- 1State Key Laboratory of Organic Geochemistry, Guangzhou Institute of Geochemistry, Chinese Academy of Sciences, Guangzhou, China
- 2CAS Center for Excellence in Deep Earth Science, Chinese Academy of Sciences, Guangzhou, China
- 3College of Earth Science and Planetary Sciences, University of Chinese Academy of Sciences, Beijing, China
The Upper Ordovician–Lower Silurian Wufeng–Longmaxi (O3w-S1l) shale is widely distributed in the Xishui area, north Guizhou, China. However, the potential and characteristics of shale gases in the Xishui area are not well evaluated, which has limited the exploration of shale gas in this area. In this study, we collected 14 fresh core samples of Wufeng–Longmaxi Formations from Well XK-3 and conducted gas desorption experiments to evaluate the potential of shale gas resources in the Xishui area. After analyzing the chemical and isotopic composition of desorption gases, the origin and genesis of the shale gas were systematically studied. Our results show that the volume of desorption gases varies from 2.14 m3/t to 6.01 m3/t, with a mean value of 4.12 m3/t, showing great potential for shale gas in this area. The positive correlation between total organic carbon (TOC) and desorption gas volume indicates that organic matter provides the main pore volume for shale gas preservation. The desorption gases are very dry, which are mainly composed of methane (99.64‰–99.74%) with a very low content of ethane, and no nitrogen, carbon dioxide, and hydrogen sulfide are detected. Based on geochemical diagrams, the desorption gases are identified as oil-associated thermogenic gases. The carbon isotopic values of methane and ethane display reversal distribution, due to the mixing of primary gases from kerogen cracking and the secondary gases from retained oil cracking and further possible cracking of heavy gaseous hydrocarbons (C3H8—C5H12) at higher thermal maturity (Ro > 2.00%). After comparing with Jiaoshiba, Changning, Weiyuan, and Dingshan areas, the carbon isotopic values of shale gases of Wufeng–Longmaxi shale also show different features at different areas, which are mainly controlled by the thermal maturity levels of the shale.
Introduction
Shale gas is mostly stored in nanoscale pores of shale with abundant organic matter in the forms of absorbed and free states (Ross and Bustin, 2008; Gao, 2015). The Upper Ordovician Wufeng Formation and the Lower Silurian Longmaxi Formation are considered the most favorable layers for shale gas exploration and development in China (Dai et al., 2020). In recent years, China had discovered several giant shale gas fields such as Jiaoshiba, Weiyuan, Changning, and Fushun (Dai et al., 2014; Dong et al., 2018). Up to 2019, the explored reserves of marine shale gas had reached 1.8 × 1012 m3, with an annual production of approximately 145 × 108 m3/a in China (Zou et al., 2020). In the past 5 y, north Guizhou has caused widespread attention for shale gas exploration (Yi and Gao, 2015; Zhang et al., 2015; Zhao et al., 2015; He et al., 2020). In 2016, commercial shale gas was discovered in Well AY-1 in Anchang anticline, Zheng’an area, and the mean production reached about 95 × 103 m3/day (Liu Y. et al., 2018). In 2017, shale gas with industrial values was also discovered in the Dingshan area (Wei et al., 2020). From the angle of the geological structure, Jiaoshiba, Changning, Dingshan, and Xishui anticlines are distributed along with the same geological structure; however, the potential of shale gas in the Xishui area is not well evaluated. One key question is the preservation of gases in the low burial thin shale layer like the Xishui area. Another question is whether there are obvious differences in geochemical and isotopic compositions in such a shallow thin shale layer in comparison with deep thick shale layers in other areas. Another observation is whether there exists carbon isotopic reversal for methane and ethane in this area, which is commonly observed in higher maturated marine shale gas in the world (Dai et al., 2014; Liu et al., 2021). In order to investigate the shale gas potential in the Xishui area, Wells XK-1, XK-2, and XK-3 were drilled by the Chinese Academy of Sciences in 2017 and 2018. Compared with Jiaoshiba and Changning areas, it is found that the Xishui area is not the depositional center of Wufeng–Longmaxi shale, and the thickness of high-quality shale is also thin (less than 30 m) due to the influence of Qianzhong paleo-uplift to the south (Yi and Gao, 2015; Zhang et al., 2016). Although the burial depth of Wufeng–Longmaxi shale is shallower than 700 m in this area, the volume of desorption gases is greater than 1 m3/t, which indicates the shale gas in these three wells have reached industrial standard (Shi et al., 2019; Sun et al., 2020). However, the geochemical and isotopic characteristics of shale gas in this area are also not well studied. In this study, 14 fresh core samples of Wufeng–Longmaxi shale from Well XK-3, Xishui area, north Guizhou, were collected with the drilling of well, and chemical and isotopic composites of the desorption gases were analyzed. The shale gas potential, gas origin, and the cause of carbon isotopic reversal were discussed after comparing with shale gas samples of Wufeng–Longmaxi shale from other areas in the Sichuan Basin.
Geological Background
The Xishui area is tectonically located in the transition zone between north Guizhou and the southeast Sichuan Basin (Figure 1), belonging to the upper part of the Yangtze block (Zhao A. et al., 2017). Because of the collision of the Yangtze block and Cathaysian block, a restricted anoxic depositional environment was formed during the period from the Late Ordovician to Early Silurian in north Guizhou (Wu et al., 2014). The Wufeng–Longmaxi shale was deposited due to two events of transgression in the periods of Late Ordovician and Early Silurian (He et al., 2020; Liu et al., 2021). The shale is distributed in the northern Guizhou area and the whole Sichuan Basin due to the influence of Qianzhong paleo-uplift (Yi and Gao, 2015; Zhang et al., 2016). The thickness of high-quality shale gradually becomes thicker from south to north, which is estimated at around 30 m in the Xishui area (Zhao A. et al., 2017; Zhao Z. et al., 2017). The regional stratigraphy of this area is shown in Figure 2. It can be seen that the exposed strata of the Xishui anticline include Cambrian, Ordovician, Lower Silurian, Permian, Triassic, Jurassic, and Cretaceous. From Figure 2, it is clear that there exist multiple unconformities in the stratigraphy, indicating the Xishui area mainly experienced four geological movements, such as Guangxi movement, Dongwu movement, Indosinian movement, and Himalayan movement. The Middle-Upper Silurian, Devonian, and Carboniferous are missing due to the erosion event related to the Guangxi movement (Yuan et al., 2013; Chen et al., 2014). Since the Late Cretaceous, the Xishui area suffered intense folding and denudation caused by the Himalayan movement. The NE–SW Xishui anticline was formed in this period, and many faults were developed in the core part of the anticline (Figure 1). In the Xishui area, the lithology of the Wufeng Formation is mainly composed of dark carbonaceous mudstone. The Longmaxi Formation can be divided into two members. The lower part is mainly composed of siliceous shale, carbonaceous shale, and calcareous shale with abundant graptolite and pyrite, while the upper part is composed of gray silty mudstone and argillaceous limestone (Figure 2). Well XK-3 is located in the core part of the Lintanchang dome-shaped anticline, and the complete well depth is 663 m (Figure 1). The thickness of high-quality shale with a TOC value of more than 2.00% is about 20 m, in which the Wufeng Formation is around 5 m and the Guanyinqiao Member is only around 34 cm.
