Corrigendum: Late Cryogenian and late Paleozoic ice ages on the São Francisco craton, east Brazil
- CPMTC, Geology Department, Universidade Federal de Minas Gerais, Belo Horizonte, MG, Brazil
The miniature paleocontinent in the region of the São Francisco River valley, in eastern Brazil, holds the record of two different glacial epochs. The late Cryogenian Jequitaí Formation from the Bambuí Group is up to 100 m thick and covers areas mainly in the central São Francisco craton. Evidences for glacial sedimentation are beautifully preserved E-W grooves and striations, dropstones within fine-grained rocks, and a full set of diamictites enclosing a rich and complex depositional history. The Jequitaí Formation is in close link with the tectonic evolution of the São Francisco paleocontinent and the West Gondwana amalgamation. From west, the precocious Paranapanema and São Francisco blocks collision in late Cryogenian flexured the foreland lithosphere and created depozones that were infilled by glacial sediments. Toward east, the rifting and opening of the Adamastor Ocean allowed thick glacial and non-glacial deposits to form through subaqueous gravitational sedimentation. From west to east, proximal and distal glaciomarine, glaciocontinental, and non-glacial resedimentation are identified and linked to the evolving continental masses and climate during the Cryogenian and beginning of Ediacaran. The late Paleozoic Santa Fé Group is the youngest record of glaciation on the São Francisco craton. It is 60–80 m thick and yields consistent and confident glacial evidences such as N-S striations on top of Cambrian sandstones, ice-rafted debris, and rain-out diamictite, all preserved in small and patchy areas in the west-central São Francisco craton. Paleocurrents suggest a northern ice center and sedimentary facies indicate deposition in continental lakes and rivers. Although late Paleozoic, its age is poorly constrained and likely correlated with the uppermost Itararé Group (Taciba Formation) of Paraná Basin in south Brazil. Deglaciation and strong isostatic adjustments make up the termination of the Santa Fé Group sedimentary record and depict a glaciocontinental system evolved on an interior stable continental crust. The late Neoproterozoic Jequitaí Formation and the late Paleozoic Santa Fé Group are parts of the earth’s sedimentary history preserving a rich record of climate, tectonic, and surface processes in part controlled by the evolving continental masses on the São Francisco craton.
Introduction
Earth’s climate has naturally fluctuated through deep time, shifting from arid to humid, hot to cold environments, shaping Earth’s landscapes, influencing (or influenced by) evolutionary changes, and ultimately challenging our understanding of its causes (Kopp et al., 2005; Goldberg et al., 2021). Glacial epochs are spread in the geological record, and the most studied occurrences that provide much data for our knowledge of past glacial processes are Cryogenian (720–635 Myr ago; Hoffman, 2011; Lang et al., 2018), late Paleozoic (ca. 300 Myr ago; Montañez and Poulsen, 2013; Dietrich et al., 2021), and Eocene to recent (from 34 Myr ago; Chiverrell and Thomas, 2010) well-exposed records.
The tectonic setting of continents can exert a strong influence on glacial dynamics and ultimately on the preservation and representativeness of the glacial record and on the basin stratigraphic arrangement (Eyles, 1993; Isbell et al., 2008; Ramstein et al., 2019). The late Neoproterozoic glacial record is well known as being influenced by plate tectonic processes (Eyles, 1993; Eyles and Januszczak, 2004; Arnaud and Etienne, 2011; Li et al., 2013; Le Heron et al., 2017; Kennedy and Eyles, 2019). The rifting of the Rodinia occurred concomitantly with major glaciations and generated many basins presenting glacial and slope-derived material. Moreover, the Rodinia breakup and Gondwana assembly are highly diachronic, featuring compressional and extensional basins alongside cratonic blocks, creating a complex interplay of subsidence mechanisms in sedimentary basins from these ages (Deynoux et al., 2006; Delpomdor et al., 2017; Uhlein et al., 2017).
During the Paleozoic, the Gondwana supercontinent included South America, Africa, India, and Australia. When centered at high latitudes in the southern hemisphere, it served as a base where ice sheets formed and grew towards low latitudes (Bishop et al., 2010; Isbell et al., 2012; Montañez and Poulsen, 2013). From the late Neoproterozoic to the late Paleozoic, the glacial records are contrastingly different and mainly caused by different tectonic and paleogeographic contexts prevailing by the time each basin has evolved. Although having a less obvious influence, independent lines of evidence support tectonic controls on late Paleozoic glaciations, including continental drift over the polar regions (Isbell et al., 2012), opening and closing of oceanic gateways (Saltzman, 2003), uplift and mountain building trapping humid air masses (Dávila et al., 2021), and others. The main difference between tectonic controls on late Neoproterozoic and late Paleozoic ice ages is probably the great effect on the sedimentary record and on the lateral correlation of strata that Neoproterozoic tectonics causes in the Precambrian records. Furthermore, perhaps because the late Paleozoic basins evolved in a period of Earth’s history with a more similar atmosphere, hydrosphere, and biosphere conditions to the present day, their sedimentary records are very similar to modern glacial records (Le Heron et al., 2019; Dietrich et al., 2021).
In this way, for at least twice, the São Francisco craton in east Brazil was the scene of glaciers and ice sheets which imprinted in the rock record a window of opportunity to investigate, elucidate and compare glacial processes and dynamics of two different ages. The Jequitaí Formation of the Bambuí Group is probably late Cryogenian and likely correlated to the global Marinoan glaciation (650–635 Ma). It formed in vast areas of the São Francisco paleocontinent during the interplay of the Rodinia breakup and the Gondwana amalgamation in West Gondwana. The younger, late Paleozoic Santa Fé Group preserves a complete set of facies and bedforms of a continental ice origin and formed probably as a discrete glaciation during the drifting of the Gondwana supercontinent.
In this contribution, we explore both glaciogenic units, presenting a review of their sedimentologic, stratigraphic and geochronologic aspects in light of recent scientific advances and introduce novel data that contribute to the discussion of tectonic control in the sedimentation of late Neoproterozoic and late Paleozoic ice ages.
Geologic settings
The São Francisco craton (SFC) in east central Brazil hosts a rock record that spans from the Paleoarchean to the Cenozoic, with distinct sedimentary successions that witness Earth processes of global significance (Figure 1). The São Francisco craton and its surrounding Neoproterozoic orogenic systems were shaped during the amalgamation of the Gondwana supercontinent, as various plates converged and collided during the Ediacaran and Cambrian (Brito-Neves et al., 1999; Alkmim et al., 2001). The basement of the SFC comprises rock units older than 1.8 Ga. Archean tonalite-trondhjemite-granodiorite gneisses (TTG), granitoids, greenstones belts, Paleoproterozoic plutons, and supracrustal successions are the main lithological assemblages of the basement exposed (Heilbron et al., 2017 and references therein).
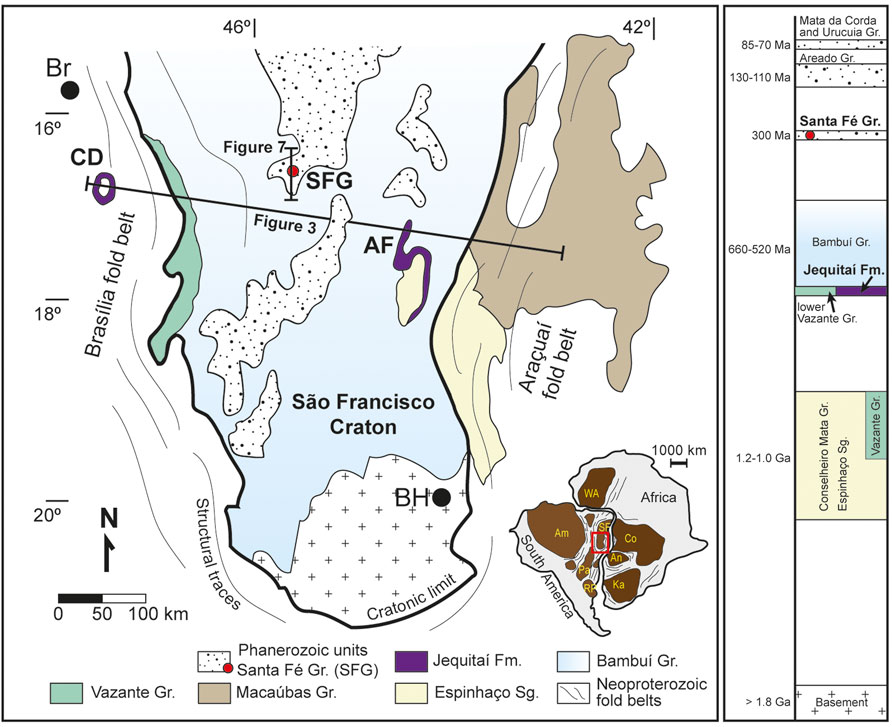
FIGURE 1. São Francisco craton in east-central Brazil is bordered by the Neoproterozoic Brasília and Araçuaí fold belts and covered by Proterozoic to Phanerozoic sedimentary units. At right, some of the sedimentary units and their approximate ages. The inset shows South America and Africa continents and their cratonic blocks in brown color. CD, Cristalina dome; AF, Água Fria ridge; Br, Brasília city; BH, Belo Horizonte city; Am, Amazonian craton; SF, São Francisco craton; Pa, Paranapanema craton; RP, Rio de la Plata craton; WA, West African craton; Co, Congo craton; An, Angola craton; and Ka, Kalahari craton.
Different basins that evolved separately are now preserved on the SFC. Some of them are relicts of the rifts/passive margins of the São Francisco paleocontinent (prior to the Gondwana amalgamation), and others developed during or much after the continent’s final collision in the Cambrian. The São Francisco paleocontinent, as the precursor of the São Francisco craton, had areas much bigger than the craton limits we know today. This is because its margins were significantly reworked during the Brasiliano/Pan-African orogenic cycle and now make part of the fold and thrust belts that surround the SFC (Alkmim et al., 2001). On the São Francisco craton, many sedimentary successions may be found: 1) The siliciclastic and carbonatic Meso to Neoproterozoic Conselheiro Mata Group (Espinhaço Supergroup), Paranoá Group and Vazante Group (Dardenne, 2000; Campos et al., 2013; Santos et al., 2013). 2) The Bambuí Group, which records one of the Cryogenian global glaciations in its base (Jequitaí Formation) and the transition from the Proterozoic to the Phanerozoic world (Uhlein et al., 2011; Uhlein et al., 2019). 3) The late Paleozoic glacial rocks of the Santa Fé Group (Campos and Dardenne, 1994; Campos and Dardenne, 1997). 4) The Cretaceous Areado, Mata da Corda and Urucuia groups, an expression of the South Atlantic Ocean opening in the interior of Brazil (Campos and Dardenne, 1997; Sgarbi et al., 2001). 5) Cenozoic sedimentary covers.
