- 1 Laboratoire EDYTEM, Université Savoie Mont Blanc, CNRS UMR, Le Bourget du Lac, France
- 2 Department of Chemical and Geological Sciences, University of Modena and Reggio Emilia, Modena, Italy
Landslides are important components of global geoheritage, but awareness of their significance and value in such terms seems scanty in the scientific community. Landslides are normally identified among various features of geological and geomorphological interest, and often considered a source of hazard. However, they are seldom identified as geosites and as part of geoheritage. This paper aims at filling these gaps by highlighting the importance of landslides in the global geoheritage. After a short introduction on the values and criteria to define landforms as geosites, based on literature review, we show to what extent landslides have been defined as geomorphosites and as part of geoheritage around the world. We then outline three aspects that should be specifically considered in the identification of landslides as geomorphosites, namely 1) past and present climate changes, 2) anthropic signature, and 3) risk perception. Finally, we describe four cases of spectacular landslides that serve as significant examples worldwide.
Introduction
Landslides are mass movements of primarily rock and/or soil on the land surface (Cruden, 1991; Cruden and Varnes, 1996; Hungr et al., 2014) and are an important landscape-shaping process, contributing significantly to sediment erosion, release, transport and deposition on hillslopes. Landslides are a great example of landforms with a broad (geo)diversity (Gray, 2004; Gray et al., 2013)—due to their variety of origin, composition, forms, processes, environment, and spatio-temporal distribution—and can result in spectacular landforms (e.g. Soldati et al., 2019). The succession and/or combination of different mass-movement processes with different rates on a variety of time scale drive the diversity and complexity of landslides, leading to an increased geodiversity of a territory (e.g., Fepuleai and Németh, 2019), and making it part of its geoheritage (Brocx and Semeniuk, 2007).
Valuing and promoting geoheritage, and particularly those elements of exceptional value (i.e. geosites) is a crucial step in the geoconservation process (Brilha, 2016). Numerous local examples of landslides around the world have been recognized as geosites (cf. Table 2). However, the role of landslides as a key landform in the promotion and enhancement of global geoheritage has so far been neglected. Geoheritage sites serve the public interest (Coratza et al., 2018), and their valorisation and conservation is a key instrument to communicate geoscience and Earth systems processes and to make them accessible to society. Scientists can transfer their knowledge on the geological changes that affect the Earth at different time and spatial scales through landscapes, landforms, and outcrops. The growing interest in geoheritage and geoconservation in the last decades has caused the rise of a number of local (e.g., Burlando et al., 2011; Migoń et al., 2018; Venturini and Pasquaré Mariotto, 2019), regional (e.g., Fuertes-Gutiérrez and Fernández-Martínez, 2010; Vasiljević et al., 2011; Ferrero et al., 2012; Migoń and Pijet-Migoń, 2016; Moufti and Németh, 2016; Szepesi et al., 2017), national (e.g., De Wever et al., 2015; Chlachula, 2020), and international (Wimbledon and Smith-Meyer, 2012; Larwood et al., 2013; Reynard and Coratza, 2013; Woo, 2017; Finney and Hilario, 2018) initiatives up to UNESCO Geoparks and to UNESCO World Heritage sites (De Vries et al., 2018; Reynard and Brilha, 2018), and advances in the cooperation between scientific researchers, public-policy makers and environmental conservationists (Brocx and Semeniuk, 2007; Henriques et al., 2011; Brilha, 2018).
Despite landslides being an ubiquitous phenomenon on Earth and having their environmental and hazardous impact been vastly documented (e.g., Petley 2012), residents of local communities generally tend to neglect or underestimate their risk (e.g., Nathan 2008). The urge of a better communication of landslides nature and impacts to society derives not only from the evidence of their disastrous consequences for human lives and infrastructures, but also for their scientific, educational, socio-economic, cultural and, perhaps paradoxically, aesthetic values (Giordan et al., 2015; Alcántara-Ayala and Moreno, 2016).
As for any other geoscience area (De Vries et al., 2018; van Wyk de Vries et al., 2018; Guilbaud et al., 2020; Vereb et al., 2020; Fepuleai et al., 2021; Geopoderes, 2022), the public should be exposed to the latest scientific discoveries and most accurate knowledge on landslides. This particularly applies when significant scientific understanding on landslides brings advances at national and international level, and when this progress is achieved studying a meaningful site.
Education and public outreach allow great progress in any geoconservation field (Németh et al., 2021) and contribute to disseminate the importance of geoheritage (Reynard and Coratza, 2016). Therefore, raising awareness of students, politicians, stakeholders, and the public on the scientific importance of landslides, on their causes - including those directly and indirectly induced by anthropic actions - on their impact in shaping the landscape, and on their hazardous and economic effects on society, can provide them with important knowledge about how landslides can affect the environment and, consequently, human life (e.g., Giordan et al., 2015). This ultimately raises awareness on the long-standing issue of landslide hazard management and benefits the development of new sustainable preventing and mitigation actions.
Landslides can have a significant socio-economic impact: over the past decades, socio-economic losses due to landslide events have been rising (e.g., Highland et al., 1998; Guzzetti, 2000; Petley et al., 2005; Peduzzi et al., 2009; Alimohammadlou et al., 2013)—for example, the ratio of deaths per 106 people per year is 100 times higher in Central America than in North America (Petley et al., 2005), and in the United States, landslides cause between one and 3.6 billion dollars each year (Highland et al., 1998), and have been classified and widely described (Winter and Bromhead, 2012). These include fatalities and injuries, disruption to infrastructure, and alteration to the access and use of transport for trading, employment, health, educational and social activities (Winter and Bromhead, 2012). Informing the population on the vulnerability of local communities and infrastructures via recreational and educational initiatives is one of the most effective tools to convey to people knowledge on the hazard and socio-economic impacts of landslides, and to involve them in preventing and mitigation actions. A valuable example of this is MurGame (murgame.ch), a free application that has been recently released by the Swiss Federal Institute for Forest, Snow and Landscape Research (WSL) that emphasises the risks associated with debris flows and allows the player to experience the effectiveness and economic impacts of different mitigation measures.
Landslides are commonly associated to damage and disaster, particularly immediately after their occurrence, when their destructive effects on land are evident. However, they do have cultural and aesthetic values that can be exploited by the recognition of geosites and by the creation of geoitineraries, combining leisure and education purposes. The scenic beauty and historical and environmental value of landslides and the landscapes in which they are present can aid to the development of sustainable tourism. Showing how landslides form, their different types, their age and the consequences of their emplacement can promote the understanding of the close interconnection between landslides-environment-society. There are stunning cases where landslide and culture meet harmoniously, such as in Bomarzo (Italy), where landslide accumulation material was used to create a historic garden with allegoric sculptures (Margottini, 2013).
This paper aims at promoting landslides as key landform in the global geological heritage. We perform a systematic literature review of how landslides are defined as geo(morpho)sites and/or as part of geoheritage around the world. We then identify three aspects that can be considered in the recognition and identification of a landslide as a geo(morpho)site. These aspects are 1) past and present climate changes, 2) anthropic signature, and 3) risk perception. We emphasise the importance of such aspects by exploring four cases of famous and spectacular landslides: the Frank Slide (Canada), the Vajont landslide (Italy), the Aoraki/Mount Cook landslide (New Zealand) and the diffused landslides of north-central Iceland, and the Island of Malta.
Identification of Landslides as Geomorphosites
Values and Criteria to Define Landforms as Geomorphosites
The concept of geoheritage, in its current form in terms of scope and objectives, is relatively new and in the late 20th century has received an increasing interest, both in conservation and tourism sectors. In particular, geomorphological heritage embraces landforms and processes that are worthy of being protected and transmitted to the future generations, as they play a key role in the understanding of Earth history, and are linked to the biological and the cultural heritage (Reynard, 2016).
The definition of geomorphosites (Panizza, 2009) and their values have been much debated in the geomorphological community (e.g., Coratza and Hobléa, 2018 and references therein) and more in general in the geological community (Brilha 2016, 2018 and references therein). According to Panizza and Piacente (2003), a geomorphosite is defined as “a landform with particular and significant geomorphological attributions, which qualify it as a component of a territory’s cultural heritage (in a broad sense)”. The attributes that may confer value to a landform are many, they are interrelated and interdependent, and may sometimes overlap and conflict. The terminology of values and attributes used in geomorphosite inventories and the assessment methodologies differ by authors and are not standardised. According to Reynard (2009) and Coratza and Hobléa (2018), two main groups of values for geomorphosites’ selection and assessment can be distinguished: 1) Scientific value: this is directly related to the scientific aspects of the sites from a geomorphological point of view; generally, the scientific value is the key or central value; 2) Additional values: these can be aesthetic, ecological and cultural, and highlight the links between geomorphology and other aspects of culture and nature. Among these, the ecological value is particularly important, as it can greatly contribute to increase the scientific meaning of a geosite (Pelfini and Gobbi, 2005; Garavaglia et al., 2010; Pelfini et al., 2010). Some authors (Reynard et al., 2007; Zouros, 2007; Bollati et al., 2012, 2013; Fassoulas et al., 2012) also consider the economic value, partially overlapping with its potential for use in educational (formal and informal) and geotourism-recreational contexts. Some studies (Mocior and Kruse, 2016; Gordon et al., 2021) also report on the functional value of landforms in providing the essential substrates, habitats, abiotic processes and cultural services that maintain physical and ecological systems at the Earth’s surface (Gray, 2004). Gray (2011) and Gray et al. (2013) refer to them as geosystem services. Despite many methods reported in literature about the evaluation of sites, the scientific literature reveals that there is still a great debate concerning values and the different criteria and indicators to be used in geosite identification and assessment. Different values are usually assessed, qualitatively or quantitatively, based on the criteria and indicators summarized in Table 1.
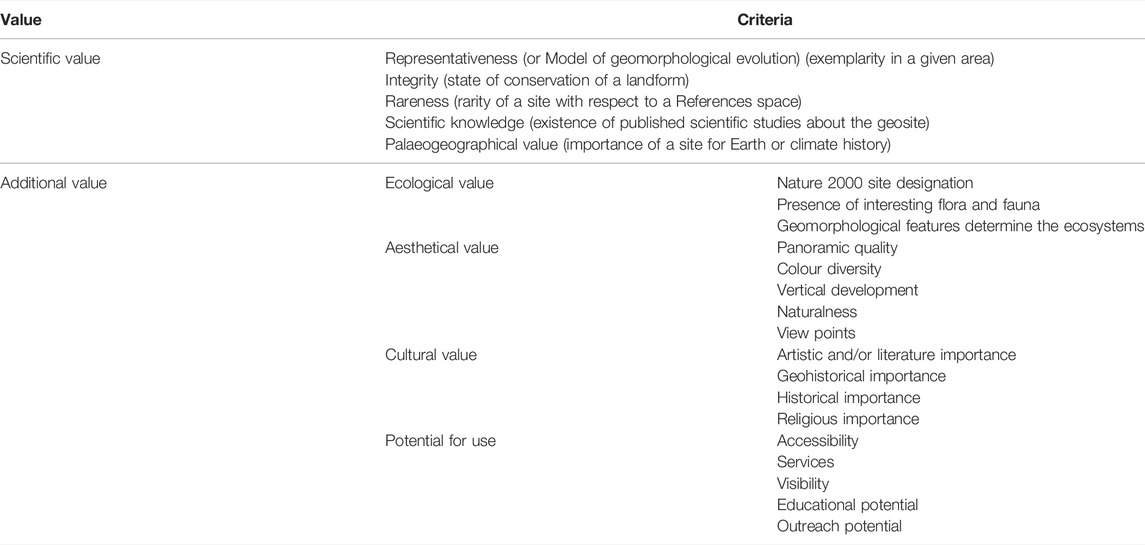
TABLE 1. The criteria and image.png indicators to assess the definition of the main values for the identification of a landform as geomorphosite.
Landslides as Geomorphosites and as Part of the Geoheritage Worldwide
To survey how previous research approached the subject of landslides as geomorphosites and as part of the geoheritage, we performed a systematic literature research. Google Scholar, Scopus and Web of Science were searched from inception until 15th December 2020 using the keywords ‘‘landslide”, ‘‘mass movement”, ‘‘slope instability”, ‘‘rock avalanche”, “rock fall”, “debris flow” in combination respectively with ‘‘geosite”, ‘‘geoheritage”, “geodiversity”, ‘‘geomorphosite”, “geopark”, “national park”, ‘‘geotourism”, “geoitinerary” (Figure 1).
We then selected the publications according to their relevance to the topic. We considered publications in English. In addition, we performed a manual search of the reference lists of the publications found. We then classified the publications into the categories “Direct link” and “Indirect link”. In the category “Direct link” fall studies that consider landslides as geosites or that find landslides being important for the geological heritage and/or from a geological perspective. This category has been subdivided into three sub-categories, depending on whether the publication 1) clearly identifies a landslide or different examples of “landslides as geosites/geomorphosites”, or 2) recognises the value of a landslide or different examples of “landslides in the geoheritage”, or 3) lists a landslide or different examples of “landslides among the features of interest” that aid to define a site of geological interest for the scientific community and the public (Table 2). In the category “Indirect link” fall studies where landslides are considered and discussed because of the hazardous impact that they can have on geosites, geomorphosites, geoitineraries, parks and locations that are of public interest for their geological and geomorphological aspects (Figure 1; Table 2). We are aware that our survey might have overlooked some references where landslides are marginally cited for their relevance in the geoheritage (e.g., Pérez-Umaña et al., 2019, 2020; Migoń and Pijet-Migoń, 2020; Quesada-Román et al., 2020), but because excluded by the web search engines for scholarly literature that we have adopted—probably because “landslide” was not in either the abstracts nor in the keywords, we have not integrated them in our study.
