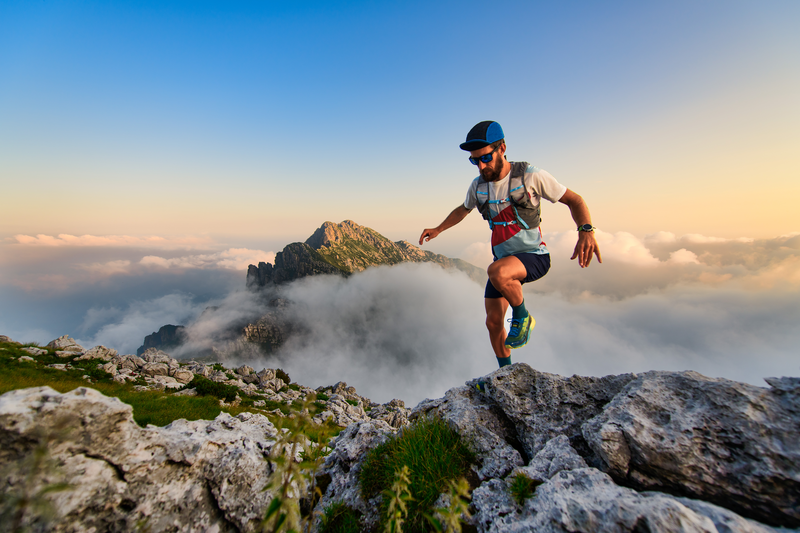
95% of researchers rate our articles as excellent or good
Learn more about the work of our research integrity team to safeguard the quality of each article we publish.
Find out more
ORIGINAL RESEARCH article
Front. Earth Sci. , 05 April 2022
Sec. Geohazards and Georisks
Volume 10 - 2022 | https://doi.org/10.3389/feart.2022.853089
The Sichuan–Tibet Railway crosses through the largest maritime glacier region in China. A large number of moraines formed after the rapid glacial retreat caused by climate warming. Moraines could induce frequent geological hazards that seriously threatened the safe construction and operation of the railway. Accordingly, moraines in this maritime glacier region have become a new challenging research topic with respect to the formation of geological hazards. Using remote-sensing image interpretations, field investigations, and dating tests, moraines and their topographic information were systematically obtained. After analyzing the geometrical distribution characteristics of the moraine accumulations, the geometrical characteristics of three typical moraine accumulation forms were generalized into corresponding mathematical models. Consequently, a method to quickly and quantitatively estimate the moraine reserves is proposed. The moraine distribution is primarily affected by the elevation, slope, river–valley morphology, and climate conditions. Old moraines that formed in the Pleistocene epoch (the Guxiang and Baiyu glacial periods) are primarily distributed below 3,500 m above sea level, while most of the new moraines that formed in the Holocene epoch (Neoglaciation and Little Ice Age) are primarily distributed above 4,000 m above sea level. Both the new and old moraines are primarily distributed within a slope range of 10–30°. Furthermore, the main river–valley morphology has a significant impact on the distribution of the old moraines, which are primarily distributed in the Zhongba–Guxiang section of the river valley where the longitudinal slope is relatively gentle. The difference in glaciation is the main reason why the new moraines distributed on the south banks in the study area are different from those on the north banks and why those distributed upstream are different from those distributed downstream. In addition, moraines are the main source of glacial debris flows. According to the presented method, the loose moraine reserves can be accurately calculated by analyzing the position, consolidation, and supply capability of the new and old moraines in each debris flow gully. It is anticipated that the presented results can be used to better understand the formation mechanisms of glacier-related hazards and improve risk assessments.
High mountain glaciers are gradually retreating as a result of global warming, subsequently exposing a large quantity of loose and unvegetated glacial sediments (moraines). Some of these unconsolidated moraines are a major source of hazards, such as glacier lake outburst floods, landslides, and debris flows. In recent years, more attention has been given to geomorphological landforms with regard to the moraine-related risk potential (Legg et al., 2014; Zaginaev et al., 2019; Kumar et al., 2019; Veh et al., 2020; Guo et al., 2021). The Parlung Tsangpo Basin is a modern glaciation center within the Qinghai–Tibet Plateau. The construction of the Sichuan–Tibet railway and the highway in operation crosse this basin and will therefore face serious glacial hazards as shown in Figure 1. Large quantities of loose and unvegetated moraines are often a source of glacial disasters. Even though considerable attention has been paid to the evaluation of potential risks of glacial hazards in this region (Cui et al., 2014; Zhang, 2016; Deng et al., 2017; Peng et al., 2020; Peng et al., 2020); however moraines’ distribution and reserve are unclear, making it impossible to accurately identify the potential risk of moraine-related disasters. So the accurate estimation of moraine reserves play a significant role in the study of glacial periods and scales and the prediction and comprehensive prevention of moraine-related disasters.
Moraines, as glacial relics, were first researched by paleontologists, meteorologists, and geologists. Different combinations of moraines distributed in different valleys can reflect changes in the paleoclimatic environment (Iturrizaga, 2008; 2018). By studying the distribution and accumulation of moraines and the biological fossils within the moraine soils, researchers can analyze glacial advance and retreat during different glacial periods, and identify the impact of glaciers on the ecological environment, biological evolution, and the evolution of the topography and landforms so as to infer the characteristics and trends of climate change (Shi et al., 2006; Zhou et al., 2010; Barr and Lovell, 2014; Korsgaard et al., 2015). Several scholars have found that the combination of moraine forms may reflect the degree of climate change affecting the glacial advances (Włodarski and Godlewska, 2016; Epshtein, 2017) and that the moraine scale can reveal the temporal scale of stable climate periods (Schrott et al., 2003; Dunlop and Clark, 2006; Ou et al., 2014). Most of these moraine distribution studies have focused on the determination and division of moraine chronological ages, as well as the evolutionary characteristics of glacial advances and retreats according to the moraine distribution. Since the Quaternary, many glacial activities have occurred on the Tibet Plateau. Moraine ridges at different locations correspond to the local glacial activities during different periods, and moraines formed in the same period show similar spatial distribution, forms, and scales (Chen et al., 2015). Topography controls not only where moraines can be formed but also if they can be preserved and easily identified. Also, the slope is one of the main factors that can affect the distribution of moraines as well as the glacial dynamics. The regional average slope where glacial moraines exist is from 7° to 25°(Peng, 1996; Libohova et al., 2016). In addition, a high relief is not conducive to the preservation of moraines so that moraines can be easily eroded and transported to relatively flat areas by gravitation and hydrodynamic forces.
