- 1State Key Laboratory of Geohazard Prevention and Geoenvironment Protection, Chengdu University of Technology, Chengdu, China
- 2Sichuan Water Conservancy Vocational College, Chengdu, China
- 3Power China Chengdu Engineering Corporation Limited, Chengdu, China
The distribution, scale, and engineering geological characteristics of riverbed overburden have become one of the key issues in the construction of water conservancy and hydropower engineering projects in southwest China. In this study, we summarize and discuss the (variation of thickness) distribution and the geogenic (formation) mechanisms of riverbed overburden in the associated rivers. This was done by compiling thickness data from constructed and planned dams. The results show that the overburden thickness is generally shallower in the upper Tibetan Plateau region, it is thickest in the marginal mountain region in the middle reaches, and shallower in the lower reaches of the mountain regions that are in contact with the Yunnan-Guizhou Plateau or Sichuan Basin. This holds true with the shallow-thick-shallow Distribution Law. Additionally, the river overburden has the characteristic of thickening gradually from the basin edge to the plateau slope. Through the genesis, source, and distribution of the aggradation deposits in the riverbed, the geogenic (formation) mechanisms of the river overburden layer is explored, and the coupling effect of tectonic-climatic-fluvial sedimentation processes on the variation of overburden thickness and spatial distribution is proposed. Finally, the geological problems encountered when engineering dams in thick overburden are analyzed, and common engineering measures are put forward. The results provide basic data support for water resources exploitation and further development of river engineering in Southwest China.
1 Introduction
The thickness of riverbed overburden poses great challenges to the construction of hydropower projects in river basins, thus it is of great theoretical value and engineering significance to study the distribution law and the geogenic (formation) mechanisms of riverbed overburden layer thickness. As is well known, rivers transport soil from the hillside to tributaries, to the main stem, and then from the main stem to the lowland, especially in mountainous areas (Fabrizio et al., 2018). The riverbeds formed are a variety of loose deposits closely related to the topography and geomorphology, parent rock lithology, geological structure, neotectonic movement, and climate environments. The formation types are complicated and its lithofacies and thickness vary greatly.
Many researchers have analyzed the exploration and experimental data of dam sites, and used numerical simulations and physical models to study riverbed overburden from the aspects of the formation mechanisms, distribution law, engineering characteristics, exploration, anti-seepage technology, and related engineering geological problems (foundation bearing capacity, seepage deformation, uneven settlement, sand liquefaction, etc.) (Hedayati Talouki et al., 2015; Michael, 2018; Luo and Huang, 2020; Yu and Shao, 2020; Zhang et al., 2021). The thickness variation of riverbed overburden has the obvious characteristics of basin and region, and there are many new achievements in the study of riverbed overburden from the dam site areas, especially in the section of the riverbed engineering area to the main stem and tributaries of rivers (Shi, 1986; Wang Y. S. et al., 2007; Xu et al., 2008; Wang, 2009; Xu et al., 2010; Yu and Shao, 2020; Yu and Shao, 2020).According to borehole data from construction sites of hydropower stations, the thickness variation of riverbed overburden from the top of Tiger Leaping Gorge to Xiakou is thinner in the upper and lower reaches of the Jinsha River, and deepest in the middle reaches (Hongyan Dam site thickness of 250 m) (Wang., 2009). The deposit layer in the Yalong river bed is often over 30 m thick and can be up to 51 m thick (Wang L. S. et al., 2007).The thickness of the riverbed overburden increases from the lower reaches to the upper reaches of the Dadu River, and suddenly decreases in the Dagangshan area of the middle reaches, and the longitudinal section of the main stem bed cover forms a “W” shape (Xu et al., 2010).The riverbed overburden of the Minjiang River appears thick in the upper reaches and thin in the lower reaches (Wang et al., 2005).Research on the distribution and geogenic mechanisms of riverbed overburden in southwest China is mainly focused on a single dam site or individual river sections, meanwhile there is less systematic research on the thickness variation of riverbed overburden in the primary rivers of Southwest China. These rivers include the Jinsha, Tsangpo, Lancang, Nujiang, etc.
Parts of the previously mentioned rivers are in the slope zone transition from the Qinghai-Tibet Plateau to the Yunnan-Guizhou Plateau and the Sichuan Basin. This is one of the regions with the most abundant water resources and also the most developed deep riverbed overburden. As for the origins of the overburden, there are many opinions, but no agreement has been reached yet. Xu et al. (2010) proposed that global climate change and eustacy (sea level rise and fall) cause the thick overburden of river valleys. Wang et al. (2005) proposed that earthquakes, heavy rains, and other disasters induce mountain collapse, landslide and debris flow, resulting in river blockage and other phenomena where the landslide front is directly deposited on the early overburden, resulting in local accumulation of deep overburden. Wang et al. (2006) proposed that during the interglacial period of the last glacial max, the river valley was strongly eroded and cut into the basal layer below the present riverbed, and then rapidly backfilled to form the deep overburdenlayer. Ding et al. (2021) investigated the 26.5 m-thick lakebed profile at the bottom of Tongzilin Dam in the Yalong River and proposed that the formation of the overburden was the result of the combined action of climate fluctuations and strong tectonic activity (local tectonic subsidence). Bridgland (2000) and Maddy et al. (2001) proposed that the reasons affecting the development of fluvial geomorphology (including riverbed cover) can be summarized as dynamic changes within the fluvial system and non-fluvial origins which are based on the geological records of the river terraces of the Thames River in the United Kingdom during the Middle Pleistocene. By considering ice dynamics, bedrock erosion, and sediment transport processes, Delaney and Adhikari (2020) simulated the sediment discharge of alpine glaciers that experienced 100 years of accelerated glacier melting, and found that during the glacier retreat sediment emissions are likely to increase significantly, and depending on the amount of sediment stored under the ice, there are additional effects based on the different glacial topography and bed roughness. Alexey et al. (2019) studied riverbed deposition of rivers in the Russian Far East under extreme rainfall conditions and found that the upper reaches of secondary tributaries and part of the estuaries of large tributaries were areas with increased deposition. Syvitski et al. (2022) reviewed that Global warming is also substantially affecting the global sediment cycle through temperature impacts (sediment production and transport, sea ice cover, glacial ice ablation and loss of permafrost), precipitation changes, desertification and wind intensities, forest fire extent and intensity, and acceleration of sea-level rise. Humans have transformed the mobilization, transport and sequestration of sediment, to the point where human action now dominates these fluxes at the global scale as seen in data from 1950 to 2010. Summarizing previous studies, it is shown that the formation of the riverbed overburden is related to several factors, such as tectonic movement, climate change, sedimentary law, geological environment, and geological disasters. However, the origin of the regional deep overburden in the primary rivers of southwest China may be more complex and diverse.
As for the classification of overburden thickness, most researchers demarcate 30 m as the boundary of thick overburden. The code for engineering geological investigation of water resources and hydropower in China (GB50487-2008) stipulates that the thickness greater than 40 m of overburden layer of is considered thick. This study adopts three categories according to the specification, namely the shallow layer (< 40 m), a deep overburden layer (40–100 m), and giant-thick overburden layer (> 100 m). For clarity the terms “thick and thin” or “thickness” is used when regarding the vertical height of the overburden layer(s) and the above terms are used when distinguishing the impact on hydrological engineering projects as shown in Table 1.
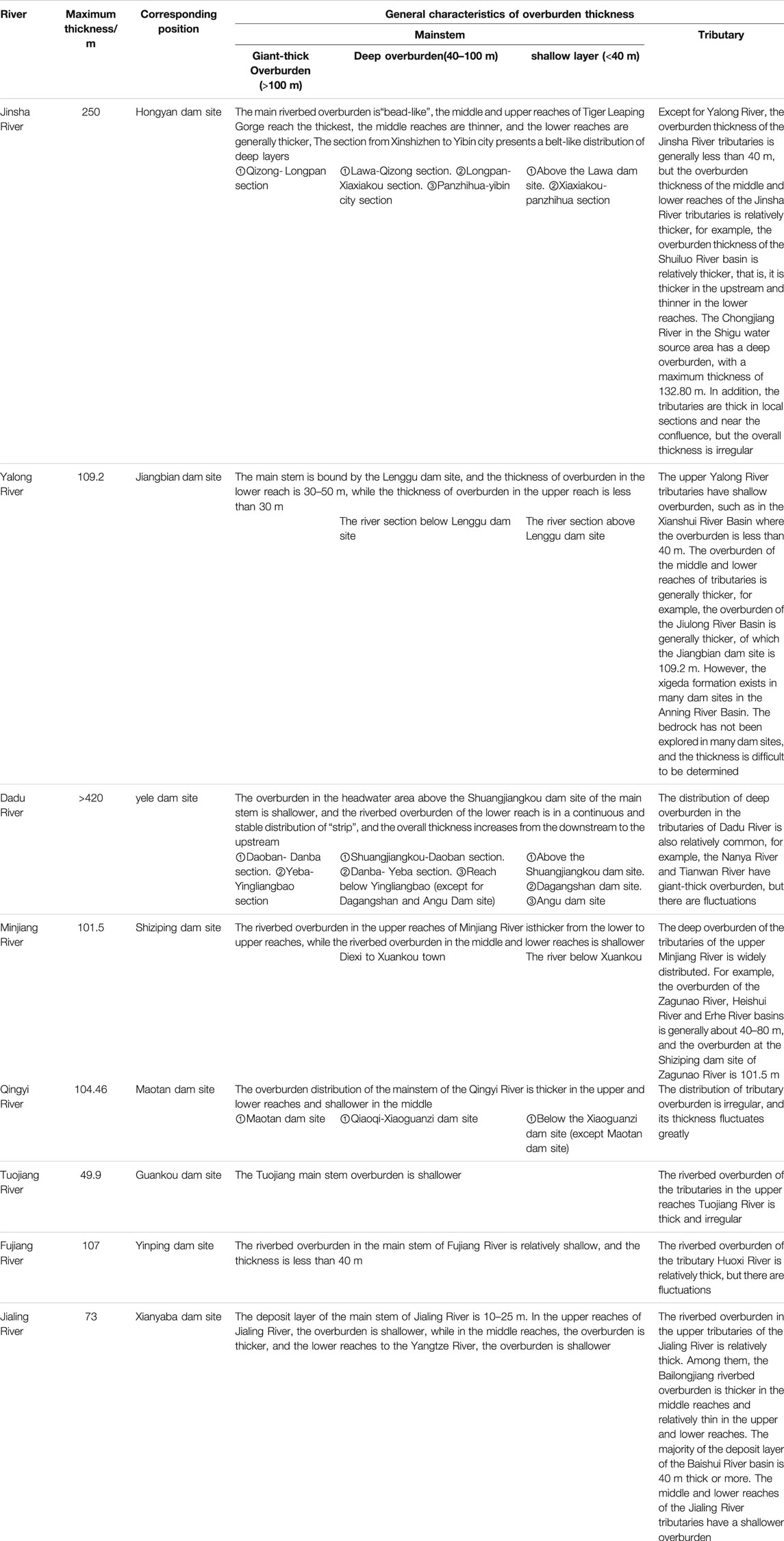
TABLE 1. The riverbed overburden thickness characteristic table in the upper Reaches of the Yangtze River.
Based on previous work, this paper systematically summarizes and explores the distribution characteristics and geogenic mechanism of riverbed overburden in major rivers of Southwest China by grading and analyzing many of the boreholes related to already built or planned dams (sluices). This will provide engineering experience, reference, and basic data support for the hydropower cascade planning, development, and site selection of river channel projects in Southwest China.
2 Riverbed Overburden Depth Distribution Characteristics
By summarizing the overburden thickness and composition data of 632 dams (sluices) that have been built or planned in Southwest China, the thickness variation of overburden in each major river basin is as follows: the Yellow River (Reach above Lanzhou) (61), the Jialing River (69), the Fujiang River (28), and the Tuojiang River (6), the Minjiang River (56), the Qingyi River (22), the Dadu River (75), the Yalong River (89), the Jinsha River (104), the Lancang River (29), the Nujiang River (36), the Lixian River (9), the Irrawaddy River (9), the Nanpan River (9) and the Yuanjiang River (4), the Yarlung Tsangpo river (26), etc. The distribution is shown in Figure 2.
