average of 4.34 (Table 2). Finally, it is concluded that the Leping Formation was deposited in warm and humid paleoclimate.
3.2.2 Organic Geochemical Analysis
The samples from more than 10 wells in the study area were tested experimentally. The results show that the TOC values of carbonaceous shale range from 13.0 to 29.4% on an average (Figure 5) and vitrinite reflectance range from 3.11 to 3.81%, and most samples are around 3.2% (Table 3). The Leping Formation is overmature source rock. This indicates that the Leping Formation shale has a high TOC content and high thermal evolution degree. Organic maceral testing shows that the main composition is vitrinite, with a part of inertinite, and little exinite (Figure 6). The organic matter type of Leping Formation carbonaceous shale is mainly type III.
3.3 Physical Characteristics
Organic and inorganic pores are well developed in the shale of the Leping formation by scanning electron microscopy of rock samples in the study area. Referring to the classification methods of Loucks et al. (2012) and Jiang et al. (2022), we divide the shale pore into interparticle pore, intraparticle pore, interbedded pore of clay minerals, intergranular pore of pyrite, organic pore, and microfracture. The interparticle pore (Figures 7A,B) is mainly formed by the arrangement and accumulation of mineral particles or diagenesis compaction. It is commonly found between crystal particles. Sometimes, some interparticle pores are filled with clay minerals. The intraparticle pore (Figures 7C,D) is formed by diagenetic transformation or dissolution of minerals. It is commonly found in minerals. The interbedded pore of clay minerals (Figures 7E,F) is cracked between the layered clay minerals. The intergranular pore (Figures 7G,H) of pyrite is the pore that remains after the pyrite crystals packed loosely during the growth process. It mostly appears as pyrite framboids. The organic pore (Figures 7I,J) is mostly formed by gas production or the gas escaped from organic matter within minerals. The formation of microfracture (Figures 7K,L) is related to stress changes caused by the sedimentary structure and diagenetic evolution (Fu et al., 2019; Zhang et al., 2021; Liu et al., 2022).
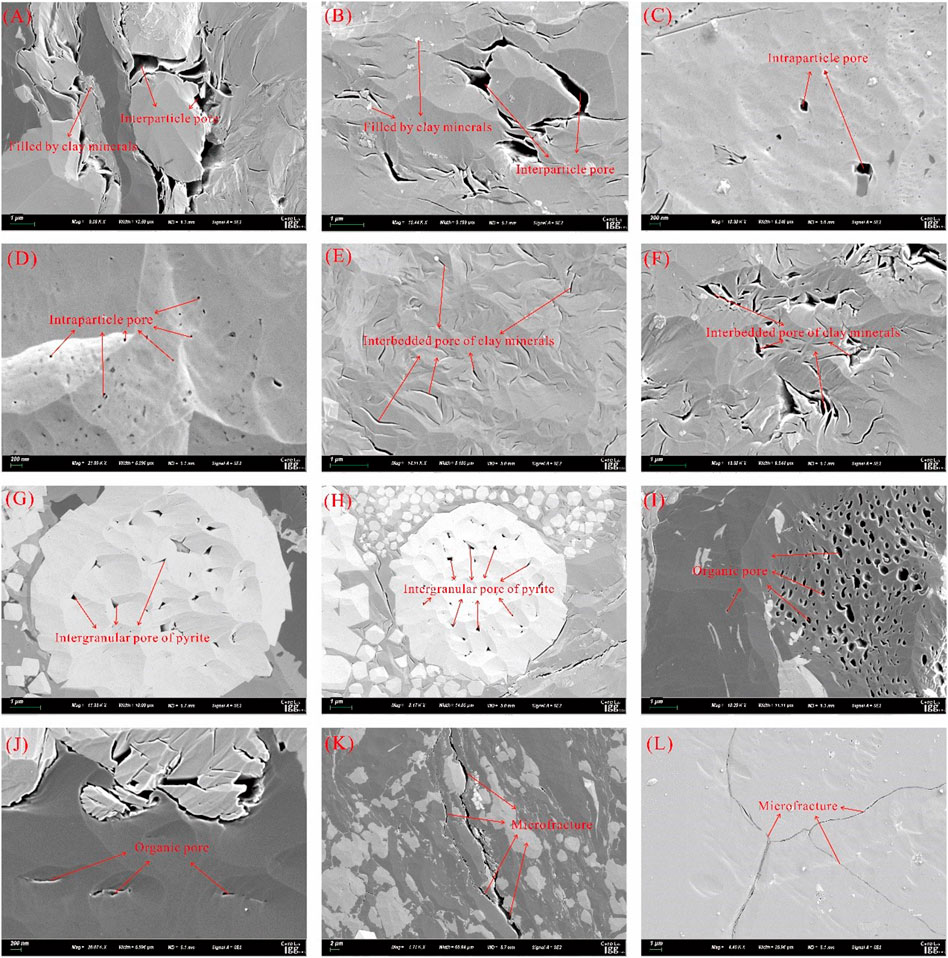
FIGURE 7. Different types of pores. (A,B) Interparticle pore. (C,D) Intraparticle pore. (E,F) Interbedded pore of clay minerals. (G,H) Intergranular pore. (I,J) Organic pore. (K,L) Microfracture.
