- Department of Geology, Lakehead University, Thunder Bay, ON, Canada
The operation of depositional processes in fluvial systems prior to the development of land-based, macrophyte vegetation has undergone considerable research. The differences in sedimentation in the channels and floodplains compared to post-vegetation systems should result in differences also existing in the deposits developed where the rivers debouch their loads into standing bodies of water. These deltaic deposits have been studied relatively less. The 1.4 Ga Kama Hill and Outan Island Formations of the Sibley Group, northwestern Ontario, provide a site to further investigate these potential differences. Four totally cored drill holes intersect this 340 m thick, prograding, deltaic to fluvial succession. It is divisible into eight lithofacies associations representing environments ranging from prodelta to the delta-top floodplain-channel assemblage. The sub-aqueous portion of the succession is composed of two thick progradational delta lobes probably caused by: deposition induced regression followed by a condensed sequence due to channel avulsion with distal repositioning, and finally channel avulsion with proximal repositioning driving another depositional induced regression. Large-scale sequence stratigraphic nomenclature does not properly describe this succession. At a smaller scale, members of progradational parasequence sets decrease in thickness from the prodelta to upper distributary-mouth bars. The deltaic deposits are compared to the modern, large Mississippi deltaic system. Sub-aqueous sub-environments are similar to this, except: 1) the lack of infauna bioturbation leads to better preservation of Sibley Delta sedimentary structures; 2) scarce organic sediment does not allow reducing diagenetic reactions, thus preserving the highly oxidized nature of the fluvial sediments; 3) the Sibley distributary-mouth bars have more medium- and coarse-grained sand than the Mississippi bars; 4) the Sibley distributary-mouth bars have abundant thick, massive, non-graded, normal graded and reverse graded sandstones, likely the result of high-discharge slurry-flows from the fluvial channels; 5) the lack of macrophytes (coastal marshes) complicates interpreting the transition from bar tops to sub-aerial environments; 6) the abandonment phase of bay-fill progradational parasequences is further condensed due to the lack of organic deposits. However, the considerable similarities between the Sibley Delta and the Mississippi Delta denote that not all pre-vegetation deltas conform to general braid and fan delta models.
Introduction
In 1997 a mineral exploration company used the winter ice of Lake Superior, Ontario, Canada, to drill ∼900 m deep cored holes through the sedimentary strata of the 1.4 Ga Sibley Group (Figure 1). Sedimentological study of these cores revealed a previously unknown ∼350 m thick deltaic assemblage (Rogala, 2003; Rogala et al., 2005). Floodplain sediments dominate the delta top with only rare channel sandstones (Fralick and Zaniewski, 2012; Ielpi et al., 2018). This contrasts with the majority of fluvial systems developed prior to the evolution of land macrophytes, which are channel dominated (Long, 2019), leading to the possibility that the subaqueous portion of the delta may supply additional information on pre-vegetation delta types.
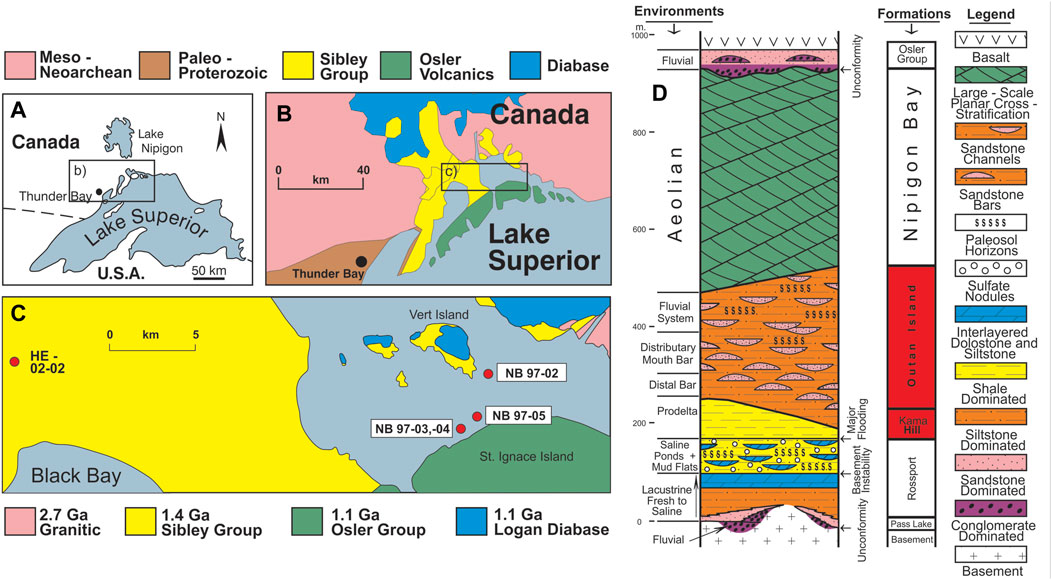
FIGURE 1. (A) Location map of the study area. Box delineates region covered in Figure 1B. (B) Outcrop area of the Sibley Group. Box delineates region covered in Figure 1C. (C) Locations of the four cored drill-holes used in this study. (D) Stratigraphy and depositional environments represented by the Sibley Group. The Kama Hill and Outan Island Formations studied here are in red.
Numerous classification schemes have been developed to differentiate various types of deltas (Gallloway, 1975; McPherson et al., 1987; Orton, 1988; Nemec, 1990; Postma, 1990; Wescott and Ethridge, 1990; Reading and Orton, 1991; Boyd et al., 1992; Dalrymple et al., 1992; Orton and Reading, 1993; Ethridge and Wescott, 1994). These classifications can be equally applied to deltas formed in non-vegetated settings. The lack of macrophytes will affect processes in the channels and floodplains on the delta top, but once the water flow exits into the sub-aqueous portion it enters a realm similar to that of vegetated deltas, with the exception of the presence of transported organics and in-fauna in the latter. Thus, the main differences between the sub-aqueous portions of deltas formed in vegetated versus non-vegetated regions will be mainly inherited from processes that occurred in the sub-aerial realm.
There is an extensive literature on sedimentation styles of fluvial systems in non-vegetated terrains (for review articles see: Long, 1978; Cotter, 1978; Long, 2004; Davies and Gibling, 2010; Davies et al., 2011; Ielpi et al., 2018; Long, 2019). Many of the aspects that are important in non-vegetated fluvial systems will influence the processes and products in the sub-aqueous portion of deltas connected to them. The intensity of chemical weathering prior to the development of land vegetation is the major determinant of clay production and is therefore important for the promotion of bank stability, floodplain extent and preservation potential. Some authors believe that larger amounts of CO2 in the Precambrian atmosphere (Kastings and Ono, 2006) would have led to more intense inorganic-driven hydrolysis reactions, and thus, more robust production of clays (Corcoran et al., 1998; Donaldson and Kemp, 1998; Hessler and Lowe, 2006; Hao et al., 2017), whereas others argue that without plant roots promoting organically-driven hydrolysis reactions clay production would have been significantly less than after rooted vegetation evolved (Fuller, 1985; Bennett and Siegel. 1987; Johnsson et al., 1991; Baars et al., 2008; Davies et al., 2011). Davies et al. (2011) also emphasize the likelihood of fluvial and aeolian removal of fines from the alluvial setting (Dalrymple et al., 1985; Davies and Gibling, 2010) and its increased abundance in marine settings. Many authors have commented on the lack of bank cohesion produced by low clay contents and lack of roots as important factors in the dominance of braided rivers in non-vegetated settings (Cotter, 1978; references in; Eriksson et al., 1998; Davies et al., 2011; Long, 2019). However, recently some examples of deep channels with lateral accretion have been reported (e.g. Ielpi and Rainbird, 2016a; Ielpi and Rainbird, 2016b). In addition, Almeida et al. (2016) infer that some lower reaches of non-vegetated fluvial systems may have developed deeper channels.
The prominence of high width to depth ratios of braided rivers in non-vegetated regions and time periods has a consequential effect on delta morphology and processes, leading to the dominance of braid or fan deltas depending on the local topography. Fan deltas (i.e. definition of Ventra and Clarke, 2018) form where a confined river valley meets a flooded area, whereas braid deltas form where a braidplain mostly composed of gravel and/or sand meets a standing body of water (i.e. definition of McPherson et al., 1987). Obviously these two types of deposits are difficult to distinguish in the rock record where their difference in slope can only be conjectured by examining grainsize and sedimentary structures to interpret distributary energy levels, or by the presence of debris-flows and sheetflood deposits (McPherson et al., 1987). Sedimentation processes on possible non-vegetated fan deltas are described by Roe (1995), Martins-Neto (1996), Gouramanis et al. (2003), Patranabis-Deb and Chaudhuri (2007), Bhattacharya and Mahapatra, (2008), Dhang and Patranabis-Deb (2011), Wendland et al. (2012), Ghandour et al. (2013), Bayet-Goll and de Carvalho (2016), de Oliveira Costa et al. (2018), Drabon et al. (2019), Vaucher et al. (2020), and Dhang and Patranabis-Deb (2011). Non-vegetated deltas that are more likely to be braid deltas are described by Vos (1981), Fralick and Miall (1989), Fedo and Cooper (1990), Haddox and Dott (1990), Hjellbakk (1993), Junnila and Young, (1995), Macnaughton and Narbonne (1999), Grazhdankin (2004), Fralick and Pufahl (2006), Rogala et al. (2007); Chakraborty and Sensarma (2008), Le Heron et al. (2010), Melvin (2015), Khalifa et al. (2015), Counts et al. (2016), Bayet-Goll and de Carvalho (2020), Muhlbauer and Fedo, (2020) and Went (2020). Due to the relative scarcity of fines capable of retaining water on the floodplains of some fan and braid deltas, as well as, or in combination with, locations in arid areas, modern examples of little-to non-vegetated fan and braid deltas exist (van Dijk et al., 2007; Ielpi et al., 2018; Hansford and Plink-Bjorklund, 2020). However, due to the lack of suitable modern analogues less is known about the stratigraphy and sedimentology of large, non-vegetated deltaic complexes that form in non-arid or non-glaciated environments with significant, fine-grained, interdistributary, delta top areas. To rectify this, detailed studies on large, pre-land macrophyte vegetation deltaic complexes with confined channels and extensive floodplains are needed.
The middle 350 m (m) of the Mesoproterozoic Sibley Group (Figure 1) represents a complete deltaic complex from prodelta to delta top fluvial system that was deposited in a semi-humid to humid setting with extensive delta-top floodplains (Rogala et al., 2007; Fralick and Zaniewski, 2012; Ielpi et al., 2018). Exposure is limited as most of the deltaic strata sub-crop under Nipigon Bay, Lake Superior. However, four fully cored drill-holes penetrate it (Figure 1), with two of these extending through the entire assemblage. This provides a two dimensional view of delta evolution over 27 km laterally. However, erosional truncation of the upper strata in one of the drill-holes limits the record of lateral extent of the assemblage to 4 km. The deltaic succession in the Sibley Group is divided between siltstone, shale and very fine-grained sandstones forming the prodelta in the Kama Hill Formation; and distal bar, distributary mouth bar and delta top deposits forming the Outan Island Formation (Rogala, 2003; Rogala et al., 2005; Rogala et al., 2007). The Outan Island Formation is further divided into the Lyon Member, consisting of the sub-aqueous portion of the delta, and the Hele Member, composed of the delta top sedimentary units (Rogala, 2003; Rogala et al., 2007; Fralick and Zaniewski, 2012).
Regional Setting
The 950 m thick, 1.4 Ga (Rogala et al., 2007) Sibley Group (Rogala et al., 2007) is present under and to the north of Lake Superior and east of Thunder Bay, Ontario (Figure 1). It is a nearly flat-lying, unmetamorphosed sedimentary succession intruded in places by 1.1 Ga diabase sills and dikes of the Mid-Continental Rift (Heaman et al., 2007). The succession is divisible into three depositional systems. The lower third of the Group, the Pass Lake and Rossport Formations (Franklin et al., 1980; Cheadle, 1986a; Cheadle, 1986b), unconformably overlies Archaen and Paleoproterozoic rocks with incised channels filled with braided fluvial deposits of the Pass Lake Formation overlying the older terrain (Franklin, 1970; Cheadle, 1986a; Cheadle, 1986b; Rogala et al., 2007). Lacustrine flooding resulted in the building of sandflats on the strandline shoreline and development of tempestites out into the lake. Progradational deltas developed in areas of maximum sediment delivery, whereas upward fining and thinning successions were deposed where creation of accommodation space outstripped sediment accumulation (Rogala et al., 2007). The lacustrine system of the Rossport Formation progressively changed from fresh to saline with the development of micritic dolomite precipitates, gypsum and halite (Cheadle, 1986a; Cheadle, 1986b; Rogala et al., 2007). The fluvial-playa system was supplied by sediment from the 1.83 Ga Trans-Hudson orogenic zone directly to the west northwest (Cheadle, 1986a; Rogala et al., 2007). However, a regional change in slope resulted in sandstone sheets entering the basin from the south causing the topography to shift to one of saline mudflats and ponds with a water body to the south (Franklin et al., 1980; Cheadle, 1986a; Cheadle, 1986b; Rogala et al., 2007).
A major hiatus, during which a flooding event occurred, separates the middle succession of the Sibley Group, the Kama Hill Formation, from the lower units (Cheadle, 1986a; Rogala et al., 2007). Rogala et al. (2007) described the lower portion of the Kama Hill Formation as: parallel laminated siltstone and fine-grained sandstone with interbeds of mudstone and ripple laminated, fine-grained sandstone. They interpreted this as a prodelta, overlain by the Lyon Member of the Outan Island Formation, which consists of coarsening-upward cycles gradually progressing from sediment similar to the fine-grained material of the Kama Hill Formation to massive, trough and hummocky cross-stratified sandstone layers commonly with wave rippled tops. Slump scars, slide blocks and chaotic intraformational conglomerates are also present. These features represent distal bar to distributary-mouth bar successions and are overlain by floodplain and channel deposits of the Hele Member, Outan Island Formation (Rogala, 2003; Rogala et al., 2007; Fralick and Zaniewski, 2012). The floodplains developed in a reasonably maderately wet climatic setting adjacent to, presumably, somewhat laterally confined, 5–8.5 m deep, sand and gravel floored distributary channels with low levees (Fralick and Zaniewski, 2012; Ielpi et al., 2018). The intervening floodplains were extensive, silt and very fine-grained sand dominated, with abundant crevasse splays feeding into floodbasins. Ripples, rip-up mud chips, and soft sediment deformation, including load and injection features are especially common, except in floodplain pond deposits, which are dominated by parallel lamination (Fralick and Zaniewski, 2012; Ielpi et al., 2018). Both paleocurrents (Cheadle, 1986a; Cheadle, 1986b) and detrital zircon U-Pb geochronology (Rogala et al., 2007) indicate the sediment was derived from the south to south east.
The upper third of the Sibley Group is formed by aeolian sandstones of the Nipigon Bay Formation. It appears to lie on a disconformity with the floodplain sediments of the Outan Island Formation and was deposited by a prevailing wind direction from the northeast (Rogala et al., 2007). It’s approximately 450 m of thickness is almost entirely large-scale, planar cross-stratified and parallel laminated medium-grained sandstone (Rogala et al., 2007).
Methodology
Nine hundred and seventy five meters of 4.5 cm diameter drill core, stored at the Conmee Core Yard, Thunder Bay Distract, of the Ontario Ministry of Northern Development and Mines, was logged on the decimeter-scale. Contacts between layers, and layer thicknesses were noted; layers being regarded as successions of the same lithofacies (see Tables). Internally laminae thicknesses were also noted; laminae thickness being defined as width of individual horizontal laminae or thickness between set boundaries for cross-laminae. Grainsize of sediment in the layers, plus any sedimentary structures were recorded. In addition to sedimentary structures created during deposition, penecontemporaneous deformation structures were also noted. Sedimentary structures and any other features of interest were recorded. The Kama Hill was differentiated from the underlying Fire Hill Member of the Rossport Formation by the presence of carbonates and diagenetic minerals in the latter. The Kama Hill is overlain by the Outan Island Formation with a gradational contact. The Outan Island Formation is overlain by aeolian sandstones of the Nipigon Bay Formation, which are distinctive from the underlying sandstones and siltstones. Graphic logs were created from the field notes at a scale of 1cm equals 1m and these were used to interpret sequence stratigraphy.
Sedimentology: Lithofacies Associations
For descriptive purposes the succession has been divided into three portions: a basal, approximately 150 m thick succession, forms a coarsening- and thickening-upward; overlain by another less well structured, approximately 100 m thick, coarsening- and thickening-upward succession; and capped by a 100 m thick heterogeneous assemblage (Figure 2). The lithofacies associations that form each of these three portions are described below.
