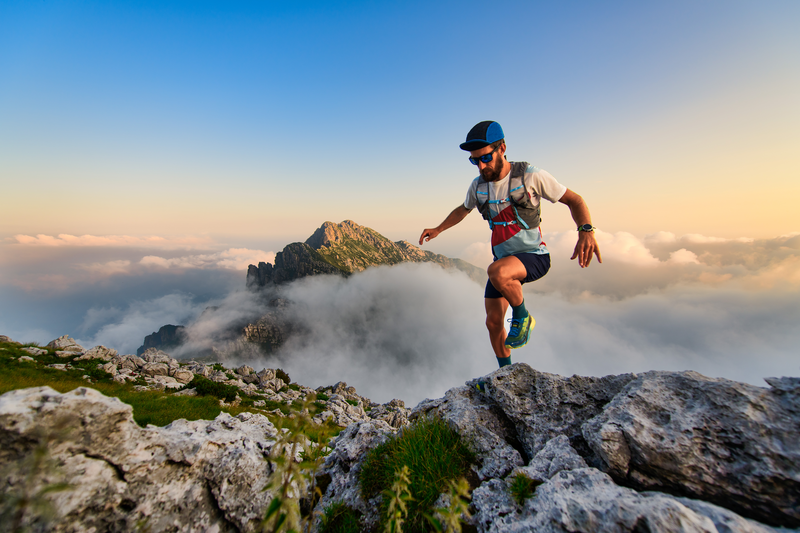
95% of researchers rate our articles as excellent or good
Learn more about the work of our research integrity team to safeguard the quality of each article we publish.
Find out more
ORIGINAL RESEARCH article
Front. Earth Sci. , 13 July 2022
Sec. Geochemistry
Volume 10 - 2022 | https://doi.org/10.3389/feart.2022.889112
This article is part of the Research Topic Isotopic Geochemistry of Natural Gas View all 14 articles
Tight gas is the fastest developing unconventional natural gas resource, becoming the principal part for gas reserves and production growth in China. The Sulige gas field is the largest gas field and also the typical low porosity and low permeability tight sandstone gas field discovered in China, with an annual natural gas output exceeding 300 billion and cumulative output exceeding 290 billion, playing an important role in ensuring national energy provision, helping China’s energy transformation, and promoting green, low-carbon, environmental protection and high-quality development. Based on sample collection and laboratory analysis, natural gas compositions including hydrocarbons, non-hydrocarbons, light hydrocarbons, and noble gases of the Sulige gas field are systematically analyzed, their genetic identifications are identified, and finally gas source originations and contribution proportions are comprehensively discussed from the perspectives of noble gases and hydrocarbon gases. The main achievements are as follows: 1) natural gases in the Sulige gas field are mainly alkane gases, with high methane content, high drying coefficient, low heavy hydrocarbon contents, low non-hydrocarbon gas contents of CO2 and N2, and relatively low noble gas contents. The helium content is relative 2 order of magnitude higher than the atmospheric value, while neon, argon, krypton, and xenon are relatively about 1–2 orders of magnitudes lower than the atmospheric values. 2) The carbon and hydrogen isotopes of alkanes are generally positive sequence distributions with some part inversion. The 3He/4He values are mainly distributed in magnitude of 10−8, the 40Ar/36Ar is ranged from 506 to 1940, the 129Xe is relative loss, and the 132Xe is relative surplus. 3) Natural gases in the Sulige gas field are typical coal-formed gases generated from a humic organic mother material with maturity from high mature to over mature according to C7 and C8 light hydrocarbons and alkane carbon isotopes. Noble gases are typical crustal genesis, mainly originating from the radioactive decay of crustal source materials. 4) The gas source correlations of noble gases and alkane gases and their quantitative evaluations on source contributions show that natural gases in the Sulige gas field are originated from Carboniferous-Permian coal measure source rocks in Ordos Basin, mainly contributed by coals and supplemented by mudstones, accounting for 55–60% and 40–45%, respectively.
Tight gas is a natural gas stored in overburden matrix permeability less than or equal to 0.1 md (air permeability less than 1 md) in tight sandstone formations. As an important unconventional gas resource, tight gas has played an important role in China’s natural gas development and been in a leading position in the process of unconventional gas development in China, becoming a major growth point for increasing natural gas reserves and production. Accelerating the exploration and development of tight gas is strategically important for the development of natural gas and the adjustment of the energy structure in China. At present, tight gas in China is widely distributed in Sichuan, Ordos, Tuha, Songliao, and Junggar basins, and mainly accumulated in Ordos and Sichuan basins. As early as 1971, China discovered the Zhongba tight gas field in the western part of Sichuan Basin. Since the mid-1990s, great breakthroughs were made in the exploration of Upper Paleozoic gas in Ordos Basin, and a large number of tight gas fields such as Yulin, Sulige, Wushengqi, Mizhi, Shenmu, and Zizhou were discovered successively. The Sulige gas field is the largest onshore mono-block natural gas field and also the largest unconventional tight gas field in China. The proven (including basic proven) gas reserves is 4.77×1012 m3 (Fu et al., 2019; Jia et al., 2021), the gas production is more than 300×108 m3 in 2021 and the cumulative gas production is more than 2,900×108 m3. It plays an important role in ensuring national energy provision security, helping China’s energy transformation and promoting green, low-carbon and high-quality development. Noble gases are considered as valuable tracers for the studies of geological processes, having great effects on gas origins and migration, crustal–mantle interaction, geotectonic etc. (Xu et al., 1979, Xu et al., 1998; Allegre et al., 1983, 1993; Ballentine et al., 1992; Battani et al., 2000; Burnard et al., 2013; Clarke et al., 1976; Du, 1989; Honda et al., 1991, 1993; Hiyagon et al., 1992; Kennedy et al., 1990; Lee et al., 2006; Liu and Xu, 1987, 1993; Lupton, 1983; Lupton and Evans, 2004; Mamyrin et al., 1970, 1984; Mark et al., 2011; Ozima and Podesek, 1983; Poreda and Farley, 1992; Poreda et al., 1986; Prinzhofer, 2013; Sano et al., 2008; Sarda et al., 1988; Shen et al., 1995; Sun et al., 1991; Wang, 1989; Wang et al., 2013, Wang et al., 2015, Wang et al., 2016, Wang et al., 2018; Wei et al., 2014; Welhan and Craig, 1983; Welhan et al., 1983; Xu et al., 1996; Xu et al., 1996, 1997). A lot of researches have been done on the geochemical characteristics, genesis, and sources of natural gas in the Sulige gas field (Dai et al., 1997; Fu et al., 2000; He et al., 2003; Dai et al., 2005; Feng et al., 2007; Hu et al., 2007; Liu et al., 2007; Hu et al., 2008; Lin et al., 2009; Dai et al., 2012; Hu et al., 2012; Li et al., 2012; Fu et al., 2013; Liu et al., 2013; Wang et al., 2013; Yu et al., 2013; Dai et al., 2013; Dai, 2014; Dai, 2014; Yang et al., 2014; Dai et al., 2016; Jia et al., 2018; Fu et al., 2019; Guo et al., 2020; Dai et al., 2021; Jia et al., 2021), but relatively little work has been done on the genesis and sources of natural gases by using the full components and isotopes of noble gases and C8 light hydrocarbons. Hence, the authors intend to systematically analyze the hydrocarbon, non-hydrocarbon, noble gas components, and isotopic characteristics of natural gases in the Sulige gas field based on a large amount of alkane gases, non-hydrocarbon gases, noble gases, and light hydrocarbons, to comprehensively identify the natural gas genesis in the largest tight gas field from the perspectives of alkane gases, noble gases, and C8 light hydrocarbons, and finally to explore the natural gas sources and their contribution proportions in the Sulige tight sandstone gas field from various perspectives, such as comparison of noble gases and conventional hydrocarbon gases. This study aims to deepen the understandings of gas genesis and sources in the Sulige tight sandstone gas field, providing references for tight sandstone gas field exploration in China.
The Ordos Basin is a multi-rotation evolutionary craton basin with stable subsidence and depressional migration, covering an area of about 37×104 km2, which is ranked the second largest oil and gas-bearing basin in China after Tarim Basin (the distribution area of Paleozoic sedimentary rocks is about 25×104 km2). In general, it has the characteristics of active surrounding structures, developed faults, stable internal structures, high in the West and low in the East, and gently inclined to the West. Tectonically, the Ordos Basin can be divided into six primary tectonic units (Figure 1), namely, Yimeng Uplift, Weibei Uplift, Western Shanxi Flexural Fold Belt, Yishan Slope, Tianhuan Depression, and the Western Margin Thrust Belt, and several secondary tectonic units. On the basis of Archean and Lower Proterozoic metamorphic rock basement, the basin has undergone five stages of tectonic evolution: Middle and Late Proterozoic Aula Valley, Early Paleozoic Shallow sea platform, Late Paleozoic Coastal Plain, Mesozoic Inland Basin, and Cenozoic Peripheral Fault Depression (Compilation group of petroleum geology of Changqing Oilfield, 1992; Yang and Pei, 1996; Kang et al., 2000; Liu et al., 2000; Yang et al., 2000; He et al., 2003; Yang et al., 2006; Hu, 2009).
FIGURE 1. Geological unit classification and the Sulige gas field distribution in Ordos Basin (Dai, 2014; Fu et al., 2019, Modifed).