A number of wells in the study area are selected to test the Leping formation physical characteristics by the nuclear magnetic resonance technology (NMR). The results showed that the Leping Formation carbonaceous shale gas reservoir in the study area has high porosity and relatively low permeability (Shan et al., 2015; Zhang et al., 2020c; Zhang et al., 2022a; Zhang et al., 2022b; Zhang et al., 2022c). The total porosity of mud shale ranges from 7.7 to 10.4% (Figure 8), and the permeability is generally less than 0.10 mD, with an average of about 0.005 mD.
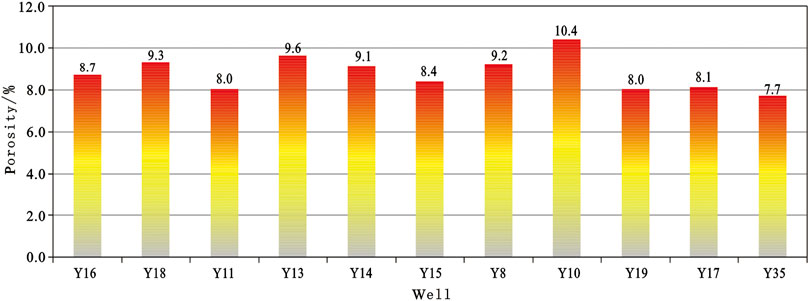
FIGURE 8. Porosity distribution histogram of the Leping Formation carbonaceous shale in the study area.
3.4 Gas-Bearing Characteristics
Gas-bearing characteristics is an important index to evaluate whether shale gas is worthy of exploration and development value. Understanding the gas bearing characteristics is the prerequisite for the next work (Daniel and Bustin, 2009; Wang and Gale, 2009; Huang et al., 2020; Zhang et al., 2022d; Zhang et al., 2022e). Based on the field gas content test of the carbonaceous shale samples from several wells in study area, it is found that Leping Formation carbonaceous shale has a high shale gas content. The total gas content of the carbonaceous shale ranges from 5.3 m3/t to 7.3 m3/t, with an average of 6.1 m3/t, showing the good gas bearing characteristics of Leping Formation (Figure 9).
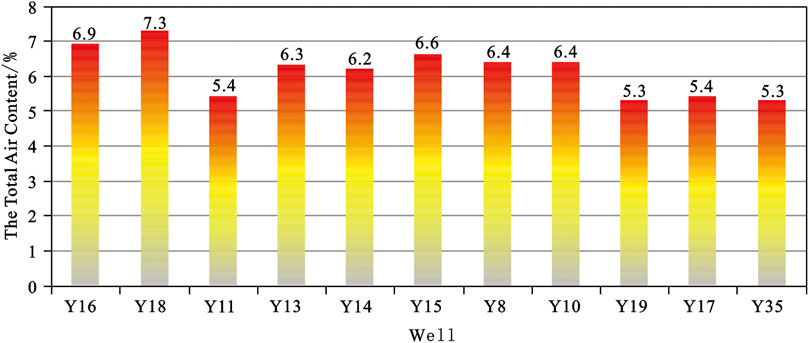
FIGURE 9. Total gas content distribution histogram of the Leping Formation carbonaceous shale in the study area.