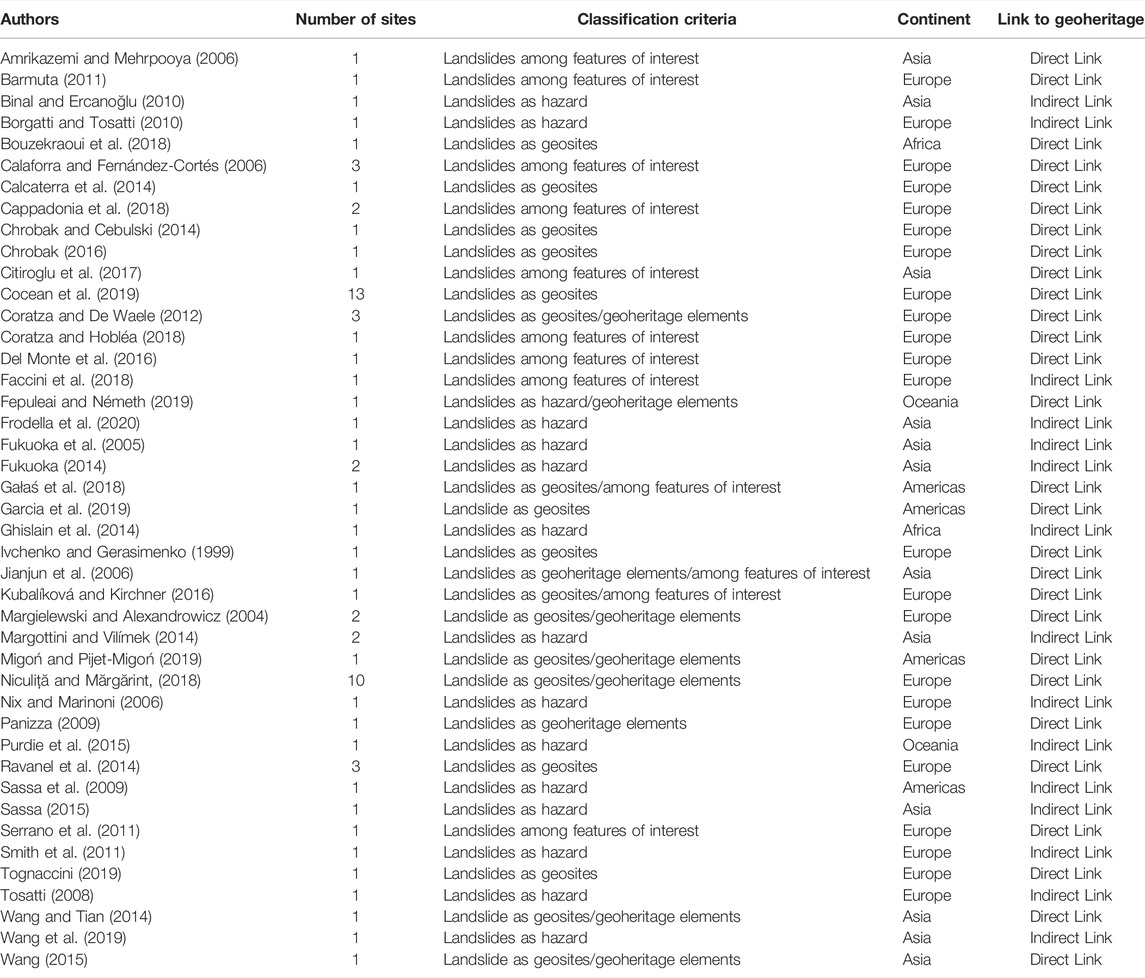
TABLE 2. Studies reporting on sites that show Direct or Indirect link between landslides and the geological heritage.
We mapped the geographic position of the study sites of the publications that record a Direct or an Indirect link between landslides and the geological heritage on the world map (Figure 2A). The predominance of studies addressing the topic of landslides in the geoheritage is in Europe (Figures 2B,C), with 47 sites with a Direct link between landslides and the geoheritage and 5 with an Indirect link. In the Asian continent there are 5 sites with a Direct link and 9 with an Indirect link. In the Americas we found 3 studies with a Direct link between landslides and the geoheritage and 1 study with an Indirect link. Finally, Africa and Oceania are the areas with the least number of studies dealing with the topic of landslides in the geoheritage, with 1 study that we classified as with Direct link and 1 with Indirect link each.
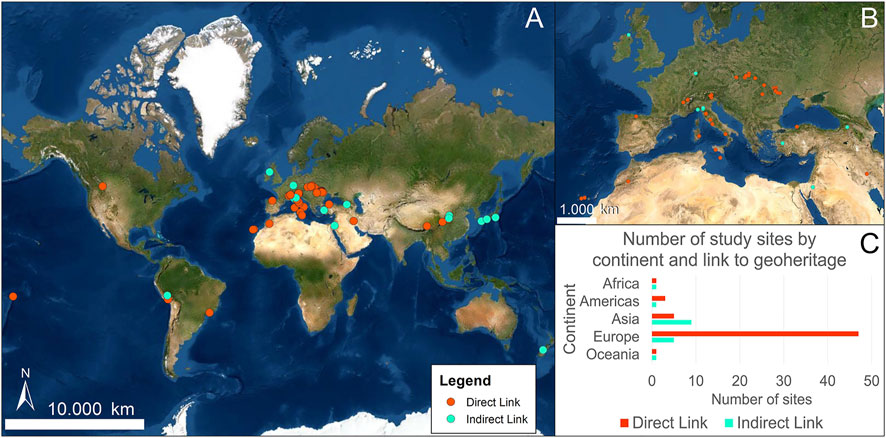
FIGURE 2. (A) World Map of the study sites in publications that record a Direct or an Indirect link between landslides and the geological heritage. Base map from World Imagery provides satellite and aerial imagery worldwide, and features imagery from Esri, Maxar, GeoEye, Earthstar Geographics, CNES/Airbus DS, USDA, USGS, AeroGRID, IGN, and the GIS User Community. (B) Map of the study sites in publications that record a Direct or an Indirect link between landslides and the geological heritage in Europe. (C) Column chart of the number of study sites by continent in publications having a Direct or an Indirect link between landslides and the geological heritage.
Despite the highest concentration of studies on landslides as geosites and as part of geoheritage concerns mass movements in Europe, the numbers of studies addressing this topic worldwide do not reflect the scientific, educational, socio-economic, cultural and aesthetic importance and impacts that slope mass movements have globally. The reasons why Europe is the continent with the highest number of studies addressing the topic of landslides as geosites and as part of geoheritage are various. Europe has a long tradition in the study and dissemination of geoheritage (Burek and Prosser, 2008; Erikstad, 2008; Wimbledon and Smith-Meyer, 2012). Over the last sixty years the interests in geoheritage and geoconservation has increasingly grown in European countries (Prosser, 2013). In the United Kingdom, the first systematic approach to geoconservation endorsed by legislation was instituted in 1949 (Thomas and Cleal, 2004). Geoparks have been promoted in Germany since the beginning of the 1990s (Frey et al., 2006). Special laws for the conservation of geoheritage were enacted in Romania since 1930s’ (Wimbledon and Smith-Meyer, 2012) and in Italy, from 2000s’, the Italian Geological Service has produced a national register of geosites. The continuous and synergistic work of specialists from universities, research institutions and non-governmental organizations in Europe allowed the identification and formal recognition of protected areas for their geological and geomorphological significance, culminating in 1993 with the establishment of ProGEO as European association for the geoconservation and with the creation of the European Network of Global Geoparks (Henriques et al., 2011; Wimbledon and Smith-Meyer, 2012). Landslides are a severe problem for society and infrastructures in many parts of Europe (Leshchinsky et al., 2017; Herrera et al., 2018; Schlögl and Matulla, 2018). There are certain areas of the continent that are most exposed to landslide hazard. Different studies (Van Den Eeckhaut and Hervás, 2012; Jaedicke et al., 2014) show that landslide hazard in Europe is not ubiquitous, and some countries are much more seriously affected than others, as landslide occurrence depends on different factors related to topography, geology, climate, land cover, and human and environmental assets. For example, Italy has the 20% of the total amount of people in Europe exposed to landslide hazard (Jaedicke et al., 2014), but it has established risk mitigation strategies in place. In Romania, landslide events are less common, and even if the hazard is low-medium, consequences of landslide events are most severe (Bălteanu et al., 2020). Furthermore, Europe has a long historical record of landslide events, having the ancient European populations produced a legacy that can record natural disasters (Migoń and Pijet-Migoń, 2019).
Even though we included the keyword “national park”, our survey led us to find only 1 study with a Direct link in North America, which could seem curious, given the highly developed network of National Parks in the US (National Park, 2022) and in Canada (National Parks of Canada, 2022), their known published landslide case histories, and the entire variety of landslide types affecting their vast territories. Furthermore, this could apply to the other continents. However, despite North America having numerous striking examples of landslides, and despite them being present in National Parks’ territories, these landslides are not considered geosites sensu stricto. Our survey indicates that further efforts should be put to appraise, develop, and protect landslides as officially recognized geo(morpho)sites worldwide.
Identification of Landslides as Part of the Geoheritage
Landslides: Neglected Landforms in the Geoheritage
Landslides are landforms already considered in the global geoheritage, but they have received scarce attention from the international landslide scientific and geoheritage community so far. Only four sites with landslides are found in the UNESCO World Heritage List (i.e., Swiss Tectonic Arena Sardona; Dolomites; Tajik National Park; Pitons, cirques and ramparts of Reunion Island); they all comprise landslides among various landforms that contribute to represent the cultural and natural diversity of the sites. There are other cases of UNESCO World Heritage Sites, such as the Dorset and East Devon Coast in the United Kingdom, that have been recognised for their outstanding combination of geological and geomorphological features, including landslides, and that meet the criteria to be globally important landslide-geosites. The Dorset and East Devon Coast site has a long history of research (Brunsden and Edmonds, 2009; May 2015; Mortimore, 2019), evident scenic value, is well-known to the public, has inspired artists’ production (Gallois, 2010; Jurassiccoast, 2022), and yet landslides of this site (Allison, 2020) are not highlighted in the description of the World Heritage properties. This dearth is not due to a lack of scientific interest. The reasons are multiple, and firstly are probably to be found in the supposed lack of aesthetic appeal of landslides, as their beauty and significance are probably more difficult to be perceived by untrained eyes (visitors and tourists). Undoubtedly, there are many landforms on Earth that are more attractive to the human eye. However, seemingly unattractive objects may appear very interesting when examined in detail and become very useful for popularising geology and geomorphology, and for raising awareness on this kind of potentially hazardous processes and the natural and human-induced causes that determined them. Also, landslides might have been overlooked because of the absence of clear guidelines on the criteria to define a landform for its outstanding universal value.
Landslides are of high geomorphological significance and are among the few landforms that tell stories about landscape evolution and environmental changes both at human and geological timescale. Furthermore, there are numerous examples of spectacular landslides around the globe and/or that are located in scenic settings, as we show in Examples of Landslide Geomorphosites Worldwide Section. It is also worth to point out that the educational aspects that can derive from the visit to a landslide do not necessarily need a breath-taking site to be fully perceived. Besides, landslides can be better appreciated from view-points, as they can be seen in their whole or their majority, they can be contextualised in the setting in which they developed, and, if still active, distance can prevent hazardous situations for the site users.
In this section, we examine three aspects that can be crucial in defining landslides as geomorphosites, namely 1) present and past climate changes, 2) anthropic signature, and 3) risk perception. These three aspects are mutually dependent and extremely topical, as seen in the very recent extreme events of Chamoli (India) and Elliot Creek (Canada) landslide-flood cascades (Geertsema et al., 2020; Kropáček et al., 2021; Shugar et al., 2021; Van Wyk de Vries et al., 2021). Landslide formation strictly depends on climate variations, which in present and past times are one of the major controlling factors in landscape modelling. In the Sixth Assessment Report of the United Nations Intergovernmental Panel on Climate Change (UN IPCC), it is highlighted that warming temperatures may cause an increase in the frequency and intensity of heavy rainfall and the degradation of the cryosphere, consequently intensifying the risk of landslides in many mass-movements prone areas. Landslides threaten human settlements and infrastructure in various environments, but risk implications are often underestimated by local communities, who are not sufficiently involved in the processes of risk management and mitigation, not being adequately informed on the landslide nature, consequences and causes. Among the latter, anthropic activity is one of the most impactful triggers for landslide initiation and development, with disastrous consequences on the environment and human beings and infrastructures. Therefore, climate changes in present and past times, anthropic signature, and risk perception are aspects that need to be considered to define a landslide as a geomorphosite.
Past and Present Climate Changes
Climate changes condition Earth surface processes and landforms in space and time. Landscapes and environments are not only altered by contemporary changes, but they were also affected by changes in the past (e.g., Thomas, 2008; Wobus et al., 2010; Brown et al., 2017). Landslides are one of the geomorphological responses to varying climatic conditions over decades and longer timescales (e.g., Borgatti and Soldati, 2010a; Borgatti and Soldati, 2010b; Crozier, 2010; Gariano and Guzzetti, 2016; Pánek, 2019; Patton et al., 2019). Paleolandslides due to the consequences of past climate changes still impact the landscape today (e.g., Kuhn et al., 2020), and they are important in understanding how climate, physical processes, and landscapes have changed in the past. Paleolandslides are also crucial in allowing the evaluation of future changing scenarios over different temporal and spatial scales, particularly because they have a nonlinear response to environmental changes. Many studies have tried to identify the different causes of paleolandslides over past-changing climates. During the Late Glacial-Holocene, paraglacial landsliding occurred as indirect consequence of deglaciation (Cossart et al., 2008; Borgatti and Soldati, 2010a; McColl, 2012; Ballantyne et al., 2014; Mercier et al., 2017; Hermanns et al., 2017). Different studies highlight the role of precipitation and temperature variability throughout various timescales in affecting frequency and magnitude of mass movements in the past (e.g., Bookhagen et al., 2005; Borgatti and Soldati, 2010a; Stoffel and Huggel, 2012; Stoffel et al., 2014). Therefore, awareness of the temporal dimension of landslide effects is essential to understand past changes and to predict future landscape trajectories through a “learning from the past” approach (e.g., Dearing et al., 2006, 2010; Brown et al., 2012). As documented in paleoenvironmental records, changes in dynamic systems as those affected by mass movements may be inevitable, so an understanding of the geomorphological effects of such landslides under changing climatic conditions is key from a geoheritage perspective (Thomas, 2001; Burt et al., 2002; Prosser et al., 2010; Micu et al., 2021).
Unanswered questions exist regarding the link between landslides and the ongoing climate change (Crozier, 2010; Korup et al., 2012; Gariano and Guzzetti, 2016). The Sixth Assessment Report of the UN IPCC states that projected increased extreme precipitation may potentially lead to cascading consequences, including landslides. The potential for landslides may be exacerbated with total precipitation rates, precipitation intensity, mountain permafrost thaw rates, glacier retreat and air temperature (Crozier, 2010; Huggel et al., 2013; Gariano and Guzzetti, 2016; Masson-Delmotte et al., 2021). Many studies focus on rainfall as the trigger for landslides (Caine, 1980; Larsen and Simon, 1993; Wieczorek and Glade, 2005; Guzzetti et al., 2008). Varying frequency or magnitude of precipitation can affect infiltration rates and pore-water pressures, impacting on slope stability. According to Haque et al., 2019, extreme rainfall linked to climate change is contributing to a higher number of deadly landslides worldwide. Current climate change might also impact slope stability by altering rates of physical and chemical weathering (Davies et al., 2001; Arenson and Springman, 2005; Harris et al., 2009; Grämiger et al., 2018). Cryospheric terrain in mountainous regions are the most susceptible to slope stability issues (e.g., Sæmundsson et al., 2021), as warming temperature cause permafrost and/or glacial ice to degrade (Paul et al., 2004; Harris, 2005; Deline et al., 2015; Huss and Hock, 2015; Pastick et al., 2015; Kos et al., 2016; Sæmundsson et al., 2021, 2018; Zemp et al., 2019; Magnin et al., 2019; Morino et al., 2019, 2021) These changes, if maintained or worsen, can have significant implications for the prevention, mitigation and adaptation strategies, sustainable development and geoheritage conservation.