The recent development of geographic information system-related technologies has made studies of large-scale glacial relics more intuitive and efficient, which is conducive to the rapid identification and comparative analysis of glacial landforms (Chen et al., 2015). Moraines formed at the margins of ice sheets and ice caps are likely to be large and readily identifiable in the modern landscape (Finlayson and Bradwell 2008; Winkler and Matthews 2010; Ojala 2016; Chandler et al., 2016). Meanwhile, ice-marginal moraines have a ridge-like form and are typically classified according to their location as glacier terminal, lateral, or lateral-frontal moraines (Chen et al., 2013; Cui 2013; Cui et al., 2015; Tonkin et al., 2016; Iturrizaga 2018). These moraines are formed through a number of processes, such as the dumping of supraglacial, englacial, and subglacial debris at glacier margins (Eyles, 1983; Heyman and Hãttestrand, 2006). Individual moraine ridges are often produced through a combination of these processes and can be formed subaerially and subaqueously (Krzyszkowski and Zeliński, 2002; Ottesen and Dowdeswell, 2006). Barr and Clark (2012) systematically interpreted the distribution characteristics of moraines from the Verkhoyansk Mountain in northeastern Russia to the Chukchi Peninsula via aerial images and digital elevation topographic data. His research findings show that most of the area (now basically ice-free) was previously covered by glaciers. Ely et al. (2017) retrieved high-resolution aerial images (with a resolution of 2 cm) of the Isfallsglaciären pre-glacier area in Lapland, Sweden, using unmanned aerial vehicles (UAVs) to produce a moraine distribution map. His study highlights the advantages of using UAVs to rapidly interpret glacial deposition landforms.
A better knowledge of sediment storage may significantly improve process modeling and the understanding of glacier-related hazards (Otto et al., 2008; Sattler et al., 2011; Chang et al., 2011; Chen and cui, 2015; Jiang et al., 2021). More detailed information concerning the volumes of potentially mobilized sediments is required, especially in densely populated mountainous regions that are vulnerable to natural hazards (Chen et al., 2015, Chen et al., 2018; Guo et al., 2020; Li et al., 2021; Cui et al. 2021; Guo et al., 2022). However, accurate quantifications of the sediment volume in drainage basins are problematic, especially in large drainage basins (Slaymaker, 1991). Different approaches have been applied to determine the volume of debris stored in a drainage basin. For a single landform, a more reproducible approach is the application of geometric forms to represent the shape of the storage body, thus approximating its volume (Curry, 1999; Shroder et al., 1999; Campbell and Church, 2003; Guo et al., 2021). Geometric forms, such as prisms and sectors of cones, are used to represent single landforms, including talus slopes, talus cones, debris cones, fans, or fluvial terraces. Parameters used for volumetric calculations are usually extracted from maps, aerial photos, or digital elevation model (DEM) data (Campbell and Church, 2003; Otto, 2006; Otto et al., 2008). For the maritime glacier region in southeastern Tibet, especially for glacial debris flow gullies, the extent to which the sediment yields are still influenced by sediments from the last glaciation is not well understood.
In this study, we systematically interpret moraines with multi-phase and high-resolution remote-sensing images and Digital Elevation Model (DEM) data according to their typical landform characteristics. We also verify the interpreted moraines based on field investigations. In addition, we propose moraine reserve calculation methods for different accumulation modes, investigate and calculate the distribution and quantity of glacial deposits over the entire watershed, and build a distribution and reserve database of the moraines. Furthermore, we analyze in detail the types and distribution characteristics of the moraines, as well as the factors that affect their distribution. This study provides a more time-efficient and reliable method for the identification of moraines around maritime glaciers, and proposes a more accurate and convenient method for the calculation of the source reserves of glacial debris flows. The results of the study, applied to debris flow prevention and control engineering, can improve the safety and economy of such ventures. In addition, we provide extremely important data for research concerning source characteristics and formation mechanisms, which also support risk assessments of glacial debris flows.
When a glacier advances, the accumulation of a large number of loose materials can occur in moraines as a result of processes such as glacier ploughing and plucking. The moraines above, in, and below a glacier and in front of the glacier tongue can be pushed forward when the glacier advances but cannot retreat when the glacier retreats. These moraines are likely to form specific landforms, indicating glacial erosion such as cirques and glacial troughs. However, moraines in and on the glacier tongue are separated from the glacier and, as the glacier melts, remain in situ and form end moraines. Meanwhile, most of the moraines left on the two sides of the glacier become lateral moraines. These glacial relics with typical topography and landform characteristics are easy to identify in the field and in high-resolution images (Iturrizaga, 2018). Therefore, different moraine types show obviously different presentation characteristics in remote-sensing images, including different forms, color tones, and image structures. Large-scale lateral moraines and end moraines generally form dikes and ridges, and there may be multiple ridges on both sides of the glacier and in the valley in front of the glacier tongue as a result of repetitive glacial advance and retreat (McBratney et al., 2003; Libohova et al., 2016; Dubey et al., 2019). These typical characteristics are different from the surrounding background in their forms, color tones, and image structures and can be identified via high-precision remote-sensing images and high-resolution digital topographic maps. In addition, the types and distributions of moraines can be obtained by analyzing the melting processes of glaciers and the changes in the glacial morphology in branch valleys. Via field investigations, the type of moraines in a study area can be classified into end moraine ridges, lateral moraine ridges, elliptical hills, moraines in trough valleys, moraines in cirques, and talus cones according to the positional relationship of the moraines relative to the glacier and the characteristics of the accumulation forms during their formation (Figure 2), referring to the classification of moraines via glacial landforms. The characteristics of various moraines are shown in Table 1.
FIGURE 2. Types of moraine [(A) Lateral moraine; (B) Terminal moraine; (C) Drumlin; (D) Moraine dam in trough valley; (E) Moraine dam in cirque; (F) Debris apron].