4 Resource Potential Analysis
Based on the analysis of sedimentary, stratigraphic, and reservoir geological conditions of the transitional shale in the Leping Formation of study area, it is concluded that the study area has a good potential for transitional shale gas exploration. According to the preliminary geological survey, drilling data, and laboratory test analysis, two favorable areas have been initially selected in the study area which can be used as breakthrough areas for the next key exploration. The optimal favorable area for the transitional carbonaceous shale of Leping Formation is 380 km2 with nearly 680 × 108 m3 of transitional shale gas resources in the A area. The optimal favorable area for the transitional carbonaceous shale of Leping Formation is 86 km2 with nearly 140 × 108 m3 of transitional shale gas resources in B area. The total accumulation of resources is nearly 800 × 108 m3 in the favorable areas (Figure 10). In July 2020, Petro China Zhejiang Oilfield Company drilled an evaluation well for the transitional carbonaceous shale gas of Leping Formation in the B area. The gas bearing test was carried out for the coal rock, shale with thin coal and shale in this set of coal measure strata. The results show that the gas content of coal rock is the highest, maximum up to 54 m3/t, the average gas content of mudstone with thin coal is 14.8 m3/t; the gas content of mudstone is low, but the average gas content can reach 3.0 m3/t. In summary, these wells show very good gas bearing characteristics which further proves that the study area has good shale gas exploration and developmental prospects (Kelts and Hsü, 1978; Zhang et al., 2022).
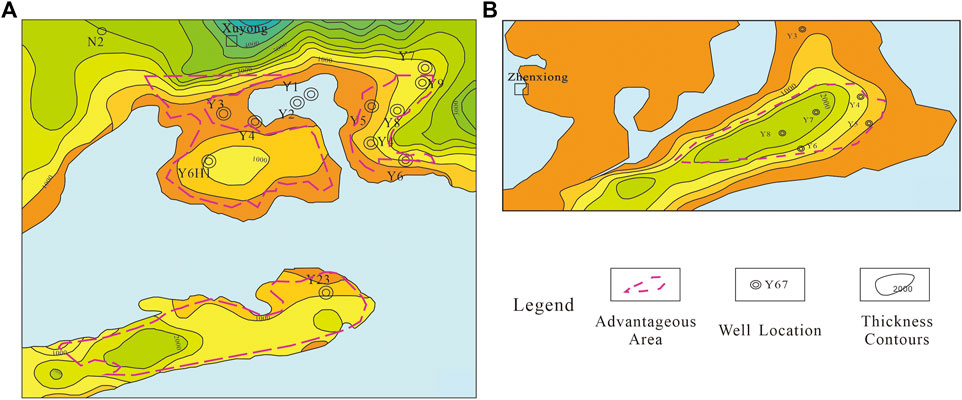
FIGURE 10. Distribution and resource quantity of transitional shale gas favorable areas in Leping Formation of the study area.
5 Conclusion
1) During the deposition period of Leping Formation, the study area experienced the retrogradation evolution process which led to the gradual deepening of the water body, and the coal measures strata deposited in the tidal flat swamp also gradually distributed upward from the east to west. There are four types of coal measure strata in the Leping Formation: carbonaceous mudstone, coal, mudstone, and sandstone. From east to west, the study area can be divided into three lithologic associations: type A (upper coal and lower sand type), type B (middle coal, lower sand, and top ash type), and type C (upper ash and lower coal type).
2) The content of brittle minerals in the transitional shale gas reservoir of Leping Formation in the study area is higher (average 66.9%), followed by clay minerals (average 33.1%). The content of chlorite is the highest in the clay minerals (41.5% on average), followed by the illite–montmorillonite mixture (35.7% on average). The TOC content is relatively high (average 17.9%); organic macerals are mainly vitrinite, followed by inertinite; kerogen type is mainly type III; vitrinite reflectance (Ro) is about 3.2%, which belongs to overmature source rock.
3) For the transitional shale gas of Leping Formation, two favorable areas are preliminarily selected in the study area, which can be used as breakthrough blocks for further exploration. The favorable area of the A area is 380 km2, and the resource amount is 680 × 108 m3; the favorable area of B district is 86 km2, and the resource amount is nearly 140 × 108 m3; the total resource accumulation is nearly 800 × 108 m3.
Data Availability Statement
The raw data supporting the conclusions of this article will be made available by the authors, without undue reservation.
Author Contributions
CS, CY, and ZZ contributed to conception and design of the study. ZZ, CZ, and KY organized the database. CS, CY, YS, and BL performed the statistical analysis. FH, HZ, and JM helped perform the analysis with constructive discussions. CS and CY wrote the first draft of the manuscript. All authors contributed to manuscript revision, read, and approved the submitted version. FZ contributed to conception and design of the study. YD performed the statistical analysis.