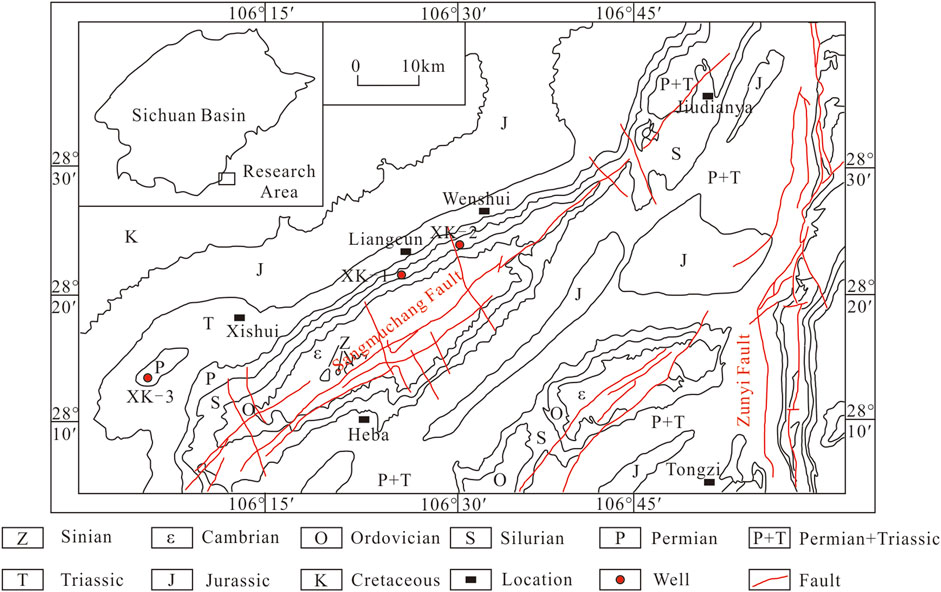
FIGURE 1. Geological map of the Xishui area, north Guizhou, with the location of Well XK-3 (figure modified from Shi et al., 2019).
Methods
The desorption gas was measured using specially designed equipment mainly composed of a sealed aluminum connected with a U-shaped glass tube. The fresh shale core samples were taken while drilling, put into the sealed aluminum immediately, and the saturated salt solution was filled into cans as quickly as possible. The desorption gas was released into a U-shaped glass tube, which is also filled with saturated salt solution, by a plastic conduit. Then, the desorption gas volume in a U-shaped glass tube can be measured using the labeled scale meter. The desorption gas volume (m3/t) at standard conditions can be calculated using the following equation:
where Vde is the desorption gas volume (m3/t) at standard conditions (P0 = 0.1 Mpa, 25°C), V is the desorption gas volume in U-shaped glass tube and aluminum can, P is the pressure of the desorption gas in U-shaped glass, P0 is the standard atmospheric pressure (P0 = 0.1 Mpa), and Mshale is the quantity of shale (t).
The chemical composition of desorption gas samples was analyzed by Agilent 6890N GC equipped with a flame ionization detector and a thermal conductivity detector. Carbon isotopic (δ13C) analysis of gases was analyzed using Isochrom GC-IRMS with the precision of ±0.5‰. Hydrogen isotopic (δ2H) analysis of gases was analyzed using IsoPrime GC-IRMS, with a precision of ±5‰.
Total organic carbon (TOC) was measured by using the LECO CS-800 analyzer, and the carbonates were removed by the hydrochloric acid (HCl) before measuring. The mineral compositions were analyzed using an Olympus X-ray Powder Diffraction (XRD) analyzer with CoKα radiation, and the powder was analyzed with a 2θ value from 5° to 55°.
The burial and thermal histories of Well XK-3 were built by PetroMod software. The first step of basin modeling is the basic input data, including stratigraphy (lithology, thickness, and age), tectonic events (unconformities, erosion, and age), and boundary conditions (paleo-water depth, heat flow, and sediment–water interface temperature) (Yao and Wang, 2016). Subsequently, the temperature and thermal maturation were calculated mainly based on burial depth and heat flow. Finally, the results of thermal maturity were required to be calibrated by the measured values. As shown in Figure 5B, the calculated thermal maturity from the Easy%Ro model (Sweeney and Burnham, 1990) was consistent with measured values.
Results
TOC and Mineral Composition of Shale
The results of total organic carbon and mineral compositions of 14 samples are listed in Table 1. The total organic carbon of studied 14 samples varies from 1.39% to 7.22%, with a mean value of 4.48%. All shale samples mainly consist of quartz, feldspar, clay minerals, calcite, dolomite, and a lower content of pyrite. All samples are clay-rich, and the content of clay minerals varies from 19.8% to 54.5%, with a mean value of 35.9%. The content of quartz varies from 21.1% to 64.4%, with a mean value of 38.7%. The positive correlation between TOC and the content of quartz can be observed (Figure 3A), which may indicate that the quartz is mainly of biogenic origin (Guo, 2014; Liang et al., 2016).
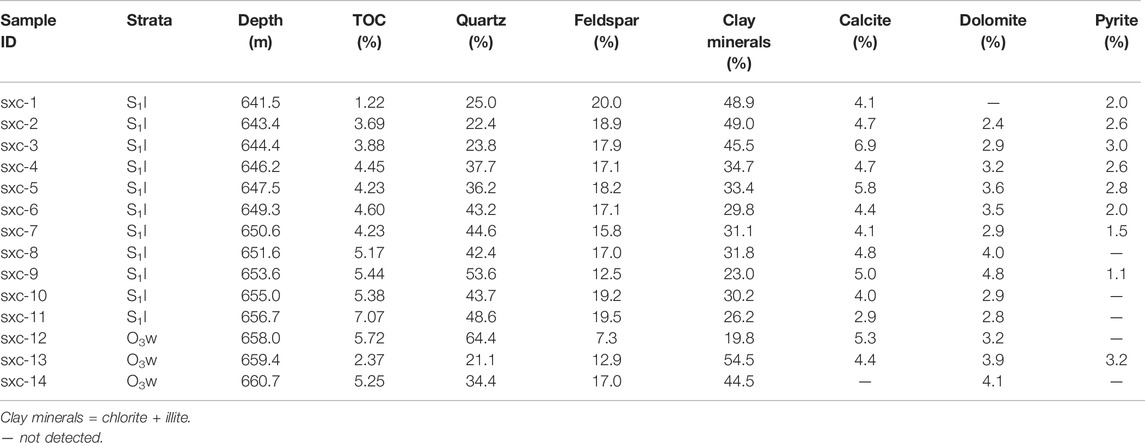
TABLE 1. TOC and mineral composition of 14 shale samples from Well XK-3, Xishui area, north Guizhou.
