- 1Chengdu University of Technology, Chengdu, Sichuan, China
- 2Institute of Exploration Technology, Chinese Academy of Geological Sciences, Chengdu, Sichuan, China
- 3Sichuan Institute of Geological Engineering Investigation Group Co. Ltd., Chengdu, Sichuan, China
The geothermal resource has become the significant constitution of renewable and clean energies in the world. This study focuses on the genetic mechanism of a high-temperature geothermal system and its engineering impact in the Woka graben, southern Tibet, via hydrochemical and isotopic analyses. The hydrochemical types are mainly SO4-Na type, SO4-Cl-Na type, and HCO3-SO4-Na type. Geothermal water is characterized as medium to alkaline affinity with low total dissolved solids. D-O isotopes indicate that geothermal water is recharged by atmospheric precipitation at the elevation of 5193–5247 m. Na-K-Mg equilibrium diagram shows partial equilibrium or mixed water, and the proportion of cold water mixing is 73–83%. The temperature ranges of shallow and deep geothermal reservoirs are from 96.85°C to 119.57°C and from 120°C to 200°C, respectively. Geothermal water is heated by melting crust and controlled by deep faults. For major construction projects in the Woka graben, detailed investigation and demonstration should be conducted to avoid the geothermal water channel as much as possible, or to divert the geothermal water and reasonably arrange the construction sequence to overcome the problem.
Introduction
Among the more than 3,000 hydrothermal activity areas identified in China, 677 are occurring in Tibet (Liao, 2018; Wang et al., 2018; Zhang and Hu, 2018). In addition, 342 geothermal manifestations are available for exploration, with the vast majority having a temperature of more than 80°C. Geothermal resource in Tibet is characterized by high-temperature, various types, and extensive distribution (Guo, 2012; Kong et al., 2014). The distribution of geothermal resources in Tibet is strictly controlled by active faults and is closely related to the regional geothermal heat flow background (Jiang et al., 2018; Tan et al., 2018). Taking the high-temperature vapor geothermal manifestations in southern Tibetan as an example, numerous active tectonic belts have developed in the N-S direction and consist of a series of uplift zones and fracture zones along the Himalayan arc tectonic belt. Afterward, a series of Quaternary graben basins were formed, in which strong modern hydrothermal activity has developed, e.g., the Langjiu, Kawu, Yangbajing, and Gudui geothermal fields (Zhang et al., 2015; Wang et al., 2016).
The Cona–Woka rift valley belt is the easternmost side of the Tibetan rift valley belt near the north–south fracture belt, by the Woka, Qiongduojiang, and Cona grabens. The graben is enriched in geothermal resources, where the second largest geothermal manifestation (Gudui geothermal field) in Tibet is distributed. The Woka geothermal manifestation is located in the Woka graben in the northern section of the Cona–Woka rift valley. Along the Zengjiuqu River valley and slope, geothermal manifestation with a length of 3 km and temperature of 43°C–68.2°C is developed, belonging to the low- to medium-temperature geothermal system. Previous studies focused on the hydrochemical characteristics and genetic mechanism of geothermal waters in Tibet (Elenga et al., 2021; Li et al., 2021; Tian et al., 2021; Wang et al., 2021; Klemperer et al., 2022; Wang et al., 2022). However, the studies on the genesis mode of the Woka geothermal springs are lacking. The geothermal reservoir and heat source are still unclear, making it difficult to evaluate the prospecting potential. So far, the Woka geothermal springs have been utilized for the bathing function. Therefore, research on the genetic mechanism of the Woka geothermal system can provide scientific guidance for the assessment of geothermal resource potential in the Woka graben and other areas in southern Tibet.
In this study, the hydrogeochemical characteristics, reservoir temperature, and subsurface cold water mixing ratio of geothermal springs in the Woka geothermal system are comprehensively studied using hydrochemical and isotopic data. Afterward, the conceptual model of a medium- to high-temperature geothermal system in the Woka geothermal zone is established. At last, the engineering significance of the geothermal system is investigated.
Study area
The study area is located in Sangri Township and Lobsa Township, 40 km away from Sangri County, Tibet. The study area has an alpine valley landform, steep bank slope, terrain slope of 30°–50°, and a height difference of 1000–1500 m. The climate in the area belongs to the plateau temperate monsoon semihumid climate zone, with an annual average temperature of 6.4°C–8.2°C and annual average precipitation of 500–600 mm, mainly concentrated from May to September, accounting for more than 89% of the annual rainfall. Rainfall has the characteristics of large annual variation and concentrated rainfall periods.
The Woka graben basin is located in the Himalayas terrane (Zhang et al., 2019a, 2022; Cao et al., 2019). The geothermal water system in the Woka graben basin is composed of the many geothermal springs distributed along the Zengjiuqu River valley in Woka graben basin, with 22 existing geothermal springs and a total flow rate of more than 20 L/s (Table 1). According to the characteristics of the underground geothermal water outcropping and the current situation of development and utilization, it can be divided into Kanai, Woka, Cuoba, and Lobosha geothermal springs. The upper part is light yellow subsandy or gravelly subsandy soil, and the lower part is mixed-colored pebbles, drift stones, and muddy pebbles. The Cretaceous–Paleocene black mica diorite (ηγβΚ2), granodiorite (γδΚ1), quartz diorite (δοK1), etc. are distributed along the watershed; the Middle to Lower Jurassic Yerba Formation (J1–2y) is exposed along the watershed, divided into three lithological sections: the first section is characterized by volcanic breccia and volcanic agglomerate; the second section is dominated by medium-acid andesite, andesite, rhyolite, and crystalline clastic tuff; and the third section is composed of sunken tuff, metamorphic sandstone, and siltstone. The geothermal geological background of the Woka graben is shown in Figure 1.