The Ordos Basin is mostly developed from bottom to top with the Middle and Upper Paleozoic Changcheng and Jixian; Lower Paleozoic Cambrian and Ordovician; Upper Paleozoic Carboniferous Benxi Formation; Permian Taiyuan Formation; Shanxi Formation; Shihezi Formation and Shiqianfeng Formation; Mesozoic Triassic, Jurassic, and Cretaceous; Cenozoic Tertiary and Quaternary; missing Upper Ordovician; and Silurian and Devonian, and the average thickness of sedimentary rocks reaches 6000 m (Hu, 2009). Marine carbonate deposits are well developed in Lower Paleozoic, and fluvial and lacustrine clastic deposits are well developed in Upper Paleozoic and Mesozoic. The Shanxi Formation of Lower Permian is divided into Shan 2 and Shan 1 members from bottom to top. The Shan 2 member which is a set of deltaic coal-formed clastic sediments, with lithology mostly of clastic sandstones or quartz sandstones, interspersed with thin layer of siltstones, mudstones, and coal seam, is one of the main gas layers in Upper Paleozoic. The Lower Shihezi Formation of Middle Permian is a set of stable distribution of fluvial–deltaic sediments and can be divided into He 8, He 7, He 6, and He 5 members. The He 8 member which is mostly composed of quartz sandstones and mudstones with unequal thickness alternate layer is one of the main gas layers in Upper Paleozoic. The Carboniferous-Permian coal measures hydrocarbon source rocks of Upper Paleozoic, with a thickness of 60–100 m, high organic matter content, high thermal evolution from high to over mature stage, and strong generation capacity are widely covered in the whole basin. The early Cretaceous is the main gas generation period for the Carboniferous-Permian coal measures hydrocarbon source rocks. The source rocks are in the high over mature stage and enter the peak of gas generation, which provides sufficient gas source for the formation of Paleozoic gas reservoir. The vertical superposition relationship between Permian-Carboniferous coal measures hydrocarbon source rocks and main reservoir such as He 8 and Shan 1 members of Permian are favorable for vertical migration and accumulation of natural gases generated in the peak of hydrocarbon generation of the source rocks. On the one hand, driven by the residual pressure of the source reservoir, the natural gases generated from the underlying Carboniferous-Permian coal measure source rocks accumulate and dissipate to the overlying Permian reservoir with the main mechanism of volumetric flow Darcy or non-Darcy seepage migration. On the other hand, due to the differences of hydrocarbon concentration caused by hydrocarbon generation, the natural gases generated in the source rock continuously diffuses to the overlying low porosity and permeability tight sandstone reservoir driven by concentration gradient (Wang et al., 2014; Wang et al., 2015). In addition, Upper Shihezi Formation has a stable distribution of fluvial and lacustrine mudstones with a thickness of about 60–120 m and constitutes the regional cover of Upper Paleozoic gas reservoir. The overlying mudstones and updip directional dense sandstones provide good direct covering and lateral sealing abilities (Yang and Pei, 1996; Kang et al., 2000; Yang et al., 2006; Hu, 2009).
The Sulige gas field is located in the north central part of Ordos Basin, tectonically in the northwestern part of the Yishan Slope, straddling three regional tectonic units: Yishan Slope, Yimeng Uplift, and Tianhuan Depression. Its administrative area is located in the Inner Mongolia Autonomous Region and the Shaanxi Province, consisting of four blocks: Central, West, East, and South. The Paleozoic strata of the Sulige gas field are lack of middle and Upper Ordovician, Silurian, Devonian, and Lower Carboniferous. The Majiagou Formation of Lower Ordovician, Benxi Formation of Upper Carboniferous, Taiyuan Formation and Shanxi Formation of Lower Permian, Lower Shihezi Formation of Middle Permian, and Upper Shihezi Formation and Shiqianfeng Formation of Upper Permian are developed from bottom to top. The Sulige gas field is mostly developed in Upper Paleozoic, and its main reservoir is the He 8 member of Middle Permian Lower Shihezi Formation of Middle Permian and the Shan 1 member of Shanxi formation of Lower Permian, with a depth of 3,200–3,500 m and a thickness of 80–100 m, followed by Shan 2 member of Shanxi Formation of Lower Permian and Ma 5 member of Majiagou Formation of Lower Ordovician. The Sulige gas field is generally characterized by a large gas-bearing area, many formations, thin single layer thickness, poor reservoir properties, strong non-homogeneity, generally low porosity and permeability, low pressure coefficient, low reserve abundance, and low production. It is a typically large distributed dense sandstone gas reservoir with low permeability, low pressure, low abundance, and river sand body as the main reservoir. In 2000, the Su6 well obtained a high production rate of 120×104 m3 per day in Shihezi Formation, which kicked off the large-scale exploration of the Sulige gas field and marked a major discovery (Dai, 2014). Since 2007, the Sulige gas field has insisted on the integration of exploration and development, and the scale of new gas reserves exceeded 5,000×108 m3 per year for seven consecutive years (Yang and Liu, 2014). At present, the exploration area of the Sulige gas field is about 5.5×104 km2, with total gas resources of 6×1012 m3, proven gas-bearing area is 3.88×104 km2, accumulated tertiary reserves reaches 4.77×1012 m3, and the natural gas production reaches 2.7×1010 m3 in 2020, which becomes a key area for increasing natural gas storage and production in the Changqing Oilfield and an important natural gas gathering area in China (Fu et al., 2019; Jia et al., 2018; Jia et al., 2021). It makes a significant contributions on guaranteeing a safe and stable gas supply in the Capital, North China, and areas around the field.
The noble gas content in natural gas is low but relatively high in air (especially argon content is as high as 0.934%), so it can easily be contaminated, which can lead to experimental analysis data to severely deviate from the real value. In order to minimize the contamination of noble gases in air, special measures are required for sampling natural gases for noble gases analysis (Wang et al., 2013; Wang et al., 2016; Wang et al., 2018):① choose a double valve high pressure–resistant steel cylinder; ② evacuate a sampling steel cylinder to below 10−1 Pa using a mechanical pump; ③ repeatedly flush the steel cylinder with natural gas for 4–6 times during wellhead sampling and intercept the middle section of gas flow. For the aforementioned noble gas analysis and testing requirements, more than 50 gas samples were collected from the Sulige gas field in Ordos Basin using high-pressure cylinder sampling, involving Permian Taiyuan Formation, Shanxi Formation, Shihezi Formation, and Shiqianfeng Formation.
The sample collection tests were completed at the Key Laboratory of Gas Formation and Development of China National Petroleum Corporation (CNPC).
(1) The natural gas component analysis was performed using an Agilent 7890A gas chromatograph, separating components by using a PLOT Al2O3 capillary column with a specification of 50 m × 0.20 mm × 0.5 μm. The sample inlet temperature was 300°C, carrier gas was helium, flow rate was 1 ml/min, splitting ratio was 50:1, and the temperature program was set to keep 10 min from 30°C, then the temperature was increased to 260°C at the heating rate of 3°C/min.
(2) Noble gas components and isotopes were analyzed using a noble gas sampling system and a Noblesse isotope mass spectrometer. The high vacuum of the system was achieved by mechanical, molecular, and ion pumps, and the sample volume of natural gas samples was controlled by an injection control system; a zirconium-based furnace and a suction pump were used to purify hydrocarbon gas, nitrogen (N2), oxygen (O2), carbon dioxide (CO2), hydrogen sulfide (H2S), trace hydrogen (H2), and other active gases in natural gas samples; enrich noble gases; measure the content of noble gas components; and further separate components according to different boiling points of noble gases. The noble gas isotope composition was determined based on the ion flow signal intensity peak height ratio method by sending it to the Noblesse noble gas isotope mass spectrometer for isotope analysis. The relative deviations of the noble gases helium, neon, argon, krypton, and xenon were ±3.36%, ±3.66%, ±1.32%, ±2.99%, and ±6.96%, respectively, and the relative deviations of the noble gases 3He/4He, 20Ne/22Ne, 21Ne/22Ne, 40Ar/36Ar, 38Ar/36Ar, 129Xe/130Xe, and 132Xe/130Xe isotopes were ±4.50%, ±1.32%, ±1.27%, ±1.39%, ±1.63%, ±1.84%, and ±2.13%, respectively.
(3) A natural gas carbon isotope analysis was performed using the Finnigan MAT Delta PLUS GC/C/IRMS isotope mass spectrometer. The gas was separated by gas chromatography, converted to CO2, and then fed into IRMS for carbon isotope determination. The chromatographic column was PLOT Q (30 m × 0.32 mm × 20 mm). The temperature rise procedure of chromatographic column was heating up from 35°C to 80°C at the rate of 8°C/min, then to 260°C at the rate of 5°C/min, and finally, kept at a constant temperature for 10 min. Each sample was analyzed three times, and the analytical standard was VPDB with an analytical accuracy of ±0.5%.
(4) The chromatographic separation column was HP PLOT Q (30 m × 0.32 mm × 20 mm). The carrier gas was helium, and the temperature rise procedure of methane column was heating up from 35°C to 80°C at the rate of 8°C/min, then to 260°C at the rate of 5°C/min, and finally, kept at a constant temperature for 10 min. Each sample was analyzed three times, and the analytical standard was SMOW with an analytical accuracy of ±0.5%.
(5) A natural gas light hydrocarbon analysis was performed by using a gas chromatograph 7890A with helium as the carrier gas and the PLOT Al2O3 capillary column of 50 m × 0.20 mm × 0.5 μm for component separation, the inlet temperature was 120°C, and liquid nitrogen cold trap for light hydrocarbon enrichment for 5 min. FID detector temperature was 320°C, the injection volume was 10–15 ml, and the ramp-up program was initiated. The initial temperature of the rise procedure was 30°C, kept at constant temperature for 15 min, and then the rise procedure was l.5°C/min to 70°C, 3°C/min to 160°C and 5°C/min to 300°C and kept at a constant temperature for 20 min, respectively.