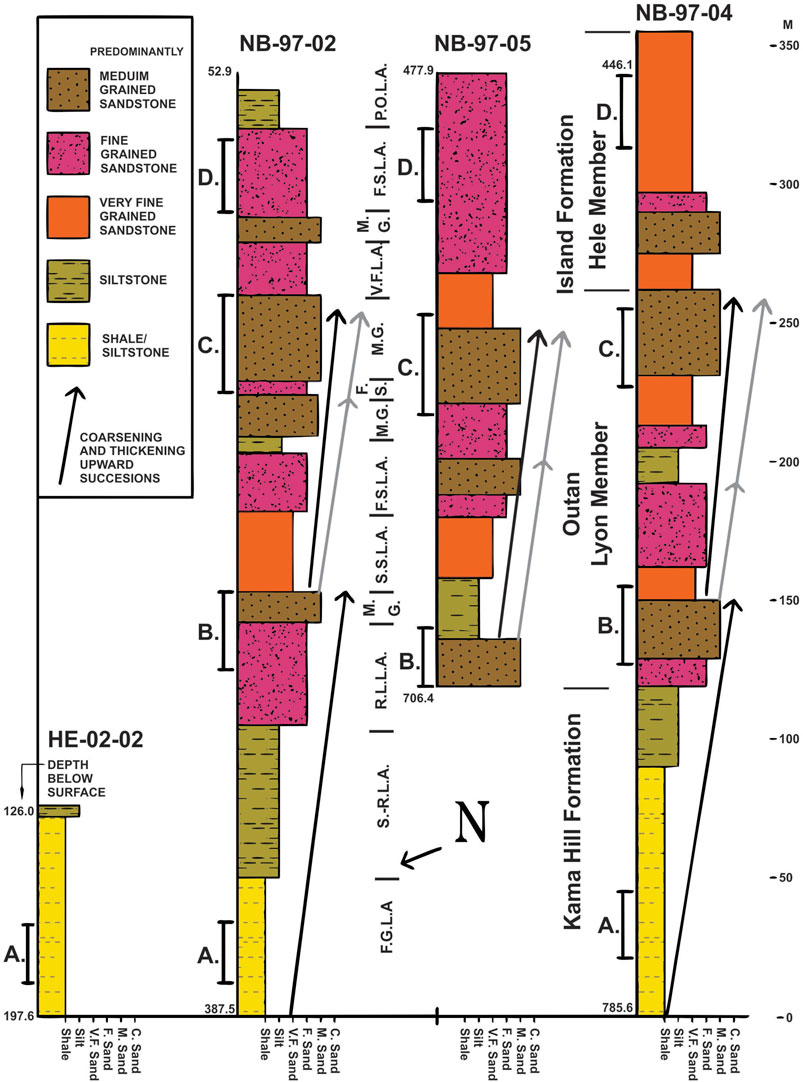
FIGURE 2. Stratigraphy of the four drill-holes that intersected the deltaic complex. Large-scale coarsening- and thickening-upwards trends are marked as are the two subsidiary coarsening upward trends in the middle coarsening upward succession. The location of lithofacies associations is marked by: F.G.L.A., Fine Grained L. A.; S.-R. L.A., Silt-Rich L.A.; R.L.L.A., Ripple Laminated L.A.; M.G., Medium Grained L.A.; S.S.L.A., Silty Sandstone L.A.; F.S.L.A., Fine-Grained Sandstone L.A.; V.F.L.A., Very Fine-Grained Sandstone L.A.; and P.O.L.A., Poorly Organized L.A. A, B, C and D with brackets refers to more detailed sections present in Figures 3, 6,8,9 respectively.
The Lower 150 m, Coarsening-Upward Succession
This succession conformably overlies the Rossport Formation, a saline mudflat assemblage (Rogala et al., 2007). The juxtaposition of sub-aerial mudflat deposits and sub-aqueous, off delta deposits requires an intervening major hiatus (Rogala et al., 2007).
Fine-Grained Lithofacies Association
The Fine-Grained L.A. overlies the Rossport Formation with an irregular contact. The overall trend of this lithofacies association is a series of coarsening-upwards parasequences (Figure 3), ranging from 5 to 30 m thick (Figure 4A), averaging 20 m, and composed of alternating beds containing shale and siltstone with less common very fine-grained sandstone. Most of the lithofacies are comprised of thin, parallel laminated siltstones (Figures 4B,C,F–H) overlain by thin mudstones and sporadic, very fine-grained sandstones with associated shales. These can be divided into: massive siltstone (Figures 4D,E); parallel laminated siltstone and contorted, parallel laminated siltstone (Figures 4F–H) parallel laminated shale and contorted, parallel laminated shale; parallel laminated sandstone; ripple laminated sandstone; lensoid layering; and wispy layering (Table 1 and Figure 4). Mud rip-ups and dewatering structures are prominent features in the upper half of this assemblage.
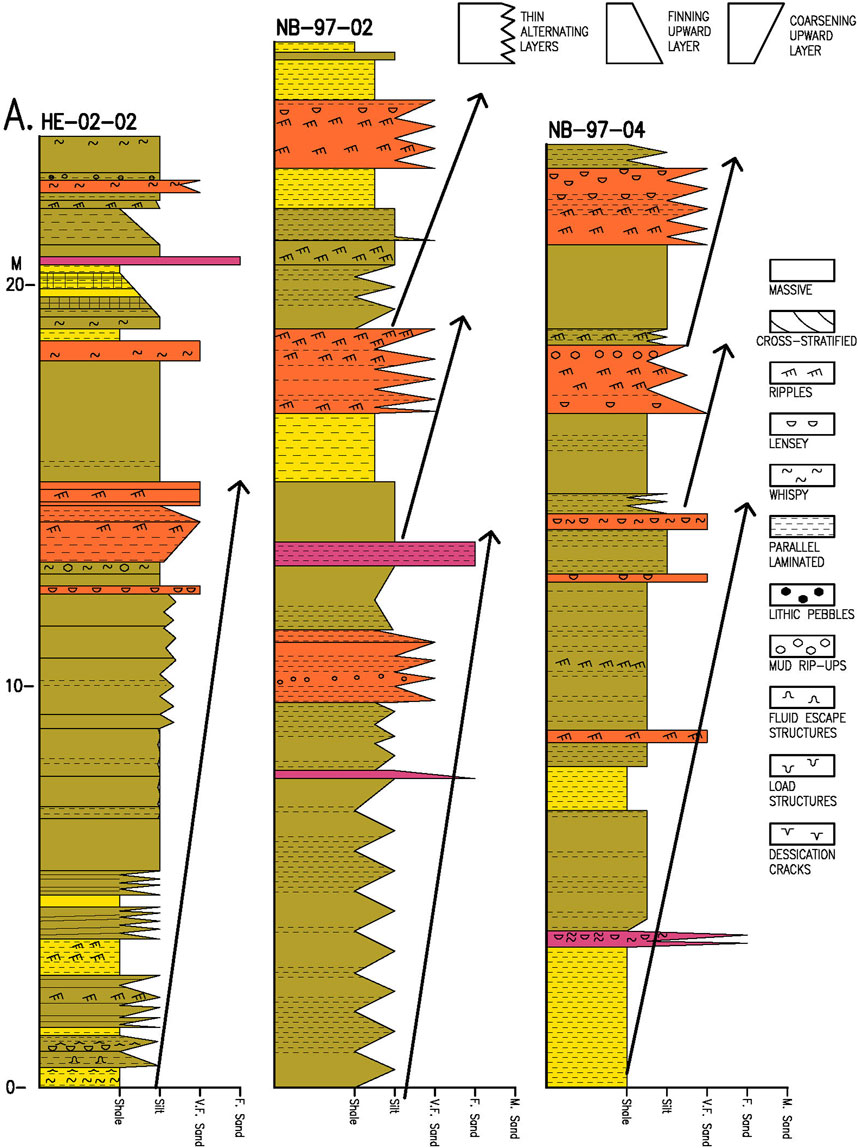
FIGURE 3. Detail of parasequences in the basal portion of the lower coarsening- and thickening-upward succession. The three parasequences in NB-97-02 are relatively aggradational, whereas the same three parasequences in NB-97-04 are progradational. This highlights that the aspect of parasequence sequencing can vary due to position of the depositional site relative to the sediment entry point, which controls development of accommodation space. The lowermost parasequence is coarsest in drill-hole 02 indicating a position closest to the sediment entry point into the subaqueous delta. The upper two parasequences are coarsest in section 04, likely caused by movement of the distributary mouth closer to this area. This positioning would result in shallower water where these two parasequences were deposited and thus they were more likely to prograde. Location of detailed sections in A depicted on Figure 2. Lensey refers to lensoid layering, which is equivalent to indistinct lenticular bedding. Wispy layering consists of contorted, thin layer fragments.
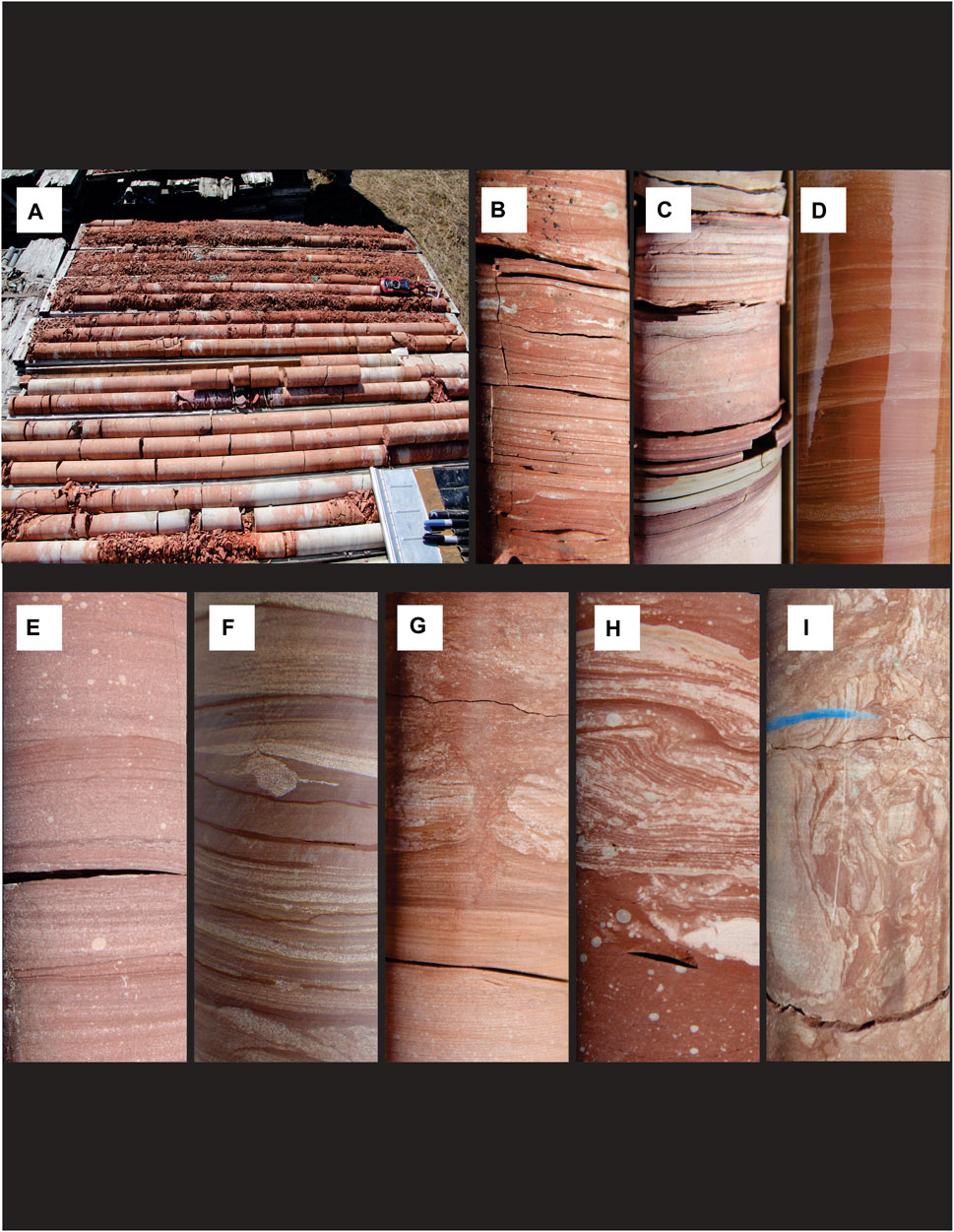
FIGURE 4. Lithofacies that are common in the lower and middle portion of the lower coarsening- and thickening-upward succession and lower portion of the upper coarsening- and thickening-upward succession. Core is 4.5 cm wide. (A) Coarsening upward, 30 m thick, progradational parasequence with red mudstone dominating the lower portion (top of photograph), light red siltstone dominating the middle of the figure and siltstone plus white sandstone present in the top (bottom of photograph). (B) Layers of parallel laminated clay-rich and clay-poor siltstone. (C) Parallel laminated green mudstone; light red siltstone; and white, very fine-grained sandstone. (D,E) Massive layers grading from clay-poor to clay-rich siltstone. Light circular areas are reduction spots where the iron was diagenetically reduced by organic carbon. (F) Massive, graded laminae with minor loading and sediment injection. Lensoid layering in bottom portion of the core. Horizontal lines were produced by drill chatter. (G) Dewatering injection. Such features are very common in the fine-grained sediment. (H) Contorted and disaggregated bedding produced by shear stress generated during slumping. (I) Slump deposit consisting of disaggregated layers.
Grey and red, massive siltstone and associated, subordinate shale dominate the lower portion of the lithofacies association. It is present as thin layers overlain by mm-scale shale laminae and successions of such layers, which are intercalated with parallel laminated shales, siltstones and silt-rich very fine-grained sandstones. Wispy layered, very fine-grained sandstones are also associated with the massive siltstones, with the contorted, discontinuous layers formed by penecontemporaneous water escape. The parallel laminated siltstones also have abundant water escape structures, which primarily consist of sills and dikes, and less common load structures. The parallel laminated siltstone units are less common in the upper 15 m of the Fine-Grained L.A. Here the laminae are thicker, with an average thickness of 7 mm, and are associated with wave rippled siltstone. Near the top of the lithofacies association the siltstone and very fine-grained sandstone laminae range from 0.5 to 2 cm in thickness. The parallel laminated, very fine-grained sandstones are not a common lithofacies and are concentrated at the top of the previously mentioned approximately 20 m thick, coarsening upwards parasequences that make up the Fine-Grained L.A. They also become more common in parasequences further up the succession. These units are all composed of mostly graded laminae of very fine-grained sands, with associated laminae of silts and clays. Ripple laminated, very fine-grained sandstone beds are most common in the middle of this lithofacies assemblage. They commonly have abundant mud flasers, but are rarely associated with dewatering structures, due to the destructive nature of the dewatering processes, which tend to transform ripples into lensoid and wispy layering. Lensoid, very fine-grained sandstone beds contain sand lenses imbedded in massive and contorted parallel laminated siltstones and more rarely silt-rich shale. The lenses represent poorly formed ripples with rounded or irregular shape. The latter are generally associated with subsequent wispy layering. The lensoid beds commonly interlayer with parallel laminated sandstones and siltstones or wispy layered sandstones. Conversely, red, parallel laminated shale and contorted, parallel laminated shale are rare and most abundant in the lower portion of the Fine Grained L.A. Individual layers of shale commonly cap other units and are generally no greater than 1 cm thick.
Silt-Rich Lithofacies Association
The Silt-Rich L. A. dominates the middle portion of the lower coarsening upward succession overlying the Fine-Grained L.A (Figure 2). The assemblage is composed of interlayered massive, parallel laminated and convolute laminated, clay-rich siltstones, and a large portion of ripple laminated, silt-rich, very fine-grained sandstone units (Table 2 and Figure 4). The first appearance of medium sized sand grains occurs scattered throughout this assemblage in the form of cross-stratified and ripple laminated layers. The lithofacies association is organization into coarsening upwards parasequences averaging 10 m thick. This lithofacies association is composed of massive, silt-rich shales; ripple laminated, silt-rich sandstone; parallel laminated and contorted, clay-rich siltstone; and parallel laminated and contorted, silt-rich sandstone.
Ripple laminated, silt-rich, fine-grained sandstones and parallel laminated siltstones are the most abundant lithofacies throughout this lithofacies association. The ripples are commonly associated with mud flasers, 1 mm or less thick, which wisp between each fine sandstone laminae on the avalanche side of the ripple. In places wave ripples alternate with convolute bedding and are associated with distinct water escape and load structures. Massive siltstone and massive shale units are composed of the same grain-sized materials present in the underlying lithofacies association. However, they more commonly appear together as silt-rich-shales in this lithofacies assemblage. Parallel laminated and contorted, clay-rich siltstones commonly cap massive siltstone layers and convolute laminated, fine-grained, silt-rich sandstones. Also present are rare, cross-stratified sandstones composed of fine- and medium-grained sand with mud rip-ups oriented parallel to the laminae. Sand injections and other water escape structures are common throughout this lithofacies assemblage and average 2 cm wide. They increase in abundance upward. Reduction spots 0.5–2 cm in diameter are very common in the lower half of the drill holes.
Ripple Laminated Sandstone Lithofacies Association
This lithofacies association overlies the Silt-Rich L. A. and consists of successions displaying a gradual increased in grainsize and convolute bedding over repeating, 20 m thick, asymmetric cycles (parasequences). The dominant primary structural lithofacies features are ripple laminated, very fine-grained sandstone and siltstone with an abundance of water escape structures resulting in areas of wispy bedding. Also present are parallel laminated, fine-grained sandstones to siltstones, wispy layered fine-grained sandstone in siltstone and hummocky cros-stratified (H.C.S.) fine-grained sandstone (Figure 5).
