- State Key Laboratory of Severe Weather, Institute of Tibetan Plateau and Polar Meteorology, Chinese Academy of Meteorological Sciences, Beijing, China
Siberian High (SH) is the dominant pressure system located in the mid-high latitudes of Eurasia during boreal wintertime. This study reveals a triggering impact of SH variation in preceding winter on the following ENSO events, and gives a possible explanation via diagnosing the SH-associated air-sea response over the tropical Pacific and North Pacific. When SH is anomalously enhanced (suppressed) during boreal winter, an Aleutian Low enhanced (suppressed) response will occur over the downstream North Pacific. The Aleutian Low response gradually evolves into a meridional dipole structure similar to the negative (positive) phase of the North Pacific Oscillation (NPO) during the following spring and early summer. Correspondingly, the oceanic response in the North Pacific features a pattern similar to the negative (positive) phase of the Victoria mode. These SH-associated air-sea responses over the subtropical North Pacific will be maintained and further delivered into the tropical Pacific through the so-called seasonal footprinting mechanism, which favors the Bjerknes feedback established around boreal summer and finally grows into a La Niña (El Niño).
Introduction
The El Niño-Southern Oscillation (ENSO) is the dominant interannual variability in the tropical Pacific, which can directly impact the weather and climate in the tropical Pacific and adjacent continents, and have remote effects worldwide through some teleconnection processes (Rasmusson and Carpenter, 1982; Philander, 1983; Jin, 1996; Trenberth et al., 1998; Paegle and Mo, 2002). Nowadays, ENSO plays an important role in seasonal and interannual climate predictions in plenty of regions (e.g., Zhang et al., 1996; McPhaden et al., 2006a; Zhang et al., 2015; Infanti and Kirtman, 2016; Luo et al., 2016). Thus, it is of great value for sciences and social benefits to understand the dynamics and climate impacts of ENSO.
In recent decades, substantial attention has been paid to the ENSO diversity and complexity, and one important part is the diversity of ENSO triggers (Timmermann et al., 2018; Ren et al., 2020). Many studies have indicated that the tropical Pacific air-sea interaction plays a fundamental role in the ENSO dynamics (Bjerknes, 1969; Neelin et al., 1998; Wang, 2018; Ren et al., 2020). However, forcings from the extratropical Pacific are also of great importance in modulating the ENSO outbreaks (e.g., Terray, 2011; Wang et al., 2011). For example, North Pacific Oscillation (NPO) can cause a sea surface temperature (SST) “footprint” when its subtropical portion (0°–20°N) forces the overlying atmosphere, resulting in zonal wind stress anomalies that favor the initiation of an ENSO event (Vimont et al., 2001, 2003; Amaya, 2019). The Victoria Mode (VM), a vital interannual-scale mode of the North Pacific SST anomaly, was also demonstrated to be a potential ENSO precursor (Ding et al., 2015a; 2015b). In addition, the anomalous SST warming in the Indian and Atlantic Oceans could influence the ENSO development via interbasin interactions (Li and Mu, 2000; Wang et al., 2017).
Previous studies were more like to seek the possible ENSO triggers in oceans, while there was relatively little attention paid to the impact from atmospheric circulation systems located on the inner continent. The Siberian High (SH) is the most vital high-pressure system in the lower troposphere over Mongolia-Siberia during boreal wintertime, which dominates the low-level atmospheric circulation and the associated anomalous events in almost the whole Asian continent (Ding, 1990; Miyazaki et al., 1999; Gong and Ho, 2002). Moreover, SH can modulate the hemispheric-scale circulation over the downstream Pacific Ocean and more distant regions (Cohen et al., 2001; Wu and Wang, 2002). Given that the tropical air-sea interaction has been found closely tied to variabilities in North Pacific (Bond et al., 2003), it is reasonable to deduce that SH may also have a remote impact on ENSO.
This paper intends to examine the possible impact of the boreal winter SH on the ENSO development in the subsequent year, and explain how the SH-associated impacts maintain and propagate to the tropical Pacific. The remainder of this paper is organized as follows. Data and Methodology introduces the data and methods. Relationship Between the Preceding SH and ENSO in the Following Winter reveals the linkage between the preceding boreal winter SH and the ENSO in the following winter. The associated physical processes of how SH affects the following ENSO development are described in Possible Mechanisms Linking the Preceding SH to ENSO. Summary and Discussion gives a summary and brief discussion.
Data and Methodology
The present study employs monthly SST data from the Met Office, Hadley Centre Sea Ice and Sea Surface Temperature dataset (HadISST), with a horizontal resolution of 1° × 1° (Rayner et al., 2003). Atmospheric variables, including monthly sea level pressure (SLP), surface wind stress, pressure-level winds, and surface upward latent heat flux are obtained from the fifth-generation European Center for Medium-Range Weather Forecasts (ECMWF) reanalysis of the global climate (ERA5; Hersbach et al., 2020) at 1° × 1° resolution. All analyses are conducted with a period of 1980–2020. Anomaly is obtained as the departure from the climatological mean of 1981–2010. In order to remove the influence of climate change, the long-term linear trend has been removed.
Following the previous studies (Gong and Ho, 2002), we focus on the variation of SH during late winter (January-February-March, JFM). Siberian High intensity (SHI) index is defined as the mean SLP over a key region (70°–120°E, 40°–60°N), which generally covers the central regions of SH (Figure 1). Niño-3.4 index, defined as the averaged SST anomalies over 5°S–5°N and 170°–120°W, is employed to represent the ENSO variability. Regression, correlation, and composite analyses are used to clarify the typical characteristics of the SH-associated air-sea responses, and their statistical significance is determined with the two-tailed Student’s t test.