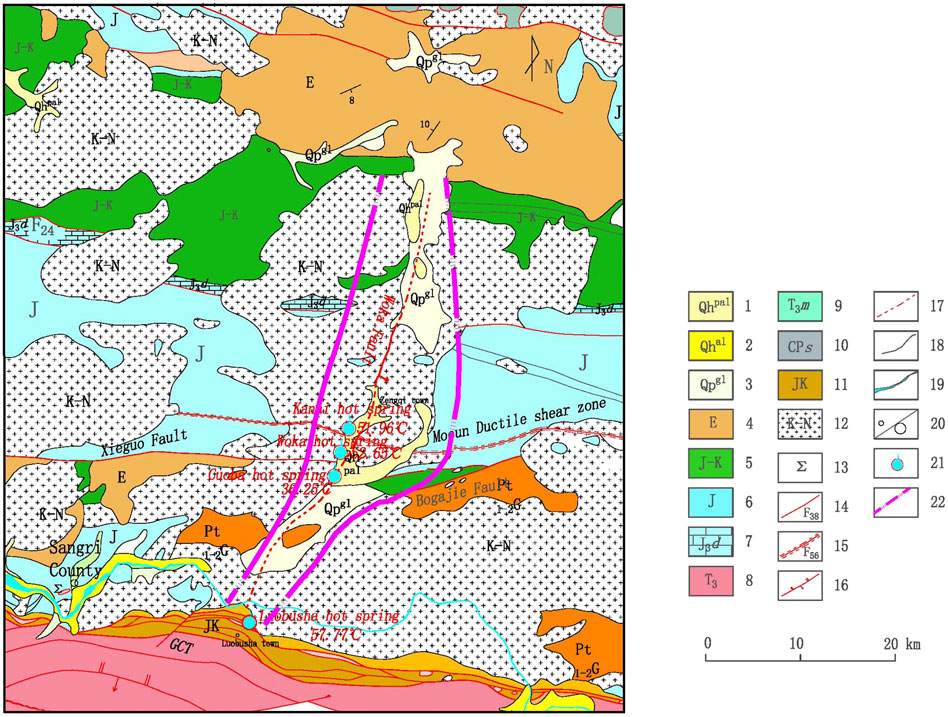
FIGURE 1. Geological background map of Oiga Graben basin. 1‐Holocene alluvial deposits; 2‐Holocene alluvium; 3‐Pleistocene glacial deposits; 4‐Paleogene volcanic rocks; 5‐Late Jurassic Cretaceous and Cretaceous; 6‐Sedimentary assemblage of Jurassic volcanic and continental clastic rocks; 7‐Duodigou formation limestone; 8‐Late Triassic Century; 9‐Mailonggang formation; 10‐Songduo group; 11‐Jurassic-Cretaceous melange; 12‐Cretaceous‐Neogene intrusive rocks; 13‐Ultrabasic block; 14‐Fault and number; 15‐Ductile shear zone and number; 16‐Reverse fault; 17‐Inferred fault; 18‐Geological boundary; 19‐River system; 20‐Town/City; 21‐Hot spring; 22‐Range of fault graben basin.
Woka basin is located in southern Tibet near the N-S development rift valley—the northern section of the Cuona—Woka rift valley, for regional extension and deformation, and the formation of the Quaternary activity is an obvious graben-type fracture basin (Cao et al., 2020; Cao et al., 2021). The total length of the rift basin is approximately 50 km, north of the Jinzhu township area, the southern end of the Luobusha chromite. Region-wise, the southern end of the Woka graben is bounded by the south-trending rebound fault zone, Yarlung Tsangpo River suture tectonic zone, and the north-trending Xela-Riduo-Palo fault in the northern section, which is interrupted by the near E-S trending development of the Mocun ductile fault and some pot faults.
The main boundary fault zone controlling the development of the Woka basin is the NNE-trending, west-trending, 50- to 60-km-long orthotropic fault on the eastern margin of the basin (Zhang et al., 2019b). In addition, there are ultra-low-resistance areas with a resistivity of less than 50 Ω m along the Zengjiuqu River valley based on several high-density physical profiles. Meanwhile, seismic activity is frequent within the Woka graben basin, and the results of fracture activity rate estimation indicate the vertical activity rate of this fault zone since MIS six ranges from 0.4 to 0.9 mm/a. Under the effect of long-term seismic activity, a network of tectonic fissures has been formed in the Woka graben basin that crosses east–west and north–south directions, providing storage space and runoff channels for the development of the underground geothermal water system in the Woka graben basin.
