- 1School of Geoscience and Technology, Southwest Petroleum University, Chengdu, China
- 2State Key Laboratory of Oil and Gas Reservoir Geology and Exploitation, Southwest Petroleum University, Chengdu, China
- 3Key Laboratory of Tectonics and Petroleum Resources (China University of Geosciences), Ministry of Education, Wuhan, China
- 4Shale Gas Evaluation and Exploitation Key Laboratory of Sichuan Province, Chengdu, China
- 5State Key Laboratory of Petroleum Resources and Prospecting, China University of Petroleum, Beijing, China
- 6Unconventional Petroleum Research Institute, China University of Petroleum, Beijing, China
- 7Research Institute of Exploration and Development, Xinjiang Oilfield Company, PetroChina, Karamay, China
- 8Karamay Vocational and Technical College, Karamay, China
- 9Petroleum Engineering School, Southwest Petroleum University, Chengdu, China
China has abundant shale gas resources with great exploration potential, and stage progress has been made in this aspect. The sedimentary environment and reservoir characteristics are important aspects of the study on shale gas accumulation. Previous studies have mostly been carried out from a “qualitative” perspective, but not from a “quantitative” one. There is a lack of comparative studies on “marine shales with different TOC contents”. This paper takes the marine shale of the first member of the Longmaxi Formation (Long 1 Fm) in southern Sichuan Basin, Southern China, as the research object. The core samples were taken to carry out analyses (mineral composition analysis, TOC content analysis, porosity analysis) and experiments (carbon dioxide and nitrogen adsorption experiments, high-pressure mercury intrusion, FIB-SEM, and FIB-HIM experiments). The element logging data were collected to conduct the quantitative comparison of genesis and pore structure characteristics of siliceous minerals in marine shale with different TOC contents in this area. The conclusions are as follows: first, a formula is used to calculate and determine whether there is excessive silicon; then the Al-Fe-Mn triangle diagram is used to analyze the genesis of excessive silicon, so as to quantitatively analyze the genesis of siliceous minerals in shale: the siliceous minerals of organic shale (1% < TOC <2%) in the member studied are almost terrigenous detrital genesis; most siliceous minerals in organic-rich shale (TOC >2%) are detrital genesis, and a small part (0–20%) are biogenic. Carbon dioxide and nitrogen adsorption experiments, as well as high-pressure mercury intrusion experiments are adopted to quantitatively characterize the whole-aperture pore structure characteristics. The pore development characteristics of different shale components are analyzed by combing FIB-SEM and FIB-HIM experiments. The organic-bearing shales in the target section of this study area mainly develop clay mineral pores (71%), and are dominated by macro-pores (57.3%) with a low number of pores, irregular-shaped pores, as well as poor storage capacity and connectivity; the organic-rich shales in the target section of this study area mainly develop organic pores (51%), and are dominated by micro-pores (32.1%) and mesopores (54%) that are large in number and elliptical-shaped, with good storage capacity and good connectivity. The results of this study help to improve the understanding of the pore size of marine shales, the origin of siliceous minerals in marine shales, and the pore structure characteristics of marine shales, which are of great theoretical and practical significance for improving the theory of shale gas formation and guiding the selection of shale gas sweet spot.
Introduction
The need for natural gas in China has increased substantially due to the rapid economic growth in recent years. Similar to North America, China is also rich in shale gas resources with enormous potential for exploration (Curtis., 2002; Guo, 2016; Zou et al., 2017; Guo et al., 2021). Since 2009, the major oil companies have successively completed five key industrial construction areas in Fuling, Changning, Weiyuan, Zhaotong, and Fushun-Yongchuan, and six evaluation breakthrough areas in Xuanhan-Wuxi, Jingmen, Southern Sichuan (Rongchang-Yongchuan and Weiyuan-Rongxian County), Southeast Sichuan (Dingshan, Wulong and Nanchuan), Meigu-Wuzishan and Yan’an, as well as seven potential research areas in Guizhou Zheng’an, Cengong, Hubei Laifeng-Xianfeng, Hunan Baojing, Longshan, Chongqing Chengkou and Zhongxian-Fengdu, with proven geological reserves of shale gas reaching 17,800 × 108 m3. This means that China has achieved phased achievements in shale gas exploration and development (Zou et al., 2015; Zou et al., 2016; Hou et al., 2020; Guo, 2021a).
Sichuan Basin is the main area for marine shale gas exploration and development in China. In the first member of the Lower Silurian Longmaxi Formation, the total organic carbon content (TOC content) exceeds 2% overall, and the rocks are organic-rich shales in the first sub-member, which is also the main reservoir stratum of shale gas (Guo et al., 2016; Kang et al., 2019; Guo et al., 2020). As exploration proceeds, a batch of prolific shale gas wells have been discovered; meanwhile, there are also some shale gas wells with little gas or no gas. There are significant differences in the shale gas production of the same block (Guo et al., 2017). The research on shale deposition and shale reservoir is important regarding the shale gas accumulation research. A series of studies on shale deposition and reservoirs have been done before the present study (Gao and Hu, 2018; Zhang et al., 2018; Wang et al., 2019; Huang et al., 2020a; Gao et al., 2020). Taking the shale samples of Longmaxi Formation and Niutitang Formation in the periphery of Chongqing as the research objects, Wang et al. (2018) studied the organic pores and development characteristics of the two sets of shales through the organic carbon content test, mineral composition analysis, equivalent vitrinite reflectance test, FIB-SEM and FIB-HIM observation in combination with the analysis of stratigraphic burial history and hydrocarbon generation history. However, the experimental method used in this paper is mainly a qualitative study of shale reservoirs, and quantitative studies have not been conducted. Taking the continental shale reservoirs in the lower sub-member of the Third Sha Member of Zhanhua Depression and marine shale reservoirs in Longmaxi Formation of Southeast Sichuan Basin as typical examples, Li et al. (2019) thoroughly analyzed the pore structure differences between continental and marine shale reservoirs by field emission scanning electron microscopy, carbon dioxide adsorption, nitrogen adsorption, high-pressure mercury intrusion analysis, Soxhlet extraction and other methods. In this paper, some experimental methods are used to quantitatively study the characteristics of shale reservoirs, but the experimental methods used are not comprehensive enough. Moreover, this paper is a comparative study of the characteristics of shale reservoirs in different sedimentary phases (terrestrial and marine), and it does not compare the shale reservoirs with different TOC contents. Qiu et al. (2019) took the shale in Lower Silurian Longmaxi Formation in the Upper Yangtze Region of southern China as an example to carry out the comparative analysis on TOC content, paleoproductivity, and redox conditions between the shale formation containing volcanic ash and the normal sedimentary shale formation. Gao et al. (2020) pointed out through literature review that both the inorganic and organic diagenesis control the shale pore structure evolution process. The studies on pore structure characteristics and diagenesis of shales conducted in this paper are mainly qualitative studies, and the quantitative aspects are slightly inadequate.
A series of studies have been done on shale sedimentation and shale reservoirs, but most of them are “qualitative” research, while the “quantitative” perspective has rarely been taken. As shale gas exploration proceeds, the gap needs to be filled. Moreover, comparative studies on “marine shales with different TOC contents” are rare. Therefore, this paper takes a quantitative and comparative perspective of the silica-mineral genesis and pore structure characteristics of marine shales with different TOC contents in Jiao Ye 1 Well from the Lower Silurian Longmaxi Formation in the southeastern Sichuan Basin, southern China (Figure 1) (Nie et al., 2020; Wang et al., 2020e; Zhang et al., 2021; Zhang et al., 2022a). This paper quantifies the presence and content of excess silicon in marine shales with different TOC contents by using Si and Al elements from elemental logging, and uses the Al-Fe-Mn triangle diagram to determine the cause of excess silicon. Besides, the “porosity petrophysical model” is utilized to quantitatively characterize the pore composition of marine shales with different TOC contents. CO2 and N2 adsorption experiments, combined with high-pressure mercury pressure experiments, were carried out to quantitatively characterize the pore volumes of marine shales with different TOC contents. FIB-SEM and FIB-HIM observations were completed to directly characterize the pore volumes of marine shales with different TOC contents.