In this study, for the remote-sensing recognition of moraines, high-resolution Gaofen-1 (GF-1, with 1-m resolution) and Gaofen-2 (GF-2, with 4-m resolution) multispectral images (image date: 2013–2016, ©China Centre for Resources Satellite Data and Application), Digital Elevation Model (DEM) data (with 5-m resolution, © the Tibet Bureau of Surveying and Mapping), and glacier data [© the Cold and Arid Regions Science Data Center (http://westdc.westgis.ac.cn/)] were used. In addition, to enhance the presentation of regional topographic characteristics, DEM data were used to generate hill-shade images to improve recognition of the moraine accumulation range. A more time-consuming polygon-based visual interpretation of the moraines, instead of the automatic extraction tool in the ArcGIS 9.3 software, was performed by integrating the high-resolution remote-sensing images and the DEM data (Figures 3A,B). Polygons were used to represent the moraines such that the location and the planar area of each moraine and the total number of moraines could be estimated. Similarly, approximately 10% of the moraines from the preliminary interpretation were verified in the field on foot or using a mini quadrotor UAV (Figure 3C) in July 2016. In addition, the formation age of the moraines can be measured using 14C samples. On the basis of existing studies (Zhou et al., 2010; Wang et al., 2013), lacustrine deposition and carbon debris in lateral moraine dams were collected as 14C dating materials to determine the formation ages of the moraines. For the sample location and age information, please see Figure 4.
FIGURE 3. (A) Sedimentary feature of moraine in remote-sensing image; (B) Topography features of moraine deposit; (C) Accumulation characteristics taken by UAV unmanned aerial vehicle (2016.09.14).
FIGURE 4. Locations and ages of moraine dating samples at the Parlung Tsangpo basin. The Cosmogenic Radio Nuclide (CRN) age is from Zhou et al (2010), Optical Stimulated Luminescence (OSL) ages are from Wang et al., 2005 and 14C ages are from testing in this study. (Site A is located at the lateral moraine in the intersection of Langqiu Gully and Parlung Tsangpo river. Site B is located at the lateral moraine at the end of glacier in Tianmogou Gully).
Different moraines accumulating to regular shapes are closely related to topographic influences. Accordingly, it is relatively easy to extract the geometries of the moraines in the study area from DEM data by applying a spatial analytical method. The geometric characteristics of the moraines can then be generalized into corresponding mathematical models to estimate their total reserves (Otto et al., 2008; 2009).
(1) For ridge-shaped moraines, such as lateral moraine ridges, end moraine ridges, and elliptical hills, their geometric characteristics are similar to those of triangular prism-shaped accumulations (Figure 5). Their volumes can be estimated according to the following formula:
where V is the sedimentary volume of the moraine ridge, h is the accumulation height of the moraine ridge, L is the accumulation length of the moraine ridge, and
(2) Talus cone-shaped moraine accumulations primarily include talus cones formed by large lateral moraine ridges (or end moraine ridges), which are destroyed nearly to a vertical plane. The moraines in steep cirques and on the slope surface under wind and freezing erosion, fall and accumulate at the foot of the slopes under the effect of gravity; accordingly, their accumulation forms are primarily fan-shaped cones. Their volumes can be calculated according to the following formula (Figure 6):
(3) The cross sections of loose moraines in trough valleys and cirques are approximately parabolic (Svensson, 1959). Their accumulation reserves can be calculated according to the shape of the cross section of the valley (Figure 7) (Institute of Mountain Hazards and Environment (IMHE), 1994):
where V is the possible reserve of loose solid materials in the valley; B is the average width of the cross section of the valley; L is the length of the loose materials in the valley; and h is the average thickness of the moraine accumulation.
FIGURE 5. The accumulation morphology and geometric model of triangular prismatic moraines. (A) Field image. (B) Corresponding sketch model.
FIGURE 6. The accumulation morphology and geometric model of debris aprons. (A) Field image. (B) Corresponding sketch model.
FIGURE 7. The accumulation morphology and geometric model of moraines in trough valley and cirque. (A) Google earth image. (B) Corresponding sketch model.
According to the Chronological data of moraine tested in this paper and the existing research achievements on moraine in Parlung Tsangpo Basin (Zhou et al., 2010; Wang et al., 2013), the formation ages of the moraines in the study area can be classified into the Penultimate Glaciation Period (also called the Guxiang glacial period, 112.9 ± 16.7–136.5 ± 5.7 ka.B.P., corresponding to glacial period MIS-6), the Last Glaciation Period (also called the Baiyu glacial period, 11.1 ± 1.9–18.5 ± 2.2 ka.B.P., corresponding to the glacial period MIS-2), Neoglaciation (3,500–1,000 a.B.P.), and the Little Ice Age (140 ± 30 a.B.P.).
The penultimate glacial period (the Guxiang glacial period) and the last glacial period (the Baiyu glacial period) belong to the Pleistocene epoch, while Neoglaciation and the Little Glacial Age belong to the Holocene epoch (Peng, 1996). Large-scale glaciers in the Pleistocene epoch entered the main valley of the Parlung Tsangpo Basin, and the moraines formed by glaciers in the Pleistocene epoch are primarily distributed on both sides of the main channel of the Parlung Tsangpo River and at the intersections between the river branches and the main river. These moraine ridges have a relatively old sedimentary age. Therefore, the moraines formed in the Pleistocene epoch are called old moraines in this study. Glaciers in the Holocene epoch, limited by their small scales, are hard to reach from the river branch mouths. They are distributed on both sides and at the terminus of the modern glacier tongue, and their lowest thresholds are generally above 3,500 m, while still maintaining relatively complete moraine forms such as lateral moraines and end moraines. The upper parts of the lateral moraines are connected with the modern glaciers. These moraines with relatively young sedimentary ages and primarily distributed in the branch valleys or on both sides of the modern glaciers are called new moraines.
On the basis of the GF-1 and GF-2 images, together with high-precision topographic data (resolutions of 5 × 5 m and 25 × 25 m, respectively) and hill-shade images, different types of moraines were identified using the presented identification methods; the moraine distribution is shown in Figure 8. According to the statistical results, 1,089 moraines with obvious sedimentary characteristics were detected in the Parlung Tsangpo Basin, covering an area of approximately 256.6 × 106 m and accounting for approximately 6% of the total study area. According to the field investigation, the moraines in the study area are mostly distributed in glacial troughs, valleys, cirques, and other relatively flat areas. The distribution of new moraines on the right bank has a relationship with the altitude and are primarily concentrated near 500 m a.s.l. close to the river channel and glacial front. Conversely, new moraines on the left bank do not have such prominent altitudinal distribution patterns and distribute widely from the river channel to the glacier terminus. Moreover, the lowest elevation moraines on the right bank are obviously higher than those at the same location on the left bank.