Sampling and methodology
In this study, four geothermal water samples were collected in January 2020 for the experiments of hydrochemistry, δ2H, δ18O, and δ13C. pH, total dissolved solids (TDS), and flow were measured in the field using a portable WTW device. The HCO3− content was in situ constraint by Gran titration. K+, Na+, Ca2+, and Mg2+ were determined via inductively coupled plasma-optical emission spectrometry, and Cl− and SO42− were measured via ion chromatography (Dionex ICS-1100) in the State Key Laboratory of Geohazard Prevention and Geoenvironment Protection, Chengdu University of Technology. The charge balance was lower than ±10%. δD and δ18O were analyzed using a mass spectrometer (MAT253), and δ13C was measured via stable isotope ratio mass spectrometry (Delta Plus XP) at the Institute of Karst Geology, Chinese Academy of Geological Science.
Results and discussion
Water–rock interaction by hydrochemical characteristics
Table 2 shows that the water chemistry of geothermal water in the Woka geothermal area is dominated by Na+, Ca2+, and K+ in cations and SO42− and Cl− in anions, followed by HCO3−. The pH values and total dissolved solid concentrations are 7.95–8.25 and 285.90–440.51 mg/L, respectively. Geothermal waters belong to medium–high alkaline water. The hydrochemistry types are mainly SO4-Na type, Cl-SO4-Na type, and HCO3-SO4-Na type.
The hydrochemical characteristics of geothermal water and bedrock fracture cold water in the fault rift basin are very different (Figure 2). The geothermal waters are enriched in H2S but poor in CO2. Their hydrochemical type is influenced by H2S, and there are more complex hydrochemical types such as SO4 type, SO4-Cl type, and HCO3-SO4 type, consistent with the Riduo geothermal spring and Gudui geothermal field in the fault rift zone. The hydrochemical type of the Luobusa geothermal spring is different from that of geothermal waters in the Woka rift, indicating that they do not belong to the same geothermal system.
As shown in the Schoeller diagram (Figure 3), all ions in the geothermal water have regular variations, revealing that the source and formation pattern of geothermal water in the basin have similar characteristics. In addition, the geothermal waters have high SO42− contents, consistent with the strong sulfur smell of the geothermal water outcrop, as typified by the Woka geothermal spring. The source of SO42− in geothermal water is mainly from geothermal water dissolution filtration of sulfate rocks, and a small part is H2S carried by deep fluids. Therefore, the higher SO42− contents in the geothermal water of Woka mainly come from the strong dissolution filtration of volcanic clastic rocks such as andesitic and rhyolitic lava dominated by the Yeba Formation strata. The saturation indices of geothermal water calculated using Phreeqc software are mostly lower than zero. The results show that most minerals, except for chalcedony and quartz, are unsaturated (Table 3), reflecting the short runoff pathway and weak water–rock reaction.
Four geothermal water samples were taken for δ13C analysis. The δ13C values of geothermal spring water are −6.49‰ to −3.45‰: the δ13C value of Kanai geothermal spring is −6.1‰, the δ13C value of Woka geothermal spring is −6.49‰, the δ13C value of Misbah geothermal spring is −5.41‰, and the δ13C value of Lobsa geothermal spring is −3.45‰ (Figure 4). Previous studies showed that the δ13C value of upper mantle material source is −8‰ to −4‰, the δ13C value of carbonate metamorphic source is −2‰ to +2‰, and the δ13C value of carbonate metamorphic source is −2‰ to +2‰. Therefore, it is presumed that mantle-sourced CO2 from deep faults was involved in the formation of geothermal waters in the study area.
Recharge source implied by D-O isotopes
Four geothermal water samples were selected for the analysis of hydrogen and oxygen isotopes. The results show that the δ2H values range from −150.8‰ to −156.3‰ and the δ18O values range from −19.46‰ to −19.86‰. In Figure 5, all samples are distributed near the global atmospheric precipitation equation line, indicating that the source is recharged by atmospheric precipitation and less infiltration of snow-melting water. The deuterium excess parameter (d) is similar between the geothermal spring and the cold spring, indicating that there is no obvious oxygen drift in the geothermal water. The result reveals the characteristics of atmospheric precipitation recharge and shallow circulation in the geothermal water system of the Woka rift. The deuterium excess parameter d of the Luobusa geothermal spring is −33.82, which shows significant oxygen drift, further supporting that it belongs to a different geothermal system from the Woka graben, with deep circulation recharge characteristics.
The isotopic composition of atmospheric precipitation has an elevation effect, and the recharge elevation of geothermal springs can be calculated using δ18O isotopic values based on this feature (Zhang et al., 2018). The calculation equation is as follows:
where H is the elevation of the recharge area, m; h is the elevation of the sampling point, m; δG is the δ18O value of the geothermal spring; δP is the δ18O value of atmospheric precipitation; and K is the δ18O elevation gradient of atmospheric precipitation (δ/100 m). The gradient value of δ18O is determined as follows: four typical cold springs were selected along the Zengjiuqu from upstream to downstream, and the δ18O was obtained by fitting the δ18O to the outcrop elevation of the spring. The curve is δ18O = −0.0037*H-107.98, R2 = 0.9473, and the height gradient obtained from the fit is 0.37‰/100 m, which is in good agreement with the height gradient of 0.31‰/100 m in Tibetan area. The elevation reference value is determined as follows: The lake water is used as the elevation reference point, and its δ18O = −15.0‰. The calculation results of the recharge elevation of each geothermal spring are shown in Table 4, and the results show that the recharge elevation of the geothermal water system in the Woka graben basin is 5193–5247 m, which is consistent with the elevation of the alpine basin where the regionally distributed snow mountains are located.