Funding
This study was supported by the open funds from the Natural Science Basic Research Plan in Shaanxi Province of China (grant no. 2019JQ-100).
Conflict of Interest
ZZ, CZ, FH, HZ, JM, and KY were employed by the Company PetroChina Zhejiang Oilfield Company.
The remaining authors declare that the research was conducted in the absence of any commercial or financial relationships that could be construed as a potential conflict of interest.
Publisher’s Note
All claims expressed in this article are solely those of the authors and do not necessarily represent those of their affiliated organizations or those of the publisher, the editors, and the reviewers. Any product that may be evaluated in this article, or claim that may be made by its manufacturer, is not guaranteed or endorsed by the publisher.
Acknowledgments
We sincerely appreciate all reviewers and the handling editor for their critical comments and constructive suggestions.
References
Chen, H. D., Qin, J. X., Wang, C. S., Shou, J. F., and Li, X. H. (1999). Sequence-Based Lithofacies and Paleogeographic Characteristics and Evolution of Permian in South China. Acta Sedimentol. Sin. 17 (4), 510–521. doi:10.1088/0256-307X/15/11/025
Chen, X., Bao, S., Hou, D., and Mao, X. (2012). Methods and Key Parameters for Shale Gas Resource Evaluation. Petroleum Explor. Dev. 39 (5), 605–610. doi:10.1016/s1876-3804(12)60082-2
China General Administration of Coalfield Geology (1996). Sedimentary Environment of Late Permian Coal-Bearing Strata in Western Guizhou, Southern Sichuan and Eastern Yunnan. Chongqing: Chongqing University Press, 362.
China General Administration of Coalfield Geology (1999). Characteristics of Permian Coal-Bearing Basin in South China and Coal Accumulation Law. Beijing: Jiangxi Science and Technology Press, 379.
Ding, W., Zhu, D., Cai, J., Gong, M., and Chen, F. (2013). Analysis of the Developmental Characteristics and Major Regulating Factors of Fractures in Marine-Continental Transitional Shale-Gas Reservoirs: A Case Study of the Carboniferous-Permian Strata in the Southeastern Ordos Basin, Central China. Mar. Petroleum Geol. 45, 121–133. doi:10.1016/j.marpetgeo.2013.04.022
Dong, D. Z., Wang, Y. M., Li, X. J., Zou, C. N., Guan, Q. Z., Zhang, C. C., et al. (2016). Breakthrough and Prospect of Shale Gas Exploration and Development in China. Nat. Gas. Ind. 36 (1), 19–32. (in Chinese with English abstract). doi:10.1016/j.ngib.2016.02.002
Ewing, T. E., Jarvie, D. M., Bowker, K. A., and Pollastro, R. M. (2006). Mississippian Barnett Shale, Fort Worth Basin, North-Central Texas: Gas-shale Play with Multi-Trillion Cubic Foot Potential: Discussion. Bulletin 90 (6), 963–966. doi:10.1306/02090605132
Fu, J., Guo, S., and Liao, G. (2019). Pore Characterization and Controlling Factors Analysis of Organic-Rich Shale from Upper Paleozoic Marine-Continental Transitional Facies in Western Ordos Basin of China. Energy Procedia 158, 6009–6015. doi:10.1016/j.egypro.2019.01.518
Gao, F. Q. (2021). Influence of Hydraulic Fracturing of Strong Roof on Mining-Induced Stress Insight from Numerical Simulation. J. Min. Strata Control Eng. 3 (2), 5–13. doi:10.13532/j.jmsce.cn10-1638/td.20210329.001
Guo, X. S., Hu, D. F., Liu, R. B., Wei, X. F., and Wei, F. B. (2018). Geological Conditions and Exploration Potential of Permian Marine-Continent Transitional Facies Shale Gas in the Sichuan Basin. Nat. Gas. Ind. 38 (10), 17–24. doi:10.3787/j.issn.1000-0976.2018.10.002
Huang, H., Li, R., Jiang, Z., Li, J., and Chen, L. (2020). Investigation of Variation in Shale Gas Adsorption Capacity with Burial Depth: Insights from the Adsorption Potential Theory. J. Nat. Gas Sci. Eng. 73, 103043. doi:10.1016/j.jngse.2019.103043