The late Cryogenian marks the beginning of continental collision between the Paranapanema block and the western margin of the São Francisco paleocontinent (ca. 650 Ma; Piauilino et al., 2021; Pimentel et al., 2011). The orogenic loading of the Brasília fold belt formed between the two continental blocks flexure the São Francisco crust at a point to create depozones of a foreland basin (Reis et al., 2017; Uhlein et al., 2017). On the SFC, Cryogenian to Ediacaran westward sediments from the Bambuí Group are interpreted as deposited in deeper settings and close to the orogenic front, while eastward sediments deposited on an uplifted basement often preserve a series of kilometer-long troughs (Reis and Suss, 2016; Uhlein et al., 2017). To the east, the margin of the São Francisco paleocontinent was rifted and facing the Adamastor Ocean during the Cryogenian (Pedrosa-Soares et al., 1998; Pedrosa-Soares et al., 2001). Sediments deposited along the eastern margin of the SFC record a history of basin opening, closure of a scissor-shaped oceanic basin, and formation of an orogenic system at 570–550 Ma (the Araçuaí fold belt) comprising an inverted continental rift (Caxito et al., 2022). The Macaúbas Group is the main representative of this stage and is composed of sandstone, conglomerate, diamictite, and deep-sea pelitic units deposited from rift to passive margin settings later deformed and metamorphosed within the Araçuaí fold belt. The Serra do Catuni Formation and its distal correlative, the Chapada Acauã Formation, from the Macaúbas Group, is known to record expressive diamictite-bearing and turbidite successions related to Cryogenian glaciations and rift subsidence (Uhlein et al., 1999; Pedrosa-Soares et al., 2011). The westward Brasília fold belt is thus tens of million years older than the eastward Araçuaí fold belt, demonstrating the diachronic continental collisions around the miniature São Francisco paleocontinent that generated a complex geotectonic context during the Cryogenian and Ediacaran periods.
After final continent collisions and amalgamation of the Western Gondwana in the late Cambrian (Brito-Neves et al., 1999), the São Francisco paleocontinent remained stable as a craton, drifting with the Gondwana supercontinent and making part of the Pangea during late Paleozoic at about 300–280 Ma.
Materials and methods
The study utilizes surface exposures of the Jequitaí Formation in the vicinities of the Água Fria ridge and of the Santa Fé Group near Santa Fé de Minas town, both in the Minas Gerais state, east Brazil. Field observations were made in a multitude of localities. Stratigraphic sections were measured during field seasons in the last years. The lithotypes, sedimentary structures, bed thicknesses, and components were documented in detail in the field and later arranged in a systematic way of sedimentary facies and facies associations. The sedimentologic, stratigraphic and geodynamic interpretations documented herein are based on our field observations and the published literature.
The Cryogenian Jequitaí Formation
Geological context
The Neoproterozoic (1,000–541 Ma) has remarked and unique biochemical characteristics, such as pulses of oxygenation of the atmosphere and oceans, and the increase of fossil record diversity with, for example, unequivocal macroalgal records and the first appearance of animals (Dong et al., 2008; Och and Shields-Zhou, 2012). The rise of atmospheric pO2, profound ecological diversifications, and the setting of plate tectonics mechanism (Hamilton, 2011) are Neoproterozoic innovations that hide intricate cause-and-effect relations and were the foundations for the Earth we know today. Beyond that, the Cryogenian Period (720–635 Ma) record the most extreme climate episodes resulting in near-global glaciations (Kirschvink, 1992; Hoffman et al., 1998; Hoffman et al., 2017). The two major Neoproterozoic glaciations, with occurrences in many continents, have come to be known as “Sturtian” (∼720–660 Ma) and “Marinoan” (∼650–635 Ma) glaciations (Condon et al., 2005; Macdonald et al., 2010; Hoffman et al., 2017; Cox et al., 2018). In east Brazil, the glaciogenic Jequitaí Formation is the base of the Bambuí Group (Dardenne, 1978; Dardenne, 2000; Uhlein et al., 2016) and although still controversial, its age is generally assigned to the Marinoan glaciation event (Caxito et al., 2012; Caxito et al., 2021; Alvarenga et al., 2014; Uhlein et al., 2016; Uhlein et al., 2017; Uhlein et al., 2019). The Jequitaí Formation is found in several regions of the São Francisco craton and one area is particularly relevant as it presents outstanding records that served as the basis for the geologic knowledge of the unit: the outskirts of the Água Fria ridge in central Minas Gerais state.
Sedimentology of the Jequitaí Formation
At the Serra da Água Fria ridge (Figure 1), the Jequitaí Formation reaches a thickness of up to 100 m and is predominantly composed of massive diamictites, sheet-like to lenticular bodies, with high variation in clast/matrix ratio (Fa2 facies from Table 1). Clasts float in a texturally and compositionally immature sand-silt matrix with quartz, clay minerals, calcite, and sericite. Some diamictite clasts are scratched, polished, and facetted. Diamictites may be clast-poor or clast-rich and composed of granule- to boulder-sized, angular to rounded clasts of sandstone, carbonate, granite, gneiss, vein quartz, and volcanic rocks (Figures 2A,B; Cukrov et al., 2005; Uhlein et al., 2011). Clast lithotypes reflect varied sedimentary sources. The stratified diamictites also contain discontinuous sandstone and few shale intercalations. The sandstone beds are scarce and are generally massive or graded, fine- to coarse-grained, sheet-like to lenticular bodies, and poorly sorted (Fa3 facies; Figure 2C). Mud-silt rhythmite or structureless mudstone is locally intercalated with diamictite (Fa5). Ice-rafted debris in shales and rhythmites are absent in the Água Fria ridge, although they are present in areas approximately 100 km south-southeast (Fa6; Figure 2D).
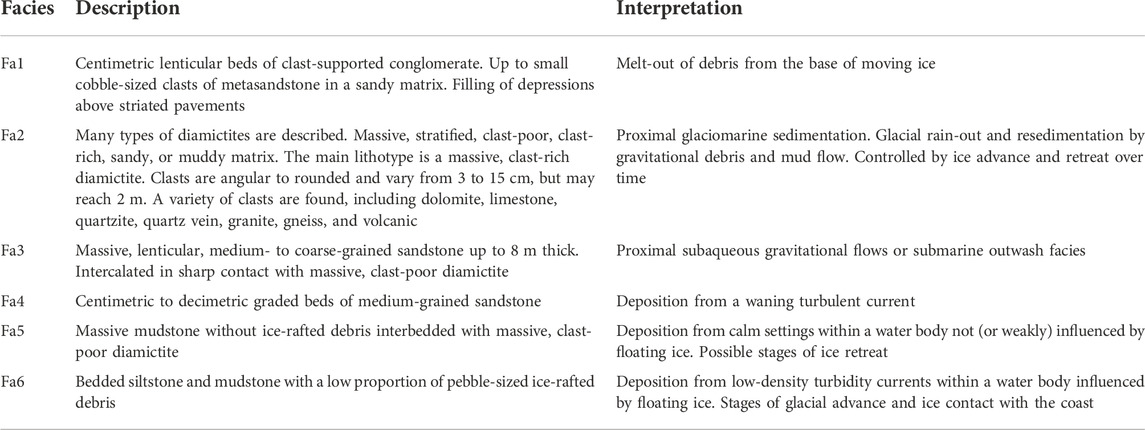
TABLE 1. Sedimentary facies of the Jequitaí Formation in the Água Fria ridge, modified from Cukrov et al. (2005).
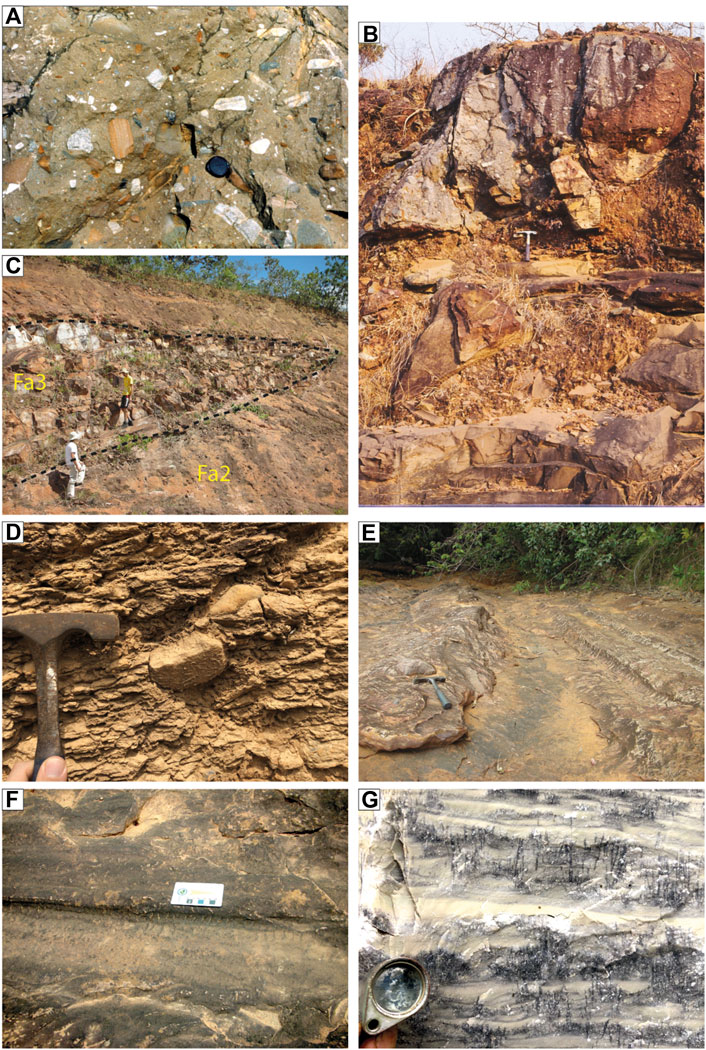
FIGURE 2. Facies characteristic and general view of the Jequitaí Formation in the Água Fria ridge. (A) Massive, clast-rich diamictite with fine matrix from facies Fa2. Note the variety of clast types and angulosity. (B) Crudely stratified clast-rich (top) and clast-poor (bottom) diamictite contact (near the hammer). (C) Massive, lenticular, medium-grained sandstone (Fa3) intercalated with clast-poor diamictite and interpreted as possible submarine outwash facies. (D) Detail of ice-rafted debris as pebble-sized clast in weathered laminated mudstone from facies Fa6. (E) Gouges with lateral ridges and internal crescent-shaped fractures at the right and fine diamictite layer covering the pavement at the left (below the hammer). Paleodirection to ENE. (F) Detail of gouge and side ridges with internal fractures. (G) Aragonite pseudomorph fans in the cap carbonate of the lower Sete Lagoas Formation.
The most outstanding feature of the Jequitaí Formation in the Água Fria ridge and the main evidence used to propose a glacial interpretation for the unit (Isotta et al., 1969) are striated pavements preserved on sandstone. East-west trending striation varies from fine, V-shaped thin scratches to U-shaped grooves up to 20 cm wide and 5 cm deep, bearing crescent-shaped cracks that consistently indicate a roughly eastward ice flow direction (Figures 2E,F). Individual grooves reach up to 18 m in length with no widening or shallowing at the ends. Isotta et al. (1969) firstly interpreted the striations as formed over an indurated quartzite pavement represented by the late Mesoproterozoic Conselheiro Mata Group (upper Espinhaço Supergroup). However, Rocha-Campos et al. (1996) reinterpreted the glacial abrasion marks as developed over a soft-sediment substratum, in the fluctuating grounding zone of a marine ice sheet. The soft-sediment interpretation is based on several pieces of evidence, such as striae inside grooves laterally covered by slumped plow ridges, clasts inside furrows partially embedded in the quartzite, and the occurrence of striated surfaces intercalated with non-scratched beds (Rocha-Campos et al., 1996). These are similar to the evidence of soft-sediment deformation in late Paleozoic records (Assine et al., 2018; Le Heron et al., 2019). A final solution to these questions requires additional and detailed work.
Continental to marine ice sheets have been proposed for the Jequitaí Formation in the Água Fria ridge (Isotta et al., 1969; Karfunkel and Hoppe 1988; Rocha-Campos et al., 1996; Uhlein et al., 1999). The scarcity of outwash deposits, the monotonous, massive, clast-poor diamictites, and the interpretation of glacial abrasion marks on unconsolidated marine sediments support the interpretation of proximal glaciomarine sedimentation in the Água Fria ridge (Uhlein et al., 1999; Uhlein et al., 2011).