Reservoir temperature
The temperature of geothermal springs, as natural outcrops of geothermal water systems, is often low because of the mixing of shallow groundwater or surface water and cannot actually represent the temperature of the geothermal reservoir (Zhang, Xu, 2018; Li et al., 2020; Chang et al., 2021). Therefore, quantitative geochemical geothermometers such as SiO2 and cation geothermometers are usually used to calculate the reservoir temperature (Fournier, 1977; Truesdell and Fournier, 1977; Fournier, 1979). As seen in Figure 6, the Kanai geothermal springs and the Woka geothermal springs all fall in the partially equilibrated zone or mixed water zone, and the Cuoba geothermal springs and the Luobosha geothermal springs fall in the immature water zone, indicating that the water–rock interaction in the geothermal water system of the Woka fault rift basin has not reached to complete equilibrium, or the geothermal water is mixed with a large proportion of cold water in a nonequilibrium state. Hence, the cation geothermometer has limitations in estimating the temperature of the reservoir. In this study, the SiO2 geothermometer is selected for the calculation of the geothermal reservoir. The geothermal reservoir temperatures range from 95.22°C to 121.66°C (no steam loss) and 96.85°C–119.57°C (maximum steam loss), respectively, measured using the SiO2 geothermometer. From the Na-K-Mg equilibrium diagram (Figure 6), it can be seen that the four outcrops of the Woka geothermal spring all fall between the 120°C and 200°C isotherms. In addition, the estimated reservoir temperatures based on the SiO2 geothermometer are all lower than this range, indicating that the geothermal water in the basin is mixed by the shallow fractured cold water or surface water in the process of gushing out of the surface.
The above analysis shows that the geothermal springs in the Woka basin have the characteristics of mixing with shallow cold water, and a mixing model can be established to estimate the initial geothermal reservoir temperature in the deep position. The mixing model equation is as follows:
where Sc is the enthalpy of cold water (J/g); Ss is the final enthalpy of spring water (J/g, the enthalpy of saturated water below 100°C is equal to the number of the temperature of water in degree Celsius; above 100°C, the relationship between temperature and enthalpy of saturated water can be found in Table 5); Sh is the initial enthalpy of geothermal water (J/g); ρcSiO2 is the mass concentration of SiO2 of cold water (mg/L); ρsSiO2 is the mass concentration of SiO2 of spring water (mg/L); ρhSiO2 is the initial mass concentration of SiO2 of geothermal water (mg/L); and X is the mixing ratio of cold water.
According to the above equation, the cold water temperature is taken as the local average annual temperature of 8.2°C in Woka, and the cold water SiO2 content is taken as the average value of cold springs in the basin. The initial temperature of geothermal water is assumed to be equal to 75°C–300°C, and the corresponding enthalpy values can be found in Table 6. The temperature at the mouth of the spring and the SiO2 content were based on the measured values. The enthalpy and the mass concentration of SiO2 at each temperature are substituted into Eqs 1, 2, respectively, to find the X1 and X2 values at different temperatures and plot the curve of X and geothermal water temperature at different temperatures (Figure 6), and the intersection point of X1 and X2 curves is the calculated reservoir temperature value.
Figure 7 shows that the reservoir temperature of Kanai geothermal spring is 200°C, and the proportion of cold water mixing is 77%; the reservoir temperature of Woka geothermal spring is 175°C, and the proportion of cold water mixing is 73%; the reservoir temperature of Amaka geothermal spring is 175°C, and the proportion of cold water mixing is 83%; thus, it can be seen that the underground geothermal water system of Woka rift basin is a high-temperature geothermal water system, but it is strongly affected by the mixing of cold water on the surface. The estimated reservoir temperature based on the mixing model is basically consistent with the reservoir temperature range on the Na-K-Mg equilibrium diagram, which can reflect the reservoir temperature value of the subsurface geothermal water system in the Woka graben basin more realistically.
Genetic mechanism
Heat source
According to previous research on high-temperature geothermal systems in Tibet, the heat source of Tibetan geothermal heat is mostly the contribution of the local melt layer in the crust, which is characterized by high conductivity and low gravity anomalies (Zhang, Tan, 2015). The melt bodies are mostly arranged in a string of beads and form extensional faults and fracture zones with linear spreading characteristics on the surface. In addition, they gradually form a fault valley system characterized by a string of bead-like fracture basins in the later geological history, which often becomes the dominant site for underground geothermal water and nurtures many geothermal fields such as Nimu, Yangbajing, and Gulu. Combined with the hydrogeochemical characteristics of the geothermal springs exposed in the Woka graben, it is believed that there is a deep local melt in the Woka graben, which provides a good heat source condition for the underground geothermal water system in the Woka graben basin.