2.1 Main Riverbed Overburden Thickness Variation Characteristics
2.1.1 The Upper Reaches of the Yangtze River
The upper reaches of the Yangtze River at the Jinsha River and its important tributaries such as the Yalong, Dadu, and Min Rivers have deep riverbed overburden (Figure 1), with diverse lithofacies and complex structures. In the upper reaches of the Yangtze River (from Yibin to Chongqing) and its tributaries, such as the Tuojiang and Jialing Rivers, the riverbed overburden is mainly composed of a single sand and gravel layer of small thickness (Table 1).
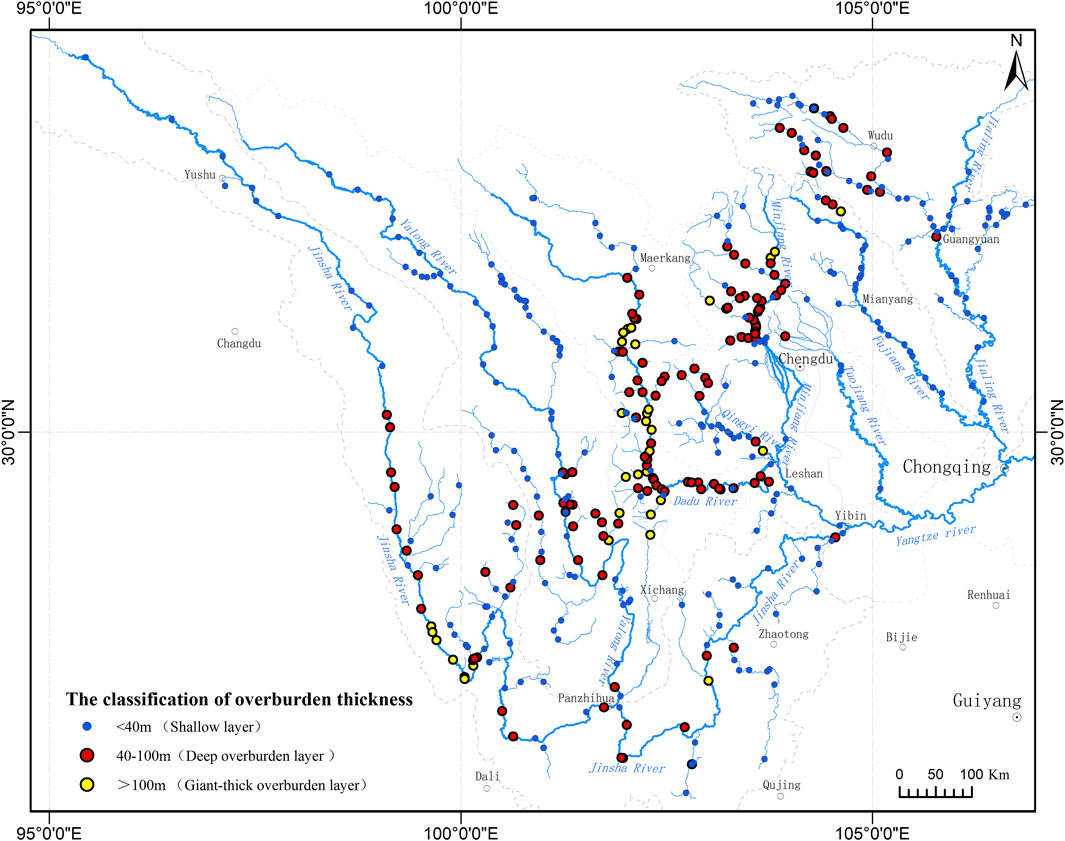
FIGURE 1. Distribution of maximum thickness of riverbed overburden in the upper reaches of the Yangtze River.
2.1.2 Nujiang River
The riverbed overburden in the Nujiang River Basin is relatively shallow. It is generally 20–40 m thick. However, the thickness of the riverbed overburden at the Songta dam site is 58–70 m. Above the Songta dam site the riverbed overburden thickness is 10–15 m. In some cases however (between the upper and lower reaches) there are almost no deposits, and the riverbed is bedrock. From the Songta dam site to the Saige dam site, the overburden layer is 25–40 m. Downstream of the Songta dam the overburden is 10–15 m. The overall thickness is shallow in the upstream and downstream portions and deep in the middle (Figure 2).
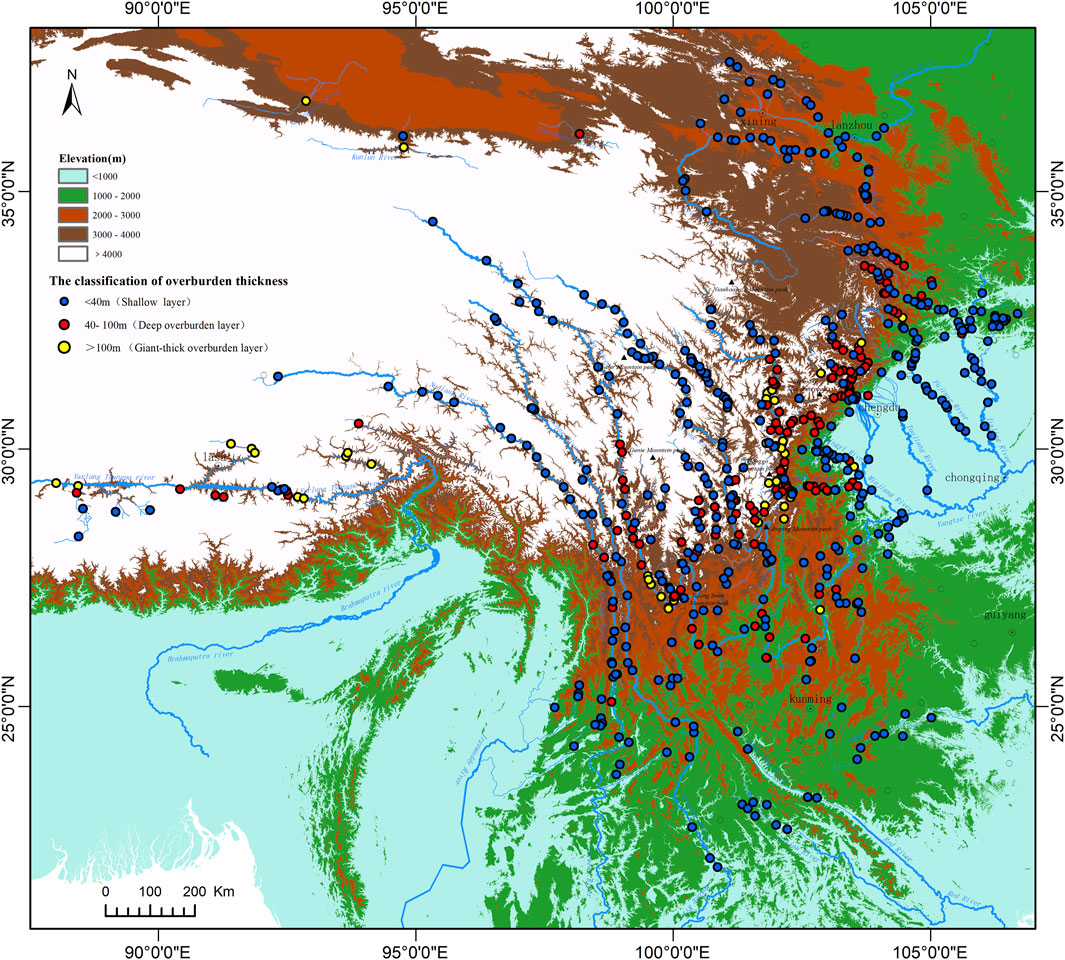
FIGURE 2. Spatial distribution of maximum thickness of riverbed overburdens of main rivers in southwest China.
2.1.3 Lancang River
The overburden in the main stem of the Lancang River between the upper and lower reaches has a consistent thickness of between 20 and 30 m. Analyzing the overburden thicknesses based on longitudinal distribution; the upstream is thinner, the middle is thicker, and the downstream is thinner. The tributaries to the Lancang have relatively thin overburden layers (Figure 2).
2.1.4 Yellow River (Above Lanzhou)
The Yellow River basin (above Lanzhou) has a relatively thin overburden thickness. The main stem riverbed overburden is 2–26 m thick and mainly composed of pebbles and gravel. The overburden of the tributary Tao and Huangshui Rivers are less than 40 m, except at the Jiudianxia dam site in the Tao River where it is 56 m. The overburden of the Tao River is thicker in the upper reaches and shallower in the middle and lower reaches.
2.1.5 Yarlung Tsangpo River
The thickness of the overburden in the middle and upper reaches of the Yarlung Tsangpo River can reach more than 120 m while the wide valley area in the lower reaches may reach hundreds of meters. The maximum thickness of the overburden in the Niyang and Lhasa Rivers is more than 100 and 400 m respectively. At the Qushui Bridge location on the Yarlung Zangbo (Tsangpo?) River in Zedang, the thickness of riverbed overburden is more than 50 m, and in the Lhasa area, the thickness of riverbed overburden is 123 m. The maximum thickness of the sand and gravel layers in the adjacent ancient riverbed as measured by electromagnetic exploration is 600 m (Yu and Shao, 2020).
2.1.6 Other River(s)
For other river basins, such as the Lixianjiang, Irrawaddy, Nanpanjiang, Yuanjiang, and others on the Yunnan-Guizhou Plateau, the overburden of the riverbeds are predominately considered shallow (<40 m). More specifically the relative overburden of the Lixianjiang and Irrawaddy are shallow, while the Nanpanjiang and Yuanjiang are thicker in comparison.
2.2 Regional Thickness Variation Characteristics
Summarizing the exploration data related to riverbed overburden; the results show that the distribution of deep riverbed overburden presents regional characteristics. According to the characteristics of topography, terrain, and the climate of the basin, they can be roughly divided into the Tibetan plateau area, marginal alpine valley area, Piedmont or marginal mountainous plain area, Yunnan-Guizhou Plateau area, and the Sichuan Basin area.
The riverbed overburden is the thickest in the alpine valley areas at the margin of the Tibetan Plateau, namely the Longmen and Hengduan Mountain areas, and it is of a consistent thickness along the following mountain ranges: Xuebaoding—Siguniang Mountain—Gongga Mountain—Jinping Mountain—Yulong Snow Mountain—Meili Snow Mountain. The contact zone between the mountains and the Yunnan-Guizhou Plateau, and the Piedmont area of the contact zone between the mountains and the Sichuan Basin has an overburden that is thicker than the mountain range in the previous example, however, the thickness of the overburden is greater in the upper river valley than in the lower river valley where it runs into the basin. The Jialing, the Tuojiang, the Fujiang, the upper reaches of the Minjiang, and the Qingyi Rivers all show the thickest overburden in the Longmenshan section. The overburden becomes thinner after it enters the Sichuan Basin. The riverbed overburden in the Yunnan-Guizhou Plateau and Sichuan basins is relatively shallow. When the Jialing, Fujiang, Tuojiang, Minjiang and other associated rivers enter the Sichuan Basin the overburden becomes thinner. Additionally, the riverbed overburden of the Li, Yuan, and Nanpan Rivers in the Yunnan-Guizhou Plateau is generally less than 40 m. For example, the thickness of the riverbed overburden of the Nanpan River is 15–25 m. After the Nujiang, Irrawaddy, and Lancang Rivers flow into Myanmar and Laos, the riverbed overburdens are generally thinner. Finally, the riverbed overburden is not uniform across the inner part of the Tibetan Plateau, but it is thinnest when it reaches the periphery. For example, the eastern margin of the Tibetan Plateau is the headwaters area of the Nujiang, Lancang, Jinsha, and other major rivers and the thickness of the riverbed overburden is predominantly less than 20 m. Additionally the riverbed overburden in the basins above Lanzhou on the Yellow River is shallower. However, the main stem of the Yarlung Tsangpo River and its tributaries, the Lhasa, and Nianchu Rivers all have a riverbed overburden that is more than 100 m in most areas. Interestingly the overburden tends to decrease near the Himalayan mountains.