There is a distinct difference in the upstream and downstream moraine distributions, as well as along the left and right banks throughout the entire basin. Figure 9 shows the longitudinal profiles of the moraines along the main river valley of the Parlung Tsangpo River on the south and north banks. There are two knickpoints in the main river channel, located near Zhongba and Guxiang (Korup and Montgomery, 2008). These points divide the basin into three sections: Ranwu to Zhongba forms the upstream section, Zhongba to Guxiang forms the midstream section, and Guxiang to Tongmai forms the downstream section. In the upstream canyon section between Ranwu and Songzong and in the midstream broad valley section between Songzong and Guxiang, the moraines account for 94% of the total reserves of the entire basin. However, in the downstream canyon section between Guxiang and Tongmai, moraines are sparsely distributed, accounting for a mere 6% of the total reserves in the entire basin. Old moraines (shown in the pink circles) on the left and right banks are primarily distributed in the section between the two knickpoints (i.e., the Zhongba–Guxiang section) and are accumulated near the main river valley. The Neoglaciation moraines are primarily distributed in the branch valleys and on both sides of the modern glaciers. This is consistent with the results of the field investigations. In the upstream Ranwu–Zhongba section, because of the steep slopes of the longitudinal profile of the river, with an average slope of 15.89%, and the strong scouring ability of the river, old moraines moving from the glacier to the valley mouth in this section are not completely preserved. In the midstream Zhongba–Guxiang section, the slope of the longitudinal profile of the river decreases, with an average slope of 6.29%, the erosivity of the river weakens, and most of the old moraines at the valley mouth are preserved. However, in the downstream Guxiang–Tongmai section, the average slope of the longitudinal profile increases to 11.38%, making the erosion capacity in the downstream section increase under the confluence effect of the Bodui Tsangpo River. Consequently, old moraines are not found in this section.
FIGURE 9. Distribution characteristic of moraine along the Parlung Tsangpo river. ((A) Moraine distribution in the north side of river. (B) Moraine distribution in the south side of river).
After the shapes and areas of the moraines were interpreted based on the remote-sensing images, the volumes of all of the moraine types were computed using the reserve estimation methods given in Section 2.4. The total volume of the accumulated glacial deposits is 10.6 × 109 m3, and the statistical results for the different moraine types in the study area are listed in Table 2.
Theold moraine primarily accumulate in lateral moraine, which account for more than 90% of the total old moraine reserves. Comparably, the new moraines deposits primarily accumulate in cirques (52% of the total new moraine reserves) or on the valley sides in the form of lateral moraine ridges (30% of the total new moraine reserves). Moraines accumulated in the form of end moraine ridges and talus cones account for only a small proportion of the total. In addition, the distribution surface area of the moraines changes remarkably. Lateral moraine ridges with relatively large areas are primarily located on the shoulders of both banks of the Parlung Tsangpo River and in the two large branch valleys (of the Bodui Tsangpo and Songzong Tsangpo rivers), and their moraine scales are much larger than those of the lateral moraine ridges near the modern glaciers. This is intimately related to the paleoclimate, glacier scale, and glacier movement characteristics that cause the formation of lateral moraine ridges (Wang et al., 2005; Wang et al., 2013; Barr and Lovell, 2014).
(1) The elevation effect
Via an analysis of the spatial distribution of the moraines formed during different glacial periods and their local elevations, a relationship between these factors was obtained, as shown in Figure 10. The statistical results show that the moraines in the study area are located within the elevation range of 2,017–6,415 m above sea level (a.s.l.), where old moraines account for 19% of the total moraines, and approximately 91% are located within an elevation range of 2,500–3,500 m a.s.l. New moraines are primarily distributed above 4,000 m a.s.l., accounting for 88% of the total moraines (Figure 10A). In general, with increasing altitude, moraines of different types distinctly vary in their distribution, evolving from lateral moraine ridges and talus cones to lateral moraines and moraines in cirques and then to moraines in cirques and talus cones (Figure 10B). In addition, below 3,500 m a.s.l., moraines primarily exist in the form of lateral moraine ridges and talus cones, while moraines of other accumulation types are rarely seen. Within the elevation range of 3,500–4,000 m a.s.l., the majority of moraines are lateral ridges and in cirques. Above 4,000 m a.s.l., moraines are mainly in cirques, the area of the lateral moraine ridges gradually decreases, and the next most abundant moraine type is talus cones.
(2) The slope effect
FIGURE 10. Distribution characteristics of different types of moraines at different elevations [(A) new and old moraines; (B) Different types of moraines].
The relationship between the moraine distribution and the topographic slope (Figure 11) indicates that old and new moraines in the study area are primarily located in a slope range of 10–20°, where the moraine distribution area accounts for approximately 36% of the total moraine area. In the slope range of 20–30°, the moraine distribution area accounts for approximately 16% of the total moraine area. However, old and new moraines are rarely seen where the slope is steeper than 40°, with such areas only accounting for 5% of the total moraine area.
(3) Influence of sunny-shade slopes
The sunny-shade slope effect and the glacier scale significantly impact on the distribution of moraines. The Parlung Tsangpo Basin presents a nearly east-west river valley, which is affected by the geological structure and maritime glaciers. Under the similar geographical conditions, in winters the sunny slope is exposed to the stronger solar radiation, longer sunshine duration, higher temperature, and larger diurnal temperature difference compared with the shady slope. Repentantly in spring glaciers prone to dramatically advance and retreat. The snowline implicating the heat-water conditions required for the development of glaciers (Shi et al., 2006). The uneven hydrothermal conditions experienced by sunny-shade slopes directly affect the position of the snowline and further determine the deposition locations of moraines. As shown in Figure 12A, the quantity of precipitation on the northern slope is much higher than that on the southern slope, making the southern slope being apt to the development of numerous large-scale glaciers than the north slope. Additionally, the freezing and wind erosion are stronger on the southern slopes. Therefore, the snowline is higher on the north bank than on the south bank (Figure 12B). This is supported by the evidence that glaciers are distributed at the higher positions on the north bank than the south bank at the same latitude, and that deposition position of the corresponding moraines are also relatively higher on the north bank.
FIGURE 12. (A) Glacier area distribution on north and south banks of Parlung Tsangpo river. (B) Characteristics of the snow-line of the North- and South-Facing slope along the Parlung Tsangpo river.
