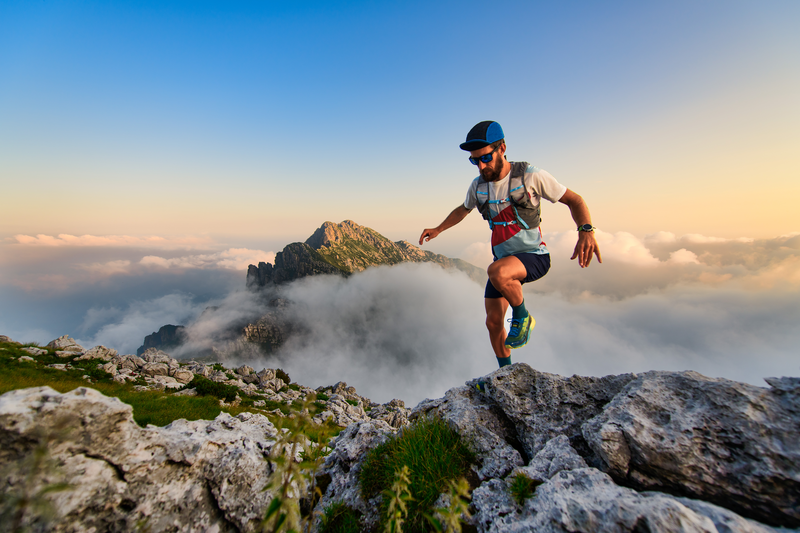
95% of researchers rate our articles as excellent or good
Learn more about the work of our research integrity team to safeguard the quality of each article we publish.
Find out more
ORIGINAL RESEARCH article
Front. Earth Sci. , 26 April 2022
Sec. Structural Geology and Tectonics
Volume 10 - 2022 | https://doi.org/10.3389/feart.2022.898414
This article is part of the Research Topic Fluid and Heat Transfer in Unconventional Reservoirs View all 15 articles
Previous studies have shown that on the surface, Jiaobei uplift and Weihai uplift have higher heat flow values than Jiaolai depression. However, the mechanism by which the deep heat flow migrates and the exposure of geothermal resources are still unclear. In this study, the geothermal field distribution, thermal conductivity, temperature logging data, and chemical composition of the geothermal fluid in the Jiaodong Peninsula were analyzed. Regarding the thermal field’s characteristics and their controlling factors, a conceptual model of heat flow diversion-accumulation between the uplift and basin areas in the Jiaodong Peninsula is proposed. The lithology of the uplift area is mainly composed of intrusive rocks and metamorphic rocks with high thermal conductivities. The lithology of the basin mainly consists of sandstone with a low thermal conductivity. The bottom of the basin, which has a low thermal conductivity and low permeability, serves as a heat insulation and water insulation roof, which causes the heat flow and the heat-carrying fluids and gases from the deep crust to be refracted and redistributed at the bottom of the basin area. As a result, the bottom of the uplift areas has a higher heat flow. In particular, the axial position in the uplift area has the highest heat flow. In addition, the geothermal resources in the Jiaodong Peninsula are mainly distributed in the V-shaped area where the upper block of the NE- and NW-trending faults intersect, and the scope of the exposure of the geothermal resources is very limited.
Geothermal resources can be considered to be renewable on the time scales of technological systems and do not require geological time scales to regenerate, as fossil fuel reserves do (coal, oil, and gas) (Rybach et al., 1999; Rybach et al., 2000). Compared with traditional fossil fuel energy, geothermal resources have obvious advantages, and various countries have accelerated the exploration and utilization of geothermal resources (Sanyal, 2004; Kang, 2013). Analyzing the thermal structure and heat flow transfer mechanism of the Earth can provide a better understanding of the formation mechanism of geothermal resources and help guide us in the exploration of geothermal resources. Low-medium temperature convective geothermal systems are widely distributed in China, especially in the eastern coastal area affected by plate collisions. This area has no additional heat source, such as intrusions, and these resources are mainly distributed in fracture zones with a normal or slightly higher thermal background. Although the Jiaodong Peninsula is rich in geothermal resources, in the actual geothermal exploration process, the exploration failure rate is high. As early as the 1880s, Chinese scholars began systematically studying the geothermal heat flow and the occurrence mechanism of geothermal resources using numerical simulations to simulate the geothermal temperature field, and they proposed the refraction and redistribution of terrestrial heat flow (Xiong and Gao, 1982; Zhang et al., 1982; Xiong and Zhang, 1984). Xie and Yu (1988) summarized the temperature field characteristics of the Sichuan Basin using temperature data from 460 wells in the Sichuan Basin, and they found that the temperature in the uplift region at the interface between the marine stratum and the continental stratum is higher, while the temperature in thee depressions is lower, and thus, the structure determines the location of the geothermal resources. This is because the uplift and basin in the same tectonic zone have different structural forms. This difference causes the heat flow to redistribute in each layer Chen et al. (1990) explored the relationship between the geothermal and geological structures and the tectonic-thermal evolution using the temperature and terrestrial heat flow data for the North China Basin. Hu and Wang (1994) conducted a comprehensive study of the terrestrial heat flow characteristics of many orogenic belts using 125 reliable datasets from south-eastern China. They found that the heat flow distribution was significantly affected by the thermal conductivity structure of the shallow crust. They investigated the effects of the thermal properties of the rocks and the fracture development on the current temperature field.
Based on previous research results, in this study, the regional geology and structure, geothermal reservoir, wellbore temperature data and temperature field, different thermal properties of the rocks, and fluid chemistry of the geothermal systems in the Jiaodong Peninsula were analyzed. In this study, the occurrence mechanism of the geothermal resources and the characteristics of and relationships between the temperature fields in the Jiaobei uplift, the Jiaolai basin, and the Weihai uplift were systematically investigated. A V-shaped structural control and thermal conductivity model, a heat flow model, and a conceptual model suitable for the Jiaodong Peninsula were developed. These results can be used to guide geothermal exploration in the Jiaodong Peninsula, thereby increasing the success rate of geothermal resource exploration.