In the last two decades, the literature on landslides as part of geoheritage has considered the link to climate change only for its negative impact on geomorphosites conservation (cf. references in Table 2 classified with “Indirect Link” in “Link to geoheritage” column). Undoubtably, addressing the potential impacts of climate change on geoheritage features is a priority. However, here we highlight the importance of adding climate-change aspects within the scientific value parameters (cf. Table 1) to be used in the identification of landslides as geomorphosites. Emphasising this aspect may aid in increasing awareness of the public with respect to the influence of past, present and future climate changes on slope instability and related risk implications. The knowledge on when, where and how ancient, historical and recent landslides develop consequently to variations in climate can be disseminated to the general public and decision makers with multiple scopes: 1) to show that landslides formed after past climate changes are still observable in the present landscape where humans and other forms of life live; 2) to convey the concept that the current climate crisis has a tangible and often hazardous impact, and therefore it is not a distant and abstract threat; 3) to enhance adaptive capacity.
Anthropic Signature
Landslides can be often induced by human activities, which can be modifications of the topography, hydrogeological perturbation, surface water-flow modifications, land-use change and degradation, inappropriate and degraded infrastructures, blasting and vibrations (Bazin, 2011; Jaboyedoff et al., 2018). Landslide hazard and vulnerability levels have increased in areas prone to landsliding (Lazzari et al., 2006; Nicolet et al., 2013) due to the expansion of economic, technological and population development (e.g., Remondo et al., 2005). Dataset on fatal non-seismic landslides worldwide in the period 2004–2016 shows that landslide occurrence triggered by human activity is increasing, therefore human disturbance may affect more future landslide incidence than climate (Froude and Petley, 2018). This is evident also at local scale. For example, in the Urseren Valley in the Central Swiss Alps, landslide area has increased by 92% in 45 years because of human-induced changes in climate and land use (Meusburger and Alewell, 2008). Optical remote sensing data analyses have revealed an increased landslide hazard after deforestation and road construction in densely populated tropical mountain regions (Guns and Vanacker, 2013; Vanacker et al., 2013).
The traumatic consequences of natural disasters on psychological responses and mental health are well documented (e.g., Pennebaker and Harber, 1993; Norris, 2005; Benedek et al., 2007), but intergenerational transmission of the memory of disasters directly linked to human actions has been less studied. Creating landslide geomorphosites that collect the lessons learned from mass movements induced by anthropic activities can raise awareness among inhabitants and stakeholders of the potential harmful effects of human actions, and of the importance of land-planning and management for prevention and reduction of landslide risk. The anthropic signature is part of the scientific value (cf. Table 1) to define a landslide as a geomorphosite. It is an aspect that can emphasise the role of human activities in landslide onset, as well as the social and economic impacts that human activities themselves can suffer from landslide occurrence.
Conversely, anthropic signature can be interpreted as human actions impacting a landslide site by making it accessible and safe. This characteristic and the human activities among the causes of landslides make the anthropic signature a unique aspect for the definition of landslides as geo(morpho)sites: the role of humans come before the landslide event by being one of the triggers and after the event by ensuring the safe use of the landslide site. Therefore, the anthropic signature can be a fundamental aspect in various moments of the history of the landslide and emphasise its scientific value in a two-way manner.
Risk Perception
Landslide risk—as the anticipated degree of damage, loss and/or costs associated to a specific landslide event—is of major concern at national and international levels, because of the threat to life and livelihood that landslides pose (Glade and Crozier, 2010; Salvati et al., 2010, 2012). It is crucial that those who might be affected are provided with knowledge, made aware of the risk, and are involved in decisions about risk management, as these actions can aid to increase the resilience of a community. In this perspective, risk perception plays a decisive role in the way dwellers coexist with the risk of potential landslide events (Tulloch and Lupton, 2003).
In general, perception of risk depends on the judgement of an individual of a particular risk (Renn, 1992; Rohrmann and Renn, 2000), which in the case of landslides can be influenced and varied by factors such as experience of previous landslide events, psychological, social and cultural values (Fischhoff et al., 1983; Morgan et al., 2001; Garrick and Gekler, 2013). These cause a discrepancy between reality and risk perception and variations of risk perception among different persons. Alarmingly, perception of risk related to geo-hydrogeological events (i.e., landslides and floods) does not match the real risk posed to the population by these phenomena (Salvati et al., 2014; Calvello et al., 2016). This gap between risk perception and the real risk posed to local communities by landslides should be reduced via incisive actions of education and communication. This need has been reiterated by many studies on landslide risk perception (Ardaya et al., 2017; Gravina et al., 2017), as informing, educating, exchanging experience, and involving the civil society in the decision processes are crucial to build awareness, with the ultimate aim for institutions and stakeholders to protect their local communities in areas susceptible to landslides.
In particular, to improve the perception of risk and to motivate locals to participate in the process of risk prevention and mitigation, the nature and potential consequences, the different aspects of local interests that could be directly and indirectly affected (Ardaya et al., 2017), but also the possibility, efficacy and cost of preventive actions (Grothmann and Reusswig, 2006; Galve et al., 2016) should landslide events occur must be clearly listed and communicated.
Introducing risk perception as a value for defining landslides as geomorphosites could be a win-win strategy for landslide-risk reduction and management purposes. Having experienced landslide events or another natural hazard event, knowledge of the local geology and geomorphology, and spending time outdoors are factors that significantly increase risk perception (Damm et al., 2013). These can almost all be experienced by accessing a landslide geomorphosite, which can also compensate the lack of the non-desirable experience of a landslide event in a positive way. A landslide geomorphosite can convey information into a form comprehensible to nontechnical users (Giordan et al., 2015), such as the expected likelihood of a landslide event of a certain magnitude at a certain location under certain conditions, and the estimated effects of a landslide event on people, structures, socio-economic activity, and surrounding environment. Communicating these elements via a landslide geomorphosite can make people aware of the risk, more prepared to this type of threat, and willing to participating and supporting policymaker decisions. This is because it has been seen that dissemination campaigns can build more resilient communities (Landeros-Mugica et al., 2016; Righi et al., 2021), promote an inclusive and sustainable collaboration among stakeholders and citizens, who are more likely to integrate with their acquired knowledges those of stakeholders and decision-makers, participate in decisions on land development planning, and positively accept regulation introduced to mitigate natural risks (e.g., Hernández-Moreno and Alcántara-Ayala, 2017).
Examples of Landslide Geomorphosites Worldwide
In this Section, we list four different cases of landslides around the world that embody the aspects presented in Identification of Landslides as Part of the Geoheritage Section, namely past and present climate changes, anthropic signature, and risk perception. We have chosen these examples because of their great scientific value, testified by the vast number of publications regarding them, for their iconicity among the landslide scientific community, and because of their spectacular scenic beauty.
The Frank Slide, Canada
The Frank Slide is located on the east slope of Turtle Mountain in southwest Alberta, Canada (Figure 3). The landslide occurred on 29th April 1903 at 4:10 am local time, and destroyed part of the mining town of Frank, killing more than 90 residents (McConnell and Brock, 1902; Cruden and Krahn, 1973; Cruden and Hungr, 1986). It deposited a debris sheet of ∼37 × 106 m3 (Nicoletti and Sorriso-Valvo, 1991) over an area of ∼2.7 km2 (McConnell and Brock, 1902), with an average thickness of 14 m (Cruden and Hungr, 1986). It consists mainly of limestone of Paleozoic age (Pedrazzini et al., 2011). The Turtle Mountain unstable geological structure was the main preparatory factor for the failure (Cruden, 2004). Fluvial erosion, water action in summit cracks, and unusual weather conditions contributed to the disaster (Beaty, 1987; Cruden, 2004), while underground coal mining remains to be precisely evaluated as a triggering factor (Cruden et al., 2009). Turtle Mountain has been subject to tens of studies (Charrière et al., 2016 and references therein), many of which are recent (Theune et al., 2006; Jaboyedoff et al., 2009; Humair et al., 2013; Froese and Moreno, 2014), which demonstrates the still alive scientific interest around this site.
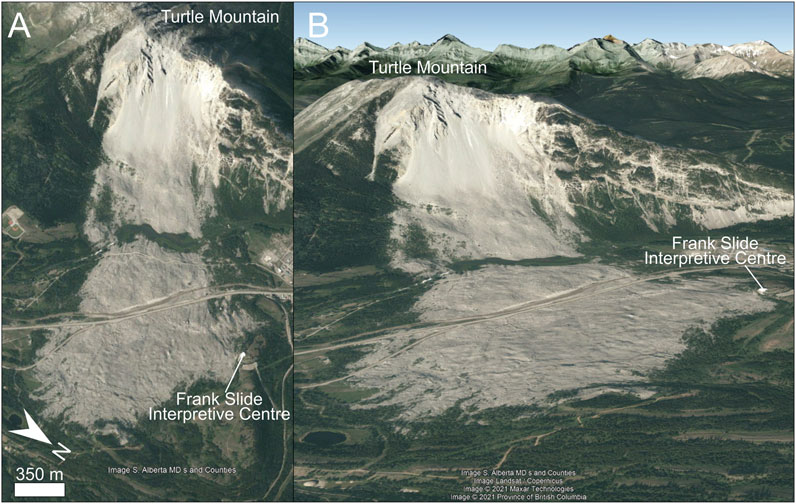
FIGURE 3. Aerial (A) and oblique (B) view of the Frank slide, which detached from Turtle Mountain on 29th April 1903; today the Frank Slide Interpretive Centre is located at the edge of the slide deposits. Images are from Google Earth.
The slide is now a Provincial Heritage Site, and along the northwest edge of the fan sits the Frank Slide Interpretive Centre (Government of Alberta, 2022; Figure 3), where tourists are welcomed and can view the slide from several telescope stations along a walking path running around the centre. To monitor further potential movements, the Turtle Mountain Monitoring Project put in place a long-term deformation, microseismic, hydrological and climatic monitoring systems (Read et al., 2005).
Despite the Interpretative Centre prolifically diffusing a culture of hazard prevention (Read et al., 2005; Cruden et al., 2009; Vallee, 2019) and successfully receiving visitors, the site has never been subject of scientific articles considering the slide as a geomorphosite. The Frank Slide is a site highly valuable in terms of geomorphology, history, natural hazard, scientific research and local tourism. Additionally, in view of the new values to define a landslide geomorphosite that we have listed and described in Identification of Landslides as Part of the Geoheritage Section, we consider this site an exceptional example of landslide geomorphosite with a high “Risk perception” value (Table 3). Visitors to an already well-established heritage site can know not just about the geology, geomorphology, scientific importance and history of this unique landslide, but also be informed about the risk that such phenomena pose to inhabited regions, so that their risk perception can be therefore enhanced. Such a geomorphosite can convey the idea of the danger posed by these phenomena because of the sadly well-known dramatic toll on lives taken by this slide—the Frank Slide is known as the deadliest landslide in Canada—and because visitors become aware that there is an active interest in monitoring and preventing landslide risk in the area via the Turtle Mountain Monitoring Project.

TABLE 3. The chosen landslide examples showcasing the new values for the identification of a landslide geomorphosite.
The Vajont Landslide, Italy
On 9th October 1963 at 10:39 pm local time, a mass of approximately 270–300 million m3 of rock and debris detached from Mount Toc, Dolomites, northeast Italy, and collapsed into the artificial lake created by the 262 m high double curved arch dam built across the Vajont valley. The wave overtopped the dam, destroying seven villages in the Piave River Valley and killing almost 2000 people (Giudici and Semenza, 1960; Ciabatti, 1964; Semenza and Ghirotti, 2000; Genevois and Ghirotti, 2005; Semenza, 2011; Delle Rose, 2012; Superchi, 2012). It is the deadliest landslide event in Europe in recorded history. The Vajont event was a human-induced disaster, as it was caused by failures of experts, who provided inadequate assessment of the possible consequences of damming the valley (UNESCO, 2008).
The Vajont dam is now easily accessible to visitors, who can also visit in Longarone, the first village to be destroyed by the wave, the Museo Longarone Vajont—Attimi di Storia (Museo, 2022). The Vajont landslide (Figure 4) is an outstanding case for a landslide geomorphosite. It has a unique scientific importance, as the complex geology and geomechanics of the landslide have been debated since the occurrence of the event (Hendron and Patton, 1985; Massironi et al., 2013; Paronuzzi et al., 2013; Wolter et al., 2016; Dykes and Bromhead, 2018), which was a turning point in the technical-scientific knowledge of landslides (Genevois and Prestininzi, 2013). The Vajont landslide fulfils two of the values that we have identified for landslide geomorphosites, namely risk perception and anthropic signature (Table 3). On the risk perception side, the Vajont landslide can aid in transmitting knowledge about the risks deriving from such cascading events to visitors. Moreover, it can convey that strengthening the collaboration between local communities, technicians and stakeholders is essential during territorial planning and management, as the opposing voice of inhabitants—who knew about the instability of Mount Toc—to the dam project remained unheard (Bianchizza and Frigerio, 2013). Since it has been demonstrated that the collective memory of the Vajont disaster seems to diminish for people not directly exposed and between generations (Raccanello et al., 2019a), promoting the landslide as a geomorphosite could improve and extend in time the risk perception of such catastrophic phenomena at local and wider levels. The anthropic signature is another tool to amplify the concept of awareness and memory of past disastrous landslides: the extensive scar visible from distance, the huge landslide body, and the dam of the Vajont valley stands as reminder of how frequency, intensity, and especially impact of landslides can be heavily conditioned by anthropic actions. The Vajont landslide has received a permanent attention from the scientific community for nearly 60 years, so we propose as the final outcome of all the knowledge gathered during this time a better perceived landslide risk and awareness of the anthropic signature on the environment by promoting the Vajont as a landslide geomorphosite.
Aoraki/Mount Cook Landslide, New Zealand
On 14th December 1991, a mass of 12 × 106 m3 of rock and ice detached from the east face of Aoraki/Mount Cook, New Zealand (Figure 5). The landslide fell onto the Tasman Glacier, travelled 7.5 km and passed less than 300 m from a mountain hut (McSaveney, 2002; Huggel et al., 2010). The failure lowered the summit of Mount Cook of ∼30 m (McSaveney, 2002) and has significantly affected the flow of Tasman Glacier (Quincey and Glasser, 2009). In this area, large slope instability phenomena are likely linked to active tectonic and climate change (McSaveney, 2002; Allen et al., 2011; Allen and Huggel, 2013). Rapid twentieth-century glacial debuttressing and permafrost warming are thought to have a role in the recent slope movements affecting the Southern Alps of New Zealand (McSaveney, 2002; Allen et al., 2009). It has been observed that unusually warm temperatures occurred days before the Mount Cook event, followed by a sudden drop in temperatures (Huggel et al., 2010). Mount Cook is a spectacular example of how warming temperatures may affect slope stability. The event from 1991 is not the only one in the area, as numerous and frequent rock avalanches have recently affected the Aoraki/Mount Cook area, with the last two occurring in 2013 and 2014 (Cox et al., 2015; Dunning et al., 2015). The frequency of these failures has been already indicated as concerning (Deline et al., 2022), and the management implications of the area in terms of risks associated to rapid glacial recession have already been identified (Purdie et al., 2020).