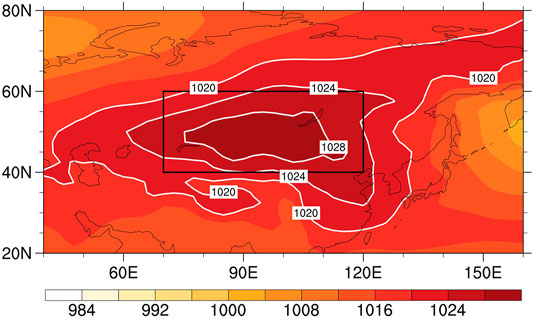
FIGURE 1. Winter (January-February-March) sea level pressure climatology. Reference period is 1981–2010. Only pressures exceeding 1020hPa are shown as white lines. The values of SLP for the box region are averaged to define the intensity index of the winter Siberian High.
Relationship Between the Preceding SH and ENSO in the Following Winter
In this section, we first examined the relationship between the winter SH and tropical Pacific SST anomalies in the following year. Figure 2 shows the correlation evolution of the JFM SH with the lagged meridional mean (5°S–5°N) of tropical SST anomalies. In total, it highly resembles a typical eastward propagation of ENSO development. A significant negative correlation between the winter SH and following SST anomalies in the equatorial Pacific establishes in the late spring, firstly occurring in the western and central Pacific. This negative correlation will be rapidly enhanced and eastward spread in the following seasons. The strongest correlation between SH and SST anomalies over the Niño-3.4 region occurs at the end of the year (November-December-January (1), NDJ (1), where (1) indicates time comes to the next year). Therefore, the preceding SH variation is likely to be a precursor of an ENSO development in the following year.
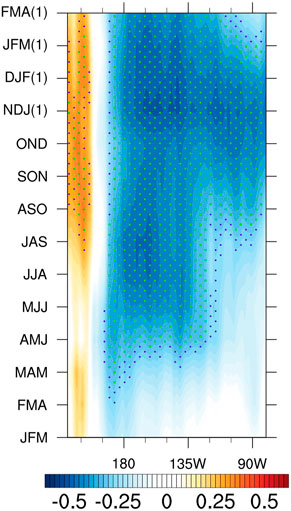
FIGURE 2. Evolution of equatorial (5°S–5°N-averaged) SST anomalies (Unit: °C) obtained by correlation on the normalized JFM SHI index. Stippled regions in green (purple) indicate anomalies significant at the 90% (95%) confidence level according to the Student’s t test.
Motivated by the high correlation between the winter SH and tropical Pacific SST anomalies in the following winter, we then examine the yearly correspondence of the normalized JFM SHI and NDJ (1) Niño-3.4 indices during the period of 1980–2020, as shown in Figure 3. The correlation coefficient of these two indices is −0.46, which is statistically significant at the 99% confidence level. In the previous winter of the three super El Niño events after 1980 (i.e., El Niños in 1982/1983, 1997/1998, and 2015/2016), the SHs were all relatively suppressed. Meanwhile, preceding some typical La Niña events (e.g., La Niñas in 1988/1989, 2010/2011), there were enhanced SHs in winter (Figure 3A). In specific, after 12 enhanced SH winters [JFM SHI index >0.5 standard deviations (STDs)], there are 6 years with a La Niña event (NDJ (1) Niño-3.4 index < -0.5 STDs) developed in the subsequent winter. Comparatively, in 9 years out of 15 suppressed winter SH years (JFM SHI index < -0.5 STDs), the Niño-3.4 region becomes anomalous warming in the following, and six of them grow into an El Niño winter (NDJ (1) Niño-3.4 index >0.5 STDs) (Figure 3B). The high correspondence of the two indices suggests again that there is a close relationship between the SH variation in the preceding winter and the development of ENSO in the following winter. Moreover, the potential impact of preceding SH on ENSO is generally symmetric between the enhanced and suppressed SH.
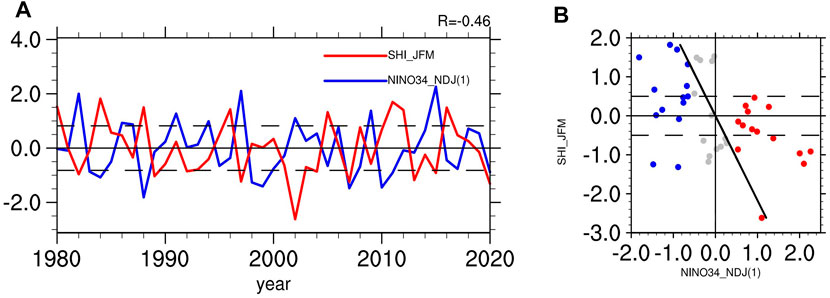
FIGURE 3. (A) Time series of the JFM SHI index and NDJ (1) Niño-3.4 index during 1980–2020. The correlation coefficient shown in the upper right corner is the correlation with JFM SHI index leading NDJ (1) Niño-3.4 index. (B) Scatter plots of the JFM SHI and NDJ (1) Niño-3.4 indices. Both indices are normalized by their standard deviations (STDs), and the red (blue) points are the years with Niño-3.4 indices greater than 0.5 STDs (less than −0.5 STDs), indicating strong El Niño (La Niña) events; grey spots are neutral events. The lines represent the regression fit. Dashed lines indicate that JFM SHI index is equal to 0.5 STDs.