The Sichuan–Tibet Railway crosses the Guxiang–Tongmai section of the Parlung Tsangpo River. Under continuous climate warming, the large amount of loose moraine deposited by rapid glacial retreat can cause giant glacial debris flows in this region. In particular, large glacial debris flows tend to block the river in the gorge section, resulting in large-scale disasters, which not only can cause huge casualties but also can endanger the safe construction and operation of the Sichuan–Tibet Railway (Cui et al., 2015; Staffler et al.2008). For example, more than 1,000 debris flows have occurred in Guxiang Gully since the 1950s; with a peak flow of 28,600 m3/s, the debris flows blocked the Parlung Tsangpo River and formed a barrier lake 1–2-km wide and 5-km long (Liu et al., 2015). The frequency and scale of the debris flows in this gully are the highest recorded in China. Debris flows in the Tianmo Gully were not active prior to 2007. However, on 4 September 2007, on July 25 and 5 September 2010, and on 11 July 2018, four large debris flows occurred in the Tianmo Gully. These events not only blocked the Parlung Tsangpo River but also destroyed the G318 Highway and caused great harm to the lives and property of local residents (Ge et al., 2014; Wang et al., 2019). This gully is also extremely rare in terms of its debris flow development speed. Glacial debris flows are special geomorphologic processes that develop in periglacial mountainous areas at high altitudes. It is necessary to evaluate the source materials for debris flows to accurately assess the hazard posed by the source area (Guo and Cui, 2020; Cao et al., 2021). This study shows that the contribution of moraines at different positions in the channel to the debris flow activity varies greatly. Old moraines with long consolidation times and good stability are primarily located in the debris flow accumulation area; therefore, they have a weak impact on the debris flow activities. Meanwhile, new moraines, with their loose structure and poor stability, are primarily located in the debris flow source area and flowing channel and, consequently, become the main suppliers for the formation and scale enlargement of debris flows (Stoffel et al., 2014). Using the proposed generalized models for moraines with different accumulation characteristics, we can quantitatively estimate the reserve of loose solid materials in glacial debris flows. An analysis of the moraine reserve and spatial distribution related to the 139 debris flows in the Parlung Tsangpo Basin indicates that 71 watersheds have thick moraine accumulation characteristics (Figure 13). According to the statistical results, 15 valleys have reserves exceeding 108 m3, 35 valleys have total reserves of 107–108 m3, 15 valleys have total reserves of 106–107 m3, five valleys have total reserves of 105–106 m3, and one valley has a total reserve of less than 105 m3.
Morainesare a sensitive indicator of climate change in eastern Tibet. Abundant moraines in this maritime glacier region induce frequent geological hazards, seriously threatening the safety of towns and roads in the mountains. This article proposed a new method to quickly identify moraines and quantitatively estimate their reserves. In this approach, the moraines were systematically identified using multi-stage and high-resolution remote-sensing images and were then validated via field investigations. Topographic information concerning typical moraine accumulations was extracted from DEM data. Then, the geometrical characteristics of three typical moraine accumulation forms were generalized into corresponding mathematical models, allowing the quick and reliable estimation of the moraine reserves. This provides a new approach to estimate the reserves of loose solid substances in periglacial regions.
The factors that affect the moraine distribution are complex and diverse. In this article, moraines were analyzed based on their temporal and spatial dimensions. The study site has experienced four main glacial periods, namely the Guxiang glacial period, the Baiyu the glacial period, Neoglaciation, and the Little Glacial Age. The old moraines that formed during the Pleistocene epoch (the Guxiang and Baiyu glacial periods) are primarily distributed at the mouth of each branch valley on both banks of the main river valley of the Parlung Tsangpo Basin. The new moraines that formed in the Holocene epoch (Neoglaciation and the Little Glacial Age) are primarily distributed in the channel of each branch valley and at the margins of the modern glaciers. In terms of the effect of spatial differences in the environmental conditions, the moraine distribution is primarily affected by the elevation, slope, river–valley morphology, and climate conditions. The results indicate that the old moraines are primarily distributed below 3,500 m a.s.l., while most new moraines are primarily distributed above 4,000 m a.s.l. Both new and old moraines are primarily distributed within a slope range of 10–30°. Moreover, the main river–valley morphology has a significant impact on the distribution of the old moraines, causing them to be primarily distributed in the Zhongba–Guxiang section of the river valley, where the longitudinal slope is relatively gentle. In addition, differences in the glaciation are the main reason why the new moraines in the study area distributed on the south banks are different from those distributed on the north banks and those distributed upstream are different from those distributed downstream.
In terms of the material source conditions for the formation of debris flows, loose moraines are accumulated during the repetitive and dramatic advances and retreats of glaciers. The material sources have diverse types, and their distribution sections are relatively long in debris flow gullies throughout the entire glacier region. These loose solid materials are more likely to participate in debris flow activities than materials from non-glacial debris-flow areas. However, the material sources of glacial debris flows are generally located in high-altitude areas above 3,000 m a.s.l., where the observation conditions are harsh and the monitoring and historical disaster data are insufficient. Accordingly, it is difficult to determine the reserve of material sources and the processes supplying materials for glacial debris activities. This seriously restricts the understanding of the formation mechanisms of glacial debris flows and the accuracy of risk assessments. Using the proposed generalized models of moraines with different accumulation characteristics, we can quantitatively estimate the reserve of loose solid sources in the debris flow channel. This will provide scientific support for hazard prevention, control, and risk assessments of glacial debris flows in alpine areas.
The original contributions presented in the study are included in the article/Supplementary Material, further inquiries can be directed to the corresponding author.
JW collated materials and wrote this manuscript. PC contributed to the conceptualization, supervision and funding acquisition. HW and GZ participated in the field investigation. QZ and XC reviewed and edited the manuscript. All authors listed have contributed sufficiently to the project to be included as authors.
This study was financially supported by the National Natural Science Foundations of China (Grant no.41941017; 42101088 and U20A20112), the Second Tibetan Plateau Scientific Expedition and Research Program (STEP) (No. 2019QZKK0906), and the Strategic Priority Research Program of Chinese Academy of Sciences (Grant no.XDA23090300). We thank Martha Evonuk, PhD, from Liwen Bianji (Edanz) (www.liwenbianji.cn/), for editing the English text of a draft of this manuscript.
The authors declare that the research was conducted in the absence of any commercial or financial relationships that could be construed as a potential conflict of interest.
All claims expressed in this article are solely those of the authors and do not necessarily represent those of their affiliated organizations, or those of the publisher, the editors and the reviewers. Any product that may be evaluated in this article, or claim that may be made by its manufacturer, is not guaranteed or endorsed by the publisher.