Toward the west, in the Cristalina dome (Figure 1), eastern margin of the Brasília fold belt, and westward of the São Francisco craton, the Jequitaí Formation is a basal pink cap dolostone with negativebit thicker (up to 250 m) and mainly different from the Água Fria ridge because of recurrent stratified diamictites and dropstones within shales. From facies association, it is interpreted as the presence of sea ice floating in a proximal to distal glaciomarine environment (Cukrov et al., 2005; Figure 3). The Cristalina dome area was likely formed in a shelf environment deeper than the Água Fria ridge area.
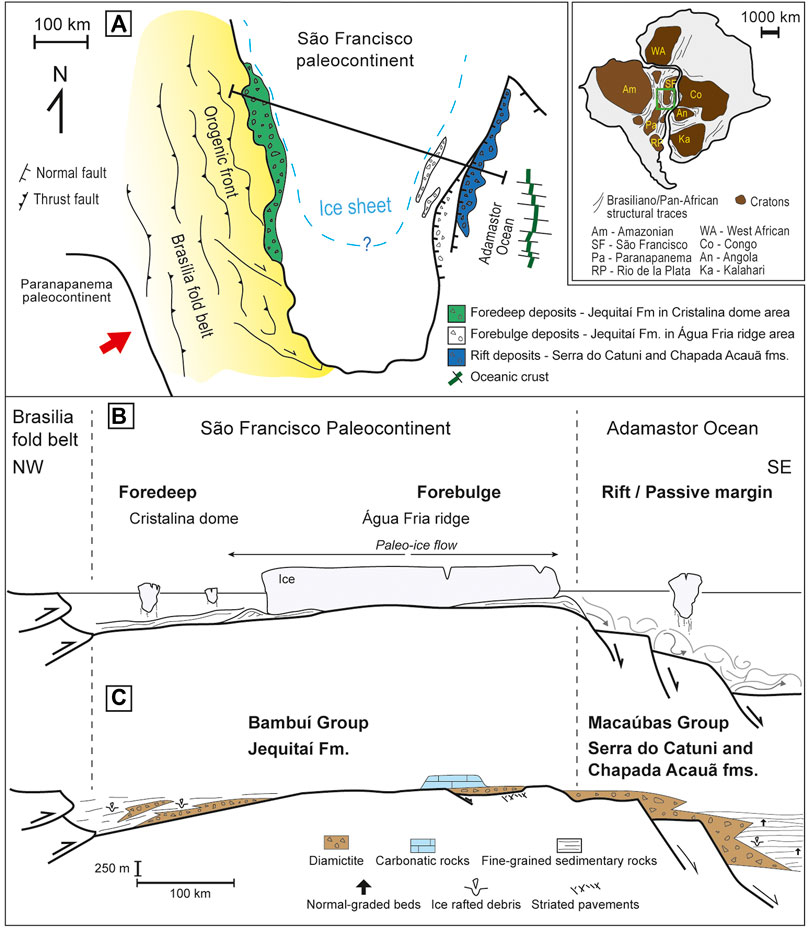
FIGURE 3. (A) Paleogeographic map of the São Francisco paleocontinent and its west and east margins affected by contrasting tectonic regimes during the Cryogenian and Ediacaran. Glacial and tectonic processes (B) and products (C) formed from the interplay of glacial sedimentation and the dynamic mechanisms of continental lithosphere. See text for facies descriptions and regional stratigraphic detailing.
Sedimentary provenance and age constraints
U-Pb detrital zircon geochronology data of the Jequitaí Formation are scarce, but suggest that source areas with ages at ca. 1.0, 1.4, 1.8, 2.0, 2.1, and 2.7 Ga were the main providers of sediments to the glacial diamictites in the Água Fria ridge (Rodrigues, 2008). From these, 3 grains yield Tonian ages from 860 to 960 Ma, probably from early Neoproterozoic gabbro dyke swarms in the east and northeastern cratonic basement (Moreira et al., 2020). These are the youngest grains ever found in the Jequitaí Formation and make up its maximum depositional age. The diamictites contain granules to large boulders of sandstone, limestone, dolostone, shale, granite, gneiss, and quartz vein. Carbonate clasts are more common towards the base, whereas crystalline basement clasts dominate towards the top (Karfunkel and Hoppe 1988). Crystalline rocks (granite, gneiss) were probably derived from the underlying cratonic basement. The Mesoproterozoic Espinhaço Supergroup and Paranoá Group—with occurrences in the SFC and the marginal fold belts—were probably important in providing sediments to the glacial system of the Jequitaí Formation, including limestone and dolostone clasts. Few beds of carbonate rocks are still present in the upper Espinhaço Supergroup and Paranoá Group (Santos et al., 2013; Martins-Ferreira et al., 2018).
In the field, the Jequitaí Formation is usually overlaid by shales of the Serra de Santa Helena Formation, an interglacial or post-glacial unit from the mixed siliciclastic-carbonatic interval of the Bambuí Group (Uhlein et al., 2019). Outcropping locally (Martins-Ferreira et al., 2013) and mainly in boreholes, carbonatic rocks from the Sete Lagoas Formation overlap Jequitaí glaciogenic rocks. The lower Sete Lagoas Formation is one of the basal Bambuí Group units and comprises a post-glacial, early Ediacaran deposit characterized by pink dolostones and limestones that bear characteristics of a post-Marinoan cap carbonate interval, such as 1) basal pink cap dolostone with negative δ13C and δ18O values that decrease up section; 2) an overlying transgressive carbonate interval with aragonite pseudomorphs (Figure 2G) and locally barite fans. In these layers, negative δ13C gradually increases upwards to around 0‰ (Caxito et al., 2012; Alvarenga et al., 2014; Okubo et al., 2018). Crockford et al. (2017) identified a strong negative Δ17O anomaly down to −1.05‰ in the barite layers. Although later disputed by Okubo et al. (2020) the primary nature of the Sete Lagoas barite, Crockford et al. (2017) suggested a global character for the geochemical Δ17O signal and used to cross-correlate Marinoan post-glacial cap carbonates around the world. More recently, from in situ U-Pb dating of calcites, Caxito et al. (2021) obtained well-fitted ages of 615.4 ± 5.9, 608.1 ± 5.1, and 607.2 ± 6.2 Ma in the aragonite pseudomorphs interval. Considering the characteristics of the Sete Lagoas Formation cap carbonate, its minimum depositional ages from in situ calcite U-Pb geochronology, and its contact relation with the Jequitaí Formation, the latter likely record the supposed late Cryogenian global Marinoan glaciation (650–635 Ma) or a younger, early Ediacaran glaciation with a termination age before ca. 610 Ma. If a younger age is assigned to the Jequitaí Formation, it could be one other piece of evidence for asynchronous late Neoproterozoic glaciations (Spence et al., 2016; Bai et al., 2020; Le Heron et al., 2020).
Geodynamic interpretations
The Jequitaí Formation bears interesting relationships with the Cryogenian rift sequences of the Araçuaí fold belt that suggest genetically linked evolutions. The Serra do Catuni Formation from the Macaúbas Group is part of the Araçuaí fold belt (Figures 1, 3). It is a kilometer-thick unit mainly composed of diamictite. Its correlation with the Jequitaí Formation is based on the distribution of facies, the thicknesses changes, and the main orientation of glacial abrasion features below the Jequitaí Formation indicating paleo-ice flow direction toward ENE; i.e., from the São Francisco paleocontinent to its eastern rifted border (Karfunkel and Hoppe, 1988; Uhlein et al., 1998; Uhlein et al., 1999). Based on the different characteristics of the diamictites and associated sediments, two distinct depositional environments can be recognized: 1) a continental to proximal glaciomarine environment on the São Francisco craton, with striated pavements and up to 100 m thick of main diamictites piled up (Jequitaí Formation); 2) a thick (up to 1.000 m), distal subaqueous gravitational sedimentation with debris and mud flow (diamictites) and turbidites (sandstone, rhythmite) represented by the Serra do Catuni Formation and also the Chapada Acauã Formation (both from the Macaúbas Group) in the Araçuaí fold belt. Although gravity flow deposits constitute a greater part of the succession, some IRD-bearing intervals are sharply distinct in the Serra do Catuni Formation. The interbedding of these deposits testifies the subaqueous distal sedimentation nature of the Serra do Catuni Formation and the basinal Chapada Acauã Formation. Thus, the Jequitaí and Serra do Catuni/Chapada Acauã formations are lateral equivalents with a proximal-distal relationship that were influenced by the breakup of continental blocks during the Cryogenian (Uhlein et al., 1999, 2011; Pedrosa-Soares et al., 2001, 2011; Castro et al., 2020).
In the Água Fria ridge, Martins-Neto and Hercos (2002) described wide grooves with inside steps with palaeodirection toward 285°, contributing to the amassed paleocurrent dataset. ENE and WNW paleo-ice flow directions thus suggest a more complex ice dispersion pattern. It is possible that the Água Fria ridge deposits and other occurrences nearby may represent a glacial center from where cratonic ice bodies dispersed toward the lowlands on both the west and east margins of the São Francisco paleocontinent during the Cryogenian. Recently, Marques et al. (2021) also correlated diamictites and shales from the lower Vazante Group (Figure 1) with the Jequitaí Formation, thus suggesting another resedimented glaciomarine Cryogenian sedimentation where today stands the transition of the Brasilia fold belt and the western São Francisco craton.
The corroboration of available data considering the timing of the initiation of the Brasiliano/Pan-African orogenic cycle suggests that the onset of the formation of the Brasilia fold belt (ca. 650 Ma; Piauilino et al., 2021; Pimentel et al., 2011), and associated flexural subsidence in the adjacent foredeep, may be chronocorrelated to the deposition of the Jequitaí Formation. The foreland basin model (Figure 3) fits well and can explain a number of key features describing the stratigraphy, the paleogeography, and the patterns of glacial dynamics during Jequitaí time: 1) the distribution of glacial and resedimented facies associations on the shelf, cratonic and deep environments; 2) the uplift of the metasedimentary cratonic basement in the Água Fria ridge and surrounding, which may be attributed at least in part to the rise of the São Francisco flexural forebulge (Souza-Filho, 1995; Reis et al., 2017); and 3) the reconstructed patterns of glacial flow, from cratonic areas toward the eastern and western adjacent lowland regions. Other subsidence mechanisms may have also operated during the Jequitaí time enhancing accommodation and contributing to the observed features that relate to the distribution of glacial facies, paleogeography, and ice dynamics.
To the east, the Macaúbas Group evolved from a deep rift system triggered by the opening of the Adamastor ocean, which is up to now constrained between 720 and 640 Ma (Pedrosa-Soares et al., 2011; Amaral et al., 2020). The eastern margin of the São Francisco palaeocontinent was rifted, steep, and deep, giving rise to successions of mostly marine strata deposited along rifted, extensional plate margin (Figure 3). Toward the west, the margin was probably gently tilted as a consequence of the transition from forebulge to foredeep depozones, which gave a few more accommodation spaces and conditions for stratified diamictites and rhythmite with dropstones controlled by glacial ice-sheet growth and decay cycles to form (Figure 3).
The Jequitaí Formation in the Cristalina dome is thinner than typical foredeep deposits (Catuneanu, 2004) and probably is the record of precocious foreland subsidence during the initial stage of continent collision. This is in agreement with the absence of younger U-Pb detrital zircon in the Jequitaí diamictite. In well-developed foreland basins, sampling of syn-collisional magmatism and magmatic arcs associated with ocean closure supply zircon grains close to the depositional age of the foreland sediment (Cawood et al., 2012). In the Cristalina dome, the Jequitaí rocks are tectonically overlaid by a thrust nappe of the Canastra Group (Cukrov et al., 2005), corroborating the development during a premature foreland setting that was later partially obliterated by the tectonic advance of the orogenic front of the Brasília fold belt. The existence of only older zircon grains is also a consequence of the interpreted ice dispersal pattern, from the paleocontinent hinterland toward its margins, thus eroding vast areas of the cratonic basement.