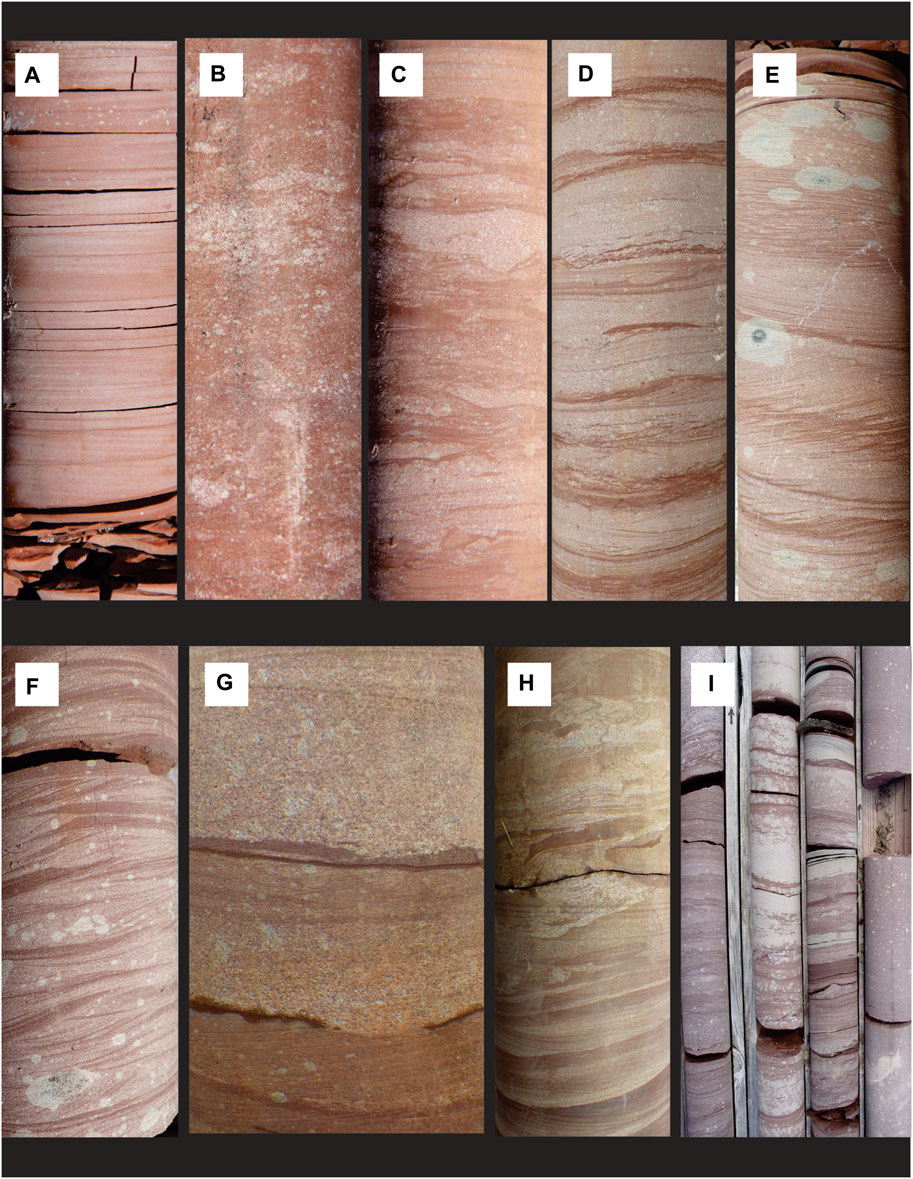
FIGURE 5. Lithofacies that are common in the middle and upper portions of the lower and upper coarsening- and thickening-upward successions. Core is 4.5 cm wide. (A) Less than 1 cm thick layers of parallel laminated siltstone with less than 1 mm thick intervening mudstones. (B) Wispy very fine-grained sand in siltstone. (C) Lensoid very fine-grained sand in siltstone. (D) Massive and parallel laminated layers of fine-grained sandstone interlaminated with current ripple laminated, fine-grained sandstone and thin, mudstone drapes. (E) Cross-lamination produced by continuous crested current ripples. The high dip angle of set boundaries denotes rapid sedimentation from suspension during ripple migration. Silty-clay flasers and drapes accentuate layering. The oval mark is an organic cored reduction spot. (F) Lingoid or lunate current ripples with flasers. (G) Layers of medium-grained sandstone grading to siltstone with drapes of mudstone that were eroded during subsequent flow events producing sand-sized clay flakes in the overlying sandstone. Horizontal lines were produced by drill chatter. (H) Layers grading from fine-grained sandstone to siltstone or mudstone overlain by an area of disrupted layering, probably due to water escape. (I) Core younging from bottom left to top right. This is a transitional area near the top of the lower coarsening-upward succession with increasing sand content and layer thickness culminating in thick, medium-grained, massive sandstone layers (e.g. core at the top of the succession at right in this box).
Current ripple laminated, very fine-grained sandstone units are extremely abundant throughout this lithofacies association. They commonly transition into lensoid (Figure 5C) or wispy (Figure 5B) siltstone with deformed sandstone lenses 0.2–0.5 cm thick, or in the case of wispy layers, swirled laminae that water escape and loading has made identifying the precursor difficult. The units range from 20 to 197cm thick, averaging 70cm. Grainsize fines upwards both within individual layers and units. The ripple laminated, very fine-grained sandstones are commonly associated with convolute bedded, clay-rich, fine-grained sandstone units with thin mud rip-up layers. Wave ripple laminated, fine- and very fine-grained sandstones with mud flasers are interlaminated with 0.1–1 cm thick red shale layers with abundant water escape structures. These layers are commonly nested within thicker sections of wispy bedded, fine-grained sandstone units; parallel laminated fine- and very fine-grained sandstones; and cross-stratified, fine-grained sandstones. Wave ripple laminated, medium-grained sandstones are also present in this L.A as are hummocky cross-stratified (H.C.S.), fine-grained sandstones. The H.C.S. is commonly overlain by parallel laminated, very fine-grained sandstone or wave ripple laminated, fine-grained sandstone. Other parallel laminated, very fine-grained sandstones grade to parallel laminated, clay-rich siltstones, which are in places capped with mud drapes or truncated by wave ripple laminated, fine-grained sandstone.
Medium-Grained Sandstone Lithofacies Association
This lithofacies association primarily lies above the Ripple Laminated L.A. at the top of the lower coarsening upward succession (Figure 6). It is largely composed of ripple laminated and cross-stratified medium-grained sandstone, lensoid and horizontally laminated, fine-grained sandstone (Table 1 and Figure 5). Additional lithofacies interlayered in this association are: massive, fine-, medium-, and coarse-grained sandstone.
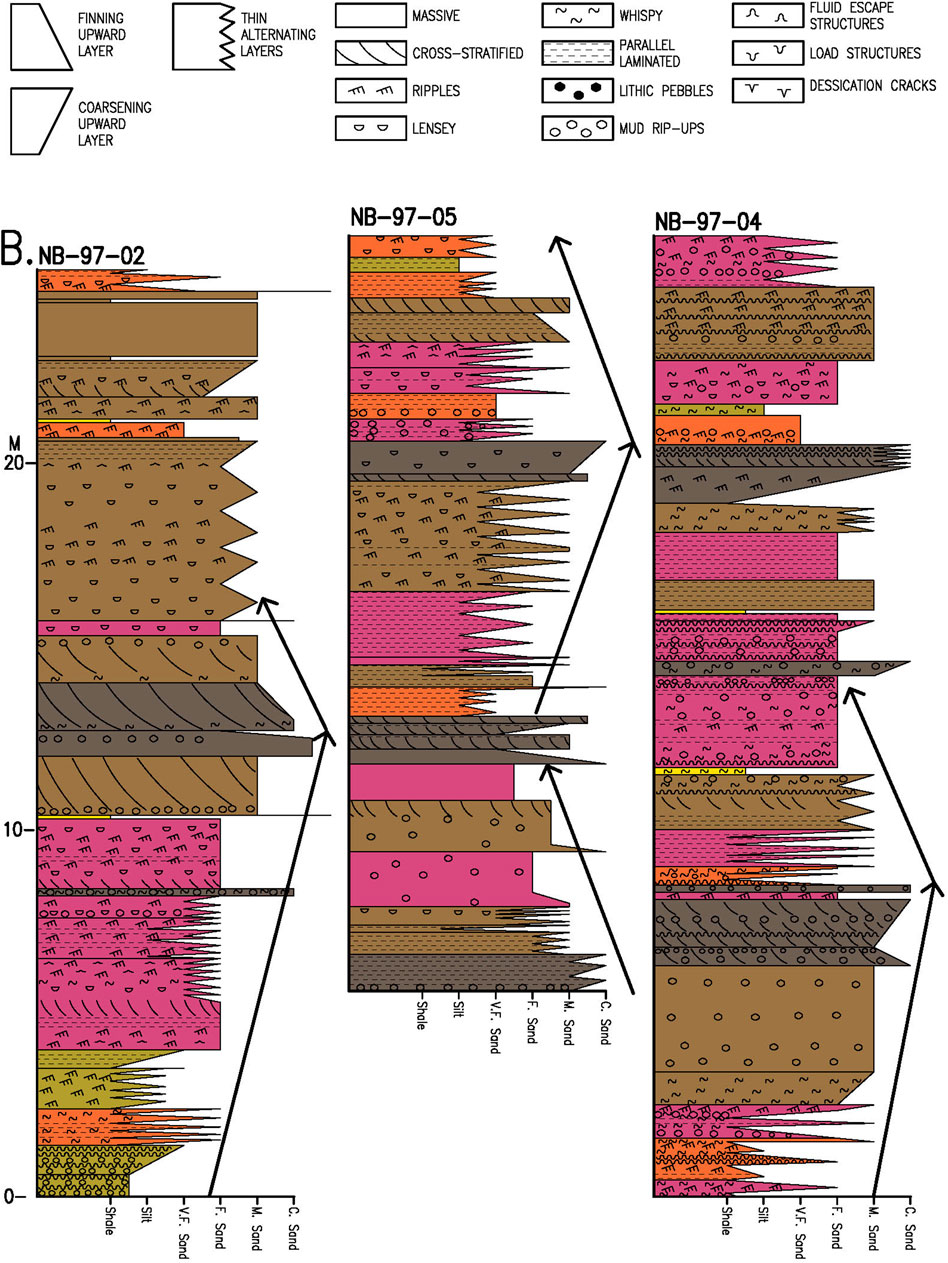
FIGURE 6. Strata forming the top of the lower coarsening- and thickening-upward succession. The lower coarsening-upward parasequences in NB-97-02 and NB-97-04 are overlain by fining upward parasequences. This may reflect the top of the distributary-mouth bar overlain by channel deposits. Hole NB-97-05 ended before penetrating the coarsening-upward parasequence, but it contains the fining-upward parasequence that overlies it. The upper portion of the strata detailed here is unstructured in NB-97-02 and NB-97-04, but continues to have a progradational and retrogradational parasequence coarsening and fining cycles in NB-97-05, possibly reflecting this are reflooding with reestablishment of a bar top distributary system. Location of detailed sections in B depicted on Figure 2.
Both current ripples and wave ripples are present, with the latter forming the upper surface of other sandstone layers. The current ripples are commonly present within thicker successions of lensoid laminae, from which they are transitional to and from. Upper flow regime, parallel laminated, fine-grained sandstone beds commonly are associated with ripple laminated and lensoid sandstone. Massive, medium-grained sandstone beds and rarer fine-grained massive sandstones are also present. The massive, medium-grained and fine-grained sandstone beds infrequently in places transition upward into upper flow regime, parallel horizontal laminated, medium-grained sandstone topped with a ripple laminated layer. However, the beds are more commonly followed by cross-stratified sandstone. The cross-stratified, medium-grained sandstones lack visible dewatering structures and mud rip-ups, but a few contain large clay blocks, some wider than the core, imbedded within the cross-stratified laminae. The coarsest beds in this lithofacies association are massive, coarse-grained sandstones, which fine upward into medium-grained sandstone, then fine-grained sandstone. These beds lack visible dewatering structures but can contain mud rip-ups concentrated near the layer’s basal contact. Compared to the previous three lithofacies, massive, fine-grained sandstones rarely occur, but where present contain a few medium sized sand grains surrounded in a matrix of fine sand grains.
The Middle, 100 m, Coarsening Upward Succession
The middle coarsening upward succession contains two subsidiary coarsening upward successions (Figure 2), with the lower one thicker in the east (NB-97-02), and the upper one thicker in the west (NB-97-04). In hierarchical fashion, a number of thinner, coarsening upward parasequences internal to those are also present. The middle coarsening upward lithofacies association is composed of three lithofacies associations (Table 2).
Silty Sandstone Lithofacies Association
This lithofacies association overlies the lower, 150 m thick, coarsening upward succession. It is relatively thin and transitions upwards into the Fine-Grained Sandstone L. A.
Parallel laminated siltstone and silt-rich, very fine-grained sandstone dominates this lithofacies association (Table 2 and Figure 7A), but in places the granular material is very clay-rich. Imbedded in this background sediment are lensoid and wispy, silt-rich very fine-grained sandstones, parallel laminated, very fine- and fine-grained sandstones and increasing amounts of wave ripple laminated, silt-rich, fine-grained sandstone near the upper contact. Unit thickness ranges from 6 to 50cm, with individual, commonly fining-upwards, laminae averaging less than 1cm thick. Mud rip-ups are abundant within a few centimetres of the basal contact of the laminae packages and in concentrated layers throughout these units. Parallel laminated siltstone, and very fine-grained to fine-grained sandstone, containing less silt, are also present. Fining-upwards beds of parallel laminated, fine-grained sandstone are commonly associated with wispy, lensoid and wave ripple laminated, silt-rich, fine-grained sandstone. Frequently these units are present as single ripple trains. Small dewatering structures, mostly composed of dikes and sills, and small mud chips are common.
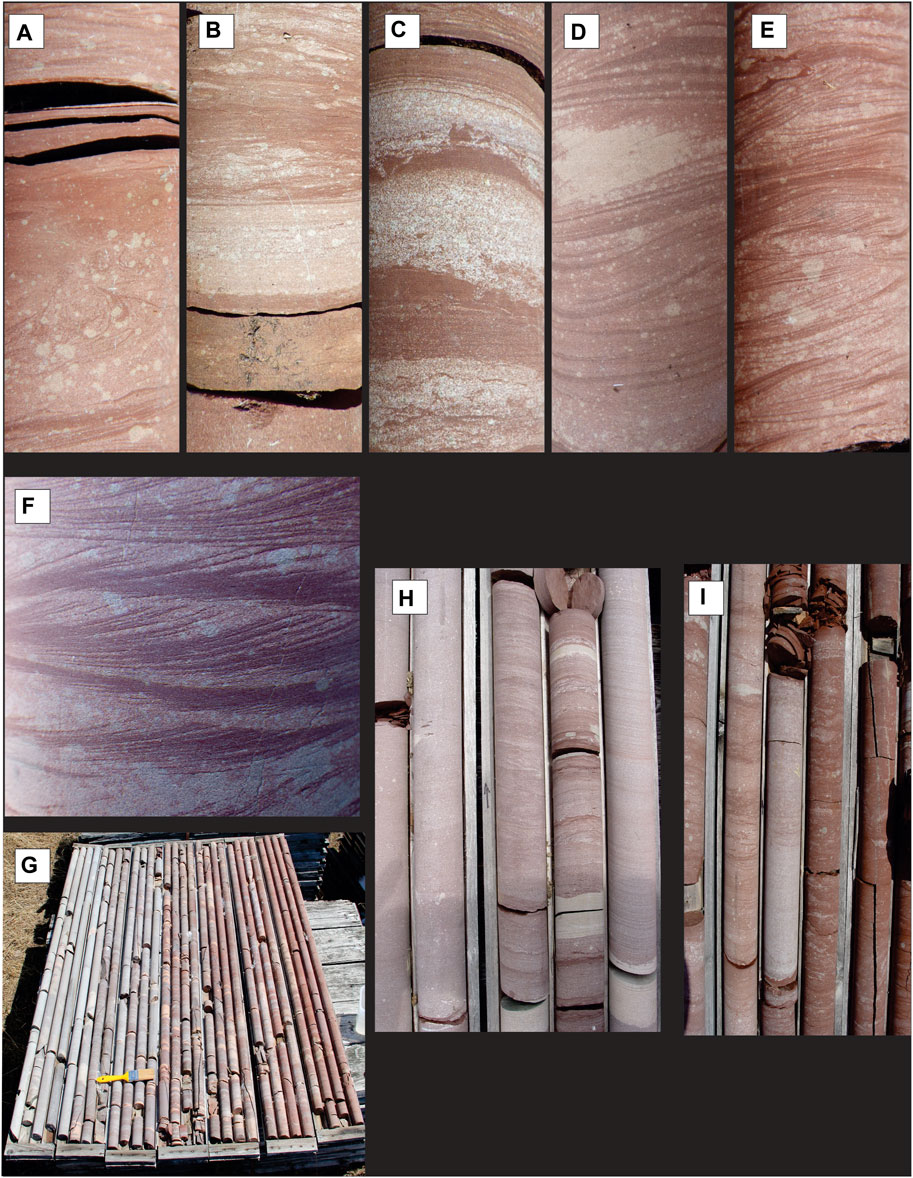
FIGURE 7. Lithofacies that are common in the upper portion of the two large-scale coarsening- and thickening-upward successions containing parasequences. Core is 4.5 cm wide. (A) Graded, massive and parallel laminated, very fine-grained sandstone and siltstone layers with small-scale erosive scouring and contorted laminae, probably due to dewatering. (B) A graded fine-grained sandstone layer erosively truncated and overlain by wispy layering. (C) Massive and parallel laminated medium-grained, graded sandstone layers, with small clayey-silt rip-ups, interlayered with clayey-silt; some of which are also graded. (D) Small-scale hummocky cross-stratification overlain by ripple lamination in medium-grained sandstone. (E) Wave ripple laminated, medium-grained sandstone with silt and clayey-silt flasers. (F) Current ripple laminated, fine-grained sandstone with clayey-silt flasers. (G) Coarsening upwards sequence near the top of the upper coarsening- and thickening-upward succession. The amount of fine- and medium-grained sandstone layers increase upward until they dominate. Bottom is at upper right; top at lower left. (H) Core from the upper portion of the second coarsening- and thickening-upward succession. Cross-stratified, medium-grained sandstones, on right, separated from massive, medium-grained sandstones, on left, by thinly layered siltstones and fine-grained sandstones. Bottom is lower left and top is upper right. (I) Core from the floodplain assemblage where lensoid layered, very fine-grained sandstones and siltstones are overlain by a sharp-sided, medium-grained crevasse splay deposit with a clay infused top, which in turn is succeeded by parallel laminated shale and clayey-siltstone. Bottom is lower left and top is upper right.