The area of the landslide hosts the Aoraki Mount Cook National Park, which has a great natural, cultural and tourist value, as it is home to New Zealand’s tallest mountain - Mount Cook is an easily accessible mecca for outdoor lovers, and holds spiritual significance for the indigenous. The Aoraki/Mount Cook is a perfect example of landslide geomorphosite showcasing the climate change value at present time (Table 3). As extreme weather events increase in frequency and magnitude due to global warming, temperature-sensitive high-mountain areas might be increasingly affected by large slope failures (e.g., Huggel et al., 2010, 2012; Stoffel and Huggel, 2012; Stoffel et al., 2014). Therefore, it is crucial to convey to visitors the message that among the cascading consequence of the human-induced present climate change there might catastrophic phenomena such as those in the Aoraki/Mount Cook area, which can affect spectacular landscapes and pose at risk lives and infrastructures. The opportunity for educating visitors on the tangible consequences (glacial retreat) of climate change in the Aoraki Mount Cook National Park (Department of Conservation/Te Papa Atawhai, 2022) has already been successfully explored (Purdie et al., 2020): visitors are aware of glacial recession and climate change, and acknowledge the contribution of human activity to global warming. Using the value of climate change to determine a landslide geomorphosite such as Aoraki/Mount Cook would strengthen an already well-established geosite and is a great opportunity to bring to the attention of the scientific community the importance of landslides in the geoheritage through a striking example.
Diffused Landslides: Tröllaskagi (Iceland) and Malta
Not only single landslides can be identified as geomorphosites, but also areas and regions widely affected by slope movements have a high geoheritage importance, as they can convey the concept that a certain landscape might be or have been in the past prone to the development of frequent and/or numerous mass wasting phenomena. In this subsection, we firstly recount on the case of mass-wasting phenomena in the Tröllaskagi peninsula, northern-central Iceland, and then on the slope failures along the northern coasts of Malta. Although these two case studies might seem different for their geography, climate, geomorphology, geological setting and history, they both show how the consequences of past climate changes have caused large mass movements that have shaped large regions.
Rock avalanches from the early Holocene are widespread in north central Tröllaskagi peninsula of Iceland (Figure 6), particularly on slopes characterised by the weathered Tertiary basalt formation (e.g., Einarsson, 1973; Whalley, et al., 1983). The abundance of slope failures in these areas of Iceland has been linked to post-glacial rebound (Cossart et al., 2014; Feuillet et al., 2014; Coquin et al., 2015, 2016; Decaulne et al., 2016) in response to fast deglaciation during the Late Weichselian, between 18 and 14 ka (Mercier et al., 2012; Mercier et al., 2017). Slope failures take the form of Deep-seated Gravitational Slope Deformations (DGSD) or rock avalanches, and it has been demonstrated that the latter are initiated because gravitational spreading acted as precursor for failures in paraglacial settings (Coquin et al., 2016; Mercier et al., 2017).
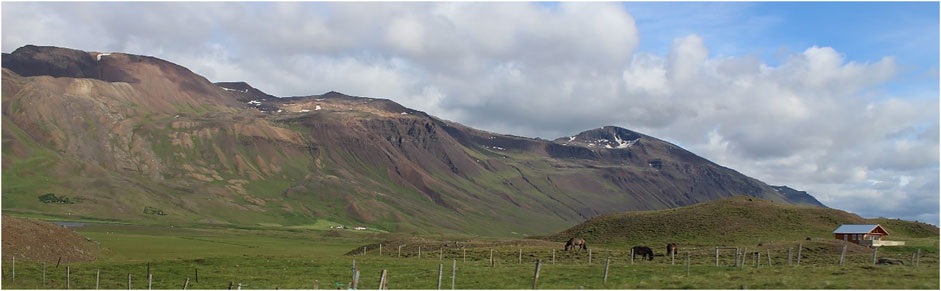
FIGURE 6. Perspective view of one of the rock avalanches shaping the landscape of the Tröllaskagi peninsula. In the background, the slope is affected by several slope failures, while in the foreground the depositional area extending to the plane is characterised by a hummocky morphology (photo by C. Morino).
The spectacular landslides in the Tröllaskagi peninsula have deeply reshaped the local topographic slopes. These large-scale gravitational phenomena are of unique kind because they testify the consequences of past climate change (Table 3), being these slope readjustments driven by debuttressing induced by Late Weichselian deglaciation. This area of Iceland can be therefore indicated as a landslide geomorphosite, allowing visitors to admire the dramatic changes in the topography generated by landslides. Geomorphologists and landslide experts should be aware that also landslides that originated in the past must be included in the geoheritage because they exemplify how variations of climatic conditions generate drastic geomorphological changes in vast areas.
The northern coasts of the Island of Malta are affected by several widespread landslides (Figure 7), mainly consisting of rock spreads evolving into spectacular block slides that characterise the geomorphological landscape, particularly along its north-western coast (Devoto et al., 2012; Soldati et al., 2019). The occurrence of these landslides is mainly controlled by tectonics, lithology and hydrogeology (Magri et al., 2008; Mantovani et al., 2022), and failures have developed through different stages related to post-glacial marine transgression, with the oldest occurring at 21 ka (Soldati et al., 2018).
The area has been included in the UNESCO World Heritage Tentative List and is located in the Il-Majjistral Nature and History Park (Majjistral, 2022), a protected area with natural, cultural and tourist interests. The Park has already been studied for its rich geomorphological heritage (Coratza et al., 2011; Cappadonia et al., 2018), and landslides have already been recognised as geosites as well as features that enhance landscape geodiversity and geotourism (Selmi et al., 2019). This area of Malta is an excellent example of diffused landslide geomorphosite, showing a variety of landslides at different stages of evolution. Furthermore, as for the case of Tröllaskagi, these diffused landslides are the result of the sensitiveness of this coastal territory to changing climate conditions and related sea-level rise, showing that the climate change value is an important one to consider when indicating a landslide geomorphosite (Table 3).
Conclusion
Landslides have been included in the global geoheritage, but so far they have received only marginal attention compared to much more popular and spectacular landforms. We have surveyed the literature to understand to what extent landslides have been considered as part of geoheritage and consequently identified as geosites/geomorphosites. We found that there are few cases of landslides defined as geomorphosites, and that the majority of them are located in Europe. In order to bring the attention of the international landslide community to the importance of landslides in the geoheritage, in addition to the commonly recognised value to define a geosite, we have defined three new values that should be considered when identifying a landslide as a geomorphosite. They include past and present climate changes, anthropic signature and risk perception. These values are strictly linked to each other and of extraordinary relevance today, given the impact that the anthropic activities and human-induced climate change are having on the environments and human communities all over the world. We report on different examples of famous and dramatic landslides around the world that exemplify and incorporate these new values. Many landslide scientists would know countless other cases of spectacular landslides that could be identified and promoted as geomorphosites, giving landslides the deserved role that they should have in the global geoheritage.
Data Availability Statement
The original contributions presented in the study are included in the article/Supplementary material, further inquiries can be directed to the corresponding author.
Author Contributions
CM, PC, and MS conceived and designed the research. CM collected, processed, and analysed the data, and prepared the manuscript. PC and MS significantly contributed to the manuscript preparation.
Funding
CM is funded by the Agence Nationale de la Recherche in the framework of the project ANR-19-CE01-0010 PERMOLARDS.
The research is part of the Project “Coastal risk assessment and mapping” funded by the EUR-OPA Major Hazards Agreement of the Council of Europe (2020–2021). Grant Number: GA/2021/08 No. 689165 (Unimore Unit Resp: Mauro Soldati).
Conflict of Interest
The authors declare that the research was conducted in the absence of any commercial or financial relationships that could be construed as a potential conflict of interest.
Publisher’s Note
All claims expressed in this article are solely those of the authors and do not necessarily represent those of their affiliated organizations, or those of the publisher, the editors and the reviewers. Any product that may be evaluated in this article, or claim that may be made by its manufacturer, is not guaranteed or endorsed by the publisher.
Acknowledgments
We thank the reviewers MN, PM, KN, and AQ-R for the insightful comments that greatly improved the quality of the manuscript.
References
Alcántara-Ayala, I., and Moreno, A. R. (2016). Landslide Risk Perception and Communication for Disaster Risk Management in Mountain Areas of Developing Countries: a Mexican Foretaste. J. Mt. Sci. 13, 2079–2093. doi:10.1007/S11629-015-3823-0
Alimohammadlou, Y., Najafi, A., and Yalcin, A. (2013). Landslide Process and Impacts: A Proposed Classification Method. Catena 104, 219–232. doi:10.1016/j.catena.2012.11.013
Allen, S., and Huggel, C. (2013). Extremely Warm Temperatures as a Potential Cause of Recent High Mountain rockfall. Glob. Planet. Change 107, 59–69. doi:10.1016/j.gloplacha.2013.04.007
Allen, S. K., Cox, S. C., and Owens, I. F. (2011). Rock Avalanches and Other Landslides in the central Southern Alps of New Zealand: A Regional Study Considering Possible Climate Change Impacts. Landslides 8, 33–48. doi:10.1007/s10346-010-0222-z
Allen, S. K., Gruber, S., and Owens, I. F. (2009). Exploring Steep Bedrock Permafrost and its Relationship with Recent Slope Failures in the Southern Alps of New Zealand. Permafrost Periglac. Process. 20, 345–356. doi:10.1002/ppp10.1002/ppp.658
Allison, R. J. (2020). “The Landslides of East Devon and West Dorset,” in Landscapes and Landforms of England and Wales (Springer), 201–213. doi:10.1007/978-3-030-38957-4_11
Amrikazemi, A., and Mehrpooya, A. (2006). “Geotourism Resources of Iran,” in Geotourism. Editors D. Newsome, and R. Dowling (UK: Routledge), 106–120.
Arenson, L. U., and Springman, S. M. (2005). Mathematical Descriptions for the Behaviour of Ice-Rich Frozen Soils at Temperatures Close to 0 °C. Can. Geotech. J. 42, 431–442. doi:10.1139/t04-109
Ballantyne, C. K., Sandeman, G. F., Stone, J. O., and Wilson, P. (2014). Rock-slope Failure Following Late Pleistocene Deglaciation on Tectonically Stable Mountainous Terrain. Quat. Sci. Rev. 86, 144–157. doi:10.1016/j.quascirev.2013.12.021
Bălteanu, D., Micu, M., Jurchescu, M., Malet, J.-P., Sima, M., Kucsicsa, G., et al. (2020). National-scale Landslide Susceptibility Map of Romania in a European Methodological Framework. Geomorphology 371, 107432.
Barmuta, M. (2011). Geotourism during Excursions in the Northeastern Part of the Gorce National Park. Geotourism/Geoturystyka, 51–62.
Bazin, S. (Editor) (2011). SafeLand deliverable 4.8.: Guidelines for landslide monitoring and early warning systems in Europe - Design and required technology. European Project SafeLand, Grant Agreement No. 226479, 153. Available at: http://www.safeland-fp7.eu
Beaty, C. B. (1987). The Frank Slide, Southwestern Alberta. Rocky Mt. Sect. Geol. Soc. Am. Decad. North. Am. Geol. Centen. F. Guid. 2, 15.
Benedek, D. M., Fullerton, C., and Ursano, R. J. (2007). First Responders: Mental Health Consequences of Natural and Human-Made Disasters for Public Health and Public Safety Workers. Annu. Rev. Public Health 28, 55–68. doi:10.1146/annurev.publhealth.28.021406.144037
Bianchizza, C., and Frigerio, S. (2013). Domination of or Adaptation to Nature? A Lesson We Can Still Learn from the Vajont. Ital. J. Eng. Geol. Env. 6, 523–531.
Binal, A., and Ercanoğlu, M. (2010). Assessment of rockfall Potential in the Kula (Manisa, Turkey) Geopark Region. Environ. Earth Sci. 61, 1361–1373. doi:10.1007/s12665-010-0454-1
Bollati, I., Pelfini, M., and Pellegrini, L. (2012). A Geomorphosites Selection Method for Educational Purposes: a Case Study in Trebbia Valley (Emilia Romagna, Italy). Geogr. Fis Din Quat. 35, 23–35.
Bollati, I., Smiraglia, C., and Pelfini, M. (2013). Assessment and Selection of Geomorphosites and Trails in the Miage Glacier Area (Western Italian Alps). Environ. Manage. 51, 951–967. doi:10.1007/s00267-012-9995-2
Bookhagen, B., Thiede, R. C., and Strecker, M. R. (2005). Late Quaternary Intensified Monsoon Phases Control Landscape Evolution in the Northwest Himalaya. Geol 33, 149–152. doi:10.1130/g20982.1
Borgatti, L., and Soldati, M. (2010a). “Landslides and Climatic Change,” in Geomorphological Hazards and Disaster Prevention. Editors I. Alcántara-Ayala, and A. S. Goudie (Cambridge: Cambridge University Press Cambridge), 87–95.
Borgatti, L., and Soldati, M. (2010b). Landslides as a Geomorphological Proxy for Climate Change: a Record from the Dolomites (Northern Italy). Geomorphology 120, 56–64. doi:10.1016/j.geomorph.2009.09.015
Borgatti, L., and Tosatti, G. (2010). Slope instability processes affecting the Pietra di Bismantova geosite (Northern Apennines, Italy). Geoheritage 2, 155–168. doi:10.1007/s12371-010-0023-8
Bouzekraoui, H., Barakat, A., El Youssi, M., Touhami, F., Mouaddine, A., Hafid, A., et al. (2018). Mapping Geosites as Gateways to the Geotourism Management in Central High-Atlas (Morocco). Quaest. Geogr. 37, 87–102. doi:10.2478/quageo-2018-0007
Brilha, J. (2018). “Geoheritage,” in Geoheritage: Assessment, Protection, and Management. Editors E. Reynard, and J. Brilha (Elsevier), 69–85. doi:10.1016/b978-0-12-809531-7.00004-6
Brilha, J. (2016). Inventory and Quantitative Assessment of Geosites and Geodiversity Sites: a Review. Geoheritage 8, 119–134. doi:10.1007/s12371-014-0139-3
Brocx, M., and Semeniuk, V. (2007). Geoheritage and Geoconservation-History, Definition, Scope and Scale. J. R. Soc. West. Aust. 90, 53–87.
Brown, A. G., Tooth, S., Bullard, J. E., Thomas, D. S. G., Chiverrell, R. C., Plater, A. J., et al. (2017). The Geomorphology of the Anthropocene: Emergence, Status and Implications. Earth Surf. Process. Landforms 42, 71–90. doi:10.1002/esp.3943
Brown, E. J., Prosser, C. D., and Stevenson, N. M. (2012). Geodiversity, Conservation and Climate Change: Key Principles for Adaptation. Scottish Geographical J. 128, 234–239. doi:10.1080/14702541.2012.725859
Brunsden, D., and Edmonds, R. (2009). “The Dorset and East Devon Coast: England's Geomorphological World Heritage Site,” in Geomorphological Landscapes of the World (Springer), 211–221. doi:10.1007/978-90-481-3055-9_22
Burek, C. V., and Prosser, C. D. (2008). The History of Geoconservation: an Introduction. Geol. Soc. Lond. Spec. Publications 300, 1–5. doi:10.1144/sp300.1
Burlando, M., Firpo, M., Queirolo, C., Rovere, A., and Vacchi, M. (2011). From Geoheritage to Sustainable Development: Strategies and Perspectives in the Beigua Geopark (Italy). Geoheritage 3, 63–72. doi:10.1007/s12371-010-0019-4
Burt, T. P., Warburton, J., and Allison, R. J. (2002). “Eroding landscapes? Past, Present and Future Perspectives,” in The British uplands: dynamics of change. JNCC Report No. 319. Editors T. P. Burt, D. B. A. Thompson, and J. Warburton (Peterborough: Joint Nature Conservation Committee), 60–66.