The Jequitaí Formation can, thus, be considered a record of a regional glaciation developed on the São Francisco craton related to highlands generated by Cryogenian uplift, either by marginal rift basins or by forebulge uplift. A glacial center or centers was developed and the marginal lowland areas were filled by glaciomarine (westward) and subaqueous gravitational sedimentation-debris flows and turbidites (eastward). An intense reworking and erosion of the glacial deposits were caused either by ice retreats or by periods of tectonic instability.
The late Paleozoic Santa Fé Group
Geological context
By the Carboniferous period, about 358 to 298 Myr ago, glaciers have once more dominated a significant portion of the Earth, reaching latitudes as low as 30° on the Gondwana supercontinent (Montañez and Poulsen, 2013; Dietrich et al., 2021). The Late Paleozoic Ice Age (LPIA) was one of the Earth’s most important Phanerozoic climatic events, probably starting modestly in the latest Devonian but reaching its apex by the middle and late Carboniferous (Gulbranson et al., 2010; Wicander et al., 2011; Cagliari et al., 2016). In Brazil, the LPIA record is abundant and mainly entrusted to the Itararé Group of Paraná Basin in south Brazil (Eyles et al., 1993; Vesely and Assine, 2006; Mottin et al., 2018; Griffis et al., 2019; Griffis et al., 2021). However, in east Brazil, on the São Francisco craton, there is one more LPIA record still poorly known: the Santa Fé Group (Figure 4). Glacial deposits such as glaciolacustrine and glaciofluvial facies were recognized as preserved inside U-shaped paleo-valleys carved on Cambrian sandstone of the Bambuí Group (Dardenne et al., 1990; Campos, 1992; Campos and Dardenne, 1994; Campos and Dardenne, 2002; Sgarbi et al., 2001).
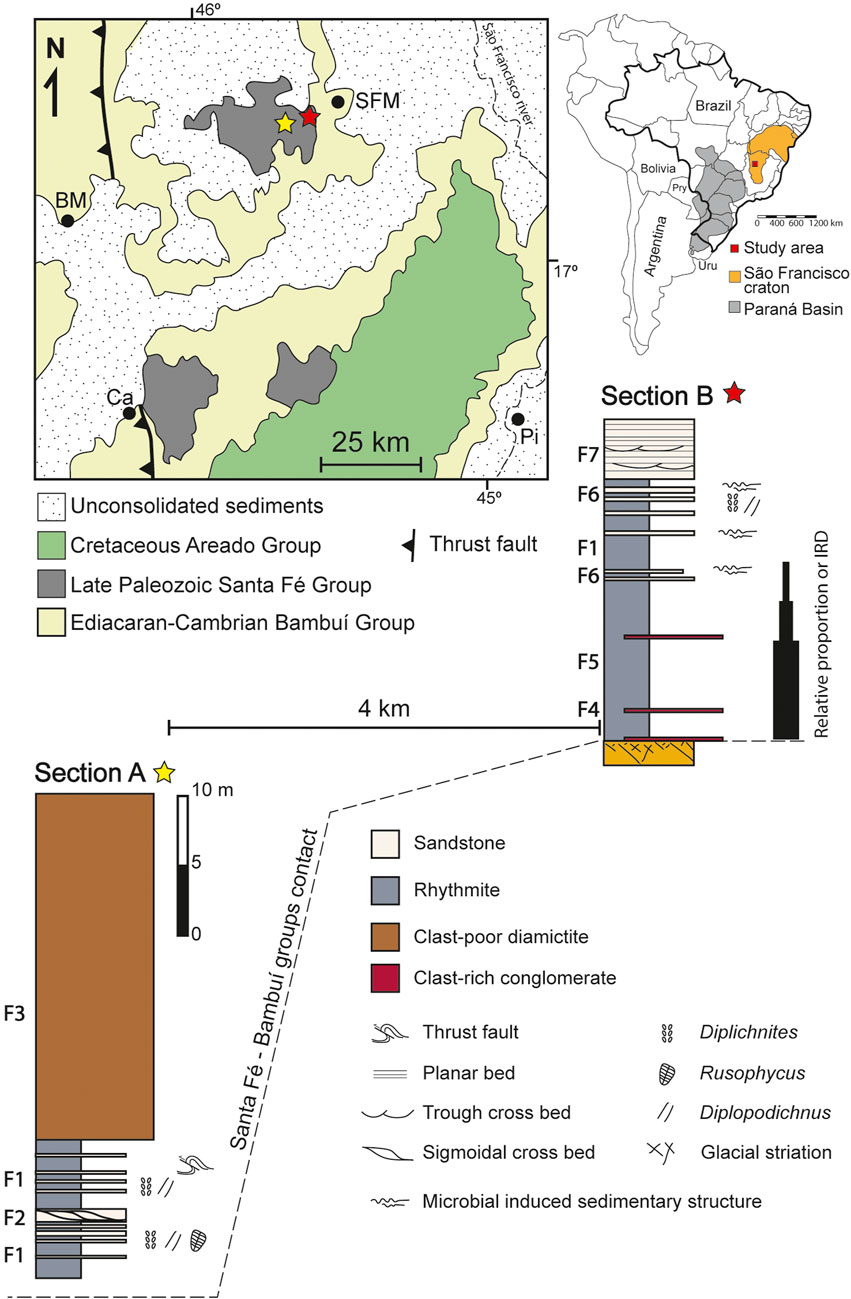
FIGURE 4. Composite section of the Santa Fé Group. Vertical displacement of sections is based on altitude. Sections A and B are located on the geological map by the yellow and red stars, respectively. See Table 2 and text for facies descriptions and interpretations. IRD = ice-rafted debris. Cities abbreviations: BM, Brasilândia de Minas; Ca, Canabrava; Pi, Pirapora; SFM, Santa Fé de Minas.
Sedimentology of the Santa Fé Group
Section A (Figure 4) initiates with mud-silt rhythmites and thin sandy intercalations (F1 from Table 2) without any ice-rafted debris (IRD). Sandstone beds locally become thicker and present trough and sigmoidal cross laminae and beds in between rhythmites (F2). Trace fossils such as Diplichnites, Diplopodichnus, and Rusophycus are abundant in almost every sandy intercalation, although the variety of ichnotaxa is low (Figure 5E). Beds of rhythmites are locally folded and thrusted toward 310–350° and may be up to 3 m-thick (Figure 5D). Up above in Section A, the mud-silt rhythmites and sandy intercalations are supplanted by circa 25 m of massive clast-poor diamictite (F3). Outsized, faceted, and exotic clasts are usual, while striated clasts are less common. No sedimentary structures such as crude stratification or graded beds are identified (Figures 6A–C).
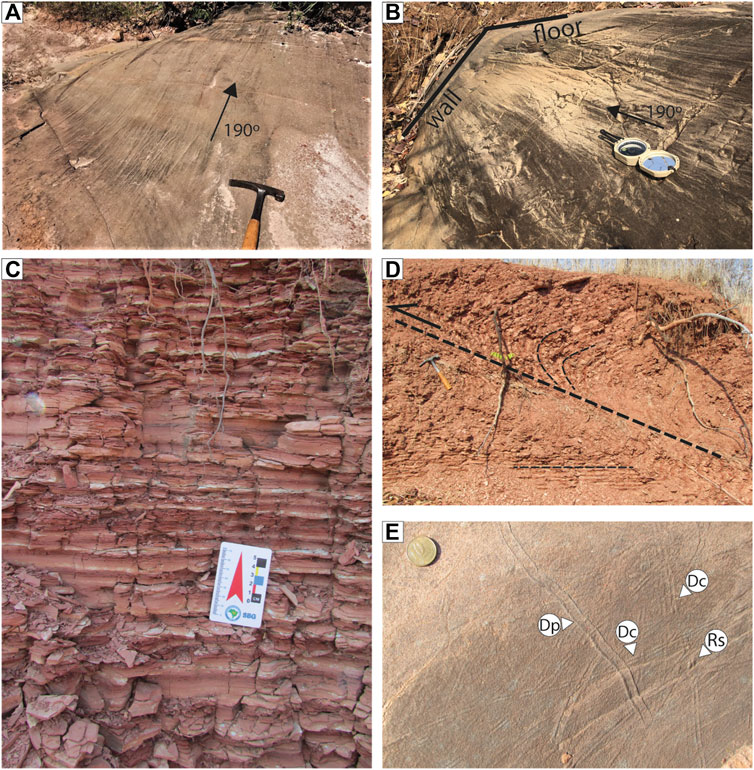
FIGURE 5. (A) Thin glacial striae on top of Cambrian sandstone. (B) Striations perpendicular to the dip of the wall rock and on the floor. (C) Prodelta rhythmites without IRD. (D) Weathered and thrusted rhythmite without ice-rafted debris. (E) Diplichnites (Dc), Diplopodichnus (Dp), and Rusophycus (Rs) trace fossils with varied preservational features in thin sandstone intercalations of the deltaic interval.
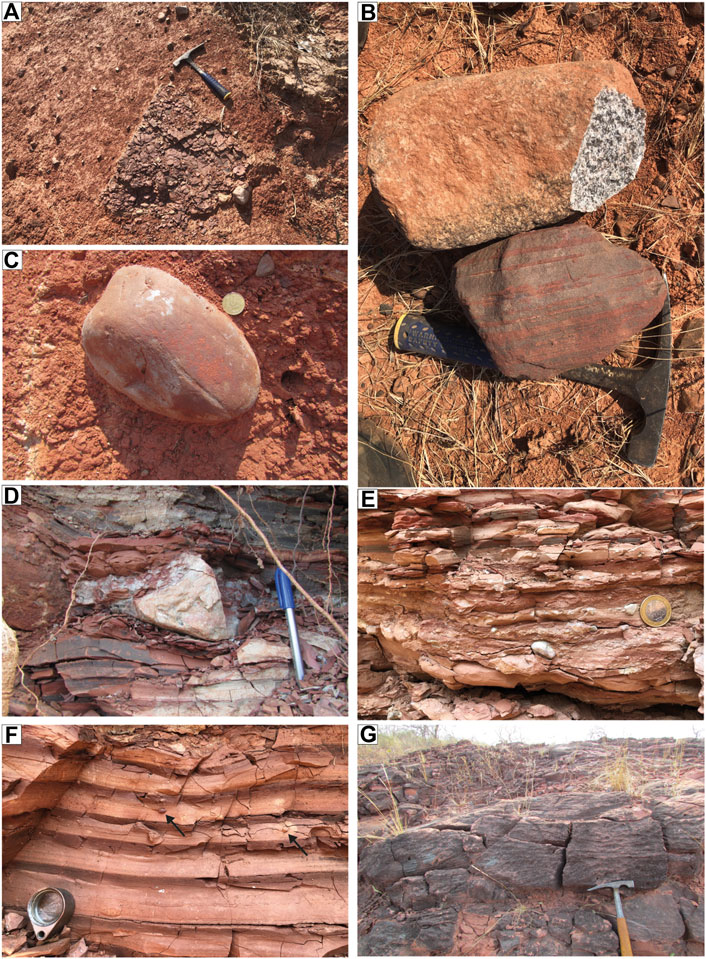
FIGURE 6. (A) Faceted sandstone boulder immersed in a clast-poor and mud-rich diamictite. (B) Cobble- and boulder-sized clasts of granite and banded iron formation from clast-poor diamictite. (C) Striated clast from diamictite of facies F3. (D) Dropstone deforming beds of rhythmites. (E), (F) Rhythmites of facies F5 with more (E) or less (F) ice-rafted debris. Arrows in (F) indicate small dropstones. It is to be noted the gradation from the silty to muddy interval and the sharp contact with the next couplet. (G) Trough cross beds in the upper sandstone of facies F7.