Barr, I. D., and Clark, C. D. (2012). Late Quaternary Glaciations in Far NE Russia; Combining Moraines, Topography and Chronology to Assess Regional and Global Glaciation Synchrony. Quat. Sci. Rev. 53 (53), 72–87. doi:10.1016/j.quascirev.2012.08.004
Barr, I. D., and Lovell, H. (2014). A Review of Topographic Controls on Moraine Distribution. Geomorphology 226, 44–64. doi:10.1016/j.geomorph.2014.07.030
Campbell, D., and Church, M. (2003). Reconnaissance Sediment Budgets for Lynn Valley, British Columbia: Holocene and Contemporary Time Scales. Can. J. Earth Sci. 40, 701–713. doi:10.1139/e03-012
Cao, C., Zhang, W., Chen, J., Shan, B., Song, S., and Zhan, J. (2021). Quantitative Estimation of Debris Flow Source Materials by Integrating Multi-Source Data: A Case Study. Eng. Geol. 291, 106222. doi:10.1016/j.enggeo.2021.106222
Chandler, B. M. P., Evans, D. J. A., and Roberts, D. H. (2016). Characteristics of Recessional Moraines at a Temperate Glacier in SE Iceland: Insights into Patterns, Rates and Drivers of Glacier Retreat. Quat. Sci. Rev. 135, 171–205. doi:10.1016/j.quascirev.2016.01.025
Chang, C. W., Lin, P. S., and Tsai, C. L. (2011). Estimation of Sediment Volume of Debris Flow Caused by Extreme Rainfall in Taiwan. Engineering Geology 123 (1), 83–90. doi:10.1016/j.enggeo.2011.07.004
Chen, J., and Cui, Z. J. (2015). Discovery of Outburst Deposits Induced by the Xuelongnang Paleolandslide-Dammed lake in the Upper Jinsha River, China and its Environmental and hazard Significance. Acta Sedimentologica Sinica 33 (2), 275–284. (in Chinese). doi:10.14027/j.cnki.cjxb.2015.02.007
Chen, J., Dai, F., Lv, T., and Cui, Z. (2013). Holocene Landslide-Dammed lake Deposits in the Upper Jinsha River, SE Tibetan Plateau and Their Ages. Quat. Int. 298, 107–113. doi:10.1016/j.quaint.2012.09.018
Chen, J., Zhou, W., Cui, Z., Li, W., Wu, S., and Ma, J. (2018). Formation Process of a Large Paleolandslide-Dammed lake at Xuelongnang in the Upper Jinsha River, SE Tibetan Plateau: Constraints from OSL and 14C Dating. Landslides 15 (12), 2399–2412. doi:10.1007/s10346-018-1056-3
Chen, R., Zhou, S., Li, Y., and Deng, Y. (2015). Glacial Geomorphology of the Parlung Zangbo Valley, southeastern Tibetan Plateau. J. Maps 12 (5), 716–724. doi:10.1080/17445647.2015.1069765
Cui, P., Chen, R., Xiang, L. Z., and Su, F. H. (2014). Risk Analysis of Mountain Hazards in Tibetan Plateau under Global Warming. Clim. Chang. Res. 10 (2), 103. doi:10.3969/j.issn.1673-1719.2014.02.004
Cui, P., Peng, J., Shi, P., Tang, H., Ouyang, C., Zou, Q., et al. (2021). Scientific Challenges of Research on Natural Hazards and Disaster Risk. Geogr. Sustainability 2 (3), 216–223. doi:10.1016/j.geosus.2021.09.001
Cui, Z. J. (2013). Diamicton and Environment. Shijiazhuang: Hebei Press of Science & Technology, 117–260. (in Chinese).
Cui, Z. J., Zhang, M., Cui, P., Chen, J., Wei, M., Chen, Y., et al. (2015). Discussion on Characteristics of Sedimentary Facies of Dammed-Lakes Outburst Deposits. Mountain Res. 33 (2), 129–140. (in Chinese). doi:10.16089/j.cnki.1008-2786.000018
Curry, A. M. (1999). Paraglacial Modification of Slope Form. Earth Surf. Process. Landforms 24 (13), 1213–1228. doi:10.1002/(sici)1096-9837(199912)24:13<1213::aid-esp32>3.0.co;2-b
Deng, M., Chen, N., and Liu, M. (2017). Meteorological Factors Driving Glacial till Variation and the Associated Periglacial Debris Flows in Tianmo Valley, South-Eastern Tibetan Plateau. Nat. Hazards Earth Syst. Sci. 17 (3), 345–356. doi:10.5194/nhess-17-345-2017
Dubey, J., Ali, S. N., Sharma, A., Morthekai, P., Singh, R., Sharma, R. K., et al. (2019). Glacial Geomorphology and Landscape Evolution of the Thangu valley, North Sikkim Himalaya, India. J. Indian Soc. Remote Sens 47 (5), 821–837. doi:10.1007/s12524-019-00943-5
Dunlop, P., and Clark, C. D. (2006). Distribution of Ribbed Moraine in the Lac Naococane Region, Central Québec, Canada. J. Maps 2 (1), 59–70. doi:10.4113/jom.2006.44
Ely, J. C., Graham, C., Barr, I. D., Rea, B. R., Spagnolo, M., and Evans, J. (2017). Using UAV Acquired Photography and Structure from Motion Techniques for Studying Glacier Landforms: Application to the Glacial Flutes at Isfallsglaciären. Earth Surf. Process. Landforms 42 (6), 877–888. doi:10.1002/esp.4044
Epshtein, O. G. (2017). Basal (Basic) Moraines: Problem of the Identification and Principles of New Classification. Lithol. Miner. Resour. 52 (2), 125–146. doi:10.1134/s0024490217010023
Eyles, N. (1983). Glacial Geology. An Introduction for Engineers and Earth Scientists. Frankfurt: Pergamon Press.