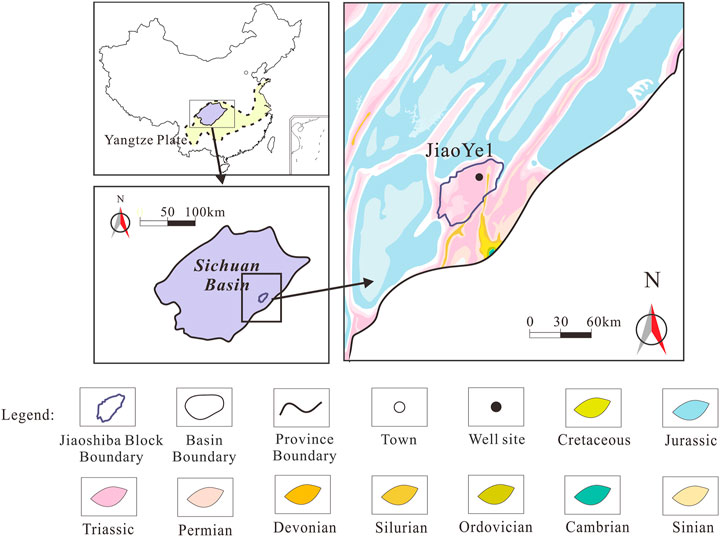
FIGURE 1. Location of Jiaoshiba Block in Southeast Sichuan Basin, South China and JiaoYe1 Well Location Map. Modified from references (Wang et al., 2020e; Nie et al., 2020; Zhang et al., 2021; Zhang et al., 2022a).
Geological Settings
Sedimentary and Stratum Characteristics
According to previous studies (Mei et al., 2012; Wang et al., 2015b; Mou et al., 2016; Zhang et al., 2020b), the interior Cratonic sagging basin was formed after the Upper Yangtze area was squeezed by the Cathaysian Plate in the Upper Ordovician-Lower Silurian. In the Upper Yangtze region, the Upper Ordovician and Lower Silurian sedimentary strata are called the Wufeng and the Longmaxi Formations, respectively. The latter can be divided into the first, second, and third members from bottom to top. This work focuses on the first member of the Longmaxi Formation, (Long 1 Fm.,) and its shale has different lithologies: the first sub-member is primarily the black organic-rich siliceous shales, while the second and third sub-members are a combination of dark grey shales, silty shales, and siltstones.
Tectonic Characteristics
According to previous studies (Li et al., 1995; Li et al., 2002; Wang and Li, 2003; Shan et al., 2021), the original continental crust in South China was split into Yangtze and Cathaysian paleo-plates in the early Mesoproterozoic. In the Lower Cambrian, the two plates had tension and a large-scale transgression occurred in them, leading to the sedimentation of a group of organic-rich shales that almost covered the entire plate. Later, the water body became shallower over time, and the lithology gradually developed from fine shales and silty shales into coarse clastic rocks, such as siltstones and sandstones, etc. Due to the extrusion and collision of the Cathaysian Plate in the Ordovician, the water body continued to get shallower, thus changing the sedimentary system of clastic rocks into carbonate rocks. In the Upper Ordovician-Lower Silurian, the sedimentary system of clastic rocks was restored due to the large-scale transgression. Consequently, a set of organic-rich shales were deposited in the deep shelf surrounded by the ancient land. In the Cambrian-Silurian, the Cathaysian Plate gradually subducted into and collided with the Yangtze Plate. The two plates merged into the unified South China Plate at the end of the Silurian (Huang et al., 2020b; Wang H. et al., 2020; Wang et al., 2020d; Li et al., 2020; Gao et al., 2021a).
Samples, Experiments, and Data Sources
A schematic diagram of the experiments used in each of the research components of this paper is shown in Figure 2. From Long 1 Formation, the core samples were taken every 2 m from the JiaoYe 1 Well. Then, we carried out the TOC content analysis experiments with Sievers 860 analyzer, the X diffraction whole-rock mineral analyses and clay mineral analyses with mineral analyzer YST-I and the porosity experiments with Poro PDP-200 tester. Partial data were collected from the literature of Guo et al. (2016). The element logging data provided by Schlumberger Company were also collected.
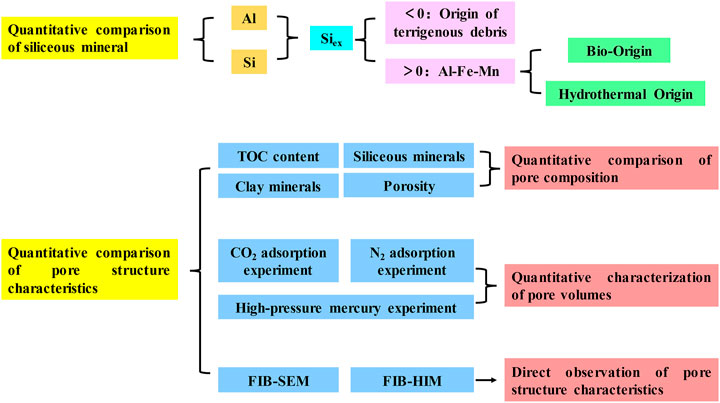
FIGURE 2. Schematic diagram of the experiments used in each of the research components of this paper.
Meanwhile, some samples with different TOC contents were selected. Then we carried out the carbon dioxide adsorption experiments with a BSD-PM1/2 instrument, the nitrogen adsorption experiments with a BSD-PS1/2/4 adsorber, and the high-pressure mercury intrusion experiments with a 3H-2000PS2 instrument. The experimental data were collected to obtain the experimental results about the joint characterization of the whole-aperture pore volume. Other samples with different TOC contents were chosen and carried out the FIB-SEM (Focused ion beam scanning electron microscopy) experiments with Helios NanoLab 660, and the FIB-HIM (Focused ion beam-Helium ion microscopy) experiments with Zeiss Orion NanoFab.
Results and Discussion
Quantitative Comparison of Siliceous Mineral Marine Shale With Different TOC Contents
The silica sources include normal terrigenous detrital deposits, hydrothermal genesis and biogenic silica under special circumstances (Bostrom et al., 1973; Murray et al., 1991; Liu and Zheng, 1993; Yang et al., 1999). Excess siliceous mineral content (Siex) refers to siliceous minerals other than normal terrigenous detrital deposits. The content of excess siliceous minerals can be calculated by the following formula:
where, Sis is the content of silicon in the sample, Unit:wt%; Als is the aluminum content in the sample, Unit:wt%; the value of (Si/Al)bg is 3.11, which is the average content in shale (Holdaway and Clayton, 1982).
The shale in the JiaoYe 1 Well from the first sub-member of Long 1 Formation is organic-rich shale (TOC >2%), and those from the second and third sub-members are organic shale (1% < TOC <2%). According to the Si and Al elements logging data provided by the Schlumberger Company and calculation formula of the excess siliceous mineral content, the Siex of the Jiao Ye 1 Well from Long 1 Fm. is calculated, and the results are shown in Figure 3.
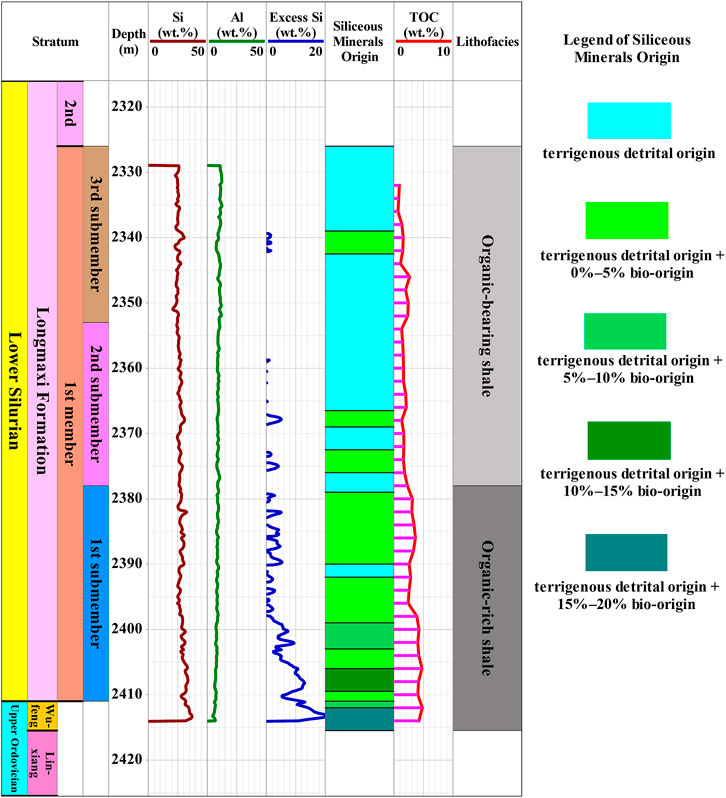
FIGURE 3. Quantitative analysis of siliceous minerals in marine shales with different TOC contents in the first member of Lower Silurian Longmaxi Formation in Jiao Ye 1 well, Yangtze area. See Figure 1 for the well location.