The alkane gas content of natural gas in the Sulige gas field occupies an absolute advantage. The highest methane content is mostly distributed in the range of 83.77%–93.87%, with an average of 91.15%. Ethane is mostly distributed in the range of 1.12%–8.30%, with an average of 4.21%. Propane is mostly distributed in the range of 0.15%–2.45%, with an average of 0.84%. The drying factor (C1/C1+) is mostly distributed in the range of 0.854–0.984, with an average of 0.940 (Table 1). The non-hydrocarbon gas nitrogen (N2) content was mostly distributed in the range of 0.60%–6.72%, with an average value of 1.62%, and carbon dioxide (CO2) content was mostly distributed in the range of 0.30%–2.97%, with an average value of 1.38%, without H2S.
TABLE 1. Data table of hydrocarbon, non-hydrocarbon, and noble gas components in some natural gas samples from the Sulige gas field in Ordos Basin.
Compared with hydrocarbon and non-hydrocarbon gases, the contents of noble gases in natural gas are relatively low. In general, the distribution of noble gas components in natural gases from the Sulige field in Ordos Basin has many characteristics (Table 1; Figure 2). 1) The helium content of natural gas samples is mostly distributed around (228.8–762.5)×10−6, with an average value of 549.1×10−6, which is about two orders of magnitude higher than the atmospheric value of 5.24×10−6 in general. 2) The neon content in natural gas samples is around (4.27–7.84) ×10−6, with an average value of 6.16×10−6, which is about 1/3 of the atmospheric value of 18.18×10−6. 3) The argon content in natural gas samples is around (20.2–213.4)×10−6, with an average value of 64.0×10−6, which is about two orders of magnitude lower than the atmospheric value of 0.934%. 4) The krypton content in natural gas samples is around (0.0103–0.0558)×10−6, with an average value of 0.0244×10−6, which is about two orders of magnitude lower than the atmospheric content value of 1.14×10−6. 5) The xenon content in natural gas samples is around (0.0043–0.0123)×10−6, with an average value of 0.0070×10−6, which is about one order of magnitude lower than the atmospheric content value of 0.078×10−6. Compared with noble gases data of previous researchers on the Weiyuan gas field in Sichuan Basin and Changshen and Xushen gas fields in Songliao Basin (Table 1), the helium content of Sulige is obviously less than that of Weiyuan and relatively larger than Changshen and Xushen, while the argon content in Weiyuan is relatively larger than that of Sulige, Changshen, and Xushen.
FIGURE 2. Components abundance of noble gas He, Ne, Ar, Kr, and Xe of natural gases in the Sulige gas field in Ordos Basin.
The carbon isotope analysis data of alkane gas components in the Sulige gas field are shown in Table 2. The methane carbon isotope δ13C1 values of natural gas samples are distributed in the range of −36.7‰ ∼ −27.6‰, with main values ranging from −35‰ ∼ −30‰ and an average value of −32.3‰. Ethane carbon isotope δ13C2 values are distributed in the range of −27.9‰ ∼ −22.4‰, with main values ranging from −26‰ ∼ −23‰ and an average value of −24.1‰. Propane carbon isotope δ13C3 values are distributed in the range of −28.9‰ ∼ −21.9‰, with the main values ranging from −28‰ ∼ −23‰ and an average value of −24.7‰. The n-butane carbon isotope δ13 nC4 values are distributed in the range of −24.1‰ ∼ −19.7‰, with main values ranging from −24‰ ∼ −21‰ and an average value of −22.5‰. The isobutane carbon isotope δ13 iC4 values are distributed in range of −24.2‰ ∼ −20‰, with main values ranging from −23‰ ∼ −20‰ and an average value of −21.6‰. The carbon dioxide isotope values of δ13CCO2 in the Sulige gas field are mostly distributed in the range of −15.3‰ ∼ −5‰, with an average value of about −10.3‰. For the hydrogen isotopes of alkane gases in the Sulige gas field, the δ2H1 values are generally distributed in the range of −203‰ ∼ −171‰, with an average of −185.3‰; the δ2H2 values are usually distributed in the range of −168‰ ∼ −151‰, with an average of −159.6‰; and the δ2H3 values are commonly distributed in the range of −165‰ ∼ −150‰, with an average of −157.6‰ (Table 3).
The carbon isotopes of alkane gases of organic origin have the characteristic of increasing the δ13C value with increasing carbon number (δ13C1<δ13C2<δ13C3<δ13C4), which is called a positive carbon isotope sequence of alkane gases; when the carbon isotope arrangement of alkane gases appears chaotic, it is called carbon isotope inversion; when δ13Ci>δ13Ci+1, it is called partial inversion of carbon isotope sequence; and when δ13C1>δ13C2>δ13C3>δ13C4 appears completely, it is called negative carbon isotope sequence of alkane gas (Dai, 2014). The carbon isotope sequence of alkane gases in Sulige gas samples generally show positive carbon isotope sequence of δ13C1<δ13C2<δ13C3<δ13C4, and some samples show δ13C2>δ13C3 inversion of carbon isotope sequence (Figure 3A).
FIGURE 3. Folding line of carbon (A) and hydrogen (B) isotope series of natural gas alkane from the Sulige gas field in Ordos Basin.
Similarly, the hydrogen isotopes of alkane gas of organic origin also have the characteristics of increasing the δ2H value with increasing carbon number (δ2HCH4<δ2HC2H6<δ2HC3H8), which is called positive hydrogen isotope sequence of alkane gas; when the carbon isotope arrangement of alkane gas appears chaotic, it is called hydrogen isotope inversion; when δ2HCiHi+2>δ2HCi+1 H2i+4 appears, it is called partial inversion of hydrogen isotope sequence; and when 2HCH4>δ2HC2H6>δ2HC3H8 appears completely, it is called negative carbon isotope sequence of alkane gas (Dai, 2014). The hydrogen isotopes of alkane in natural gas samples from the Sulige field generally show the characteristics of δ2HCH4<δ2HC2H6<δ2HC3H8 positive hydrogen isotope sequence, and some of samples show δ13C2>δ13C3 inversion of alkane gas hydrogen isotopes (Figure 3B).
Stable carbon isotope values are the most reliable and commonly used methods for natural gas genesis, with methane isotope values generally ranging of −55‰ ∼ −35‰ for oil-typed gas and −35‰ ∼ −22‰ for coal-formed gas. Since methane carbon isotopes are strongly influenced by the degree of thermal evolution of source rocks, ethane isotopes are usually little influenced by the maturity of thermal evolution of source rocks and have good parent material inheritance effects, so the ethane carbon isotope value of δ13C2 = −28.5‰ is generally used as the threshold for determining oil-typed gas and coal-formed gas (Dai et al., 1989; Dai, 1992, 2014). The δ13C2 values of natural gas from the Sulige gas field in Ordos Basin are distributed in the range of −27.9‰ ∼ −22.4‰, with main values ranging from −26‰ ∼ −23‰ and an average value of −24.1‰. The natural gas δ13C2 is significantly heavier than −28.5‰, which is significantly different from oil-typed gas, and can be identified as typical coal-formed gas based on ethane carbon isotopes. According to carbon isotopes of methane, ethane, and propane proposed by Dai (1993), the majority of the carbon isotope data points of the gas samples distribute in coal-formed gas area, and some of points fall in or near mixed carbon isotope inversion series area (Figure 4), indicating that natural gases from the Sulige gas field is generally coal-formed gas.
FIGURE 4. Identification of δ13C1-δ13C2-δ13C3 alkane gas genesis in the Sulige gas field, Ordos Basin (plate According to Dai, 1993; Modified).
CO2 is an important non-hydrocarbon gas component in natural gases. The CO2 content in the Sulige gas field mostly distributes in the range of 0.30%–2.97%, and the average value is 1.38%. The δ13CCO2 is mostly distributed in the range of −15.3‰ ∼ −5‰, and the average value is −10.3‰. 1) When the CO2 content is less than 15%, δ13CCO2 < −10‰ is organic CO2; 2) when δ13CCO2 ≥ −8‰, all are inorganic CO2; and 3) when the CO2 content is greater than 60%, all are inorganic CO2 (Dai et al., 1989; Dai, 1992). According to the identification chart of different CO2 genesis by Dai et al. (1992) (Figure 5), the CO2 in the Sulige gas field is mostly organic in general with small part inorganic.
FIGURE 5. Identification of organic and inorganic carbon dioxide in natural gases from the Sulige gas field (plate according to Dai, 1989).
(1) The 3He/4He values (R) of He in natural gas samples from the Sulige gas field mostly distribute around (2.89–7.30)×10−8 (0.021–0.052Ra), with an average of 4.81×10−8 (0.034Ra). From the He-R/Ra genesis identifications of noble gases in natural gas, it can be found that the 3He/4He values of natural gas in the Sulige gas field generally distribute between 0.01<R/Ra<0.10, and the sample points all fall in a typical crust genesis area (Table 4; Figure 6). It indicates that noble gas helium in natural gas from the Sulige gas field is mostly of typical crustal genesis, and the proportion of crustal genesis helium is greater than 98.8%, originating from the decay of crustal genesis radioactive elements U and Th.
FIGURE 6. Genesis identification chart of He and R/Ra of natural gases in the Sulige gas field, Ordos Basin (Plate according to Wang et al. 2013, Wang et al. 2016).