Transport channel
The interlocking fracture system in the Woka basin provides storage space and transport channels for the formation of geothermal water. From the point of view of the development of the geothermal water system, the intersection of fractures in different directions is the dominant part of geothermal water storage, transportation, enrichment, and even discharge and is the area with intensive geothermal water activity. The main heat control structure of the Woka geothermal area can be divided into the northern Regan–Songduo fault zone, the central Mocun fault zone, and the southern Yarlung–Zangbo River tectonic zone. They are distributed in the E-W direction and cut by the N-S direction Cuona–Woka fault. These tectonic intersections constitute the favorable location of the geothermal water system. In particular, the intersection of NS-oriented faults and EW-oriented faults is not only a channel for geothermal water recharging but also a space for geothermal water transportation and storage. Hence, it is the most active part of the geothermal water system, and the existence of a low-resistance zone has a resistivity of less than 100 Ω m, which is approximately 300 m wide, along the geothermal spring outcrop zone of the Zengjiuqu River Valley.
Recharging source
The analysis of hydrogeochemical characteristics shows that the geothermal springs in the study area are mainly recharged by atmospheric precipitation and snow-melting water. The geothermal water is affected by water–rock action and cold water mixing in the circulation process, showing different types of water chemistry. The geothermal springs in the area are mixed with cold water in varying proportions from 73 to 83%. The hydrogen and oxygen isotopic characteristics of the geothermal springs show that the geothermal springs in the area are recharged at elevations from 5193 to 5247 m.
Reservoir affinity
Based on the hydrogeochemical characteristics of the geothermal springs and their reservoir characteristics, the reservoirs in the area can be divided into two types: Quaternary pore and bedrock fracture.
Quaternary pore sediment reservoir
According to the results of physical prospecting, the thickness of the fourth series loose cover layer of Zengjiuqu in the Woka area is 50–150 m. In addition, its lithology is sand and gravel, with a dense sand layer or geothermal water colloid layer as a cover layer locally. In the process of recharge and runoff, the geothermal water gushes out along the buried Woka fault developed in the basement of the riverbed or the boundary faults on both sides of the Woka rift basin. Then, geothermal water enters into the pore space of the Quaternary loose sediments with good permeability along the valley of Zengjiuqu, forming a good Quaternary pore sediment reservoir.
The outcrop of geothermal water in this geothermal reservoir is mainly a warm water swamp. In addition, the outcrop along Zengjiuqu is wide. The closer distance to the surface water is, the more obvious the mixing effect is, and the temperature is lower. However, the borehole can reveal underground geothermal water with a higher temperature than the natural outcrop geothermal spring.
Bedrock fracture type reservoir
This reservoir is mainly distributed in the bedrock on both sides of Zengjiuqu, and its aquifer is composed of Cretaceous–Paleocene granite intrusive rocks, which are influenced by active faults, and the tectonic fissures are developed, with rich water reserve. The development of tectonic fissures is not only an advantageous channel for geothermal water transportation but also an excellent space for geothermal water storage. Most of the geothermal springs flow along the fissures in granite, which is the main reservoir of geothermal water in the area.
Conceptual model for geothermal system in the Woka graben
Because of the continuous and strong plate collision activities of the Eurasian plate, intensive and frequent structural deformation has been formed on the Tibetan Plateau. It creates a deep melt-type magma source area in the lower crust and a local low-velocity melt layer in the middle and forms shallow intrusive magma capsules and local banded melt bodies at different depths within a certain depth in the upper crust, providing the necessary heat source for the formation of the underground geothermal water system on the Tibetan Plateau. Therefore, the Tibetan Plateau geothermal resources are mostly uplifted mountain convection type. The conceptual model of the subsurface geothermal water system within the Woka graben basin was established by synthesizing the research results of many geothermal fields in the Tibetan region, such as Yangpaijing and Nimu-Naqu geothermal fields (Figure 8).
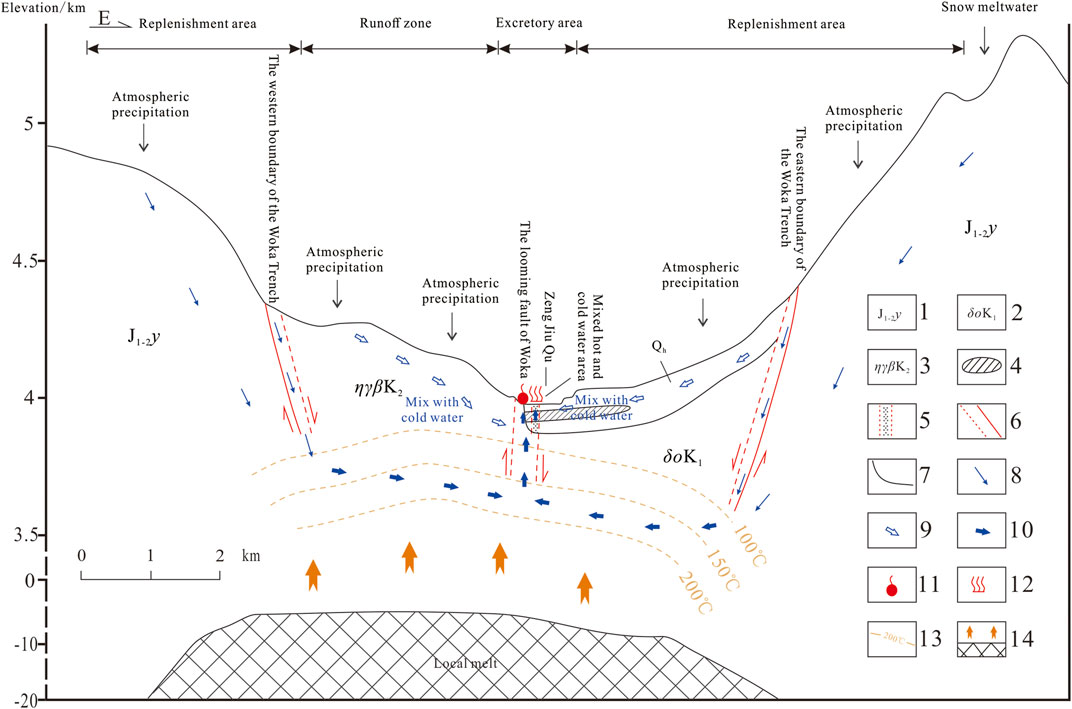
FIGURE 8. Genetic model of geothermal waters in the Woka Graben basin. 1-Middle to Lower Jurassic Yeba formation; 2-Lower Cretaceous quartz diorite; 3-Middle Cretaceous monzogranite;4-Tight sand cover; 5-Channel of Quaternary thermal reservoir; 6-Measured or inferred fault; 7‐Geological boundary; 8‐Cold water and transport direction; 9‐Mixed cold water and its migration direction; 10‐Geothermal water and flow direction; 11‐Geothermal spring; 12‐Warm water swamp; 13‐Inferred temperature contour; 14‐Heat source.