Section B is 4 km east from section A (Figure 4) and initiates with striated pavements carved on partially silicified sandstones from the uppermost Bambuí Group (Figures 5A,B). Millimeter-wide simple striations and crescentic fractures develop on horizontal and vertical erosional surfaces and indicate the main SSW (200–190°) ice flow movement. A few short striaes point toward 220–230° and are cut by the SSW ones. The Santa Fé glacial erosion pavements are up to now known from two different sites and both yield sedimentary thicknesses of no more than 25 m of sedimentary pile between the striated pavement below and the recent erosional surface above. Moreover, the basement of the two sites is circa 40 m above other contacts between the Bambuí and Santa Fé groups, suggesting that the contact between the basement and the glaciogenic is highly uneven, undulated and that the preserved glacial pavements are from basement highs. Above the striated pavements, just over 10 m of rhythmites with dropstones are found covering the basement sandstone. These are well-cyclic silt/sand-mud couplets intercalations with more or less IRD (Facies F5, Figures 6D–F). Couplets are 2–3 cm thick and the clasts are mainly found in silty/sandy beds. Occasionally, it may have a number of coarse-grained fragments that call it a conglomerate is appropriate. Up in the section, IRD disappears and beds of sandstones with microbial-induced sedimentary structures and trace fossils start to intercalate with rhythmites (F6). It culminates with up to 5 m of medium to coarse-grained sandstone (F7, Figure 6G).
Facies F1 and F2 from the base of Section A (Figure 4; Table 2) are interpreted as deltaic facies from a non-ice contact lake margin (Figure 5C) and are probably the sedimentological expression of the lake before the initiation of the main glacial period. Two mechanisms may be interpreted as causing the fold and thrust deformation found in the basal rhythmites: 1) soft-sediment deformation from slumps in the prodelta setting during gravitational collapse; 2) fold and thrust of glaciotectonic origin when a glacier advance reaches an area in which a suitable decollement layer, (e.g., clay) comes nearer to the surface. Both interpretations are likely and are not mutually contrary, as glacial advance may push marginal sediments triggering gravity-driven slumps (Wright and Anderson, 1982). Nevertheless, considering the difference between the shear direction and the ice flow direction and the absence of IRD in facies F1 and F2, it is more likely a pure gravitational collapse interpretation.
The high proportion of mud matrix summed to the local intense chemical weathering on the diamictites (F3) may have obliterated delicate structures that could exist. The clasts’ nature and at least 2 km of lateral continuity suggest that these were rain-out diamict formed from a high ratio of fines from meltwater plume and IRD during a maximum glacial advance stage. Cobble to boulder-sized clasts of banded iron formations are surprisingly common within diamictite (Figure 6B). Due to the rarity of such lithotype in basement rocks and the interpreted SSW ice movement, the probable source are iron deposits located 500 km to NE, in the Bahia state (Borges et al., 2015).
Facies F5 above striated pavements from section B (Figure 4; Table 2) may be interpreted as ancient varves, in which seasonal variation in meltwater and sediment input produces a coarse sediment layer deposited during spring and summer when significant supraglacial melting occurs, and fine-grained sediment during winter, when melting is suppressed, allowing the fines to settle. However, the demonstration of seasonal control on ancient glacial sediments is tough and more data is needed. Another possibility is that F5 rocks are rhythmically laminated sediments accumulated in the glaciolacustrine setting as a result of deposition by discrete-event turbidity currents (turbidites) with no evident seasonal control; in this case, they should not be classified as varvites. Progressive deglaciation during intense glacial retreat may have formed rhythmites influenced by different rates of meltwater supply from streams (Figure 7). The upward decrease of IRD flux in these rhythmites may roughly reflect the volume of icebergs and can be used to infer depletion in iceberg production over time (Zolitschka et al., 2015). The increase in sandstone beds between rhythmites culminating with sandstone from facies F7 in the upper section B is interpreted as a regressive tendency from glaciolacustrine to glaciofluvial sedimentation fed by meltwater within a periglacial setting (Figure 7).
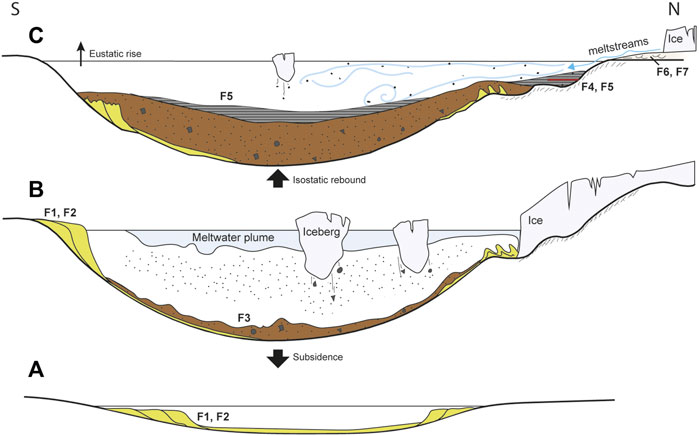
FIGURE 7. Stages of glaciolacustrine sedimentation of the Santa Fé Group. (A) Pre-glacial stage with shallow waters and deltaic margins; (B) ice advance, deepening of the lake due to ice sheet subsidence, and deposition of rain out diamict. Deltaic sedimentation may continue to form in glaciolacustrine margins; and (C) ice retreat and intensification of melt streams, reworking, and periglacial settings. Rhythmites with more or less ice-rafted debris may be seasonally controlled (varvites) or low-density turbidites.
Age constraints and glacial dynamics
The depositional age of the Santa Fé Group is poorly constrained based on trace fossils and interpreted as late Paleozoic by Campos and Dardenne (1994). Paleomagnetic data on diamictites also indicated a Carboniferous to Permian age and yielded a paleolatitude of 44°S (Brandt and Ernesto, 2006). In the Paraná Basin in south Brazil, the Taciba Formation (uppermost Itararé Group) is interpreted as a proximal glaciomarine unit yielding similar SSW sediment transport (Mottin et al., 2018), which is in contrast with the lower and middle Itararé Group and with the general view of S and SE glacial centers (Rosa et al., 2016). The Taciba Formation is early Permian (Asselian-Sakmarian age) based on palynomorphs (Souza and Marques-Toigo, 2003; Mottin et al., 2018) and invertebrates (Taboada et al., 2016), and represents the final deglacial sequence of the LPIA in the Paraná Basin (Mottin and Vesely, 2021). It records successive stages of ice retreat and advance culminating in base-level fall and the development of paleosol on diamictites below the post-glacial, coal-bearing Rio Bonito Formation (Mottin et al., 2018). If a correlation between the Santa Fé Group and the uppermost Itararé Group is correct, then the first should record a similar early Permian deglacial evolution.
During deglaciation, large quantities of meltwater are released into the oceans causing glacio-eustatic sea-level rise (Lambeck et al., 2012). The progressive ice retreat also imply in crustal unloading and rapid uplift of the lithosphere, provoking glacio-isostatic adjustments and relative sea-level fall (Boulton, 1990). Thus, the net sea-level change is a resultant of the glacio-eustatic sea-level rise and glacio-isostatic adjustments during deglacial evolution of a glaciated ocean margin or lake (Dietrich et al., 2017; Dietrich et al., 2019). In coastal areas, close to the maximal extent of the former ice-sheet margins, the deglaciation initiates with relative sea-level fall caused by high rates of crustal uplift and glacio-isostatic adjustments. Later, decreasing rates of crustal uplift are suppressed by the large input of meltwater, causing moderate glacio-eustatic sea-level rise. In such areas, hypothetically sedimentary successions would yield glaciomarine muds below delta front deposits overlaid by transgressive, post-glacial sand and muds (Hein et al., 2014). In continental areas formerly covered by ice, the glacio-isostatic adjustments remain the dominant process controlling regional surface water-level throughout the deglacial evolution (Dietrich et al., 2017), and a continuous regressive stacking pattern from glacial muds to delta plain and fluvial deposits is observed.
The upper Santa Fé Group (Section B from Figure 4) shows a regressive pattern. Rhythmites with decreasing content of IRD (F5) give place to rhythmites without IRD (F1) intercalated by thin beds of sandstone (F6) that are overlaid by fluvial sandstones (F7). The gradual absence of ice involvement on sedimentation summed to the regressive tendency are interpreted as a deglacial evolution mainly controlled by glacio-isostatic adjustments, as expected for a glacioterrestrial depositional system evolved on a stable continental crust (Nutz et al., 2015) (Figure 7). The SSW paleo-ice flow direction preserved in the Santa Fé Group and in the Taciba Formation is unique in the context of late Paleozoic glaciations in Brazil. Along with the similar deglacial evolution, the Santa Fé Group from east-central Brazil may be tentatively correlated with the Taciba Formation from the uppermost Itararé Group in south Brazil. Further north, other late Paleozoic glacial basins are found (Viviani et al., 2000), although no confident paleo-ice flow directions were up to now measured and published. Nevertheless, late Paleozoic glaciations may have occurred in lower latitudes in Brazil and the geographic extension may be wider than previously thought. At the very beginning of the Permian, during the last sighs of the LPIA, ice-spreading centers may have been relocated to N and NE in Western Gondwana.
Conclusion
On the São Francisco craton in east Brazil, the late Cryogenian and late Paleozoic glacial epochs are recorded in the sedimentary rocks of the Jequitaí Formation and Santa Fé Group, respectively. However, although having a common glacial nature, both records are remarkably different. These contrasts are mainly caused by different tectonic and paleogeographic contexts prevailing by the time each basin has evolved.
The glaciogenic Jequitaí Formation from the Bambuí Group has a probable late Cryogenian age and developed in consonance with large scale continental reconfiguration. While glacial conditions were achieved on the São Francisco paleocontinent, its surrounding areas were rifted or thrusted in a diachronic process of Western Gondwana assembly. The formation of the Brasília fold belt in the west triggered W-E flexural subsidence and foredeep to forebulge depozones on the São Francisco paleocontinent that were the locus of deposition of the Jequitaí Formation in the Cristalina dome area (west) and in the Água Fria ridge (east). By the same time, on the opposite margin, rifting of the lithosphere and opening of the Adamastor ocean created large accommodation spaces and subaqueous sediment gravity flows that formed the Serra do Catuni and Chapada Acauã formations from the Macaúbas Group. The latters are mixed glacial and non-glacial sequences recording the taphrogenic phase of the Araçuaí fold belt. Thrusting and mountain building in the west and rifting in the east shaped the glacial environments, sedimentary processes, and facies. The Jequitaí Formation can, thus, be considered a record of a regional glaciation developed on the São Francisco paleocontinent and related to high- and lowlands generated by either eastern marginal rift basins (Macaubas basin - resedimentation through mass flows and turbidites) and by western forebulge-foredeep mechanisms toward the Brasília fold belt, with glaciomarine sedimentation. The Jequitaí Formation record late Cryogenian glacial dynamics influenced by the evolving continental masses from the former Rodinia to the latter Gondwana supercontinents.