(2) From the noble gas Ne-20Ne/22Ne, 20Ne/22Ne-21Ne/22Ne, and 3He/4He-20Ne/22Ne genesis identifications (Figure 7), it can be found that the 20Ne/22Ne values of natural gas samples from the Sulige gas field are mostly distributed in the range of 9.56–9.74, and 21Ne/22Ne values are mostly distributed in the range of 0.0295–0.0315. The 20Ne is relatively deficient, while the 21Ne and 22Ne are relative excess. It indicates that neon in natural gas from the Sulige gas field is dominated by typical crustal genesis, which is a result of variable depositional environments of uranium, thorium, oxygen, and fluorine elements in crust. The 20Ne/22Ne ratios of Changshen and Xushen gas fields are larger than 9.8, reflecting the relative atmospheric excess of 20Ne. It indicates that neon in natural gas from Changshen and Xushen fields has significant mantle genesis neon mixing characteristics.
FIGURE 7. Genesis identification chart of Ne and 20Ne/22Ne (A), 20Ne/22Ne and 21Ne/22Ne (B), and 3He/4He and 20Ne/22Ne (C) of natural gases in the Sulige gas field in Ordos Basin (Plate according to Wang et al. 2013, Wang et al. 2016).
(3) The 40Ar/36Ar values of natural gas samples from the Sulige gas field are relatively high and widely distributed, mostly in the range of 506–1940, with an average value of 1148, while the 38Ar/36Ar values are mostly distributed in range of 0.1709–0.1959, with an average value of 0.1909 (Table 4). According to the 3He/4He–40Ar/36Ar genesis identification chart, it can be found that the noble gas 3He/4He values in the Sulige gas field generally distribute around (2.89–7.30)×10−8, with an average value of 4.81×10−8, and the 3He/4He and 40Ar/36Ar have an overall negative correlation growth. It shows that helium and argon in natural gases from the Sulige gas field have the characteristics of typical crustal origin genesis (Figure 8). In contrast to Yingcheng Formation in Changshen and Xushen gas fields, the 3He/4He is larger than 1.4×10−6 and the 3He/4He is positively correlated with 40Ar/36Ar, indicating that the noble gases have significant mantle genesis mixing characteristics.
FIGURE 8. Genesis identification chart of 3He/4He and 40Ar/36Ar of natural gases in the Sulige gas field in Ordos Basin (Plate according to Wang et al. 2013, Wang et al. 2016).
(4) The 129Xe/130Xe values of natural gas in the Sulige gas field are mostly distributed in the range of 6.362–6.479 with an average value of 6.436, while the 132Xe/130Xe values are mostly distributed in the range of 6.629–6.731 with an average value of 6.670 (Table 4). According to the noble gas 129Xe/130Xe–132Xe/130Xe genesis identification, it can found that the 129Xe in the Sulige gas field is generally deficient relative to atmosphere and the 132Xe is excess relative to atmosphere, and the gas sample points fall into a crustal genesis region (Figure 9), indicating that the noble gas xenon in natural gas is mostly of crustal genesis. While the 129Xe in Changshen and Xushen gas fields in Songliao Basin is excess relative to the atmosphere, which has the characteristics of significant mantle genesis mixing.
FIGURE 9. Genesis identification chart of 129Xe/130Xe and 132Xe/130Xe of natural gases in the Sulige gas field in Ordos Basin (Plate according to Wang et al. 2013, Wang et al. 2016).
Light hydrocarbons are C5–10 hydrocarbon compounds with a boiling point less than 200°C, including n-alkanes, isoalkanes, cycloalkanes, and aromatic compounds, which are very important components of natural gas and crude oil and contain rich geochemical information. The geochemistry of light hydrocarbons can be used to study the parent material types and genesis, depositional environments, maturities, and secondary migration of natural gases. Some researchers have established genetic identification indexes and charts for the composition of C7 light hydrocarbon series (n-heptane, methylcyclohexane, and dimethylcyclopentane), C5–7 aliphatic compositions (n-alkanes, isoalkanes, and cycloalkanes), light hydrocarbon monomer hydrocarbon carbon isotope ratios (benzene, toluene, cyclohexane, and methylcyclohexane), C6–7 aromatic and branched alkane combinations, benzene and toluene content, methylcyclohexane, and aromatic alkane—paraffin—to identify coal-formed and oil-typed gas (Leythauser et al., 1979; Leythauser et al., 1980; Hu et al., 1990; Dai, 1992; Dai, 1993; Jiang et al., 1999; Li et al., 2001; Li et al., 2003; Hu et al., 2007; Hu et al., 2010; Hu et al., 2012). At present, the studies of light hydrocarbons in natural gases have become a hot spot in the field of oil and gas geology. C5–7 and C7 light hydrocarbon compositions have been widely used in oil and gas exploration and geological studies; however, few researches have been conducted on C8 light hydrocarbon compounds. There is a lack of methods to identify the genesis and sources of coal-formed gas and oil-typed gas by using C8 light hydrocarbon parameters. In this study, based on the researches of C5–7 and C7 light hydrocarbons, the distribution characteristics and genetic identifications of C8 light hydrocarbons were carried out.
The C7 light hydrocarbon compounds include n-heptane, methylcyclohexane, and dimethylcyclopentane. The n-heptane is mostly from algae and bacteria, which is a good maturity indicator. The methylcyclohexane is mostly from higher plant lignin and cellulose, which is a good parameter to reflect the type of terrestrial parent material. The dimethylcyclopentane is mostly from lipid-like compounds of aquatic organisms, which is a characteristic of oil-typed gas light hydrocarbons (Chung et al., 1998; Chung et al., 1991; Whiticar and Snowdon, 1999). Hu et al. (2007) analyzed 173 coal-formed gas samples in China and found that 92% of the gas samples had a methylcyclohexane volume fraction greater than 50%. Therefore, the dominant distribution of methylcyclohexane in a C7 light hydrocarbon system is a major characteristic of coal-formed light hydrocarbons. According to the ternary diagram of relative content of the C7 light hydrocarbon compounds system of natural gases from the Sulige gas field (Figure 10A), the methylcyclohexane is distributed in the range of 53.9%–77%, with an average of 66%; the n-heptane is distributed in the range of 9.6%–28.6%, with an average of 15%; the dimethylcyclopentane is distributed in the range of 11.5%–30%, with an average of 19%. All gas samples of the Sulige gas field is distributed in the lower left corner and show that methylcyclohexane content is dominant. So, the natural gases from the Sulige gas field are typical coal-formed gas mostly generated from humic parent material.
FIGURE 10. Ternary diagram of the C7 compositions (A) and C5–7 (B) light hydrocarbons system in the Sulige gas field (Plate according to Hu et al., 2007)
The ternary diagram of relative contents of C5–7 aliphatic composition of n-alkanes, isoalkanes, and cycloalkanes is also commonly used to identify different types of natural gases. According to the ternary diagram of C5–7 aliphatic composition of n-alkanes, isoalkanes, and cycloalkanes of natural gases from the Sulige gas field (Figure 10B), it can be seen that n-alkane content of Sulige natural gas samples in the range of 12.2%–25.9%, with an average of 19.2%; isoalkane content in the range of 21%–62.1%, with an average of 49%; and cycloalkane content in the range of 14.5%–68%, with an average of 31.8%. The C5–7 aliphatic compositions show that natural gases from the Sulige gas field are rich in isoparaffins and cycloalkanes, indicating that natural gases from the Sulige gas field are typical coal-formed gases mostly originated from humic parent material.
Previous studies suggest that the light hydrocarbon fraction of humic parent material is rich in isoalkanes and aromatics (Leythaeuser et al., 1979; Leythaeuser et al., 1980), and the light hydrocarbon fraction of condensate from terrestrial parent material is rich in cycloalkanes (Snowdon and Powell, 1982). Therefore, the relative content of C8 aliphatic compositions of n-alkanes, isoalkanes, and cycloalkanes can also be used to identify natural gas genesis. In this study, based on a large number of experimental analysis and statistics of light hydrocarbons, the following indicators and ternary diagram were established for the identification of coal-formed gas and oil-typed gas by the relative contents of C8 light hydrocarbon n-alkane, isoalkane, and cycloalkane compositions. 1) When 0 ≤ C8 n-alkane relative content ≤ 20%, 20% ≤ C8 cycloalkane relative content ≤ 100%, and 0% ≤ C8 isomeric alkane relative content ≤ 70%, natural gas is generally coal-formed gas. 2) When 20% ≤ C8 n-alkane relative content ≤ 100%, 0 ≤ C8 cycloalkane relative content ≤ 50%, and 20% ≤ C8 isoalkane relative content ≤ 60%, natural gas is generally oil-typed gas. According to the ternary diagram of C8 aliphatic compositions for natural gases from the Sulige gas field (Figure 11A), n-alkane content of natural gas samples is in the range of 4%–18.2%, with an average of 7.9%; isoalkane content in the range of 10%–64.9%, with an average of 52.6%; and cycloalkane content in the range of 21.4%–86.3%, with an average of 39.5%. The C8 aliphatic compositions show that natural gases from the Sulige gas field is rich in isoalkanes and cycloalkanes, indicating that natural gases from the Sulige gas field are typical coal-formed gases generated from humic parent material.
FIGURE 11. Ternary diagram of C8 light hydrocarbon aliphatic (A) and C8 light hydrocarbon compounds (B) in natural gases from the Sulige gas field.