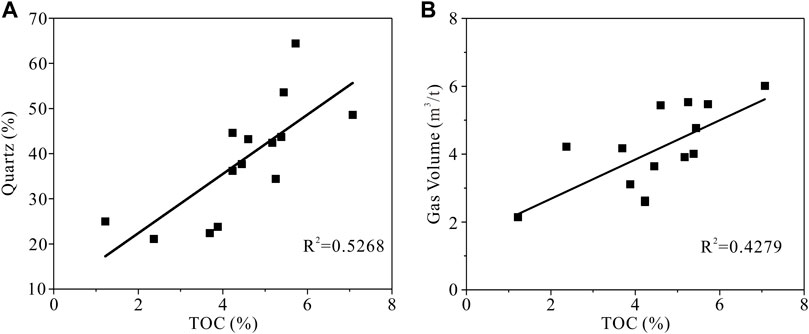
FIGURE 3. Total organic carbon vs. quartz (A) and total organic carbon vs. desorption gas volume (B) of 14 samples of Wufeng–Longmaxi shale from Well XK-3.
Gas Volume and Molecular Composition of Desorption Gases
The results of desorption experiments show that the gas volume ranges from 2.14 m3/t to 6.01 m3/t, with a mean value of 4.12 m3/t (Table 2). All samples show the gas volume exceeds 1 m3/t, which indicates it reaches the industrial standard. The positive correlation between TOC and gas volume (Figure 3B) indicates that the organic matter provides the main pore volume for the preservation of shale gas. The molecular composition of desorption gases is dominantly hydrocarbon gases, in which methane and ethane meanly account for 99.70% (99.64‰–99.74%) and 0.30% (0.26‰–0.36%), respectively, and no carbon dioxide, hydrogen sulfide, and nitrogen are detected. The lower wetness of shale gas from Wufeng–Longmaxi shale is very common in Sichuan Basin and its adjacent areas, such as Changning, Jiaoshiba, and Weiyuan areas (Dai et al., 2014; Feng et al., 2016).
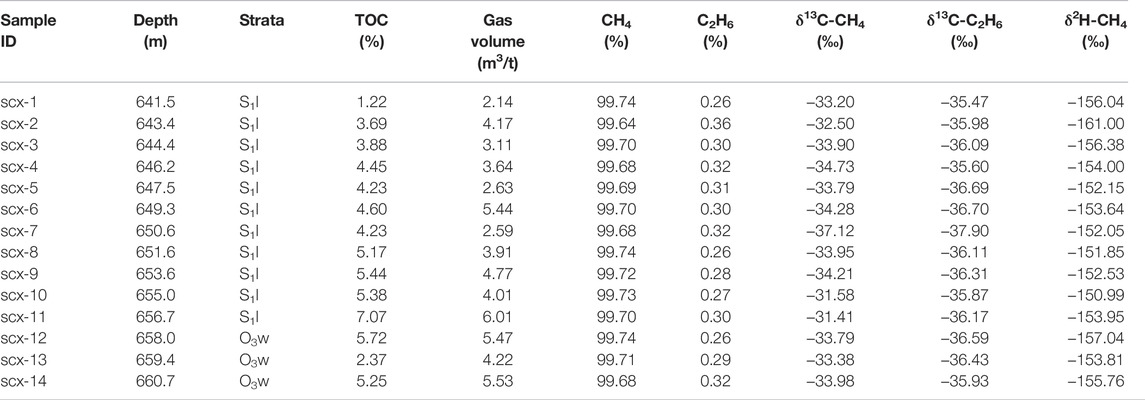
TABLE 2. Gas volume and geochemical characteristics of desorption gases from Well XK-3, Xishui area, north Guizhou.
Isotopic Composition of Desorption Gas
The carbon isotopic results (Table 2) show that the methane carbon isotopic values (δ13C-CH4) of desorption gases range from −37.41‰ to 31.21‰, with a mean value of −33.70‰. The ethane carbon isotopic values (δ13C-C2H6) range from −37.90‰ to −35.47‰, with a mean value of −36.27‰. The carbon isotopic values of desorption gases show an obvious reversal distribution (Figure 4A, δ13C-CH4 > δ13C-C2H6), which is similar to other areas, such as Changning, Weiyuan, Jiaoshiba, and Dingshan in the Sichuan Basin (Dai et al., 2014; Dai et al., 2016; Feng et al., 2016; Yang et al., 2017; Zhong et al., 2019). Figure 4B shows that shale gas samples of Well XK-3 fall in the post-rollover zone with the wetness lower than 1.2%, suggesting an extremely high maturity (Dai et al., 2014). In addition, the δ2H-CH4 (‰) values range from −157.07‰ to −159.99‰, with a mean value of −154.37‰, which is similar to Weiyuan (with a mean value of 141.50‰), Jiaoshiba (with a mean value of 148.95‰), and Changning (with a mean value of 148.00‰) areas.
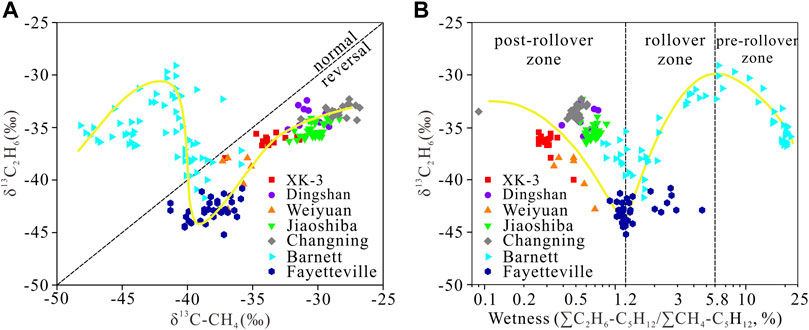
FIGURE 4. Plot of δ13C-CH4 vs. δ13C-C2H6 (A) and the wetness vs. δ13C-C2H6 (B) of shale gases from different areas. Data sources: the Dingshan gas field (Zhong et al., 2019); (2) the Weiyuan gas field (Dai et al., 2014); (3) the Jiaoshiba gas field (Dai et al., 2016; Yang et al., 2017); (4) the Changning gas field (Dai et al., 2016; Feng et al., 2016); (5) the Barnett shale in the Fort Worth Basin (Zumberge et al., 2012); and (6) the Fayetteville shale in the Arkoma Basin (Zumberge et al., 2012).