The Jiaodong Peninsula is located on the eastern margin of the North China Craton, in the Pacific Ocean tectonic area, and it is part of the Pacific Rim tectonic-magmatic belt (Figure 1) (Chen, 2009; Qiu et al., 2014). In addition to the wide distribution of geothermal resources, the gold resources in the Jiaodong Peninsula are extensive. The most important common feature of the geothermal resources and gold resources is that they are both controlled by the regional faults. The widely distributed regional faults not only provide the tectonic space and migration channels for the gold deposits (Lv et al., 2015), but they also provide a channel for the deep migration and upward-flow of geothermal liquids (Jin et al., 1999). Previous studies on the mineralization mechanism of the gold resources investigated the basic geology and structure of the Jiaodong Peninsula in detail, providing detailed geological data for the study of the heat flow related to the geothermal resources in the Jiaodong Peninsula.
FIGURE 1. Location of the Jiaodong Peninsula (modified from Chen et al., 2013).
The study area has many medium-intensity intrusive rocks related to the oceanic subduction from the Jurassic to the Early Cretaceous (Liu et al., 2018). The dikes are very well developed. The intrusive rocks were formed in different periods, with an average density of 10 intrusions per km2. There was no obvious mixing of crustal material during their ascent. They originated from the lithospheric mantle rather than the asthenosphere mantle (Tan et al., 2006).
The crystalline basement of the platform in this area was formed in the Archean-Proterozoic. The sedimentary caprock of the platform was formed in the Paleozoic. Before this period, the Jiaodong Peninsula was a stable continental block, and the terrestrial heat flow was low. The destruction of the North China Craton (NCC) and the lithospheric thinning had not yet occurred (Lin et al., 2013; He, 2015; Hu et al., 2021). The Late Mesozoic destruction of the eastern NCC and the accompanying magmatism were controlled by prolonged thermomechanical-chemical erosion due to low-angle subduction, steepening, and rollback of the Paleo-Pacific Oceanic lithosphere (Liang et al., 2020). The Mesozoic and Cainozoic era were important stages of intense crustal activity in the Jiaodong area. In particular, during the Yanshanian, the upper mantle was strongly active, and this activity was accompanied by crustal movement, forming a series of NE-SW and NNE-SSW trending compressive-torsional faults and NW-SE trending tensional faults. These faults are considered to be secondary faults in the regional Tanlu fault zone (Mao et al., 2005). For example, the regional Muping-Jimo fault zone is an important fault that controls the boundary of the Jiaolai Basin, and its extension in the Cretaceous controlled the formation and development of the Jiaolai Basin. The Taocun Fault is inferred to be a deep-cutting fault that cuts through the Moho based on seismic detection (Pan et al., 2015). The tectonic activity and intrusive rocks determine the distribution of the tectonic units in the Jiaodong Peninsula, and they clearly divide the Jiaodong Block into three structural units: the Jiaobei uplift, Weihai uplift, and Jiaolai depression. The geology, structure, thermal properties of the rocks, and crustal structure of the three different structural units indirectly determine the geothermal background in the Jiaodong Peninsula.
It can be seen from Figure 2 that the lithologies of the Jiaobei uplift and Weihai uplift mainly consist of Archean and Proterozoic metamorphic rocks and Mesozoic intrusive rocks. The lithology of the Jiaolai Basin is mainly Cretaceous sandstone, and the thickness of the sediments in some areas is greater than 7 km. The depth of the detachment system is 8–10 km, and it controls the origin of the Jiaolai Basin (Zhang et al., 2006). The sedimentary age of the Wawukuang Formation at the bottom of the Jiaolai Basin is Early Cretaceous. The source of these sediments was mainly the denudation-transport-deposition of the metamorphic rocks in the early Jiaobei uplift and Weihai uplift.
Terrestrial heat flow is considered to be an important parameter for studying the geodynamic processes of the formation and evolution of orogenic belts (Lee, 1970; Sclater and Francheteau, 2010; Majorowicz and Wybraniec, 2011). Based on heat flow data from the Precambrian shields in North America and South Africa, Jaupart and Mareschal (1999) found that large-scale variations in the bulk crustal heat production are well-documented and imply significant differences in the deep lithospheric thermal structure. In thick lithosphere, surficial heat flow measurements record the time averaged heat production in the lithospheric mantle, which is not in equilibrium with the instantaneous heat production.
In China, Hu et al. (2000) created a new compilation of heat flow data for the continental area of China, including 862 observations from different sites. The relationships between the heat flow and geological ages based on this new dataset indicate that the surface heat flow is obviously more dependent on the most recent tectonic-thermal activity than on the age of the orogeny. Different tectonic units have different heat flow backgrounds owing to their different tectonic-thermal evolutions. Terrestrial heat flow is also an important basis for understanding the regional temperature field. Based on its geothermal and geological background, the Jiaodong Peninsula lies in the high heat flow zone on the west coast of the Pacific Rim, which is the junction zone between the North China Plate and the Yangtze Plate. The structures and intrusive rocks are well developed, which provide a good channel for the formation, conduction, and storage of geothermal resources. In general, the Jiaodong Peninsula was a stable rock platform before the Mesozoic, and during this period, the terrestrial heat flow of the entire Jiaodong Peninsula was low. Since the Mesozoic, the Jiaodong Peninsula has entered a stage of rift development and has begun a slow warming process. From the Late Cretaceous to the Early Tertiary, the Jiaodong Peninsula was in a higher temperature stage. Since the Late Tertiary, the temperature conditions in the Jiaodong Peninsula have gradually begun to decline, but the high geothermal background developed in the previous period has been retained (Chen et al., 1988).