C8 light hydrocarbon system compounds include cis-1,3-dimethylcyclohexane, n-octane, and 2-methylheptane. Dimethylcyclohexane is mostly derived from lignin and cellulose of higher plants, reflecting the characteristics of terrestrial parent material. While n-octane is mostly from algae and bacteria, 2-methylheptane is mostly from lipid-like compounds of aquatic organisms, both reflecting the characteristics of oil-typed gas light hydrocarbons. Similarly, we also established a ternary diagram to identify coal-formed gas and oil-typed gas by the relative contents of cis-1,3-dimethylcyclohexane, n-octane, and 2-methylheptane of C8 light hydrocarbon system compounds: 1) when 40% ≤ cis-1,3-dimethylcyclohexane relative content ≤ 100%, n-octane relative percentage content ≤ 40%, and 2-methylheptane relative percentage content ≤ 40%, natural gas is generally coal-formed gas. 2) When 0 ≤ cis-1,3-dimethylcyclohexane relative content ≤ 40%, 30% ≤ n-octane relative percentage ≤ 100%, and 0 ≤ 2-methylheptane relative percentage ≤ 50%, natural gas is generally oil-typed gas. According to the ternary diagram of C8 light hydrocarbon cis-1,3-dimethylcyclohexane, n-octane, and 2-methylheptane in the Sulige gas field, the relative contents of cis-1,3-dimethylcyclohexane, n-octane, and 2-methylheptane are 31.1%–92.2%, with an average of 56.6%; 2-methylheptane is 3.3%–36.3%, with an average of 20.1%; n-octane is 4.5%–46.9%, with an average of 23.3%; and n-octane is 4.5%–46.9%, with an average of 20.1%. The relative contents of 2-methylheptane is in the range of 3.3%–36.3%, with an average of 20.1%; the relative contents of n-octane is in the range of 4.5%–46.9%, with an average of 23.3%. Therefore, the aliphatic compositions of the C8 light hydrocarbon compounds in the Sulige gas field is rich in cis-1,3-dimethylcyclohexane. The gas samples from the Upper Paleozoic tight gas field in the Sulige gas field fall into the coal-formed gas region (Figure 11B) and show typical coal-formed gas generated from humic parent material.
In total, two sets of source rocks are developed in Paleozoic of Ordos Basin: Carboniferous-Permian coal measure source rocks of Upper Paleozoic and marine carbonate source rocks of Lower Paleozoic. The Carboniferous-Permian coal measure source rocks are considered as the main source rocks in Ordos Basin (Chen et al., 1993; Dai et al., 1996; Dai et al., 2003; Dai et al., 2005). The coal measure source rocks of Carboniferous-Permian in Ordos Basin are widely distributed throughout the basin, mainly including coals and dark mudstones in Shanxi Formation, Taiyuan Formation, and Benxi Formation. The coals of Benxi, Taiyuan, and Shanxi Formations basically cover the whole basin and are main source rocks of Upper Paleozoic. The cumulative thickness of coals ranges from 4 to 40 m. Dark mudstones are developed in Benxi, Taiyuan, and Shanxi Formations, mainly in Taiyuan Formation and Shan2 member of Shanxi Formation, and widely distribute in upper Paleozoic with total thickness from 20 to 80 m. In Yan’an-Wuqi area in the southern part of Ordos Basin, the highest thermal evolution maturity of source rocks reach 2.8%, decreasing in a ring-like pattern toward north and south and basin margin. The thermal evolution maturity of most parts of the basin are above 1.5%, generally entering high mature to over mature stage.
According to the genetic identification, the natural gases of the Sulige gas field are typical coal-formed gas, mainly from coal measure source rocks of humic parent material. Combing the typical characteristics of existed Paleozoic source rocks in Ordos Basin, it is concluded that the natural gases of the Sulige gas field originate from Carboniferous-Permian coal measure source rocks. Furthermore, by calculating the natural gas maturity of the Sulige gas field, the calculated Ro values of natural gas maturity are mainly distributed from 1.0% to 2.0%, and the maturity of natural gas in southern part of the Sulige gas field is relative higher, while the maturity of natural gas in northern part is relative lower, having a similar trend with maturity of Carboniferous-Permian coal measure source rocks in Ordos Basin. It indicates that the natural gas in the Sulige gas field mainly originates from Carboniferous-Permian coal measure source rocks and also reflects that the natural gas in the Sulige gas field is mainly generated from near source rocks. The coal measure source rocks of Carboniferous-Permian in Ordos are characterized by widespread hydrocarbon generation, and the area with hydrocarbon generation intensity greater than 12×108 m3/km2 accounting for 71.6% of the total basin. The source rocks of the Sulige gas field and its nearby area have a gas generation intensity of (12–30)×108 m3/km2 (Li et al., 2012; Zhao et al., 2013a).
Therefore, the natural gases from the Sulige gas field are typical coal-formed gas in high mature to over mature stage, mainly generating from coal measure source rocks of Upper Paleozoic Carboniferous-Permian in Ordos Basin.
Based on the noble gas isotope analysis, the gas source comparison study of Ordos Sulige gas was further conducted by using helium and argon isotopes and argon isotope age accumulation effect. The 3He/4He values (R) of gas samples from the Sulige gas field mainly distribute around (2.89–7.30)×10−8 (0.021∼0.052Ra),with an average value of 4.81×10−8 (0.034Ra), which is in the order of 10−8 with an overall distribution in 0.01<R/Ra<0.10. It shows that noble gas helium in natural gas of the Sulige gas field is a typical crustal source genesis, and the proportion of crustal source contribution is more than 98.8%, mainly coming from decay of crustal source radioactive elements U and Th. Therefore, comparative gas source studies can be performed by using natural gas argon isotope age accumulation effect. The 40Ar/36Ar values of gas samples from the Sulige gas field are relatively high and widely distributed, mainly in the range of 506–1940, with an average value of 1148. The average value is in the range of 920–1450 for natural gas generated from Carboniferous-Permian source rocks (Shen et al., 1995). Furthermore, based on the relationship between argon isotopes of clastic strata and parent source stratigraphic age proposed by Xu et al. (1996), parent source stratigraphic age of natural gases in the Sulige gas field is estimated to be about 299 Ma, which is in the range of 250∼355 Ma for Carboniferous-Permian coal measure source rocks in Upper Paleozoic of Ordos Basin. Therefore, the natural gases in the Sulige gas field mainly originate from Carboniferous-Permian coal measure source rocks of Upper Paleozoic. The comparative study of noble gas source correlations in the Sulige gas field by using age accumulation effect of argon isotopes of natural gas provides evidences for gas source correlation from a noble gas point of view, which is consistent with the results obtained by conventional gas source correlation methods by alkane gas isotopes, further deepening the understandings of gas sources in the Sulige gas field.
Since coal measure source rocks usually contain coals and mudstones, the average content of K in Chinese coals is about 0.214% and the average content of potassium in Chinese mudstones is about 2.67%. Due to great differences on potassium content between coals and mudstones, the 40Ar/36Ar values in natural gas produced from coals is relatively low and in natural gas produced from mudstones in relatively high (Liu and Xu, 1987, 1993; Zhang et al., 2005). Therefore, the source contribution proportions of coals and mudstones can be quantitatively studied by the noble gas argon isotope 40Ar/36Ar. The distribution of 40Ar/36Ar in the natural gases of the Sulige gas field ranges from 506 to 1940, and the average value is about 1148. The minimum value of 40Ar/36Ar in Upper Paleozoic natural gas samples of the Sulige gas field is approximated as the end element value of natural gas generated from coals, and the maximum value of 40Ar/36Ar in Upper Paleozoic natural gas samples of the Sulige gas field is approximated as the end element value of natural gas generated from mudstones. So, the source contribution proportions of coals and mudstones in Carboniferous-Permian coal measure source rocks are calculated as 55% and 45%, respectively, by using noble gas argon isotope of the 40Ar/36Ar method.
In addition, the contribution proportions of coals and mudstones in the coal measure source rocks of the Sulige gas field was also quantitatively investigated by using the alkane gas methane carbon isotope method. By comparing the data with previous analyses, the heaviest methane carbon isotope δ13C1 of the positive sequence of alkane gases carbon isotopes in the Sulige gas field analyzed here is −28.4‰, which can be approximated as the methane carbon isotope end element value of natural gas from coals. The lightest methane carbon isotope δ13C1 with positive sequence of alkane gas isotope in Upper Paleozoic of Ordos Basin was found to be −38.1‰ (Dai, 2014), which can be used as the approximate end element value of methane carbon isotope for natural gas production from mudstones. In this study, the average value of methane carbon isotope δ13C1 in the Sulige gas field is about −32.3‰, and the contribution of coals and mudstones in Carboniferous-Permian coal measure source rocks is calculated as 60% and 40% separately from the alkanes carbon isotope quantification.
Therefore, the Sulige gas field in Ordos Basin is mainly derived from coal measure source rocks of Carboniferous-Permian, and the contribution of coals mainly accounting for 55–60% and the contribution of mudstones accounting for 40–45%.
1. The Sulige gas field is mainly alkane gases with high methane content; low heavy hydrocarbon contents; high drying coefficient; low non-hydrocarbon gases contents, mainly CO2 and N2; and relatively low noble gas contents in general. The helium content is slightly higher than atmospheric content by about two orders of magnitude, while neon, argon, krypton, and exon contents are relatively lower than atmospheric values by about 1,2,2,1 orders of magnitude.
2. Natural gas alkane carbon and hydrogen isotopes are generally distributed in positive sequences, with a few parts inversion. The 3He/4He values are mainly distributed around (2.89–7.30)×10−8, with an average of 4.81×10−8. The 40Ar/36Ar values are distributed in a wide range, from 506 to 1940, with an average value of 1148. The 129Xe is relatively deficient, while the 132Xe is relatively surplus.