In summary, the main rivers in Southwest China generally have deep riverbed overburden, where the thickness is generally between 10 and 100—m, local sections can reach into the hundreds of meters. Generally, the thickness of riverbed overburden is shallower in the upper reaches of the Tibetan Plateau, thickest in the marginal mountain areas of the middle reaches, and shallower in the contact areas of the lower reaches. The Yunnan-Guizhou Plateau and Sichuan Basin show a shallow-thick-shallow distribution pattern. Overall, it has the characteristics of thickening gradually from the basin edge to the plateau slope, and the river overburden also has the characteristics of thickening from the downstream to the upstream (Figure 2).
3 Preliminary Study on Formation Mechanisms of Riverbed Overburden
The formation types of riverbed overburden are complex. Different rivers, different sections of the same river, and even different time periods of the same river section may have different causes (Luo, 1995). Based on the engineering geological data, this paper explores the distribution characteristics of the existing riverbed overburden, and then analyzes the formation mechanisms of the riverbed overburden in the primary rivers of Southwest China.
3.1 Characteristics of Overburden Material Composition
The main sources of riverbed overburden in Southwest China are mainly alluvial-proluvial deposits, glacial (glaciofluvial, moraine) deposits, dammed lake deposition, and landslide and collapse deposits.
3.1.1 Alluvial-Proluvial Deposits
The alluvial-proluvial sediments in riverbeds in Southwest China can be classified as coarse as boulders or fine to sand (and loess?). The dominant species is gravel that is mainly composed of drift pebbles intercalated with sand, others are gravelly sand and sandy structure with good sorting and roundness. In addition, the sand is usually lenticular in the horizontal or has an oblique laminar distribution. The bed of the mountain river is narrow, the lateral swing is limited, the floodplain is not developed, and downcutting is obvious. The normal thickness of alluvial-proluvial deposits is generally less than 30 m (Xu et al., 2008).
Alluvial-proluvial deposits are mainly distributed in the upper and lower portions of the deep and huge thick overburden reaches. The bottom layer is partially cemented and generally unable to move. The deposits are widely distributed in riverbeds and terraces.
3.1.2 Glacial Deposits
(1) Characteristics
Glacial deposits are one of the most important quaternary deposits in northwest Yunnan, Western Sichuan, and Tibet. Glacial moraines and glaciofluvial deposits are the major contributors to the overburden layers. The glacial deposits in China almost all belong to giant-grained or boulder soil and crushed stone soil. The glacial deposits lack intermediate grain size (or discontinuous deposits), and the lithology mainly depends on the lithology of local parent rocks (Zhang et al., 2009).
(2) Distribution range
Glacial deposits mainly exist in riverbank slopes and terrace zones. With the development of hydropower and accurate drilling data of the riverbed, there is a range of glacial deposits differing in thickness at the bottom of some riverbeds.
The glacial deposits found in the studied riverbeds are distributed into separate layers. This distribution varies from the central Longmen mountains to the Hengduan mountains. Specifically, the glacial deposits were distributed in different periods in the mountains between Minshan to Mangkang and the Yunling river tributaries, and around the Yulong Mountains, Haba Mountains, White snow Mountains, Gongga Mountains, Siguniang mountains and other high mountains. The deposits are oriented around the river tributaries of the riverbeds and valley terraces. For example, there are several glacial deposits in the Minjiang and Qingyi rivers around Siguniang Mountain. The glacial deposits in the Minjiang River are mainly distributed in the upper reaches of the main stem, the tributary Erhe River, and the upper reaches of the Zagunao River. This is especially noted in the northeast and east slopes of Siguniang Mountain. Glacial deposits in the Qingyi River are distributed in the upper east river basin, in the southwest slope of Siguniang Mountain and the southeast slope of Jiajinshan Mountain.
Glacial deposits in the Dadu River around the Gongga Mountains are distributed in the middle and upper reaches of the river. The main stem is mainly distributed in the reaches between the Qionglai Mountains and the Daxueshan Mountains. Glacial deposits in the tributaries are mainly distributed in the upper reaches of the Nanya River, the upper reaches of the Tianwan River, the lower reaches of the Yanzigou River, the whole basin of the Wasihe River and the Geshizahe River, etc.
Glacial deposits in the Yalong River around Saneiri are distributed in the Litang River section on the eastern slope of the Shaluli Mountains. The glacial deposits of the Jinsha River near the Yulong Mountains are distributed in the upper reaches between the Shaluli Mountains and the Mangkang-Yunling Mountains and the Qizong-Tacheng section of the Tiger Leaping Gorge.
The sequence of distribution of glacial deposits is given in the following examples. Along the margins of the Tibetan Plateau and in mountain canyon valleys glacial deposits are primarily distributed at the bottom and middle layers of the riverbed. At the contact zone between the mountains and the Yunnan-Guizhou Plateau and the Sichuan Basin, the deposits are primarily distributed on the river terraces. In the Jinsha River, for example, glacial deposits are located at the bottom of the riverbed in the upper reaches. Near Shigu, they are in the middle layer of the riverbed mixed with other deposits. In the lower reaches they are above the river terraces. Essentially, the lower altitude of the Sichuan Basin accounts for the higher distribution of glacial deposits. For example, the shaft overburden on the left and right sides of the Xiluodu dam site is mainly composed of glacial and glaciofluvial deposits with a thickness of 42–59 m (He and You, 2013). At the same time, the middle and lower layers of the Yarlung Tsangpo riverbed are mostly glacial deposits (Figure 3).
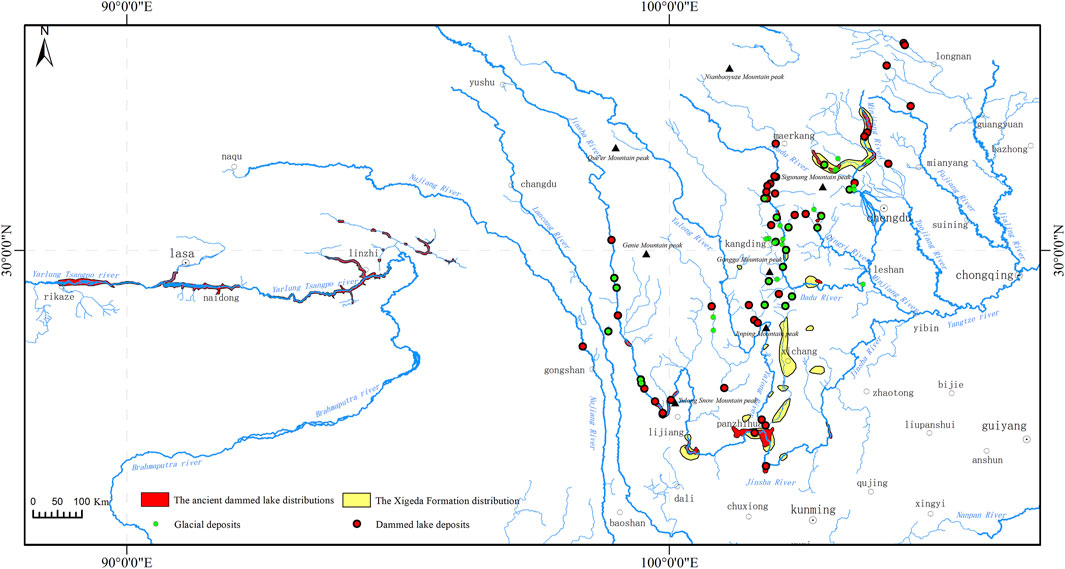
FIGURE 3. Distribution of Main Causes of RiverBed Overburden in Southwest China (Distribution of ancient dammed lakes, according to Wang Y. S. et al., 2007; Li et al., 2010; Zhang et al., 2020; Wang et al., 2021.Distribution of Xigeda Formation, according to Xu and Liu, 2011).
Analyzing longitudinal distributions between Hengduan Mountain and Longmen Mountain, no glacial deposits were found in the riverbed of the Jialing and Yellow Rivers in the north, or from the Nujiang and Lancang Rivers in the south, however glacial deposits were found on the terraces of the river valleys. In the middle area of the previously mentioned river sections, glacial deposits are found in the riverbed. In addition, there are large accumulations in the valleys of the Hengduan Mountains, many of which are of single or mixed glacial origins. Tu et al. (2008) found that since the Quaternary, which was influenced by glacier movement, a layer of glaciofluvial and moraine deposits has been widely accumulated along the middle reaches of the Dadu River at an altitude of 700–1500 m, with a maximum thickness of more than 100 m and the thinnest point is just a few meters.
(3) Geogenic (Formation) Mechanism(s)
The eastern part of the Tibetan Plateau is divided into three glacial periods: the last glacial period, the penultimate glacial period, and the antepenultimate glacial period. There were no quaternary ice sheets, but some intermittent distribution of ice caps and mountain glacial centers formed by high mountains, planation surfaces, and high-level basins (Li et al., 1991). The high mountains in the Hengduan Mountain range have experienced several glacial periods. As the glaciers melted, the water carried a large amount of moraine debris along the way that finally collected in the river valleys and basins. In the middle and upper reaches of the Dadu River, Gongga Mountain is the most concentrated and developed area of glaciers in the Hengduan Mountains. The moraines of the last glacial period (Dali Glaciation of late Pleistocene) are distributed below 2000 m, and the ancient glacial erosion and accumulation remains are distributed in bands from the center to the periphery downstream of these mountains. The lower limit of the distribution ranges from about 2000 m above sea level on the edge of the plateau to more than 5,000 m above sea level in the interior of the plateau (Shi and Zheng, 1995).
3.1.3 Dammed Lake Deposits
(1) Characteristics
Dammed lake deposition is primarily composed of interbedded silty clay and silty fine sand. It is typically of uniform grain size in thick layers ∼ huge thick layers with obvious horizontal bedding, and few other fossils except spores and pollen (Zhang et al., 2020).
(2) Distribution range
The dammed lake depositions are primarily found as a mix with other deposits or as a formation of separate layers generally found in riverbed overburden (Figure 2). These deposits are consistent with those of dammed paleolakes. For example, the ancient Shigu Lake retains thick lacustrine sediments in the middle of the riverbed of the Tiger Leaping Gorge section, the Jinsha-Longjie section of the ancient Longjie Lake, as well as the riverbeds of Zhaizicun, Xuelongbu, Temi, Qiaojia Lake, Xigda Lake, Taoyuan Lake, Daju Lake, Shigu Lake, Jintang and other dammed paleolakes have thick lacustrine sediments.
The dammed paleolakes existed in Diexi and downstream in the Minjiang River Basin, as well as in the Zagunao River, a tributary of the Minjiang River (Wang L. S. et al., 2007). These have layers of dammed lake deposits in the Futang and Diexi sections of the main stem of the Minjiang River, Shiziping in the tributary of the Zagunao River, and the Yuzixi II in the tributary of the Erhe River.
The Tongzilin dammed paleolake in the Yalong River is mainly distributed in the Tongzilin-Ertan section. The Lingguan River dammed paleolake (Li et al., 2010) in the upper reaches of the Qingyi River has a thick layer of dammed lake depositions in the riverbed segments such as Xiaoguanzi, Minzhi, and Zuijugou.
Dammed lake depositions are widely distributed in the middle and upper reaches of the main stem of the Dadu River, and dammed paleolakes (deposits?) such as Kairaocun and Jiajun have been discovered (Zhong and Ji, 2012).
Since the late Pleistocene, several river-blocking events have occurred in the south-north river system in Southwest China, forming several dammed lake deposits (Li et al., 2010). The dammed lake depositions are roughly distributed along the Gongga Mountain-Yulong Mountain line and extend along the Jinsha and Dadu River valleys to the Tibetan plateau. The specific distribution area is in the intersection of the Tibetan Plateau, Yunnan Plateau, and Sichuan Basin where there is an abrupt change in elevation.