Burial and Thermal Histories of Well XK-3
For discussing the origin of desorption gas, we also built the geological model of Well XK-3 by using PetroMod software with the constrain of measured maturity data (Ro%) from publications (Zhao et al., 2015; Guo et al., 2016). The results of burial and thermal histories of Wufeng–Longmaxi shale from Well XK-3 are shown in Figure 5 (Zhao et al., 2015; Guo et al., 2016). It can be seen that Wufeng–Longmaxi shale of Well XK-3 experienced four stages of deep burial and uplift. The shale in Well XK-3 experienced a long-term erosion caused by Guangxi movement in the Late Silurian and the burial depth of Wufeng–Longmaxi shale is shallow (<1,500 m), when the temperature of Wufeng–Longmaxi shale is about 70°C and the maturity (Ro%) of the shale is about 0.40%, showing an immature stage. During the Permian period, there was short-term quick erosion due to the Dongwu movement (approximately 259 Ma). At that period, the burial depth of Wufeng–Longmaxi shale was also lower than 1,500 m, the temperature was approximately 90°C, and the maturity (Ro%) was about 0.50%, which was still in the immature stage. After the Dongwu movement, the Wufeng–Longmaxi shale of Well XK-3 entered into a long-term continuous deep burial stage and a short-term erosion related to the Indosinian movement occurred during the Late Triassic period. Meanwhile, the temperature and maturity (Ro%) of Wufeng–Longmaxi shale increased with the burial depth. Since the middle Triassic (approximately 235 Ma), the Wufeng–Longmaxi shale has been in the main oil stage (Ro = 0.70–1.00%); the burial depth was more than 2,700 m, and the temperature was about 120°C. Subsequently, the shale entered into the wet gas stage at the Late Jurassic (Ro > 1.30%, approximately 150 Ma) and dry gas stage at the early stage of Late Cretaceous (Ro > 2.00%, approximately 100 Ma), successively. The burial depth of Wufeng–Longmaxi reached the maximum depth (approximately 5,500 m) at the Late Cretaceous. At the same time, the temperature of the Wufeng–Longmaxi shale in Well XK-3 reached 190°C, and the maturity of the shale reached the current level (around 2.21%). Then, the shale of Well XK-3 uplifted and was eroded because of the Himalayan movement. Currently, the burial depth of Wufeng–Longmaxi shale in this area is about 650 m, and the temperature is about 37°C.
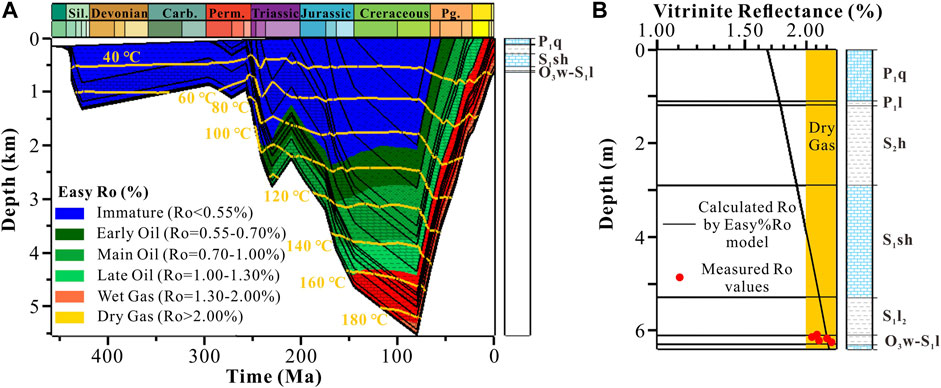
FIGURE 5. Burial and thermal histories of Wufeng–Longmaxi shale of Well XK-3 (A) and thermal model calibration (B).
Discussion
Origin of Desorption Gases
Whiticar’s diagrams (Whiticar, 1999) could be used to determine the origin of natural gases based on the chemical and isotopic compositions of natural gases. As Figure 6A shows, shale gases from Well XK-3, Dingshan, Changning, Weiyuan, and Jiaoshiba are of thermogenic origin, which is different from the gases from Barnett and Fayetteville shales. The δ13C-CH4 vs. CH4/(C2H6+C3H8) diagram (Figure 6B) shows that shale gases from Barnett and Fayetteville shales fall into the type II zone. On the contrary, shale gases from Well XK-3, Dingshan, Changning, and Jiaoshiba areas almost fall into the zone shifting from the typical type II kerogen gases, except for the Weiyuan area. This phenomenon may be caused by the extremely high content of methane and heavier carbon isotopic value at a relatively higher thermal maturity stage (Ro > 2.00%) (Liu et al., 2016; Yang et al., 2017). The δ13C-C2H6 values could be used to classify two types of natural gases. Dai (2011) proposed that natural gases with δ13C-C2H6 < −28.5‰ are oil-associated gases, while the gases with δ13C-C2H6 > −29‰ are coal-derived gases. Although there are some different viewpoints regarding this threshold (Milkov et al., 2020), a range of −27.0‰ to −29.0‰ for δ13C-C2H6 value is acceptable for distinguishing oil-derived gases. As shown in Table 2, the δ13C-C2H6 values of the gas samples are between −37.90‰ and −35.47‰, suggesting that the desorption gases of Wufeng–Longmaxi shale from Well XK-3 are oil-associated gases, which is similar to other areas in the Sichuan Basin (Figure 4).
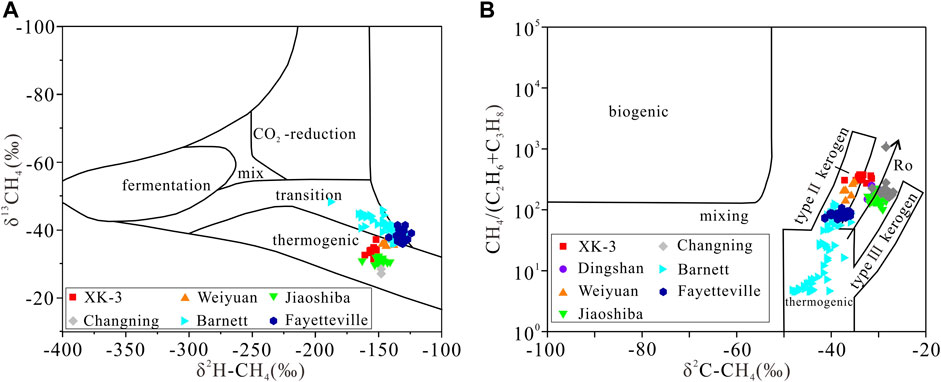
FIGURE 6. Plot of δ2H-CH4 vs. δ13C-CH4 (A) and δ13C-CH4 vs. CH4/(C2H6+C3H8) (B) of shale gases from different areas (modified from Whiticar 1999). Data sources: (1) the Dingshan gas field (Zhong et al., 2019); (2) the Weiyuan gas field (Dai et al., 2014); (3) the Jiaoshiba gas field (Dai et al., 2016; Yang et al., 2017); (4) the Changning gas field (Dai et al., 2016; Feng et al., 2016); (5) the Barnett shale in the Fort Worth Basin (Zumberge et al., 2012); and (6) the Fayetteville shale in the Arkoma Basin (Zumberge et al., 2012).