Finlayson, A. G., and Bradwell, T. (2008). Morphological Characteristics, Formation and Glaciological Significance of Rogen Moraine in Northern Scotland. Geomorphology 101 (4), 607–617. doi:10.1016/j.geomorph.2008.02.013
Fu, P., and Yi, C. L. (2009). Relationships between the heights of moraines and lengths of former glaciers in Tibet and surrounding mountains. Geomorphology 103 (2), 205–211. doi:10.1016/j.geomorph.2008.04.023
Ge, Y. G., Cui, P., Su, F. H., Zhang, J. Q., and Chen, X. Z. (2014). Case history of the disastrous debris flows of Tianmo Watershed in Bomi County, Tibet, China: Some mitigation suggestions. Journal of Mountain Science 11 (5), 1253–1265. doi:10.1007/s11629-014-2579-2
Guo, C., and Cui, Y. (2020). Pore Structure Characteristics of Debris Flow Source Material in the Wenchuan Earthquake Area. Eng. Geology. 267, 105499. doi:10.1016/j.enggeo.2020.105499
Guo, J., Wang, J., LiYi, Y. S. J., and Yi, S. (2021). Discussions on the Transformation Conditions of Wangcang Landslide-Induced Debris Flow. Landslides 18, 1833–1843. doi:10.1007/s10346-021-01650-4
Guo, J., Yi, S., Yin, Y., Cui, Y., Qin, M., LiWang, T. C. Y., et al. (2020). The Effect of Topography on Landslide Kinematics: a Case Study of the Jichang Town Landslide in Guizhou, China. Landslides 17, 959–973. doi:10.1007/s10346-019-01339-9
Guo, C., Cui, Y., Xu, W. J., Yin, Y. Z., Li, Y., and Jin, W. (2022). Numerical investigation of the landslide-debris flow transformation process considering topographic and entrainment effects: a case study. Landslides. doi:10.1007/s10346-021-01791-6
Heyman, J., and Hättestrand, C. (2006). Morphology, Distribution and Formation of Relict Marginal Moraines in the Swedish Mountains. Geografiska Annaler: Ser. A, Phys. Geogr. 88 (4), 253–265. doi:10.1111/j.0435-3676.2006.00299.x
Hu, E. (2010). The moraine topography measurement in Rongbuk valley of Mount Everest and its geomorphic significance. Master Dissertation. Graduate School of Chinese Academy of Sciences. (in Chinese)
Institute of Mountain Hazards and Environment (IMHE) (1994). Flood, Debris Flow, Landslide Hazard and Control. Science Publications (in Chinese).
Iturrizaga, L. (2018). Glacial Landform Assemblages and Pedestal Moraines in the Cordillera Blanca (Peru). Geomorphology 318, 283–302. doi:10.1016/j.geomorph.2018.06.012
Iturrizaga, L. (2008). Paraglacial Landform Assemblages in the Hindukush and Karakoram Mountains. Geomorphology 95 (1-2), 27–47. doi:10.1016/j.geomorph.2006.07.030
Jiang, Z., Fan, X., Siva Subramanian, S., Yang, F., Tang, R., Xu, Q., et al. (2021). Probabilistic Rainfall Thresholds for Debris Flows Occurred after the Wenchuan Earthquake Using a Bayesian Technique. Eng. Geology. 280, 105965. doi:10.1016/j.enggeo.2020.105965
Korsgaard, N. J., Schomacker, A., Benediktsson, Í. Ö., Larsen, N. K., Ingólfsson, Ó., and Kjær, K. H. (2015). Spatial Distribution of Erosion and Deposition during a Glacier Surge: Brúarjökull, Iceland. Geomorphology 250, 258–270. doi:10.1016/j.geomorph.2015.09.010
Korup, O., and Montgomery, D. R. (2008). Tibetan Plateau River Incision Inhibited by Glacial Stabilization of the Tsangpo Gorge. Nature 455 (7214), 786–789. doi:10.1038/nature07322
Krzyszkowski, D., and Zieliński, T. (2002). The Pleistocene End Moraine Fans: Controls on Their Sedimentation and Location. Sediment. Geol. 149 (1-3), 73–92. doi:10.1016/s0037-0738(01)00245-7
Kumar, A., Bhambri, R., Tiwari, S. K., Verma, A., Gupta, A. K., and Kawishwar, P. (2019). Evolution of Debris Flow and Moraine Failure in the Gangotri Glacier Region, Garhwal Himalaya: Hydro-Geomorphological Aspects. Geomorphology 333, 152–166. doi:10.1016/j.geomorph.2019.02.015
Legg, N. T., Meigs, A. J., Grant, G. E., and Kennard, P. (2014). Debris Flow Initiation in Proglacial Gullies on Mount Rainier, Washington. Geomorphology 226, 249–260. doi:10.1016/j.geomorph.2014.08.003
Li, Y., Meng, X., Guo, P., Dijkstra, T., Zhao, Y., Chen, G., et al. (2021). Constructing Rainfall Thresholds for Debris Flow Initiation Based on Critical Discharge and S-Hydrograph. Eng. Geology. 280, 105962. doi:10.1016/j.enggeo.2020.105962
Libohova, Z., Winzeler, H. E., Lee, B., Schoeneberger, P. J., Datta, J., and Owens, P. R. (2016). Geomorphons: Landform and Property Predictions in a Glacial Moraine in Indiana Landscapes. Catena 142, 66–76. doi:10.1016/j.catena.2016.01.002
Liu, J. K., Cheng, Z. L., and Li, Q. H. (2015). Meteorology Conditions for Frequent Debris Flows from Guxiang valley in Tibet, China. Sci. Technol. Eng. 33 (1), 95–102. doi:10.1659/MRD-JOURNAL-D-12-00053.1
McBratney, A. B., Mendonça Santos, M. L., and Minasny, B. (2003). On Digital Soil Mapping. Geoderma 117, 3–52. doi:10.1016/s0016-7061(03)00223-4
Ojala, A. E. K. (2016). Appearance of De Geer Moraines in Southern and Western Finland - Implications for Reconstructing Glacier Retreat Dynamics. Geomorphology 255, 16–25. doi:10.1016/j.geomorph.2015.12.005
Ottesen, D., and Dowdeswell, J. A. (2006). Assemblages of Submarine Landforms Produced by Tidewater Glaciers in Svalbard. J. Geophys. Res-earth 111 (F1). doi:10.1029/2005jf000330
Otto, J.-C., Schrott, L., Jaboyedoff, M., and Dikau, R. (2009). Quantifying Sediment Storage in a High alpine valley (Turtmanntal, Switzerland). Earth Surf. Process. Landforms 34 (13), 1726–1742. doi:10.1002/esp.1856
Otto, J. C., Götz, J., and Schrott, L. (2008). “Sediment Storage in Alpine Sedimentary Systems-Quantification and Scaling Issues,” in Sediment Dynamics in Changing Environments (New Zealand: IAHS Press), 258–265.
Otto, J. C. (2006). Paraglacial Sediment Storage Quantification in the Turtmann Valley, Swiss Alps. Germany: University of Bonn. https://nbn-resolving.org/urn:nbn:de:hbz:5N-09254.
Ou, X., Lai, Z., Zhou, S., and Zeng, L. (2014). Timing of Glacier Fluctuations and Trigger Mechanisms in Eastern Qinghai-Tibetan Plateau during the Late Quaternary. Quat. Res. 81 (3), 464–475. doi:10.1016/j.yqres.2013.09.007
Peng, B. Z. (1996). Natural Geography and Resources of Namjagbarwa Peak Area. Beijing: Science Press, 102–111. (in Chinese).