To explore the typical characteristics of the potential impacts of the preceding SH, we select the historical years when apparent SH variation leads to the following ENSO events and conduct a composite analysis on the atmospheric and oceanic anomalies during these years. The six La Niña years with enhanced SH (1984, 1988, 2005, 2008, 2010, 2011) are identified as years with the JFM SHI index exceeding 0.5 STDs and NDJ (1) Niño-3.4 index lower than -0.5 STDs. Similarly, six El Niño years with suppressed SH (1982, 1997, 2002, 2004, 2009, 2015) are years with the JFM SHI index lower than -0.5 STDs and NDJ (1) Niño-3.4 index higher than 0.5 STDs. As shown in Figure 4A, when the SH is anomalously enhanced during the boreal wintertime, there is no significant synchronized SST response in the tropical Pacific, but some easterly wind anomalies occur over the warm pool. In the North Pacific, there is a significant low-level westerly wind anomaly over around 30°–40°N, with a strong anticyclonic atmospheric circulation at its southern flank. Accordingly, there are weak SST warming responses excited underlying the anomalous winds (Figure 4A). Coming to the late spring (April-May-June, AMJ), the subtropical anticyclone and corresponding horseshoe-shaped SST response are further intensified. The negative SST anomalies in the subtropical Pacific are enhanced and southward extend to the tropical central Pacific and eastern Pacific. In addition, the easterly wind anomalies only covering the warm pool in the preceding season also extend to the tropical central-eastern Pacific (Figure 4B).
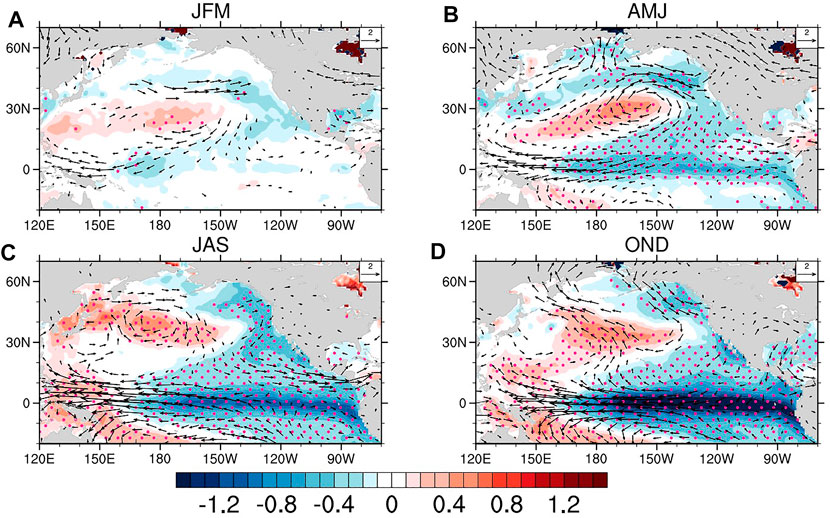
FIGURE 4. Composite SST (°C) and 850-hPa winds (vectors; m s−1) in the La Niña years with enhanced SH and El Niño years with suppressed SH (multiply by a factor of -1) during (A) January-February-March (JFM), (B) April-May-June (AMJ), (C) July-August-September (JAS) and (D) October-November-December (OND). Stippled regions indicate anomalies significant at the 90% confidence level. Only wind anomalies significant at the 90% confidence level are plotted.
Entering the second half of the year, the meridional horseshoe-shaped pattern of SST response in the North Pacific shows little changes except the westward extending of warming SST to the Asian coasts and warm pool area. In the tropics, the negative SST anomalies are further enhanced, and the center of anomalies gradually extends from the western Pacific to the central-eastern Pacific. Meanwhile, the easterly wind anomalies develop and cover the whole Pacific equatorial band (Figures 4C,D). These variations can be attributed to the positive feedback of zonal wind and SST gradient, namely, the so-called Bjerknes feedback (Bjerknes, 1969; Neelin et al., 1998; Wang, 2018; Ren et al., 2020). The above composite analysis implies that the North Pacific may be a critical passway of the SH impacts, where the anomalous signal maintains and propagates before the Bjerknes feedback established in the second half of the year.
Possible Mechanisms Linking the Preceding SH to ENSO
The above results have shown that the JFM SH, as a precursor, may influence the occurrence and development of ENSO. There is no doubt that the strong tropical Bjerknes feedback dominates the development of ENSO during the second half of the year. However, how the preceding SH initiates the Bjerknes feedback in the first half of the year remains unclear. Given that North Pacific may be the vital passway, next, we examined the evolutionary features of North Pacific air-sea anomalies associated with the SH during the first half of the year to clarify the possible mechanisms.
Figure 5 illustrates regressions of the North Pacific SLP and 850-hPa wind anomalies in JFM and AMJ upon the JFM SHI index. The JFM SLP anomalies exhibit a negative response over the mid-latitude North Pacific, resembling an enhanced Aleutian Low (AL) anomaly (Figure 5A) (Overland et al., 1999). The corresponding variation between the SH and AL reflects the connectivity among atmospheric centers of action. Referring to previous work (Chen et al., 2020), an AL intensity (ALI) index is defined to describe the strength variation of AL (Table 1). As shown in Figure 6, the AL response over the North Pacific to the JFM SH begins quickly and remains throughout the winter and spring. The negative correlation reaches the highest when AL lags SH about 1 month. Affected by the enhanced AL, significant low-level westerly wind anomalies occur at the southern flank of AL. Besides, there are easterly wind anomalies confined over the warm pool (Figure 5A). Moreover, the westerly wind anomalies at the southern flank of AL are opposite to the background winds, which may cause the warm SST anomalies observed in the central subtropical North Pacific (Figure 7A) by weakening the local evaporation (Figure 8A) (Chen et al., 2020).