The temperature field can reflect the characteristics of the heat energy in the upper crust. Studying the characteristics of the temperature field is conducive to enhancing our understanding of the distribution of the heat energy in the crust. Li et al. (1997) created a 1:200,000 heat flow contour map of the Jiaodong Peninsula based on the SiO2 values of 578 reliable water samples from the Jiaodong Peninsula (Figure 3). It can be seen from Figure 3 that the heat flow values in the Jiaobei uplift and Weihai uplift are obviously higher than those in the Jiaolai depression. The values of the silica heat flow in the uplift areas are generally greater than 60 mW/m2, with a maximum of 85 mW/m2, while the values in the basin are generally less than 60 mW/m2. Compared with the geothermal heat flow of 47.15 mW/m2 in northern China, the Jiaodong Peninsula has a higher background terrestrial heat flow, especially in the anticlinal axis of the Jiaobei uplift and Weihai uplift where the heat flow is the highest (>80 mW/m2). In addition, Jiang et al. (2016) used two boreholes in Jiaodong Peninsula to calculate the heat flow in this area, and their results indicate that the Bohai Bay Basin has high heat-flow values. The regional distribution pattern of high heat flow values exhibits NE-SW and NNE-SSW trends, which is basically consistent with the strikes of the NE-SW and NNE-SSW faults in the Jiaodong Peninsula. In addition, it can be seen that the average heat flow decreases gradually with increasing distance from the anticlinal axis, and eventually, it becomes almost the same as the regional average heat flow value.
FIGURE 3. Terrestrial heat flow contours (A) and profiles (B) in the Jiaodong Peninsula based on silica temperatures (modified from Li et al., 1997).
According to the temperature of natural hot springs in the Jiaodong Peninsula, it was found that the closer to the center of the axis of the uplift area, the higher the temperature of the hot springs. The Baoquan hot spring (67°C), Hongshuilan hot spring (71°C), and Qili hot spring (66°C) are located along the axis of the Weihai uplift area and have significantly higher temperatures than the Tangcun hot spring (51°C) and the Xingcun hot spring (28°C), which are located farther from the axis. The temperature of the Xingcun hot spring, which is located near the contact zone between the Jiaolai Basin and Weihai uplift, is only 30°C (Table 1). Based on this, there are obvious differences in the surface heat flow in the different tectonic units such as the Jiaobei uplift, Jiaolai Basin, and Weihai uplift, and the terrestrial heat flow in different regions of the same tectonic unit also vary.
The Jiaodong Peninsula contains 16 hot springs. To determine the distribution of the geothermal resources, a 1:2,000 geological survey of the 16 geothermal fields was conducted, and we identified the main thermal conduction fault and water conduction fault. In addition, the reservoir channel was also identified. Geological survey and geophysical explanation methods were used to identify the locations of the faults controlling the distribution of the geothermal field. Temperature logging was used to delineate the distribution range of the geothermal field, with the 25°C isotherm as the boundary of the geothermal fields (Figures 4, 8).
In addition, we collected samples of the geothermal fluids from these 16 hot springs in accordance with the sampling specifications, and then, we sent the water samples to the laboratory for water quality analysis. The geochemistry data were used to calculate the reservoir temperatures of the geothermal fields (Table 1).
It can be seen from Figure 4 that the lithology of the geothermal field is relatively simple, mainly including the monzogranite from the Late Yanshanian in the Mesozoic. The surface of the geothermal field is covered by the fine sand of the Yihe Formation and the coarse sand of the Linyi Formation. There are three main faults controlling the geothermal field. F1 is the main fault zone in this area. Its strike is NW-SE, its dip is NE, its inclination is 60–70°, its width is about 20 m, and there are breccia and fault mud in the fault zone, so it is a water-blocking fault F2 also strikes NW-SE. Its dip is NE, and its width is about 10 m. It is a secondary fault of the F1 fault, and the former is a water-conducting fault. F3 is a NNE-SSW-oriented compression-torsion fault, and it is a heat-conducting fault. The high-permeability fracture zone formed by the intersection of the F2 fault and F3 fault provides a channel for the upward flow of the geothermal fluids, which forms the geothermal fluid channel of the Yujia hot spring. In this study, we define the boundary of the geothermal field as the 25°C isotherm. The geothermal wells in this geothermal field are mainly distributed to the north of F2 and the west of F1, and there is no obvious geothermal anomaly in the foot walls of F1 and F3.
In past decades, to identify more geothermal fields in the Jiaodong Peninsula, many boreholes have been drilled in the geothermal field, and most of the boreholes were drilled without finding geothermal resources. In this study, we collected the temperature logs of 18 wells to estimate the temperature conditions in this area. The temperature logging was conducted at least 1 month after the completion of the drilling. Thus, the temperature was considered to be stable. Figure 6 shows the positions of the wells in the area, and Figure 7 shows the temperature logs with depth.
To better compare the differences between the wells in the geothermal fields and the wells outside of the geothermal fields, in Figures 5, 6, we plotted the Yujia, Zhaoyuan, and Wendeng hot springs for comparison (No. 13, No. 17, and No. 18, respectively).
FIGURE 5. Locations of the temperature logging wells (Figure 6).
FIGURE 6. Temperature logging curves (Figure 5).
Leapfrog Geothermal is a 3D modeling visualization and resource management software developed by ARANZ Geo (Applied Research Associates Ltd.). It includes geoscientific input from GNS Science and meets the 3D computing needs of the geothermal industry. Leapfrog Geothermal is based on implicit modeling methods that represent the geology, structure, geophysical, and reservoir data using fitted mathematical functions. Complex geological models are built by combining measured field data, specialist interpretations, and user editing. The Leapfrog software is easy to use, and it allows the quick and efficient creation of 3D models. It is also routinely updated (Alcaraz et al., 2011; Tri et al., 2017; Wulaningsih et al., 2017).
The area of the Jiaodong Peninsula is very large, so we just take the Weihai uplift area as an example to illustrate the heat distribution of the hot springs and wells in the Jiaodong Peninsula (Figure 7). In Figure 7, the modeled area is shown by the blue square, and the faults, hot springs, and boreholes distribution are within the modeled section.