3. The carbon isotopes of alkanes in the Sulige gas field indicate that the natural gases are high mature to over mature coal-formed gas. The genetic identification of C7 and C8 light hydrocarbons reflect that the natural gases are typical coal-formed gases, mainly originating from humic source rocks. The comprehensive identification of noble gas isotopes indicate that noble gases are typical crustal genesis, mainly generated from radioactive decay of U, Th, and other elements of crust. The CO2 in the natural gases are mainly organic in general and partly inorganic.
4. The gas source correlations of noble gases and alkane gases and quantitative evaluation of gas source contributions show that the natural gases in the Sulige gas field mainly come from Carboniferous-Permian coal measure source rocks in Ordos Basin, where the contribution proportion of coals accounts for 55–60%, while the contribution proportion of mudstones accounts for 40–45%. The evaluation results of noble gas argon isotope and alkane gas carbon isotope are basically compatible, further deepening the understandings on gas sources of natural gases in the Sulige gas field.
The original contributions presented in the study are included in the article/Supplementary Material; further inquiries can be directed to the corresponding authors.
All authors listed have made a substantial, direct, and intellectual contribution to the work and approved it for publication.
This study was sponsored by the National Key Research & Development Plan Project (2021YFA0719004), the Program of PetroChina (2018D-500802, 2019B-0604, and 2016ycq03), the Major Project of National Special Science and Technology (2016ZX05007-003), and the Strategic Priority Research Program of the Chinese Academy of Sciences (XDA14010403). Thanks to reviewers for their constructive suggestions. We declare that all sources of funding received for the research being submitted in the manuscript.
All authors are affiliated with Research Institute of Petroleum Exploration and Development/PetroChina, Changqing Oilfield/Petrochina, China University of Petroleum and University of Chinese Academy of Science. The authors declare that this study received funding from National Key Research & Development Plan Project, PetroChina Company Limited, Major Project of National Special Science and Technology, Chinese Academy of Science. The funders were involved in the study design, collection, analysis, interpretation of data, the writing of this article or the decision to submit it for publication.
All claims expressed in this article are solely those of the authors and do not necessarily represent those of their affiliated organizations, or those of the publisher, the editors, and the reviewers. Any product that may be evaluated in this article, or claim that may be made by its manufacturer, is not guaranteed or endorsed by the publisher.
Allègre, C. J., Sarda, P., and Staudacher, T. (1993). Speculations about the Cosmic Origin of He and Ne in the Interior of the Earth. Earth Planet. Sci. Lett. 117, 229–233. doi:10.1016/0012-821x(93)90129-w
Allègre, C. J., Staudacher, T., Sarda, P., and Kurz, M. (1983). Constraints on Evolution of Earth's Mantle from Rare Gas Systematics. Nature 303, 762–766. doi:10.1038/303762a0
Ballentine, C. J., and O'Nions, R. K. (1992). The Nature of Mantle Neon Contributions to Vienna Basin Hydrocarbon Reservoirs. Earth Planet. Sci. Lett. 113, 553–567. doi:10.1016/0012-821x(92)90131-e
Battani, A., Sarda, P., and Prinzhofer, A. (2000). Basin Scale Natural Gas Source, Migration and Trapping Traced by Noble Gases and Major Elements: the Pakistan Indus Basin. Earth Planet. Sci. Lett. 181 (1/221), 229–249. doi:10.1016/s0012-821x(00)00188-6
Burnard, P., Zimmermann, L., and Sano, Y. (2013). “The Noble Gases as Geochemical Tracers: History and Background,” in The Noble Gases as Geochemical Tracers, Advances in Isotope Geochemistry. Editor P. Burnard (New york Dordrecht London: Springer Berlin Heidelberg), 1–15. doi:10.1007/978-3-642-28836-4_1
Chen, A. D., Zhang, W. Z., and Xu, Y. C. (1993). Isotopic Characteristics and Application of Thermal Simulation Products of Hydrocarbon Generation in Sedimentary Rocks. Chin. Sci. Part B 23 (2), 9. CNKI: SUN: JBXK.0.1993-02-014.
Chung, E., Aldom, J. E., Chagla, A. H., Kostrzynska, M., Lee, H., and Palmateer, G. (1998). Detection of Cryptosporidium Parvum Oocysts in Municipal Water Samples by the Polymerase Chain Reaction. J. Microbiol. Methods 33 (2), 0–180. doi:10.1016/s0167-7012(98)00050-5
Chung, H. M., Rooney, M. A., and Claypool, G. E. (1991). “Thermal Maturity of Oils,” in Organic Geochemistry Advances and Applications in Energy and the Natural Environment, Organic Geochemistry. 15th Meeting of European Association of Organic Geochemists Poster Abstracts. Editor D. Manning, 14.
Clarke, W. B., Jenkins, W. J., and Top, Z. (1976). Determination of Tritium by Mass Spectrometric Measurement of 3He. Int. J. Appl. Radiat. Isotopes 27, 515–522. doi:10.1016/0020-708x(76)90082-x
Compilation group of petroleum geology of Changqing Oilfield (1992). Petroleum Geology of China (Volume 12) (Changqing Oilfield). Beijing: Petroleum Industry Press, 10–90. (in Chinese).
Dai, J., Ni, Y., Huang, S., Gong, D., Liu, D., Feng, Z., et al. (2016). Secondary Origin of Negative Carbon Isotopic Series in Natural Gas. J. Nat. Gas Geoscience 1 (1), 1–7. doi:10.1016/j.jnggs.2016.02.002
Dai, J. X., Chen, J. F., and Zhong, N. N. (2003). Large Gas Fields in China and Their Gas Sources. Beijing: Science Press, 83–163. (In Chinese).
Dai, J. X. (2014). Coal-derived Large Gas Fields and Their Gas Sources in China. Beijing: Science Press, 212–233.
Dai, J. X. (1993). Hydrocarbon Isotope Characteristics of Natural Gas and Identification of Various Natural Gases. Nat. Gas. Geosci. 4 (2), 1–40. (In Chinese with English abstract).
Dai, J. X. (1992). Identification and Distinction of Various Alkenes Gases. Sci. China (Series B) 35 (10), 1246–1257.
Dai, J. X., Li, J., Ding, W. W., Hu, G. Y., Luo, X., Tao, S. Z., et al. (2005). Geochemical Characters of the Giants Gas Accumulations with over One Hundred Billion Cubic Meters Reserves in China. Pet. Explor. Dev. 32 (4), 16–23. (In Chinese).
Dai, J. X., Ni, Y. Y., and Dong, D. Z. (2021). 2021-2025 Is a Period of Great Development of China's Natural Gas Industry: Suggestions on the Exploration and Development of Natural Gas during the 14th Five-Year Plan in China. Nat. Gas. Geosci. 32 (1), 1–16. doi:10.1016/j.jnggs.2021.08.001
Dai, J. X., Ni, Y. Y., and Wu, X. Q. (2012). Tight Gas in China and its Significance in Exploration and Exploitation. Petroleum Explor. Dev. 39 (3), 257–264. (In Chinese with English abstract). doi:10.1016/s1876-3804(12)60043-3
Dai, J. X., Ni, Y. Y., and Wu, X. Q. (2013). Tight Sandstone Gas in China and its Significance in Exploration and Development. Petroleum Explor. Dev. 39 (3), 257–264.
Dai, J. X., and Qi, H. F. (1989). The Relationship between δ13C and Ro in Coal Derived Hydrocarbon in China. Chin. Sci. Bull. 34 (9), 690–692.
Dai, J. X., Song, Y., and Zhang, H. F. (1996). Main Controlling Factors for the Formation of Large and Medium-Sized Gas Fields in China. Chin. Sci. Ser. D 26 (6), 481–487. CNKI: SUN: JDXK.0.1996-06-000.
Dai, J. X., Song, Y., and Zhang, H. F. (1997). Natural Gas Accumulation Zones in China. Beijing: Science Press. (In Chinese).
Feng, Q., Geng, A. S., Liao, Z. W., and Zhang, X. L. (2007). Carbon and Hydrogen Isotopic Compositions of Coal-Genetic Natural Gases and Their Applications in Accumulation in Upper Palezoic. Ordos Basin 36 (3), 261–266.
Fu, J. H., Duan, X. W., and Xi, S. L. (2000). Characteristics of Upper Paleozoic Gas Reservoirs in Ordos Basin. Nat. Gas. Ind. 20 (6), 17–19. (In Chinese with English abstract).
Fu, J. H., Fan, L. Y., Liu, X. S., and Huang, D. J. (2019). Gas Accumulation Conditions and Key Exploration & Development Technologies in Sulige Gas Field. Acta Pet. Sin. 40 (2), 240–256. doi:10.7623/syxb201902013
Fu, J. H., Guo, S. B., Liu, X. S., and Wang, Y. G. (2013). Shale Gas Accumulation Condition and Exploration and Exploration Potential of the Upper Paleozoic Shanxi Formation in Ordos Basin. J. Jilin Univ. (Earth Sci. Ed. 43 (2), 382–389. doi:10.13278/j.cnki.jjuese.2013.02.001
Guo, Q., Nie, J. W., Li, J. F., Li, J. F., Feng, X. P., and Xeng, Z. B. (2020). Research and Application Effect of Natural Gas Accumulation Model in Western Area of Sulige Gas Field. Prog. Geophys. (in Chinese) 35 (5), 1784–1791. doi:10.6038/pg2020DD0496
He, Z. X., Fu, J. H., and Xi, S. L. (2003). Geological Characteristics of Reservoir Formation in Sulige Atmospheric Field. Journal of petroleum 24 (2), 6–12. (In Chinese with English abstract).