Fine-Grained Sandstone Lithofacies Association
The fine-grained sandstone lithofacies association occurs at two levels in the middle coarsening upward succession. Its first appearance is low in the succession, above the Silty Sandstone L. A. The second occurrence is at the base of the second subsiduary coarsening upward cycle below the Medium-Grained Sandstone L. A., at the top of the large-scale coarsening upwards succession. The lower occurrence is dominated by rippled, lensoid and wispy, fine-grained sandstone in the lower reaches and rippled and convolute bedded fine-grained sandstone near the top; whereas the upper occurrence is comprised of mostly parallel laminated fine-grained sandstone and shales (Table 2 and Figure 4).
The lower occurrence has abundant current and wave ripple laminated layers, commonly composed of a higher concentration of medium-grained sand than the other layers. Frequently these units contain thin mud rip-up layers, water escape structures, mud injections and loading features. Thinner layers of parallel laminated, fine-grained sandstone overlain by shale; convolute laminated layers; cross-stratified, fine-grained sandstone; and coarse-grained ripple laminated and cross-stratified sandstone are sporadically present.
The second occurrence is comprised mostly of interlayered wispy and graded, parallel laminated, fine- and medium-grained sandstone with shale drapes and rip-ups. Cross-stratified and hummocky cross-stratified, medium-grained sandstone layers and current and wave ripple laminated fine-grained sandstone are significantly less common. Massive, medium-grained sandstone are rarely present. Though rare the massive sandstone units present in this lithofacies association are distinctive with one reaching 2.3 m thick and containing large mud and silt rip-ups. The beds are either reverse or normal graded with the latter upward transitional into parallel laminated, fine-grained sandstone capped by either cm-scale thicknesses of shale or wave ripples. This lithofacies association is followed by the Medium-Grained Sandstone L. A.
Medium-Grained Sandstone Lithofacies Association
Two occurrences of this lithofacies association are present in the middle coarsening upward succession of drill-holes NB-97-02 and NB-97-05 (Figure 2). One occurs near the middle of the succession and the other caps the succession. Drill-hole NB-97-04 only contains one assemblage of medium- and coarse-grained sandstone at the top of the coarsening-up succession.
The lower occurrence is dominated by cross-stratified, massive and parallel laminated sandstones with mud rip-up clasts (Table 2 and Figure 7). Lensoid layers become more common in the upper portion of this association. The cross-stratified, medium-grained sandstones form stacked successions of cross-stratified lenses 13–93cm thick associated with relatively thinner massive sandstones. Layers of mud rip-ups are rare in the cross-stratified sandstone, but common in the massive ones, in places creating coarse-tail grading. The massive sandstones average 30cm thick and are separated by shale layers less than 1 cm thick, but lack load features. Some of these medium-grained sandstones grade upwards into parallel laminated, fine-grained sandstones. One 355 cm thick massive bed contains in its upper portion: parallel laminated medium-grained sandstone, which then grades into cross-stratified medium-grained sandstone and finally transitions into wispy ripple laminated medium-grained sandstone increasing in clay content upwards. Also present in the lower occurrence of this lithofacies association are layers of parallel laminated, medium-grained sandstones; lensoid and wispy ripple laminated, medium-grained sand in silt-rich shale; massive fine-grained sandstone; and parallel laminated shale.
The upper Medium-Grained Sandstone L.A. at the top of the 100 m thick coarsening upwards succession (Figure 8) continues its upwards coarsening trend within the association (Figure 7G). The strata capping the coarsening upward succession in NB-97-02 are notably coarser grained than those in NB-97-05, which in turn is coarser grained than NB-97-04 (Figure 8).
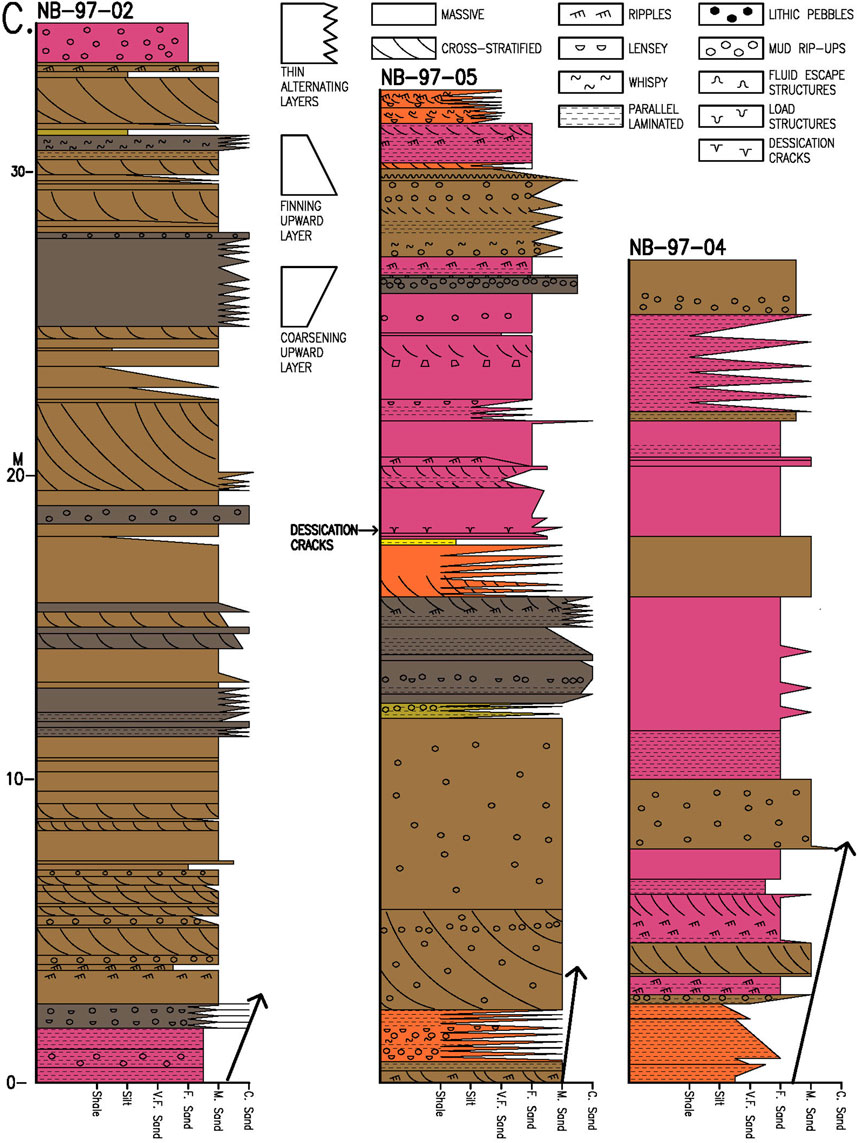
FIGURE 8. Detail of strata present at the top of the middle coarsening-upward succession. The underlying coarsening upward parasequence is overlain by unstructured distributary-mouth bar top sediments. The interval is dominated by medium- and fine-grained, massive and trough cross-stratified sandstones. The overall grainsize decreases from NB-97-05 in the northeast to NB-97-04, 4 km to the southwest. Location of detailed sections in C depicted on Figure 2.
The lithofacies association is dominated by thick massive and cross-stratified sandstones (Figure 7H). The massive sandstones average 125cm thick and range up to 276 cm thick with shale-rich tops. Small pebbles occur near the base of some, with pebbles, and rarer cobbles, in others scattered throughout the layer. Some massive sandstones are mixtures of medium-, coarse- and fine-grained sand with no organized internal structure, whereas others are normal or reverse graded. Successions of massive sandstone alternate with series of cross-stratified sandstone. The cross-stratified, medium- and fine-grained sandstones in places have pebbly sand in their scour fills and some laminae consist of coarse-grained sand. Stacked successions of troughs reach up to 3 m thick, though most are thinner and separated by cm-scale layers of shale. The parallel laminated, coarse- and medium-grained sandstones are graded to parallel laminated, fine-grained sandstones. Subsidiary units of ripple laminated, medium-grained sandstone; massive, fine-grained sandstone; parallel laminated, fine-grained sandstone; and lensoid and wispy, fine-grained sandstone with siltstone are also present.
Upper, 100 m, Heterogeneous Assemblage
The two thick coarsening-up successions described thus far share similar lithofacies successions in comparable order of appearance. This does not apply to the strata forming the upper portion of the deltaic succession. The lithofacies represented by the rocks in the upper portion of hole NB-97-02 are somewhat coarser grained than those in NB-97-05 and considerably coarser grained on average than those in NB-97-04 (Figures 3, 9); representing a fining trend to the southwest across 4 km (Figure 2). A further description of the delta-top strata in drill-hole NB-97-02 is given in Ielpi et al. (2018), and especially Fralick and Zaniewski (2012).
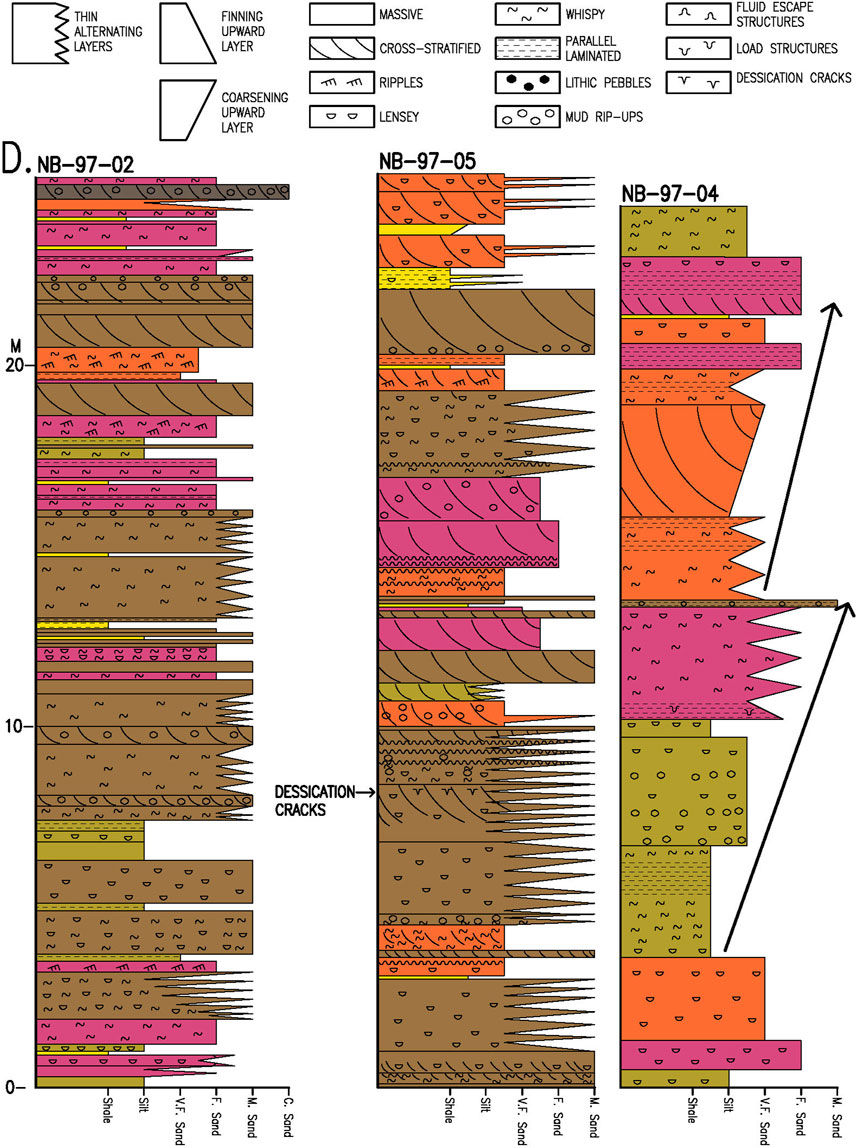
FIGURE 9. Detail of strata in the upper heterogeneous assemblage. Similar to the strata forming the top of the middle coarsening-upwards assemblage grainsize fines from NB-97-05 in the northeast to NB-97-04 in the southwest (Figure 8). In addition the finer grained sediment in NB-97-04 are is organized into approximately 10 m thick coarsening upwards cycles. Location of detailed sections in D depicted on Figure 2.
Very Fine-Grained Sandstone Lithofacies Association
Immediately above the medium-grained sandstone lithofacies association marking the top of the middle coarsening upward succession is the Very Fine-Grained Sandstone L.A. In all three drill cores the lithofacies association displays fining upwards then coarsening upwards trends. It is coarsest in NB-97-02. Relative to other lithofacies associations very few mud rip-ups are present.
Parallel laminated, very fine-grained sandstones separated by thinner siltstone or shale laminae are a common constituent of this lithofacies association (Table 3). Units average 30cm thick and are separated by thinner siltstone or shale laminae. These are interbedded with very fine- and fine-grained, small-scale cross-stratified sandstones; massive, very fine- and fine-grained sandstone; and, lensoid and wispy, very fine-grained sandstone. Layers with well-preserved wave ripples are common. In some areas, ripples composed of very fine-grained sandstone appear almost lensoid and are surrounded by shale-rich siltstone or are draped with mud flasers up to a few cms thick. One 6 cm thick layer of hummocky cross-stratification is also present. All of these lithofacies contain siltstone and shale laminae.
Medium-Grained Sandstone Lithofacies Association
The Very Fine-Grained Sandstone L. A. coarsens upward into layers of the Medium-Grained Sandstone L.A., and culminating in drill-hole NB-97-02 with a fining-upward 8.5 m thick occurrence dominantly composed of massive, medium-grained sandstones, with minor sections of cross-stratified, medium-grained sandstones and a 43 cm thick pebble conglomerate near its base. Massive, medium-grained sandstone beds are up to 240 cm thick and successions of cross-stratified sandstones reach 79 cm thick. Minor lensoid, rippled sandstone and silty shale separate some layers. Minor wispy and parallel laminated, fine-grained sandstones; lensoid, rippled sandstone; and silty shale are also present as thin layers.
The medium-grained sandstone section in drill-hole NB-97-05 lacks the large units of massive, medium-grained sandstone greater than 2 m thick present in NB-97-02, but does have several moderately thick medium-grained, cross-stratified sandstone beds. These have clay-rich, fine-grained sandstone laminae, mud drapes, and, one or two ripple trains on their upper surface. The first desiccation cracks appear associated with this succession. The trough cross-stratified sandstones are interlayered with ripple laminated, medium- and fine-grained sandstone lenses in encasing siltstone, averaging 120cm thick. Rare fine-grained, massive sandstones up to 50cm thick and thin, fine-grained, wispy and parallel lamination are also present.
The occurrence of the Medium-Grained Sandstone L. A. in drill-hole NB-97-04 contains more cross-stratified sandstone and lensoid sandstone units than the other drill core. The overall grain-size is finer than in NB-97-02 and -05. The cross-stratified sandstones average 150cm thick and are composed of varying amounts of fine- and medium-grained sand. They are interlayered with lensoid, very fine-grained sandstone units averaging 40cm thick; massive, medium-grained sandstones, which are less than 50cm thick; or parallel laminated and low angle cross-stratified sandstone. The lensoid sandstone successions are up to 208cm thick with fine-grained sandstone ripples in siltstone. The massive sandstone average 10cm thick and are composed of very fine-, fine- and medium-grained sand. The finer grained sandstone layers grade upwards to siltstone and layers of massive and parallel laminated shale are also present.