Wedepohl (1971), Adachi et al. (1986), and Yamamoto (1987) proposed a method to determine whether siliceous minerals are hydrothermal or biogenic genesis by Al-Fe-Mn triangular diagram. In this paper, the test values of Al, Fe and Mn elements in the formation with excess silicon content in the first member of Lower Silurian Long Fm. in Jiao Ye 1 Well are projected on the triangle diagram, as shown in Figure 4. It is found that the numerical values are basically distributed in the biogenesis area, which indicates that excess silicon content is biogenic. Based on the findings, the vertical siliceous source map of shale in the first member of Lower Silurian Longmaxi Formation in Jiao Ye 1 Well can be accurately drawn, as shown in Figure 3. It can be seen that the organic shale in the second and third sub-members of Long 1 Fm. are basically terrigenous detrital silicon, and the organic-rich shale in the first sub-member of Long 1 Fm. contains biogenic silicon. Among the members with biogenic silicon, the biogenic silicon content of more than half of the members is between 0 and 5%, and that in some members is 5–15%, with the highest being 15–20%.
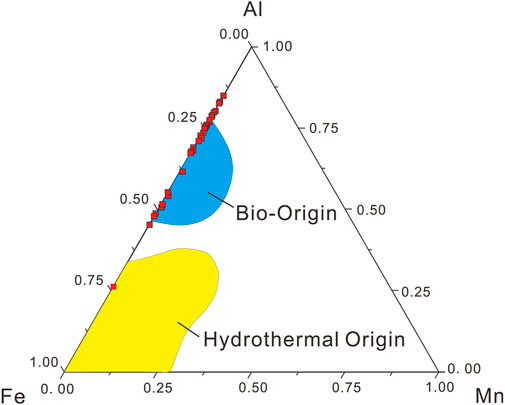
FIGURE 4. The source of siliceous minerals in the first member of Lower Silurian Longmaxi Formation of Jiao Ye 1 Well containing excess silicon content is analyzed by Al-Fe-Mn triangle diagram, indicating that the excess silicon content is biogenesis. See Figure 1 for the well location.
Quantitative Comparison of Pore Structure Characteristics of Marine Shales With Different TOC Contents
Quantitative Comparison of Pore Composition of Marine Shales With Different TOC Contents
From Long 1 Fm. in this study, the core samples were taken from the JiaoYe 1 Well. Next, we carried out TOC content analyses, porosity experiments and mineral composition analyses. See Figure 5 for the stratigraphic division and geochemical data analysis results in this area. Based on the porosity data statistics of each sub-member in Long 1 Fm., we found that the average total porosity is 4.60% in the first sub-member, 3.72% in the second sub-member, and 5.36% in the third sub-member. According to TOC content and mineral composition analyses, in the Long 1 Fm., the brittle minerals content and TOC content in shales decrease gradually from the lower first sub-member (average value is 65.9 and 3.58%, respectively) to the upper third sub-member (average 46.8 and 1.72%, respectively), while the clay minerals content increases gradually (from 34.1 to 53.2%). See these data in Table 1.
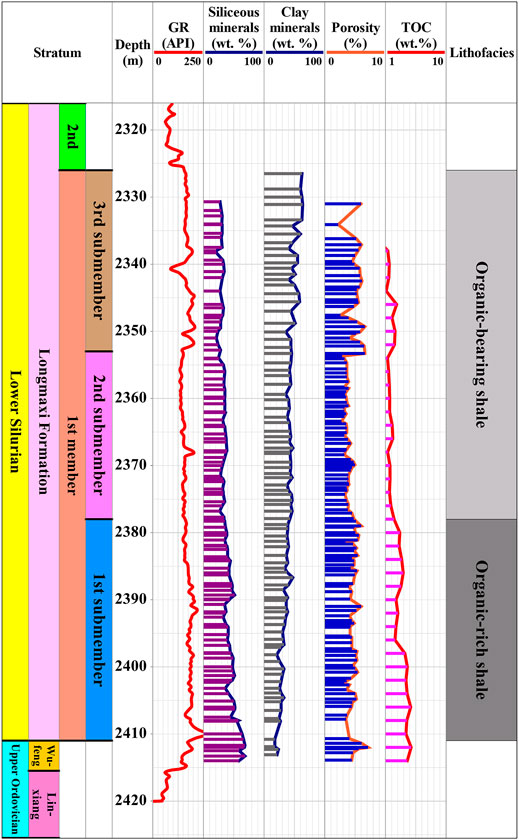
FIGURE 5. The divided sub-members in JiaoYe 1 Well in Long 1 Fm. and analysis results of mineral composition, TOC content and porosity. See Figure 1 for the well location.
In Long 1 Fm., each sub-member has different mineral composition and TOC content, which means that the pore composition varies as well. According to the reservoir condition, shale pores can be divided into organic pores, brittle mineral pores, and clay mineral pores. Guo et al. (2016) quantitatively characterized the contribution of each type to shale pores through the petrophysical model of porosity (Guo et al., 2016). Although the total porosity is similar among sub-members, the porosity composition is different because of the various mineral composition and TOC content in shales. For the total porosity in Long 1 Fm., the proportion of the clay mineral pores increases gradually from the lower first sub-member (average 43%) to the upper third sub-member (average 71%), while the proportion of the organic pores decreases gradually from the lower first sub-member (average 51%) to the upper third sub-member (average 24%) (Guo et al., 2016).
Quantitative Characterization of Pore Volumes of Marine Shales With Different TOC Contents
CO2 and N2 adsorption experiments were carried out on the samples in this study for characterization, which has overlapped area inevitably so the weighted average method (Ji et al., 2015; Wang et al., 2016a; Wang et al., 2016b; Tang et al., 2017; Gao et al., 2021b; Gao., 2021) was adopted to process the pore volume and pore-specific surface area data. Besides, high-pressure mercury experiments were carried out for the distribution of micro- (<2 nm), meso- (2–50 nm), and macropores (>50 nm), respectively. For Long 1 Fm. in this study, quantitative characterization (Figure 6) was conducted on the pore volume of shales with different TOC content. See the statistical data in Table 1. In the first sub-member with high TOC content, It is found that the pore volume proportion of micropores and mesopores are relatively larger (32.1 and 54%, respectively), and the volume proportion of macropores is relatively smaller (13.9%). In the third sub-member with gradually decreasing TOC content and gradually increasing clay minerals, the pore volume proportion of micropores and mesopores decreased gradually (10.3 and 32.5%, respectively), and the pore volume proportion of macropores increased gradually (57.3%). This means that micropores and mesopores are mainly developed in organic pores, and macropores are mainly developed in clay mineral pores.