Causes of the Carbon Isotopic Reversal in Well XK-3
The carbon isotopic reversal is commonly observed in Wufeng–Longmaxi shale gas in the Sichuan Basin (Figure 4A). Previous studies interpreted and summarized the following causes of this phenomenon: 1) mixing gases with different thermal maturity (Hao and Zou, 2013; Xia et al., 2013; Liu Q. et al., 2018; Zhao et al., 2019); 2) redox reactions with transition metals and water (Burruss and Laughrey, 2010; Feng et al., 2016); 3) depressurization during uplifting (Milkov et al., 2020); and 4) thermochemical sulfate reduction (Hao et al., 2008; Liu et al., 2019).
In order to discuss the cause of carbon isotopic reversal in this area, we recovered the generation history of hydrocarbons of Wufeng–Longmaxi shale of Well XK-3 with consideration of oil secondary cracking based on Pepper’s hydrocarbon generation kinetics (Pepper and Corvi, 1995), and the results are shown in Figure 7. During the relatively lower maturity stage (Ro < 1.00%), a considerable amount of gases was mainly from kerogen cracking, and no or little retained oil cracking before oil cracking (Figure 7). δ13C increased with the carbon number, and the carbon isotopic distribution was normal (Figures 4A, 7; δ13C-CH4 < δ13C-C2H6); thus, the carbon isotopic reversal was not observed due to the same origin of gases. With the increase in thermal maturity and burial depth, the open system was gradually converted into a closed system. When thermal maturity (Ro%) reached over 1.00% (165 Ma, T = 160°C), the retained oil started cracking (Figures 5A, 7). The retained oil was mainly cracked and converted into wet gases and generated lighter δ13C-C2H6 values (Liu Q. et al., 2018). As a result, δ13C-C2H6 becomes lighter due to the mixing of gases from kerogen cracking and retained oil cracking (Dai et al., 2017; Liu Q. et al., 2018). Although δ13C-CH4 also becomes lighter, the extent of change for δ13C-CH4 is not as obvious as δ13C-C2H6 (Figure 4A). The main reason causing isotopic reversal is that the retained oil mainly cracked into wet gases with a little methane, and methane was mainly from kerogen cracking (Xia et al., 2013). During this stage, δ13C-C2H6 becomes more negative with the increase in thermal maturity, and reversal carbon isotopic distribution (δ13C-CH4 > δ13C-C2H6) occurs with increasing contribution of wet gases from retained oil cracking (Figure 4). Therefore, we proposed that mixing gases from kerogen and retained oil cracking might be the main reason for the carbon isotopic reversal in this area.
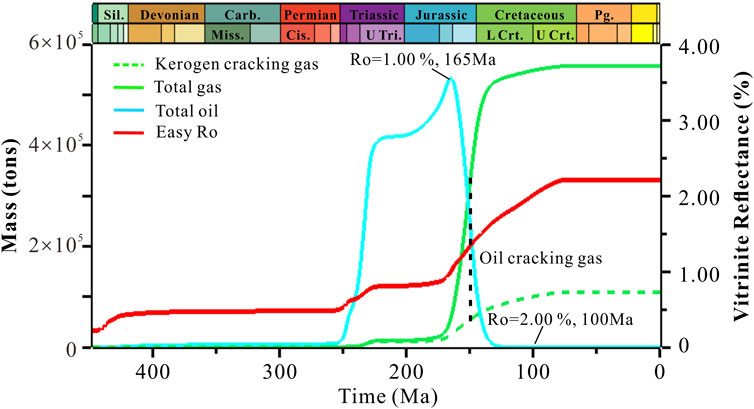
FIGURE 7. Generation history of hydrocarbons of Wufeng–Longmaxi shale of Well XK-3, Xishui area, north Guizhou.
Differences and Causes of Carbon Isotopic Values in the Sichuan Basin
In this study, we also collected carbon isotopic data of Wufeng–Longmaxi shale gas from Changning, Weiyuan, Dingshan, and Jiaoshiba areas. Although the values of δ13C-CH4 and δ13C-C2H6 for shale gas are distributed in the reversal zone, we still found that there existed differences in the carbon isotopic values of shale gases in different areas, Sichuan Basin (Figure 8). The mean values of δ13C-CH4 are −36.21‰ in Weiyuan (Dai et al., 2014), −33.70‰ in Well XK-3, −30.73‰ in Dingshan (Zhong et al., 2019), −30.59‰ in Jiaoshiba (Dai et al., 2016; Yang et al., 2017), and −28.34‰ in Changning (Feng et al., 2016), respectively. The mean values of δ13C-C2H6 are −39.31‰ in Weiyuan (Dai et al., 2014), −36.27‰ in Well XK-3, −34.09‰ in Dingshan (Zhong et al., 2019), −35.09‰ in Jiaoshiba (Dai et al., 2016; Yang et al., 2017), and −33.44‰ in Changning (Feng et al., 2016), respectively. As shown in Figure 8, the carbon isotopic values of Well XK-3 and Weiyuan are lighter than those of the other areas, and gases from Changning show the heaviest carbon isotopic values for both methane and ethane. We regard that this difference may be controlled by thermal maturity. The current maturities of Wufeng–Longmaxi shale from Weiyuan, Xishui, Dingshan, Jiaoshiba, and Changning are 2.00%–2.20% (Feng et al., 2016), 1.80%–2.20% (Zhao A. et al., 2017), 2.14%–2.24% (Wei et al., 2017), 2.50%–2.80% (Feng et al., 2018), and 2.50%–3.00% (Chen et al., 2017), respectively. It is obvious that there exists a positive correlation between δ13C-CH4 or δ13C-C2H6 and thermal maturity (Figure 8).
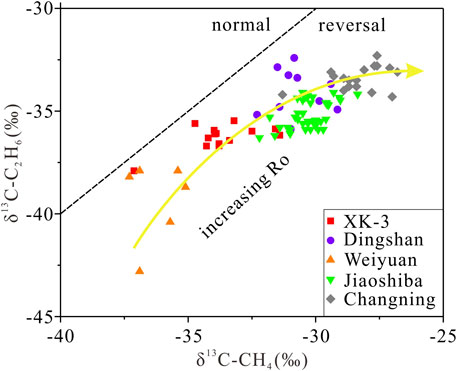
FIGURE 8. Plot of δ13C-CH4 vs. δ13C-C2H6 of shale gases of Longmaxi shale in different areas, Sichuan Basin. Data sources: (1) the Dingshan gas field (Zhong et al., 2019); (2) the Weiyuan gas field (Dai et al., 2014); (3) the Jiaoshiba gas field (Dai et al., 2016; Yang et al., 2017); and (4) the Changning gas field (Dai et al., 2016; Feng et al., 2016).
