- 1Key Laboratory of Desert and Desertification, Northwest Institute of Eco-Environment and Resources, Chinese Academy of Sciences, Lanzhou, China
- 2State Key Laboratory of Loess and Quaternary Geology, Institute of Earth Environment, Chinese Academy of Sciences, Xi’an, China
- 3Department of Geosciences, University of Arizona, Tucson, AZ, United States
- 4Key Laboratory of Orogenic Belts and Crustal Evolution, Ministry of Education, School of Earth and Space Sciences, Peking University, Beijing, China
Late Cenozoic wildfire evolution in Inner Asia has been attributed to both ice-volume modulating precipitation changes and surface uplift of the Tibetan Plateau. Whether this is the case or not requires additional research and wildfire records from older periods. In this study, 251 microcharcoal samples from the Huatugou section in the western Qaidam Basin are used to reconstruct the early Oligocene-middle Miocene wildfire history of the northern Tibetan Plateau. The results show that wildfires remained relatively frequent before ∼26 Ma, then reduced gradually until ∼14 Ma, and finally increased slightly but still at low level between 14 and 12 Ma. The wildfire variations can be correlated to the steppe-based dryness changes, and both of which are coincident with global temperature changes. We infer that mean annual temperature might have played a dominant role in controlling wildfire frequencies in the northern Tibetan Plateau through modulating atmospheric moisture content. This conclusion is in line with previous studies including microcharcoal-based wildfire records of 18–5 Ma successions from the Qaidam Basin as well as soot-based wildfire records from Quaternary glacial–interglacial cycles of the Chinese Loess Plateau.
Introduction
The Earth’s surface has experienced wildfires since the appearance of terrestrial plants around 420 million years ago (Ma) (Scott and Glasspool, 2006). Wildfire is driven by factors such as carbon-rich vegetation, seasonally dry climates, atmospheric oxygen, widespread lightning, and volcanic ignition sources (Bowman et al., 2009). Wildfire records can therefore provide useful information about the evolution of vegetation, climate, atmosphere, and human society (Dale et al., 2001; Bowman et al., 2009; Edwards et al., 2010; Burton, 2011; Miao et al., 2016b).
For example, charred grass cuticles from tropical Africa have been used as a proxy for late Cenozoic wildfires, which indicates essentially dry periods since 8 Ma (Morley and Richards, 1993). Charred grains from southern Africa have also been used as a wildfire proxy but showing that wildfire reached a maximum at 7–6 Ma ago under moderately dry conditions (Hoetzel et al., 2013). Similarly, wildfire and its potential links with monsoonal precipitation have been studied in inner Asia for the past 7 Ma (Zhou et al., 2014) or 16 Ma (Hui et al., 2021). Wildfire patterns in East Asia have changed during the last 13 Ma, possibly influenced by regional hydroclimatic changes (Shen et al., 2018). In East Asia, the high-resolution soot record shows unique and distinct glacial–interglacial cycles that are synchronous with marine δ18O records, suggesting that aridity, which was driven by global ice volume, controlled wildfire intensity during the past 2.6 Ma (Han et al., 2020). Similar correlations between global temperature and regional wildfire intensity have also been found in the northern Tibetan Plateau for the middle Miocene-early Pliocene (18–5 Ma) period, while tectonic activities of the northern Tibetan Plateau may have only played a secondary role (Miao et al., 2019).
Globally, late Cenozoic wildfire patterns varied between different places, and a diverse range of driving mechanisms have been proposed (Bond, 2015), such as precipitation amount, degree of aridity, and hydroclimatic conditions, etc., that are further driven by either solar radiation (Han et al., 2020), atmospheric circulations (Jia et al., 2003), seasonality (Shen et al., 2018), or topographic changes (Miao et al., 2019). These hypotheses are largely based on evidence from individual sites, which may not extend to other sites or different time intervals. Extending such site-specific records to regional scales will help improve our understanding of the driving forces of wildfire.
In contrast to the late Cenozoic sequences, wildfire records of earlier periods are scarce. At present, the only wildfire record that covers the whole Cenozoic period comes from the northern Pacific Ocean (Herring, 1985), and the only record from the southern hemisphere (Australia) covers the middle Eocene (Kershaw et al., 2002). Records from the South China Sea cover periods since the early Oligocene (Jia et al., 2003). However, none of these Paleogene records can be directly compared to the late Cenozoic wildfire records on land due to their far localities and wildfire proxies. In this study, we focus on sedimentary records of the microcharcoals from the Qaidam Basin to reconstruct the early Oligocene-middle Miocene wildfire history of the northern Tibetan Plateau, and then integrate this new record with our previous reconstruction from the same basin for the middle Miocene-early Pliocene (Miao et al., 2019), to assess the drivers of wildfire in this region over a long period.