(3) Formation Mechanism
Dammed lake deposits are from lakes formed by dammed or regional subsidence (fault depression or depression) due to the river flowing through the area. For example, the Xuelongnang and Temi dammed paleolakes in the upper reaches of the Jinsha River were probably formed by large landslides triggered by seismic activity in the area (Chen and Cui, 2015; Chen J. et al., 2021). The dammed paleolakes in the Benzilan-Qiaojia section of the Jinsha River are mostly formed by landslides or glacial deposits blocking the Jinsha River (Zhang et al., 2020).
Due to the continuous uplifting process of the Tibetan plateau, the increased erosion of the river has resulted in a large number of dammed lake deposits being distributed downstream. There may be multiple dammed river events in the same river section, many of which are preserved in the formation of terraces. For example, Zhang et al. (2007) studied the lacustrine lamellar clay layer and its formation by landslide debris flow blocking the river in the early to middle of the late Pleistocene as found under the fourth terrace on both sides of the valley in the Benzilan area of the Jinsha River. Some researchers have also found landslide dammed paleolakes in the Lancang and Nujiang River basins, mainly preserved on river terraces. Additionally, the Gushui paleo-lake in the Lancang River in the Gushui area of Deqin County, Yunnan Province, was formed in the late last interglacial period of the early Pleistocene and the early interglacial period of the last Pleistocene, it was found at the base of the third and fourth terraces (Zhang and Zhao, 2008). A further example is the sand-layer of a barrier lake formation that is about 100 m thick in the ninth terrace of the Nujiang River valley in the Daojie-Huitongqiao section which was formed during the middle and late Pliocene between 4.2 and 2.6 Ma (Zhao et al., 2012).
Based on the current dating of the riverbed overburden, it has been concluded that the dammed lake depositions have formed starting from the Late Pleistocene and Holocene eras and continued to the present. The upper age limit of dammed lake depositions in the Benzilan-Qiaojia section of the Jinsha River is from the late Pleistocene, and the lower age limit is from the late to the early Pleistocene (Zhang et al., 2020). The dammed lake depositions in the middle reaches of the Dadu River were formed from 18 Ka to 11 Ka (Li C. Z. et al., 2015). The lacustrine deposit at the Tongzilin dam site in the Yalong River started at 25 Ka and lasted to ∼10 Ka (Ding et al., 2021). The dammed lake depositions in Dixi, from Dixi to the lower reaches of the Minjiang River and its tributary the Zagunao River, were dated to about 20 Ka BP (Wang Y. S. et al., 2007). This period from the late Pleistocene to Holocene, is also the period of accelerated rise of the Tibetan plateau (Li et al., 1979).
There are also some lacustrine sediments widely distributed in Southwest China, mainly preserved in terrace form, such as the lacustrine sediments of the Xigda Formation, which are 4.2–3.3 or 2.6–1.8 Ma BP in the middle and late Pliocene or to the early Pleistocene (Zhao et al., 2008). The Longjie silts along the valley of the Jinsha River from Sanduizi of Panzhihua City to Baimakou of Wuding County belong to the late Pleistocene (Li H. L. et al., 2015).
3.1.4 Geological Hazard Deposits
(1) Characteristics
Colluvial deposits are a primary source of riverbed overburden in Southwest China. The colluvial deposits are a loose, non-cohesive soil containing rock fragments. The particle size composition of landslide deposits varies greatly, and the particle size distribution range is very wide. Debris flow deposits are mainly composed of gravel, sand, silt, and clay, and the grain gradation varies significantly.
(2) Distribution range
The deposits formed by geological disasters, such as hillside collapse, landslide and debris flow, are primarily distributed in mountain rivers where the riverbed has a large gradient. These deposits have a short transient time in the river and are usually found in small, isolated pockets dotted in the longitudinal direction of the river. Examples of this are in the Wudong section of the Jinsha River and the Xiaoguanzi section or the Qingyi River. The sediments are seldom distributed in bands, instead they are mixed with other sediments to form layers during river cutting. This can be seen in the Tiger Leaping Gorge section of the Jinsha River and the Danba to Luding section of the Dadu River. Large landslides and debris flows are typical mechanisms in blocking a river. Once the dam breaks, the depositions will form a long strip-shaped distribution pattern downstream. As the Tibetan plateau continues to rise, the capacity for river erosion is increased. Consequently, the remains of the disaster sediment are eroded, leaving little residual sediment in the riverbed. However, a large quantity of sediment is retained in the valley terrace and bank slopes. For example, the Xuenongnang dammed paleolake on the upper reaches of the Jinsha River was formed by an earthquake-induced landslide. After the dam broke, the depositions were distributed within a range of 3.5 km from the downstream side (Chen and Cui, 2015). Additionally, disaster deposits exist in the form of accumulation predominantly along riverbanks as a result of the deep river cutting.
(3) Formation Mechanism(s)
The formation mechanisms for geological hazard deposits are heavily influenced by external factors such as earthquakes and rainstorms, this may also be coupled with rapid uplift and deep river cutting. This is a result of the very active internal and external geodynamic processes in the eastern margin of the Qinghai-Tibet Plateau. Both large and giant landslides, hillside collapses and debris flows occur frequently in mountain canyon valleys, forming deep accumulations in local areas. The concentrated occurrence time of paleo-landslides in mountain canyon valleys of the Dadu River basin and the upper reaches of the Minjiang River basin is 20–30 Ka, and the highest frequency of the paleo-landslides in the Dadu River is 15–25 Ka, speculated to have occurred in the late Pleistocene. The strong tectonic activity of the Dadu River Basin resulted in frequent large-scale landslides, consequently a large volume of paleo-landslide deposits remains in the Dadu River basin today (Zhang et al., 2021). The Xuelongnang and Temi dammed paleolakes in the upper reaches of the Jinsha River were probably formed by large-scale landslides triggered and blocked by paleo-seismic activity in this area (Chen and Cui, 2015; Chen Y. et al., 2021). The age of the existing geological disaster deposits is mainly in the 20–30 Ka range, which is in the glacial peak of the last glacial period.
3.2 Geogenic Mechanisms
3.2.1 Overburden Formation Mechanisms in Primary Rivers
The riverbed overburden thickness of the main rivers in Southwest China presents a shallow layer with a single origin that is primarily alluvium or alluvial-pluvial. The formation of deep or huge thick riverbed overburden is the result of multiple genesis. The controlling factors vary in different reaches of each river. According to the material characteristics of current riverbed overburden, the genesis of main river overburden is discussed.
(1) Minjiang River
The riverbed overburden in the Minjiang River basin was formed by dammed lake deposition, glacial sediments and alluvial-proluvial deposits. From the regional distribution data, there are dammed lake deposits of differing thickness in the riverbed of the main and tributary rivers in the upper reaches of the Minjiang River, especially in the Diexi section. The bottom of the overburden layer of the main stem and the left bank tributaries of the Minjiang River in the east slope of Siguiniang Mountain is mostly glaciofluvial sediments.
The deep riverbed overburden in the upper reaches of the Minjiang River is mainly distributed in the middle portion of the Longmen Mountains. During the uplifting process of the Tibetan plateau, the main peaks of Qionglai and Sionglai Mountains, formed from the surrounding highlands and above the snow line, becoming the local center of quaternary glaciation. During the glacial period, glaciers developed on Qionglai Mountain, During the interglacial period, the glaciers melted anddeep river cutting occurred carrying a large amount of the glacial moraine deposits into the Minjiang River which was subsequently deposited in the Minjiang River valley. During the uplift of Longmen Mountain, seismic activity resulted in the river also uplifting causing the deep valley to form, the result of which was increased erosion deposits blocking the river. Wang L. S. et al. (2007) studied landslide derived and other small-scale ancient barrier lakes in Dixi, Maoxian, Wenzhen, Mianyang, Guogou, and Lixian in the upper reaches of the Minjiang River. Thi indicated that earthquakes and rainstorms lead to (hillside) collapse, landslide and debris flows blocking the river, or the landslide front is deposited directly on the early overburden, which resulted in local accumulation of deep overburden.
(2) Qingyi River
The deep riverbed overburden is mainly deposited in the upper reaches of the Qingyi River. The origin of the overburden in the main source Baoxing River basin is complex. The riverbed overburden in the East River showed that the bottom of the river is ice-water sediment, and the middle layer is alluvial-pluvial, debris flow accumulation, colluvial and barrier deposits, and the surface layer is the modern river alluvium. The riverbed of the Xihe River has a deep overburden. The bottom of the riverbed overburden is an alluvial-proluvial layer, the middle is a dammed lake deposition layer, and the surface is a modern river alluvial layer. These are typical at the Chujugou Power Station sluice site.
The source of the Qingyi River is the Qionglai and Jiajinshan Mountains, both of which are areas with intense crustal uplift occurring from the late to recent periods. The local climate characteristics, leading to frequent geological disasters such as debris flow and river blocking events, and forming dammed lake deposition in the riverbed are primary sources. The thickness of the river deposits at the Xiaoguanzi dam site area reaches a maximum depth of 87 m and has 10 distinct layers according to its origin. The sixth and eighth layers are dammed lake deposits and the fifth and seventh layers are debris flow deposits; it was found that the left bank of the Baoxing River was blocked by two extra-large debris flows in Guangou (Peng, 1996). In addition, the Donghe River basin is located on the southeast slope of Siguniang Mountain. The glaciation of Siguniang Mountain led to the existence of glacial deposits at the bottom of the riverbed.
(3) Dadu River
The deep overburden in the Dadu River basin is distributed continuously and stably in “bands” and has the characteristics of a continuous regional development in spatial distribution. According to the material origin of the overburden, the Dajinchuan Section (above Danba County reach) is composed of dammed lacustrine and alluvial-pluvial deposits. The middle and upper reaches of the Dadu River (above Dagangshan) are mainly composed of glaciofluvial, dammed lake, and alluvial-diluvial deposits. The lower reaches of Dagang Mountain are mainly composed of alluvial-pluvial deposits.
Tectonic movement controlled the development of the Dadu River valley. Based on the north-south structure control the Dadu River valley above Shimian county was an “L” shape. Below Shimian county, due to the east-west uplift block of Shimian-Ebian, the river turns sharply to the east. Under the action of neo-tectonic movement, the broad valley basins of the Jinchuan intermountain depression, the Jiajun tectonic adaptive basin, the Hanyuan fault depression basin, and other wide valley basins were formed.
During the late Pleistocene Dali glacial period, moraines piled up in the middle and upper reaches of the Dadu River valley forming weir dams. Meanwhile, the terminal moraine of the tributary also blocked the Dadu River valley forming barrier lakes allowing lacustrine sediments to be deposited in the riverbed. As the climate warmed in the postglacial period, the melting of the glaciers and the increased flowing water broke the dam. The glacier descending height was higher in the Dajinchuan section increasing the strength of the erosion. The glacier descending height was lower in the upper part of Dagang Mountain, the weir dam was taller, the lake deposits were thicker, the river erosion had not cut through the moraine, and the glacier accretion overburden was developed before the current riverbed. The moraines in the lower reaches of Dagang Mountain are mainly preserved on the river terraces (Shi, 1986).
The deep overburden of the Dadu River is the result of the composite accumulation of tectonic subsidence and glaciation (Shi, 1986). The Dajinchuan reach is a tectonic multilayer accretion, and the middle and upper reaches of the Dadu River are the products of the last glacial period (Dali Glaciation of late Pleistocene) and tectonic-controlled adaptive valley basins (such as Jiajun). Areas below Dagangshan are mainly the result of tectonic control, such as faulted basins (e. g. Hanyuan basins).
(4) Yalong River
The riverbed overburden in the upper reaches of the Yalong River is relatively shallow, generally less than 40m, primarily located in the eastern margin of the Tibetan plateau, and the river erosion rate is relatively high. The deep overburden of the Yalong River is mainly distributed in the middle and lower reaches. The deposit layer can be divided into three parts along the depth, the upper layer is modern river deposit; the middle layer is composed of alluvial, pluvial, barrier lacustrine, and is comparatively thick; the bottom layer is alluvial and colluvial deposits (Wang et al., 2006; Wang Y. S. et al., 2007). The overburden of the lower reaches of the Yalong River is composed of the middle layer attributed to deposits originating from the Tongzilin dammed lake. The dam site of the Tongzilin Hydropower Station has developed a 51 m thick deposit profile, which is generally composed of a lower alluvial layer (10 m thick), middle lacustrine layer (26 m thick) and upper alluvial layer (15 m thick) (Ding et al., 2021).