After the Gondwana had assembled and drifted to higher latitudes, glacial conditions were once more achieved on the São Francisco craton by the late Paleozoic. The Santa Fé Group is tentatively correlated with the Taciba Formation from the uppermost Itararé Group of Paraná Basin. From paleo-ice flow directions, an ice center may have existed toward NNE in Brazil during the earliest Permian, and moving bodies of ice spread toward SSW, forming the Santa Fé Group in three stages: 1) deltaic margins free of ice influence; 2) ice advance and deposition of rain out diamictite and rhythmites with ice-rafted debris in glaciolacustrine environments; 3) ice retreat, melt streams, reworking, and periglacial settings. The Santa Fé Group terminates with a phase of deglaciation controlled by isostatic adjustments from the glacial retreat and lithospheric unloading.
The Cryogenian Jequitaí Formation and the late Paleozoic Santa Fé Group are parts of the Earth’s glaciation history preserving a rich record of climate, tectonic, surface processes, and landscape evolution of the São Francisco craton in ancient times. Differences in its sedimentary and stratigraphic patterns are in part related to the tectonic evolution of continental crust during the late Neoproterozoic and late Paleozoic.
Data availability statement
The original contributions presented in the study are included in the article/Supplementary Material; further inquiries can be directed to the corresponding author.
Author contributions
GU and AU contributed to the implementation of the research, analysis of the results, and writing of the manuscript.
Funding
CNPq (432556/2018-4) and FAPEMIG (APQ-02240-21).
Acknowledgments
The authors thank P. Dietrich, D. Le Heron and G. Cornamusini for reviewing the manuscript and F. Vesely for editorial handling. This work was made possible with the institutional support of the Federal University of Minas Gerais and its Dean of Research (PRPq), the Geology Department, and the Centro de Pesquisa Prof. Manoel Teixeira da Costa (CPMTC). AU is a fellow of CNPq.
Conflict of interest
The authors declare that the research was conducted in the absence of any commercial or financial relationships that could be construed as a potential conflict of interest.
Publisher’s note
All claims expressed in this article are solely those of the authors and do not necessarily represent those of their affiliated organizations, or those of the publisher, the editors, and the reviewers. Any product that may be evaluated in this article, or claim that may be made by its manufacturer, is not guaranteed or endorsed by the publisher.
References
Alkmim, F. F., Marshak, S., and Fonseca, M. A. (2001). Assembling West Gondwana in the neoproterozoic: Clues from the São Francisco craton region, Brazil. Geol. 29 (4), 319–322. doi:10.1130/0091-7613(2001)029<0319:awgitn>2.0.co;2
Alvarenga, C. J. S., Santos, R. V., Vieira, L. C., Lima, B. A. F., and Mancini, L. H. (2014). Meso-Neoproterozoic isotope stratigraphy on carbonates platforms in the Brasília Belt of Brazil. Precambrian Res. 251, 164–180. doi:10.1016/j.precamres.2014.06.011
Amaral, L., Caxito, F. A., Pedrosa-Soares, A. C., Queiroga, G., Babinski, M., Trindade, R., et al. (2020). The ribeirão da folha ophiolite-bearing accretionary wedge (Araçuaí orogen, SE Brazil): New data for cryogenian plagiogranite and metasedimentary rocks. Precambrian Res. 336, 105522. doi:10.1016/j.precamres.2019.105522
Arnaud, E., and Etienne, J. L. (2011). “Recognition of glacial influence in Neoproterozoic sedimentary successions,”. The geological record of neoproterozoic glaciations. Editors E. Arnaud, G. P. Halverson, and G. Shields-Zhou (London: Geological Society of London, Memoir), 36, 39–50.
Assine, M. L., Santa Ana, H., Veroslavsky, G., and Vesely, F. F. (2018). Exhumed subglacial landscape in Uruguay: Erosional landforms, depositional environments, and paleo-ice flow in the context of the late Paleozoic Gondwanan glaciation. Sediment. Geol. 369, 1–12. doi:10.1016/j.sedgeo.2018.03.011
Bai, H., Kuang, H., Liu, Y., Peng, N., Chen, X., and Wang, Y. (2020). Marinoan-aged red beds at shennongjia, south China: Evidence against global-scale glaciation during the cryogenian. Palaeogeogr. Palaeoclimatol. Palaeoecol. 559, 109967. doi:10.1016/j.palaeo.2020.109967
Bishop, J. W., Montañez, I. P., and Osleger, D. A. (2010). Dynamic carboniferous climate change, arrow canyon, Nevada. Geosphere 6, 1–34. doi:10.1130/ges00192.1
Borges, J. O., Cruz, S. C. P., Barbosa, J. S. F., and Santos, E. S. (2015). Structural framework of rocks of the Lagoa D'anta mine area, iron-manganese Urandi-Caetité-Licínio de Almeida District, Bahia, Brasil. Braz. J. Geol. 45 (2), 173–192. doi:10.1590/23174889201500020002
Boulton, G. S. (1990). “Sedimentary and sea level changes during glacial cycles and their control on glacimarine facies architecture,”. Glacimarine environments: Processes and sediments. Editors J. A. Dowdeswell, and J. D. Scourse (London: Geological Society, London, Special Publications), 53, 15–52.
Brandt, D., and Ernesto, M. (2006). Resultados paleomagnéticos preliminares do grupo Santa Fé (paleozoico da bacia sanfranciscana): Implicações sobre a idade e paleolatitude da sedimentação glacial. Rev. Bras. Geof. 24, 199–207. doi:10.1590/s0102-261x2006000200004
Brito-Neves, B. B., Campos-Neto, M. C., and Fuck, R. A. (1999). From Rodinia to western Gondwana: An approach to the Brasiliano-Pan african cycle and orogenic collage. Episodes 22, 155–166. doi:10.18814/epiiugs/1999/v22i3/002
Cagliari, J., Philipp, R. P., Buso, V. V., Netto, R. G., Hillebrand, P. K., Lopes, R. C., et al. (2016). Age constraints of the glaciation in the Paraná Basin: Evidence from new U–Pb dates. J. Geol. Soc. Lond. 173, 871–874. doi:10.1144/jgs2015-161
Campos, J. E. G. (1992). A glaciação permo-carbonífera nas regiões de Canabrava e Santa Fé de Minas. Dissertação de Mestrado. Brasília: Instituto de Geociencias, Univ de Brasília, 104.
Campos, J. E. G., and Dardenne, M. A. (1994). A glaciação neopaleozóica na porção meridional da Bacia Sanfranciscana. Rev. Bras. Geociências 23, 65–76. doi:10.25249/0375-7536.19946576
Campos, J. E. G., and Dardenne, M. A. (1997). Estratigrafia e Sedimentação da Bacia Sanfranciscana: uma revisão. Rev. Bras. Geociências 27, 269–282. doi:10.25249/0375-7536.1997269282
Campos, J. E. G., Dardenne, M. A., Freitas-Silva, F. H., and Martins-Ferreira, M. A. C. (2013). Geologia do Grupo Paranoá na porção externa da Faixa Brasília. Braz. J. Geol. 43 (3), 461–476. doi:10.5327/z2317-48892013000300004
Campos, J. E. G., and Dardenne, M. A. (2002). “Pavimentos estriados do Grupo Santa Fé - neopaleozóico da Bacia Sanfranciscana, MG,” in Sítios Geológicos e Paleontológicos do Brasil. Editors C. Schobbenhaus, D. A. Campos, E. T. Queiroz, M. Winge, and M. Berbert-Born (Brasília: DNPM CPRM SIGEP), 161–164.
Castro, M. P., Queiroga, G. N., Martins, M., Pedrosa-Soares, A. C., Dias, L., Lana, C., et al. (2020). Provenance shift through time in superposed basins: From Early Cryogenian glaciomarine to Late Ediacaran orogenic sedimentations (Araçuaí Orogen, SE Brazil). Gondwana Res. 87, 41–66. doi:10.1016/j.gr.2020.05.019
Catuneanu, O. (2004). Retroarc foreland systems––evolution through time. J. Afr. Earth Sci. 38, 225–242. doi:10.1016/j.jafrearsci.2004.01.004
Cawood, P. A., Hawkesworth, C. J., and Dhuime, B. (2012). Detrital zircon record and tectonic setting. Geology 40, 875–878. doi:10.1130/g32945.1
Caxito, F. A., Halverson, G. P., Uhlein, A., Stevenson, R., Gonçalves Dias, T., and Uhlein, G. J. (2012). Marinoan glaciation in east central Brazil. Precambrian Res. 200, 38–58. doi:10.1016/j.precamres.2012.01.005
Caxito, F. A., Lana, C., Frei, R., Uhlein, G. J., Sial, A. N., Dantas, E. L., et al. (2021). Goldilocks at the dawn of complex life: Mountains might have damaged ediacaran–cambrian ecosystems and prompted an early cambrian greenhouse world. Sci. Rep. 11, 20010. doi:10.1038/s41598-021-99526-z
Caxito, F. A., Hartmann, L. A., Heilbron, M., Pedrosa-Soares, A. C., Bruno, H., Basei, M. A. S., et al. (2022). Multi-proxy evidence for subduction of the neoproterozoic Adamastor ocean and wilson cycle tectonics in the south atlantic Brasiliano orogenic system of western Gondwana. Precambrian Res. 376, 106678. doi:10.1016/j.precamres.2022.106678
Chiverrell, R. C., and Thomas, G. S. P. (2010). Extent and timing of the last glacial maximum (LGM) in britain and Ireland: A review. J. Quat. Sci. 25, 535–549. doi:10.1002/jqs.1404
Condon, D., Zhu, M., Bowring, S., Wang, W., Yang, A., and Jin, Y. (2005). U-Pb Ages from the neoproterozoic doushantuo formation, China. Science 308, 95–98. doi:10.1126/science.1107765
Cox, G. M., Isakson, V., Hoffman, P. F., Gernon, T. M., Schmitz, M. D., Shahin, S., et al. (2018). South Australian U-Pb zircon (CAID-TIMS) age supports globally synchronous Sturtian deglaciation. Precambrian Res. 315, 257–263. doi:10.1016/j.precamres.2018.07.007
Crockford, P. W., Hodgskiss, M. S. W., Uhlein, G. J., Caxito, F. A., Hayles, J. A., and Halverson, G. P. (2017). Linking paleocontinents through triple oxygen isotope anomalies. Geology 46 (2), 179–182. doi:10.1130/g39470.1
Cukrov, N., Alvarenga, C. J. S., and Uhlein, A. (2005). Litofácies da glaciação neoproterozoica nas porções sul do cráton do São Francisco: Exemplos de Jequitaí (MG) e Cristalina (GO). Rev. Bras. Geociências 35 (1), 69–76. doi:10.25249/0375-7536.20053516976
Dardenne, M. A., Gonzaga, G. M., and Campos, J. E. G. (1990). Descoberta de pavimentos estriados de origem glacial sobre arcóseos da Formação Tres Marias, na região de Santa Fé de Minas, MG. Rev. Esc. Minas 54 (4), 65–66.
Dardenne, M. A. (1978). Síntese sobre a estratigrafia do Grupo Bambuí no Brasil Central SBG, Congresso Brasileiro de Geologia, 30, Recife. Anais 2, 597–610.
Dardenne, M. A. (2000). “The Brasília fold belt,” in Proceedings of the 31st international geological congress on the tectonic evolution of south America. Editors U. G. Cordani, E. J. Milani, A. Thomaz Filho, and D. A. Campos (Rio de Janeiro, 231–263.