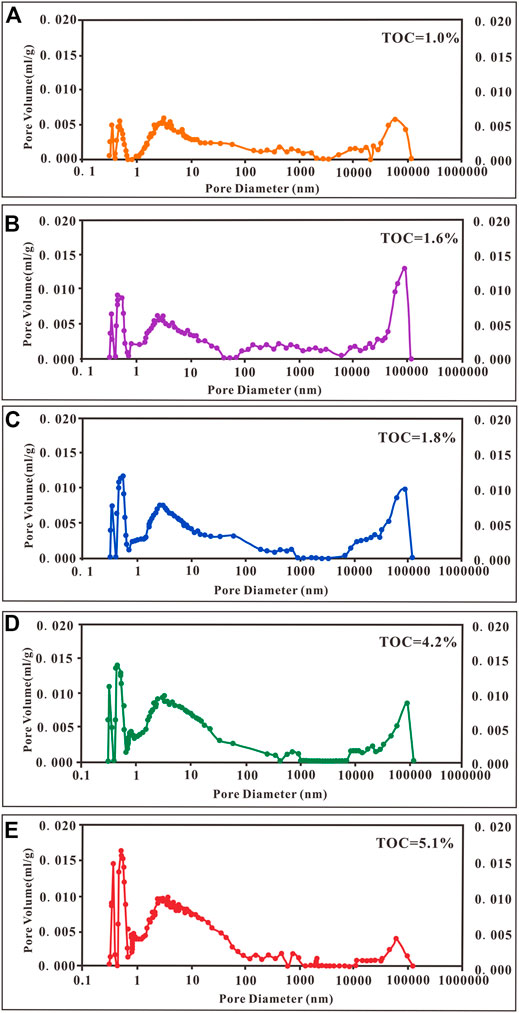
FIGURE 6. The whole-aperture quantitative characterization on pore volume of the shales with different TOC content in JiaoYe 1 Well in the Long 1 Fm.: Characteristics about. (A): 2337 m, TOC = 1.0%; (B): 2358 m, TOC = 1.6%; (C): 2369 m, TOC = 1.8%; (D): 2396 m, TOC = 4.2%; (E): 2406 m, TOC = 5.1%. See Figure 1 for the well location. Modified from reference (Tang et al., 2016).
Direct Observation of Pore Structure Characteristics of Marine Shales With Different TOC Contents
For an in-depth analysis on the characteristics of shaly pore structure in different strata, we observed the organic pores in the first sub-member and the clay mineral pores in the third sub-member of the JiaoYe 1 Well in Long 1 Fm. during FIB-SEM experiments and FIB-HIM experiments.
The FIB-SEM images show that the grayscale of pores is the largest, and the grayscale of each shaly material composition decreases as the molecular weight drops, which means that the grayscale of organic matters is higher than that of inorganic minerals in FIB-SEM images (Ji et al., 2014; Ji et al., 2016; Guo,2021b; He et al., 2021; Huang et al., 2021; Zhang et al., 2022b). For the Long 1 Fm., it is found that most pores are organic pores in the first sub-member with large pore diameter (micropores, mesopores and macropores with diameter less than 200 nm), and the pores are mostly elliptical (Figure 7A); the number of clay mineral pores is less than that of organic pores in the third sub-member, while with larger pore diameter than that of organic pores (macropores with a diameter of 200nm∼1 μm), and the pores are mostly irregular (Figure 7C).
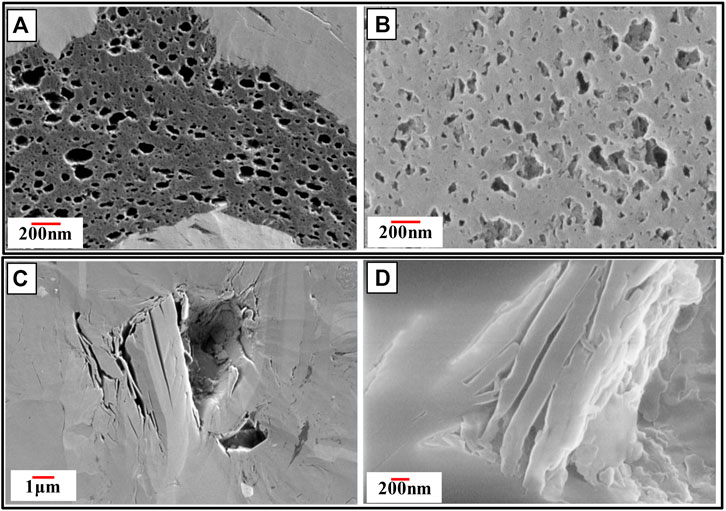
FIGURE 7. In JiaoYe 1 Well in the Long 1 Fm.: FIB-SEM and FIB-HIM images of shales in the first sum-member and third member. In JiaoYe 1 Well in the Long 1 Fm., 2402 m: Organic pores: (A) FIB-SEM image, (B) FIB-HIM image; In JiaoYe 1 Well in the third member of Longmaxi Formation, 2339 m: Clay Mineral Pores: (C) FIB-SEM Image, (D) FIB-HIM Image.
The FIB-HIM experiments can be adopted to further observe the internal stereo structure of the pores and display the two-dimensional images with three-dimensional effects (Zhang et al., 2020a; Zhang et al., 2020c; Liu et al., 2021a; Liu et al., 2021b; Wang et al., 2021). The grayscale of FIB-HIM images is right the opposite of that of FIB-SEM images, and the grayscale of each shaly material composition increases with the decreasing molecular weight. This means that the grayscale of organic matter is lower than that of inorganic minerals in FIB-SEM images (Bernard et al., 2012a; Bernard et al., 2012b; Dillinger and Esteban, 2014; Wang et al., 2017; Kou et al., 2022). For the Long 1 Fm., FIB-HIM experiments were carried out on the FIB-SEM samples with similar depth. It is found that there are foramina embedded in the macropores in the organic pores of JiaoYe 1 well in the first sub-member (Figure 7B). The porosity development characteristics of “foramina embedded in the macropores” not only increase the reservoir space and specific surface area of organic matters, but also provides a seepage channel for shale gas, as well as enhances the connectivity of organic matters (Zuo et al., 2019; Wang G. et al., 2020; Wang J. et al., 2020; Xia et al., 2020; Yu et al., 2022). Relatively isolated pores are fewer and mainly developed in the clay mineral pores in the second and the third sub-members (Figure 7D), with relatively poor reservoir capacity and connectivity (Nie et al., 2012; Dillinger and Esteban, 2014; Wang et al., 2015a; Guo et al., 2016).
Patterns of Pore Structure Characteristics
Based on the above analysis, the patterns of pore structure characteristics of marine shales with different TOC contents were summarized, as shown in Figure 8. The organic shales in the second and third sub-members of Long 1 Fm. mainly develop clay mineral pores, mainly macropores, and the pore development is isolated, with a small number of pores. The pores are mostly irregular, with poor reservoir capacity and connectivity (Figure 8A). The organic-rich shales in the first sub-member of Longmaxi Formation mainly develop organic pores, mainly micropores and mesopores, which are great in quantity and mostly elliptical. It presents the development characteristics of “small pores in big pores”, with large reservoir space and sound connectivity (Figure 8B).
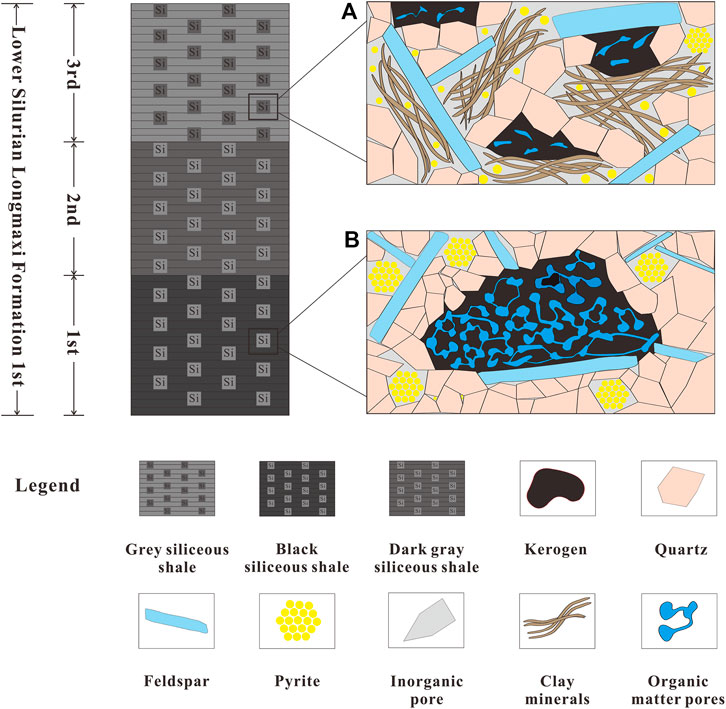
FIGURE 8. Patterns of pore structure characteristics of marine shales with different TOC contents (A): Organic shale; (B) Organic-rich shale.