The atmospheric precipitation and alpine ice and snow melt water on both sides of the Woka graben basin are the main recharge sources of geothermal water, and the cold water receiving the recharge keeps seeping down along the fractures developed by the tectonic activity. In addition, cold water is heated by the local strip melt at a certain depth to provide a heat source and thus forms geothermal water involved with deep gas components. Then, geothermal water begins to flow upward, controlled by the fault-controlled bedrock fracture type reservoir. During the ascent of geothermal water along the river valley or the basin boundary fractures, its temperature decreases because of the mixing of the shallow cold water. In Zengjiuqu River valley, geothermal water enters into the pore aquifer of the fourth system loose rock type with good permeability along the bedrock fracture reservoir. In addition, the dense sand layer or geothermal water cementation layer is used as the cover layer, forming the Quaternary pore type shallow reservoir, which is mixed with the surface water during the rising of geothermal water and forms a warm water swamp along the riverbed.
The effect of high-temperature geothermal water on underground construction
The Woka graben is adjacent to Mochukongka Qu in the north and reaches the valley of Yarlung Tsangpo River in the south, which is an essential place for the Sichuan–Tibet railway line. Because of the construction of the Lhasa–Linzhi railway, many major projects such as hydropower and road construction are further planned in the area. According to the above analysis, the geological structure of Woka graben is complex, with active fracture development and strong hydrothermal activity, and its deep reservoir temperature can reach more than 120°C. Hence, it belongs to the high- and medium-temperature geothermal system. Engineering construction will be affected by the enrichment of geothermal resources.
As for the engineering thermal damage, the water temperature of the surface outcrop in the Woka rift valley is 36.25°C–57.77°C, and the calculated reservoir temperature is 97.86°C–125.56°C. The water temperature in the deep or good geothermal channels will be even higher. After the project exposure, there will be a large flow of high-temperature geothermal water caused by the surge water disaster; the sulfate content of geothermal water in the graben is 94.4–147 mg/L, and toxic and harmful gases such as H2S will be escaped after the project exposure. Therefore, the construction of the project in the geothermal development area of the rift valley will pose a great threat to the safety of construction workers because of the high-temperature geothermal water and harmful gases, leading to a significant increase in project costs.
As for the corrosiveness of the project, the pH value of the geothermal water in the rift valley is 6.71–8.25, the HCO3− content is 31–214 mg/L, the Mg2+ content is 0.026–7.85 mg/L, and the SO42− content is 94.4–147 mg/L, which is not corrosive to the project. However, the Cl− ion content of the Luobusa geothermal spring is 4060 mg/L, which is more than 1000 mg/L. It is a corrosive hazard to the tunneling equipment as well as the tunneling facilities, which must be considered in tunnel construction. Therefore, for major construction projects in the Woka graben, a detailed investigation should be conducted to avoid the geothermal water channel or to divert the geothermal water and reasonably arrange the construction sequence to overcome the problem.
Conclusion
(1) The geothermal water system in the Woka graben belongs to the medium- to high-temperature geothermal system, and the geothermal heat shows a belt-like distribution along the Woka semiburied fracture. The geothermal water is medium alkaline water with a TDS concentration of 150.8–744 mg/L. The hydrochemical types are mainly SO4-Na type, SO4-Cl-Na type, and HCO3-SO4-Na type.
(2) The hydrogeochemical characteristics show that the Woka geothermal water system has the characteristics of atmospheric precipitation recharge and shallow circulating groundwater and is subject to the mixing effect of fracture diving or surface water. In addition, the geothermal water is in the range of partial equilibrium or mixed water and immature water on the Na-K-Mg equilibrium diagram.
(3) The reservoir temperatures range from 97.86°C to 125.56°C, estimated using a silica geothermometer. The geothermal water was subject to the mixing of shallow fissure cold water or surface water. By establishing the reservoir temperature mixing model, the initial reservoir temperature of geothermal water and the proportion of mixed cold water are determined. The temperature range of shallow and deep geothermal reservoirs is from 96.85°C to 119.57°C and from 120°C to 200°C, respectively, and the proportion of mixed cold water is 73–83%.