The generation history of Wufeng–Longmaxi shale of Well XK-3 shows the retained oil stopped cracking into gases when thermal maturity reached 2.00% (Figures 5A, 7, approximately 100 Ma, T = 185°C). The current thermal maturity of Wufeng–Longmaxi shale is over 2.00%, which indicates that Wufeng–Longmaxi shale had experienced the stage of retained oil cracking into wet gases. It is worth noting that gaseous hydrocarbons are still generated and increased when thermal maturity is greater than 2.00% (Figure 7). This increasing part of generated gases might be from kerogen cracking because the cracking of retained oil is stopped. Meanwhile, heavier gaseous hydrocarbons are further cracked and converted to lighter gaseous hydrocarbons, and this process is also controlled by thermal maturity (Hao and Zou, 2013; Liu Q. et al., 2018). The current maturity (Ro%) values of Wufeng–Longmaxi shale in Changning and Jiaoshiba areas are higher than those in Xishui, Weiyuan, and Dingshan areas. It may indicate that Wufeng–Longmaxi shale in Changning areas experienced a longer period of heavier gases cracking into lighter gases. Thus, the carbon isotopic values of shale gases in Changning and Jiaoshiba are heavier than those of other areas.
Conclusion
The gas desorption experiments on 14 fresh core samples of Wufeng–Longmaxi Formations collected from Well XK-3 show the volume of desorption gases varies from 2.14 m3/t to 6.01 m3/t, with a mean value of 4.12 m3/t, suggesting great potential of shale gas resources for Wufeng–Longmaxi shale in the Xishui area, north Guizhou. Our results indicate that the shallow and thin shale still enjoys the high capability of gas resources. From the angle of the regional structure, the belt along the Jiaoshiba, Changning, Dingshan, and Xishui anticlines is the most prospective exploration target for further exploration of shale gas. The positive relationship between desorption gas volume and TOC indicates that organic matter can provide more pore volume for shale gas storage. The desorption gases are typically dry gases, which are mainly composed of methane (99.64%–99.74%), with a little ethane (0.26%–0.36%) and no detected nitrogen, carbon dioxide, and hydrogen sulfide. The desorption gases are thermogenic origin and oil-associated gases. The carbon isotopic reversal is mainly caused by the mixing of gases from kerogen primary cracking and retained oil secondary cracking. The further cracking of heavy gaseous hydrocarbons during higher thermal maturity (Ro > 2.00%) may lead to the carbon isotopic differences of shale gases from Wufeng–Longmaxi shale in different areas, Sichuan Basin. The difference of geochemical and isotopic compositions in such a shallow thin shale layer is the maturation of the gases in comparison with deep thick shale layers in other areas, which should cause the attention for further studies and explorations.
Data Availability Statement
The raw data supporting the conclusion of this article will be made available by the authors, without undue reservation.
Author Contributions
YW and HG presented the idea and conceived this study. SS, YS, and YW designed the field works and experiments. SS and YS performed the experiments. SS, YW and HG interpreted the data and wrote the manuscript.
Funding
This study was supported by the Strategic Priority Research Program of the Chinese Academy of Sciences (XDA14010103, XDB10010300), the National Natural Science Foundation of China (No. 41702151), and the National Science and Technology Major Project of China (No. 2017ZX05008-002-030).
Conflict of Interest
The authors declare that the research was conducted in the absence of any commercial or financial relationships that could be construed as a potential conflict of interest.
Publisher’s Note
All claims expressed in this article are solely those of the authors and do not necessarily represent those of their affiliated organizations, or those of the publisher, the editors, and the reviewers. Any product that may be evaluated in this article, or claim that may be made by its manufacturer, is not guaranteed or endorsed by the publisher.
Acknowledgments
The authors thank Prof. Xiao Li and Dr. Guanfang Li, Institute of Geology and Geophysics, Beijing, China, to assist with core sample collection. We also thank the editor and reviewers for their critical and constructive comments, which have improved this manuscript significantly. This is contribution No.IS-3156 from GIGCAS.
References
Burruss, R. C., and Laughrey, C. D. (2010). Carbon and Hydrogen Isotopic Reversals in Deep basin Gas: Evidence for Limits to the Stability of Hydrocarbons. Org. Geochem. 41, 1285–1296. doi:10.1016/j.orggeochem.2010.09.008
Chen, X., Fan, J., Chen, Q., Tang, L., and Hou, X. (2014). Toward a Stepwise Kwangsian Orogeny. Sci. China Earth Sci. 57 (3), 379–387. doi:10.1007/s11430-013-4815-y
Dai, J. (2011). Significance of the Study on Carbon Isotopes of Alkane Gases. Nat. Gas Industry. 31 (12), 1–6. doi:10.3787/j.issn.1000-0976.2011.12.001
Dai, J., Dong, D., Ni, Y., Hong, F., Zhang, S., Zhang, Y., et al. (2020). Some Essential Geological and Geochemical Issues about Shale Gas Research in China. Nat. Gas Geosci. 31 (6), 745–760. doi:10.11764/j.issn.1672-1926.2020.05.016
Dai, J., Ni, Y., Gong, D., Feng, Z., Liu, D., Peng, W., et al. (2017). Geochemical Characteristics of Gases from the Largest Tight Sand Gas Field (Sulige) and Shale Gas Field (Fuling) in China. Mar. Pet. Geology. 79, 426–438. doi:10.1016/j.marpetgeo.2016.10.021
Dai, J., Zou, C., Dong, D., Ni, Y., Wu, W., Gong, D., et al. (2016). Geochemical Characteristics of marine and Terrestrial Shale Gas in China. Mar. Pet. Geology. 76, 444–463. doi:10.1016/j.marpetgeo.2016.04.027