The following analyzes the reasons why the pore structure characteristics of marine shales with different TOC contents are developed differently. The organic-bearing shales in the sub-members 2 and 3 of Long 1 formation have relatively low TOC contents, and inorganic minerals are mainly clay minerals; while the contents of siliceous minerals such as quartz and feldspar are low. Intergranular pores are developed in clay minerals. Due to the effect of overburden pressure and the lack of support from rigid minerals such as quartz and feldspar, some of the clay minerals were compressed, causing the residual clay mineral pores to develop more isolated and irregular pores that are small in number, with poor storage capacity and connectivity. The organic-rich shale in the sub-member 1 of the Long 1 Formation has a relatively high TOC content, and inorganic minerals are mostly siliceous minerals such as quartz and feldspar, but the clay mineral content is relatively low. The organic-rich shales in the sub-member 1 of the Long 1 formation have a kerogen type of I and II1 as they are all marine shales. With the gradually deepened thermal evolution when entering the immature stage (Ro > 2%), part of type I and II1 kerogen are directly converted to natural gas, and the other part is first converted to liquid hydrocarbons and then to natural gas, thus forming organic pores within the kerogen. The organic pores are protected by rigid minerals such as quartz and feldspar and are highly resistant to compression. As a result, these organic pores are mostly in an elliptical shape and large in number with the distribution of “large pores over small pores”. Besides, they have large storage space and strong connectivity.
Two quantitative analysis methods are applied in this paper: (1) Quantitative analysis of shale silica mineral genesis: The data are from elemental logging data or data obtained from the main trace element analysis of the cores. The Si and Al contents, combined with the calculation formula, are used to determine the presence of excess Si and to calculate its amount; and then the Al-Fe-Mn triangle is used to analyze the cause of the excess Si. This can quantitatively analyze the siliceous mineral genesis of shale and provide a basis for the study of shale depositional environment. (2) Quantitative analysis methods of pore structure characteristics of shale: (1) data are obtained from TOC content analysis, mineral composition analysis, rock density analysis and porosity analysis of shale cores; the “porosity petrophysical model” is used to quantitatively characterize the pore composition of marine shale; (2) data are obtained from adsorption experiments of carbon dioxide and nitrogen; high-pressure mercury pressure experiments, FIB-SEM experiments, and FIB-HIM experiments. A combination of these five experiments was used to quantitatively characterize the pore structure of shale micro and nano full pore size. The above methods can be applied not only to the study of marine shale, but also to the study of terrestrial shale and marine-terrestrial transitional shale, providing important technical support for the analysis of shale depositional environment and shale reservoir characterization.
Summary and Conclusion
This paper studied the marine shales in Long 1 Fm. (located in the Jiaoshiba Block in Southeast Sichuan Basin). The core samples were taken from the newly drilled shale gas exploration wells. The mineral composition analysis, TOC content analysis, porosity analyses, carbon dioxide adsorption experiment, nitrogen adsorption experiment, high-pressure mercury intrusion experiment, FIB-SEM experiments and FIB-HIM experiments were carried out, and the element logging data were collected to quantitatively compare the genesis of siliceous minerals and pore structure characteristics of marine shales with different TOC contents. The conclusions are as follows:
1) The existence of excess silicon content was determined according to the contents of silicon and aluminum, as well as the results of calculation by formula, and then the Al-Fe-Mn triangle diagram analysis method used to analyze the genesis of excess silicon content was adopted to quantitatively analyze the genesis of siliceous minerals of shales. The siliceous minerals of organic shale in the target formation of study area are almost terrigenous detrital genesis; while the siliceous minerals in organic-rich shales contain 0–20% biogenic silicon.
2) The full aperture pore structure characteristics of shale can be quantitatively characterized by carbon dioxide adsorption experiment (characterizing the micropores < 2 nm), nitrogen adsorption experiment (characterizing the mesopores from 2 to 50 nm), and high-pressure mercury intrusion experiment (characterizing the macropores >50 nm). Besides, combined with FIB-SEM experiments and FIB-HIM experiments, the pore development characteristics of different components of shale can be analyzed. Clay mineral pores are mainly developed in the organic shales of the target formation in this study area, which are mainly large pores, small in quantity, irregular and poor in reservoir capacity and connectivity. The organic-rich shales in the target formation of this study area mainly develop organic pores, which are mainly composed of a large number of oval micropores and mesopores, with good reservoir capacity and connectivity.
Data Availability Statement
The raw data supporting the conclusions of this article will be made available by the authors, without undue reservation.
Author Contributions
KZ, YS, and ZJ contributed to the conception and design of the study. KZ organized the database. YS performed the statistical analysis. KZ, YS, and ZJ wrote the first draft of the manuscript. DX, LL, XY, PL, FH, LT, XW, LZ, JJ, ZZ, and XC wrote the sections of the manuscript. All authors contributed to manuscript revision, read, and approved the submitted version.
Funding
This study was supported by the National Natural Science Foundation of China (No. 42102192, No. 42130803, and No. 42072174), the open experiment fund of Southwest Petroleum University (2021KSP02029), the open fund of Shale Gas Evaluation and Exploitation Key Laboratory of Sichuan Province (YSK2022010), the open fund from the State Key Laboratory of Petroleum Resources and Prospecting (PRP/open-2107), the open fund of Key Laboratory of Tectonics and Petroleum Resources (China University of Geosciences), Ministry of Education, Wuhan (TPR-2020-07), the open funds from the State Key Laboratory of Shale Oil and Gas Enrichment Mechanisms and Effective Development (G5800-20-ZS-KFGY012), and the Science and Technology Cooperation Project of the CNPC-SWPU Innovation Alliance.
Conflict of Interest
Author DX is employed by Xinjiang Oilfield Company, PetroChina.
The remaining authors declare that the research was conducted in the absence of any commercial or financial relationships that could be construed as a potential conflict of interest.
Publisher’s Note
All claims expressed in this article are solely those of the authors and do not necessarily represent those of their affiliated organizations, or those of the publisher, the editors and the reviewers. Any product that may be evaluated in this article, or claim that may be made by its manufacturer, is not guaranteed or endorsed by the publisher.
Acknowledgments
We sincerely appreciate all reviewers and the handling editor for their critical comments and constructive suggestions.