(4) Based on the isotope elevation effect characteristics, the recharge elevations of the geothermal water system in the Woka graben basin are from 5193 to 5247 m, mainly recharged by atmospheric rainfall and alpine snow-melting water, and the geothermal water comes from the release of mantle-sourced CO2 from deep regional faults.
(5) Some geothermal waters possess high Cl− concentrations, leading to the corrosiveness of the project. For major construction projects in the Woka graben, a detailed investigation should be conducted to avoid the geothermal water channel or to divert the geothermal water and reasonably arrange the construction sequence to overcome the problem.
Data availability statement
The original contributions presented in the study are included in the article/Supplementary Material; further inquiries can be directed to the corresponding author.
Author contributions
WZ: Investigation, data curation, and writing—original draft. SW: Data curation and methodology. MX: Writing—review and editing and conceptualization.
Conflict of interest
SW is employed by the Sichuan Institute of Geological Engineering Investigation Group Co., Ltd.
The remaining authors declare that the research was conducted in the absence of any commercial or financial relationships that could be construed as a potential conflict of interest. The reviewer YL declared a shared affiliation with the author WZ to the handling editor at the time of review.
Publisher’s note
All claims expressed in this article are solely those of the authors and do not necessarily represent those of their affiliated organizations, or those of the publisher, the editors, and the reviewers. Any product that may be evaluated in this article, or claim that may be made by its manufacturer, is not guaranteed or endorsed by the publisher.
Supplementary material
The Supplementary Material for this article can be found online at: https://www.frontiersin.org/articles/10.3389/feart.2022.895884/full#supplementary-material
References
Cao, H-W., Li, G-M., Zhang, R-Q., Zhang, Y-H., Zhang, L-K., Dai, Z-W., et al. (2021). Genesis of the Cuonadong tin polymetallic deposit in the tethyan himalaya: evidence from geology, geochronology, fluid inclusions and multiple isotopes. Gondwana Res. 92, 72–101. doi:10.1016/j.gr.2020.12.020
Cao, H-W., Li, G-M., Zhang, Z., Zhang, L-K., Dong, S-L., Xia, X-B., et al. (2020). Miocene Sn polymetallic mineralization in the tethyan himalaya, southeastern tibet: a case study of the cuonadong deposit. Ore Geol. Rev. 119, 103403. doi:10.1016/j.oregeorev.2020.103403
Cao, H-W., Zhang, Y-H., Santosh, M., Li, G-M., Hollis, S. P., Zhang, L-K., et al. (2019). Petrogenesis and metallogenic implications of cretaceous magmatism in central lhasa, tibetan plateau: a case study from the lunggar Fe skarn deposit and perspective review. Geol. J. 54, 2323–2346. doi:10.1002/gj.3299
Chang, X-W., Xu, M., Jiang, L-W., Li, X., and Zhang, Y-H. (2021). Hydrogeochemical characteristics and formation of low-temperature geothermal waters in mangbang-longling area of western yunnan, China. J. Chem. 2021, 1–13. doi:10.1155/2021/5527354
Elenga, H. I., Tan, H., Su, J., and Yang, J. (2021). Origin of the enrichment of B and alkali metal elements in the geothermal water in the Tibetan Plateau: Evidence from B and Sr isotopes. Geochemistry 81, 125797. doi:10.1016/j.chemer.2021.125797
Fournier, R. (1977). Chemical geothermometers and mixing models for geothermal systems. Geothermics 5, 41–50. doi:10.1016/0375-6505(77)90007-4
Fournier, R. O. (1979). Geochemical and hydrologic considerations and the use of enthalpy-chloride diagrams in the prediction of underground conditions in hot-spring systems. J. Volcanol. Geotherm. Res. 5, 1–16. doi:10.1016/0377-0273(79)90029-5
Guo, Q. (2012). Hydrogeochemistry of high-temperature geothermal systems in China: a review. Appl. Geochem. 27, 1887–1898. doi:10.1016/j.apgeochem.2012.07.006
Jiang, Z., Xu, T., Owen, D. D. R., Jia, X., Feng, B., Zhang, Y., et al. (2018). Geothermal fluid circulation in the Guide Basin of the northeastern tibetan Pplateau: isotopic analysis and numerical modeling. Geothermics 71, 234–244. doi:10.1016/j.geothermics.2017.10.007
Klemperer, S. L., Zhao, P., Whyte, C. J., Darrah, T. H., Crossey, L. J., Karlstrom, K. E., et al. (2022). Limited underthrusting of India below Tibet: (3)He/(4)He analysis of thermal springs locates the mantle suture in continental collision. Proc. Natl. Acad. Sci. U. S. A. 119, e2113877119. doi:10.1073/pnas.2113877119
Kong, Y., Pang, Z., Shao, H., Hu, S., and Kolditz, O. (2014). Recent studies on hydrothermal systems in China: a review. Geotherm. Energy 2, 19. doi:10.1186/s40517-014-0019-8
Li, X., Huang, X., Liao, X., and Zhang, Y. (2020). Hydrogeochemical characteristics and conceptual model of the geothermal waters in the xianshuihe fault zone, southwestern china. Int. J. Environ. Res. Public Health 17, 500. doi:10.3390/ijerph17020500
Li, X., Qi, J., Yi, L., Xu, M., Zhang, X., Zhang, Q., et al. (2021). Hydrochemical characteristics and evolution of geothermal waters in the eastern himalayan syntaxis geothermal field, southern tibet. Geothermics 97, 102233. doi:10.1016/j.geothermics.2021.102233
Liao, Z. (2018). Hot springs in southwestern China. Therm. Springs Geotherm. Energy Qinghai-Tibetan Plateau Surroundings chapter 6, 79–163. doi:10.1007/978-981-10-3485-5_6
Tan, H., Su, J., Xu, P., Dong, T., and Elenga, H. I. (2018). Enrichment mechanism of Li, B and K in the geothermal water and associated deposits from the kawu area of the tibetan plateau: constraints from geochemical experimental data. Appl. Geochem. 93, 60–68. doi:10.1016/j.apgeochem.2018.04.001
Tian, J., Pang, Z., Liao, D., and Zhou, X. (2021). Fluid geochemistry and its implications on the role of deep faults in the genesis of high temperature systems in the eastern edge of the Qinghai Tibet Plateau. Appl. Geochem. 131, 105036. doi:10.1016/j.apgeochem.2021.105036
Truesdell, A., and Fournier, R. (1977). Procedure for estimating the temperature of a hot-water component in a mixed water by using a plot of dissolved silica versus enthalpy. USGS J. Res. 5, 49–52.