Fine-Grained Sandstone Lithofacies Association
The Fine-Grained Sandstone L. A. lies immediately above the Medium-Grained Sandstone L. A. in drill-holes NB-97-02 and NB-97-04. This lithofacies is coarsest in NB-97-02 and fines upwards in both drill-holes. Composed largely of thick successions of fine- and very fine-grained sandstones, this lithofacies association also has isolated interlayered areas of siltstone and a few thin layers of medium-grained sandstone. Very few mud rip-ups are present, relative to other lithofacies associations.
In drill-hole NB-97-02 this lithofacies association is dominated by ripple laminated sandstone. Medium- and fine-grained sand lenses and contorted wisps imbedded in silt are the most common unit. They are interlayered with current ripple laminated, medium-grained sandstones and wave ripple laminated, fine-grained sandstones. They have sharp contacts with other units and contain abundant mud rip-ups near these areas. In places these units alternate with parallel laminated siltstone, massive siltstone and massive shales.
Parallel laminated, fine-grained sandstones and siltstones dominate the fine-grained sandstone lithofacies in drill-hole NB-97-04. They average 40cm thick with well sorted laminae, which can be either normal or reverse graded. These units are commonly found present interlayered with parallel laminated shale and lensoid, fine-grained sandstone. Rare cross-stratified, medium- and fine-grained sandstones, some capped with ripple laminae, are also present.
Poorly Organized Sandstone Lithofacies Association
This lithofacies association forms the upper most assemblage in all three drill-holes (Figure 2) and is overlain by aeolian sandstone of the Nipigon Bay Formation. It is not dominated by any type of lithology but constitutes a mixture of units with differing grainsize and sedimentary structures. Overall, mud rip-ups and dewatering structures and sand injections are very common within this lithofacies assemblage.
Wispy, silt-rich, fine-grained sandstones are the most frequently occurring unit (Table 3). The layers lack a well-organized internal structure with the ripple lenses deformed by water escape. Lensoid sandstones have better defined internal layering, consisting of approximately 1cm thick, ripple lenses of very fine- and fine-grained sand in silt. They are abundant but do not occur in large continuous units, but rather, are isolated pods or lenses surrounded by generally parallel laminated or wispy silt-rich shale. Graded, parallel laminated sandstone, siltstone and shale are more common in drill-hole NB-97-04. They are interlayered with wispy, silt-rich, fine-grained sandstone, and wave rippled siltstone and fine-grained sandstone.
The coarsest units in this lithofacies association are cross-stratified and massive sandstones with mixtures of fine-to medium-grained sand and, very rarely, coarse sand. Some of these 20–30 cm thick massive sandstones are graded with increasing clay content near their tops (Figure 7) and development of protosols in their upper few centimeters (Fralick and Zaniewski, 2012). They are more common in drill-holes NB-97-02 and NB-97-05.
Sedimentology: Depositional System
An expansive literature on deltaic deposits, both ancient and modern, exists and it is beyond the scope of this paper to review it all for comparison purposes with this deltaic assemblage. Therefore, most of this discussion centers on the similarities and differences the Sibley Delta has with a modern example; the Mississippi Delta. The Mississippi was chosen because: 1) it is well vegetated and is located in a humid, sub-tropical area (Coleman and Wright, 1975; Coleman et al., 1998) compared with a humid near equatorial location for the Sibley Delta (Robertson, 1973); 2) it is comparable in size to the Sibley delta, with large progradational parasequences, due to major lobe switching, 50–150 m thick for the Mississippi (Fisk et al., 1954; Coleman and Wright, 1975) and 100–150 m thick for the Sibley; 3) it has comparably sized small-scale parasequences, produced by crevasse deltas formed in interdistributary bays, 3–16 m thick for the Mississippi (Elliot, 1974; Coleman and Wright, 1975) and 10 m thick for the Sibley; 4) and a large volume of research has been published on the Mississippi, including pictures of core (Coleman and Prior, 1980, 1982) that can be used for comparison.
Rogala et al. (2007) recognized that the strata in the Kama Hill and Outan Island Formations were deposited in a deltaic environment and divided it into a prodelta, distal bar, distributary-mouth bar and floodplain-channel assemblage. They recognized slump block conglomerates (c.f. Klein et al., 1972; Coleman and Prior, 1980) and slump scars filled with slide blocks (c.f. Nemec and Steel, 1988; Fralick and Miall, 1989); the presence of wave ripples and hummocky cross-stratification indicating wave modification; and low-angle, cross-stratified sandstones capping the distributary-mouth bar, similar to beach deposits capping modern Mississippi delta sand bars (Coleman and Prior, 1980). Thus, a framework has already been laid for further discussion.
Prodelta
Prodelta deposits form the furthest offshore deltaic sediments. They are deposited by rainout from suspension in inter- and over-flows and reflect the sediment and water supply of the source river and its fluctuations through time (Reading and Collinson, 1996). This is manifested in the sediment record by sedimentation from rainout dominating over layers with evidence of deposition from traction currents (Table 1 and Figure 2). Coleman and Prior (1982) observed parallel lamination in alternating finely-graded silts and clays in prodelta sediments of the Mississippi, which were highlighted by slight colour changes caused by varying content of organic matter.
The Fine-Grained L. A. forms the Sibley prodelta up to where it transitions into distal bar deposits (shale/siltstone on Figure 2). The main lithofacies within this association are: 1) massive siltstone; 2) parallel laminated siltstone; 3) parallel laminated shale; 4) sporadic, parallel laminated and ripple laminated, very fine-to fine-grained sandstone; 5) lensey or wispy siltstone and sandstone (Figure 4). These fine-grained lithofacies (Table 1) are present in other pre-vegetation prodeltas, such as the Mesoproterozoic Açurua Formation in present day Brazil, which is dominated by: 1) massive, wavy, and parallel laminated shale with siltstone and fine-grained sandstone; 2) massive or 3) parallel laminated, fine- to coarse-grained sandstone; and very fine- to fine-grained, rippled sandstone (Magalhaes et al., 2015).
Layers of ripple laminated sandstone probably represent larger than average flood events in the fluvial system that allowed the hyperpycnal underflow to extend into the prodelta area. Alternatively some may be the result of geostrophic flow below storm wave base transporting sand from the distributary-mouth bar onto the prodelta (e.g. Piper and Panagos, 1981).
Compared to the numerous slump deposits present on the Mississippi prodelta (Coleman and Prior, 1982; Lindsay et al., 1984), possibly reflecting a higher slope, the Sibley prodelta has far fewer slumps, though they are present (Figure 4H, I) (Rogala et al., 2007). In addition numerous smaller post-depositional modifications, such as fluid escape structures and sediment loading are present (Figure 4G). The former was also noted in the Mississippi prodelta sediments and attributed to excess pore fluid pressures (Coleman and Prior, 1982).
The deposits formed by physical sedimentation processes are similar in the Sibley and Mississippi prodelta assemblages. The main differences relate to the low abundance of organic matter in the Sibley deposits. Bioturbation is non-existent, providing a better record of sedimentary structures than in cores of Mississippi sediment (Coleman and Prior, 1982). Without organic debris mixed with the Sibley sediment low reduction potential Eh levels could not develop. The organic-rich, black to dark brown clays of the Mississippi prodelta contrast with the red, oxidised clays and siltstones of the Sibley Delta. Only scattered reduction spots record the presence of inconsequential amounts of organic carbon; further evidence for limited mid-Proterozoic primary productivity (Crockford et al., 2018).
Distal Bar
The transition from the distal bar to the prodelta occurs where the sediment plume exiting the distributary mouth during an average flood event deposits enough sediment to lower its density and become buoyant. Shoreward of this zone ripple laminated, coarse silt and fine-grained sand will record the importance of hyperpycnal driven underflows in the distal bar (Coleman and Gagliano, 1965; Fisher et al., 1969; Wright and Coleman, 1974).
Distal bar deposits are found on the seaward sloping margin of the distributary-mouth bar, and overlie the pro-delta sequence vertically and landward (Coleman and Prior, 1982). They coarsen from the pro-delta, with an increased concentration of ripple laminated silts and sands (Coleman and Prior, 1982). The lower portion of the Sibley Delta distal bar is dominated by the Silt-Rich L.A. (Figure 2 and Table 1) in the Lower Coarsening Upward Succession and the Silty Sandstone L.A. (Table 2) in the Middle Coarsening Upward Succession. The upper strata of the distal bar are dominantly current ripple laminated, very fine-grained sandstone (Figure 5E, F) of the Ripple Laminated Sandstone L.A. (Table 1) in the Lower Coarsening Upward Succession and the Fine-Grained Sandstone L.A. (Table 2) in the Middle Coarsening Upward Succession. Hummocky cross-stratified fine-grained sandstones are present but not abundant (Figure 7D).
These assemblages are similar to distal bars of the Mississippi, which, in their lower areas, are composed of parallel laminated silts and clays with numerous thin graded and ripple laminated sand and silt layers (Coleman and Prior, 1980; Coleman and Prior, 1982). Small burrows cause some bioturbation and shell fragments are common. Higher in the Mississippi distal bar deposits of a variety of structures associated with both oscillatory and unidirectional currents become much more common (Coleman and Prior 1982). These consist of ripple lamination, starved current ripples, small scour and fill structures and graded sand layers (Coleman and Prior, 1982). The Sibley distal bar deposits are similar to this and also similar to the distal bars in the lower Naeringselva Member of the Båsnaering Formation, which is dominated by: 1) parallel laminated mudstone; 2) current-rippled siltstone; 3) structure-less mudstone; and 4) wave-rippled, fine-grained sandstone (Pickering, 1984). In addition it is similar to the pro-delta to distal delta-front facies in the Aҫurua Formation (Magalhaes et al., 2015).
Mud flasers, both wavy flaser bedding and bifurcated wavy flaser bedding (definition of Reineck and Wunderlich, 1968), and sub-millimeter clay drapes are very common in the ripple laminated, coarse-grained siltstones and fine-grained sandstones (Figures 5, 7D-F). Though flaser bedding is commonly associated with tidal currents (Reineck and Wunderlich, 1968; Terwindt and Breusers, 1972; Reineck and Singh, 1973) it has also been described in ephemeral fluvial channel deposits (Martin, 2000) and perennial point bar deposits (Bhattacharya, 1997). More significantly, ripple lamination in offshore deposits of the Mississippi Delta commonly have very similar flaser bedding, lenticular bedding and thin clay drapes developed (Coleman and Prior, 1982). The only significant difference is that the Mississippi cores have organics mixed with the clay flasers and laminae, and are reduced, whereas those in the Sibley Delta are oxidized. Although, aside from the effects of biota, the lamination types in the prodelta and distal bar are very similar to those of the Mississippi Delta, a conspicuous difference exists. Small, red mud chips are present in the sediment of the Sibley Delta. This is similar to the Sibley floodplain deposits (Fralick and Zaniewski, 2012, Figure 6; Ielpi et al., 2018; Figures 8I,J) where the lack of vegetation caused increased drying conditions on the uppermost layer of sediment generating large quantities of small mud chips. Probably horton overland flow and floodplain inundation and drainage during overbank flow also delivered these intraformational mud chips to the distributary channels, which flushed them offshore.
Distributary-Mouth Bar
Distributary mouth bars are areas of shoaling associated with the seaward terminus of a distributary channel, and result from a decrease in velocity and the subsequent reduction in carrying capacity of the river as it leaves its confined channel and enters the open-body of water, resulting in rapid deposition of sediment (Coleman and Prior, 1982; Reading and Collinson, 1996). Due to the shallow nature of the overlying water they are subject to extensive wave reworking.
The Fine-Grained Sandstone L.A. marks the first distributary mouth bar deposit in the Outan Island Formation. It contains rippled, lensoid, wispy, and parallel laminated, fine-grained sandstone, and parallel laminated shale. Ripple laminations, convolute bedding, probably caused by slumping, and rarer cross-stratification, some of which is hummocky, plus thick reverse and normal graded, massive, medium-grained sandstones are present higher in the distributary-mouth bars. The upper half of the distributary-mouth bars are dominated by the Medium-Grained Sandstone L.A. Cross-stratified, massive and parallel laminated, medium-grained sandstone gains importance here. Finer grained lithologies are present as lensoid and wispy ripple laminated medium-grained sandstone, massive fine-grained sandstone and parallel laminated shale. Superimposed on this overall trend progradational parasequences, averaging 6.5 m thick, are present.
This contrasts with the distributary-mouth bars of the Mississippi Delta. They are dominated by fine-grained sandstone with ripple laminae and contorted laminae interlayered with silt and silty-clay. Near the bar tops small-scale cross-stratification becomes more pronounced (Coleman and Prior, 1982). The Sibley distributary-mouth bars have, on average, coarser sands and bedforms characteristic of higher flow velocities. This results in a steeper energy gradient between the distributary-mouth bar and the distal bar. To facilitate delivery of coarser sediment the Sibley fluvial system required either a higher slope or was prone to more variable and higher discharge events. The greater heterogeneity of layer thicknesses, grainsizes and sedimentary structures in the Sibley Delta indicates the latter was the control (e.g. Fielding et al., 2018). Especially the presence of massive, fine- and medium-grained sandstone layers, up to 355 cm thick, is compatible with high-discharge events containing large sediment loads. These layers can be normal or reverse graded and where normal graded commonly progress from massive to parallel laminated to cross-stratified to rippled, or a portion of this sequence. Others are non-graded, and some are poorly sorted. The graded layers with Bouma sequences (Bouma, 1962) are classic turbidites and the other variations can be interpreted using that as a base. Massive, non-graded sands have been interpreted as either freezing of sandy debris flows or continuous deposition by a high density turbidity current with a basal layer of hindered settling due to grainflow or liquefied/fluidized processes (Stow et al., 1994). The reverse graded examples were probably transported in part by, and deposited under the influence of, dispersive pressure in a highly concentrated flow (Lowe, 1982). The normally graded massive sandstones are typical A division turbidites (Bouma, 1962; S3; Lowe, 1982) deposited due to an unsupported collapsing dispersion (Allen, 1991) and a high sedimentation rate (Walton, 1967). Additionally, researchers have defined four differing processes that can occur in a high-concentration, basal flow layer which lead to deposition of a massive bed. These are deposition from a basal: traction carpet (Dzulynski and Sanders, 1962; Hiscott, 1994; Sohn, 1997; and; Cartigny et al., 2013); layer of laminar flow (Middleton and Hampton, 1976; Postma et al., 1988); collapsing laminar sheared layer (Vrolijk and Southard, 1997); and quick bed (Middleton, 1967; Parkash and Middleton, 1970). In addition a low concentration, sheet flow basal layer can also lead to deposition of massive sand (Hernandez-Moreira et al., 2020). These processes can be active during resedimentation of slumped material. In addition to slump generated currents hyperpycnal flows originating during flood events in fluvial systems and flowing in sub-aqueous channels down the delta front into deep water have been described as depositing massive layers (e.g. alpine lakes: Sturm and Matter, 1978; Lambert and Giovanoli, 1988). More applicable examples come from fan deltas (Massari, 1984; Dabrio and Polo, 1988; Massari and Parea, 1990; Nemec, 1990), and in particular fjords of British Columbia (Kostaschuk, 1985; Parea et al., 1987; Bornhold and Prior, 1990; Zeng et al., 1991; Bornhold et al., 1994). There, turbidity currents have been recorded travelling down sub-aqueous channels from the delta top. These deposited successions of massive, meter-scale, fine and medium-grained sands (Zeng et al., 1991). They were probably generated as highly concentrated underflows (Prior et al., 1987). This work was expanded on by Bornhold et al. (1994) who discovered that sediment that built up during low flow conditions in fluvial channels and distributary-mouth bars was swept onto the steep delta slope and down sub-aqueous channels during floods. These systems transported the sediment into deep water settings. The Sibley Delta thick, massive sandstones are most prominent in the proximal distributary-mouth bar sediments, a low slope area of shallow water. However, though the mechanism supplying energy to the flow must be different the process may be similar. Alluvial fans present on the east side of Laguna de Bustillos in the foothills of the Sierra Madre of northwest Mexico have small aeolian dunes migrating across the surface that fall into dry stream channels during the dry season (personal observation, PWF). During the beginning of the rainy season mid-afternoon downpours remobilize the sand plugs as slurry-like flows down the channels and deposit the sand as sheets on extensive sandflats dipping at extremely low angles into the lake. A similar process, initiated by large floods, possibly remobilized sand, which had accumulated in Sibley fluvial channels during low, or no, flow events could have produced the massive sandstone beds up to 355 cm thick (e.g. Martin and Turner, 1998; Muhlbauer and Fedo, 2020). The rapid sedimentation from suspension would not allow any reworking into laminations (Walton, 1967; Allen, 1991).
A lack of macrophytic biota are more noticeable in this sub-environment. Normally near the top of distributary-mouth bar deposits there is an increase in organic matter from the floodplain and river which form “coffee grind deposits” (Coleman and Prior, 1982). Also large pieces of organic matter concentrate on the channel-proximal distributary-mouth bar as this is the shallowest portion of the distributary-bar area. However these deposits are not present in the Sibley distributary mouth bar because there was limited organic matter to deposit.