For the surface data, the 1:500,000 scale geological map was used. The faults in this area are mainly the Mishan fault, the Wenquan fault, and the Xizicheng fault, and the hot springs and borehole wellhead locations (coordinates) were also input into the Leapfrog software. The subsurface information consists of the lithology of the Weihai uplift, which is relatively simple, with a very thin Quaternary sand layer at the surface and granite below. In this area, we mainly used nine hot springs and three boreholes with temperature logs and lithology data to build the model. The boundaries of the model are listed in Table 2.
The tertiary structural units in the study area mainly include the Jiaobei uplift, Jiaolai Basin, and Weihai uplift. The main structural traces include the Rushan-Weihai anticline, Qixia anticline, and Jiaolai syncline. The anticline structure is ridge-type with a narrow upper part and wide lower part, which is beneficial to the lateral migration and redistribution of geothermal fluids (Zhao et al., 2017). The overall strike of the faults is consistent with the strain at the boundary between the Eurasian Plate and Pacific Plate, which easily causes earthquakes (Chen et al., 2004). The NE-SW and NNE-SSW trending faults mainly compressive faults and torsional faults and mainly conduct heat. The NW-SE faults are mainly tensional or tensional-shear faults and mainly conduct water.
Figure 8 presents a typical geothermal field distribution map of the Jiaodong Peninsula, including the Hongshuilan, Wenshi, Daying, and Xiao hot springs, with the 25°C isotherm as the boundary of the geothermal fields. It can be seen from Figure 8 that the distribution range of the geothermal fields, such as the Hongshuilan, Wenshi, Daying, and Xiao hot springs, are mainly distributed within the V-shaped area where the upper block of the NE- and NW-trending faults intersect. The geothermal reservoir is controlled by the NE- and NW-trending faults and has an irregular columnar shape.
FIGURE 8. Typical geothermal field distribution in the Jiaodong Peninsula: (A) Hongshuilan, (B) Wenshi, (C) Daying, and (D) Xiao hot springs.
It can be seen from the 1:2,000 geological geothermal survey of the 16 geothermal fields that the geothermal resources in the Jiaodong Peninsula are mainly distributed in the V-shaped area where the upper block of the NE-SW and NW-SE trending faults intersect (Figure 8). The geothermal reservoir is controlled by the NE-SW and NW-SE trending faults and has an irregular columnar shape. Using Visual Modflow, in this study, a V type structural control and thermal conductivity model was developed (Figure 9). As can be seen from Figure 8, A-A’ and B-B’ are faults with different trends. Fault A-A’ is water conducting and fault B-B’ is thermal conducting. The surface water goes into the crust with the deep circulation, and when the water reaches the intersection of the water conducting and thermal conducting faults, the cool water is heating via heat convention. Then, the hot water flows upward along the fracture zone.
In the Weihai uplift area, the granite is widely distributed, and the Quaternary sand layer is very thin, i.e., less than 50 m in the geothermal field. Thus, in this stratigraphic model the lithology can be divided into two layers: an upper thin sand layer and a lower granite layer. Since the sand layer is very thin compared to the granite layer, it is not possible to show the sand layer in this model. The main faults are the Mishan fault, the Wenquan fault, and the Xizicheng fault (Figure 10).
FIGURE 10. Stratigraphic model of the Weihai uplift area (see Figure 8).
The temperature data were used to create a 3D temperature model, which sheds light on the importance of the major geological structures and their relationships to the heat flow (Figure 11).
FIGURE 11. 3D temperature model of the Weihai uplift, including the Mishan fault, the Wenquan fault, and the hot springs (Figure 10).
It can be seen from Figure 11 that in the Weihai uplift, the hot springs are mainly distributed around the Wenquan fault, and the hot springs are distributed almost on a line along it. The temperature along the Wenquan fault is much higher than in other locations in the model area. In addition, along the Wenquan fault, the temperature is the highest at the Hongshuilan hot spring (Figure 12).
FIGURE 12. Temperature profile A-B (Figure 10).
To study the reservoir temperature characteristics of the hot springs in the Jiaodong Peninsula and to preliminarily determine their temperature, geothermal fluid samples were collected from 16 natural hot springs in the Jiaodong Peninsula and the water was analyzed. Owing to the mixing of seawater, the water samples from some of the hot springs not only contained a large amount of Cl− but also large amounts of Na+ and K+. The Na+, K+, and other cations are not easy to balance with the surrounding rock. Therefore, in this study, the cation temperature scale method could not be used and the SiO2 temperature scale (silica temperature) was used to calculate the reservoir temperature.
The formula for calculating the reservoir temperature T (°C) based on the SiO2 temperature scale is as follows:
where S is the SiO2 concentration of the water (mg/L).
According to the SiO2 temperature scale, the reservoir temperature range of the hot springs is 106–137°C (Table 3), so all of the reservoir temperatures are lower than 150°C, making this a low-medium temperature geothermal system. This means up to now there is no high-temperature heat source similar to magmatic intrusions in this region through which the geothermal water flows.
Figure 13 shows the lithology and temperature logs for the Zangnan well (No. 14 in Figures 6, 7). As can be seen from Figure 13, the cap rock is gravel sand, and the cap is very thin (only 10 m). The lithology is mainly monzonitic granite. In fact, in the Jiaodong Peninsula, the cap rocks of all of the geothermal fields are mainly composed of gravel sand, and the caps are very thin, with thicknesses of mostly less than 50 m.
As can be seen from Figure 7, the temperature curve of the well in the geothermal field is not linear, but the temperature logs of the wells outside of the geothermal fields are linear. This indicates that outside of the geothermal fields, there are no obvious anomalies in the geothermal gradient for this type of well, and it is mainly based on the normal terrestrial heat flow heating the groundwater. The temperature gradient outside of the geothermal fields mainly ranges from 1.9°C/100 m to 2.3°C/100 m.
Based on the above analysis, the boreholes outside of the geothermal fields have normal geothermal gradients. No high temperature heat source such as magma was found in this area, and no obvious geothermal heat anomalies were found in the deep crust. Most of the heat anomalies in the boreholes come from the geothermal fluids. The main heat source of the water was the terrestrial heat flow and heat-conducting faults.