The origin of overburden of the Upper-Yalong River is dominated by deposits that are caused by blocking, while the middle and upper Yalong River sections are dominated by deposits typically associated with a composite origin. The deep overburden is caused by the second layer accretion sequence, and the dating of the accretion layer are 10–20 Ka bp (Wang Y. S. et al., 2007). This period was during the last glacial maximum when glaciers were widely developed. The decreased river flow coupled with the low potential energy and increased glacial debris resulted in the river filling and damming, forming a thick multi-geogenic accretion overburden. During the post-glacial period, the rivers incised rapidly, but did not cut through the accretion layer. Therefore, the deep overburden in the Yalong River is a climatic geogenic model formed during the last glacial maximum (Wang L. S. et al., 2007).
(5) Jinsha River
The riverbed overburden in the Jinsha River can be roughly divided into four sections according to the thickness: the source area (above the Lawa dam site) (<40 m), the lawa dam site to Daju section (>40 m), the Daju to Panzhihua city (<40 m), and the section below Panzhihua city (>40 m). Among them, the riverbed overburden in the source area and Daju to Panzhihua city is thinner, with a predominant alluvial layer, the cause of which may be mainly tectonic. The source area is in the Tibetan Plateau, which has been in the process of continuous uplift, and therefore the river erosion capacity is greater than the accumulation rate. The main reason for the thin riverbed overburden from Daju to Panzhihua city may be that it is in the same tectonic region, the Yangtze platform, so the uplift and subsidence of the crust and river erosion are at roughly the same level.
The separate layers of overburden from the Lawa dam site to the Daju section are composed of dammed lake deposits, glacial deposits, and deposits of geological hazards. Wang (2009) proposed that the thick riverbed overburden in this reach was formed by the ancient Gulongpan fault basin under the influence of neo-tectonic movement.
The Jinsha River overburden from Panzhihua city to Yibin city are primarily composed of alluvial-proluvial sediments in the top and bottom layers, and dammed lake deposits or mixed sediments in the middle layers. Ge et al. (2006) studied the Jinsha River from Tuoding to Yibin city and proposed that climate change directly affected the precipitation conditions of the Jinsha River basin. During the rainy period, the river channels were strongly incised, developing deep grooves, and included mass wasting of the bank slopes. During dry periods, the Jinsha River cuts down slowly, and the river channels form large deposits.
(6) Yarlung Tsangpo River
According to the available geological data, the riverbed overburden of the main Yarlung Tsangpo River and its tributaries, such as the Lhasa and Nianchu Rivers, has a large burial depth, mainly affected by glaciation and tectonic factors. The stratigraphic structure of the longitudinal riverbed overburden in the Yarlung Tsangpo River is basically a binary structure, with the upper layer being a modern alluvial-pluvial layer and the middle and lower layers primarily consisting of glacial moraine deposits.
The main stem of Yarlung Tsangpo River is characterized by a narrow and wide valley and thick river and lake deposits. Wang et al. (2014) studied the riverbed thickness from Xetongmen to the Yalu Tsangpo Canyon and found that, due to the variation in uplift rates of different river sections, the uplift rates of the canyon section were high, while the uplift rates of the wide valley section were low, the bedrock surface of the canyon river channel were several hundred meters higher than the sediment-bedrock interface of the broad valley river section in its upper reaches. The result was the formation of a huge “sediment reservoir.” Over the past million years, the pebble sediment had been filling this sediment reservoir forming a thick layer. At the same time, it also caused the valley floor to rise and widen, turning the v-shaped valley into a u-shaped valley (Figure 4).
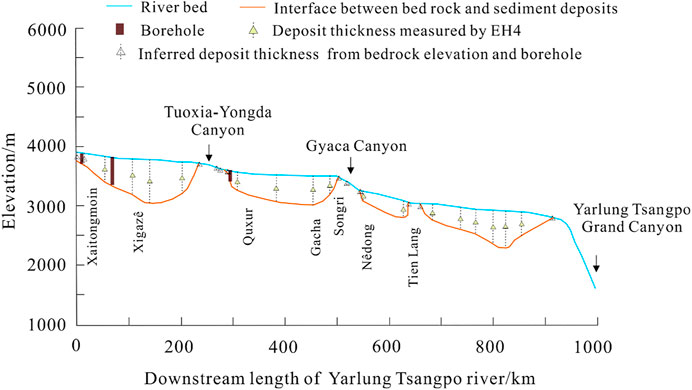
FIGURE 4. Longitudinal profiles of interface between bed rock and sediment deposits along the Yalu Tsangpo River Valley (Wang et al., 2014, modification).
Lacustrine sediments of dammed paleolakes are widely distributed along the valley of the Yarlung Tsangpo River basin across the southeastern Tibetan Plateau. There are several ancient dammed lakes in the middle and lower reaches of the Yarlung Tsangpo River, such as Dazhuka, Jiedexiu, Gega, Yigong, Zhongyu, Talu, Guxiang, Songzong, Yupu, Dongjiu, Lulang and others. The spatial distribution of these paleolakes may be controlled by the north-south normal fault system or follow active fault lines such as the Jiali fault. The paleolakeshad developed since the last glacial period, mainly during the last glacial maximum and the early Holocene, lasting for thousands to tens of thousands of years (Wang et al., 2021).
(7) Other river(s)
The deep riverbed overburden in the Jialing and Tuojiang River basins are mainly distributed in the Longmen Mountain area. Among them are the Jialing River tributaries; the Bailong River, the Baishui River, and the Fujiang branch of the Liuhuo River have developed deep overburden with zonal distribution. These deep overburden layers are mainly composed of alluvial or alluvial-proluvial deposits. Additionally, there are collapse deposits on the side of the river and dammed lake deposits in some sections of the river.
The deep riverbed overburden of the Nujiang, Yellow, and Lancang Rivers are mainly distributed and are similar in having a point-type distribution, where its origin is mainly formed by collapse, landslides, and debris flow.
3.2.2 Discussion on the Geogenic Mechanisms of Thick Riverbed Overburden in Southwest China
According to existing and ongoing research achievements the formation of deep overburden is very complicated with a deposit composition of fluvial and non-fluvial origins.
The change of dynamic conditions in the river system can only control the self-organization process of low-level streams (Yi et a1., 2021). It can cause the river to accumulate and cut down, but the influence is generally limited to a certain reach of a river, the time scale of the influence is short (10–1000 a), and the overburden is shallow (<40 m) (Maddy et a1., 2001).
There are many non-fluvial factors (contributing to the geogenic mechanisms), including erosion base level change, tectonic movement, climate change and so on (Bridgland, 2000; Alexey et al., 2019; Delaney and Adhikari, 2020); Xu et al. (2008) proposed that the existence of deep riverbed overburden in Southwest China was directly related to the increase and decrease in sea level since the Quaternary. However, some studies found that the influence of sea level rise and fall on river geomorphology development was only limited to the reach near the estuary (mainly downstream). It has little impact on the middle and upper reaches of inland rivers (Merritts et a1., 1994).
Tectonic movement and climate change are two important factors controlling the riverbed overburden thickness. However, the coupling of tectonic movement and climate change in the evolution of riverbed overburden is of primary concern.
Tectonic movement is a major contributor to the formation and evolution of rivers. Macroscopically, the Tibetan Plateau controls the sedimentary and biogeochemical exchange between mountains and oceans, and there are dynamic linkages and coevolution between tectonic plates and river systems, the macro-structure of the river system in the eastern Tibetan Plateau is controlled by tectonic movement (Yi et a1., 2021) A large number of low-temperature thermochronology studies have revealed the rapid exhumation of the southeastern margin of the plateau in Cenozoic stages at about 60–40 Ma BP, 30–20 Ma BP and 20–0 Ma BP (Clift and Sun, 2006; Liu et al., 2018). The South Slope of the Western Kunlun Mountains Plateau is the highest, and the southeast part of the Qinghai-Xizang Plateau, which flows east to the north of Tibet and is the source of the Yangtze and Yellow rivers, is the lowest, and descends by a steep slope (fault) to the mountains on the border of western Sichuan and the Yunnan-Guizhou Plateau. This general tilt from northwest to southeast is undoubtedly an important factor in controlling the flow of rivers like the Jinsha River, the Salween River, and the Lancang River (Li et al., 1979). On a smaller scale, the integral block uplift of the Tibetan plateau also has local differences. From the point of view of the specific river reach, the river spans different tectonic units, and the ascending and the up-and-down movement between tectonic units further contribute to river erosion and accumulation change, forming the riverbed overburden aggradation layer (Xu et al., 2008). For example, the longitudinal profile of the Dadu riverbed from the upper to the lower reaches shows a gentle-steep-gentle fold shape. The thickness of the valley deposits on the steep slope is different, the thin part is about 20 m, and the steep slope is in the Sichuan-Yunnan south-north tectonic belt, which is obviously related to the continuous uplift of neotectonics (Luo, 1995).
The variation in topography caused by tectonic movement not only affects slope stability, but also affects climate. The uplift on the eastern margin of the Tibetan Plateau, increases the potential energy of flowing water to carry out strong erosion and river cutting. There are various types of gravity based physical and geological processes active on these slopes. At the same time, in the middle-lower reaches of many rivers in Southwest China, the longitudinal gradient of the riverbed becomes steeper as it moves down the valley. As the steepness increases river erosion and cutting are also increased, and the physical and geological effects such as valley slope collapse, landslide and debris flow also increases, and these in turn form dammed lakes and colluvium deposits. The periodic uplift of the Tibetan Plateau and the resulting water and heat conditions, combined with global climate changes, have caused four alternating glacial and interglacial periods to occur on the plateau (Li et al., 1979). Some mountains in the eastern margin of the Tibetan Plateau formed glaciers when the mountains uplifted above the height of the snow line during the last glacial period. Some mountains at higher altitudes developed quaternary glacial periods, such as Mt. Gongga and Mt. Yulong, etc., but at least 12 relatively low-lying mountains between 4,200 m and 4,500 m only developed during the last glacial period. These mountains were strongly uplifted by about 1000 m due to the Gonghe Movement in the late Pleistocene causing them to move above the snow line and thus were only affected by the last glacial period (Cui et al., 2011). During the late Pleistocene, the glaciers advanced along these river valleys (Li et al., 1979). As a result of intense erosion of the upper source of the glacier, the longitudinal slope of the bedrock valley floor is shallower than that of the modern riverbed, and the overburden of the valley floor gradually thickens from the marginal mountain mouth of the basin to the upper reaches, forming deep deposits (e.g., Minjiang River valley) (Luo, 1995). At the same time, the strong rise of the Tibetan Plateau changed the atmospheric circulation, the monsoon had become dominant, and impacted the occurrence and development of quaternary sediments of different geogenic types in the valley (An et al., 2006).
As the climate warms (the glacial-interglacial transition), glacier melting accelerates in the alpine valley region, and river flow velocity and flux increase. According to model simulations, river sediment discharge increases 3–19 times after 100 years of accelerated glacial retreat (Delaney and Adhikari, 2020). Such high-speed sediment discharge leads to a reduction in the sedimentation of the riverbed, and only thinner sediments can be formed on the original overburden of the river valley. During the transition from the interglacial period to the glacial period, the climate gradually becomes colder and more unstable, which may lead to higher flood frequency and intensity, causing rivers to undercut. As the climate begins to cool, vegetation coverage decreases or even disappears, and as the average temperature continues to decrease, the vegetation coverage also decreases or even disappears. This cooling combined with the increased freeze-thaw action, more sediments enter the river, and the combination of higher sediment flux and lower stream flow cause the increased deposition of river overburden (Bridgland, 2000; Maddy et al., 2001; Liu et al., 2006) studied the four sets of lake terraces and lacustrine sediments of the Yarlung Tsangpo Grand Canyon and its tributary the Niyang River, using 14C dating found that during glacial advances the growth of the Zelunglung glacier on Mt. Namche Barwa formed ice dams and barrier lakes in the Yarlung Zangbo River, and developed corresponding lacustrine deposits. During the interglacial period the ice dam broke, the lake water was released and the lacustrine sediments decreased. In addition, according to the study, the rapid melting of plateau glaciers during the interglacial period was the trigger for earthquakes. During the last deglacial period, the rapid melting and erosion of mountain glaciers (or ice caps) unloading in the Tibetan Plateau might have induced frequent seismic activity in this area (Zhong et al., 2021).