Wang, P., Chen, X., Shen, L., Wu, K., Huang, M., Xiao, Q., et al. (2016). Geochemical features of the geothermal fluids from the mapamyum non-volcanic geothermal system (Western Tibet, China). J. Volcanol. Geotherm. Res. 320, 29–39. doi:10.1016/j.jvolgeores.2016.04.002
Wang, Y., Gu, H., Li, D., Lyu, M., Lu, L., Zuo, Y., et al. (2021). Hydrochemical characteristics and Genesis analysis of geothermal fluid in the zhaxikang geothermal field in cuona county, southern tibet. Environ. Earth Sci. 80, 415. doi:10.1007/s12665-021-09577-8
Wang, Y., Li, L., Wen, H., and Hao, Y. (2022). Geochemical evidence for the nonexistence of supercritical geothermal fluids at the yangbajing geothermal field, southern tibet. J. Hydrology 604, 127243. doi:10.1016/j.jhydrol.2021.127243
Wang, Y., Zheng, C., and Ma, R. (2018). Review: safe and sustainable groundwater supply in China. Hydrogeol. J. 26, 1301–1324. doi:10.1007/s10040-018-1795-1
Zhang, W., Tan, H., Zhang, Y., Wei, H., and Dong, T. (2015). Boron geochemistry from some typical tibetan hydrothermal systems: origin and isotopic fractionation. Appl. Geochem. 63, 436–445. doi:10.1016/j.apgeochem.2015.10.006
Zhang, X., and Hu, Q. (2018). Development of geothermal resources in China: a review. J. Earth Sci. 29, 452–467. doi:10.1007/s12583-018-0838-9
Zhang, Y., Xu, M., Li, X., Qi, J., Zhang, Q., Guo, J., et al. (2018). Hydrochemical characteristics and multivariate statistical analysis of natural water system: a case study in kangding county, southwestern China. Water 10, 80. doi:10.3390/w10010080
Zhang, Y., Cao, H., Hollis, S., Tang, L., Xu, M., Jiang, J., et al. (2019a). Geochronology, geochemistry and Sr-Nd-Pb-Hf isotopes of the early paleogene gabbro and granite from central lhasa, southern tibet: petrogenesis and tectonic implications. Int. Geol. Rev. 61, 868–894. doi:10.1080/00206814.2018.1476187
Zhang, Y., Wang, Y., Wang, W., Liu, J., and Yuan, L. (2019b). Zircon U-Pb-Hf isotopes and mineral chemistry of early cretaceous granodiorite in the lunggar iron deposit in central Lhasa, Tibet Y, China. J. Central South Univ. 26, 3457–3469. doi:10.1007/s11771-019-4266-5
Keywords: formation mechanism, high-temperature geothermal system, hydrochemical characteristics, D-O-C isotopes, engineering influence
Citation: Zhang W, Xu M and Wu S (2022) Study on the genetic mechanism of high-temperature geothermal system and its engineering impact in the Woka graben, Tibet. Front. Earth Sci. 10:895884. doi: 10.3389/feart.2022.895884
Received: 14 March 2022; Accepted: 11 July 2022;
Published: 10 August 2022.
Edited by:
Xiaoyan Zhao, Southwest Jiaotong University, ChinaReviewed by:
Zhao Liu, Hebei GEO University, ChinaYanyan Li, Chinese Academy of Geological Sciences (CAGS), China
Copyright © 2022 Zhang, Xu and Wu. This is an open-access article distributed under the terms of the Creative Commons Attribution License (CC BY). The use, distribution or reproduction in other forums is permitted, provided the original author(s) and the copyright owner(s) are credited and that the original publication in this journal is cited, in accordance with accepted academic practice. No use, distribution or reproduction is permitted which does not comply with these terms.
*Correspondence: Mo Xu, xm@cdut.edu.cn