The thermal structure of the lithosphere controls many of the properties of and processes in Earth’s crust (Furlong and Chapman, 2013). Based on comparative analysis of the temperature conditions in the geothermal anomaly area and the non-geothermal anomaly area, it is proposed that the geothermal fluid heat accumulation mode in the Jiaodong area is mainly a three-source heat accumulation mode. Here, we take the Wenquan hot spring as an example to analyze the heat source of the geothermal fields in the Jiaodong Peninsula. The heat source of the geothermal liquids mainly comes from 1) hydrothermal convection within the thermal conducting faults. This is the most important heat source for the geothermal fields in the Jiaodong Peninsula. 2) The second source is heat conduction via the terrestrial heat flow. It can be seen in Figure 3 that the heat flow values in the Jiaobei uplift and Weihai uplift are obviously higher, which provides the basic heat for this area. 3) The third heat source is conduction-convection during groundwater migration. When the surface water flows downward and deep migration occurs, the water absorbs the heat from the surrounding rock (Figure 14). The most important heat source is the hydrothermal convection heat accumulation in the thermal conduction fault zone. In particular, the deep and large cross-crust faults conduct the heat from the crust and upper mantle. Because these faults have stronger water conductivity, the faults become strong water-rich and water-conducting channels. The deep heat flow is absorbed by the deep fluids along these channels, so this is the main heat source of the natural hot springs in this area. The second heat source is conduction heat accumulation from terrestrial heat flow, which mainly occurs when there is no obvious thermal anomaly. This is the main heat source of the geothermal resources in the non-geothermal anomaly areas. The third heat source is groundwater transport and conduction-convective heat accumulation. The surface water supplied by rainfall migrates to deeper depths, the heat from the surrounding rock is continuously absorbed, and heat is continuously accumulated. These three heat sources are the main heat accumulation methods of the geothermal resources in the Jiaodong Peninsula.
FIGURE 14. Recharge, runoff, drainage, and heat sources of the Wenquan hot springs geothermal field.
The outflow of heat from the Earth’s interior, i.e., the terrestrial heat flow, and the temperature field at depth are determined by deep-seated tectonic processes. Analyzing the regional heat flow pattern is a useful tool for studying the structures of the crust and lithospheric (Horai and Shankland, 1987; David, 1994). The factors influencing the shallow temperature field in the Jiaodong Peninsula include the structural distribution, the thermal properties of the different strata, the heat storage distribution, and groundwater activity (Rhee, 1975; Roy et al., 1981; Rybach and Bunterbarth, 1982; Blackwell and Steele, 1989; Pribnow et al., 2013).
Owing to the strong activity in the upper mantle and the crustal movements during the Yanshanian, during the formation of the Jiaobei uplift and the Weihai uplift, the central axis became more fractured relative to the two-wings due to the compressive stress. There are a series of NE-SW, NNE-SSW, and NW-SE faults, and the lithology is mainly Mesozoic, Archean and Proterozoic monzonitic granites. Previous studies have shown that the thermal conductivity increases with increasing pressure, and it increases nonlinearly with increasing axial pressure. The higher the porosity is, the higher the thermal conductivity of the granite is (Zhao et al., 1995; Durutürk et al., 2002; Chen et al., 2016). Therefore, in the Jiaodong Peninsula where granite is widely distributed, the more fractured uplift axis and the fault zone are characterized by higher thermal conductivities and faster heat transfer. The fracture zone provides a channel for the upwelling of the deep heat flow.
The thermophysical parameters of rocks mainly refer to physical properties such as the thermal conductivity, thermal diffusivity, specific heat capacity, and radioactive heat generation rate, which are important indicators for characterizing the thermophysical properties of rocks (Xu, 1992). The thermophysical properties of rocks directly affect the thermal generation, storage, and transmission of heat between the various layers. They are indispensable parameters for studying the temperature distribution and heat transfer (Song et al., 2019).
The lithology of the Jiaobei uplift and Weihai uplift is dominated by granitic intrusive rocks. The average thermal conductivity of the granite in the Sanshandao borehole in Laizhou, Jiaobei uplift, is 2.64–3.56 W/m•K and that of gneiss is 2.24–3.12 W/m•K (Jiang et al., 2016). The thermal conductivities of 32 granite samples measured in the Dong hot spring area are 2.34–3.68 W/m•K. The thermal conductivities of 20 granite samples measured in the Tangbo hot dry rock exploration hole in the Weihai uplift area are 2.29–3.63 W/m•K. It can be seen that the thermal conductivities of the granitic rocks in the Jiaodong uplift area are 2.2–3.6 W/m•k, while the thermal conductivity of the granite in the Zhangzhou area of the eastern part of China is 3.19 W/m•K. The thermal conductivity of felsic and mafic rocks is 2.52 W/m•K (Wang et al., 2015), which is similar to the thermal conductivity of the granite in the Jiaodong Peninsula. The Jiaolai Basin is dominated by sandstone. The thermal conductivity of sandstone (1.84 W/m•K) is generally lower than that of granite (Zhou et al., 2011). In addition, the sandstone has a much lower permeability than the granite.
The shape of the reservoir and the movement of the geothermal fluids are also the main factors affecting the distribution of the local temperature field. The reservoir’s shape determines the flow space of the fluids inside the Earth. The geothermal fluid carrying the heat also affects the distribution of the temperature around the reservoir. This is also the main reason for the local thermal anomalies on the surface of the Jiaodong Peninsula.
As can be seen from Figure 15, during upwelling, the heat flow from the interior of the Earth reaches the Moho surface first and then enters the crust. The undulations of the Moho surface are opposite to those of the surface. When the deep heat flow reaches this interface, there is no obvious difference. However, because the lithology in the uplift area is granite and that in the basin is sandstone, the difference in the thermal conductivities and permeabilities of the different rocks lead to the bottom (A1) becoming a heat insulating and water insulating surface. When the heat flow reaches to the bottom (A1) of the Jiaolai Basin, the heat flow changes direction and is redistributed along the bottom of A1 toward both sides of the uplifts, which have a higher thermal conductivity. The rising heat flow accumulates at a certain depth in the crust (areas B1 and B2), which results in the uplift area having a higher temperature. This explains why the silica heat flow value in the uplift area is higher than the silica heat flow value in the basin (Figure 3).