Jiang, T., Jin, Z., Liu, G., Hu, Z., Chen, X., Liu, Z., et al. (2022). Investigating the Pore Structure Characteristics and Reservoir Capacities of Lower Jurassic Continental Shale Reservoirs in the Northeastern Sichuan Basin, China. Front. Earth Sci. 10, 886907. doi:10.3389/feart.2022.886907
Kang, H. P., Xu, G., Wang, B. M., Wu, Y. Z., Jiang, P. F., Pan, J. F., et al. (2019). Forty Years Development and Prospects of Underground Coal Mining and Strata Control Technologies in China. J. Min. Strata Control Eng. 1 (1), 013501. doi:10.13532/j.jmsce.cn10-1638/td.2019.02.002
Kelts, K., and Hsü, K. J. (1978). “Freshwater Carbonate Sedimentation,” in Lakes. Editor A. Lerman (New York, NY: Springer), 295–323. doi:10.1007/978-1-4757-1152-3_9
Kuang, L. C., Dong, D. Z., He, W. Y., Wen, S. M., Sun, S. S., Li, S. X., et al. (2020). Geological Characteristics and Development Potential of Transitional Shale Gas in the East Margin of the Ordos Basin, NW China. Petroleum Explor. Dev. 47 (3), 435–446. doi:10.1016/S1876-3804(20)60066-0
Lerman, A., and Baccini, P. (1978). Lakes: Chemistry, Geology, Physics. New York, NY: Springer-Verlag 17 (1-2), 72–74. doi:10.1016/0013-7952(81)90028-4
Li, H. (2022). Research Progress on Evaluation Methods and Factors Influencing Shale Brittleness: A Review. Energy Rep. 8, 4344–4358. doi:10.1016/j.egyr.2022.03.120
Li, Y., Wang, Z., Pan, Z., Niu, X., Yu, Y., and Meng, S. (2019). Pore Structure and its Fractal Dimensions of Transitional Shale: A Cross-Section from East Margin of the Ordos Basin, China. Fuel 241 (Apr.1), 417–431. doi:10.1016/j.fuel.2018.12.066
Liang, D. G., Guo, T. L., Chen, J. P., Bian, L. Z., and Zhao, Z. (2008). Some Progresses on Studies of Hydrocarbon Generation and Accumulation in Marine Sedimentary Regions, Southern China (Part1): Distribution of Four Suits of Regional Marine Source Rocks. Mar. Orig. Pet. Geol. 13 (02), 1–16. (in Chinese with English abstract). doi:10.3969/j.issn.1672-9854.2008.02.001
Liang, X., Wang, G. C., Xu, Z. Y., Zhang, J. H., Chen, Z. P., Xian, C. G., et al. (2016). Comprehensive Evaluation Technology for Shale Gas Sweet Spots in the Complex Marine Mountains, South China: A Case Study from Zhao Tong National Shale Gas Demonstration Zone. Nat. Gas. Ind. 36 (1), 33–42. (in Chinese with English abstract). doi:10.1016/j.ngib.2016.02.003
Liang, X., Xu, Z. Y., Zhang, Z., Wang, W. X., Zhang, J. H., Lu, H. L., et al. (2020). Breakthrough of Shallow Shale Gas Exploration in Taiyang Anticline Area and its Significance for Resource Development in Zhaotong, Yunnan Province, China. Petroleum Explor. Dev. 47 (1), 11–28. doi:10.1016/s1876-3804(20)60002-7
Liu, J. P., Liu, D. X., Hu, H. Y., Jiao, P. F., Wang, T., Xie, Z. T., et al. (2022). Pore Structure Characteristics of Marine Continental Transitional Facies Shale of Shanxi Formation in the Eastern Margin of Ordos Basin. China Sci. 17 (1), 21–30. doi:10.3969/j.issn.2095-2783.2022.01.004
Loucks, R. G., Reed, R. M., Ruppel, S. C., and Hammes, U. (2012). Spectrum of Pore Types and Networks in Mudrocks and a Descriptive Classification for Matrix-Related Mudrock Pores. Bulletin 96 (6), 1071–1098. doi:10.1306/08171111061
Luo, J. X., He, B. Y., and Wang, R. (2014). Lithofacies Palaeogeography of the Late Permian Wujiaping Age in the Middle and Upper Yangtze Region, China. J. Palaeogeogr. 3 (04), 384–409. doi:10.3724/SP.J.1261.2014.00063
Ma, Y. S., Chen, H. D., and Wang, G. Li. (2009). Sequence Stratigraphy and Paleogeography in Southern China. Beijing: Science Press, 603.