Dai, J., Zou, C., Liao, S., Dong, D., Ni, Y., Huang, J., et al. (2014). Geochemistry of the Extremely High thermal Maturity Longmaxi Shale Gas, Southern Sichuan Basin. Org. Geochem. 74, 3–12. doi:10.1016/j.orggeochem.2014.01.018
Dong, D., Shi, Z., Guan, Q., Jiang, S., Zhang, M., Zhang, C., et al. (2018). Processes, Challenges and Prospects of Shale Gas Exploration in Wufeng-Longmaxi Reservoirs in the Sichuan Basin. Nat. Gas Industry 38 (4), 8. doi:10.3787/j.issn.1000-0976.2018.04.008
Feng, W., Wang, F., Guan, J., Zhou, J., Wei, F., Dong, W., et al. (2018). Geologic Structure Controls on Initial Productions of Lower Silurian Longmaxi Shale in south China. Mar. Pet. Geology. 91, 163–178. doi:10.1016/j.marpetgeo.2018.01.001
Feng, Z., Liu, D., Huang, S., Wu, W., Dong, D., Peng, W., et al. (2016). Carbon Isotopic Composition of Shale Gas in the Silurian Longmaxi Formation of the Changning Area, Sichuan Basin. Pet. Exploration Develop. 43 (5), 1–9. doi:10.11698/PED.2016.05.0010.1016/s1876-3804(16)30092-1
Gao, B. (2015). Geochemical Characteristics of Shale Gas from Lower Silurian Longmaxi Formation in the Sichuan Basin and its Geological Significance. Nat. Gas Geosci. 26 (6), 1173–1182. doi:10.11764/j.issn.1672-1926.2015.06.1173
Guo, S., Guo, J., Liu, C., Zhang, L., Guo, X., and Xiao, P. (2016). Shale Gas Accumulation Potential of Lower Silurian Longmaxi Formation in Northern Guizhou. J. Cent. South Univ. (Science Technology) 47 (6), 1973–1980. doi:10.11817/j.issn.1672-7207.2016.06.021
Guo, X. (2014). Rules of Two-Factor Enrichment for Marine Shale Gas in Southern China-Understanding from the Longmaxi Formation. Acta Geologica Sinica 88 (7), 1209–1218. doi:10.1111/1755-6724.12347
Hao, F., Guo, T., Zhu, Y., Cai, X., Zou, H., and Li, P. (2008). Evidence for Multiple Stages of Oil Cracking and Thermochemical Sulfate Reduction in the Puguang Gas Field, Sichuan Basin, China. Bulletin 92 (5), 611–637. doi:10.1306/01210807090
Hao, F., and Zou, H. (2013). Cause of Shale Gas Geochemical Anomalies and Mechanisms for Gas Enrichment and Depletion in High-Maturity Shales. Mar. Pet. Geology. 44, 1–12. doi:10.1016/j.marpetgeo.2013.03.005
He, Y., Xiang, K., An, Y., Yi, C., Yang, Z., and Yu, N. (2020). Geological Characteristics and Favorable Areas Prediction of Shale Gas in Wufeng-Longmaxi Formation in Zheng'an Area of North Guizhou. Geol. Survery China 7 (3), 21–29. doi:10.19388/j.zgdzdc.2020.0303
Liang, C., Jiang, Z., Cao, Y., Wu, M., Guo, L., and Zhang, C. (2016). Deep-water Depositional Mechanisms and Significance for Unconventional Hydrocarbon Exploration: A Case Study from the Lower Silurian Longmaxi Shale in the southeastern Sichuan Basin. Bulletin 100 (05), 773–794. doi:10.1306/02031615002
Liu, Q., Jin, Z., Wang, X., Yi, J., Meng, Q., Wu, X., et al. (2018a). Distinguishing Kerogen and Oil Cracked Shale Gas Using H, C-Isotopic Fractionation of Alkane Gases. Mar. Pet. Geology. 91, 350–362. doi:10.1016/j.marpetgeo.2018.01.006
Liu, Q., Li, P., Jin, Z., Liang, X., Zhu, D., Wu, X., et al. (2021). Preservation of Organic Matter in Shale Linked to Bacterial Sulfate Reduction (BSR) and Volcanic Activity under marine and Lacustrine Depositional Environments. Mar. Pet. Geology. 127, 104950. doi:10.1016/j.marpetgeo.2021.104950
Liu, Q., Wu, X., Wang, X., Jin, Z., Zhu, D., Meng, Q., et al. (2019). Carbon and Hydrogen Isotopes of Methane, Ethane, and Propane: A Review of Genetic Identification of Natural Gas. Earth-Science Rev. 190, 247–272. doi:10.1016/j.earscirev.2018.11.017
Liu, Y., Zhang, J., Ren, J., Liu, Z., Huang, H., and Tang, X. (2016). Stable Isotope Geochemistry of the Nitrogen-Rich Gas from Lower Cambrian Shale in the Yangtze Gorges Area, South China. Mar. Pet. Geology. 77, 693–702. doi:10.1016/j.marpetgeo.2016.07.020
Liu, Y., Zhang, J., Zhang, P., Liu, Z., Zhao, P., Huang, H., et al. (2018b). Origin and Enrichment Factors of Natural Gas from the Lower Silurian Songkan Formation in Northern Guizhou Province, south China. Int. J. Coal Geology. 187, 20–29. doi:10.1016/j.coal.2018.01.004
Milkov, A. V., Faiz, M., and Etiope, G. (2020). Geochemistry of Shale Gases from Around the World: Composition, Origins, Isotope Reversals and Rollovers, and Implications for the Exploration of Shale Plays. Org. Geochem. 143, 103997. doi:10.1016/j.orggeochem.2020.103997
Pepper, A. S., and Corvi, P. J. (1995). Simple Kinetic Models of Petroleum Formation. Part I: Oil and Gas Generation from Kerogen. Mar. Pet. Geology. 12 (3), 291–319. doi:10.1016/0264-8172(95)98381-e
Ross, D. J. K., and Bustin, R. M. (2008). Characterizing the Shale Gas Resource Potential of Devonian-Mississippian Strata in the Western Canada Sedimentary basin: Application of an Integrated Formation Evaluation. Bulletin 92 (1), 87–125. doi:10.1306/09040707048
Shangbin, C., Yanming, Z., Si, C., Yufu, H., Changqing, F., and Junhua, F. (2017). Hydrocarbon Generation and Shale Gas Accumulation in the Longmaxi Formation, Southern Sichuan Basin, China. Mar. Pet. Geology. 86, 248–258. doi:10.1016/j.marpetgeo.2017.05.017
Shi, S., Sun, Y., Guo, H., Deng, R., Cheng, C., and Wang, Y. (2019). Geochemical and thermal Evolution of Wufeng-Longmaxi Shale and its prospect in the Xishui Area, North Guizhou. Geochimica 48 (6), 567–579. doi:10.19700/j.0379-1726.2019.06.005
Sun, J., Xiao, X., Wei, Q., Cheng, P., Tian, H., and Wu, Y. (2020). Gas in Place and its Controlling Factors of the Shallow Longmaxi Shale in the Xishui Area, Guizhou, China. J. Nat. Gas Sci. Eng. 77, 1–11. doi:10.1016/j.jngse.2020.103272