Dávila, F. M., Martina, F., Parra, M., and Ávila, P. (2021). Effects of uplift on Carboniferous exhumation and mountain glaciations in pericratonic areas of SW Gondwana, central Argentina. Tectonics 40 (12), e2021TC006855. doi:10.1029/2021TC006855
Delpomdor, F. R., Tack, L., and Preat, A. R. (2017). Facies and micromorphology of the neoproterozoic upper diamictite formation in the democratic republic of Congo: New evidence of sediment gravity flow. Geol. Belg. 20, 69–79. doi:10.20341/gb.2017.004
Deynoux, M., Affaton, P., Trompette, R., and Villeneuve, M. (2006). Pan-African tectonic evolution and glacial events registered in Neoproterozoic to Cambrian cratonic and foreland basins of West Africa. J. Afr. Earth Sci. 46, 397–426. doi:10.1016/j.jafrearsci.2006.08.005
Dietrich, P., Ghienne, J.-F., Schuster, M., Lajeunesse, P., Nutz, A., Deschamps, R., et al. (2017). From outwash to coastal systems in the portneuf-forestville deltaic complex (québec north shore): Anatomy of a forced regressive deglacial sequence. Sedimentology 64, 1044–1078. doi:10.1111/sed.12340
Dietrich, P., Ghienne, J. F., Lajeunesse, P., Normandeau, A., Deschamps, R., and Razin, P. (2019). Deglacial sequences and glacio-isostatic adjustment: Quaternary compared with Ordovician glaciations. Special Publications, 475. London: Geological Society, 149–179.
Dietrich, P., Griffis, N. P., Le Heron, D. P., Montañez, I. P., Kettler, C., Robin, C., et al. (2021). Fjord network in Namibia: A snapshot into the dynamics of the late paleozoic glaciation. Geology 49 (12), 1521–1526. doi:10.1130/g49067.1
Dong, L., Xiao, S., Shen, B., Yuan, X., Yan, X., and Peng, Y. (2008). Restudy of the worm-like carbonaceous compression fossils Protoarenicola, Pararenicola, and Sinosabellidites from early Neoproterozoic successions in North China. Palaeogeogr. Palaeoclimatol. Palaeoecol. 258 (3), 138–161. doi:10.1016/j.palaeo.2007.05.019
Eyles, C. H., Eyles, N., and Franca, A. B. (1993). Glaciation and tectonics in an active intracratonic basin: The late palaeozoic Itararé group, Paraná Basin, Brazil. Sedimentology 40, 1–25. doi:10.1111/j.1365-3091.1993.tb01087.x
Eyles, N. (1993). Earth's glacial record and its tectonic setting. Earth-Science Rev. 35 (1-2), 1–248. doi:10.1016/0012-8252(93)90002-o
Eyles, N., and Januszczak, N. (2004). “Zipper-rift”: A tectonic model for Neoproterozoic glaciations during the breakup of Rodinia after 750 Ma. Earth-Science Reviews 65, 1–73
Goldberg, S. L., Present, T. M., Finnegan, S., and Bergmann, K. D. (2021). A high-resolution record of early Paleozoic climate. Proc. Natl. Acad. Sci. U. S. A. 118 (6), e2013083118. doi:10.1073/pnas.2013083118
Griffis, N. P., Montañez, I. P., Mundil, R., Richey, R., Isbell, J., Fedorchuk, N., et al. (2019). Coupled stratigraphic and U-Pb zircon age constraints on the late Paleozoic icehouse-to-greenhouse turnover in south-central Gondwana. Geology 47, 1146–1150. doi:10.1130/g46740.1
Griffis, N. P., Montañez, I. P., Mundil, R., Le Heron, D. P., Dietrich, P., Kettler, C., et al. (2021). High-latitude ice and climate control on sediment supply across SW Gondwana during the late Carboniferous and early Permian. GSA Bull. 133, 2113–2124. doi:10.1130/b35852.1
Gulbranson, E. L., Montañez, I. P., Schmitz, M. D., Limarino, C. O., Isbell, J. L., Marenssi, S. A., et al. (2010). High-precision U-Pb calibration of Carboniferous glaciation and climate history, Paganzo Group, NW Argentina. Geol. Soc. Am. Bull. 122, 1480–1498. doi:10.1130/b30025.1
Hamilton, W. B. (2011). Plate tectonics began in Neoproterozoic time, and plumes from deep mantle have never operated. Lithos 123, 1–20. doi:10.1016/j.lithos.2010.12.007
Heilbron, M., Cordani, U. G., and Alkmim, F. F. (2017). “The São Francisco craton and its margins,” in The São Francisco craton, eastern Brazil. Editors M. Heilbron, U. G. Cordani, and F. F. Alkmim (Springer), 3–14.
Hein, C. J., Fitzgerald, D. M., Buynevich, I. V., Van Heteren, S., and Kelley, J. T. (2014). “Evolution of paraglacial coasts in response to changes in fluvial sediment supply,” in Sedimentary coastal zones from high to low latitudes: Similarities and differences. Editors I. P. Martini, and H. R. Wanless (London: Geological Society, London, Special Publications), 388, 247–280.
Hoffman, P. F., Kaufman, A. J., Halverson, G. P., and Schrag, D. P. (1998). A neoproterozoic snowball Earth. Science 281, 1342–1346. doi:10.1126/science.281.5381.1342
Hoffman, P. F., Abbot, D. S., Ashkenazy, Y., Benn, D. I., Brocks, J. J., Cohen, P. A., et al. (2017). Snowball Earth climate dynamics and Cryogenian geology-geobiology. Sci. Adv. 11, e1600983. doi:10.1126/sciadv.1600983
Hoffman, P. F. (2011). Strange bedfellows: Glacial diamictite and cap carbonate from the Marinoan (635 Ma) glaciation in Namibia. Sedimentology 58, 57–119. doi:10.1111/j.1365-3091.2010.01206.x
Isbell, J. L., Cole, D. I., and Catuneanu, O. (2008). “Carboniferous-Permian glaciation in the main Karoo Basin, South Africa: Stratigraphy, depositional controls, and glacial dynamics,”. Resolving the late paleozoic ice age in time and space. Editors C. R. Fielding, T. D. Frank, and J. L. Isbell (Boulder, CO: Geological Society of America Special Paper), 441, 71–82.
Isbell, J. L., Henry, L. C., Gulbranson, E. L., Limarino, C. O., Fraiser, M. L., Koch, Z. J., et al. (2012). Glacial paradoxes during the late paleozoic ice age: Evaluating the equilibrium line altitude as a control on glaciation. Gondwana Res. 22, 1–19. doi:10.1016/j.gr.2011.11.005
Isotta, C. A. L., Rocha-Campos, A. C., and Yoshida, R. (1969). Striated pavement of the upper precambrian glaciation in Brazil. Nature 222, 466–468. doi:10.1038/222466a0
Karfunkel, J., and Hoppe, A. (1988). Late proterozoic glaciation in central-eastern Brazil: Synthesis and model. Palaeogeogr. Palaeoclimatol. Palaeoecol. 65, 1–21. doi:10.1016/0031-0182(88)90108-3
Kennedy, K., and Eyles, N. (2019). Subaqueous debrites of the grand conglomérat formation, democratic republic of Congo: A model for anomalously thick neoproterozoic: ‘‘glacial’’ diamictites. J. Sediment. Res. 89, 935–955. doi:10.2110/jsr.2019.51
Kirschvink, J. L. (1992). “Late proterozoic low-latitude global glaciation: The snowball Earth,” in The proterozoic biosphere: A multidisciplinary study. Editors J. W. Schopf, and C. Klein (Cambridge: Cambridge University Press), 51–52.
Kopp, R. E., Kirschvink, J. L., Hilburn, I. A., and Nash, C. Z. (2005). The paleoproterozoic snowball Earth: A climate disaster triggered by the evolution of oxygenic photosynthesis. Proc. Natl. Acad. Sci. U. S. A. 102 (32), 11131–11136. doi:10.1073/pnas.0504878102
Lambeck, K., Purcell, A., and Dutton, A. (2012). The anatomy of interglacial sea levels: The relationship between sea levels and ice volumes during the last interglacial. Earth Planet. Sci. Lett. 315–316, 4–11. doi:10.1016/j.epsl.2011.08.026
Lang, X., Chen, J., Cui, H., Man, L., Huang, K., Fu, Y., et al. (2018). Cyclic cold climate during the nantuo glaciation: Evidence from the cryogenian nantuo formation in the yangtze block, south China. Precambrian Res. 310, 243–255. doi:10.1016/j.precamres.2018.03.004
Le Heron, D. P., Dietrich, P., Busfield, M. E., Kettler, C., Bermanschlager, S., and Grasemann, B. (2019). Scratching the surface: Footprint of a late Carboniferous ice sheet. Geology 47, 1034–1038. doi:10.1130/g46590.1
Le Heron, D. P., Eyles, N., and Busfield, M. E. (2020). The laurentian neoproterozoic glacial interval: Reappraising the extent and timing of glaciation. Austrian J. Earth Sci. 113, 59–70. doi:10.17738/ajes.2020.0004
Le Heron, D. P., Tofaif, S., Vandyk, T., and Ali, D. O. (2017). A diamictite dichotomy: Glacial conveyor belts and olistostromes in the Neoproterozoic of Death Valley, California, USA Geology 45 (1), 31–34. doi:10.1130/G38460.1
Li, Z-X., Evans, D. A. D., and Halverson, G. P. (2013). Neoproterozoic glaciations in a revised global palaeogeography from the breakup of Rodinia to the assembly of Gondwanaland. Sediment. Geol. 294, 219–232. doi:10.1016/j.sedgeo.2013.05.016
Macdonald, F. A., Schmitz, M. D., Crowley, J. L., Roots, C. F., Jones, D. S., Maloof, A. C., et al. (2010). Calibrating the cryogenian. Science 327, 1241–1243. doi:10.1126/science.1183325
Marques, C. S. S., Uhlein, A., and Uhlein, G. J. (2021). Stratigraphy and U-Pb geochronology of the basal units of the Vazante group: A lateral correlation with the glaciogenic jequitai formation (Minas Gerais, Brazil). J. S. Am. Earth Sci. 108, 103204. doi:10.1016/j.jsames.2021.103204
Martins-Ferreira, M. A. C., Campos, J. E. G., and Alvarenga, C. J. S. (2013). A formação Jequitaí na região de Vila Boa, GO[/title]: [subtitle]exemplo de sedimentação por geleiras terminais no neoproterozóico. Braz. J. Geol. 43 (2), 373–384. doi:10.5327/z2317-48892013000200012
Martins-Ferreira, M. A. C., Campos, J. E. G., and Von Huelsen, M. G. (2018). Tectonic evolution of the Paranoá basin: New evidence from gravimetric and stratigraphic data. Tectonophysics 734-735, 44–58. doi:10.1016/j.tecto.2018.04.004
Martins-Neto, M. A., and Hercos, C. M. (2002). “Sedimentation and tectonic setting of Early Neoproterozoic glacial deposits in southeastern Brazil,”. Precambrian sedimentary environments: A modern approach to ancient depositional systems. Editors W. Altermann, and P. L. Corcoran (New Jersey: International Association of Sedimentologists. Special Publications), 33, 383–403.