Interdistributary Bays
Distributary-mouth-bar deposits are generally overlain by either interdistributary bay or distributary channel deposits (Coleman and Gagliano 1964, 1965; Coleman and Wright, 1975; Coleman and Prior, 1982). Modern interdistributary bay deposits include areas of open water within the active delta which may be completely surrounded by marsh or distributary levees, and partially open to the sea. Interdistributary bay deposits of the Mississippi Delta consist of 3–16 m thick, stacked, asymmetric, coarsening- and thickening upwards cycles (Coleman and Gagliano, 1964; Coleman and Gagliano, 1965; Coleman and Wright, 1975; Coleman and Prior, 1982). These progress upwards from parallel laminated, organic-rich, silts and silty clays to sandy stringers intercalated with the latter, to thin sand layers which are graded or rippled, to cross-stratified sands interlayered with ripple laminated silty sand, and finally emergent organic-rich marsh deposits (Coleman and Prior, 1982). The 10 m thick asymmetric, coarsening- and thickening-upwards cycles overlying the distributary-mouth bar deposits in drill-hole NB-97-04 (Figure 9) are very similar to these interdistributary bay deposits. Sediment in the lower area of the lower parasequence depicted in Figure 9 belong to the Silt-Rich L.A. with predominantly clay-rich siltstone and shale interlayered on the millimeter-to centimeter-scale. Ripple lamination becomes more prevalent upwards, especially in layers of fine-grained sandstone, which also gain in importance upwards. The upper parasequence begins with wispy layered, very fine-grained sandstone and progresses upward to cross-stratified, fine-grained sandstone. Combined they form a progradational parasequence set and probably represent progressive cyclic infilling of the interdistributary bay. The lack of terrestrial macrophyte debris in the Sibley interdistributary bay deposits is the only feature that distinguishes them from those of the Mississippi.
Delta Top
Following a rapid decrease in grainsize a coarsening-upward trend exists above the top of the distributary-mouth bar sediments of the upper coarsening and thickening-upward assemblage (Figure 2). This gives the appearance of another distal bar to distributary mouth bar cycle, especially as the upper coarse-grained interval in NB-97-04 and NB-97-05 both contain a layer of low-angle, cross-stratified, fine-grained sandstone, which may represent an interdistributary beach at the top of the bar. This contrasts with NB-97-02, which contains a similar coarsening upward succession but it climaxes at its top with an 8.5 m thick fining upward succession with an extraformational conglomerate at its base. The 20 m thick succession below it and separating it from the top of the distributary-mouth bar contains desiccation cracks. Thus, here it is probable that the bar is overlain by floodplain that is incised by a fluvial channel. Numerical modelling by Almeida et al. (2016) predicted that such pre-vegetation channels formed in low slope environments (e.g. delta tops) would have more bank cohesion, be deeper, and possibly meandering, compared to sheet-braid channels common in higher slope fluvial systems without macrophytes. The channel system on the Sibley delta top conforms to this prediction. None of the distributary-mouth bar deposits have fluvial channel sediments erosively cuting into them, therefore making it likely that the drill-holes went through an area away from the main channel. NB-97-02 was closest to that channel and as the channel built out past the bar overbank flow deposited floodplain sediments on the bar top. NB-97-05 and NB-97-04 were laterally further away from the river mouth so the sediment bypass resulted in finer grained sediments being deposited on the bar top there until overbank flow, and in the case of drill-hole NB-97-04 probably crevasse splays, built sub-aerial conditions out into those areas. However, the location of drill-hole NB-97-04 was distant enough from the main channel that restricted sedimentation resulted in its reflooding and developing a series of interdistributary bay fill cycles, with the prograding parasequence sets averaging 10 m thick. Sediment deposited on the sub-aerial delta top is described and interpreted further in Ielpi et al. (2018) and Fralick and Zaniewski (2012).
The succession of sediments overlying the distributary-mouth bar contrasts more than other Sibley sub-environments with the Mississippi Delta. The organic-rich costal swamps, marshes and wet-land sediments of the latter are absent, with profound effects on the nearshore depositional systems of the former. The highstand surfaces at the top of the bay-fill parasequences, lacking organics, are more condensed. The sub-aerial sediments overlying the distributary-mouth bar are quite heterolithic and vertically variable, lacking the stabilizing effects of vegetation. For further discussion of these and other effects see Fralick and Zaniewski (2012) and Ielpi et al. (2018).
Sequence Stratigraphy
Sequence stratigraphic nomenclature of Coe and Church, (2005) is used here. For a further discussion of terminology see: Miall (1997), Posamentier and Allen (1999), and Catuneanu (2006).
The effects of sequence stratigraphy are most visible in the coastal to shallow marine realm (Coe and Church, 2005). This is reflected in the Kama Hill – Outan Island delta system, which formed on cratonic crust covered by an eperic sea or by an extremely large lacustrine area inland from the cratonic edge. The delta comprises a large-scale progradational system with offshore, fine-grained sediment at its base and various sub-aerial environments at its top, which were overlain by aeolian dune fields. The entire deltaic assemblage is the thickest member of a hierarchy of asymmetric stratigraphic cycles.
Parasequences form the smallest asymmetrical stratigraphic cycles. The lower and middle portions of the first coarsening- and thickening-upward succession are organized into 5–30 m thick parasequences that coarsen upwards from shale to fine-grained sandstone (Figures 3, 4A). They are generally correlatable between the drill-holes. These are caused by either: an increase in sediment delivery to the area, or a fall in relative sea-level. As repositioning of distributary sediment feeder systems, either by rapid avulsion or incremental channel switching is a common attribute of deltaic systems, it is likely that channel repositioning to a location better able to deliver sediment to this offshore area was the cause of the initiation of parasequences in this system. Individual parasequences were generally ended abruptly because of a decrease in sediment supply and development of a flooding surface; though that nomenclature is misleading as this portion of the delta is all sub-aqueous. This can be explained by the channel moving away from the area. Most of these parasequences form a progradational parasequences set, which builds up to the distributary-mouth bar at the top of the lower coarsening- and thickening-upward succession.
The final progradational parasequence of the set is overlain by a fining upward, retrogradational parasequence (central area of Figure 6). This represents an increase in accommodation space and/or a phased-in decrease in sediment delivery. A step-wise avulsion through time of the feeder distributary channel would result in the offshore retrogradational parasequences as progressively more sediment exited during floods through a levee breach. The lower coarsening- and thickening-upward succession is abruptly overlain by a flooding surface and the beginning of the middle coarsening- and thickening-upward succession.
The Middle Coarsening-Upward Succession is composed of a large-scale progradational parasequence set of two thick parasequences. The lower one is thicker in the northeast and the upper one is thicker in the southwest. This gives the appearance of two overlapping depositional lobes forming the Middle Coarsening-Upward Succession. These are further composed of thinner parasequences averaging 10 m thick. These progradational parasequences end abruptly with the coarser and thicker sandstones of the distributary-mouth bars, which do not exhibit parasequence development (Figure 8). Heterogeneous delta top deposits overlie the distributary-mouth bar, with uncommon, thinning- and fining-upward channel sandstones (e.g. Figure 3, 75–83 meters, of Fralick and Zaniewski, 2012) forming the only well structured, meter-scale zones in drill-holes NB-97-2 and NB-97-5 (Figure 9). Their retrogradational appearance probably represents the progressive diversion of water and sediment away from that distributary channel through a deepening levee breach into another developing distributary channel. The interdistributary bay deposits of drill-hole NB-97-4 contain a progradational parasequence set composed of 2, 10 m thick parasequences (Figure 9). As described earlier, these represent infilling events, probably the result of progressive levee breaching providing sediment access to, and eventually filling, an interdistributary bay. These would be matched by upward fining (retrogradational) parasequences in the proximal distributary mouth bar, e.g. similar to those at the top of the distributary mouth bar in Figure 6. In this scenario the flooding surface separating the interdistributary bay coarsening- and thickening-upwards parasequences would have been produced by deposition self-sealing the levee break and sediment starvation plus subsidence causing reflooding of the area. Another progressive levee break would produce the overlying progradational parasequence.
Putting this deltaic succession into a larger sequence stratigraphic context is difficult. In deltaic settings changing sediment supply is an important factor in determining progradational and retrogradational assemblages. If a relatively constant subsidence rate and eustatic sealevel are assumed all of the depositional cycles present in the core studied here can be explained by changes in sediment supply rate; i.e. autocyclic changes that are common in deltaic environments. Coe and Church (2005) note that in systems with very high sediment supply accommodation space will be overwhelmed with detritus even during intervals of sea-level rise leading to aggradational and retrogradational parasequences not being able to develop. Without these a very large amount of stratigraphic control is necessary to identify complete sequence stratigraphic cycles.
Fitting sequence stratigraphic nomenclature to the progradational successions that are present on Figure 2 highlights the problems. The Lower Coarsening-Upwards Succession has the characteristics of a highstand systems tract where decreasing accommodation space leads to parasequence progradation (Figure 3). Though, regressions caused by sediment supply being greater than subsidence rate with a constant sea-level do not easily fit into sequence stratigraphic nomenclature, which is based on an absolute sea-level change. This is highlighted by what happened next in the stratigraphy. The sequence boundary overlies the highstand systems tract and is denoted by the development of retrogradational parasequences (Figure 6). In modern deltas this transition is due to lobe abandonment by the fluvial system and wave reworking of the now sediment starved area (Penland et al., 1988). Next the falling stage systems tract should be present, except it cannot develop if a sea-level drop does not cause a forced regression (Coe and Church, 2005). The lowstand systems tract also cannot develop as sediment starvation combined with subsidence induced flooding cause’s transgression rather than regression. This results in the sequence boundary, transgressive surface and maximum flooding surface all occurring in the uppermost distributary mouth bar (Figure 6) and adjacent area of fine-grained sediment above it. The transgressive systems tract comes next, but instead of reflecting drowning of the delta, accommodation space is only created in the disused lobe while progradation and a highstand systems tract operates in the newly formed lobe.
The main problem is that in sequence stratigraphy changes in depositional style is conceptualized as driven by changes in absolute sea-level. This is commonly thought of as following a sine curve progressing from highstand systems tract, ending with a sequence boundary at the top of the curve and then continuing through falling stage systems tract, lowstand systems tract and transgressive systems tract up to highstand systems tract again. However, in deltaic systems where the primary variable is rate of sediment supply, rather than sea-level change, as a lobe progrades and an area becomes closer to the river mouth sediment supply increases. This continues until channel avulsion causes an abrupt decrease in sediment supply leading to subsidence rate gaining importance and increasing accommodation space. Thus, a condensed sequence develops until channel avulsion moves a river mouth into a position where it can deliver sediment to the area and start the development of progradational parasequences again. In this type of system the falling stage to highstand transgressive system tract nomenclature is at best misleading and at worst inapplicable.
Conclusions
The large-scale structure of the Mesoproterozoic Sibley Delta consists of a lower, 150 m thick, coarsening- and thickening-upward succession, an upper, 100 m thick coarsening- and thickening-upward succession, both representing delta lobe progradation, overlain by an interdistributary bay – floodplain – channel assemblage (Figure 10). Delta lobe progradation and abandonment was probably controlled by sediment supply from a distributary channel resulting in decreased accommodation space culminating in channel extension and leading to avulsion restricting sediment supply to the delta lobe. Subsidence, low sediment supply and increasing accommodation space produced a condensed sequence until further distributary channel avulsion increased sediment supply causing the second lobe to prograde. Internally the lobes are composed of progradational parasequence sets that decrease in thickness upward through the lobe, averaging 20 m in the prodelta 10 m in the lower distributary-mouth bars and only 6.5 m near bar tops. This may be the result of decreased accommodation space causing increased erosive removal or bypass of sediment.
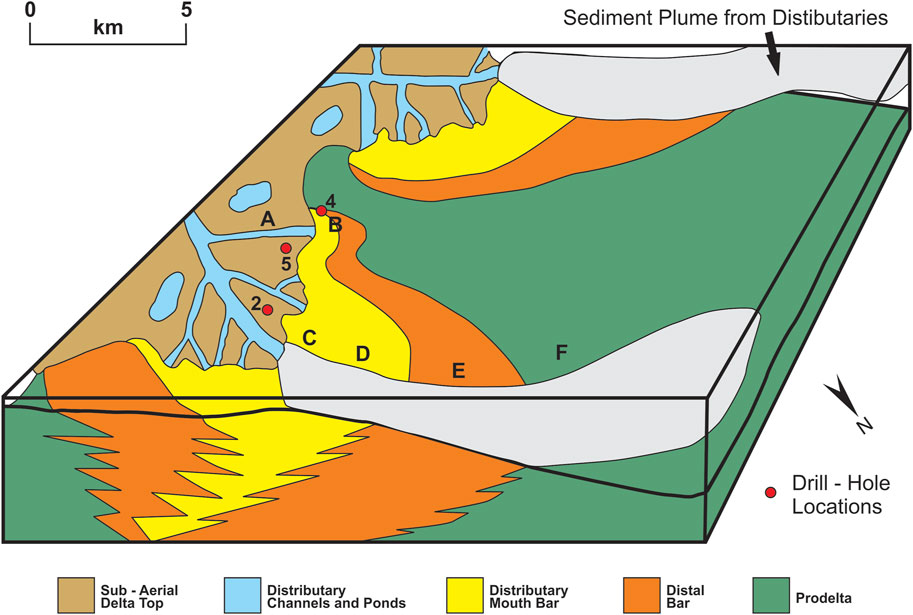
FIGURE 10. Schematic block diagram depiction of the sedimentary system responsible for the deltaic deposits. The positions of NB-97-02, NB-97-04 and NB-97-05 are where they would be at the end of deposition of the deltaic system. The side branches off the main channel represent levee failures supplying sediment to the distributary-mouth bar (drill-hole NB-97-05) and interdistributary bays (B; drill-hole NB-97-04). The proximal distributary-mouth bar (C; drill-hole NB-97-02) is dominated by medium- and coarse-grained, massive sandstone and cross-stratified medium-grained sandstone, whereas further offshore on the distributary-mouth bar (D) fine-grained sandstones become more numerous. Hyperpycnal flows (underflows) dominate sedimentation on the distal bar (E), whereas hypopycnal flows (overflows) dominate the prodelta (F).
The scale of the delta is similar to the modern, highly vegetated, Mississippi Delta system and therefore it was used for comparison. The prodelta of the Sibley Delta is mostly composed of graded and/or parallel laminated, thin layers of siltstone and shale deposited from suspension in hypopycnal flows during intense discharge events. Closer to the river mouth most flows retained enough sediment to be hyperpycnal and deposited very fine-grained sand with current ripples forming the distal bar. Wave reworking, and the production of oscillatory ripples, was also common in this environment. The grainsizes, layer thicknesses and sedimentary structures in both of these deltaic sub-environments are similar to those in the modern Mississippi Delta. The main differences are the lack of infauna causing bioturbation of the fine-grained layers and the relative scarcity of organic matter in general limiting the content of reductants in the sediment and leading to the highly oxidized sediment delivered from the fluvial system not being reduced.
The distributary-mouth bars in the Sibley Delta are somewhat coarser grained and contain a larger amount of higher velocity bedforms than those of the Mississippi. However, the most conspicuous difference is the presence of thick massive fine-and medium-grained sandstone beds in the Sibley bars, especially near the bar top, proximal to the distributary mouth. These represent mass-flow deposits with the sediment kept in suspension by turbulence and to a lesser degree dispersive pressure. They may have formed from a two-step process where 1) sand accumulated in the distributary channels during low or no flow periods due to the slow current causing the channel to partially infill, or aeolian sand dunes migrating over the floodplain cascaded into the channel. 2) During a major flood the water surging down the channel entrained a large amount of the sand creating a slurry flow which exited the distributary mouth and rapidly decelerated depositing a thick, massive bed.
As the distributary channel extended into the basin it did not cut through the distributary-mouth bar in any areas later penetrated by the drill-holes. NB-97-02 received the largest amount of medium- and coarse-grained sand, indicating the distributary-mouth bar there was closest to the river mouth. The distributary-mouth bar in drill-hole NB-97-04 is overlain by a series of progradational parasequences similar in thickness and location to interdistributary bay deposits of the Mississippi system (Coleman and Gagliano, 1964; Coleman and Gagliano, 1965; Coleman and Wright, 1975; Coleman and Prior, 1982). Individually they record the progressive change from deposition of fine rainout deposits, mostly sourced from floods overtopping the levees, to entry into the bay of sands sourced from a deepening levee break. As the sandy fan built upward it would have self-sealed the levee, diminishing the sediment supply to the crevasse fan, and ongoing subsidence and erosion would have progressively drowned it.
With some important exceptions, the sub-aqueous portion of this delta is comparable to the Mississippi system. From this it can be inferred that the sub-aerial fluvial system likely shared similarities with lower width to depth ratio rivers rather than typical braided systems. This is consistent with the dominance of floodplain sediments on the Sibley Delta top with only one 8.5 m deep channel present in the 170 m of core through this sub-aerial environment. This agrees with numeric modelling that predicted low slope pre-vegetation fluvial channels would be deeper and possibly meandering compared to higher slope sheet-braid channel systems (Almeida et al., 2016). Thus, this delta system has substantial differences with most of the non-vegetated fan and braid deltas described in the majority of literature listed in the Introduction. Though the type of non-vegetated delta represented by the Sibley Delta may have been rare prior to the Devonian Period, given the correct circumstances it, obviously, could develop.
Data Availability Statement
The original contributions presented in the study are included in the article/Supplementary Material, further inquiries can be directed to the corresponding author.