Bustillos Ardaya, A., Evers, M., and Ribbe, L. (2017). What influences disaster risk perception? Intervention measures, flood and landslide risk perception of the population living in flood risk areas in Rio de Janeiro state, Brazil. Int. J. Disaster Risk Reduction 25, 227–237. doi:10.1016/j.ijdrr.2017.09.006
Caine, N. (1980). The Rainfall Intensity - Duration Control of Shallow Landslides and Debris Flows. Geografiska Annaler: Ser. A, Phys. Geogr. 62, 23–27. doi:10.1080/04353676.1980.11879996
Calaforra, J. M., and Fernández-Cortés, Á. (2006). “Geotourism in Spain: Resources and Environmental Management,” in Geotourism. Editors D. Newsome, and R. Dowling (UK: Routledge), 227–248.
Calcaterra, D., Guida, D., Budetta, P., De Vita, P., Di Martire, D., and Aloia, A. (2014). “Moving Geosites: How Landslides Can Become Focal Points in Geoparks,” in Latest Trends in Engineering Mechanics, Structures, Engineering Geology, Proceedings of the 7th International Conference on Engineering Mechanics, Structures, Engineering Geology (EMESEG 14) (Italy: Salerno), 162–171.
Calvello, M., Papa, M. N., Pratschke, J., and Nacchia Crescenzo, M. (2016). Landslide Risk Perception: a Case Study in Southern Italy. Landslides 13, 349–360. doi:10.1007/s10346-015-0572-7
Cappadonia, C., Coratza, P., Agnesi, V., and Soldati, M. (2018). Malta and Sicily Joined by Geoheritage Enhancement and Geotourism within the Framework of Land Management and Development. Geosciences 8, 253. doi:10.3390/geosciences8070253
Charrière, M., Humair, F., Froese, C., Jaboyedoff, M., Pedrazzini, A., and Longchamp, C. (2016). From the Source Area to the deposit: Collapse, Fragmentation, and Propagation of the Frank Slide. Bulletin 128, 332–351.
Chlachula, J. (2020). Geoheritage of East Kazakhstan. Geoheritage 12, 1–17. doi:10.1007/s12371-020-00514-y
Chrobak, A., and Cebulski, J. (2014). Landslides in the Polish Carpathians as the Potential Educational Geosites. Curr. Issues Tour. Res. 4, 38–49.
Chrobak, A. (2016). Valorisation and Categorisation of the Geosites in the Podtatrze Area. Southern Poland: Geotourism/Geoturystyka.
Cocean, P., Hognogi, G.-G., Pop, A.-M., Bejan, I., and David, N. (2019). Anthropic Valorisation of Vulnerable Areas Affected by Deep-Seated Landslides. Geoheritage 11, 1855–1868. doi:10.1007/s12371-019-00397-8
Coquin, J., Mercier, D., Bourgeois, O., Cossart, E., and Decaulne, A. (2015). Gravitational Spreading of Mountain Ridges Coeval with Late Weichselian Deglaciation: Impact on Glacial Landscapes in Tröllaskagi, Northern Iceland. Quat. Sci. Rev. 107, 197–213. doi:10.1016/j.quascirev.2014.10.023
Coquin, J., Mercier, D., Bourgeois, O., Feuillet, T., and Decaulne, A. (2016). Is Gravitational Spreading a Precursor for the Stífluhólar Landslide (Skagafjörður, Northern Iceland)? geomorphologie 22, 9–24. doi:10.4000/geomorphologie.11295
Coratza, P., Bruschi, V. M., Piacentini, D., Saliba, D., and Soldati, M. (2011). Recognition and Assessment of Geomorphosites in Malta at the Il-Majjistral Nature and History Park. Geoheritage 3, 175–185. doi:10.1007/s12371-011-0034-0
Coratza, P., and De Waele, J. (2012). Geomorphosites and Natural Hazards: Teaching the Importance of Geomorphology in Society. Geoheritage 4, 195–203. doi:10.1007/s12371-012-0058-0
Coratza, P., and Hobléa, F. (2018). “The Specificities of Geomorphological Heritage,” in Geoheritage: Assessment, Protection, and Management. Editors E. Reynard, and J. Brilha (Elsevier), 87–106. doi:10.1016/b978-0-12-809531-7.00005-8
Coratza, P., Vandelli, V., and Soldati, M. (2018). Environmental Rehabilitation Linking Natural and Industrial Heritage: a Master Plan for Dismissed Quarry Areas in the Emilia Apennines (Italy). Environ. Earth Sci. 77, 1–16. doi:10.1007/s12665-018-7642-9
Cossart, E., Braucher, R., Fort, M., Bourlès, D. L., and Carcaillet, J. (2008). Slope Instability in Relation to Glacial Debuttressing in alpine Areas (Upper Durance Catchment, southeastern France): Evidence from Field Data and 10Be Cosmic ray Exposure Ages. Geomorphology 95, 3–26. doi:10.1016/j.geomorph.2006.12.022
Cossart, E., Mercier, D., Decaulne, A., Feuillet, T., Jónsson, H. P., and Saemundsson, Þ. (2014). Impacts of post-glacial Rebound on Landslide Spatial Distribution at a Regional Scale in Northern Iceland (Skagafjörður). Earth Surf. Process. Landforms 39, 336–350. doi:10.1002/esp.3450
Cox, S. C., McSaveney, M. J., Spencer, J., Allen, S. K., Ashraf, S., Hancox, G. T., et al. (2015). Rock Avalanche on 14 July 2014 from Hillary Ridge, Aoraki/Mount Cook, New Zealand. Landslides 12, 395–402. doi:10.1007/s10346-015-0556-7
Crozier, M. J. (2010). Deciphering the Effect of Climate Change on Landslide Activity: A Review. Geomorphology 124, 260–267. doi:10.1016/j.geomorph.2010.04.009
Cruden, D. M. (1991). A Simple Definition of a Landslide. Bull. Int. Assoc. Eng. Geology. 43, 27–29. doi:10.1007/bf02590167
Cruden, D. M. (2004). “Before the Frank Slide: Preparatory and Triggering Causes from Maps and Photographs,” in Proceedings of the 57th Canadian Geotechnical Conference and the 5th Joint CGS/IAH-CNC Conference (IEEE), 1–6.
Cruden, D. M., and Hungr, O. (1986). The Debris of the Frank Slide and Theories of Rockslide-Avalanche Mobility. Can. J. Earth Sci. 23, 425–432. doi:10.1139/e86-044
Cruden, D. M., and Krahn, J. (1973). A Reexamination of the Geology of the Frank Slide. Can. Geotech. J. 10, 581–591. doi:10.1139/t73-054
Cruden, D. M., Martin, C. D., Culshaw, M. G., Reeves, H. J., Jefferson, I., and Spink, T. W. (2009). A century of Risk Management at the Frank Slide, Canada. Eng. Geol. Tomorrow’s Cities Geol. Soc. London Spec. Publ., 22.
Cruden, D. M., and Varnes, D. (1996). “Landslide Types and Processes,” in Landslides Investigation and Mitigation. Editors A. Turner, and R. Shuster (Washington, DC: IEEE), 36–75.
Damm, A., Eberhard, K., Sendzimir, J., and Patt, A. (2013). Perception of Landslides Risk and Responsibility: a Case Study in Eastern Styria, Austria. Nat. Hazards 69, 165–183. doi:10.1007/s11069-013-0694-y
Davies, M. C. R., Hamza, O., and Harris, C. (2001). The Effect of Rise in Mean Annual Temperature on the Stability of Rock Slopes Containing Ice-Filled Discontinuities. Permafrost Periglac. Process. 12, 137–144. doi:10.1002/ppp10.1002/ppp.378
De Wever, P., Alterio, I., Egoroff, G., Cornée, A., Bobrowsky, P., Collin, G., et al. (2015). Geoheritage, a National Inventory in France. Geoheritage 7, 205–247. doi:10.1007/s12371-015-0151-2
Dearing, J. A., Battarbee, R. W., Dikau, R., Larocque, I., and Oldfield, F. (2006). Human-environment Interactions: Learning from the Past. Reg. Environ. Change 6, 1–16. doi:10.1007/s10113-005-0011-8
Dearing, J. A., Braimoh, A. K., Reenberg, A., Turner, B. L., and Van der Leeuw, S. (2010). Complex Land Systems: the Need for Long Time Perspectives to Assess Their Future. Ecol. Soc. 15, 1–19. doi:10.5751/es-03645-150421
Decaulne, A., Cossart, E., Mercier, D., Feuillet, T., Coquin, J., and Jónsson, H. P. (2016). An Early Holocene Age for the Vatn Landslide (Skagafjörður, central Northern Iceland): Insights into the Role of Postglacial Landsliding on Slope Development. The Holocene 26, 1304–1318. doi:10.1177/0959683616638432
Del Monte, M., D’Orefice, M., Luberti, G. M., Marini, R., Pica, A., and Vergari, F. (2016). Geomorphological Classification of Urban Landscapes: the Case Study of Rome (Italy). J. Maps 12, 178–189. doi:10.1080/17445647.2016.1187977
Deline, P., Gruber, S., Delaloye, R., Fischer, L., Geertsema, M., Giardino, M., et al. (2015). “Ice Loss and Slope Stability in High-Mountain Regions,” in Snow and Ice-Related Hazards, Risks and Disasters (Elsevier), 521–561. doi:10.1016/b978-0-12-394849-6.00015-9
Deline, P., Hewitt, K., Shugar, D., and Reznichenko, N. (2022). “Rock Avalanches onto Glaciers,” in Landslide Hazards, Risks, and Disasters (Elsevier), 269–333. doi:10.1016/b978-0-12-818464-6.00010-x
Delle Rose, M. (2012). Some Insights about the Relation Among Seismic Activity, Tectonic Structures and Rockslide Kinematics at the Vajont Dam Site. Bull. Seismol. Soc. Am. 94 (1), 44–50.
Department of Conservation/Te Papa Atawhai (2022). Department of Conservation/Te Papa Atawhai. Available at: https://www.doc.govt.nz/parks-and-recreation/places-to-go/canterbury/places/aoraki-mount-cook-national-park/?tab-id=50578.
Devoto, S., Biolchi, S., Bruschi, V. M., Furlani, S., Mantovani, M., Piacentini, D., et al. (2012). Geomorphological Map of the NW Coast of the Island of Malta (Mediterranean Sea). J. Maps 8, 33–40. doi:10.1080/17445647.2012.668425
Dunning, S. A., Rosser, N. J., McColl, S. T., and Reznichenko, N. V. (2015). Rapid Sequestration of Rock Avalanche Deposits within Glaciers. Nat. Commun. 6, 1–7. doi:10.1038/ncomms8964
Dykes, A. P., and Bromhead, E. N. (2018). New, Simplified and Improved Interpretation of the Vaiont Landslide Mechanics. Landslides 15, 2001–2015. doi:10.1007/s10346-018-0998-9
Einarsson, T. (1973). “Geology of Iceland: Regional Arctic Geology of the Nordic Countries,” in Arctic Geology. Editor M. Pitcher (Mere Am Assoc Pet Geol), 171–175.
Erikstad, L. (2008). History of Geoconservation in Europe. Geol. Soc. Lond. Spec. Publications 300, 249–256. doi:10.1144/sp300.19
Faccini, F., Gabellieri, N., Paliaga, G., Piana, P., Angelini, S., and Coratza, P. (2018). Geoheritage Map of the Portofino Natural Park (Italy). J. Maps 14, 87–96. doi:10.1080/17445647.2018.1433561
Fassoulas, C., Mouriki, D., Dimitriou-Nikolakis, P., and Iliopoulos, G. (2012). Quantitative Assessment of Geotopes as an Effective Tool for Geoheritage Management. Geoheritage 4, 177–193. doi:10.1007/s12371-011-0046-9
Fepuleai, A., Németh, K., and Muliaina, T. (2021). Geopark Impact for the Resilience of Communities in Samoa, SW Pacific. Geoheritage 13, 1–23. doi:10.1007/s12371-021-00578-4
Fepuleai, A., and Németh, K. (2019). Volcanic Geoheritage of Landslides and Rockfalls on a Tropical Ocean Island (Western Samoa, SW Pacific). Geoheritage 11, 577–596. doi:10.1007/s12371-018-0306-z
Ferrero, E., Giardino, M., Lozar, F., Giordano, E., Belluso, E., and Perotti, L. (2012). Geodiversity Action Plans for the Enhancement of Geoheritage in the Piemonte Region (north-western Italy). Ann: Geophys, 55.
Feuillet, T., Coquin, J., Mercier, D., Cossart, E., Decaulne, A., Jónsson, H. P., et al. (2014). Focusing on the Spatial Non-stationarity of Landslide Predisposing Factors in Northern Iceland. Prog. Phys. Geogr. Earth Environ. 38, 354–377. doi:10.1177/0309133314528944
Finney, S. C., and Hilario, A. (2018). “GSSPs as International Geostandards and as Global Geoheritage,” in Geoheritage (Elsevier), 179–189. doi:10.1016/b978-0-12-809531-7.00010-1
Fischhoff, B., Lichtenstein, S., Derby, S. L., Slovic, P., and Keeney, R. (1983). Acceptable Risk. Cambridge University Press.
Frey, M.-L., Schäfer, K., Büchel, G., and Patzak, M. (2006). in Geoparks–a Regional, European and Global Policy. Geotourism. Editors D. Newsome, and R. Dowling (UK: Routledge), 123–145.
Frodella, W., Elashvili, M., Spizzichino, D., Gigli, G., Adikashvili, L., Vacheishvili, N., et al. (2020). Combining Infrared Thermography and Uav Digital Photogrammetry for the protection and Conservation of Rupestrian Cultural Heritage Sites in Georgia: A Methodological Application. Remote Sensing 12, 892. doi:10.3390/rs12050892
Froese, C. R., and Moreno, F. (2014). Structure and Components for the Emergency Response and Warning System on Turtle Mountain, Alberta, Canada. Nat. Hazards 70, 1689–1712. doi:10.1007/s11069-011-9714-y
Froude, M. J., and Petley, D. N. (2018). Global Fatal Landslide Occurrence from 2004 to 2016. Nat. Hazards Earth Syst. Sci. 18, 2161–2181. doi:10.5194/nhess-18-2161-2018
Fuertes-Gutiérrez, I., and Fernández-Martínez, E. (2010). Geosites Inventory in the Leon Province (Northwestern Spain): a Tool to Introduce Geoheritage into Regional Environmental Management. Geoheritage 2, 57–75.
Fukuoka, H., Sassa, K., Wang, G., Wang, F., Wang, Y., and Tian, Y. (2005). “Landslide Risk Assessment and Disaster Management in the Imperial Resort Palace of Lishan, Xian, China (C101-4),” in Landslides. Editors K. Sassa, H. Fukuoka, F. Wang, and G. Wang (Berlin: Springer), 81–89.
Gałaś, A., Paulo, A., Gaidzik, K., Zavala, B., Kalicki, T., Churata, D., et al. (2018). Geosites and Geotouristic Attractions Proposed for the Project Geopark Colca and Volcanoes of Andagua, Peru. Geoheritage 10, 707–729.