McLennan, S. M., Hemming, S., McDaniel, D. K., and Hanson, G. N. (1993). Geochemical Approaches to Sedimentation, Provenance, and Tectonics. Special Pap. Geol. Soc. Am. 284, 21–40. doi:10.1130/SPE284-p21
Nesbitt, H. W., and Young, G. M. (1982). Early Proterozoic Climates and Plate Motions Inferred from Major Element Chemistry of Lutites. Nature 299 (5885), 715–717. doi:10.1038/299715a0
Ross, D. J. K., and Marc Bustin, R. (2009). The Importance of Shale Composition and Pore Structure upon Gas Storage Potential of Shale Gas Reservoirs. Mar. Petroleum Geol. 26 (6), 916–927. doi:10.1016/j.marpetgeo.2008.06.004
Shan, C., Zhang, T., Guo, J., Zhang, Z., and Yang, Y. (2015). Characterization of the Micropore Systems in the High-Rank Coal Reservoirs of the Southern Sichuan Basin, China. Bulletin 99 (11), 2099–2119. doi:10.1306/07061514240
Shan, C., Zhang, T., Liang, X., Zhang, Z., Zhu, H., Yang, W., et al. (2018). Influence of Chemical Properties on CH 4 Adsorption Capacity of Anthracite Derived from Southern Sichuan Basin, China. Mar. Petroleum Geol. 89, 387–401. doi:10.1016/j.marpetgeo.2017.10.008
Shan, C. A., Zhang, T., Liang, X., Shu, D., Zhang, Z., Wei, X., et al. (2019). Effects of Nano-Pore System Characteristics on CH4 Adsorption Capacity in Anthracite. Front. Earth Sci. 13 (1), 75–91. doi:10.1007/s11707-018-0712-1
Shan, C. A., Zhang, T. S., Liang, X., Hu, R. R., and Zhao, W. W. (2020). Nanopore Structure Characteristics of High–Rank Vitrinite–And Inertinite–Coal. Acta Pet. Sin. 41, 723–736. doi:10.7623/syxb202006007
Shao, L. Y., Liu, H. M., Tian, B. L., and Zhang, P. F. (1998). Sedimentary Evolution and its Controls on Coal Accumulation for the Late Permian in the Upper Yangtze Area. Acta Sedimentol. Sin. 16 (2), 55–60.
Shao, L. Y., Gao, C. X., Zhang, C., Wang, H., Guo, L. J., and Gao, C. H. (2013). Sequence-palaeogeography and Coal Aaccumulation of Late Permian in Southwestern china. Acta Sedimentol. Sin. 31 (5), 856–866. doi:10.14027/j.cnki.cjxb.2013.05.006
Shen, S. Z., Zhang, H., Zhang, Y. C., Yuan, D. X., Chen, B., He, W. H., et al. (2019). Permian Integrative Stratigraphy and Timescale of China. Sci. China (Earth Sci. 62 (01), 154–188. doi:10.1007/s11430-017-9228-4
Su, W., Hu, S., Jiang, Q., Zhang, J., Huang, S., Jiang, H., et al. (2020). Sedimentary Responses to the Dongwu Movement and the Influence of the Emeishan Mantle Plume in Sichuan Basin, Southwest China: Significance for Petroleum Geology. Carbonates Evaporites 35 (4), 108. doi:10.1007/s13146-020-00638-w
Sun, L. C. (2020). Study on Permian Lithofacies Paleogeography in the Southeastern Margin of Sichuan Basin. Shandong Univ. Sci. Technol. 6, 46–48. doi:10.27275/d.cnki.gsdku.2020.001221
Wang, F. P., and Gale, J. F. W. (2009). Screening Criteria for Shale-Gas Systems. Gulf Coast Assoc. Geol. Soc. Trans. 59, 779–793.