Peng, J. B., Cui, P., and Zhuang, J. Q. (2020). Challenges to Engineering Geology of Sichuan-Tibet Railway. Chin. J. Rock Mech. Eng. 39 (12), 2377–2389. doi:10.13722/j.cnki.jrme.2020.0446
Staffler, H., Pollinger, R., Zischg, A., and Mani, P. (2008). Spatial variability and potential impacts of climate change on flood and debris flow hazard zone mapping and implications for risk management. Natural Hazards and Earth System Sciences 8 (3), 539–558. doi:10.5194/nhess-8-539-2008
Stoffel, M., Mendlik, T., Schneuwly-Bollschweiler, M., and Gobiet, A. (2014). Possible impacts of climate change on debris-flow activity in the Swiss Alps. Climatic Change 122 (1), 141–155. doi:10.1007/s10584-013-0993-z
Sattler, K., Keiler, M., Zischg, A., and Schrott, L. (2011). On the Connection between Debris Flow Activity and Permafrost Degradation: a Case Study from the Schnalstal, South Tyrolean Alps, Italy. Permafrost Periglac. Process. 22 (3), 254–265. doi:10.1002/ppp.730
Schrott, L., Hufschmidt, G., Hankammer, M., Hoffmann, T., and Dikau, R. (2003). Spatial Distribution of Sediment Storage Types and Quantification of valley Fill Deposits in an alpine basin, Reintal, Bavarian Alps, Germany. Geomorphology 55 (1-4), 45–63. doi:10.1016/s0169-555x(03)00131-4
Shi, Y. F., Cui, Z. J., and Su, Z. (2006). The Quaternary Glaciations and Environmental Variations in China. Shijiazhuang: Hebei Press of Science & Technology, 89–115. (in Chinese).
Shroder, J. F., Scheppy, R. A., and Bishop, M. P. (1999). Denudation of Small Alpine Basins, Nanga Parbat Himalaya, Pakistan. Arctic, Antarctic, Alpine Res. 31 (2), 121–127. doi:10.1080/15230430.1999.12003289
Slaymaker, O. (1991). Mountain Geomorphology: A Theoretical Framework for Measurement Programmes. Catena 18, 427–437. doi:10.1016/0341-8162(91)90047-2
Svensson, H. (1959). Is the Cross-Section of a Glacial valley a Parabola? J. Glaciol. 3 (25), 362–363. doi:10.1017/s0022143000017032
Tonkin, T. N., Midgley, N. G., Cook, S. J., and Graham, D. J. (2016). Ice-cored Moraine Degradation Mapped and Quantified Using an Unmanned Aerial Vehicle: A Case Study from a Polythermal Glacier in Svalbard. Geomorphology 258, 1–10. doi:10.1016/j.geomorph.2015.12.019
Veh, G., Korup, O., and Walz, A. (2020). Hazard from Himalayan Glacier lake Outburst Floods. Proc. Natl. Acad. Sci. U.S.A. 117 (2), 907–912. doi:10.1073/pnas.1914898117
Wang, J., Cui, Y., Choi, C. E., and Ng, C. W. W. (2019). The Effect of Climate Change on alpine Mountain Hazards Chain: a Case Study in Tianmo Ravine, Tibet, China. Proc. 8th Int. Congress Environ. Geotechnics 3, 461–470. doi:10.1007/978-981-13-2227-3_57
Wang, J., Kassab, C., Harbor, J. M., Caffee, M. W., Cui, H., and Zhang, G. (2013). Cosmogenic Nuclide Constraints on Late Quaternary Glacial Chronology on the Dalijia Shan, Northeastern Tibetan Plateau. Quat. Res. 79 (3), 439–451. doi:10.1016/j.yqres.2013.01.004
Wang, X. L., Zou, S. B., and Zhou, S. Z. (2005). Climatic Significance Indicated by valley Glacier Variation in Boduizangbu on Southeast Tibetan Plateau. Proc. Int. Geosci. Remote Sensing Symp. (IEEE) IGARSS 8, 5168–5170. doi:10.1109/IGARSS.2005.1526847
Winkler, S., and Matthews, J. A. (2010). Observations on Terminal Moraine-ridge Formation during Recent Advances of Southern Norwegian Glaciers. Geomorphology 116 (1-2), 87–106. doi:10.1016/j.geomorph.2009.10.011
Włodarski, W., and Godlewska, A. (2016). Sedimentary and Structural Evolution of a Pleistocene Small-Scale Push Moraine in Eastern Poland: New Insight into Paleoenvironmental Conditions at the Margin of an Advancing Ice Lobe. Quat. Sci. Rev. 146, 300–321. doi:10.1016/j.quascirev.2016.06.014
Zhang, B. B. (2016). Study on debris flow characteristics in temperate glacier area of Parllon Tsangpo.Master Dissertation. Southwest Jiaotong University. (in Chinese)
Zaginaev, V., Petrakov, D., Erokhin, S., Meleshko, A., Stoffel, M., and Ballesteros-Cánovas, J. A. (2019). Geomorphic Control on Regional Glacier lake Outburst Flood and Debris Flow Activity over Northern Tien Shan. Glob. Planet. Change 176, 50–59. doi:10.1016/j.gloplacha.2019.03.003
Keywords: Glacial moraine, Remote sensing, Estimating sediment reserves model, Debris flow, Parlung Tsangpo
Citation: Wang J, Cui P, Wang H, Zhang G, Zou Q and Chen X (2022) Novel Approach to Estimating Glacial Moraine Reserves in the Parlung Tsangpo Basin. Front. Earth Sci. 10:853089. doi: 10.3389/feart.2022.853089
Received: 12 January 2022; Accepted: 14 March 2022;
Published: 05 April 2022.
Edited by:
Chong Xu, Ministry of Emergency Management, ChinaReviewed by:
Jian Chen, China University of Geosciences, ChinaCopyright © 2022 Wang, Cui, Wang, Zhang, Zou and Chen. This is an open-access article distributed under the terms of the Creative Commons Attribution License (CC BY). The use, distribution or reproduction in other forums is permitted, provided the original author(s) and the copyright owner(s) are credited and that the original publication in this journal is cited, in accordance with accepted academic practice. No use, distribution or reproduction is permitted which does not comply with these terms.
*Correspondence: Peng Cui, cGVuZ2N1aUBpbWRlLmFjLmNu
Disclaimer: All claims expressed in this article are solely those of the authors and do not necessarily represent those of their affiliated organizations, or those of the publisher, the editors and the reviewers. Any product that may be evaluated in this article or claim that may be made by its manufacturer is not guaranteed or endorsed by the publisher.
Research integrity at Frontiers
Learn more about the work of our research integrity team to safeguard the quality of each article we publish.