Hiyagon, H., Ozima, M., Marty, B., and Zashu, S. (1992). Noble Gases in Submarine Glasses from Mid Oceanic Ridge and Loihi Seamount: Constraints on Early History of the Earth. Gechim. Cosmochim. Acta 56, 1301.
Honda, M., McDougall, I., Patterson, D. B., and Doulgeris, A. (1991). Possible Solar Noble-Gas Component in Hawaiian Basalts. Nature 349, 149–151. doi:10.1038/349149a0
Honda, M., Patterson, D. B., McDougall, I., and Falloon, T. J. (1993). Noble Gases in Submarine Pillow Basalt Glasses from the Lau Basin: Detection of a Solar Component in Back-Arc Basalts. Earth Planet. Sci. Lett. 120, 135. doi:10.1016/0012-821x(93)90235-2
Hu, A. P., Li, J., Zhang, W. Z., Li, Z. S., Hou, L., and Liu, Q. Y. (2008). Geochemical Characteristics and Origin of Gases from the Upper, Lower Paleozoic and the Mesozoic Reservoirs in the Ordos Basin, China. Science in China Series D Earth Sciences 51 (Suppl. 1), 183–194. doi:10.1007/s11430-008-5005-1
Hu, G. Y., Li, J., Ma, C. H., Li, Z. S., Zhang, M., and Zhou, Q. (2007). Characteristics and Implications of the Carbon Isotope Fractionation of Desorbed Coalbed Methane in Qinshui Coalbed Methane Field, China. Earth Science Frontiers 14 (6), 267–272. doi:10.1016/s1872-5791(08)60015-9
Hu, G. Y., Luo, X., and Li, Z. S. (2010). Geochemical Characteristics and Origin of Light Hydrocarbons in Biogenic Gas. Science China (Earth Sciences) 53 (6), 832–843. doi:10.1007/s11430-010-3072-6
Hu, G. Y., Wang, W. S., and Liao, F. R. (2012). Geochemical Characteristics and its Influencing Factors of Light Hydrocarbon in Coal-Derived Gas: A Case Study of Sichuan Basin. Acta Petrologica Sinica 28 (3), 905–916.
Hu, T. L., Ge, B. X., and Chang, Y. G. (1990). The Development and Application of Fingerprint Parameters for Hydrocarbons Absorbed by Source Rocks and Light Hydrocarbon in Natural Gas. Petroleum Geology & Experiment 12 (4), 375–379. (in Chinese).
Hu, W. R. (2009). Introduction to Low Permeability Oil and Gas, Volume I, the Rapidly Rising Ordos Basin. Beijing: Petroleum Industry Press. (In Chinese).
Jia, A. L., He, D. B., Wei, Y. S., and Li, Y. L. (2021). Predictions on Natural Gas Development Trend in China for the Next Fifteen Years. Natural Gas Geoscience 32 (1), 17–27. doi:10.1016/j.jnggs.2021.04.005
Jia, A. L., Wang, G. T., Meng, D. W., Guo, Z., Ji, G., and Chen, L. H. (2018). Well Pattern Infilling Stragergy to Enhance Oil Recovery of Giant Low-Permeability Tight Gas Field: a Case Study of Sulige Gas field,Ordos Basin. Acta Petrolei Sinica 39 (7), 802–813. doi:10.7623/syxb201807007
Jiang, Z. S., Hu, G. Y., Li, Z. S., Zhang, Y., and Xie, Z. Y. (1999). The Novel Approach of the Correlation between Paleozoic Natural Gas and Source Rock in Ordos Basin. Acta Sedimentologica Sinica 17 (Sup), 820–823.
Kang, Z. L., Fu, C. D., and Cui, S. F. (2000). Introduction to Large and Medium-Sized Gas Fields in China. Beijing: Petroleum Industry Press. (In Chinese).
Kennedy, B. M., Hiyagon, H., and Reynolds, J. H. (1990). Crustal Neon: A Striking Uniformity. Earth Planet Science Letters 98, 277–286. doi:10.1016/0012-821x(90)90030-2
Lee, J. Y., Marti, K., Severinghaus, J. P., Kawamura, K., Yoo, H. S., Lee, J. B., et al. (2006). A Redetermination of the Isotopic Abundances of Atmospheric Ar. Geochim. Cosmochim. Acta 70 (17), 4507–4512. doi:10.1016/j.gca.2006.06.1563
Leythaeuser, D., Schaefer, R. G., and Cornford, C. (1979). Generation and Migration of Light Hydrocarbon (C2-C7) in Sedimentary Basin. Organic Geochemistry 1, 191–204. doi:10.1016/0146-6380(79)90022-6
Leythaeuser, D., Schaefer, R. G., and Yükler, A. (1980). Diffusion of Light Hydrocarbons through Near-Surface Rocks. Nature 284 (5756), 522–525. doi:10.1038/284522a0
Li, J., Luo, X., Li, Z. S., Jiang, Z. S., Hu, G. Y., Xie, Z. Y., et al. (2003). New Issue of Carbon Isotope Composition of Toluene to Be as Index of Gas-Rock Correlation. Natural Gas Geoscience 14 (3), 177–180.
Li, J., Xie, Z. Y., Li, Z. S., Luo, X., Hu, G. Y., and Gong, S. (2001). Gas-Source Correlation of Natural Gas in Kuqa Depression, Tarim Basin. Petroleum Exploration and Development 28 (5), 29–32. CNKI:SUN:SKYK.0.2001-05-007.
Li, X. Q., Li, J., Wang, K. D., Kong, L. X., Feng, S. B., Huang, X. B., et al. (2012). Charging, Migration and Accumulation Characteristics of Natural Gas in the Sulige Low Permeability Sandstone Large Gas Field. Geological Science and Technology Information 31 (3), 55–62.
Lin, L. B., Lin, H. B., Hou, M. C., Chen, H. D., and Dong, G. Y. (2009). Geochemistry and Accumulation of the Upper Paleozoic Natural Gas in the Sulige Gas Field, Ordos Basin. Sedimentary Geology and Tethyan Geology 29 (2), 77–82.
Liu, G., Sun, M., and Zhao, Z. (2013). Characteristics and Accumulation Mechanism of Tight Sandstone Gas Reservoirs in the Upper Paleozoic, Northern Ordos Basin, China. Petroleum Science 10 (4), 442–449. doi:10.1007/s12182-013-0294-1
Liu, Q. Y., Liu, W. H., Xu, Y. C., Li, J., and Chen, M. J. (2007). Geochemistry of Natural Gas and Crude Composition of Gas-Generated Contribution for Various Source Rocks in Sulige Gas field,Ordos Basin. Natrual Gas Geosicence 18 (5), 697–705.
Liu, W. H., and Xu, Y. C. (1987). “Relationship between Argon in Natural Gas and Kalium-Argon in Source Rock,” in Lanzhou Institute of Geology, Chinese Academy of Science. Research Annual Report for Biology, Gas Geochemistry Laboratory (Lanzhou: Gansu Science and Technology Press), 191–200.
Liu, W. H., and Xu, Y. C. (1993). The Significance of Helium and Argon Isotopic Composition in Natural Gases. Chinese Science bulletin 38 (9), 818–821.
Liu, X. S., Xi, S. L., and Fu, J. H. (2000). Natural Gas Generation of Upper Paleozoic in Ordos Basin. Natural gas industry 20 (6), 19–23. (In Chinese with English abstract).
Lupton, J. E., and Evans, L. (2004). The Atmospheric Helium Isotope Ratio: Is it Changing? Geophys. Res. Lett. 18 (3), 482–485.
Lupton, J. E. (1983). Terrestrial Inert Gases: Isotopic Tracer Studies and Clues to Primordia1 Components in the Mantle. Annual Review Earth Plant Science 11 (5), 371–414. doi:10.1146/annurev.ea.11.050183.002103
Mamyrin, B. A., Anufrriev, G. S., Kamenskii, I. L., and Tolstikhin, I. N. (1970). Determination of the Isotopic Composition of Air Helium. Geochem. Int. 7, 498–505.
Mamyrin, B. A., and Tolstikhin, I. N. (1984). Helium Isotopes in Nature. Amsterdam: Elsevier, 287. Developments in geochemistry 3.
Mark, D. F., Stuart, F. M., and Podesta, M. (2011). New High-Precision Measurements of the Isotopic Composition of Atmospheric Argon. Geochim. Cosmochim. Acta 75 (23), 7494–7501. doi:10.1016/j.gca.2011.09.042
Ozima, M., and Podesek, F. A. (1983). Noble Gas Geochemistry. London: Cambridge University Press, 1–252.
Poreda, R. J., and Farley, K. A. (1992). Noble Gases in Samoan Xenoliths. Earth Planet. Sci. Lett. 113, 129–144. doi:10.1016/0012-821x(92)90215-h
Poreda, R. J., Jenden, P. D., Kaplan, I. R., and Craig, H. (1986). Mantle Helium in Sacramento Basin Natural Gas Wells. Geochimica et Cosmoehimiea Acta 50 (12), 2 847. doi:10.1016/0016-7037(86)90231-0
Prinzhofer, A. (2013). “Noble Gas in Oil and Gas Accumulations,” in The Noble Gases as Geochemical Tracers, Advances in Isotope Geochemistry. Editor P. Burnard (New york Dordrecht London: Springer Berlin Heidelberg), 225–247. doi:10.1007/978-3-642-28836-4_9
Sano, Y., Tominaga, T., and Takahata, N. (2008). Accurate Measurement of Atmospheric Helium Isotopes. Anal. Sci. 24 (4), 521–525. doi:10.2116/analsci.24.521
Sarda, P., Staudacher, T., and Allegre, C. J. (1988). Neon Isotopes in Submarine Basalts. Earth Planet. Sci. Lett. 91, 73–88. doi:10.1016/0012-821x(88)90152-5
Shen, P., Xu, Y. C., and Liu, W. H. (1995). Applied Models of Rare Gas Geochemistry in the Research of Natural Gases. Acta Sedimentologica Sinica 13 (2), 48–57.