Montañez, I. P., and Poulsen, C. J. (2013). The late paleozoic ice age: An evolving paradigm. Annu. Rev. Earth Planet. Sci. 41, 629–656. doi:10.1146/annurev.earth.031208.100118
Moreira, H. F., Danderfer, A., Costa, A. F. O., Bersan, S. M., Lana, C. C., and Queiroga, G. N. (2020). Record of Early Tonian mafic magmatism in the central Espinhaço (Brazil): New insights for break-up of the Neoproterozoic landmass ancestor of São Francisco-Congo paleocontinent. Geosci. Front. 11, 2323–2337. doi:10.1016/j.gsf.2020.02.007
Mottin, T. E., and Vesely, F. F. (2021). Formação Taciba: Última manifestação glacial no Paraná. BPGeo. 78, 65–82. doi:10.5380/geo.v78i0.79352
Mottin, T. E., Vesely, F. F., Rodrigues, M. C. N. L., Kipper, F., and Souza, P. A. (2018). The paths and timing of late Paleozoic ice revisited: New stratigraphic and paleo-ice flow interpretations from a glacial succession in the upper Itararé Group (Paraná Basin, Brazil). Palaeogeogr. Palaeoclimatol. Palaeoecol. 490, 488–504. doi:10.1016/j.palaeo.2017.11.031
Nutz, A., Ghienne, J.-F., Schuster, M., Dietrich, P., Roquin, C., Hay, M. B., et al. (2015). Forced regressive deposits of a deglaciation sequence: Example from the late quaternary succession in the lake saint-jean basin (québec, Canada). Sedimentology 62, 1573–1610. doi:10.1111/sed.12196
Och, L. M., and Shields-Zhou, G. A. (2012). The Neoproterozoic oxygenation event: Environmental perturbations and biogeochemical cycling. Earth-Science Rev. 110, 26–57. doi:10.1016/j.earscirev.2011.09.004
Okubo, J., Muscente, A., Luvizotto, G. L., Uhlein, G. J., and Warren, L. (2018). Phosphogenesis, aragonite fan formation and seafloor environments following the Marinoan glaciation. Precambrian Res. 311, 24–36. doi:10.1016/j.precamres.2018.04.002
Okubo, J., Klyukin, Y. I., Warren, L. V., Sublett, D. M., Bodnar, R. J., Gill, B. C., et al. (2020). Phosphogenesis, aragonite fan formation and seafloor environments following the Marinoan glaciation, 105628.
Pedrosa-Soares, A. C., Vidal, P., Leonardos, O. H., and Brito-Neves, B. B. (1998). Neoproterozoic oceanic remnants in eastern Brazil: Further evidence and refutation of an exclusively ensialic evolution for the Arac ̧uaı ́-West Congo Orogen. Geol. 26, 519–522. doi:10.1130/0091-7613(1998)026<0519:norieb>2.3.co;2
Pedrosa-Soares, A. C., Noce, C. M., Wiedemann, C. M., and Pinto, C. P. (2001). The araã§uaã-west-Congo orogen in Brazil: An overview of a confined orogen formed during gondwanaland assemblyfined orogen formed during gondwanland assembly. Precambrian Res. 110, 307–323. doi:10.1016/s0301-9268(01)00174-7
Pedrosa-Soares, A. C., Babinski, M., Noce, C. M., Maximiliano, M., Queiroga, G., and Vilela, F. (2011). “The neoproterozoic Macaúbas group, Araçuaí orogen, SE Brazil,”. The geological record of neoproterozoic glaciations. Editors E. Arnaud, G. P. Halverson, and G. Shields- Zhou (London: Geological Society, London, Memmoirs), 36, 523–534.
Piauilino, P. F., Hauser, N., and Dantas, E. L. (2021). From passive margin to continental collision: Geochemical and isotopic constraints for E-MORB and OIB-like magmatism during the neoproterozoic evolution of the southeast Brasília Belt. Precambrian Res. 359, 105345. doi:10.1016/j.precamres.2019.105345
Pimentel, M. M., Rodrigues, J. B., Della Giustina, M. E. S., Junges, S., Matteini, M., and Armstrong, R. (2011). The tectonic evolution of the neoproterozoic Brasília belt, central Brazil, based on shrimp and LA-ICPMS U-Pb sedimentary provenance data: A review. J. S. Am. Earth Sci. 31, 345–357. doi:10.1016/j.jsames.2011.02.011
Ramstein, G., Godderis, Y., Donnadieu, Y., Sepulchre, P., Fluteau, F., Zhang, Z., et al. (2019). Some illustrations of large tectonically driven climate changes in Earth history. Tectonics 38, 4454–4464. doi:10.1029/2019tc005569
Reis, H. L. S., Suss, J. F., Fonseca, R. C. S., and Alkmim, F. F. (2017). Ediacaran forebulge grabens of the southern São Francisco basin, SE Brazil: Craton interior dynamics during West Gondwana assembly. Precambrian Res. 302, 150–170. doi:10.1016/j.precamres.2017.09.023
Reis, H. L. S., and Suss, J. F. (2016). Mixed carbonate-siliciclastic sedimentation in forebulge grabens: An example from the ediacaran Bambuí group, São Francisco basin, Brazil. Sediment. Geol. 15, 83–103. doi:10.1016/j.sedgeo.2016.04.004
Rocha-Campos, A. C., Young, G. M., and Santos, P. R. (1996). Re-examination of a striated paviment near Jequitaí, MG: Implications for Proterozoic stratigraphy and glacial geology. An. Acad. Bras. Ciências 68 (4), 593.
Rodrigues, J. B. (2008). Proveniência de sedimentos dos grupos Canastra, Ibiá, Vazante e Bambuí: Um estudo de zircões detríticos e Idades Modelo Sm-Nd. PhD Thesis. Brasília: Univ. Brasília, 141. unpublished.
Rosa, L. E. M., Vesely, F. F., and França, A. B. (2016). A review on late paleozoic ice-related erosional landforms in the Paraná Basin: Origin and paleogeographical implications. Braz. J. Geol. 46, 147–166. doi:10.1590/2317-4889201620160050
Santos, M. N., Chemale, F., Dussin, I. A., Martins, M., Assis, T. A. R., Jelinek, A. R., et al. (2013). Sedimentological and paleoenvironmental constraints of the Statherian and Stenian Espinhaço rift system, Brazil. Sediment. Geol. 290 (15), 47–59. doi:10.1016/j.sedgeo.2013.03.002
Sgarbi, G. N. C., Sgarbi, P. B. A., Campos, J. E. G., Dardenne, M. A., and Penha, U. C. (2001). “Bacia Sanfranciscana: o registro Fanerozóico da Bacia do São Francisco,” in Bacia do São Francisco: Geologia e Recursos Naturais. Editors C. P. Pinto, and M. A. Martins-Neto (Belo Horizonte: SBG, Belo Horizonte), 93–138.
Souza, P. A., and Marques-Toigo, M. (2003). An overview on the palynostratigraphy of the upper paleozoic strata of the Brazilian Paraná Basin. Rev. Mus. Argent. Cienc. Nat. 5, 205–214. doi:10.22179/revmacn.5.49
Souza-Filho, R. G. (1995). O Arcabouço Estrutural da porção externa da Faixa Aracuaí na Serra do Cabral (MG) e o contraste de estilos deformacionais entre os supergrupos Espinhaço e São Francisco. MSc thesis. Ouro Preto: Universidade Federal de Ouro Preto, 150p.
Spence, G., Le Heron, D. P., and Fairchild, I. J. (2016). Sedimentological perspectives on climatic, atmospheric and environmental change in the Neoproterozoic Era. Sedimentology 63, 253–306. doi:10.1111/sed.12261
Taboada, A. C., Neves, J. P., Weinschutz, L. C., Pagani, M. A., and Simões, M. G. (2016). Eurydesma-lyonia fauna (early permian) from the Itararé group, Paraná Basin (Brazil): A paleobiogeographic W-E trans-gondwanan marine connection. Palaeogeogr. Palaeoclimatol. Palaeoecol. 449, 431–454. doi:10.1016/j.palaeo.2016.02.022
Uhlein, A., Trompette, R., and Egydio-Silva, M. (1998). Proterozoic rifting and closure, SE border of the São Francisco Craton, Brazil. J. S. Am. Earth Sci. 11, 191–203. doi:10.1016/s0895-9811(98)00010-8
Uhlein, A., Trompette, R., and Alvarenga, C. (1999). Neoproterozoic glacial and gravitational sedimentation on a continental rifted margin: The jequitaí-macaúbas sequence (Minas Gerais, Brazil). J. S. Am. Earth Sci. 12, 435–451. doi:10.1016/s0895-9811(99)00032-2
Uhlein, A., Alvarenga, C. J. S., Dardenne, M. A., and Trompette, R. R. (2011). “The glaciogenic Jequitaí Formation, southeastern Brazil,” in The geological record of neoproterozoic glaciations. Memoirs 36. Editors E. Arnaud, G. P. Halverson, and G. Shields- Zhou (London: Geological Society), 541–546.
Uhlein, G. J., Uhlein, A., Halverson, G. P., Stevenson, R., Caxito, F. A., Cox, G. M., et al. (2016). The carrancas formation, Bambuí group: A record of pre-marinoan sedimentation on the southern São Francisco craton, Brazil. J. S. Am. Earth Sci. 71, 1–16. doi:10.1016/j.jsames.2016.06.009
Uhlein, G. J., Uhlein, A., Stevenson, R., Halverson, G. P., Caxito, F. A., and Cox, G. M. (2017). Early to late Ediacaran conglomeratic wedges from a complete foreland basin cycle in the southwest São Francisco Craton, Bambuí Group, Brazil. Precambrian Res. 299, 101–116. doi:10.1016/j.precamres.2017.07.020
Uhlein, G. J., Uhlein, A., Pereira, E., Caxito, F. A., Okubo, J., Warren, L. V., et al. (2019). Ediacaran paleoenvironmental changes recorded in the mixed carbonate siliciclastic Bambuí Basin, Brazil. Palaeogeogr. Palaeoclimatol. Palaeoecol. 517, 39–51. doi:10.1016/j.palaeo.2018.12.022
Vesely, F. F., and Assine, M. L. (2006). Deglaciation sequences in the permo-carboniferous Itararé group, Paraná Basin, southern Brazil. J. S. Am. Earth Sci. 22, 156–168. doi:10.1016/j.jsames.2006.09.006
Viviani, J. B., Roch-Campos, A. C., and Ernesto, M. (2000). Late paleozoic glacial sedimentation in northeastern Brazil: New results. An. Acad. Bras. Cienc. 72, 598. doi:10.1590/s0001-37652000000400012
Wicander, R., Clayton, G., Marshall, J. E. A., Troth, I., and Racey, A. (2011). Was the latest devonian glaciation a multiple event? New palynological evidence from Bolivia. Palaeogeogr. Palaeoclimatol. Palaeoecol. 305, 75–83. doi:10.1016/j.palaeo.2011.02.016
Wright, R., and Anderson, J. B. (1982). The importance of sediment gravity flow to sediment transport and sorting in a glacial marine environment: Eastern Weddell Sea, Antarctica. Geol. Soc. Am. Bull. 93 (10), 951–963. doi:10.1130/0016-7606(1982)93<951:tiosgf>2.0.co;2
Keywords: glaciomarine, glaciolacustrine, Gondwana supercontinent, LPIA, late Neoproterozoic
Citation: Uhlein GJ and Uhlein A (2022) Late Cryogenian and late Paleozoic ice ages on the São Francisco craton, east Brazil. Front. Earth Sci. 10:900101. doi: 10.3389/feart.2022.900101
Received: 20 March 2022; Accepted: 03 October 2022;
Published: 24 October 2022.
Edited by:
Fernando Vesely, Federal University of Paraná, BrazilReviewed by:
Dan Le Heron, University of Vienna, AustriaGianluca Cornamusini, University of Siena, Italy
Copyright © 2022 Uhlein and Uhlein. This is an open-access article distributed under the terms of the Creative Commons Attribution License (CC BY). The use, distribution or reproduction in other forums is permitted, provided the original author(s) and the copyright owner(s) are credited and that the original publication in this journal is cited, in accordance with accepted academic practice. No use, distribution or reproduction is permitted which does not comply with these terms.
*Correspondence: Gabriel J. Uhlein, guhlein@ufmg.br