References
Adachi, M., Yamamoto, K., and Sugisaki, R. (1986). Hydrothermal Chert and Associated Siliceous Rocks from the Northern Pacific: Their Geological Significance as Indication of Ocean ridge Activity. Sediment. Geology. 47 (1), 125–148. doi:10.1016/0037-0738(86)90075-8
Bernard, S., Horsfield, B., Schulz, H.-M., Wirth, R., Schreiber, A., and Sherwood, N. (2012a). Geochemical Evolution of Organic-Rich Shales with Increasing Maturity: A STXM and TEM Study of the Posidonia Shale (Lower Toarcian, Northern Germany). Mar. Pet. Geology. 31 (1), 70–89. doi:10.1016/j.marpetgeo.2011.05.010
Bernard, S., Wirth, R., Schreiber, A., Schulz, H.-M., and Horsfield, B. (2012b). Formation of Nanoporous Pyrobitumen Residues during Maturation of the Barnett Shale (Fort Worth Basin). Int. J. Coal Geology. 103, 3–11. doi:10.1016/j.coal.2012.04.010
Boström, K., Kraemer, T., and Gartner, S. (1973). Provenance and Accumulation Rates of Opaline Silica, Al, Ti, Fe, Mn, Cu, Ni and Co in Pacific Pelagic Sediments. Chem. Geology. 11 (2), 123–148. doi:10.1016/0009-2541(73)90049-1
Curtis, J. B. (2002). Fractured Shale-Gas Systems. AAPG Bull. 86 (11), 1921–1938. doi:10.1306/61eeddbe-173e-11d7-8645000102c1865d
Dillinger, A., and Esteban, L. (2014). Experimental Evaluation of Reservoir Quality in Mesozoic Formations of the Perth Basin (Western Australia) by Using a Laboratory Low Field Nuclear Magnetic Resonance. Mar. Pet. Geology. 57, 455–469. doi:10.1016/j.marpetgeo.2014.06.010
Gao, F. (2021). Influence of Hydraulic Fracturing of strong Roof on Mining-Induced Stress Insight from Numerical Simulation. J. Mining Strata Control. Eng. 3 (2), 023032. doi:10.13532/j.jmsce.cn10-1638/td.20210329.001
Gao, Y., Cai, X., and He, X. (2021a). Challenges and Development Direction of Experimental Testing Technology for Shale Gas Exploration and Development. Reservoir Eval. Develop. 11 (1), 30. doi:10.13809/j.cnki.cn32-1825/te.2021.02.004
Gao, Z., Fan, Y., Xuan, Q., and Zheng, G. (2020). A Review of Shale Pore Structure Evolution Characteristics with Increasing thermal Maturities. Adv. Geo-energy Res. 4 (3), 247–259. doi:10.46690/ager.2020.03.03
Gao, Z., and Hu, Q. (2018). Pore Structure and Spontaneous Imbibition Characteristics of marine and continental Shales in China. Bulletin 102 (10), 1941–1961. doi:10.1306/03291817297
Gao, Z., Liang, Z., Qinhong Hu, Q., Jiang, Z., and Xuan, Q. (2021b). A New and Integrated Imaging and Compositional Method to Investigate the Contributions of Organic Matter and Inorganic Minerals to the Pore Spaces of Lacustrine Shale in China. Mar. Pet. Geology. 127, 104962. doi:10.1016/j.marpetgeo.2021.104962
Guo, H., Ji, M., and Sun, Z. (2021). Energy Evolution Characteristics of Red sandstone under Cyclic Load. J. Mining Strata Control. Eng. 3 (4), 043019. doi:10.13532/j.jmsce.cn10-1638/td.20211008.001
Guo, T. (2021a). Geological Characteristics and Exploration Prospect of Carbonate Source Rock Gas in Sichuan Basin. J. Southwest Pet. Univ. (Science Techn. Edition) 43 (1), 1. (in Chinese with English abstract). doi:10.11885/j.issn.16745086.2020.10.20.03
Guo, T. (2016). Key Geological Issues and Main Controls on Accumulation and Enrichment of Chinese Shale Gas. Pet. Exploration Develop. 43 (3), 317–326. (in Chinese with English abstract). doi:10.1016/s1876-3804(16)30042-8
Guo, T. (2021). Progress and Research Direction of Deep Shale Gas Exploration and Development. Reservoir Eval. Develop. 11 (1), 1–6. doi:10.13809/j.cnki.cn32-1825/te.2021.01.001
Guo, X., Hu, D., Li, Y., Wei, Z., Wei, X., and Liu, Z. (2017). Geological Factors Controlling Shale Gas Enrichment and High Production in Fuling Shale Gas Field. Pet. Exploration Develop. 44, 513–523. doi:10.1016/s1876-3804(17)30060-5
Guo, X., Hu, D., and Wei, Z. (2016). Discovery and Exploration of Fuling Shale Gas Field. China Pet. Exploration 21 (3), 24–37. (in Chinese with English abstract). doi:10.3969/j.issn.1672-7703.2016.03.003
Guo, X., Li, Y., and Tenger, B. (2020). Hydrocarbon Generation and Storage Mechanisms of deepwater Shelf Shales of Ordovician Wufeng Formation Silurian Longmaxi Formation in Sichuan basin, china. Pet. Exploration Develop. 000 (001), 204–213. doi:10.1016/s1876-3804(20)60019-2
He, Z., Nie, H., and Jiang, T. (2021). Challenges and Countermeasures of Effective Development with Large Scale of Deep Shale Gas in Sichuan Basin. Reservoir Eval. Develop. 11, 1–11. doi:10.13809/j.cnki.cn32-1825/te.2021.02.001
Holdaway, H. K., and Clayton, C. J. (1982). Preservation of Shell Microstructure in Silicified Brachiopods from the Upper Cretaceous Wilmington Sands of Devon. Geol. Mag. 119, 371–382. doi:10.1017/s0016756800026285
Hou, E., Cong, T., and Xie, X. (2020). Ground Surface Fracture Development Characteristics of Shallow Double Coal Seam Staggered Mining Based on Particle Flow. J. Mining Strata Control. Eng. 2 (1), 013521. doi:10.13532/j.jmsce.cn10-1638/td.2020.01.002
Huang, H., Li, R., Chen, W., Chen, L., Jiang, Z., Xiong, F., et al. (2021). Revisiting Movable Fluid Space in Tight fine-grained Reservoirs: A Case Study from Shahejie Shale in the Bohai Bay Basin, NE China. J. Pet. Sci. Eng. 207, 109170. doi:10.1016/j.petrol.2021.109170
Huang, H., Li, R., Jiang, Z., Li, J., and Chen, L. (2020a). Investigation of Variation in Shale Gas Adsorption Capacity with Burial Depth: Insights from the Adsorption Potential Theory. J. Nat. Gas Sci. Eng. 73, 103043. doi:10.1016/j.jngse.2019.103043
Huang, H., Li, R., Xiong, F., Hu, H., Sun, W., Jiang, Z., et al. (2020b). A Method to Probe the Pore-Throat Structure of Tight Reservoirs Based on Low-Field NMR: Insights from a Cylindrical Pore Model. Mar. Pet. Geology. 117, 104344. doi:10.1016/j.marpetgeo.2020.104344
Ji, W., Song, Y., Jiang, Z., Chen, L., Li, Z., Yang, X., et al. (2015). Estimation of marine Shale Methane Adsorption Capacity Based on Experimental Investigations of Lower Silurian Longmaxi Formation in the Upper Yangtze Platform, South China. Mar. Pet. Geology. 68, 94–106. doi:10.1016/j.marpetgeo.2015.08.012
Ji, W., Song, Y., Jiang, Z., Meng, M., Liu, Q., Chen, L., et al. (2016). Fractal Characteristics of Nano-Pores in the Lower Silurian Longmaxi Shales from the Upper Yangtze Platform, South China. Mar. Pet. Geology. 78, 88–98. doi:10.1016/j.marpetgeo.2016.08.023
Ji, W., Song, Y., Jiang, Z., Wang, X., Bai, Y., and Xing, J. (2014). Geological Controls and Estimation Algorithms of Lacustrine Shale Gas Adsorption Capacity: a Case Study of the Triassic Strata in the Southeastern Ordos Basin, China. Int. J. Coal Geology. 134-135 (135), 61–73. doi:10.1016/j.coal.2014.09.005
Kang, H., Xu, G., and Wang, B. (2019). Forty Years Development and Prospects of Underground Coal Mining and Strata Control Technologies in China. J. Mining Strata Control. Eng. 1 (1), 013501. doi:10.13532/j.jmsce.cn10-1638/td.2019.02.002
Kou, Z., Zhang, D., Chen, Z., and Xie, Y. (2022). Quantitatively Determine CO2 Geosequestration Capacity in Depleted Shale Reservoir: A Model Considering Viscous Flow, Diffusion, and Adsorption. Fuel 309, 122191. doi:10.1016/j.fuel.2021.122191
Li, K., Kong, S., Xia, P., and Wang, X. (2020). Microstructural Characterisation of Organic Matter Pores in Coal-Measure Shale. Adv. Geo-energy Res. 4 (4), 372–391. doi:10.46690/ager.2020.04.04
Li, T., Jiang, Z., and Li, Z. (2019). Analysis of Differences in Pore Structure between continental and marine Shale Reservoirs. Pet. Geology. Recovery Efficiency 26, 65. doi:10.13673/j.cnki.cn37-1359/te.2019.01.007
Li, Z.-X., Li, X.-h., Zhou, H., and Kinny, P. D. (2002). Grenvillian continental Collision in South China: New SHRIMP U-Pb Zircon Results and Implications for the Configuration of Rodinia. Geol 30 (2), 163–166. doi:10.1130/0091-7613(2002)030<0163:gccisc>2.0.co;2
Li, Z.-X., Zhang, L., and Powell, C. M. (1995). South China in Rodinia: Part of the Missing Link between Australia-East Antarctica and Laurentia? Geol 23 (5), 407–410. doi:10.1130/0091-7613(1995)023<0407:scirpo>2.3.co;2
Liu, B., He, S., and Meng, L. (2021a). Sealing Mechanisms in Volcanic Faulted Reservoirs in Xujiaweizi Extension, Northern Songliao Basin, Northeastern China. AAPG Bull. 2021 (20315), 210. doi:10.1306/03122119048
Liu, B., Sun, J., Zhang, Y., He, J., Fu, X., Yang, L., et al. (2021b). Reservoir Space and Enrichment Model of Shale Oil in the First Member of Cretaceous Qingshankou Formation in the Changling Sag, Southern Songliao Basin, NE China. Pet. Exploration Develop. 48 (3), 608–624. doi:10.1016/s1876-3804(21)60049-6
Liu, J., and Zheng, M. (1993). Geochemistry of Hydrothermal Sedimentary Silicalite. Acta Geol. Sichuan 13 (2), 110–118. (in Chinese with English abstract).