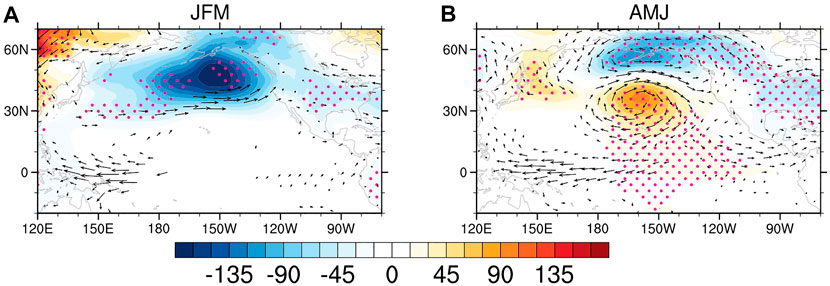
FIGURE 5. Regression of 850-hPa winds (vectors; m s−1) and SLP (shading; hPa) anomalies in (A) JFM, (B) AMJ onto the normalized JFM SHI index. Stippled regions indicate SLP anomalies significant at the 90% confidence level. Only wind anomalies significant at the 90% confidence level are plotted.
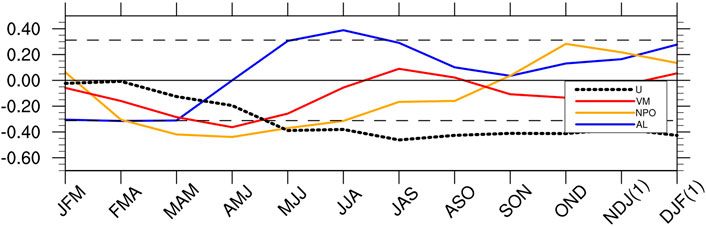
FIGURE 6. Lag correlation coefficients between the JFM SHI and the AL (blue), NPO (yellow), VM (red), U (black) indices during 1979–2020. The U index is defined as the regional mean 10-m zonal wind anomaly in the equatorial eastern-central Pacific (10°S–10°N, 180° E–120°W). The horizontal axis represents the target month of used AL, NPO, VM, and U indices. The dashed lines indicate the correlation coefficient significant at the 95% confidence level. Time notations indicate the year alongside and (1) indicate time comes to the next year.
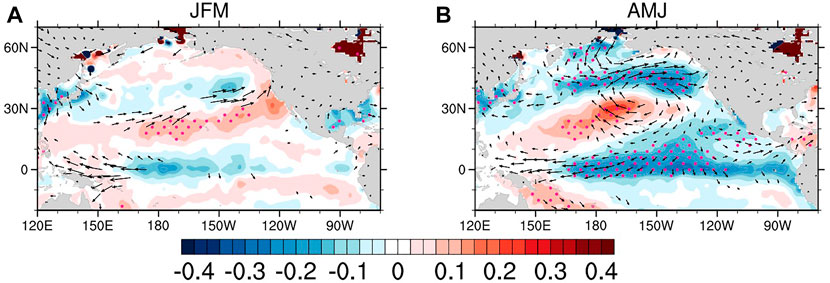
FIGURE 7. Regression of SST anomalies (°C) and 10-m winds (vectors; m s−1) anomalies in (A) JFM and (B) AMJ onto the normalized JFM SHI index. Stippled regions indicate anomalies significant at the 90% confidence level. Only wind anomalies significant at the 90% confidence level are plotted.
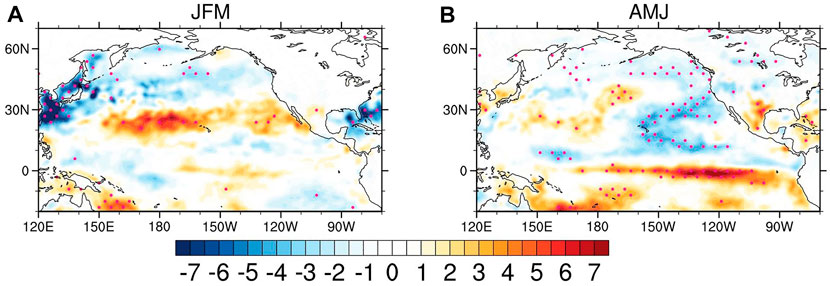
FIGURE 8. Regression of surface upward latent heat flux (shading; W m−2) anomalies in (A) JFM, (B) AMJ onto the normalized JFM SHI index. Stippled regions indicate latent heat flux anomalies significant at the 90% confidence level. Downward fluxes are positive, indicating the transfer of energy from the atmosphere to the ocean.
The anomalous AL response in the mid-latitude North Pacific diminishes rapidly in spring (Figure 5B). The SH-related SLP anomalies change from the anomalous AL pattern to a meridional dipole pattern with centers in the 60°N and 30° N North Pacific, respectively, which is similar to the pattern of NPO (Wallace and Gutzler, 1981). Several studies have suggested that such variation may be attributed to the interaction between the synoptic-scale eddy activity and mean flow and associated vorticity transportation. Westerly wind anomalies at the southern flank of the enhanced AL are accompanied by an increased synoptic-scale eddy activity, which will excite an anomalous anticyclonic circulation in the subtropical North Pacific (Chen et al., 2014; Chen et al., 2020). Connected with atmospheric circulation changes, a remarkable northeast-southwest dipole mode of SST anomalies occurs in the North Pacific in late spring (AMJ), namely, the so-called VM pattern (Bond et al., 2003; Ding et al., 2015b). The positive SST anomalies in the central subtropical North Pacific are enhanced. Moreover, significant negative SST anomalies occur at the northern and southern flanks of the positive anomalies, extending from the coast of North America to the central subtropical North Pacific (Figure 7B).