Through geothermal geological survey and heat conduction and water conduction structural analysis of the 16 hot springs in the Jiaodong Peninsula, the mechanisms of the geothermal resources in Jiaodong Peninsula were comprehensively analyzed. We propose a V-type structural control and thermal conductivity model. Based on the heat flow distribution, the logging data for the wells, and the thermophysical parameters of the rocks in the Jiaodong Peninsula, a 3D model of the temperature and lithology and a conceptual model were established. The main conclusions of this study are as follows.
(1) The geothermal resources in the Jiaodong Peninsula are mainly distributed in the V-shaped area where the upper block of the NE- and NW-trending faults intersect. The geothermal reservoir is controlled by the NE- and NW-trending faults and has an irregular columnar shape.
(2) The lithology in the uplift area is mainly granite, that in the basin is mainly sandstone, and the heat flow value in the uplift area is much higher than that in the basin. Due to the differences between the thermophysical parameters of the different rocks, the heat flow changes direction at the bottom of the basin and redistributes toward both sides to the uplifts, which results in the uplift area having a higher heat flow value. This demonstrates the diversion and accumulation mode of the heat flow in the deep crust in the Jiaodong Peninsula.
(3) For the geothermal fluids in the Jiaodong Peninsula, the heat is mainly supplied by the terrestrial heat flow and the heat-conducting faults. Up to now, there is no high-temperature heat source similar to magmatic intrusions in this area. The heat of the geothermal liquids mainly comes from three sources: hydrothermal convection in the thermal conducting faults, heat conduction via terrestrial heat flow, and conduction-convection during groundwater migration.
The original contributions presented in the study are included in the article/Supplementary Material, further inquiries can be directed to the corresponding author.
Conceptualization, MS and FK; investigation, MS, TY, SG, XG, and XY; writing—original draft preparation, MS; writing—review and editing, FK; supervision, FK; project administration, MS; funding acquisition, FK. All authors have read and agreed to the published version of the article.
This research was supported by the National Natural Science Foundation of China (grant numbers U1906209, 42072331) and financial foundation of Yantai City (SDGP37600202102000097).
The authors declare that the research was conducted in the absence of any commercial or financial relationships that could be construed as a potential conflict of interest.
All claims expressed in this article are solely those of the authors and do not necessarily represent those of their affiliated organizations, or those of the publisher, the editors and the reviewers. Any product that may be evaluated in this article, or claim that may be made by its manufacturer, is not guaranteed or endorsed by the publisher.
We thank UNU Geothermal Training Programme for applying for 6 months free use of leapfrog for me, and thank Vivi Dewi Mardiana Nusantara who comes from PT Pertamina Geothermal Energy, Indonesia and Erick Jiménez for their help in the establishment of the leapfrog model, and also thank LetPub for its linguistic assistance during the preparation of this manuscript. We are grateful to editors and reviewers for their constructive comments and valuable suggestions that significantly improved this manuscript.
Alcaraz, S. A., Lane, R., Spragg, K., Milicich, S. D., and Bignall, G. (2011). “3-D Geological Modelling Using New Leapfrog Geothermal Software,” in Proceedings, 36th Workshop on Geothermal Reservoir Engineering. doi:10.3901/JME.2008.10.294
Blackwell, D., and Steele, J. (1989). Thermal Conductivity of Sedimentary Rocks: Measurement and Significance. New York: Springer. doi:10.1007/978-1-4612-3492-0-2
Chen, B., Chen, C., Kaban, M. K., Du, J., Liang, Q., and Thomas, M. (2013). Variations of the Effective Elastic Thickness over China and Surroundings and Their Relation to the Lithosphere Dynamics. Earth Planet. Sci. Lett. 363, 61–72. doi:10.1016/j.epsl.2012.12.022
Chen, L. (2009). Lithospheric Structure Variations between the Eastern and central North China Craton from S- and P-Receiver Function Migration. Phys. Earth Planet. Interiors. 173, 216–227. doi:10.1016/j.pepi.2008.11.011
Chen, M., Wang, J., Wang, J., Xiao, D., Yang, S., Xiong, L., et al. (1990). The Characteristics of the Geothermal Field and its Formation Mechanism in the North China Down-Faulted basin (In Chinese with English Abstract). Acta Geologica Sinica. (1), 80–91.
Chen, Y., Franco, P., and Lai, Y. (2004). Metallogenic Time and Tecctonic Setting of the Jiaodong Gold Province, Eastern china (In Chinese with English Abstract). Acta Petrologica Sinica. 20 (4), 907–922.
Chen, Z., Wu, X., Chen, F., Wang, H., and Sun, D. (2016). Research of thermal Conductive Characteristics of Granite under Uniaxial Compression. Coal Eng. 48 (9), 113–116.
Deming, D. (1994). Estimation of the thermal Conductivity Anisotropy of Rock with Application to the Determination of Terrestrial Heat Flow. J. Geophys. Res. 99 (B11), 22087–22091. doi:10.1029/94jb02164
Durutürk, Y. S., Demirci, A., and Keçeciler, A. (2002). Variation of thermal Conductivity of Rocks with Pressure. CIM Bull. (95), 67–71. Available at: https://www.researchgate.net/publication/290823333.
Furlong, K. P., and Chapman, D. S. (2013). Heat Flow, Heat Generation, and the thermal State of the Lithosphere. Annu. Rev. Earth Planet. Sci. 41, 385–410. doi:10.1146/annurev.earth.031208.100051
He, L. (2015). Thermal Regime of the north china Craton: Implications for Craton Destruction. Earth-Science Rev. 140, 14–26. doi:10.1016/j.earscirev.2014.10.011
Horai, K., and Shankland, T. (1987). 8. Thermal Conductivity of Rocks and Minerals. Methods Exp. Phys. 24 (1), 271–302. doi:10.1016/S0076-695X(08)60589-X
Hu, S., He, L., and Wang, J. (2000). Heat Flow in the continental Area of China: a New Data Set. Earth Planet. Sci. Lett. 179 (2), 407–419. doi:10.1016/S0012-821X(00)00126-6
Hu, S., and Wang, J. (1994). Heat Flow Characteristics of Orogenic Belts in southeastern China (In Chinese with English Abstract). Geol. Rev. 40 (5), 387–394.