Gallois, R. (2010). The Failure Mechanism of the 1839 Bindon Landslide. Devon, UK: almost right first time.
Galve, J. P., Cevasco, A., Brandolini, P., Piacentini, D., Azañón, J. M., Notti, D., et al. (2016). Cost-based Analysis of Mitigation Measures for Shallow-Landslide Risk Reduction Strategies. Eng. Geology. 213, 142–157. doi:10.1016/j.enggeo.2016.09.002
Garavaglia, V., Pelfini, M., and Bollati, I. (2010). The Influence of Climate Change on Glacier Geomorphosites: the Case of Two Italian Glaciers (Miage Glacier, Forni Glacier)investigated through Dendrochronology. geomorphologie 16, 153–164. doi:10.4000/geomorphologie.7895
Garcia, M. D. G. M., Lama, E. A. D., Martins, L., Mazoca, C. E. M., and Bourotte, C. L. M. (2019). Inventory and Assessment of Geosites to Stimulate Regional Sustainable Management: the Northern Coast of the State of São Paulo, Brazil. Acad. Bras. Cienc. 91, e20180514. doi:10.1590/0001-3765201920180514
Gariano, S. L., and Guzzetti, F. (2016). Landslides in a Changing Climate. Earth-Science Rev. 162, 227–252. doi:10.1016/j.earscirev.2016.08.011
Garrick, B. J., and Gekler, W. C. (2013). The Analysis, Communication, and Perception of Risk. Springer Science & Business Media.
Geertsema, M., Menounos, B., Bullard, G., Carrivick, J. L., Clague, J. J., Dai, C., et al. (2020). “landslide, tsunami, and outburst flood‐a hazard cascade associated with rapid deglaciation at Elliot Creek, British Columbia, Canada,” in Geophysical Research Letters. doi:10.1029/2021GL096716
Genevois, R., and Prestininzi, A. (Edition) (2013). International Conference on Vajont ‐ 1963‐2013: thoughts and analyses after 50 years since the catastrophic landslide. Italian Journal of Engineering Geology and Environment ‐ Book Series No.6. Rome: Sapienza Universitá Editrice.
Geopoderes (2022). Geoheritage for Geohazard Resilience and Bringing Geology & Geoheritage to Life. Available at: https://www.geopoderes.com.
Ghislain, Z. T., Guimolaire, N. D., Armand, K. D., Merlin, G. D., and Pierre, K. (2014). Study of Multi-Origin Hazards and Assessment of Associated Risks in the Lefo Caldera (Bamenda Volcano, Cameroon Line). Ijg 05, 1300–1314. doi:10.4236/ijg.2014.511107
Giordan, D., Manconi, A., Allasia, P., and Bertolo, D. (2015). Brief Communication: On the rapid and efficient monitoring results dissemination in landslide emergency scenarios: The Mont de la Saxe case study. Nat. Hazards Earth Syst. Sci. 15, 2009–2017. doi:10.5194/NHESS-15-2009-2015
Giudici, F., and Semenza, E. (1960). Studio geologico del serbatoio del Vajoint. Unpubl. Rep. Sade. Part. A. 21.
Glade, T., and Crozier, M. J. (2010). Landslide Geomorphology in a Changing Environment. Geomorphology 120, 1–2. doi:10.1016/j.geomorph.2009.09.018
Gordon, J. E., Crofts, R., Gray, M., and Tormey, D. (2021). Including Geoconservation in the Management of Protected and Conserved Areas Matters for All of Nature and People. Int. J. Geoheritage Park. 9, 323–334. doi:10.1016/j.ijgeop.2021.05.003
Government of Alberta (2022). Frank Slide Interpretive Centre. Available at: https://frankslide.ca/.
Grämiger, L. M., Moore, J. R., Gischig, V. S., and Loew, S. (2018). Thermomechanical Stresses Drive Damage of Alpine valley Rock walls during Repeat Glacial Cycles. J. Geophys. Res. Earth Surf. 123, 2620–2646.
Gravina, T., Figliozzi, E., Mari, N., and De Luca Tupputi Schinosa, F. (2017). Landslide Risk Perception in Frosinone (Lazio, Central Italy). Landslides 14, 1419–1429. doi:10.1007/s10346-016-0787-2
Gray, M., Gordon, J. E., and Brown, E. J. (2013). Geodiversity and the Ecosystem Approach: the Contribution of Geoscience in Delivering Integrated Environmental Management. Proc. Geologists' Assoc. 124, 659–673. doi:10.1016/j.pgeola.2013.01.003
Gray, M. (2011). Other Nature: Geodiversity and Geosystem Services. Envir. Conserv. 38, 271–274. doi:10.1017/s0376892911000117
Grothmann, T., and Reusswig, F. (2006). People at Risk of Flooding: Why Some Residents Take Precautionary Action while Others Do Not. Nat. Hazards 38, 101–120. doi:10.1007/s11069-005-8604-6
Guilbaud, M., van Wyk de Vries, B., Nemeth, K., Vereb, V., Hagos, M., Manrique, N., et al. (2020). “Unesco Igcp Project 692. Geoheritage for Geohazard Resilience: a Global Initiative to Share Knowledge, Raise Awareness and Communicate about Natural Hazards,” in Oxford Geoheritage Virtual Conference (Oxford: Springer), 67–68.
Guns, M., and Vanacker, V. (2013). Forest Cover Change Trajectories and Their Impact on Landslide Occurrence in the Tropical Andes. Environ. Earth Sci. 70, 2941–2952. doi:10.1007/s12665-013-2352-9
Guzzetti, F. (2000). Landslide Fatalities and the Evaluation of Landslide Risk in Italy. Eng. Geology. 58, 89–107. doi:10.1016/s0013-7952(00)00047-8
Guzzetti, F., Peruccacci, S., Rossi, M., and Stark, C. P. (2008). The Rainfall Intensity-Duration Control of Shallow Landslides and Debris Flows: an Update. Landslides 5, 3–17. doi:10.1007/s10346-007-0112-1
Haque, U., Da Silva, P. F., Devoli, G., Pilz, J., Zhao, B., Khaloua, A., et al. (2019). The Human Cost of Global Warming: Deadly Landslides and Their Triggers (1995-2014). Sci. Total Environ. 682, 673–684. doi:10.1016/j.scitotenv.2019.03.415
Harris, C., Arenson, L. U., Christiansen, H. H., Etzelmüller, B., Frauenfelder, R., Gruber, S., et al. (2009). Permafrost and Climate in Europe: Monitoring and Modelling thermal, Geomorphological and Geotechnical Responses. Earth-Science Rev. 92, 117–171. doi:10.1016/j.earscirev.2008.12.002
Harris, C. (2005). “Climate Change, Mountain Permafrost Degradation and Geotechnical hazard,” in Global Change and Mountain Regions (Dordrecht: Springer), 215–224.
Hendron, A. J., and Patton, F. D. (1985). The Vaiont Slide, a geotechnical analysis based on how geologic observations of the failure surface. U.S. Army Corps of Engineers Report 85, Number 5, 104p.
Henriques, M. H., dos Reis, R. P., Brilha, J., and Mota, T. (2011). Geoconservation as an Emerging Geoscience. Geoheritage 3, 117–128. doi:10.1007/s12371-011-0039-8
Hermanns, R. L., Schleier, M., Böhme, M., Blikra, L. H., Gosse, J., Ivy-Ochs, S., et al. (2017). “Rock-avalanche Activity in W and S Norway Peaks after the Retreat of the Scandinavian Ice Sheet,” in Workshop on World Landslide Forum (Springer), 331–338. doi:10.1007/978-3-319-53483-1_39
Hernández-Moreno, G., and Alcántara-Ayala, I. (2017). Landslide Risk Perception in Mexico: a Research Gate into Public Awareness and Knowledge. Landslides 14, 351–371.
Herrera, G., Mateos, R. M., García-Davalillo, J. C., Grandjean, G., Poyiadji, E., Maftei, R., et al. (2018). Landslide Databases in the Geological Surveys of Europe. Landslides 15, 359–379. doi:10.1007/s10346-017-0902-z
Highland, L. M., Godt, J. W., Howell, D., and Savage, W. (1998). El Nino 1997-98; Damaging Landslides in the San Francisco Bay Area. Denver, CO: US Dept. of the Interior, US Geological Survey, National Landslide, 2327–6932.
Huggel, C., Clague, J. J., and Korup, O. (2012). Is Climate Change Responsible for Changing Landslide Activity in High Mountains? Earth Surf. Process. Landforms 37, 77–91. doi:10.1002/esp.2223
Huggel, C., Gruber, S., Korup, O., and Shroder, J. F. (2013). “13.17 Landslide Hazards and Climate Change in High Mountains,” in Reference Module in Earth Systems and Environmental Sciences, from Treatise on Geomorphology. Editor J. F. Shroder (Amsterdam: Elsevier), 288–301. doi:10.1016/b978-0-12-374739-6.00367-5
Huggel, C., Salzmann, N., Allen, S., Caplan-Auerbach, J., Fischer, L., Haeberli, W., et al. (2010). Recent and Future Warm Extreme Events and High-Mountain Slope Stability. Phil. Trans. R. Soc. A. 368, 2435–2459. doi:10.1098/rsta.2010.0078
Humair, F., Pedrazzini, A., Epard, J.-L., Froese, C. R., and Jaboyedoff, M. (2013). Structural Characterization of Turtle Mountain Anticline (Alberta, Canada) and Impact on Rock Slope Failure. Tectonophysics 605, 133–148. doi:10.1016/j.tecto.2013.04.029
Hungr, O., Leroueil, S., and Picarelli, L. (2014). The Varnes Classification of Landslide Types, an Update. Landslides 11, 167–194. doi:10.1007/s10346-013-0436-y
Huss, M., and Hock, R. (2015). A New Model for Global Glacier Change and Sea-Level Rise. Front. Earth Sci. 3, 54. doi:10.3389/feart.2015.00054
Ivchenko, A., and Gerasimenko, N. (1999). Geosites of the Ukrainian Carpathians-Draft Candidate List. Polish Geol. Inst. Spec. Pap. 2, 47–52.
Jaboyedoff, M., Couture, R., and Locat, P. (2009). Structural Analysis of Turtle Mountain (Alberta) Using Digital Elevation Model: toward a Progressive Failure. Geomorphology 103, 5–16. doi:10.1016/j.geomorph.2008.04.012
Jaboyedoff, M., Michoud, C., Derron, M.-H., Voumard, J., Leibundgut, G., Sudmeier-Rieux, K., et al. (2018). “Human-induced Landslides: toward the Analysis of Anthropogenic Changes of the Slope Environment,” in Landslides and Engineered Slopes. Experience, Theory and Practice. Editors S. Aversa, L. Cascini, L. Picarelli, and C. Scavia (Lonfon: CRC Press), 217–232. doi:10.1201/9781315375007-12
Jaedicke, C., Van Den Eeckhaut, M., Nadim, F., Hervás, J., Kalsnes, B., Vangelsten, B. V., et al. (2014). Identification of Landslide hazard and Risk ‘hotspots’ in Europe. Bull. Eng. Geol. Environ. 73, 325–339.
Jianjun, J., Xun, Z., and Youfang, C. (2006). “Geological Heritage in China,” in Geotourism. Editors D. Newsome, and R. Dowling (Oxford: Elsevier Butterworth-Heinemann), 140–154. doi:10.1016/b978-0-7506-6215-4.50016-6
Jurassiccoast (2022). Jurassic Coast Trust. Available at: https://jurassiccoast.org/news-events/jurassic-coast-artist-collaboration-takes-shape/.
Keskin Citiroglu, H., Isik, S., and Pulat, O. (2017). Utilizing the Geological Diversity for Sustainable Regional Development, a Case Study-Zonguldak (NW Turkey). Geoheritage 9, 211–223. doi:10.1007/s12371-016-0196-x
Korup, O., Görüm, T., and Hayakawa, Y. (2012). Without Power? Landslide Inventories in the Face of Climate Change. Earth Surf. Process. Landforms 37, 92–99. doi:10.1002/esp.2248
Kos, A., Amann, F., Strozzi, T., Delaloye, R., von Ruette, J., and Springman, S. (2016). Contemporary Glacier Retreat Triggers a Rapid Landslide Response, Great Aletsch Glacier, Switzerland. Geophys. Res. Lett. 43, 12–466. doi:10.1002/2016gl071708
Kropáček, J., Vilímek, V., and Mehrishi, P. (2021). A Preliminary Assessment of the Chamoli Rock and Ice Avalanche in the Indian Himalayas by Remote Sensing. Springer.
Kubalíková, L., and Kirchner, K. (2016). Geosite and Geomorphosite Assessment as a Tool for Geoconservation and Geotourism Purposes: a Case Study from Vizovická Vrchovina Highland (Eastern Part of the Czech Republic). Geoheritage 8, 5–14.
Kuhn, D., Hermanns, R. L., Torizin, J., Fuchs, M., Redfield, T., Eilertsen, R., et al. (2020). “Forkastningsfjellet Rock Slide, Spitsbergen: State of Activity in a Changing Climate,” in Understanding and Reducing Landslide Disaster Risk. WLF 2020. ICL Contribution to Landslide Disaster Risk Reduction. Editors V. Vilímek, F. Wang, A. Strom, K. Sassa, P. Bobrowsky, and K. Takara (Springer), 183–189. doi:10.1007/978-3-030-60319-9_21
Landeros-Mugica, K., Urbina-Soria, J., and Alcántara-Ayala, I. (2016). The Good, the Bad and the Ugly: on the Interactions Among Experience, Exposure and Commitment with Reference to Landslide Risk Perception in México. Nat. Hazards 80, 1515–1537. doi:10.1007/s11069-015-2037-7
Larsen, M. C., and Simon, A. (1993). A Rainfall Intensity-Duration Threshold for Landslides in a Humid-Tropical Environment, Puerto Rico. Geografiska Annaler. Ser. A, Phys. Geogr. 75, 13–23. doi:10.2307/521049
Larwood, J. G., Badman, T., and McKeever, P. J. (2013). The Progress and Future of Geoconservation at a Global Level. Proc. Geologists' Assoc. 124, 720–730. doi:10.1016/j.pgeola.2013.04.001
Lazzari, M., Geraldi, E., Lapenna, V., and Loperte, A. (2006). Natural Hazards vs Human Impact: an Integrated Methodological Approach in Geomorphological Risk Assessment on the Tursi Historical Site, Southern Italy. Landslides 3, 275–287. doi:10.1007/s10346-006-0055-y
Leshchinsky, B., Olsen, M. J., Mohney, C., Glover-Cutter, K., Crook, G., Allan, J., et al. (2017). Mitigating Coastal Landslide Damage. Science 357, 981–982. doi:10.1126/science.aao1722
Magnin, F., Etzelmüller, B., Westermann, S., Isaksen, K., Hilger, P., and Hermanns, R. L. (2019). Permafrost Distribution in Steep Slopes in Norway: Measurements, Statistical Modelling and Geomorphological Implication. Earth Surf. Dyn. Discuss. 7, 1–35. doi:10.5194/esurf-2018-90
Magri, O., Mantovani, M., Pasuto, A., and Soldati, M. (2008). Geomorphological Investigation and Monitoring of Lateral Spreading along the north-west Coast of Malta. Geogr. Fis. e Din. Quat. 31, 171–180.