The thickness of riverbed overburden is a geological phenomenon of the coupling effect of tectonic, climatic, and fluvial sedimentary processes during the Quaternary period. Take Qiaojia County section of the main stem of the Jinsha River as an example, the thickness of the overburden layer is nearly 900 m as revealed by wide-area electromagnetic resistivity sounding profile. Additionally, its lowest point is 100 m below sea level. According to the latest drilling data, the platform overburden thickness of Qiaojia County is more than 733 m (Shan et al., 2021; Cheng et al., 2022).
The formation of riverbed overburden in the Qiaojia section of the Jinsha River is mostly controlled by tectonic activity and fluvial sedimentation. Initially, the deposition process is controlled by these two processes (Figures 5, 6). The Qiaojia County section is mainly controlled by Xiaojiang fault and Zemuhe fault, forming a nearly elliptical pull-apart Basin that formed 1.1 ma ago and ended at about 34 ka (Li et al., 2016; Shan et al., 2021). According to the 14C dating data, the relative subsidence time of the basin stopped at about 34 ka, and its average subsidence rate was about 1–2 mm/year, slightly higher than the erosion rate of 0.4 mm/year in the Qiaojia section of the Jinsha River (Huang et al., 2010; Shan et al., 2021), indicating that it was the river accumulation stage.
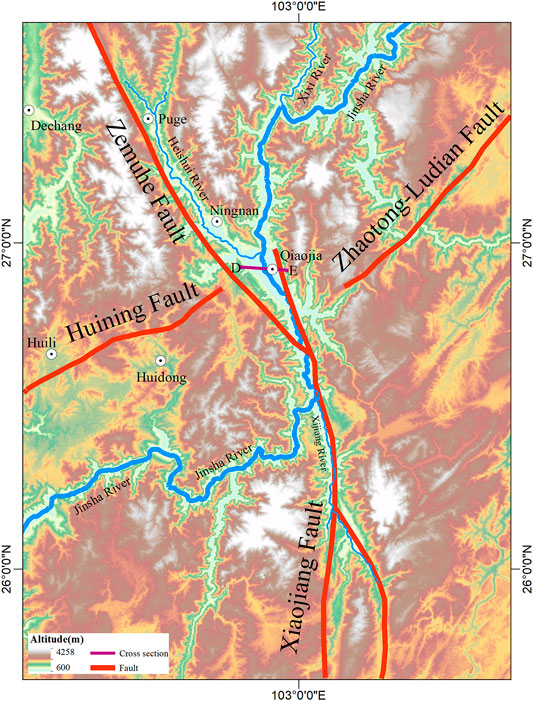
FIGURE 5. Tectonic setting of the Qiaojia Basin (Shan et al., 2021, modification).
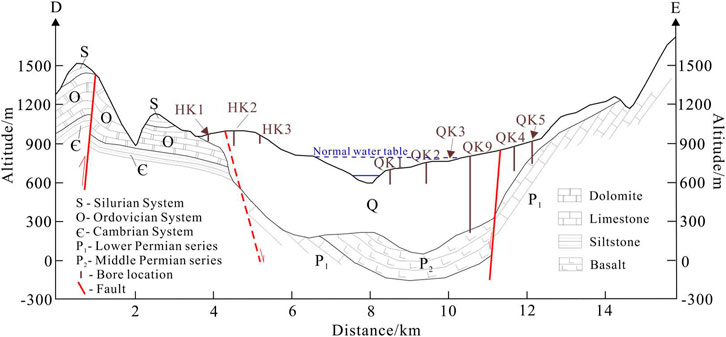
FIGURE 6. Geological model of the Qiaojia Basin (Shan et al., 2021, modification).
At the same time, the tectonic and fluvial processes affected the evolution of drainage catchment and backflow, and then affected the sediment. Before the Jinsha River was connected, there was a south-flow paleo-drainage system extending along the Xiaojiang fault zone in the Qiaojia section. The upper reaches of the Xixi River flowed into the ancient Honghe River system after passing the Paleo-Xiaojiang River. According to the heavy mineral analysis of paleo-fluvial sediments in the overburden of this section, the provenance area is close to Qiaojia basin, which is inferred to be the product of the Paleo-Jinsha River flowing south. There are three main layers of paleo-fluvial alluvial facies, accounting for about 50% of the total thickness (Table 2). The late Quaternary uplift of the Yunnan Plateau blocked the Xixihe-Baihetan-Qiaojia paleo-drainage from flowing south, capturing the upstream erosion process of the ancient Duiping River (one of the branches of the Niulanjiang paleo-drainage). This resulted in the reversed flow of the Qiaojia River segment to the north, through the Jinsha River (Li et al., 2009; Cheng et al., 2022).
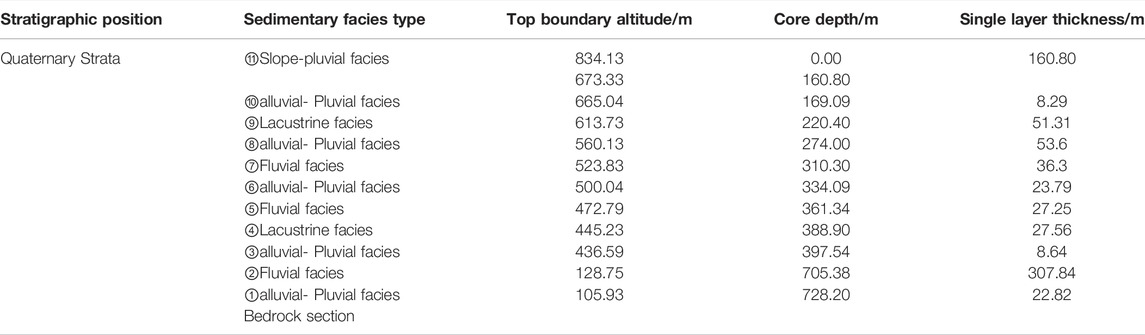
TABLE 2. Sedimentary sequence revealed by boreholesin Qiaojia Basin(Cheng et al., 2022, modification).
The effects of climate change should now also be considered as an important factor in the formation of overburden. Luoji Mountain in the upper reaches of Zemu River on the north side of Qiaojia Basin still retains well-preserved Quaternary moraines of Penultimate Glaciation (MIS 6) early stages of the last Glaciation(MIS 4) and late stage of the Last Glaciation (MIS 2) (Tang et al., 2021) Since the last glacial period, the sedimentary climate in this region was dry and cold, with less precipitation, and the sediments were mainly from physical weathering. According to stratigraphic sporopollen analysis, the overburden in this section transitioned through a dry-cold grassland environment, mild-humid environment, warm-humid environment, and a modern dry-hot climate. The sporopollen assemblage reveals that the environmental evolution cycle was consistent with the sedimentary cycle. Each sedimentary layer of the overburden in this section is composed of multiple cyclic deposits with a coarse-fine grain size change (Shan et al., 2021).
Finally, the coupling effect of the three factors is conclusive. Both tectonic activity and climate change lead to frequent landslides, floods and debris flows in this section, which then affect the river geological processes. The overburden in this section can be traced to lacustrine sediments, debris flow alluvium and slope-alluvium, accounting for about 50% of the total thickness (Cheng et al., 2022). Among them, the evidence suggests that the lacustrine sediments are from dammed lake deposits. There are many ancient landslide bodies near Qiaojia basin, and there is a huge ancient landslide residue about 3 km away from the lower boundary of the Qiaojia Basin, which certainly blocked the Jinsha River and formed a large barrier lake, providing conditions for the static water deposition (Li et al., 2016).
4 Hydroengineering Geological Problems and Treatment of Deep Overburden
The spatial distribution of hydropower resources in China are uneven as they are affected by topography and climate conditions. The hydropower resources in major rivers such as the Jinsha River in Southwest China are very rich, thus, it has become the center of hydroengineering construction in China. Flood control and hydroelectric are two of the main engineering activities in Southwest China. The construction of dams often encounters thick overburden. The diverse genesis of deep overburden restricts the site and dam type for hydroelectric power stations, These are often based on differences in lithology, sediment grading, structure, particle shape, density, and degree of cementation. Altogether it increases the complexity of the dam junction layout and adds further complexity to the foundation design.
Faced with complex and thick overburden and the general risk of dam construction, there are two main treatment methods for overburden: excavate all the overburden layers, or alternately, partial excavation of the overburden layers.
4.1 Excavation of all the Overburden
When the overburden layer is relatively thin and its removal will not add further technical problems and will not increase the amount of engineering and investment too great, the overburden layer can be removed completely and treated according to the conventional damming technology on bedrock.
For example, the thickness of the riverbed overburden at the Wudongde Hydropower Station on the Jinsha River is 52–73 m. After all the overburden was excavated, a hyperbolic thin arch dam was built on the limestone bedrock. The dam has a maximum height of 265 m. For the Shuangjiangkou Hydropower Station on the Dadu River, the riverbed overburden depth is 48–57 m (partial 67.8 m). The decision was made to excavate all the overburden layer at the bottom of the core wall, build the core wall directly on granite, and construct a 314 m high rockfill dam with a gravel-soil vertical core (Liu, 2015). In both cases the removal of the relatively thin overburden did not add unnecessary complexity and additional financial burden onto the project allowing the dam to be built on bedrock.
4.2 Partial Excavation of Overburden
When the thickness of the overburden is very large, the cost of excavation may be too high and overall construction becomes more difficult. It may be decided to retain part of the overburden and build the dam directly on the overburden layer, but the engineering characteristics of the thick overburden often result in three major problems: dam foundation deformation, dam foundation seepage, and/or sand liquefaction. These three problems are directly related to the safety and financial investment of the project.
(1) Dam foundation deformation
The riverbed overburden layer of the dam foundation may have different geogenic types, a complex structure, differing thicknesses of each layer, and different physical and mechanical properties, each of which adversely affect the stress distribution and overall deformation of the dam body, core, and anti-seepage walls. Therefore, based on the specific situation of the project it is necessary to reinforce the overburden layer within a certain range of the dam foundation, this increases the strength and stability of the foundation and reduces the uneven deformation of the dam body. the reinforcement measures such as the excavation and replacement, consolidation grouting, vibro-replacement stone columns and Jet Grouting are often adopted.
The Pubugou Hydropower station dam in the main stem of the Dadu River is located on a deep overburden with a depth of 78 m. The uneven deformation of the dam’s foundation is the key to dam foundation treatment. All soils above 670 m of the originally designed foundation surface should have been excavated. During construction, the soft soil and soil layers which are susceptible to liquefaction were found in the dam foundation. The result was the final foundation surface was excavated to a depth of 667 m, and a backfill measure was adopted to 670 m of the dam foundation design height. At the same time, 10 m deep consolidation grouting was carried out under the dam foundation. The results show that the overburden after grouting meets the design requirements of foundation bearing capacity and deformation modulus, and the continued operation of the reservoir indicates that these measures were successful (Xue et al., 2012).
The original design of the composite geomembrane inclined-wall rockfill dam of the Renzonghai Hydropower Station was to excavate all the silt loam layers within 20 m of the dam foundation. Two additional problems presented themselves in this plan, first because it is difficult to excavate silt loam and second, the resulting pile up after excavation which may cause soil erosion and pollution of the river channel, it was decided to reinforce the foundation by vibro-replacement stone columns in the project implementation stage (Fan and Wang, 2012).