Hu, Y., Liu, S., Fu, J., Sun, G., Gao, L., and Guo, R. (2021). Neoarchean-early Paleoproterozoic Granitoids, the Geothermal Gradient and Geodynamic Evolution in the Hengshan Terrane, north china Craton. Gondwana Res. 94 (2), 143–163. doi:10.1016/j.gr.2021.03.004
Jaupart, C., and Mareschal, J. C. (1999). The thermal Structure and Thickness of continental Roots. Lithos. 48, 93–114. doi:10.1016/S0419-0254(99)80007-X
Jiang, G., Tang, X., Rao, S., Gao, P., Zhang, L., Zhao, P., et al. (2016). High-quality Heat Flow Determination from the Crystalline Basement of the South-East Margin of North China Craton. J. Asian Earth Sci. 118, 1–10. doi:10.1016/j.jseaes.2016.01.009
Jin, B., Zhang, Y., and Luan, G. (1999). Characteristics of Geothermal Resources in Jiaodong Peninsula (In Chinese with English Abstract). Ludong Univ. J. (Natural Sci. Edition). 15 (4), 297–301. doi:10.16030/j.cnki.issn.1000-3665.2000.05.010
Kang, F. (2013). Sustainable Yield and its Assessment of Geothermal Reservoirs in china. Trans. - Geothermal Resour. Counc. 37 (4), 843–852.
Lee, W. H. K. (1970). On the Global Variations of Terrestrial Heat-Flow. Phys. Earth Planet. Interiors 2 (5), 332–341. doi:10.1016/0031-9201(69)90026-0
Li, X., Liu, B., Sun, X., and Wang, Y. (1997). Relationship between the Silica Heat Flow and Regional Geological Conditions in Shandong Peninsula (In Chinese with English Abstract). J. Ocean Univ. Qingdao 27 (1), 75–83. doi:10.16441/j.cnki.hdxb.1997.01.011
Liang, Y., Guo, W., Ma, Y., and Zhao, E. (2020). Geochemical Evidence for Thickening and Thinning of Lower Crust beneath the Jiaodong Peninsula: Implications for Late Mesozoic Destruction of the Eastern North China Craton. Lithosphere. 2020 (1), 1–17. doi:10.2113/2020/8831664
Lin, Z. T., Zhu, B., Lin, X., Chen, Y., and Liu, Y. Z. (2013). Study on the Mechanism of the Anodic Oxidation of PAN-Based Carbon Fibers by Cyclic Voltammetry. Adv. Mater. Res. 774-776 (5), 832–835. doi:10.4028/www.scientific.net/amr.774-776.832
Liu, C., Deng, J., Li, S., Xiao, Q., Jin, T., Sun, H., et al. (2018). Discussions on Crust-Mantle Structure during the Formation of Yanshanian of Large-Super Large Scale Deposits in the Jiaodong Gold Ore District: Constraints from Ore-Forming Igneous Assemblage (In Chinese with English Abstract). Earth Sci. Front. 25 (6), 296–307. doi:10.13745/j.esf.sf.2018.11.16
Lv, C., Wu, G., and Boyko, K. J. (2015). A Study of Distribution and Charge of Regional Tectonic Fluid in Goldfields of Jiaodong Peninsula (In Chinese with English Abstract). Earth Sci. Front. 22 (4), 113–121. doi:10.13745/j.esf.2015.04.013
Majorowicz, J., and Wybraniec, S. (2011). New Terrestrial Heat Flow Map of Europe after Regional Paleoclimatic Correction Application. Int. J. Earth Sci. (Geol Rundsch) 100 (4), 881–887. doi:10.1007/s00531-010-0526-1
Mao, J., Li, H., Wang, Y., Zhang, C., and Wang, Y. (2005). The Relationship between Mantle-Derived Fluid and Gold Ore-Formation in the Eastern Shandong Peninsula: Evidences from D-O-C-S Isotopes. Acta Geologica Sinica. 79 (6), 839–857. doi:10.1360/gs050303
Pan, S., Wang, F., Zheng, Y., Duan, Y., Liu, L., Deng, X., et al. (2015). Crustal Velocity Structure beneath Jiaodong Peninsula and its Tectonic Implications (In Chinese with English Abstract). Chin. J. Geophys. 58 (9), 3251–3263. doi:10.6038/cjg20150920
Pribnow, D., Williams, C. F., Sass, J. H., and Keating, R. (2013). Thermal Conductivity of Water‐saturated Rocks from the KTB Pilot Hole at Temperatures of 25 to 300 °C. Geophys. Res. Lett. 23 (4), 391–394. doi:10.1029/95gl00253
Qiu, N., Zuo, Y., Chang, J., and Li, W. (2014). Geothermal Evidence of Meso-Cenozoic Lithosphere Thinning in the Jiyang sub-basin, Bohai Bay Basin, Eastern North China Craton. Gondwana Res. 26 (3-4), 1079–1092. doi:10.1016/j.gr.2013.08.011
Rhee, S. K. (1975). Porosity-Thermal Conductivity Correlations for Ceramic Materials. Mater. Sci. Eng. 20 (1), 89–93. doi:10.1016/0025-5416(75)90134-2
Roy, R. F., Beck, A. E., and Touloukian, Y. S. (1981). Thermophysical Properties of Rocks. Phys. Prop.Rocks Min. 2 (2), 409–502.