Wang, J., Zhang, X. B., and Chen, J. Fa. (2005). Evaluation of the Hydrocarbon-Generating Characteristic for Coal-Bearing Hydrocarbon-Source Rocks of Suligemiao Gas Field. Coal Geol. Explpration 33 (2), 26–29. doi:10.3969/j.issn.1001-1986.2005.02.008
Wang, J., Zhang, C., Zheng, D., Song, W. D., and Ji, X. F. (2020). Stability Analysis of Roof in Goaf Considering Time Effect. J. Min. Strata Control Eng. 2 (1), 013011. doi:10.13532/j.jmsce.cn10-1638/td.2020.01.005
Xiao, J., Hao, Q. Q., Zhang, S. D., and Fan, S. W. (2020). Influence of Oil Well Casing on the Law of Strata Pressure in Working Face. J. Min. Strata Control Eng. 2 (1), 013522. doi:10.13532/j.jmsce.cn10-1638/td.2020.01.003
Yang, C., Zhang, J., Tang, X., Ding, J., Zhao, Q., Dang, W., et al. (2017). Comparative Study on Micro-pore Structure of Marine, Terrestrial, and Transitional Shales in Key Areas, China. Int. J. Coal Geol. 171, 76–92. doi:10.1016/j.coal.2016.12.001
Yang, H. Z., Zhao, S. X., Liu, Y., Wu, W., Xia, Z., Wu, T., et al. (2019). Main Controlling Factors of Enrichment and High-Yield of Deep Shale Gas in the Luzhou Block, Southern Sichuan Basin. Nat. Gas. Ind. 39 (11), 55–63. doi:10.3787/j.issn.1000-0976.2019.11.007
Yu, X., Bian, J. Q., and Liu, C. Y. (2022). Determination of Energy Release Parameters of Hydraulic Fracturing Roof Near Goaf Based on Surrounding Rock Control of Dynamic Pressure Roadway. J. Min. Strata Control Eng. 4 (1), 013016. doi:10.13532/j.jmsce.cn10-1638/td.20210908.001
Zhang, K., Peng, J., Liu, W., Li, B., Xia, Q., Cheng, S., et al. (2020a2020). The Role of Deep Geofluids in the Enrichment of Sedimentary Organic Matter: A Case Study of the Late Ordovician-Early Silurian in the Upper Yangtze Region and Early Cambrian in the Lower Yangtze Region, South China. Geofluids 2020, 1–12. doi:10.1155/2020/8868638
Zhang, K., Jia, C., Song, Y., Jiang, S., Jiang, Z., Wen, M., et al. (2020b). Analysis of Lower Cambrian Shale Gas Composition, Source and Accumulation Pattern in Different Tectonic Backgrounds: A Case Study of Weiyuan Block in the Upper Yangtze Region and Xiuwu Basin in the Lower Yangtze Region. Fuel 263 (2020), 115978. doi:10.1016/j.fuel.2019.115978
Zhang, K., Peng, J., Wang, X., Jiang, Z., Song, Y., Jiang, L., et al. (2020c). Effect of Organic Maturity on Shale Gas Genesis and Pores Development: A Case Study on Marine Shale in the Upper Yangtze Region, South China. Open Geosci. 12 (2020), 1617–1629. doi:10.1515/geo-2020-0216
Zhang, J. Z., Li, X. Q., Zou, X. Y., Xie, Z. Y., Zhang, X. Q., Li, Y. Y., et al. (2021). Pore Structure Characteristics of a Marine-Continental Coal-Bearing Shale Reservoir and its Effect on the Shale Gas-Containing Property. Geochimica 50 (5), 478–491. doi:10.19700/j.0379-1726.2021.05.004
Zhang, K., Song, Y., Jia, C., Jiang, Z., Han, F., Wang, P., et al. (2022a). Formation Mechanism of the Sealing Capacity of the Roof and Floor Strata of Marine Organic-Rich Shale and Shale Itself, and its Influence on the Characteristics of Shale Gas and Organic Matter Pore Development. Mar. Petroleum Geol. 140 (2022), 105647. doi:10.1016/j.marpetgeo.2022.105647
Zhang, K., Jiang, S., Zhao, R., Wang, P., Jia, C., and Song, Y. (2022b). Connectivity of Organic Matter Pores in the Lower Silurian Longmaxi Formation Shale, Sichuan Basin, Southern China: Analyses from Helium Ion Microscope and Focused Ion Beam Scanning Electron Microscope. Geol. J. 2022, 1–13. doi:10.1002/gj.4387