The correlation evolution of indices between the JFM SHI and associated air-sea responses in the North Pacific also reflects the aforementioned processes (Figure 6). The SHI index poorly correlates with the NPO and VM indices in late winter. While in spring, when the relationship between SH and AL decays, the correlation between JFM SH and NPO becomes significant and can last throughout the whole summer, indicating that the SH-related atmospheric response gradually changes into an NPO-like pattern. Meanwhile, the negative correlation between the VM and JFM SH also becomes significant in late spring.
Previous studies suggested that the NPO-induced SLP anomalies linking with the North Pacific trade winds can excite surface and subsurface temperature anomalies in the equatorial Pacific, which is beneficial to developing ENSO events (Vimont et al., 2001; Vimont et al., 2003; Anderson et al., 2013). Moreover, the SST anomalies featuring a VM pattern can also transfer the anomaly from extratropical North Pacific to equatorial Pacific and support the occurrence of ENSO events (Ding et al., 2015a; Ding et al., 2015b). When SH is enhanced in the preceding winter, the NPO-like pattern in late spring features an anticyclonic circulation over the subtropical North Pacific. The northeasterly wind anomalies at its southern side strengthen the trade winds (Figure 5B), thus sharpening the evaporation over the subtropical northeastern Pacific (Figure 8B) and leading to the local cooling anomalies (Figure 7B). The cooling anomalies can further strengthen the northeasterly wind anomalies and evaporation through the so-called wind-evaporation-SST feedback, which can sustain and stretch to the central equatorial Pacific during the spring and summer (Xie and Philander, 1994; Chiang and Vimont, 2004). The coupled easterly winds and cooling SST anomalies extending to the equator will enhance the zonal air-sea interaction over the equatorial Pacific. Besides, the associated off-equatorial wind stress curl anomalies may lead to a discharge of the subsurface heat content (Ding et al., 2015b; Amaya, 2019). Both processes contribute to establishing the tropical Bjerknes feedback, favoring the subsequent ENSO development. As shown in Figure 6, the negative correlation between zonal wind anomalies in the central-eastern equatorial Pacific and JFM SHI is insignificant until summer. With the development of ENSO, this negative correlation will last in the second half of the year.
Summary and Discussion
As one of the vital atmospheric circulation systems in the northern hemisphere during boreal wintertime, SH has remote impacts on the hemispheric-scale atmospheric circulation over the downstream regions. Our study found that the SH variation in the previous winter is well correlated with the tropical Pacific SST anomalies in the following year, which can be used as an atmospheric precursor of ENSO. An enhanced SH in the previous winter is likely to trigger a La Niña-related SST anomalous pattern in the central-eastern equatorial Pacific in the following winter. Conversely, a suppressed SH may lead to an El Niño event.
Through diagnosing the air-sea responses over the North Pacific and tropical Pacific, we gave a possible explanation of how the SH impacts maintain, propagate, and eventually trigger an ENSO in the following year. As summarized schematically in Figure 9, in response to a strengthened boreal winter SH, an enhanced AL response will occur over the downstream North Pacific. During the late spring (AMJ), this anomalous AL response gradually evolves into an NPO-like SLP structure. Correspondingly, the North Pacific SST anomalies feature a typical VM pattern. In the subtropical North Pacific, the NPO-like atmospheric circulation and VM-associated SST anomalies cause a cooling SST “footprint.” This cooling SST and associated northeasterly wind anomalies can be enhanced and delivered to the central Pacific through the seasonal footprinting mechanism. That contributes to the rapid development of the tropical Bjerknes feedback in summer and eventually results in a La Niña event. Similarly, the North Pacific air-sea responses will be in the opposite phase when a suppressed SH occurs, contributing to an El Niño event.
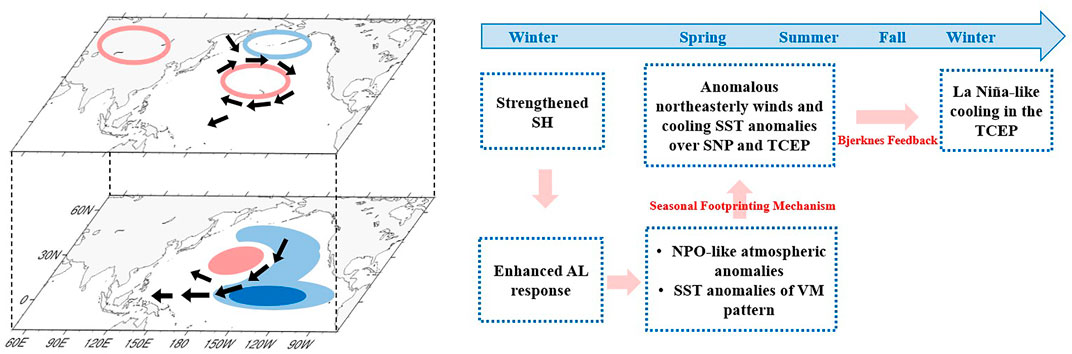
FIGURE 9. A mechanism diagram showing the processes linking the SH to the subsequent winter ENSO. Red (blue) circles indicate positive (negative) SLP anomalies. Red (blue) shading denotes positive (negative) SST anomalies. Black arrows are surface wind anomalies. SNP and TCEP denote subtropical North Pacific and tropical central-eastern Pacific, respectively.