(2) Dam Foundation Seepage
Due to the nature of riverbed overburden and its variable sedimentary makeup there is a strong tendency for dam foundation seepage. The specific reasons for foundation seepage are that the overburden has coarse grain, boulders, low permeability resistance, strong permeability, and the more common local overhead. More specifically seepage deformation failure forms, such as contact scouring and soil piping resulting from glacial deposits, alluvial-proluvial deposits, and colluvial-sliding deposits add to foundation seepage. Engineering measures must necessarily be taken to prevent seepage and premature dam failure. Curtain grouting of the dam foundation or the construction of a concrete anti-seepage wall to cut off the seepage path and reduce hydraulic gradient are common methods (Hedayati Talouki et a1., 2015; Luo and Huang, 2020). The seepage control measures mainly include the vertical cutoff technique, the horizontal anti-seepage technique, and joint seepage control. In China, the concrete cut-off wall or the combination of cut-off wall and curtain grouting are the most common for the construction of high earth-rock dams on a deep overburden layer. This is mainly due to their mature technology and their highly effective ability to protect against permeability. Horizontal anti-seepage techniques are more suitable for dam foundations with weak permeability. When the overburden is thick and the vertical anti-seepage conditions are unfavorable or are too cost intensive, the horizontal anti-seepage scheme should be studied and adopted, but the effect of the Horizontal anti-seepage technique is limited. For tall and medium height dams, complex strata and projects with large anti-seepage requirements, the final technique should be carefully selected.
A typical example is the Tarbela Dam in Pakistan, this high dam of 147 m was built on a 230 m thick overburden layer. Using horizontal seepage prevention measures, the dam used a length of 1,432 m of clay paving seepage prevention. The result was that after initial water filling the dam foundation permeability was unacceptably high. During water storage in 1974, there were more than 100 collapse pits, in 1978 after a dumping soil treatment, the overall seepage and the seepage of the dam foundation has tended to be stable (Dang and Fang, 2011).
(3) Sand Liquefaction
Loose sand layers are typical in thick riverbed overburden and are susceptible to liquefaction. In particular, the sand lens interlayers are mostly distributed in the nearshore along the river. The thickness is generally less than 2 m, and the maximum thickness is about 13 m. The liquefaction of sand layers often occurs during earthquakes. Sand liquefaction has several harmful effects, such as sand-boils and waterspouts, ground subsidence, inducing high-speed landslides, creating foundation instability, etc. Sand liquefaction results when the pore water pressure increases, the effective stress of the soil skeleton decreases, and the soil is transformed from solid to liquid.
Chen et al. (2013) has reviewed the site liquefaction phenomenon during earthquakes over the past 20 years and concluded that the depth of soil liquefaction can reach 20 m, however, below 20 m sand liquefaction becomes more difficult. For a sand layer that is in the location of the dam foundation where it is judged to be possible for liquefaction, the measures of excavating or changing the soil should be taken. When excavation is difficult or uneconomical, artificial infill measures may be taken, or concrete diaphragm wall or other methods can be used to enclose the liquefiable layer under the foundation. The possible liquefied sand layer of the dam foundation of the Huangjinping Hydropower Station on the Dadu River was treated using basic excavation and setting the pressure on the slope downstream of the dam. The foundation of Jinping Hydropower station in the Yalong River prevents the liquefaction of the Sand Lens body through the technique of consolidation irrigation.
5 Conclusion
Based on the analysis of the exploration data of riverbed overburden in the primary river basins of Southwest China, the results show that:
(1) The riverbed overburden in Southwest China shows a certain regularity. The riverbed overburden in the Tibetan Plateau is shallower in the headstream area, it is thickest in the middle reaches along the mountain canyon valleys, and entering the plain area, and into the piedmont slope areas and the Yunnan Plateau, the overburden is shallower.
(2) The deep overburden in most river reaches can be vertically divided into three layers. The middle layer is a sequence of aggradation of multi-genesis deposits, mainly consisting of glacial deposits, damming lake deposits, and landslide and debris flow caused by geological disasters. The Glacial deposits are mainly distributed in the valley belt around the mountain which is above the snow line. Dammed lake deposits and geological disaster deposits are widely distributed, mainly in mountain canyon valleys where the riverbed gradient is large. Typically, the upper layers are modern river deposits, while the bottom layers are made up of paleo-riverbed deposits.
(3) The combination of regional tectonic movement, climate change, and river system development are major contributing factors to the origin of the aggradation resulting in the deep overburden of major rivers in Southwest China, this includes the Jinsha, Dadu, Yalong, Qingyi, Min, and Yarlung Tsangpo Rivers. However, the overburden of each river is the result of a complex action of multiple auxiliary sources guided by the effects of these main controlling factors. Due to the specific topography and geomorphology, geological environment, drainage evolution, and the local differential ascending and descending movement, there are clearly differences in the genesis of each river basin. However, no matter what factor or multiple factors are at work, the coupling of tectonic-climatic-fluvial sedimentation processes always affects the thickness variation and spatial distribution of riverbed overburden in Southwest China.
(4) In hydroengineering projects over thick overburden of riverbeds, two primary treatment methods are adopted: to remove the overburden completely or to remove it partially. When the dam foundation is placed on the overburden layer, there are three primary engineering problems that need to be addressed: dam foundation deformation, dam foundation seepage, and sand liquefaction.
Data Availability Statement
The raw data supporting the conclusion of this article will be made available by the authors, without undue reservation.
Author Contributions
ZY contributed to the data acquisition, investigation and wrote the original draft. MX and XK contributed to the data acquisition, the conception and design of the study. LG and SZ contributed to the data acquisition and graphic editing. The authors would like to thank Joshua Schmidt for his help on the English editing of the manuscript. All authors have read and agreed to the published version of the manuscript.
Funding
This research is supported by the State Key Laboratory of Geohazard Prevention and Geoenvironment Protection at Chengdu University of Technology (SKLGP2018Z018);Power China Chengdu Engineering Corporation Limited (P238-2014);The College Science Foundation of Sichuan Water Conservancy Vocational College (KY2021-07).
Conflict of Interest
SZ was employed by Power China Chengdu Engineering Corporation Ltd.
The remaining authors declare that the research was conducted in the absence of any commercial or financial relationships that could be construed as a potential conflict of interest.
Publisher’s Note
All claims expressed in this article are solely those of the authors and do not necessarily represent those of their affiliated organizations, or those of the publisher, the editors and the reviewers. Any product that may be evaluated in this article, or claim that may be made by its manufacturer, is not guaranteed or endorsed by the publisher.
Supplementary Material
The Supplementary Material for this article can be found online at: https://www.frontiersin.org/articles/10.3389/feart.2022.895769/full#supplementary-material
References
An, Z. S., Zhang, P. Z., Wang, E. Q., Wang, S. M., Qiang, X. K., Li, L., et al. (2006). Changes of the Monsoon-Arid Environment in China and Growth of the Tibetan Plateau since the Miocene. Quat. Sci. 26 (5), 678–693.
Bridgland, D. R. (2000). River Terrace Systems in North-West Europe: an Archive of Environmental Change, Uplift and Early Human Occupation. Quat. Sci. Rev. 19, 1293–1303. doi:10.1016/S0277-3791(99)00095-5
Chen, G. X., Jin, D. D., Chang, X. D., Li, X. J., and Zhou, G. L. (2013). Review of Soil Liquefaction Characteristics during Major Earthquakes in Recent Twenty Years and Liquefaction Susceptibility Criteria for Soils. Rock Soil Mech. 34 (10), 2737–2755+2795. doi:10.16285/j.rsm.2013.10.005
Chen, J., Cui, Z. J., Chen, R. C., and zheng, X. X. (2021a). The Origin and Evlution of Temi Paleolandslide-Dammed Lake in the Upper Jinsha River. Earth Sci. Front. 28 (2), 085–093. doi:10.13745/j.esf.sf.2020.9.9
Chen, J., and Cui, Z. J. (2015). Discevery of Outhurst Deposits Induced by the Xuelongnang Paleolandslide-Dammed Lake in the Upper Jinsha River,China and its Environmental and Hazard Significance. Acta Sedimentol. Sin. 33 (02), 275–284. doi:10.14027/j.cnki.cjxb.2015.02.007
Chen, Y., Wu, B., Xiong, Z., Zan, J., Zhang, B., Zhang, R., et al. (2021b). Evolution of Eastern Tibetan River Systems Is Driven by the Indentation of India. Commun. Earth Environ. 2, 256. doi:10.1038/s43247-021-00330-4
Cheng, W. Q., Tai, Z. H., Xiang, F., Huang, H. X., Zhou, Y. H., Song, L., et al. (2022). The Characteristics of Fluvial Deposition and Heavy Minerals in Qiaojia Basin: Indication for the Transfixion of Jinsha River, Southwestern China. J. Chenghu Univ. Technol. Sci. Technol. Ed. 49 (1), 84–93+103.
Clift, P. D., and Sun, Z. (2006). The Sedimentary and Tectonic Evolution of the Yinggehai-Song Hong Basin and the Southern Hainan Margin, South China Sea: Implications for Tibetan Uplift and Monsoon Intensification. J. Geophys. Res. 111 (B6), a–n. doi:10.1029/2005JB004048
Cui, Z. J., Chen, Y. X., Zhang, W., Zhou, S. Z., Zhou, L. P., Zhang, M., et al. (2011). Research History, Glacial Chronology and Origins of Quaternary Glaciations in China. Quat. Sci. 31 (5), 749–763.
Dang, L. C., and Fang, G. D. (2011). Major Technical Problems of Constructing Dam on Deep Overburden. Water Power 37 (2), 24–28+45.
Delaney, I., and Adhikari, S. (2020). Increased Subglacial Sediment Discharge in a Warming Climate: Consideration of Ice Dynamics, Glacial Erosion, and Fluvial Sediment Transport. Geophys. Res. Lett. 47. doi:10.1029/2019GL085672
Ding, Y., Zhang, X., He, Z., Lu, C., and Bao, S. (2021). Sedimentary Environment of a Dammed Lake Buried in the Modern Riverbed of the Yalong River During the Last Glacial Maximum and its Implication for Fluvial Geomorphic Evolution. Geomorphology 378, 107588. doi:10.1016/j.geomorph.2020.107588
Fan, Y., and Wang, L. H. (2012). Application of Vibro Replacement Stone Column in Renzonghai Weak Dam Foundation Reinforcement Treatment. China Water Power & Electrification 93 (11), 55–60.
Ge, Z. S., Liu, Q. Y., Xu, Q. M., Li, H. Z., Xue, G. F., Mei, Y. T., et al. (2006). The Geomorphic Evolution and Characteristics of the River Bed Lower Reaches of Jinshajiang River. Quat. Sci. 26 (03), 421–428.
He, W. Z., and You, F. (2013). Shaft Construction in the Deep Overburden Layer Segment of Xiluodu Hydropower Station Hydropower and New Energy, 30 32–35. doi:10.13622/j.cnki.cn42-1800/tv.2013.05.011
Hedayati Talouki, H., Lashkaripour, G. R., Ghafoori, M., and Saba, A. A. (2015). Assessment and Presentation of a Treatment Method to Seepage Problems of the Alluvial Foundation of Ghordanloo Dam, NE Iran. J. Geol. Soc. India 85, 377–384. doi:10.1007/s12594-015-0227-2
Huang, D., Yang, d. Y., Li, l. P., Shi, C. A., Lu, F., Ge, Z. S., et al. (2010). Incision Rates of the Baihetan Section of the Jinsha River. Quat. Sci. 30 (5), 872–876.
Li, C. Z., Wang, S. B., Fu, J. L., and Jiang, F. C. (2015a). Sedimentary Environment Evolutionary Characteristics of Longjie Silt Layer in the Middle Reaches of the Jinsha River,Yunnan. Geol. Bull. China 34 (1), 113–120.
Li, H. L., Zhang, Y. Q., and Li, J. H. (2010). Meridional River Systems and Ancient Dammed Lakes on the East Margin of the Tibetan Plateau. Quat. Sci. 30 (4), 812–824.
Li, H. L., Zhang, Y. Q., Qiao, Y. S., Zhu, F. S., and Li, J. H. (2015b). A Study of the Sediments in the Middle Reaches of the Dadu River on the Eastern Margin of the Tibetan Plateau and its Seismogeological Implications. Geol. Bull. China 34 (1), 104–112.