Rybach, L., and Buntebarth, G. (1982). Relationships between the Petrophysical Properties Density, Seismic Velocity, Heat Generation, and Mineralogical Constitution. Earth Planet. Sci. Lett. 57 (2), 367–376. doi:10.1016/0012-821x(82)90157-1
Rybach, L., Mégel, T., and Eugster, W. J. (2000). “At what Time Scale Are Geothermal Resources Renewable?” in World Geothermal Congress 2000 (Japan: Kyushu-Tohoku), 867–872.
Rybach, L., Mégel, T., and Eugster, W. J. (1999). How Renewable Are Geothermal Resources? Trans. - Geothermal Resour. Counc. 23, 563–566.
Sanyal, S. K. (2004). Sustainability and Renewability of Geothermal Power Capacity. Trans. - Geothermal Resour. Counc. 28, 804–817. doi:10.1007/978-1-4614-5820-3-229
Sclater, J. G., and Francheteau, J. (1970). The Implications of Terrestrial Heat Flow Observations on Current Tectonic and Geochemical Models of the Crust and Upper Mantle of the Earth. Geophys. J. Int. 20 (5), 509–542. doi:10.1111/j.1365-246X.1970.tb06089.x
Song, X., Jiang, M., Peng, Q., and Xiong, P. (2019). Thermal Property Parameters and Influencing Factor Analysis of Main Rock Strata in Guizhou Province (In Chinese with English Abstract). Acta Geologica Sinica. 93 (8), 2092–2103. doi:10.19762/j.cnki.dizhixuebao.2019116
Tan, J., Wei, J., Yang, C., Feng, B., Tan, W., and Guo, D. (2006). Geochemstry and Tectonic Setting of Dikes in the Guocheng Area, Jiaodong Peninsula. Acta Geologica Sinica 80 (8), 1177–1188. doi:10.1111/j.1745-4557.2006.00081.x
Wang, A., Sun, Z., Liu, J., Hu, B., Wan, J., and Yang, L. (2015). Radiogenic Heat Production of Rocks from Zhangzhou, Southeast China and its Implications for thermal Regime of Lithosphere. Sci. Technology Rev. 33 (24), 41–45. doi:10.3981/j.issn.1000-7857.2015.24.007
Wulaningsih, T., Fujii, Y., and Tsutsumi, S. (2017). “A 3D Model of the Yamagawa Geothermal System: Insights into Reservoir Structure and Future Field Management,” in Proceedings 39th New Zealand Geothermal Workshop.
Xie, X., and Yu, H. (1988). The Characteristics of the Regional Geothermal Field in Sichuan Basin (In Chinese with English Abstract). J. Chengdu Coll. Geology. (4), 110–117.
Xiong, L., and Gao, W. (1982). Characteristics of Geothermal in Uplift and Depression (In Chinese with English Abstract). Acta Geophysica Sinica. 25 (5), 60–68.
Xiong, L., and Zhang, J. (1984). Mathematical Simulation of Refract and Redistribution of Heat Flow (In Chinese with English Abstract). Chin. J. Geology. (4), 445–454.
Xu, Z. (1992). A Discussion of Factors Influencing Thermophysical Characteristics of Rocks and Their Mechanisms (In Chinese with English Abstract). Pet. Exploration Development 19 (6), 85–89.
Zhang, J., Sun, H., and Xiong, L. (1982). Mathematical Simulating of Regional Geothermal Field and Case Analysis (In Chinese with English Abstract). Chin. J. Geology. (3), 315–321. doi:10.1007/BF01033890
Zhang, Y., Li, J., Liu, Z., Ren, F., and Yuan, J. (2006). Detachment Systems in Deep of Jiaolai basin and Their Regional Tectonic Significance. Oil Gas Geology. 27 (4), 504–511. doi:10.11743/ogg20060410
Zhao, X., Li, F., Zeng, J., and Jin, F. (2017). The Geological Characteristics and Origin of Deep Geothermal Water in Baxian Sag. Earth Sci. Front. 24 (3), 210–218. doi:10.13745/j.esf.2017.03.019
Zhao, Y., Yang, S., Zhang, W., Liang, X., and Ma, L. (1995). An Experimental Study of Rock thermal Conductivity under Different Temperature and Pressure (In Chinese with English Abstract). Prog. Geophys. 10 (1), 104–113.
Zhou, Y., Ji, Y., Zhang, S., and Wan, L. (2011). Characteristics and Controlling Factors on Physical Properties of Low-Permeability sandstone of the Laiyang Formation in the Laiyang Sag, Jiaolai Basin (In Chinese with English Abstract). Acta Petrolei Sinica 32 (4), 611–620. doi:10.1007/s12182-011-0123-3
Keywords: Jiaodong Peninsula, uplift and basin, refraction and redistribution, V-shaped area, heat flow accumulation
Citation: Shi M, Kang F, Yin T, Gao S, Sui H, Guo X and Yu X (2022) Occurrence Mechanism of Convective Geothermal Systems in Jiaodong Peninsula, China. Front. Earth Sci. 10:898414. doi: 10.3389/feart.2022.898414
Received: 17 March 2022; Accepted: 04 April 2022;
Published: 26 April 2022.
Edited by:
Wenjing Lin, Chinese Academy of Geological Sciences, ChinaReviewed by:
Bo Feng, Jilin University, ChinaCopyright © 2022 Shi, Kang, Yin, Gao, Sui, Guo and Yu. This is an open-access article distributed under the terms of the Creative Commons Attribution License (CC BY). The use, distribution or reproduction in other forums is permitted, provided the original author(s) and the copyright owner(s) are credited and that the original publication in this journal is cited, in accordance with accepted academic practice. No use, distribution or reproduction is permitted which does not comply with these terms.
*Correspondence: Fengxin Kang, a2FuZ2Zlbmd4aW5AMTI2LmNvbQ==
Disclaimer: All claims expressed in this article are solely those of the authors and do not necessarily represent those of their affiliated organizations, or those of the publisher, the editors and the reviewers. Any product that may be evaluated in this article or claim that may be made by its manufacturer is not guaranteed or endorsed by the publisher.
Research integrity at Frontiers
Learn more about the work of our research integrity team to safeguard the quality of each article we publish.