This study links the preceding SH variation and following ENSO development through air-sea interactions over North Pacific. However, it should be noticed that these North Pacific air-sea interactions may be sensitive to the background interdecadal time scales. For example, the Arctic Oscillation showing a substantial interdecadal variability could significantly affect the seasonal footprinting mechanism (Yeo et al., 2012; Chen et al., 2013). Some interdecadal variabilities, such as Pacific Decadal Oscillation, can directly change the linkage between North Pacific and tropical Pacific (Vimont et al., 2003; Krishnamurthy and Krishnamurthy, 2014). How the SH-ENSO relationship will change under different interdecadal modulation, and the detailed mechanisms are worthy of being further investigated. Besides, there may be more possible ways of the SH impact on following ENSO. For example, SH is one of the critical components of the East Asian Winter Monsoon (EAWM) system. Associated with the EAWM variation, there may be anomalous Madden-Julian Oscillation activity and westerly winds over the western equatorial Pacific, thus significantly impacting the following ENSO development (Lengaigne et al., 2004; McPhaden et al., 2006b; Chen et al., 2017). This hypothesis and its detailed mechanism need to be further diagnosed in the future.
The impact of preceding SH on the following ENSO complements the understanding of the complexity and diversity of ENSO developments. It also provides a new explanation for the onset of ENSO events without apparent precursors in the tropical Pacific. However, this understanding is still in infancy, and further numerical validation needs to be carried out in the future. The SH variation is relatively easy to monitor and represent compared to other extratropical ENSO precursors, such as the NAO and VM. Besides, it precedes around 1 year before the following ENSO, which occurs even prior to the spring predictability barrier of ENSO (Webster and Yang, 1992). Thus, the SH variation promises to be an effective long-leading predictor of ENSO, which can help improve the seasonal-interannual ENSO prediction and weaken the predictability barrier if reasonably used.
Data Availability Statement
Publicly available datasets were analyzed in this study. This data can be found here: The Met Office, Hadley Centre Sea Ice and Sea Surface Temperature dataset (HadISST): https://www.metoffice.gov.uk/hadobs/hadisst/, and the ERA-5 dataset of the European Centre for Medium-Range Weather Forecasts: https://cds.climate.copernicus.eu/cdsapp#!/dataset/reanalysis-era5-pressure-levels-monthly-means?tab=overview.
Author Contributions
JF, ML, and RW contributed to conception and design of the study. JF organized the database. JF and SZ performed the statistical analysis. JF wrote the first draft of the manuscript. ML, RW, and YW contributed to manuscript revision, read, and approved the submitted version.
Funding
This work was jointly supported by the National Natural Science Foundation of China under Grant 42105067, 41975094, and the Basic Research and Operational Special Project of CAMS (2021Z007).
Conflict of Interest
The authors declare that the research was conducted in the absence of any commercial or financial relationships that could be construed as a potential conflict of interest.
Publisher’s Note
All claims expressed in this article are solely those of the authors and do not necessarily represent those of their affiliated organizations or those of the publisher, the editors, and the reviewers. Any product that may be evaluated in this article, or claim that may be made by its manufacturer, is not guaranteed or endorsed by the publisher.
References
Amaya, D. J. (2019). The Pacific Meridional Mode and ENSO: a Review. Curr. Clim. Change Rep. 5, 296–307. doi:10.1007/s40641-019-00142-x
Anderson, B. T., Perez, R. C., and Karspeck, A. (2013). Triggering of El Niño Onset through Trade Wind-Induced Charging of the Equatorial Pacific.. Geophys. Res. Lett. 40, 1212–1216. doi:10.1002/grl.50200
Bjerknes, J. (1969). Atmospheric Teleconnections from the Equatorial Pacific1. Mon. Wea. Rev. 97, 163–172. doi:10.1175/1520-0493(1969)097<0163:atftep>2.3.co;2
Bond, N. A., Overland, J. E., Spillane, M., and Stabeno, P. (2003). Recent Shifts in the State of the North Pacific. Geophys. Res. Lett. 30, a–n. doi:10.1029/2003GL018597
Chen, S. F., Yu, B., and Chen, W. (2014). An Analysis on the Physical Process of the Influence of AO on ENSO. Clim. Dyn. 42, 973–989. doi:10.1007/s00382-012-1654-z