Zhang, K., Song, Y., Jiang, Z., Yuan, X., Wang, X., Han, F., et al. (2022c). Research on the Occurrence State of Methane Molecules in Postmature Marine Shales-A Case Analysis of the Lower Silurian Longmaxi Formation Shales of the Upper Yangtze Region in Southern China. Front. Earth Sci. 10, 864279. doi:10.3389/feart.2022.864279
Zhang, K., Jiang, Z., Song, Y., Jia, C., Yuan, X., Wang, X., et al. (2022d). Quantitative Characterization for Pore Connectivity, Pore Wettability, and Shale Oil Mobility of Terrestrial Shale with Different Lithofacies-A Case Study of the Jurassic Lianggaoshan Formation in the Southeast Sichuan Basin of the Upper Yangtze Region in Southern China. Front. Earth Sci. 10, 864189. doi:10.3389/feart.2022.864189
Zhang, K., Song, Y., Jiang, Z., Xu, D., Li, L., Yuan, X., et al. (2022e). Quantitative Comparison of Genesis and Pore Structure Characteristics of Siliceous Minerals in Marine Shale with Different TOC Contents-A Case Study on the Shale of Lower Silurian Longmaxi Formation in Sichuan Basin, Southern China. Front. Earth Sci. 10, 887160. doi:10.3389/feart.2022.887160
Zhang, Q., Qiu, Z., Zhang, L. F., Wang, Y. M., Xiao, Y. F., Liu, D., et al. (2022). Reservoir Characteristics and its Influence on Transitional Shale: An Example from Permian Shanxi Formation Shale, Daning-Jixian Blocks, Ordos Basin. Nat. Gas. Geosci. 33 (3), 396–407. doi:10.11764/j.issn.1672-1926.2021.07.002
Zhao, Z. J., Zhou, H., Chen, X., Liu, Y. H., Zhang, Y. B., Liu, Y. E., et al. (2012). Sequence Lithofacies Paleography and Favorable Exploration Zones of the Permianin Sichuan Basin and Adjacent Areas, China. Acta Pet. Sin. 33 (S2), 35–51. doi:10.1016/S1002-0160(08)60059-4
Zheng, H. R., and Hu, Z. Q. (2010). Tectonic-lithofacies Palaeogeographic Atlas of Premesozoic China. Beijing: Geological Publishing House.
Zou, C., Dong, D., Wang, S., Li, J., Li, X., Wang, Y., et al. (2010). Geological Characteristics and Resource Potential of Shale Gas in China. Petroleum Explor. Dev. 37 (6), 641–653. doi:10.1016/s1876-3804(11)60001-3
Zou, C. N., Dong, D. Z., Wang, Y. M., Li, X. J., Huang, J. L., Wang, S. F., et al. (2015). Shale Gas in China: Characteristics, Challenges and Prospects (Ⅰ). Petroleum Explor. Dev. 42 (6), 689–701. (in Chinese with English abstract). doi:10.1016/s1876-3804(15)30072-0
Keywords: transitional facies, shale gas, reservoir characteristics, leping formation, southern sichuan basin, resource potential
Citation: Shan C, Ye C, Zhang Z, Zou C, He F, Zhang H, Mei J, Yin K, Shi Y, Li B, Zhang F and Diao Y (2022) Reservoir Characteristics and Resource Potential Analysis of Transitional Shale Gas in Southern Sichuan Basin. Front. Earth Sci. 10:909469. doi: 10.3389/feart.2022.909469
Received: 31 March 2022; Accepted: 25 April 2022;
Published: 09 June 2022.
Edited by:
Shu Jiang, The University of Utah, United StatesReviewed by:
Mengdi Sun, Northeast Petroleum University, ChinaHaihua Zhu, Southwest Petroleum University, China
Wei Yang, Guizhou Institute of Technology, China
Copyright © 2022 Shan, Ye, Zhang, Zou, He, Zhang, Mei, Yin, Shi, Li, Zhang and Diao. This is an open-access article distributed under the terms of the Creative Commons Attribution License (CC BY). The use, distribution or reproduction in other forums is permitted, provided the original author(s) and the copyright owner(s) are credited and that the original publication in this journal is cited, in accordance with accepted academic practice. No use, distribution or reproduction is permitted which does not comply with these terms.
*Correspondence: Congchen Ye, WTA5OTYwOTIyQDE2My5jb20=; Fengqi Zhang, MTU1MjA1NDE3QHFxLmNvbQ==; Yifan Diao, MTkwNjc3OTI2MUBxcS5jb20=