Mei, L., Deng, D., and Shen, C. (2012). Tectonic Dynamics and marine Hydrocarbon Accumulation of Jiangnan-Xuefeng Uplift. Geol. Sci. Techn. Inf. 31 (5), 85–93. (in Chinese with English abstract).
Mou, C., Wang, X., and Wang, Q. (2016). Relationship between Sedimentary Facies and Shale Gas Geological Conditions of the Lower Silurian Longmaxi Formation in Southern Sichuan Basin and its Adjacent Areas. J. Palaeogeogr. 18 (3), 457–472. (in Chinese with English abstract). doi:10.7605/gdlxb.2016.03.032
Murray, R. W., Buchholtz Ten Brink, M. R., Gerlach, D. C., Russ, G. P., and Jones, D. L. (1991). Rare Earth, Major, and Trace Elements in Chert from the Franciscan Complex and Monterey Group, California: Assessing REE Sources to fine-grained marine Sediments. Geochimica et Cosmochimica Acta 55 (7), 1875–1895. doi:10.1016/0016-7037(91)90030-9
Nie, H., Bao, S., and Gao, B. (2012). A Study of Shale Gas Preservation Conditions for the Lower Paleozoic in Sichuan Basin and its Periphery. Earth Sci. Front. 19 (3), 280–294. (in Chinese with English abstract).
Nie, H., Zhang, B., and Liu, G. (2020). Geological Factors Contributing to High Shale Gas Yield in the Wufeng-Longmaxi Fms of Sichuan Basin: A Case Study of Well JY6-2HF in Fuling Shale Gas Field. Oil Gas Geology. 41 (3), 463–473. (in Chinese with English abstract). doi:10.11743/ogg20200303
Qiu, Z., Lu, B., and Chen, Z. (2019). Discussion of the Relationship between Volcanic Ash Layers and Organic Enrichment of Black Shale: A Case Study of the Wufeng-Longmaxi Gas Shales in the Sichuan Basin. Acta Sedimentologica Sinica 37 (6), 1296–1308. doi:10.14027/j.issn.1000-0550.2019.088
Shan, S., Wu, Y., and Fu, Y. (2021). Shear Mechanical Properties of Anchored Rock Mass under Impact Load. J. Mining Strata Control. Eng. 3 (4), 043034. doi:10.13532/j.jmsce.cn10-1638/td.20211014.001
Tang, X., Jiang, Z., Jiang, S., Cheng, L., and Zhang, Y. (2017). Characteristics and Origin of Iin-Ssitu Gas Desorption of the Cambrian Shuijingtuo Formation Shale Gas Reservoir in the Sichuan Basin, China. Fuel 187, 285–295. doi:10.1016/j.fuel.2016.09.072
Tang, X., Jiang, Z., Jiang, S., Wang, P., and Xiang, C. (2016). Effect of Organic Matter and Maturity on Pore Size Distribution and Gas Storage Capacity in High-Mature to Post-Mature Shales. Energy Fuels 30 (11), 8985–8996. doi:10.1021/acs.energyfuels.6b01499
Wang, G., Ju, Y., Yan, Z., and Li, Q. (2015a). Pore Structure Characteristics of Coal-Bearing Shale Using Fluid Invasion Methods: A Case Study in the Huainan-Huaibei Coalfield in China. Mar. Pet. Geology. 62, 1–13. doi:10.1016/j.marpetgeo.2015.01.001
Wang, G., Pang, Y., and Ren, H. (2020a). Intelligent Coal Mining Pattern and Technological Path. J. Mining Strata Control. Eng. 2 (1), 013501. doi:10.13532/j.jmsce.cn10-1638/td.2020.01.001
Wang, H., Kou, Z., and Guo, J. (2020b). A Semi-analytical Model for the Transient Pressure Behaviors of a Multiple Fractured Well in a Coal Seam Gas Reservoir. J. Pet. Sci. Eng. 2020, 198. doi:10.1016/j.petrol.2020.108159
Wang, J., and Li, Z. (2003). History of Neoproterozoic Rift Basins in South China: Implications for Rodinia Break-Up. PreCambrian Res. 122 (1/4), 141–158. doi:10.1016/s0301-9268(02)00209-7
Wang, J., Zhang, C., and Zheng, D. (2020c). Stability Analysis of Roof in Goaf Considering Time Effect. J. Mining Strata Control. Eng. 2 (1), 013011. doi:10.13532/j.jmsce.cn10-1638/td.2020.01.005
Wang, P., Jiang, Z., Chen, L., Yin, L., Li, Z., Zhang, C., et al. (2016a). Pore Structure Characterization for the Longmaxi and Niutitang Shales in the Upper Yangtze Platform, South China: Evidence from Focused Ion Beam-He Ion Microscopy, Nano-Computerized Tomography and Gas Adsorption Analysis. Mar. Pet. Geology. 77, 1323–1337. doi:10.1016/j.marpetgeo.2016.09.001
Wang, P., Jiang, Z., Ji, W., Zhang, C., Yuan, Y., Chen, L., et al. (2016b). Heterogeneity of Intergranular, Intraparticle and Organic Pores in Longmaxi Shale in Sichuan Basin, South China: Evidence from SEM Digital Images and Fractal and Multifractal Geometries. Mar. Pet. Geology. 72, 122–138. doi:10.1016/j.marpetgeo.2016.01.020
Wang, P., Jiang, Z., and Li, P. (2018). Organic Matter Pores and Evolution Characteristics of Shales in the Lower Silurian Longmaxi Formation and the Lower Cambrian Niutitang Formation in Periphery of Chongqing. Nat. Gas Geosci. 29 (7), 997–1008. doi:10.11764/j.issn.1672-1926.2018.06.012
Wang, P., Jiang, Z., Yin, L., Chen, L., Li, Z., Zhang, C., et al. (2017). Lithofacies Classification and its Effect on Pore Structure of the Cambrian marine Shale in the Upper Yangtze Platform, South China: Evidence from FE-SEM and Gas Adsorption Analysis. J. Pet. Sci. Eng. 156, 307–321. doi:10.1016/j.petrol.2017.06.011
Wang, R., Hu, Z., and Dong, L. (2021). Advancement and Trends of Shale Gas Reservoir Characterization and Evaluation. Oil Gas Geology. 42 (1), 54. doi:10.11743/ogg20200105
Wang, R., Hu, Z., Long, S., Liu, G., Zhao, J., Dong, L., et al. (2019). Differential Characteristics of the Upper Ordovician‐Lower Silurian Wufeng‐Longmaxi Shale Reservoir and its Implications for Exploration and Development of Shale Gas In/around the Sichuan Basin. Acta Geologica Sinica ‐ English Edition 93 (3), 520–535. doi:10.1111/1755-6724.13875
Wang, R., Nie, H., and Hu, Z. (2020d). Controlling Effect of Pressure Evolution on Shale Gas Reservoirs: A Case Study of the Wufeng–Longmaxi Formation in the Sichuan Basin. Nat. Gas Industry 40 (10), 1. doi:10.3787/j.issn.1000-0976.2020.10.001
Wang, X., Jiang, Z., Zhang, K., Wen, M., Xue, Z., Wu, W., et al. (2020e). Analysis of Gas Composition and Nitrogen Sources of Shale Gas Reservoir under strong Tectonic Events: Evidence from the Complex Tectonic Area in the Yangtze Plate. Energies 13 (1), 281. doi:10.3390/en13010281
Wang, Y., Dong, D., and Li, X. (2015b). Stratigraphic Sequence and Sedimentary Characteristics of Lower Silurian Longmaxi Formation in the Sichuan Basin and its Peripheral Areas. Nat. Gas Industry 35 (3), 12–21. (in Chinese with English abstract). doi:10.3787/j.issn.1000-0976.2015.03.002