Chen, S., Chen, W., Wu, R., Yu, B., and Graf, H.-F. (2020). Potential Impact of Preceding Aleutian Low Variation on El Niño-Southern Oscillation during the Following Winter. J. Clim. 33, 3061–3077. doi:10.1175/JCLI-D-19-0717.1
Chen, S., Chen, W., Yu, B., and Graf, H.-F. (2013). Modulation of the Seasonal Footprinting Mechanism by the Boreal spring Arctic Oscillation. Geophys. Res. Lett. 40, 6384–6389. doi:10.1002/2013GL058628
Chen, X., Li, C., Ling, J., and Tan, Y. (2017). Impact of East Asian winter Monsoon on MJO over the Equatorial Western Pacific. Theor. Appl. Climatol 127, 551–561. doi:10.1007/s00704-015-1649-x
Chiang, J. C. H., and Vimont, D. J. (2004). Analogous Pacific and Atlantic Meridional Modes of Tropical Atmosphere-Ocean Variability*. J. Clim. 17, 4143–4158. doi:10.1175/JCLI4953.1
Cohen, J., Saito, K., and Entekhabi, D. (2001). The Role of the Siberian High in Northern Hemisphere Climate Variability. Geophys. Res. Lett. 28, 299–302. doi:10.1029/2000GL011927
Ding, R., Li, J., Tseng, Y.-H., and Ruan, C. (2015a). Influence of the North Pacific Victoria Mode on the Pacific ITCZ Summer Precipitation. J. Geophys. Res. Atmos. 120, 964–979. doi:10.1002/2014JD022364
Ding, R., Li, J., Tseng, Y.-H., Sun, C., and Guo, Y. (2015b). The Victoria Mode in the North Pacific Linking Extratropical Sea Level Pressure Variations to ENSO. J. Geophys. Res. Atmos. 120, 27–45. doi:10.1002/2014JD022221
Gong, D.-Y., and Ho, C.-H. (2002). The Siberian High and Climate Change over Middle to High Latitude Asia. Theor. Appl. Climatology 72, 1–9. doi:10.1007/s007040200008
Hersbach, H., Bell, B., Berrisford, P., Hirahara, S., Horányi, A., Muñoz‐Sabater, J., et al. (2020). The ERA5 Global Reanalysis. Q.J.R. Meteorol. Soc. 146, 1999–2049. doi:10.1002/qj.3803
Infanti, J. M., and Kirtman, B. P. (2016). North American Rainfall and Temperature Prediction Response to the Diversity of ENSO. Clim. Dyn. 46, 3007–3023. doi:10.1007/s00382-015-2749-0
Jin, F.-F. (1996). Atmosphere Interaction, the Pacific Cold Tongue, and the El Niño-Southern Oscillation. Science 274, 76–78. doi:10.1126/science.274.5284.76
Krishnamurthy, L., and Krishnamurthy, V. (2014). Influence of PDO on South Asian Summer Monsoon and Monsoon-ENSO Relation. Clim. Dyn. 42, 2397–2410. doi:10.1007/s00382-013-1856-z
Lengaigne, M., Boulanger, J.-P., Menkes, C., Delecluse, P., and Slingo, J. (2004). “Westerly Wind Events in the Tropical Pacific and Their Influence on the Coupled Ocean-Atmosphere System: A Review,” in Earth’s Climate. Editors C. Wang, S. Xie, and J. Carton (American Geophysical Union), 147, 49–69. doi:10.1029/147GM03Geophysical Monograph Series
Li, C., and Mu, M. (2000). Relationship between East Asian winter Monsoon, Warm Pool Situation and ENSO Cycle. Chin.Sci.Bull. 45, 1448–1455. doi:10.1007/BF02898885
Luo, J.-J., Yuan, C., Sasaki, W., Behera, S. K., Masumoto, Y., Yamagata, T., et al. (2016). “Current Status of Intraseasonal-Seasonal-To-Interannual Prediction of the Indo-Pacific Climate,” in Indo-Pacific Climate Variability and Predictability. Editors T. Yamagata, and S. Behera (World Scientific Publisher World Scientific Series on Asia-Pacific Weather and Climate), 7, 63–107. doi:10.1142/9789814696623_0003
McPhaden, M. J., Zebiak, S. E., and Glantz, M. H. (2006a). ENSO as an Integrating Concept in Earth Science. Science 314, 1740–1745. doi:10.1126/science.1132588
McPhaden, M. J., Zhang, X., Hendon, H. H., and Wheeler, M. C. (2006b). Large Scale Dynamics and MJO Forcing of ENSO Variability. Geophys. Res. Lett. 33, L16702. doi:10.1029/2006GL026786
Miyazaki, S., Yasunari, T., and Adyasuren, T. (1999). Abrupt Seasonal Changes of Surface Climate Observed in Northern Mongolia by an Automatic Weather Station. J. Meteorol. Soc. Jpn. 77, 583–593. doi:10.2151/jmsj1965.77.2_583
Neelin, J. D., Battisti, D. S., Hirst, A. C., Jin, F.-F., Wakata, Y., Yamagata, T., et al. (1998). ENSO Theory. J. Geophys. Res. 103 (C7), 14261–14290. doi:10.1029/97JC03424
Overland, J. E., Adams, J. M., and Bond, N. A. (1999). Decadal Variability of the Aleutian Low and its Relation to High-Latitude Circulation*. J. Clim., 12. 15422–21548. doi:10.1175/1520–0442(1999)012<1542:DVOTAL>2.010.1175/1520-0442(1999)012<1542:dvotal>2.0.co;2
Paegle, J. N., and Mo, K. C. (2002). Linkages between Summer Rainfall Variability over South America and Sea Surface Temperature Anomalies. J. Clim. 15, 1389–1407. doi:10.1175/1520-0442(2002)015<1389:LBSRVO>2.0.CO;2>
Philander, S. G. H. (1983). El Niño Southern Oscillation Phenomena. Nature 302, 295–301. doi:10.1038/302295a0
Rasmusson, E. M., and Carpenter, T. H. (1982). Variations in Tropical Sea Surface Temperature and Surface Wind Fields Associated with the Southern Oscillation/El Niño. Mon. Wea. Rev. 110, 354–384. doi:10.1175/1520-0493(1982)110<0354:vitsst>2.0.co;2