Sweeney, J. J., and Burnham, A. K. (1990). Evaluation of a Simple Model of Vitrinite Reflectance Based on Chemical Kinetics. AAPG Bull. 74 (10), 1559–1570. doi:10.1306/0c9b251f-1710-11d7-8645000102c1865d
Wei, X., Liu, Z., Wang, Q., Wei, F., and Yuan, T. (2020). Analysis and Thinking of the Difference of Wufeng-Longmaxi Shale Gas Enrichment Conditions between Dingshan and Jiaoshiba Areas in southeastern Sichuan Basin. Nat. Gas Geosci. 31 (8), 1041–1050. doi:10.11764/j.issn.1672-1926.2020.02.011
Wei, X., Zhao, Z., Wang, Q., Liu, Z., Zhou, M., and Zhang, H. (2017). Comprehensive Evaluation on Geological Conditions of the Shale Gas in Upper Ordovician Wufeng Formation-Lower Silurian Longmaxi Formation in Dingshan Area, Qijiang, Southeastern Sichuan. Geol. Rev. 63 (1), 153–164. doi:10.16509/j.georeview.2017.01.014
Whiticar, M. J. (1999). Carbon and Hydrogen Isotope Systematics of Bacterial Formation and Oxidation of Methane. Chem. Geology. 161, 219–314. doi:10.1016/s0009-2541(99)00092-3
Wu, Y., Fan, T., Zhang, J., Jiang, S., Li, Y., Zhang, J., et al. (2014). Characterization of the Upper Ordovician and Lower Silurian Marine Shale in Northwestern Guizhou Province of the Upper Yangtze Block, South China: Implication for Shale Gas Potential. Energy Fuels 28 (6), 3679–3687. doi:10.1021/ef5004254
Xia, X., Chen, J., Braun, R., and Tang, Y. (2013). Isotopic Reversals with Respect to Maturity Trends Due to Mixing of Primary and Secondary Products in Source Rocks. Chem. Geology. 339, 205–212. doi:10.1016/j.chemgeo.2012.07.025
Yang, R., He, S., Hu, Q., Hu, D., and Yi, J. (2017). Geochemical Characteristics and Origin of Natural Gas from Wufeng-Longmaxi Shales of the Fuling Gas Field, Sichuan Basin (China). Int. J. Coal Geology. 171, 1–11. doi:10.1016/j.coal.2016.12.003
Yao, X., and Wang, Y. (2016). Assessing Shale Gas Resources of Wufeng-Longmaxi Shale (O3w-S1l) in Jiaoshiba Area, SE Sichuan (China) Using Petromod I: Burial and thermal Histories. Pet. Sci. Technol. 34 (11-12), 1000–1007. doi:10.1080/10916466.2016.1176041
Yi, T., and Gao, D. (2015). Characteristics and Distribution Pattern of Shale Gas Reservoir in Longmaxi Formation in Guizhou Province. Coal Geology. Exploration 43 (3), 22–32. doi:10.3969/j.issn.1001-1986.2015.03.005
Yuan, Y., Sun, D., li, S., and Lin, J. (2013). Caledonian Erosion Thickness Reconstruction in the Sichuan Basin. Chin. J. Geology. 48 (3), 581–591.
Zhang, P., Zhang, J., Huang, Y., Tang, X., Wang, Z., and Peng, J. (2015). Characteristics and Gas Content Evaluation of Wufeng-Longmaxi Formation Shale in Well Xiye-1. Resour. Industries 17 (4), 48–55. doi:10.13776/j.cnki.resourcesindustries.20150514.012
Zhang, P., Zhang, J., Liu, H., and Huang, Y. (2016). Accumulation Conditions of Shale Gas from Lower Silurian Longmaxi Formation in Guizhou. J. Cent. South Univ. (Science Technology) 47 (9), 3085–3092. doi:10.11817/j.issn.1672-7207.2016.09.024
Zhao, A., Yu, Q., Lei, Z., Tan, J., Zhou, W., Zhao, G., et al. (2017a). Geological and Microstructural Characterization of the Wufeng-Longmaxi Shale in the Basin-Orogen Transitional Belt of North Guizhou Province, China. j nanosci nanotechnol 17 (9), 6026–6038. doi:10.1166/jnn.2017.14522
Zhao, L., He, Y., Yang, P., Chen, H., and An, Y. (2015). Characteristics of Lower Palaeozoic Hydrocarbon Source Strata and a Primary Study of the Shale Gas Accumulation in Northern Guizhou Province. Geology. China 42 (6), 1931–1943.
Zhao, W., Zhang, S., He, K., Zeng, H., Hu, G., Zhang, B., et al. (2019). Origin of Conventional and Shale Gas in Sinian-Lower Paleozoic Strata in the Sichuan Basin: Relayed Gas Generation from Liquid Hydrocarbon Cracking. Bulletin 103 (6), 1265–1296. doi:10.1306/11151817334
Zhao, Z., Li, R., Feng, W., Yu, Q., Yang, H., and Zhu, L. (2017b). Enrichment Conditions and Favorable Zone Prediction of Wufeng–Longmaxi Shale Gas Reservoirs in the Northern Yunnan–Guizhou Provinces, China. Nat. Gas Industry 37 (12), 26–34. doi:10.3787/j.issn.1000-0976.2017.12.004
Zhong, C., Qin, Q., Fan, C., and Hu, D. (2019). Geochemical Characteristics of Shale Gas and its Response to thermal Maturity (Ro) in the Longmaxi Formation, Dingshan Area, Southeast Sichuan. Pet. Sci. Technol. 37 (11), 1270–1278. doi:10.1080/10916466.2018.1558241
Zou, C., Pan, S., Jing, Z., Gao, J., Yang, Z., Wu, S., et al. (2020). Shale Oil and Gas Revolution and its Impact. Acta Petrolei Sinica 41 (1), 1–12. doi:10.7623/syxb202001001
Keywords: Wufeng–Longmaxi (O3w-S1l) shale, desorption gases, thermogenic origin, shale gas, isotopic reversal, thermal maturity
Citation: Shi S, Wang Y, Sun Y and Guo H (2022) The Volume and Geochemical Characteristics of Desorption Gases From Wufeng–Longmaxi (O3w-S1l) Shale in the Xishui Area, North Guizhou, China. Front. Earth Sci. 10:879959. doi: 10.3389/feart.2022.879959
Received: 20 February 2022; Accepted: 07 March 2022;
Published: 13 April 2022.
Edited by:
Yunyan Ni, Research Institute of Petroleum Exploration and Development (RIPED), ChinaReviewed by:
Quanyou Liu, SINOPEC Petroleum Exploration and Production Research Institute, ChinaGuodong Zheng, Northwest Institute of Eco-Environment and Resources (CAS), China
Copyright © 2022 Shi, Wang, Sun and Guo. This is an open-access article distributed under the terms of the Creative Commons Attribution License (CC BY). The use, distribution or reproduction in other forums is permitted, provided the original author(s) and the copyright owner(s) are credited and that the original publication in this journal is cited, in accordance with accepted academic practice. No use, distribution or reproduction is permitted which does not comply with these terms.
*Correspondence: Yunpeng Wang, d2FuZ3lwQGdpZy5hYy5jbg==