Majjistral (2022). Il-Majjistral Nature and History Park. Available at: https://majjistral.org/.
Mantovani, M., Bossi, G., Dykes, A. P., Pasuto, A., Soldati, M., and Devoto, S. (2022). “Coupling Long-term GNSS Monitoring and Numerical Modelling of Lateral Spreading for Hazard Assessment Purposes,” in Engineering Geology 296, 106466. doi:10.1016/j.enggeo.2021.106466
Margielewski, W., and Alexandrowicz, Z. (2004). Diversity of Landslide Morphology as a Part of Geoconservation Pattern in the Polish Carpathians. Polish Geol. Inst. Spec. Pap. 13, 65–71.
Margottini, C. (2013). “The Monsters grove of Bomarzo (Central Italy): from Rock Fall to Landscape Architecture,” in Landslide Science and Practice (Springer), 511–519. doi:10.1007/978-3-642-31319-6_67
Margottini, C., and Vilímek, V. (2014). The ICL Network on "Landslides and Cultural & Natural Heritage (LACUNHEN)". Landslides 11, 933–938. doi:10.1007/s10346-014-0510-0
Massironi, M., Zampieri, D., Superchi, L., Bistacchi, A., Ravagnan, R., Bergamo, A., et al. (2013). Geological Structures of the Vajont Landslide. Ital. J. Eng. Geol. Environ. 2013, 573–582.
Masson-Delmotte, V., Zhai, P., Pirani, A., Connors, S. L., Péan, C., Berger, S., et al. (2021). IPCC, 2021: Climate Change 2021: The Physical Science Basis. Contribution of Working Group I to the Sixth Assessment Report of the Intergovernmental Panel on Climate Change. Available at: https://www.ipcc.ch/report/ar6/wg1/(Accessed September 24, 2021).
May, V. (2015). Coastal Cliff Conservation and Management: the Dorset and East Devon Coast World Heritage Site. J. Coast. Conserv. 19, 821–829. doi:10.1007/s11852-014-0338-8
McColl, S. T. (2012). Paraglacial Rock-Slope Stability. Geomorphology 153-154, 1–16. doi:10.1016/j.geomorph.2012.02.015
McConnell, R. G., and Brock, R. W. (1902). Report on the Great Landslide at Frank, Alberta, Canada. Can. Dep. Inter. Annu. Rep. 14, 97–112. doi:10.1016/B978-0-444-41507-3.50010-6
McSaveney, M. J. (2002). Recent Rockfalls and Rock Avalanches in Mount Cook national park, New Zealand. Rev. Eng. Geol. 15, 35–70. doi:10.1130/reg15-p35
Mercier, D., Coquin, J., Feuillet, T., Decaulne, A., Cossart, E., Jónsson, H. P., et al. (2017). Are Icelandic Rock-Slope Failures Paraglacial? Age Evaluation of Seventeen Rock-Slope Failures in the Skagafjörður Area, Based on Geomorphological Stacking, Radiocarbon Dating and Tephrochronology. Geomorphology 296, 45–58. doi:10.1016/j.geomorph.2017.08.011
Mercier, D., Cossart, E., Decaulne, A., Feuillet, T., Jónsson, H. P., and Sæmundsson, Þ. (2012). The Höfðahólar Rock Avalanche (Sturzström): Chronological Constraint of Paraglacial Landsliding on an Icelandic Hillslope. The Holocene 23, 432–446. doi:10.1177/0959683612463104
Meusburger, K., and Alewell, C. (2008). Impacts of Anthropogenic and Environmental Factors on the Occurrence of Shallow Landslides in an alpine Catchment (Urseren Valley, Switzerland). Nat. Hazards Earth Syst. Sci. 8, 509–520. doi:10.5194/nhess-8-509-2008
Micu, M., Micu, D., and Soldati, M. (2021). “Mass Movements in Changing Mountainous Environments,” in Reference Module in Earth Systems and Environmental Sciences (Elsevier). In press. doi:10.1016/b978-0-12-818234-5.00175-9
Migoń, P., and Pijet-Migoń, E. (2020). Late Palaeozoic Volcanism in central Europe—Geoheritage Significance and Use in Geotourism. Geoheritage 12, 1–13.
Migoń, P., and Pijet-Migoń, E. (2019). Natural Disasters, Geotourism, and Geo-Interpretation. Geoheritage 11, 629–640.
Migoń, P., and Pijet-Migoń, E. (2016). Overlooked Geomorphological Component of Volcanic Geoheritage—Diversity and Perspectives for Tourism Industry, Pogórze Kaczawskie Region, SW Poland. Geoheritage 8, 333–350.
Migoń, P., Różycka, M., and Michniewicz, A. (2018). Conservation and Geotourism Perspectives at Granite Geoheritage Sites of Waldviertel, Austria. Geoheritage 10, 11–21.
Mocior, E., and Kruse, M. (2016). Educational Values and Services of Ecosystems and Landscapes - an Overview. Ecol. Indicators 60, 137–151. doi:10.1016/j.ecolind.2015.06.031
Morgan, K. M., DeKay, M. L., Fischbeck, P. S., Morgan, M. G., Fischhoff, B., and Florig, H. K. (2001). A Deliberative Method for Ranking Risks (II): Evaluation of Validity and Agreement Among Risk Managers. Risk Anal. 21, 923. doi:10.1111/0272-4332.215162
Morino, C., Conway, S. J., Balme, M. R., Helgason, J. K., Sæmundsson, Þ., Jordan, C., et al. (2021). The Impact of Ground-Ice Thaw on Landslide Geomorphology and Dynamics: Two Case Studies in Northern Iceland. Landslides 18, 1–28. doi:10.1007/s10346-021-01661-1
Morino, C., Conway, S. J., Sæmundsson, Þ., Helgason, J. K., Hillier, J., Butcher, F. E. G., et al. (2019). Molards as an Indicator of Permafrost Degradation and Landslide Processes. Earth Planet. Sci. Lett. 516, 136–147. doi:10.1016/j.epsl.2019.03.040
Mortimore, R. N. (2019). Late Cretaceous Stratigraphy, Sediments and Structure: Gems of the Dorset and East Devon Coast World Heritage Site (Jurassic Coast), England. Proc. Geologists' Assoc. 130, 406–450. doi:10.1016/j.pgeola.2018.05.008
Museo (2022). Museo Longarone Vajont – Attimi di Storia. Available at: https://www.attimidistoria.it/.
Nathan, F. (2008). Risk Perception, Risk Management and Vulnerability to Landslides in the hill Slopes in the City of La Paz, Bolivia. A Preliminary Statement. Disasters 32, 337–357. doi:10.1111/j.1467-7717.2008.01043.x
National Park (2022). National Park Foundation. Available at https://www.nationalparks.org/(Accessed January 14, 2022).
National Parks of Canada (2022). The Canadian Encyclopedia. Available at: https://www.thecanadianencyclopedia.ca/en/article/national-parks-of-canada (Accessed January 14, 2022).
Németh, B., Németh, K., and Procter, J. N. (2021). Visitation Rate Analysis of Geoheritage Features from Earth Science Education Perspective Using Automated Landform Classification and Crowdsourcing: A Geoeducation Capacity Map of the Auckland Volcanic Field, New Zealand. Geosciences 11, 480.
Nicolet, P., Foresti, L., Caspar, O., and Jaboyedoff, M. (2013). Shallow Landslide's Stochastic Risk Modelling Based on the Precipitation Event of August 2005 in Switzerland: Results and Implications. Nat. Hazards Earth Syst. Sci. 13, 3169–3184. doi:10.5194/nhess-13-3169-2013
Nicoletti, P. G., and Sorriso-Valvo, M. (1991). Geomorphic Controls of the Shape and Mobility of Rock Avalanches. Geol. Soc. Am. Bull. 103, 1365–1373. doi:10.1130/0016-7606(1991)103<1365:gcotsa>2.3.co;2
Niculiţă, M., and Mărgărint, M. C. (2018). Landslides and Fortified Settlements as Valuable Cultural Geomorphosites and Geoheritage Sites in the Moldavian Plateau, North-Eastern Romania. Geoheritage 10, 613–634.
Nix, T., and Marinoni, O. (2006). “Quantitative Landslide Risk Analysis and Risk Evaluation for Publicly Accessible Geosites,” in International Association for Engineering Geology Conference (Nottingham: The Geological Society of London), 222.
Norris, F. H. (2005). Psychosocial Consequences of Natural Disasters in Developing Countries: What Does Past Research Tell Us about the Potential Effects of the 2004 Tsunami? Washington, DC: Department of Veterans Affairs.
Pánek, T. (2019). Landslides and Quaternary Climate Changes—The State of the Art. Earth-science Rev. 196, 102871.
Panizza, M. (2009). “Geomorphodiversity of the Dolomites and Some Remarks on Recent Rockfalls,” in Proceedings of the International Conference ‘Landslide Processes (Springer), 6–7.
Paronuzzi, P., Rigo, E., and Bolla, A. (2013). Influence of Filling-Drawdown Cycles of the Vajont Reservoir on Mt. Toc Slope Stability. Geomorphology 191, 75–93. doi:10.1016/j.geomorph.2013.03.004
Pastick, N. J., Jorgenson, M. T., Wylie, B. K., Nield, S. J., Johnson, K. D., and Finley, A. O. (2015). Distribution of Near-Surface Permafrost in Alaska: Estimates of Present and Future Conditions. Remote Sensing Environ. 168, 301–315. doi:10.1016/j.rse.2015.07.019
Patton, A. I., Rathburn, S. L., and Capps, D. M. (2019). Landslide Response to Climate Change in Permafrost Regions. Geomorphology 340, 116–128. doi:10.1016/j.geomorph.2019.04.029
Paul, F., Kääb, A., Maisch, M., Kellenberger, T., and Haeberli, W. (2004). Rapid Disintegration of Alpine Glaciers Observed with Satellite Data. Geophys. Res. Lett. 31, 1. doi:10.1029/2004gl020816
Pedrazzini, A., Jaboyedoff, M., Froese, C. R., Langenberg, C. W., and Moreno, F. (2011). Structural Analysis of Turtle Mountain: Origin and Influence of Fractures in the Development of Rock Slope Failures. Geol. Soc. Lond. Spec. Publications 351, 163–183. doi:10.1144/sp351.9
Peduzzi, P. (2009). Global Assessment Report on Disaster Risk Reduction. Patterns, Trends, and Drivers. Geneva, Switzerland: The UN Office for Disaster Risk Reduction.
Pelfini, M., Garavaglia, V., and Bollati, I. (2010). Dendrogeomorphological Investigations for Assessing Ecological and Educational Value of Glacial Geomorpho-Sites. Two Examples from the Italian Alps. Regolini-Bissig Géraldine.
Pelfini, M., and Gobbi, M. (2005). Enhancement of the Ecological Value of Forni Glacier (Central Alps) as a Possible Geomorphosite: New Data from Arthropod Communities. Geogr. Fis. e Din. Quat. 28, 211–217.
Pennebaker, J. W., and Harber, K. D. (1993). A Social Stage Model of Collective Coping: The Loma Prieta Earthquake and the Persian Gulf War. J. Soc. Issues 49, 125–145. doi:10.1111/j.1540-4560.1993.tb01184.x
Pérez-Umaña, D., Quesada-Román, A., De Jesús Rojas, J. C., Zamorano-Orozco, J. J., Dóniz-Páez, J., and Becerra-Ramírez, R. (2019). Comparative Analysis of Geomorphosites in Volcanoes of Costa Rica, Mexico, and Spain. Geoheritage 11, 545–559.
Pérez-Umaña, D., Quesada-Román, A., and Tefogoum, G. Z. (2020). Geomorphological Heritage Inventory of Irazú Volcano, Costa Rica. Int. J. Geoheritage Park. 8, 31–47.
Petley, D. (2012). Global Patterns of Loss of Life from Landslides. Geology 40, 927–930. doi:10.1130/G33217.1
Petley, D. N., Dunning, S. A., and Rosser, N. J. (2005). “The Analysis of Global Landslide Risk through the Creation of a Database of Worldwide Landslide Fatalities,” in Landslide Risk Management. Editors O. Hungr, R. Fell, R. Couture, and E. Eberhardt (London: CRC Press), 377–384.
Prosser, C. D., Burek, C. V., Evans, D. H., Gordon, J. E., Kirkbride, V. B., Rennie, A. F., et al. (2010). Conserving Geodiversity Sites in a Changing Climate: Management Challenges and Responses. Geoheritage 2, 123–136. doi:10.1007/S12371-010-0016-7
Prosser, C. D. (2013). Our Rich and Varied Geoconservation Portfolio: the Foundation for the Future. Proc. Geologists' Assoc. 124, 568–580. doi:10.1016/j.pgeola.2012.06.001
Purdie, H., Gomez, C., and Espiner, S. (2015). Glacier Recession and the Changing rockfall hazard: Implications for Glacier Tourism. N. Z. Geog. 71, 189–202. doi:10.1111/nzg.12091
Purdie, H., Hutton, J. H., Stewart, E., and Espiner, S. (2020). Implications of a Changing alpine Environment for Geotourism: A Case Study from Aoraki/Mount Cook, New Zealand. J. Outdoor Recreation Tourism 29, 100235. doi:10.1016/j.jort.2019.100235
Quesada-Román, A., Zangmo, G. T., and Pérez-Umaña, D. (2020). Geomorphosite Comparative Analysis in Costa Rica and Cameroon Volcanoes. Geoheritage 12, 1–14.
Quincey, D. J., and Glasser, N. F. (2009). Morphological and Ice-Dynamical Changes on the Tasman Glacier, New Zealand, 1990-2007. Glob. Planet. Change 68, 185–197. doi:10.1016/j.gloplacha.2009.05.003
Raccanello, D., Gobbo, C., Corona, L., De Bona, G., Hall, R., and Burro, R. (2019a). Long-Term Intergenerational Transmission of Memories of the Vajont Disaster. Psychol. Trauma.. doi:10.1037/tra0000528
Ravanel, L., Bodin, X., and Deline, P. (2014). Using terrestrial laser scanning for the recognition and promotion of high-alpine geomorphosites. Geoheritage 6 (2), 129–140.
Read, R. S., Langenberg, W., Cruden, D. M., Field, M., Stewart, R., Bland, H., et al. (2005). “Frank Slide a century Later: the Turtle Mountain Monitoring Project,” in Landslide Risk Management. Editors O. Hungr, R. Fell, R. Couture, and E. Eberhardt (London: CRC Press), 723–734.
Remondo, J., Bonachea, J., and Cendrero, A. (2005). A Statistical Approach to Landslide Risk Modelling at basin Scale: from Landslide Susceptibility to Quantitative Risk Assessment. Landslides 2, 321–328. doi:10.1007/s10346-005-0016-x
Renn, O. (1992). “Concepts of Risk: a Classification,” in Social Theories of Risk. Editors S. Krimsky, and D. Golding (Westport, CT: Praeger Publishers), 53–79.