Author Contributions
PF planned the project, helped log core and wrote the manuscript. RJ logged core and wrote her thesis on the subject. RM conducted background research on delta systems.
Funding
Natural Sciences and Engineering Research Council of Canada Discovery Grant to PF.
Conflict of Interest
The authors declare that the research was conducted in the absence of any commercial or financial relationships that could be construed as a potential conflict of interest.
Publisher’s Note
All claims expressed in this article are solely those of the authors and do not necessarily represent those of their affiliated organizations, or those of the publisher, the editors and the reviewers. Any product that may be evaluated in this article, or claim that may be made by its manufacturer, is not guaranteed or endorsed by the publisher.
Acknowledgments
Appreciation is expressed to Sophie Kurucz and Matthew Svensson who helped log the core and to Jason Heroux for drafting the figures. Appreciation is extended to the three reviewers whose recommendations greatly improved the manuscript. The research was supported by a Natural Sciences and Engineering Research Council of Canada Discovery grant to PF.
References
Allen, J. R. L. (1991). The Bouma Division A and the Possible Duration of Turbidity Currents. J. Sediment. Petrology. 61, 291–295. doi:10.1306/d42676f4-2b26-11d7-8648000102c1865d
Baars, C., Hefin Jones, T., and Edwards, D. (2008). Microcosm Studies of the Role of Land Plants in Evaluating Soil Carbon Dioxide and Chemical Weathering. Glob. Biogeochem. Cycles. 22, 1–9. doi:10.1029/2008gb003228
Bayet-Goll, A., and de Carvalho, C. N. (2020). Architectural Evolution of a Mixed-Influenced Deltaic Succession: Lower-To-Middle Ordovician Armorican Quartzite in the Southwest Central Iberian Zone, Penha Garcia Formation (Portugal). Int. J. Earth Sci. (Geol Rundsch). 109, 2495–2526. doi:10.1007/s00531-020-01915-8
Bayet-Goll, A., and Neto de Carvalho, C. (2016). Ichnology and Sedimentology of a Tide-Influenced delta in the Ordovician from the Northeastern Alborz Range of Iran (Kopet Dagh Region). Lethaia. 49, 327–350. doi:10.1111/let.12150
Bennett, P., and Siegel, D. I. (1987). Increased Solubility of Quartz in Water Due to Complexing by Organic Compounds. Nature. 326, 684–686. doi:10.1038/326684a0
Bhattacharya, A. (1997). On the Origin of Non-tidal Flaser Bedding in point Bar Deposits of the River Ajay, Bihar and west Bengal. NE India. Sedimentology. 44, 973–975. doi:10.1111/(ISSN)1365-3
Bhattacharya, H., and Mahapatra, S. (2008). Evolution of the Proterozoic Rift Margin Sediments-North Singhbhum Mobile Belt, Jharkhand-Orissa, India. Precambrian Res. 162, 302–316. doi:10.1016/j.precamres.2007.07.021
Bornhold, B., and Prior, D. B., (1990). Morphology and Sedimentary Processes on the Subaqueous Noeick River delta, British Colombia, Cadada. Editor A. Colella, and D. B. Prior) Coerse-Grained Deltas. International Association of Sedimentologists, Special Publication 10, 169–181.
Bornhold, B., Ren, P., and Prior, D. B. (1994). High Frequency Turbidity Currents in British Columbia Fjords. Geo-Marine Lett. 14, 138–243. doi:10.1007/bf01274059
Bouma, A. H. (1962). Sedimentology of Some Flysch Deposits: A Graphic Approach to Facies Interpretation. Amsterdam: Elsevier, 168.
Boyd, R., Dalrymple, R., and Zaitlin, B. A. (1992). Classification of Clastic Coastal Depositional Environments. Sediment. Geology. 80, 139–150. doi:10.1016/0037-0738(92)90037-r
Cartigny, M. J. B., Eggenhuisen, J. T., Hansen, E., and Postma, G. (2013). Concentration-dependent Flow Stratification in Experimental High-Density Turbidity Currents and Their Relevance to Turbidite Facies Models. J. Sediment. Res. 83, 1047–1065. doi:10.2110/jsr.2013.71
Chakraborty, T., and Sensarma, S. (2008). Shallow marine and Coastal Eolian Quartz Arenites in the Neoarchean-Palaeoproterozoic Karutola Formation, Dongargarh Volcano-Sedimentary Succession, central India. Precambrian Res. 162, 284–301. doi:10.1016/j.precamres.2007.07.024
Cheadle, B. A. (1986a). Alluvial-playa Sedimentation in the Lower Keweenawan Sibley Group, Thunder Bay District, Ontario. Can. J. Earth Sci. 23, 527–542. doi:10.1139/e86-053
Cheadle, B. (1986b). Stratigraphy and Sedimentation of the Middle Proterozoic Sibley Group, Thunder Bay District, Ontario. London, Ont: Ph.D. thesis, The University of Western Ontario.
Coe, A. L., and Church, K. D. (2005). Sequence Stratigraphy and Sea-Level Change. In The Sedimentary Record of Sea-Level Change. Editor A. Coe. The Open University, Cambridge University Press, 57–134.
Coleman, J. M., and Gagliano, S. M. (1964). Cyclic Sedimentation in the Mississippi River Deltaic plain. Trans. Gulf Coast Associations Geologic Societies. 14, 67–80.
Coleman, J. M., and Gagliano, S. M., (1965). Sedimentary Structures: Mississippi River Deltaic plain. In Sediment. Structures their Hydrodynamic Interpretation. Ed. G. V. Middleton. Society of Economic Paleontology and Mineralogy, Special Publication 12, 133–148.doi:10.2110/pec.65.08.0133
Coleman, J. M., and Prior, D. B. (1980). Deltaic Sand Bodies, 15. Houston: American Association of Petroleum Geologists, Short Course, 171.
Coleman, J. M., and Prior, D. B. (1982). Deltaic Environment of Deposition. In. Sandstone Depositional Environments. Editor P. A. Scholle, and D. R. Spearing. American Association of Petroleum Geologists, Memoir 31, 139–178.
Coleman, J. M., Roberts, H. H., and Stone, G. W. (1998). Mississippi River Delta: An Overview. J. Coastal Res. 14, 698–716.
Coleman, J. M., and Wright, L. D. (1975). Modern River Deltas: Variability of Processes and Sand Bodies. In. Deltas, Models for Exploration. Ed. M. L. Broussard. Houston Geological Society, 99–149.
Corcoran, P. L., Mueller, W. U., and Chown, E. H. (1998). Climatic and Tectonic Influences on Fan Deltas and Wave- to Tide-Controlled Shoreface Deposits: Evidence from the Archaean Keskarrah Formation, Slave Province, Canada. Sediment. Geology. 120, 125–152. doi:10.1016/s0037-0738(98)00030-x
Costa, A. F. d. O., Danderfer, A., and Lana, C. (2018). Stratigraphic and Geochronological Characterization of the Mato Verde Group, Central Espinhaço (Brazil): An Eocalymmian Rifting Record in the Western Domain of the Congo-São Francisco Paleocontinent. J. South Am. Earth Sci. 84, 16–33. doi:10.1016/j.jsames.2018.03.005
Cotter, E. (1978). The Evolution of Fluvial Style with Special Reference to the central Appilation Paleozoic. In. Fluvial Sedimentology. Editor A. D. Miall. Canadian Society of Petroleom Geologists, Memoir 5, 361–384.
Counts, J. W., Rarity, F., Ainsworth, R. B., Amos, K. J., Lane, T., Morón, S., et al. (2016). Sedimentological Interpretation of an Ediacaran delta: Bonney Sandstone, South Australia. Aust. J. Earth Sci. 63, 257–273. doi:10.1080/08120099.2016.1180322
Crockford, P. W., Hayles, J. A., Bao, H., Planavsky, N. J., Bekker, A., Fralick, P. W., et al. (2018). Triple Oxygen Isotope Evidence for Limited Mid-proterozoic Primary Productivity. Nature 559, 613–616. doi:10.1038/s41586-018-0349-y
Dabrio, C. J., and Polo, M. D. (1988). Late Neogene Fan Deltas and Associated Coral Reefs in the Almanzora Basin, Almeria Province, southeastern Spain. Editor W. Nemec, and R. J. Steel. Fan Deltas – Sedimentology and Tectonic Settings. Blackie, Glasgow, 354–367.
Dalrymple, R. W., Narbonne, G. M., and Smith, L. (1985). Eolian Action and the Distribution of Cambrian Shales in North America. Geol 13, 607–610. doi:10.1130/0091-7613(1985)13<607:eaatdo>2.0.co;2
Dalrymple, R. W., Zaitlin, B. A., and Boyd, R. (1992). Estuarine Facies Models; Conceptual Basis and Stratigraphic Implications. J. Sediment. Res. 62, 1130–1146. doi:10.1306/d4267a69-2b26-11d7-8648000102c1865d
Davies, N. S., and Gibling, M. R. (2010). Cambrian to Devonian Evolution of Alluvial Systems: the Sedimentological Impact of the Earliest Land Plants. Earth-Science Rev. 98, 171–200. doi:10.1016/j.earscirev.2009.11.002
Davies, N. S., Gibling, M. R., and Rygel, M. C. (2011). Alluvial Facies Evolution during the Palaeozoic Greening of the Continents: Case Studies, Conceptual Models and Modern Analogues. Sedimentology 58, 220–258. doi:10.1111/j.1365-3091.2010.01215.x
de Almeida, R. P., Marconato, A., Freitas, B. T., and Turra, B. B. (2016). The Ancestors of Meandering Rivers. Geology 44, 203–206. doi:10.1130/g37534.1
Dhang, P. C., and Patranabis-Deb, S. (2011). Lithostratigraphy of the Chattisgarh Supergroup Around Singhora-Saraipali Area and its Tectonic Implication. J. Geol. Soc. India 77, 493–515.
Dijk, M., Postma, G., and Kleinhans, M. (2007). “Autogenic Cycles of Sheet and Channelised Flow on Fluvial Fan-Deltas,” in Autogenic Cycles of Sheet and Channelized Flow on Fluvial Fan-Deltas (River: Coastal and Estuarine Morphodynamics), 823–828. doi:10.1201/noe0415453639-c104
Donaldson, J. A., and de Kemp, E. A. (1998). Archaean Quartz Arenites in the Canadian Shield: Examples from the Superior and Churchill Provinces. Sediment. Geology. 120, 153–176. doi:10.1016/s0037-0738(98)00031-1
Drabon, N., Heubeck, C. E., and Lowe, D. R. (2019). Evolution of an Archean Fan delta and its Implications for the Initiation of Uplift and Deformation in the Barberton Greenstone Belt, South Africa. J. Sediment. Res. 89, 849–874. doi:10.2110/jsr.2019.46
Dzulynski, S., and Sanders, J. E. (1962). Current marks on Firm Mud Bottoms. Trans. Conn. Acad. Arts Sci. 42, 5–96.
Elliott, T. (1974). Interdistributary bay Sequences and Their Genesis. Sedimentology 21, 611–622. doi:10.1111/j.1365-3091.1974.tb01793.x
Eriksson, P. G., Condie, K. C., Tirsgaard, H., Mueller, W. U., Altermann, W., Miall, A. D., et al. (1998). Precambrian Clastic Sedimentation Systems. Sediment. Geology. 120, 5–53. doi:10.1016/s0037-0738(98)00026-8
Ethridge, F. G., and Wescott, W. A. (1994). Tactonic Setting, Recognition and Hydrocarbon reserve Potential of Fan-delta Deposits. Editor E. H. Koster, and R. J. Steel. Sedimentology of Gravels and Conglomerates. Canadian Society of Petroleum Geologists, Memoir 10, 217–235.
Fedo, C. M., and Cooper, J. D. (1990). Braided Fluvial to marine Transition: the Basal Lower Cambrian Wood Canyon Formation, Southern Marble Mountains, Mojave Desert, California. J. Sediment. Petrology 60, 220–234. doi:10.1306/212f915b-2b24-11d7-8648000102c1865d
Fielding, C. R., Alexander, J., and Allen, J. P. (2018). The Role of Discharge Variability in the Formation and Preservation of Alluvial Sediment Bodies. Sediment. Geology. 365, 1–20. doi:10.1016/j.sedgeo.2017.12.022
Fisher, W. L., Brown, L. F., Scott, A. J., and McGowen, J. H. (1969). Delta Systems in the Exploration for Oil and Gas. Austin: Bureau of Economic Geology, University of Texas, 78.
Fisk, H. N., Kolb, C. R., McFarlan, E., and Wilbert, L. J. (1954). Sedimentary Framework of the Modern Mississippi delta [Louisiana]. J. Sediment. Res. 24, 76–99. doi:10.1306/d4269661-2b26-11d7-8648000102c1865d
Fralick, P., and Pufahl, P. K. (2006). Iron Formation in Neoarchean Deltaic Successions and the Microbially Mediated Deposition of Transgressive Systems Tracts. J. Sediment. Res. 76, 1057–1066. doi:10.2110/jsr.2006.095
Fralick, P. W., and Miall, A. D. (1989). Sedimentology of the Lower Huronian Supergroup (Early Proterozoic), Elliot Lake Area, Ontario, 63. Canada: Sedimentary Geology, 127–153.
Fralick, P. W., and Zaniewski, K. (2012). Sedimentology of a Wet, Pre-vegetation Floodplain Assemblage. Sedimentology 59, 1030–1049. doi:10.1111/j.1365-3091.2011.01291.x
Franklin, J. M., McIlwaine, W. H., Poulsen, K. H., and Wanless, R. K. (1980). Stratigraphy and Depositional Setting of the Sibley Group, Thunder Bay District, Ontario, Canada. Can. J. Earth Sci. 17, 633–651. doi:10.1139/e80-060
Franklin, J. M. (1970). Metalogeny of the Proterozoic Rocks of Thunder Bay District, Ontario. London, Ontario: Unpublished PhD Thesis, University of Western Ontario, 317.
Fuller, A. O. (1985). A Contribution to the Conceptual Modelling of Pre-Devonian Fluvial Systems. Trans. Geol. Soc. South Africa 88, 189–194.
Gallloway, (1975). Process Framework for Describing the Morphologic and Stratigraphic Evolution of Deltaic Depositionl Systems. Ed. M. L. Broussard. Deltas, Models for Exploration. Houston Geological Society, Houston, Texas, 87–98.
Ghandour, I. M., Tawfik, H. A., Maejima, W., and Abdel-Hameed, A.-M. T. (2013). Sedimentary Facies and Sequence Stratigraphy of the Cambrian Araba Formation, Gebel Somr El-Qaa'a, North Wadi Qena, Egypt. Neues Jahrbuch für Geologie und Paläontologie. 268, 149–174. doi:10.1127/0077-7749/2013/0324
Gouramanis, C., Webb, J. A., and Warren, A. A. (2003). Fluviodeltaic Sedimentology and Ichnology of Part of the Silurian Grampians Group, Western Victoria. Aust. J. Earth Sci. 50, 811–825. doi:10.1111/j.1440-0952.2003.01028.x
Grazhdankin, D. (2004). Late Neoproterozoic Sedimentation in the Timan Foreland. Geol. Soc. Lond. Mem. 30, 37–46. doi:10.1144/gsl.mem.2004.030.01.04
Haddox, C. A., and Dott, R. H. (1990). Cambrian Shoreline Deposits in Northern Michigan. J. Sediment. Petrology 60, 697–716. doi:10.1306/212f9250-2b24-11d7-8648000102c1865d
Hansford, M. R., and Plink-Björklund, P. (2020). River Discharge Variability as the Link between Climate and Fluvial Fan Formation. Geology 48, 952–956. doi:10.1130/g47471.1
Hao, J., Sverjensky, D. A., and Hazen, R. M. (2017). A Model for Late Archean Chemical Weathering and World Average River Water. Earth Planet. Sci. Lett. 457, 191–203. doi:10.1016/j.epsl.2016.10.021
Heaman, L. M., Easton, R. M., Hart, T. R., Hollings, P., MacDonald, C. A., and Smyk, M. (2007). Further Refinement to the Timing of Mesoproterozoic Magmatism, Lake Nipigon Region, Ontario. Can. J. Earth Sci. 44, 1055–1086. doi:10.1139/e06-117
Hernandez-Moreira, R., Jafarinik, S., Sanders, S., Kendall, C. G., Parker, G., and Viparelli, E. (2020). Emplacement of Massive Deposits by Sheet Flow. Sedimentology 67, 1951–1972.
Hessler, A. M., and Lowe, D. R. (2006). Weathering and Sediment Generation in the Archean: An Integrated Study of the Evolution of Siliciclastic Sedimentary Rocks of the 3.2Ga Moodies Group, Barberton Greenstone Belt, South Africa. Precambrian Res. 151, 185–210. doi:10.1016/j.precamres.2006.08.008
Hiscott, R. N. (1994). Traction-carpet Stratification in Turbidites – Fact or Fiction? J. Sediment. Res. A64, 209–214.
Hjellbakk, A. (1993). A 'flash-Flood' Dominated Braid delta in the Upper Proterozoic Næringselva Member, Varanger Peninsula, Northern Norway. Norwegian J. Geology. 73, 63–80.