Snowdon, L. R., and Powell, T. G. (1982). Immature Oil and Condensate-Modification of Hydrocarbon Generation Model for Terrestrial Organic Matter. AAPG Bulletin 66 (6), 755–788. doi:10.1306/03b5a313-16d1-11d7-8645000102c1865d
Sun, M. L., Xu, Y. C., and Wang, X. B. (1991). Mass Spectrometer Analysis for He Isotope in Natural Gases. Journal of instrumental analysis 10 (5), 50–55. (in Chinese with English abstract).
Wang, J., Zhang, X. B., and Chen, J. F. (2005). Evaluation of the Hydrocarbon-Generating Characteristic for Coal-Bearing Hydrocarbon-Source Rocks of Suligemiao Gas Field. Coal Geology & Exploration 33 (2), 26–29.
Wang, X. B., Chen, J. F., and Li, J. (2014). Analysis and Influencing Factors of Rock Diffusion Coefficient in High Temperature and High Pressure Tight Gas Reservoir. Journal of China University of Petroleum (NATURAL SCIENCE EDITION) 38 (3), 25–31. (In Chinese with English abstract).
Wang, X. B., Chen, J. F., and Li, J. (2015). Discussion on the Role and Contribution of Diffusion in the Formation of Low Porosity and Permeability Tight Sandstone Gas Reservoir. Journal of China University of Petroleum (Natural Science Edition) 39 (5), 58–64. (In Chinese with English abstract).
Wang, X. B., Chen, J. F., Li, Z. S., Li, J., Wang, D. L., Wang, Y. F., et al. (2016). Noble Gases Geochemical Characteristics and Gas Source Correlation for Dabei Gas Field in Kuche Depression, Tarim Basin. Energy Exploration & Exploitation 34 (1), 113–128. doi:10.1177/0144598715623673
Wang, X. B., Li, Z. S., Li, J., Wang, D. L., Chen, J. F., Xie, Z. Y., et al. (2013). Technique for Total Composition and Isotope Analyses of Noble Gases. Acta Petrolei Sinica 34 (S.1), 70–77. doi:10.7623/syxb2013S1008
Wang, X. B. (1989). Noble Gas Isotope Geochemistry and Cosmochemistry. Beijing: Science Press, 7–22.
Wang, X. B., Wei, G. Q., Li, J., Chen, J. F., Gong, S., Li, Z. S., et al. (2018). Geochemical Characteristics and Origins of Noble Gases of the Kela 2 Gas Field in the Tarim Basin, China. Marine and Petroleum Geology 89, 155–163. doi:10.1016/j.marpetgeo.2017.02.013
Wei, G. Q., Wang, D. L., Wang, X. B., Li, J., Li, Z. S., Xie, Z. Y., et al. (2014). Characteristics of Noble Gases in Gaoshiti—Moxi Large Gas Field in Sichuan Basin. Petroleum Exploration and Development 41 (5), 533–538. doi:10.1016/s1876-3804(14)60069-0
Welhan, J. A., and Craig, H. (1983). “Methane, Hydrogen and Helium in Hydrothermal Fluids at 21°N on the East Pacific Rise,” in Hydrothermal Processes at Seafloor Spreading Centers. Editors P. A. Rona, K. Bostrom, and L. Laubier (New York: Plenum Press), 391–410. doi:10.1007/978-1-4899-0402-7_17
Welhan, J. A., Poreda, R. J., Rison, W., Poreda, R., and Craig, P. (1983). Geothermal Gases of the Mud Volcano Area, Yellowstone Park. Eos (Transactions, American Geophysical Union) 64, 882.
Whiticar, M. J., and Snowdon, L. R. (1999). Geochemical Characterization of Selected Western canada Oils by C5-C8 Compound Specific Isotope Correlation (CSIC). Organic Geochemistry 30 (7-8), 1127–1161. doi:10.1016/S0146-6380(99)00093-5
Xu, C., Shen, P., Liu, W. H., Tao, M. X., Sun, M. L., and Du, J. G. (1998). Noble Gas Geochemistry Study in Natural Gas. Beijing: Science Press, 1–231.
Xu, S., Xu, Y. C., Shen, P., Shun’ichi, N., and Hiroshi, W. (1997). Noble Gas Isotopes in Natural Gases from Central and Northwest China. Chinese Science bulletin 41 (12), 115–118. doi:10.1007/bf02882496
Xu, S., Xu, Y. C., Shen, P., Wang, X. B., Du, J. G., Shun’ichi, N., et al. (1996). The Isotopic Composition and its Geological Significance of Neon in Natural Gas of Eastern Basin in China. Chinese Science bulletin 41 (21), 970–l972.
Xu, Y. C., Wang, X. B., Wu, R. M., Shen, P., Wang, Y. X., and He, Y. P. (1979). Noble Gas Isotopic Composition of Natural Gases. Geochimica 8 (9), 271–282. (in Chinese).
Yang, H., and Liu, X. S. (2014). Progress of Paleozoic Coal-Derived Gas Exploration in Ordos Basin, West China. Petroleum Exploration & Development 41 (2), 129–137. doi:10.1016/s1876-3804(14)60017-3
Yang, H., Xi, S. L., and Wei, X. S. (2006). Analysis of Natural Gas Exploration Potential in Sulige Area. Natural gas industry 26 (12), 45–48. (In Chinese with English abstract).
Yang, H., Zhang, J., and Wang, F. Y. (2000). Characteristics of Paleozoic Gas Bearing System in Ordos Basin. Natural gas industry 20 (6), 7–11. (In Chinese with English abstract).
Yang, J. J., and Pei, X. G. (1996). Natural Gas Geology in China (Volume IV): Ordos Basin. Beijing: Petroleum Industry Press. (In Chinese).
Yu, C., Huang, S. P., Gong, D. Y., Liao, F. R., Li, J., and Sun, Q. W. (2013). Partial Reversal Cause of Carbon and Hydrogen Isotope Compositions of Natural Gas: a Case Study in Sulige Gas Field, Ordos Basin. Acta Petrolei Sinica 34 (Suppl. 1), 92–101. doi:10.7623/syxb2013s1011
Zhang, D. W., Liu, W. H., Zheng, J. J., Wang, X. F., and Nan, Q. Y. (2005). Identification of Main Gas Source in Kuqa Depression Using Argon Isotope Ratios. Geochimica 34 (4), 405–409. (in Chinese with English abstract).
Zhao, W. Z., Bian, C. S., and Xu, Z. H. (2013a). Similarities and Differences in Reservoir Formation between Sulige Gas Field and Xujiahe Formation Gas Field in Central Sichuan. Petroleum exploration and development 40 (4), 429–438. doi:10.1016/s1876-3804(13)60054-3
Keywords: Ordos basin, Sulige gas field, tight gas, noble gases, C7 and C8 light hydrocarbon, genetic identification, gas source correlation
Citation: Xiaobo W, Lianhua H, Jian L, Chunxia Y, Liyong F, Jianfa C, Chunlin Z, Jianying G, Jixian T, Yue Z and Chunlong Y (2022) Geochemical Characteristics and Gas Source Contributions of Noble Gases of the Sulige Large Tight Gas Field of Upper Paleozoic in Ordos Basin, China. Front. Earth Sci. 10:889112. doi: 10.3389/feart.2022.889112
Received: 03 March 2022; Accepted: 27 May 2022;
Published: 13 July 2022.
Edited by:
Maowen Li, SINOPEC Petroleum Exploration and Production Research Institute, ChinaReviewed by:
Peng Li, SINOPEC Petroleum Exploration and Production Research Institute, ChinaCopyright © 2022 Xiaobo, Lianhua, Jian, Chunxia, Liyong, Jianfa, Chunlin, Jianying, Jixian, Yue and Chunlong. This is an open-access article distributed under the terms of the Creative Commons Attribution License (CC BY). The use, distribution or reproduction in other forums is permitted, provided the original author(s) and the copyright owner(s) are credited and that the original publication in this journal is cited, in accordance with accepted academic practice. No use, distribution or reproduction is permitted which does not comply with these terms.
*Correspondence: Li Jian, bGlqaWFuNjlAcGV0cm9jaGluYS5jb20uY24=; Yang Chunxia, eWFuZ2N4ODNAcGV0cm9jaGluYS5jb20uY24=; Fan Liyong, bHlmYW4xMjNfY3FAcGV0cm9jaGluYS5jb20uY24=; Yang Chunlong, eWFuZ2NodW5sb25nNjlAcGV0cm9jaGluYS5jb20uY24=
Disclaimer: All claims expressed in this article are solely those of the authors and do not necessarily represent those of their affiliated organizations, or those of the publisher, the editors and the reviewers. Any product that may be evaluated in this article or claim that may be made by its manufacturer is not guaranteed or endorsed by the publisher.
Research integrity at Frontiers
Learn more about the work of our research integrity team to safeguard the quality of each article we publish.