Reynard, E., and Brilha, J. (2018). “Geoheritage,” in Geoheritage (Elsevier), 3–9. doi:10.1016/b978-0-12-809531-7.00030-7
Reynard, E., and Coratza, P. (2013). Scientific Research on Geomorphosites. A Review of the Activities of the IAG Working Group on Geomorphosites over the Last Twelve Years. Geogr. Fis. Dinam. Quat. 36, 159–168.
Reynard, E., and Coratza, P. (2016). The Importance of Mountain Geomorphosites for Environmental Education: Examples from the Italian Dolomites and the Swiss Alps. Acta Geogr. Slov Reynard E 56, 291–303. doi:10.3986/ags.1684
Reynard, E., Fontana, G., Kozlik, L., and Scapozza, C. (2007). A Method for Assessing "scientific" and "additional Values" of Geomorphosites. Geogr. Helv. 62, 148–158. doi:10.5194/gh-62-148-2007
Reynard, E. (2009). “The Assessment of Geomorphosites,” in Geomorphosites. Editors E. Reynard, P. Coratza, and G. Regolini-Bissig (München, Germany: Pfeil Verlag), 63–71.
Reynard, E. (2016). “The Importance of Mountain Geomorphosites for Environmental Education: Examples from the Italian Dolomites and the Swiss Alps,” in Acta Geographica Slovenica (Elsevier), 291–303. doi:10.3986/ags.1684
Righi, E., Lauriola, P., Ghinoi, A., Giovannetti, E., and Soldati, M. (2021). Disaster Risk Reduction and Interdisciplinary Education and Training. Prog. Disaster Sci. 10, 100165. doi:10.1016/j.pdisas.2021.100165
Rohrmann, B., and Renn, O. (2000). “Risk Perception Research,” in Cross-cultural Risk Perception. Editors O. Renn, and B. Rohrmann (Springer), 11–53. doi:10.1007/978-1-4757-4891-8_1
Salvati, P., Bianchi, C., Fiorucci, F., Giostrella, P., Marchesini, I., and Guzzetti, F. (2014). Perception of Flood and Landslide Risk in Italy: a Preliminary Analysis. Nat. Hazards Earth Syst. Sci. 14, 2589–2603. doi:10.5194/nhess-14-2589-2014
Salvati, P., Bianchi, C., Rossi, M., and Guzzetti, F. (2012). “Flood Risk in Italy,” in Changes of Flood Risk in Europe. Editor Z. Kundzewicz (UK: IAHS Press), 277–292.
Salvati, P., Bianchi, C., Rossi, M., and Guzzetti, F. (2010). Societal Landslide and Flood Risk in Italy. Nat. Hazards Earth Syst. Sci. 10, 465–483. doi:10.5194/nhess-10-465-2010
Sæmundsson, Þ., Morino, C., and Conway, S. J. (2022). Mass-Movements in Cold and Polar Climates. In: Treatise on Geomorphology. Editor Shroder, J.J.F. (Elsevier: Academic Press) 5, 350–370. doi:10.1016/B978-0-12-818234-5.00117-6
Sæmundsson, Þ., Morino, C., Helgason, J. K., Conway, S. J., and Pétursson, H. G. (2018). The Triggering Factors of the Móafellshyrna Debris Slide in Northern Iceland: Intense Precipitation, Earthquake Activity and Thawing of Mountain Permafrost. Sci. Total Environ. 621, 1163–1175. doi:10.1016/j.scitotenv.2017.10.111
Sassa, K., Fukuoka, H., and Carreno, R. (2009). “Landslide Investigation and Capacity Building in the Machu Picchu - Aguas Calientes Area (IPL C101-1),” in Landslides–Disaster Risk Reduction. Editors K. Sassa, and P. Canuti (Berlin: Springer), 229–248. doi:10.1007/978-3-540-69970-5_13
Sassa, K. (2015). “Landslide Risk Assessment at Cultural Heritage Sites,” in In Engineering Geology for Society and Territory (Cham: Springer), 79–103. doi:10.1007/978-3-319-09057-3_6
Schlögl, M., and Matulla, C. (2018). Potential Future Exposure of European Land Transport Infrastructure to Rainfall-Induced Landslides throughout the 21st century. Nat. Hazards Earth Syst. Sci. 18, 1121–1132.
Selmi, L., Coratza, P., Gauci, R., and Soldati, M. (2019). Geoheritage as a Tool for Environmental Management: A Case Study in Northern Malta (Central Mediterranean Sea). Resources 8, 168. doi:10.3390/resources8040168
Semenza, E., and Ghirotti, M. (2000). History of the 1963 Vaiont Slide: the Importance of Geological Factors. Bull. Eng. Geology. Environ. 59, 87–97. doi:10.1007/s100640000067
Semenza, E. (2011). The Story of Vaiont: Told by the Geologist Who Discovered the Landslide. Ferrara: K-FLASH PUBLISHER.
Serrano, E., José, J., and Trueba, G. (2011). Environmental education and landscape leisure. Geotourist map and geomorphosites in the Picos de Europa National Park. Geoj. Tour. Geosites 8, 295–308.
Shugar, D. H., Jacquemart, M., Shean, D., Bhushan, S., Upadhyay, K., Sattar, A., et al. (2021). A Massive Rock and Ice Avalanche Caused the 2021 Disaster at Chamoli, Indian Himalaya. Science 373, 300–306. doi:10.1126/science.abh4455
Smith, B. J., Pellitero Ondicol, R., and Alexander, G. (2011). Mapping Slope Instability at the Giant's Causeway and Causeway Coast World Heritage Site: Implications for Site Management. Geoheritage 3, 253–266. doi:10.1007/s12371-010-0021-x
Soldati, M., Barrows, T. T., Prampolini, M., and Fifield, K. L. (2018). Cosmogenic Exposure Dating Constraints for Coastal Landslide Evolution on the Island of Malta (Mediterranean Sea). J. Coast. Conserv. 22, 831–844. doi:10.1007/s11852-017-0551-3
Soldati, M., Devoto, S., Prampolini, M., and Pasuto, A. (2019). “The Spectacular Landslide-Controlled Landscape of the Northwestern Coast of Malta,” in Landscapes and Landforms of the Maltese Islands. Editors R. Gauci, and J. Schembri (Springer), 167–178. doi:10.1007/978-3-030-15456-1_14
Stoffel, M., and Huggel, C. (2012). Effects of Climate Change on Mass Movements in Mountain Environments. Prog. Phys. Geogr. Earth Environ. 36, 421–439. doi:10.1177/0309133312441010
Stoffel, M., Tiranti, D., and Huggel, C. (2014). Climate Change Impacts on Mass Movements - Case Studies from the European Alps. Sci. Total Environ. 493, 1255–1266. doi:10.1016/j.scitotenv.2014.02.102
Superchi, L. (2012). The Vajont Rockslide: New Techniques and Traditional Methods to Re-evaluate the Catastrophic Event. Available at: https://www.semanticscholar.org/paper/The-Vajont-rockslide%3A-new-techniques-and-methods-to-Superchi/66de06c67e09abce62f016703fbd3f7d09415a21.
Szepesi, J., Harangi, S., Ésik, Z., Novák, T. J., Lukács, R., and Soós, I. (2017). Volcanic Geoheritage and Geotourism Perspectives in Hungary: a Case of an UNESCO World Heritage Site, Tokaj Wine Region Historic Cultural Landscape, Hungary. Geoheritage 9, 329–349. doi:10.1007/s12371-016-0205-0
Theune, U., Rokosh, D., Sacchi, M. D., and Schmitt, D. R. (2006). Mapping Fractures with GPR: A Case Study from Turtle Mountain. Geophysics 71, B139–B150. doi:10.1190/1.2335515
Thomas, B. A., and Cleal, C. J. (2004). Geological Conservation in the United Kingdom. L. Sci. Pol. 2, 269.
Thomas, M. F. (2001). Landscape Sensitivity in Time and Space - an Introduction. CATENA 42, 83–98. doi:10.1016/S0341-8162(00)00133-8
Thomas, M. F. (2008). Understanding the Impacts of Late Quaternary Climate Change in Tropical and Sub-tropical Regions. Geomorphology 101, 146–158. doi:10.1016/j.geomorph.2008.05.026
Tognaccini, S. (2019). The Proposed Geosite of Volano Landslide and a Geomorphological Itinerary within the Valdelsa Basin (Montespertoli, Tuscany, Italy). Geoheritage 11, 1447–1460. doi:10.1007/s12371-019-00392-z
Tosatti, G. (2008). Slope Instability Affecting the Canossa Geosite (Northern Apennines, Italy). Geogr. Fis Din Quat. 31, 239–246.
UNESCO (2008). International Year of Planet Earth - Global Launch Event 12-13 February 2008. Available at: https://en.lswn.it/events/international-year-of-planet-earth-global-launch-event-12-13-february-2008/(Accessed September 24, 2021).
Vallee, M. (2019). Falling in Place: Geoscience, Disaster, and Cultural Heritage at the Frank Slide, Canada's Deadliest Rockslide. Space Cult. 22, 66–76. doi:10.1177/1206331218795829
Van Den Eeckhaut, M., and Hervás, J. (2012). State of the Art of National Landslide Databases in Europe and Their Potential for Assessing Landslide Susceptibility, hazard and Risk. Geomorphology 139-140, 545–558. doi:10.1016/j.geomorph.2011.12.006
Van Wyk de Vries, B., Byrne, P., Delcamp, A., Einarson, P., Göğüş, O., Guilbaud, M.-N., et al. (2018). A Global Framework for the Earth: Putting Geological Sciences in Context. Glob. Planet. Change 171, 293–321. doi:10.1016/j.gloplacha.2017.12.019
Van Wyk de Vries, B., Vereb, V., Karátson, D., Lagmay, M., Navarro, M., and Karoly, N. (2018). “Rich and Resilient Volcanic Territories Maintained by Geoheritage,” in EGU General Assembly Conference Abstracts (Springer), 4472.
Van Wyk de Vries, M., Bhushan, S., Jacquemart, M., Deschamps-Berger, C., Berthier, E., Gascoin, S., et al. (2021). Pre-collapse Motion of the February 2021 Chamoli Rock-Ice Avalanche, Indian Himalaya. Nat. Hazards Earth Syst. Sci. Discuss., 1–29.
Vanacker, V., Balthazar, V., and Molina, A. (2013). “Anthropogenic Activity Triggering Landslides in Densely Populated Mountain Areas,” in Landslide Science and Practice. Editors C. Margottini, and P. Canuti (Berlin: Springer), 163–167. doi:10.1007/978-3-642-31337-0_21
Vasiljević, D. A., Marković, S. B., Hose, T. A., Smalley, I., Basarin, B., Lazić, L., et al. (2011). The Introduction to Geoconservation of Loess-Palaeosol Sequences in the Vojvodina Region: Significant Geoheritage of Serbia. Quat. Int. 240, 108–116.
Venturini, C., and Pasquaré Mariotto, F. (2019). Geoheritage Promotion through an Interactive Exhibition: A Case Study from the Carnic Alps, NE Italy. Geoheritage 11, 459–469. doi:10.1007/s12371-018-0299-7
Vereb, V., van Wyk de Vries, B., Hagos, M., and Karátson, D. (2020). Geoheritage and Resilience of Dallol and the Northern Danakil Depression in Ethiopia. Geoheritage 12, 1–34. doi:10.1007/s12371-020-00499-8
Wang, G. (2015). Geoheritage Features in Xi'an, China: Cuihua Rock Avalanche Likely Originating from an Ancient Earthquake. Geoheritage 7, 285–297. doi:10.1007/s12371-014-0132-x
Wang, G., Ye, W., and Lv, Y. (2019). Loess Geoheritage and Geohazard Protective Measures at Luochuan Loess National Geopark in NW China. Geoheritage 11, 1089–1100. doi:10.1007/s12371-019-00354-5
Wang, L., and Tian, M. (2014). A Discussion on the Development Model of Earthquake Relic Geopark - a Case Study of the Qingchuan Earthquake Relic Geopark in Sichuan Province, China. J. Cult. Heritage 15, 459–469. doi:10.1016/J.CULHER.2013.11.007
Whalley, W. B., Douglas, G. R., and Jonsson, A. (1983). The Magnitude and Frequency of Large Rockslides in Iceland in the Postglacial. Geografiska Annaler: Ser. A, Phys. Geogr. 65, 99–110. doi:10.2307/52072410.1080/04353676.1983.11880077
Wieczorek, G. F., and Glade, T. (2005). “Climatic Factors Influencing Occurrence of Debris Flows,” in Debris-flow Hazards and Related Phenomena. Editors M. Jakob, and O. Hungr (Chichester: Springer), 325–362.
Wimbledon, W. A., and Smith-Meyer, S. (Editors) (2012). Geoheritage in Europe and its Conservation. Oslo: ProGEO.
Winter, M. G., and Bromhead, E. N. (2012). Landslide Risk: Some Issues that Determine Societal Acceptance. Nat. Hazards 62, 169–187. doi:10.1007/s11069-011-9987-1
Wobus, C. W., Tucker, G. E., and Anderson, R. S. (2010). Does Climate Change Create Distinctive Patterns of Landscape Incision? J. Geophys. Res. Earth Surf. 115, 1. doi:10.1029/2009jf001562
Wolter, A., Stead, D., Ward, B. C., Clague, J. J., and Ghirotti, M. (2016). Engineering Geomorphological Characterisation of the Vajont Slide, Italy, and a New Interpretation of the Chronology and Evolution of the Landslide. Landslides 13, 1067–1081. doi:10.1007/s10346-015-0668-0
Woo, K. S. (2017). “Role of Iucn Wcpa Geoheritage Specialist Group for Geoheritage Conservation and Recognition of World Heritage Sites, Global Geoparks and Other Protected Areas,” in EGU General Assembly Conference Abstracts (Springer), 1137.
Zemp, M., Huss, M., Thibert, E., Eckert, N., McNabb, R., Huber, J., et al. (2019). Global Glacier Mass Changes and Their Contributions to Sea-Level Rise from 1961 to 2016. Nature 568, 382–386. doi:10.1038/s41586-019-1071-0
Keywords: landslide, geomorphosite, geosite, geoheritage, climate change, anthropic signature, risk perception
Citation: Morino C, Coratza P and Soldati M (2022) Landslides, a Key Landform in the Global Geological Heritage. Front. Earth Sci. 10:864760. doi: 10.3389/feart.2022.864760
Received: 28 January 2022; Accepted: 23 February 2022;
Published: 25 March 2022.
Edited by:
Marcelo Cohen, Federal University of Pará, BrazilReviewed by:
Mihai Niculita, Alexandru Ioan Cuza University, RomaniaPiotr Migoń, University of Wrocław, Poland
Karoly Nemeth, Massey University, New Zealand
Adolfo Quesada-Román, University of Costa Rica, Costa Rica
Copyright © 2022 Morino, Coratza and Soldati. This is an open-access article distributed under the terms of the Creative Commons Attribution License (CC BY). The use, distribution or reproduction in other forums is permitted, provided the original author(s) and the copyright owner(s) are credited and that the original publication in this journal is cited, in accordance with accepted academic practice. No use, distribution or reproduction is permitted which does not comply with these terms.
*Correspondence: Paola Coratza, cGFvbGEuY29yYXR6YUB1bmltb3JlLml0