Ielpi, A., Fralick, P., Ventra, D., Ghinassi, M., Lebeau, L. E., Marconato, A., et al. (2018). Fluvial Floodplains Prior to Greening of the Continents: Stratigraphic Record, Geodynamic Setting, and Modern Analogues. Sediment. Geology. 372, 140–172. doi:10.1016/j.sedgeo.2018.05.009
Ielpi, A., and Rainbird, R. H. (2016b). Highly Variable Precambrian Fluvial Style Recorded in the Nelson Head Formation of Brock Inlier (Northwest Territories, Canada). J. Sediment. Res. 86, 199–216. doi:10.2110/jsr.2016.16
Ielpi, A., and Rainbird, R. H. (2016a). Reappraisal of Precambrian Sheet-Braided Rivers: Evidence for 1·9 Ga Deep-Channelled Drainage. Sedimentology 63, 1550–1581. doi:10.1111/sed.12273
Johnsson, M. J., Stallard, R. F., and Lundberg, N. (1991). Controls on the Composition of Fluvial Sands from a Tropical Weathering Environment: Sands of the Orinoco River Drainage basin, Venezuela and Colombia. Geol. Soc. America Bull. 103, 1622–1647. doi:10.1130/0016-7606(1991)103<1622:cotcof>2.3.co;2
Junnila, R. M., and Young, G. M. (1995). The Paleoproterozoic Upper Gowganda Formation, Whitefish Falls Area, Ontario, Canada: Subaqueous Deposits of a Braid delta. Can. J. Earth Sci. 32, 197–209. doi:10.1139/e95-016
Kasting, J. F., and Ono, S. (2006). Palaeoclimates: the First Two Billion Years. Phil. Trans. R. Soc. B 361, 917–929. doi:10.1098/rstb.2006.1839
Khalifa, M. K. H., Jones, B. G., and Hlal, O. (2015). Sedimentary Facies Analysis and Paleogeographic Significance of the Latest Silurian to Early Devonian Winduck Interval in the Darling Basin, Western New South Wales, Australia. Aust. J. Earth Sci. 62, 307–330. doi:10.1080/08120099.2015.1020449
Klein, G. d., deMELO, U., and Favera, J. C. D. (1972). Subaqueous Gravity Processes on the Front of Cretaceous Deltas, Recôncavo Basin, Brazil. Geol. Soc. America Bull. 83, 1469–1492. doi:10.1130/0016-7606(1972)83[1469:sgpotf]2.0.co;2
Kostaschuk, R. A. (1985). River Mouth Processes in a fjord-delta, British Columbia, Canada. Mar. Geology. 69, 1–23. doi:10.1016/0025-3227(85)90131-8
Lambert, A., and Giovanoli, F. (1988). Records of Riverbome Turbidity Currents and Indications of Slope Failures in the Rhone delta of Lake Geneva. Limnol. Oceanogr. 33, 458–468. doi:10.4319/lo.1988.33.3.0458
Le Heron, D. P., Armstrong, H. A., Wilson, C., Howard, J. P., and Gindre, L. (2010). Glaciation and Deglaciation of the Libyan Desert: The Late Ordovician Record. Sediment. Geology. 223, 100–125. doi:10.1016/j.sedgeo.2009.11.002
Lindsay, J. F., Prior, D. B., and Coleman, J. M. (1984). Distributary-mouth Bar Development and Role of Submarine Landslides in delta Growth, South Pass, Mississippi Delta. Bull. Am. Assoc. Pet. Geologists 68, 1732–1743. doi:10.1306/ad46198a-16f7-11d7-8645000102c1865d
Long, D. G. F. (2019). Archean Fluvial Deposits: A Review. Earth-Science Rev. 188, 148–175. doi:10.1016/j.earscirev.2018.11.009
Long, D. G. F. (2004). Precambrian Rivers. In The Precambrian Earth: Tempos and Events. Editor P. G Eriksson. Elsevier, Amsterdam, 660–663.
Long, D. G. F. (1978). Proterozoic Stream Deposits: Some Problems of Recognition and Interpretation of Ancient sandy Fluvial Systems. Fluvial Sedimentology. Can. Soc. of Petroleum Geologists, Memoir. 5, 313–331.
Lowe, D. R. (1982). Sediment Gravity Flows: II. Depositional Models with Special Reference to the Deposition of High-Density Turbidity Currents. J. Sediment. Petrology 52, 279–297. doi:10.1306/212F7F31-2B24-11D7-8648000102C1865D
Macnaughton, R. B., and Narbonne, G. M. (1999). Evolution and Ecology of Neoproterozoic-Lower Cambrian Trace Fossils, NW Canada. Palaios 14, 97–115. doi:10.2307/3515367
Magalhães, A. J. C., Scherer, C. M. S., Raja Gabaglia, G. P., and Catuneanu, O. (2015). Mesoproterozoic delta Systems of the Açuruá Formation, Chapada Diamantina, Brazil. Precambrian Res. 257, 1–21. doi:10.1016/j.precamres.2014.11.016
Martin, A. J. (2000). Flaser and Wavy Bedding in Ephemeral Streams: a Modern and an Ancient Example. Sediment. Geology. 136, 1–5. doi:10.1016/s0037-0738(00)00085-3
Martin, C. A. L., and Turner, B. R. (1998). Origins of Massive-type Sandstones in Braided River Systems. Earth-Science Rev. 44, 15–38. doi:10.1016/s0012-8252(98)00019-1
Martins-Neto, M. A. (1996). Lacustrine Fan-Deltaic Sedimentation in a Proterozoic Rift basin: the Sopa-Brumadinho Tectonosequence, southeastern Brazil. Sediment. Geology. 106, 65–96. doi:10.1016/0037-0738(95)00152-2
Massari, F., and Parea, G. C. (1990). “Wave-dominated Gilbeert-type Gravel Deltas in the Hinterland of the Gulf of Taranto (Pleistocene, Southern Italy),” in Coarse-Grained Deltas. International Association of Sedimentologists, Special Publication. Editors A. Colella, and D. B. Prior (Hoboken, NJ: Wiley), 10, 311–331.
Massari, F. (1984). Resedimented Conglomerates of a Miocene Fan-delta Complex, Southern Alps, Italy. In Sedimentology of Gravels and Conglomerates. Editor E. H. Koster, and R. J. Steel. Canadian Society of Petroleum Geology, Memoir 10, 259–277.
McPherson, J. G., Shanmugam, G., and Moiola, R. J. (1987). Fan-deltas and Braid Deltas: Varieties of Coarse-Grained Deltas. Geol. Soc. America Bull. 99, 331–340. doi:10.1130/0016-7606(1987)99<331:fabdvo>2.0.co;2
Melvin, J. (2015). Lithostratigraphy and Depositional History of Upper Ordovician and Lowermost Silurian Sediments Recovered from the Qusaiba-1 Shallow Core Hole, Qasim Region, central Saudi Arabia. Rev. Palaeobotany Palynology 212, 3–21. doi:10.1016/j.revpalbo.2014.08.014
Middleton, G. V. (1967). Experiments on Density and Turbidity Currents: Iii. Deposition of Sediment. Can. J. Earth Sci. 4, 475–505. doi:10.1139/e67-025
Middleton, G. V., and Hampton, M. A., 1976. Subaqueous Sediment Transport and Deposition by Sediment Gravity Flows. In Marine Sediment Transport and Environmental Management. Editor D. J. Stanley, and D. J. P. Swift. Wiley, New York, 197–218.
Muhlbauer, J. L., and Fedo, C. M. (2020). Architecture of a River-Dominated, Wave- and Tide-Influenced, Pre-vegetation Braid delta: Cambrian Middle Member of the Wood Canyon Formation, Southern Marble Mountains, California, U.S.A. J. Sediment. Res. 90, 1011–1036. doi:10.2110/jsr.2020.023
Nemec, W. (1990). “Aspects of Sediment Movement on Steep delta Slopes,” Editors A. Colella, and D. B. Prior (Coarse-Grained Deltas. International Association of Sedimentologists , Special Publication), 10, 29–73.
Nemec, W., and Steel, R. J. (1988). What Is a Fan Deelta and How Do We Recognizeit? In Fan Deltas: Sedimentology and Tectonic Settings. Editor W. Nemec, and R. J. Steel. Blackie, London, 3–13.
Orton, G. J. (1988). A Spectrum of Middle Ordovician Fan Deltas and Braid-plain Deltas North Wales: a Consequence of Varying Fluvial Clastic Input. In Fan Deltas: Sedimentology and Tectonic Settings. Editor W. Nemec, and R. J. Steel. Blackie, London, 23–49.
Orton, G. J., and Reading, H. G. (1993). Variability of Deltaic Processes in Terms of Sediment Supply, with Particular Emphasis on Grain Size. Sedimentology 40, 475–512. doi:10.1111/j.1365-3091.1993.tb01347.x
Parkash, B., and Middleton, G. V. (1970). Downcurrent Textural Changes in Ordovician Turbidite Greywackes. Sedimentology 14, 259–293. doi:10.1111/j.1365-3091.1970.tb00195.x
Patranabis-Deb, S., and Kumar Chaudhuri, A. (2007). A Retreating Fan-delta System in the Neoproterozoic Chattisgarh Rift basin, central India: Major Controls on its Evolution. Bulletin 91 (6), 785–808. doi:10.1306/11270605182
Penland, S., Boyd, R., and Suter, J. R. (1988). Transgressive Depositional Systems of the Mississippi delta plain: a Model for Barrier Shoreline and Shelf Sand Development. J. Sediment. Petrology 58, 932–949. doi:10.1306/212f8ec2-2b24-11d7-8648000102c1865d
Pickering, K. T. (1984). Facies, Facies-Associations and Sediment Transport/deposition Processes in a Late Precambrian Upper basin-slope/pro-delta, Finnmark, N. Norway. Geol. Soc. Lond. Spec. Publications 15, 343–362. doi:10.1144/gsl.sp.1984.015.01.23
Piper, D. J. W., and Panagos, A. G. (1981). Growth Patterns of the Acheloos and Evinos Deltas, Western Greece. Sediment. Geology. 28, 111–132.
Posamentier, H. W., and Allen, G. P. (1999). Siliciclastic Sequence Stratigraphy – Concepts and Applications. Soc. Sediment. Geology. Concepts Sedimentology Paleontol. 7, 204.
Postma, G. (1990). Depositional Architecture and Facies of River and Fan Deltas: a Synthesis. Editor A. Colella, and D. B. Prior. Coarse-Grained Deltas. International Association of Sedimentologists, Special Publication 10, 13–27.
Postma, G., Nemec, W., and Kleinspehn, K. L. (1988). Large Floating Clasts in Turbidites: a Mechanism for Their Emplacement. Sediment. Geology. 58, 47–61. doi:10.1016/0037-0738(88)90005-x
Prior, D. B., Bornhold, B. D., Wiseman, W. J., and Lowe, D. R. (1987). Turbidity Current Activity in a British Columbia Fjord. Science 237, 1330–1333. doi:10.1126/science.237.4820.1330
Reading, H. G., and Collinson, J. D. (1996). “Clastic Coasts,” in Sedimentary Environments: Processes, Facies and Stratigraphy. Editor H. G. Reading (Blackwell Science), 154–231. doi:10.1126/science.237.4820.1330
Reading, H. G., and Orton, G. J. (1991). “Sediment Caliber: a Control on Facies Models with Special Reference to Deep Sea Depositional Systems,” in Contraversies in Moder Geology. Editor D. W. Muller, J. A. McKenzie, and H. Weissert (Academic Press, London), 85–111.
Reineck, H.-E., and Wunderlich, F. (1968). Classification and Origin of Flaser and Lenticular Bedding. Sedimentology 11, 99–104. doi:10.1111/j.1365-3091.1968.tb00843.x
Reineck, H. E., and Singh, I. B. (1973). Depositional Sedimentary Environments – with Reference to Terrigenous Clastics. Berlin: Springer-Verlag, 439.
Robertson, W. A. (1973). Pole Position from Thermally Cleaned Sibley Group Sediments and its Relevance to Proterozoic Magnetic Stratigraphy. Can. J. Earth Sci. 10, 180–193. doi:10.1139/e73-020
Roe, S. L. (1995). Stacked Fluviodeltaic Cycles in the Upper Proterozoic Godkeila Member, Varanger Peninsula, Northen Norway. Norwegian J. Geology. 75, 229–242.
Rogala, B., Fralick, P. W., Heaman, L. M., and Metsaranta, R. (2007). Lithostratigraphy and Chemostratigraphy of the Mesoproterozoic Sibley Group, Northwestern Ontario, Canada. Can. J. Earth Sci. 44, 1131–1149. doi:10.1139/e07-027
Rogala, B., Fralick, P. W., and Metsaranta, R. (2005). Stratigraphy and Sedimentology of the Mesoproterozoic Sibley Group and Related Igneous Intrusions, Northwestern Ontario: Lake Nipigon Region Geoscience Initiative. Ontario Geol. Surv. open file Rep. 6174, 128.
Rogala, B. (2003). The Sibley Group: A Lithostratigraphic, Geochemical and Paleomagnetic Study. LakeheadUniversity, Thunder Bay, Ontario Canada: Unpublished MSc Thesis, 254.
Sohn, Y. H. (1997). On Traction-Carpet Sedimentation. J. Sediment. Res. 67, 502–509. doi:10.1306/d42685ae-2b26-11d7-8648000102c1865d
Stow, D. A. V., Braakenburg, N. E., and Johansson, M. (1994). Atlas of deepwater, Massive Sands. Southampton, United Kingdom: Southampton University. Unpublished Report.
Sturm, M., and Matter, A., 1978. Turbidites and Varves in Lake Brienz (Switzerland): Deposition of Clastic Detritus by Density Currents. In Modern and Ancient Lake Sediments. Editor A. Matter, and M. E. Tucker International Association of Sedimentologists, Special Publication 2, 147–168.doi:10.1002/9781444303698.ch8
Terwindt, J. H. J., and Breusers, H. N. C. (1972). Experiments on the Origin of Flaser, Lenticular and Sand-clay Alternating Bedding. Sedimentology 19, 85–98. doi:10.1111/j.1365-3091.1972.tb00237.x
Vaucher, R., Vaccari, N. E., Balseiro, D., Muñoz, D. F., Dillinger, A., Waisfeld, B. G., et al. (2020). Tectonic Controls on Late Cambrian-Early Ordovician Deposition in Cordillera oriental (Northwest Argentina). Int. J. Earth Sci. (Geol Rundsch) 109, 1897–1920. doi:10.1007/s00531-020-01879-9
Ventra, D., and Clarke, L. E. (2018). Geology and Geomorphology of Alluvial and Fluvial Fans: Current Progress and Research Perspectives, 440. London: The Geological Society of London, Special Publications, 1–21. doi:10.1144/sp440.16
Vos, R. G. (1981). Sedimentology of an Ordovician Fan delta Complex, Western Libya. Sediment. Geology. 29, 153–170. doi:10.1016/0037-0738(81)90005-1
Vrolijk, P. J., and Southard, J. B. (1997). Experiments on Rapid Deposition of Sand from High Velocity Flows. Geosci. Can. 24, 45–54.
Walton, E. K. (1967). The Sequence of Internal Structures in Turbidites. Scottish J. Geology. 3, 306–317. doi:10.1144/sjg03020306
Wendland, C., Fralick, P., and Hollings, P. (2012). Diamondiferous, Neoarchean Fan-delta Deposits, Western Superior Province, Canada: Sedimentology and Provenance. Precambrian Res. 196-197, 46–60. doi:10.1016/j.precamres.2011.11.007
Went, D. J. (2020). Fluvial Shoal Water Deltas: Pre‐vegetation Sedimentation through the Fluvial-marine Transition, Lower Cambrian, English Channel Region. Sedimentology 67, 330–363. doi:10.1111/sed.12645
Westcott, W. A., and Ethridge, E. G. (1990). Fan Deltas – Alluvial Fans in Coastal Settings. In Alluvial Fans: A Field Approach. A. H. Rachocki, and M. Church Wiley, 195–211.
Wright, L. D., and Coleman, J. M. (1974). Mississippi River Mouth Processes: Effluent Dynamics and Morphologic Development. J. Geology. 82, 751–778. doi:10.1086/628028
Keywords: pre-vegetation, delta, precambrian sedimentation, sibley group, massive sandstones, delta parasequences
Citation: Jones R, Marcelissen R and Fralick P (2022) Sedimentology and Stratigraphy of a Large, Pre-Vegetation Deltaic Complex. Front. Earth Sci. 10:875838. doi: 10.3389/feart.2022.875838
Received: 14 February 2022; Accepted: 04 April 2022;
Published: 04 May 2022.
Edited by:
Darrel G. F. Long, Laurentian University, CanadaReviewed by:
Andre Marconato, University of São Paulo, BrazilRobert Rainbird, Geological Survey of Canada, Canada
Jason Muhlbauer, University of Tennessee at Chattanooga, United States
Copyright © 2022 Jones, Marcelissen and Fralick. This is an open-access article distributed under the terms of the Creative Commons Attribution License (CC BY). The use, distribution or reproduction in other forums is permitted, provided the original author(s) and the copyright owner(s) are credited and that the original publication in this journal is cited, in accordance with accepted academic practice. No use, distribution or reproduction is permitted which does not comply with these terms.
*Correspondence: Philip Fralick, philip.fralick@lakeheadu.ca