Wedepohl, K. H. (1971). Environmental Influences on the Chemical Composition of Shales and Clays. Phys. Chem. Earth 8, 307–333. doi:10.1016/0079-1946(71)90020-6
Xia, Y., Lu, C., and Yang, G. (2020). Experimental Study on Axial Fracture Cutting and Fracturing of Abrasive Jet in Hard Roof Hole. J. Mining Strata Control. Eng. 2 (3), 033522. doi:10.13532/j.jmsce.cn10-1638/td.20200522.001
Yamamoto, K. (1987). Geochemical Characteristics and Depositional Environments of Cherts and Associated Rocks in the Franciscan and Shimanto Terranes. Sediment. Geology. 52, 65–108. doi:10.1016/0037-0738(87)90017-0
Yang, J., Wang, D., Mao, J., Zhang, Z., Zhang, Z., and Wang, Z. (1999). The Petrochemical Research Method for Silicalite and its Application to the “Jingtieshan Type” Iron Deposits. Acta Petrologica et Mineralogical 18 (2), 108–118. (in Chinese with English abstract). doi:10.3969/j.issn.1000-6524.1999.02.002
Yu, X., Bian, J., and Liu, C. (2022). Determination of Energy Release Parameters of Hydraulic Fracturing Roof Near Goaf Based on Surrounding Rock Control of Dynamic Pressure Roadway. J. Mining Strata Control. Eng. 4 (1), 013016. doi:10.13532/j.jmsce.cn10-1638/td.20210908.001
Zhang, K., Jia, C., Song, Y., Jiang, S., Jiang, Z., Wen, M., et al. (2020a). Analysis of Lower Cambrian Shale Gas Composition, Source and Accumulation Pattern in Different Tectonic Backgrounds: A Case Study of Weiyuan Block in the Upper Yangtze Region and Xiuwu Basin in the Lower Yangtze Region. Fuel 263, 115978. doi:10.1016/j.fuel.2019.115978
Zhang, K., Jiang, S., Zhao, R., et al. (2022a). Connectivity of Organic Matter Pores in the Lower Silurian Longmaxi Formation Shale, Sichuan Basin, Southern China: Analyses from Helium Ion Microscope and Focused Ion Beam Scanning Electron Microscope. Geol. J., 1–13. doi:10.1002/gj.4387
Zhang, K., Peng, J., and Liu, W. (2020b). The Role of Deep Geofluids in the Enrichment of Sedimentary Organic Matter: a Case Study of the Late Ordovician-Early Silurian in the Upper Yangtze Region and Early Cambrian in the Lower Yangtze Region, south China. Geouids 2020, 8868638. doi:10.1155/2020/8868638
Zhang, K., Peng, J., and Wang, X. (2020c). Effect of Organic Maturity on Shale Gas Genesis and Pores Development: A Case Study on marine Shale in the Upper Yangtze Region, South China. Open Geosciences 12 (2020), 1617–1629. doi:10.1515/geo-2020-0216
Zhang, K., Song, Y., Jiang, Z., Yuan, X., Wang, X., Han, F., et al. (2022b). Research on the Occurrence State of Methane Molecules in Postmature Marine Shales-A Case Analysis of the Lower Silurian Longmaxi Formation Shales of the Upper Yangtze Region in Southern China. Front. Earth Sci. 10, 864279. doi:10.3389/feart.2022.864279
Zhang, L., Kou, Z., Wang, H., Zhao, Y., Dejam, M., Guo, J., et al. (2018). Performance Analysis for a Model of a Multi-wing Hydraulically Fractured Vertical Well in a Coalbed Methane Gas Reservoir. J. Pet. Sci. Eng. 166, 104–120. doi:10.1016/j.petrol.2018.03.038
Zhang, Z., Zhang, K., Song, Y., Jiang, Z., Jiang, S., Wang, P., et al. (2021). Study on the Formation Mechanism of Shale Roof, Floor Sealing, and Shale Self-Sealing: A Case of Member I of the Upper Ordovician Wufeng Formation-Lower Silurian Longmaxi Formation in the Yangtze Region. Front. Earth Sci. 9, 764287. doi:10.3389/feart.2021.764287
Zou, C., Dong, D., Wang, Y., Li, X., Huang, J., Wang, S., et al. (2016). Shale Gas in China: Characteristics, Challenges and Prospects (II). Pet. Exploration Develop. 432, 182–196. doi:10.1016/s1876-3804(16)30022-2
Zou, C., Dong, D., and Wang, Y. (2015). Shale Gas in China: Characteristics, Challenges and Prospects (I). Pet. Exploration Develop. 42 (6), 689–701. (in Chinese with English abstract). doi:10.1016/s1876-3804(15)30072-0
Zou, C., Zhao, Q., and Dong, D. (2017). Geological Characteristics, Main Challenges and Future prospect of Shale Gas. J. Nat. Gas Geosci. 2 (5-6), 273–288. doi:10.1016/j.jnggs.2017.11.002
Zuo, J., Yu, M., and Hu, S. (2019). Experimental Investigation on Fracture Mode of Different Thick Rock Strata. J. Mining Strata Control. Eng. 1 (1), 013007. doi:10.13532/j.jmsce.cn10-1638/td.2019.02.008
Nomenclature
Als the aluminum content in the sample, unit:wt.%Clay minerals, unit:wt%
FIB-HIM Focused ion beam-Helium ion microscopy
FIB-SEM Focused ion beam-scanning electron microscopy Pore Diameter, unit:nm Pore volume, unit:ml/g Porosity, unit:%
Siex Excess siliceous mineral content, unit:wt% Siliceous minerals, unit:wt%
Sis the content of silicon in the sample, unit:wt%
(Si/Al)bg 3.11, which is the average content in shale
TOC Total organic carbon, unit:wt%
Keywords: marine shales, quantitative analysis, genesis of siliceous minerals, organic pores, clay mineral pores, connectivity
Citation: Zhang K, Song Y, Jiang Z, Xu D, Li L, Yuan X, Liu P, Han F, Tang L, Wang X, Zhang L, Jiang J, Zheng Z and Chen X (2022) Quantitative Comparison of Genesis and Pore Structure Characteristics of Siliceous Minerals in Marine Shale With Different TOC Contents–A Case Study on the Shale of Lower Silurian Longmaxi Formation in Sichuan Basin, Southern China. Front. Earth Sci. 10:887160. doi: 10.3389/feart.2022.887160
Received: 01 March 2022; Accepted: 04 March 2022;
Published: 22 March 2022.
Edited by:
Shu Jiang, The University of Utah, United StatesReviewed by:
Tingwei Li, Guangzhou Marine Geological Survey, ChinaTao Jiang, Chinese Academy of Geological Science, China
Ling Tang, CNOOC Research Institute Ltd., China
Copyright © 2022 Zhang, Song, Jiang, Xu, Li, Yuan, Liu, Han, Tang, Wang, Zhang, Jiang, Zheng and Chen. This is an open-access article distributed under the terms of the Creative Commons Attribution License (CC BY). The use, distribution or reproduction in other forums is permitted, provided the original author(s) and the copyright owner(s) are credited and that the original publication in this journal is cited, in accordance with accepted academic practice. No use, distribution or reproduction is permitted which does not comply with these terms.
*Correspondence: Yan Song, c3lhQHBldHJvY2hpbmEuY29tLmNu