Rayner, N. A., Parker, D. E., Horton, E., Folland, C. K., Alexander, L. V., Rowell, D., et al. (2003). Global Analyses of Sea Surface Temperature, Sea Ice, and Night marine Air Temperature since the Late Nineteenth century. J. Geophys. Res. 108, 4407. doi:10.1029/2002JD002670
Ren, H.-L., Zheng, F., Luo, J.-J., Wang, R., Liu, M., Zhang, W., et al. (2020). A Review of Research on Tropical Air-Sea Interaction, ENSO Dynamics, and ENSO Prediction in China. J. Meteorol. Res. 34, 43–62. doi:10.1007/s13351-020-9155-1
Terray, P. (2011). Southern Hemisphere Extra-tropical Forcing: a New Paradigm for El Niño-Southern Oscillation. Clim. Dyn. 36, 2171–2199. doi:10.1007/s00382-010-0825-z
Timmermann, A., An, S.-I., Kug, J.-S., Jin, F.-F., Cai, W., Capotondi, A., et al. (2018). El Niño-Southern Oscillation Complexity. Nature 559, 535–545. doi:10.1038/s41586-018-0252-6
Trenberth, K. E., Branstator, G. W., Karoly, D., Kumar, A., Lau, N.-C., and Ropelewski, C. (1998). Progress during TOGA in Understanding and Modeling Global Teleconnections Associated with Tropical Sea Surface Temperatures. J. Geophys. Res. 103, 14291–14324. doi:10.1029/97JC01444
Vimont, D. J., Battisti, D. S., and Hirst, A. C. (2001). Footprinting: A Seasonal Connection between the Tropics and Mid-latitudes. Geophys. Res. Lett. 28, 3923–3926. doi:10.1029/2001GL013435
Vimont, D. J., Wallace, J. M., and Battisti, D. S. (2003). The Seasonal Footprinting Mechanism in the Pacific: Implications for ENSO*. J. Clim. 16, 26682–32675. doi:10.1175/1520-0442(2003)016<2668:TSFMIT>2.0.CO10.1175/1520-0442(2003)016<2668:tsfmit>2.0.co;2
Wallace, J. M., and Gutzler, D. S. (1981). Teleconnections in the Geopotential Height Field during the Northern Hemisphere winter. Mon. Wea. Rev. 109 (4), 7842–8812. doi:10.1175/1520-0493(1981)109<0784:TITGHF>2.0.CO10.1175/1520-0493(1981)109<0784:titghf>2.0.co;2
Wang, L., Yu, J.-Y., and Paek, H. (2017). Enhanced Biennial Variability in the Pacific Due to Atlantic Capacitor Effect. Nat. Commun. 8, 14887. doi:10.1038/ncommsl488710.1038/ncomms14887
Wang, X., Wang, C., Zhou, W., Wang, D., and Song, J. (2011). Teleconnected Influence of North Atlantic Sea Surface Temperature on the El Niño Onset. Clim. Dyn. 37, 663–676. doi:10.1007/s00382-010-0833-z
Webster, P. J., and Yang, S. (1992). Monsoon and ENSO: Selectively Interactive Systems. Q.J R. Met. Soc. 118, 877–926. doi:10.1002/qj.49711850705
Wu, B., and Wang, J. (2002). Winter Arctic Oscillation, Siberian High and East Asian winter Monsoon. Geophys. Res. Lett. 29, 3–1. doi:10.1029/2002GL015373
Xie, S.-P., and Philander, S. G. H. (1994). A Coupled Ocean-Atmosphere Model of Relevance to the ITCZ in the Eastern Pacific. Tellus A 46, 340–350. doi:10.1034/j.1600-0870.1994.t01-1-00001.x
Yeo, S.-R., Kim, K.-Y., Yeh, S.-W., and Kim, W. (2012). Decadal Changes in the Relationship between the Tropical Pacific and the North Pacific. J. Geophys. Res. 117, a–n. doi:10.1029/2012JD017775
Yihui, D. (1990). Build-up, Air Mass Transformation and Propagation of Siberian High and its Relations to Cold Surge in East Asia. Meteorl. Atmos. Phys. 44, 281–292. doi:10.1007/BF01026822
Yu, J.-Y., and Kim, S. T. (2011). Relationships between Extratropical Sea Level Pressure Variations and the Central Pacific and Eastern Pacific Types of ENSO. J. Clim. 24, 708–720. doi:10.1175/2010JCLI3688.1
Zhang, R., Sumi, A., and Kimoto, M. (1996). Impact of El Niño on the East Asian Monsoon. J. Meteorol. Soc. Jpn. 74, 49–62. doi:10.215/jmsj1965.74.1_4910.2151/jmsj1965.74.1_49
Keywords: Siberian High, El Niño-Southern Oscillation, North Pacific, seasonal footprinting mechanism, wind-evaporation-SST feedback
Citation: Fu J, Liu M, Wang R, Wang Y and Zhao S (2022) Possible Impact of Boreal Winter Siberian High on ENSO Development in the Following Year. Front. Earth Sci. 10:885846. doi: 10.3389/feart.2022.885846
Received: 28 February 2022; Accepted: 28 March 2022;
Published: 14 April 2022.
Edited by:
Ruihuang Xie, Ocean University of China, ChinaCopyright © 2022 Fu, Liu, Wang, Wang and Zhao. This is an open-access article distributed under the terms of the Creative Commons Attribution License (CC BY). The use, distribution or reproduction in other forums is permitted, provided the original author(s) and the copyright owner(s) are credited and that the original publication in this journal is cited, in accordance with accepted academic practice. No use, distribution or reproduction is permitted which does not comply with these terms.
*Correspondence: Minghong Liu, bGl1bWluZ2hvbmcxOEBtYWlscy51Y2FzLmFjLmNu