Li, J. J., Wen, S. X., Zhang, Q. S., Wang, F. B., Zheng, B. X., and Li, B. Y. (1979). Discussion on the Timing, Magnitude and Style of Uplift of the Tibetan Plateau. Sci. Sin. (6), 608–616.
Li, J. J., Zhou, S. Z., and Pan, B. T. (1991). The Problems of Quaternary Glaciations in the Eastern Part of Qinghai-Xizang Plateau. Quat. Sci. (3), 193–203.
Li, L. P., Yang, D. Y., Huang, D., Ge, Z. S., Xu, Q. M., and Wang, X. Y. (2009). Drainage Evolution of QiaoJia-XinShiZhen Section of Jinsha River. Quat. Sci. 29 (2), 327–333.
Li, T.-t., Pei, X.-j., Huang, R.-q., and Jin, L.-d. (2016). The Formation and Evolution of the Qiaojia Pull-Apart Basin, North Xiaojiang Fault Zone, Southwest China. J. Mt. Sci. 13 (6), 1096–1106. doi:10.1007/S11629-015-3778-1
Liu, S. H. (2015). Safety Assessment of Hydraulic Structures Built on Overburden Layer. Dam Saf. (1), 46–63.
Liu, Y. P., Montgomery, D. R., Hallet, B., Tang, W. Q., Zhang, J. L., and Zhang, X. Y. (2006). Quaternary Glacier Blocking Events at the Entrance of Yarlungzangbo Great Canyon, Southeast Tibet. Quat. Sci. 26 (1), 52–62.
Liu-Zeng, J., Zhang, J., McPhillips, D., Reiners, P., Wang, W., Pik, R., et al. (2018). Multiple Episodes of Fast Exhumation since Cretaceous in Southeast Tibet, Revealed by Low-Temperature Thermochronology. Earth Planet. Sci. Lett. 490, 62–76. doi:10.1016/j.epsl.2018.03.011
Luo, S. C. (1995). Cognition of Geological Problems of Thick Overburden Layer. Hydroelectr. Power (4), 21–24+58.
Luo, Y., and Huang, Y. (2020). Effect of Open-Framework Gravel on Suffusion in Sandy Gravel Alluvium. Acta Geotech. 15 (9), 2649–2664. doi:10.1007/s11440-020-00933-9
Maddy, D., Bridgland, D., and Westaway, R. (2001). Uplift-driven Valley Incision and Climate-Controlled River Terrace Development in the Thames Valley, UK. Quat. Int. 79, 23–36. doi:10.1016/S1040-6182(00)00120-8
Mahinov, A., Shevtsov, M., and Tkachenko, A. (2019). The Influence of Disastrous Floods on Riverbeds Processes in the Far East of Russia. MATEC Web Conf. 265, 06004. doi:10.1051/matecconf/201926506004
Marra, F., Motta, L., Brock, A. L., Macrì, P., Florindo, F., Sadori, L., et al. (2018). Rome in its Setting. Post-glacial Aggradation History of the Tiber River Alluvial Deposits and Tectonic Origin of the Tiber Island. PLos One 13 (3), e0194838–24. doi:10.1371/journal.pone.0194838
Merritts, D. J., Vincent, K. R., and Wohl, E. E. (1994). Long River Profiles, Tectonism, and Eustasy: A Guide to Interpreting Fluvial Terraces. J. Geophys. Res. 99 (B7), 14031–14050. doi:10.1029/94JB00857
Nield, M. C. (2018). Grout Curtain Construction at Bolivar Dam, Ohio. Environ. Eng. Geoscience 24, 121–136. doi:10.2113/gseegeosci.24.1.121
Peng, S. X. (1996). Preliminary Study on Lacustrine Deposit under Sluicegate at Xiaoguanzi Hydroelectric Power Station. J. Geol. Hazards Environ. Preserv. 7 (4), 34–36. doi:10.1007/bf02652053
Shan, Z. G., Cheng, W. Q., Wang, P., Wang, Y. S., Li, D. Z., and Chen, J. P. (2021). Sedimentary Construction and Mechanism of QiaoJia Basin in JinSha River-Evidence from Driling and Geophysical Exploration. Proceedings of 2021 national engineering geology Academic Annual Conference. 230–239.
Shi, J. L. (1986). Study on the Riverbed Thick Overburden Layer and Engineering Geological Problems of Dadu River. Sichuan Water Power 9 (3), 10–15.
Shi, Y. F., and Zheng, B. X. (1995). Studies on Altitude Climatic Environment in the Middle and East Parts of Tibetan Plateau during Quaternary Maximum Glaciation. J. Glaciol. Geocryol. 17 (2), 97–112.
Syvitski, J., Ángel, J. R., Saito, Y., Overeem, I., Vörösmarty, C. J., Wang, H., et al. (2022). Earth's Sediment Cycle during the Anthropocene. Nat. Rev. Earth Environ. 3, 179–196. doi:10.1038/s43017-021-00253-w
Tang, Q. Y., Zhang, W., Liu, L., Chai, L., Li, Y. P., Zhang, L. Q., et al. (2021). Glacier Scale and Paleoclimate Reconstruction since Penultimate Glaciation in the Qingshui Valley, Luoji Mountain, Western Sichuan. J. Glaciol. Geocryol. 43 (6), 1869–1877.
Tu, G. X., Huang, R. Q., and Deng, H. (2008). Deformation and Failure Mechanism of an Outwash Deposit under Scouring of River Water. J. Eng. Geol. 14 (5), 598–604.
Wang, L. S., Wang, X. Q., Xu, X. N., and Cui, J. (2007b). What Happened on the Upstream of Minjiang River in Sichuan Province 20, 000 Years Ago. Earth Sci. Front. 14 (4), 189–196.
Wang, L. S., Yang, L. Z., Wang, X. Q., and Duan, L. P. (2005). Discovery of Huge Ancient Dammed Lake on Upstream of Minjiang River in Sichuan, China. J. Chenghu Univ. Technol. Sci. Technol. Ed. 32 (1), 1–11.
Wang, P., Wang, H. Y., Hu, G., Qin, J. T., and Li, C. P. (2021). A Preliminary Study on the Development of Dammed Paleolakes in the Yarlung Tsangpo River Basin,southeastern Tibet. Earth Sci. Front. 28 (2), 035–045. doi:10.13745/j.esf.sf.2020.9.18
Wang, Q. G. (2009). Causes of Riverbed Deep Cover and Engineering Significance of Tiger Leaping Gorge Reach of Jinsha River. Chin. J. Rock Mech. Eng. 28 (7), 1455–1466.
Wang, Y. S., Huang, R. Q., Duan, H. P., and Wei, M. (2006). An Intensive Erosion in the Last Glaciation in the West of China. J. Chenghu Univ. Technol. Sci. Technol. Ed. 33 (1), 73–76.
Wang, Y. S., Luo, Y. H., Tang, X. J., and Sun, Y. M. (2007a). Characteristics of the Thick Deposit Layer Underlain with Unloaded Bedrock Forming the River Bed of Yalong River and Their Implications in Dam Construction. J. Eng. Geol. 15 (2), 164–168.
Wang, Z. Y., Yu, G. A., Wang, X. Z., and Liu, L. (2014). Impact of Techtonic Motion on Sediment Storage and Morphology of Yalu Tsangpo Valley. J. Sediment Res. (2), 1–7. doi:10.16239/j.cnki.0468-155x.2014.02.001
Xu, Q., Chen, W., Jin, H., and Zhang, Z. Y. (2010). Characteristics and Distribution of Thick Overburdens along the Dadu River Valley. Quat. Sci. 30 (1), 30–36.
Xu, Q., Chen, W., and Zhang, Z. Y. (2008). New View on Forming Mechanism of Deep Overburden on Riverbed in Southwest China. Adv. Earth Sci. 23 (5), 448–456.
Xu, Z. M., and Liu, W. L. (2011). Some Problems in the Study of the Genesis of Xigeda Formation. Earth Sci. Front. 18 (5), 256–270.
Xue, S. D., Lu, J. M., and Pang, B. (2012). Treatment of Deep Thick Cover Layer of Dam Foundation of Pubugou Hydropower Station. Yangtze River 43 (04), 35–38. doi:10.16232/j.cnki.1001-4179.2012.04.024
Yu, T., and Shao, L. (2020). Study on the Dynamic Characteristics of Dam Foundation on Deep Riverbed Overburden with Soft Soil Layer. Rock Soil Mech. 41 (1), 267–277. doi:10.16285/j.rsm.2019.0407
Zhang, X. B., Liu, Y., Hu, K. H., Hu, C. H., Dai, B., and Liu, W. M. (2020). Geological Implication of the Jinsha River Flowing Eastward,Ancient Dammed Lake Deposition,and Quaternary River Evolution in China. Mt. Res. 38 (6), 805–815. doi:10.16089/j.cnki.1008-2786.000557
Zhang, Y. S., Liu, X. Y., Wu, R. A., Guo, C. B., and Ren, S. S. (2021). Cognization, Characteristics, Age and Evolution of the Ancient Landslides along the Deep-Cut Valleys on the Eastern Tibetan Plateau,China. Earth Sci. Front. 28 (2), 94–105. doi:10.13745/j.esf.sf.2020.9.10
Zhang, Y. S., Qu, Y. X., Wang, X. L., Yao, X., and Guo, C. B. (2009). On the Engineering Geological Classification of Quaternary Glacial Deposits Is Southwestern Mountain Area of China. J. Eng. Geol. 17 (5), 581–589.
Zhang, Y. S., Zhao, X. T., and Hu, D. G. (2007). An Ancient Landslide-Dammed Lake Found in the Jinsha River Valley Near Benzilan, Dêqên, Yunnan and its Significance. Geol. Bull. China 26 (8), 970–975.
Zhang, Y. S., and Zhao, X. T. (2008). Sedimentary Characteristics of the Quaternary Damed Lake in the Lancang River Near the Dêqên Guoshui Area, Yunnan and its Significance. Acta Geol. Sin. 82 (2), 262–268+296.
Zhao, X. T., Hu, D. G., and Zhang, Y. S. (2008). Genesis and Age of the Gravels Underlying the Xigeda Formation of Panzhihua,Sichuan,China,and Valley Development of the Ancient Jinsha River. Acta Geosci. Sin. 29 (1), 1–12.
Zhao, X. T., Tong, Y. B., Wu, Z. H., Ye, P. S., and Hu, D. G. (2012). Buried Gravels and Dammed-Lake Sediments of the Pliocene Nujiang River Discovered in the Daojie Basin,western Yunnan Province and Their Magneto-Stratigraphic Evidence. Geol. Bull. China 31 (2/3), 227–234.
Zhong, H. P., and Ji, F. (2012). Preliminary Study on Sedimentary Characteristics and Evolution Process of Jiajun Ancient Dammed Lake in Dadu River. China Water Transp. 12 (4), 182–184.
Keywords: southwest China, river, overburden, distribution, geogenic mechanisms
Citation: Yan Z, Xu M, Kang X, Guo L and Zhang S (2022) Distribution Characteristics and Geogenic Mechanisms of Riverbed Overburden in Southwest China. Front. Earth Sci. 10:895769. doi: 10.3389/feart.2022.895769
Received: 14 March 2022; Accepted: 04 May 2022;
Published: 23 June 2022.
Edited by:
Xiaoyan Zhao, Southwest Jiaotong University, ChinaReviewed by:
Chengcheng Li, China University of Geosciences Wuhan, ChinaJianping Chen, Jilin University, China
Copyright © 2022 Yan, Xu, Kang, Guo and Zhang. This is an open-access article distributed under the terms of the Creative Commons Attribution License (CC BY). The use, distribution or reproduction in other forums is permitted, provided the original author(s) and the copyright owner(s) are credited and that the original publication in this journal is cited, in accordance with accepted academic practice. No use, distribution or reproduction is permitted which does not comply with these terms.
*Correspondence: Mo Xu, xm@cdut.edu.cn