Geological Setting and Sampling
Geological Setting
The Qaidam Basin, covering an area of 200,000 km2 and lying at an average elevation of 2,800 m, is the largest topographic depression on the Tibetan Plateau. The current climate in the basin is arid to hyper-arid, with a mean annual precipitation of less than 300 mm in the east, and less than 50 mm in the west. The basin locates in cold climate zone with mean annual temperatures ranging between 2°C and 4°C. The westerlies are the dominant atmospheric circulation pattern throughout the year, while the East Asian monsoon only reaches the southeastern basin during summer (Wu et al., 1980) (Figure 1A). Vegetation in the basin is dominated by desert species, which grow on gravelly lake margins and well-drained soils on alluvial fans. The main species include Amaranthaceae (Chenopodioideae), Ephedra, Nitraria, Tamarix, and Asteraceae, etc. Occasionally, Picea and Sabina, two types of woods can be observed on high mountains in the eastern basin (Miao et al., 2019).
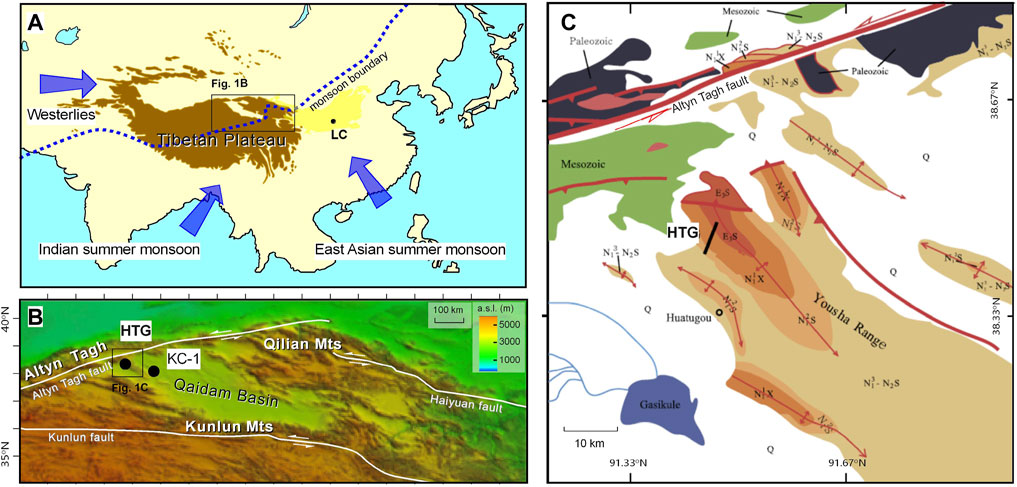
FIGURE 1. Physiographic map of inner to eastern Asia, showing the locations for the wildfire study. (A). Geographic map of the Tibetan Plateau, showing the study area and a simplified representation of atmospheric circulation. LC section in the Loess Plateau (yellow area) is for reference (Han et al., 2020). (B). Morphotectonic map of Qaidam Basin and the location, of the HTG section that was sampled in this study, and of the KC-1 core (Miao et al., 2019). (C). Geological map of the Youshashan Range in the western Qaidam Basin. E3S: Shangganchaigou Formation, N11X: Xiayoushashan Formation, N21S: Shangyoushashan Formation, N31–N2S: Shizigou Formation, redrawn from (Chang et al., 2015).
Geomorphologically, the Qaidam Basin is bounded by the Altyn Tagh to the northwest, the East Kunlun Shan to the south, and the Qilian Shan to the northeast (Figure 1B). Tectonically, the basin is bounded by the Altyn Tagh fault, Kunlun fault, and North Qaidam-Qilian Shan thrust belts (Song and Wang, 1993; Yin et al., 2007). Cenozoic stratigraphic units in the basin were deposited mainly in fluvial-lacustrine environments. Although disputes exist concerning the stratigraphic ages, there is a consensus that the depositional center of the basin has shifted southeastward along the axis of the basin from the early Cenozoic to the present (Song and Wang, 1993; Métivier et al., 1998; Wang et al., 2006; Yin et al., 2007; Cheng et al., 2014). Provenance analysis indicates that the Cenozoic deposits in the Qaidam basin were mainly sourced from surrounding mountains (Rieser et al., 2005; Jian et al., 2013; Cheng et al., 2016; Wang et al., 2017; Lu et al., 2019; Nie et al., 2020; Cheng et al., 2021).
Study Site and Age Constraints
The Huatugou (HTG) section (bottom: 38.43°N, 90.89°E; top: 38.36°N, 90.88°E) lies at the southwestern side of the Youshashan anticline in the western Qaidam Basin (Figure 1C) and is about 4,360 m thick (Rieser et al., 2005; Song and Wang, 1993). Strata in the section include the early Oligocene to late Miocene Shangganchaigou, Xiayoushashan, and Shangyoushashan formations. Detailed lithofacies analysis indicates that the strata between 200 and 2,700 m are dominated by marginal lacustrine facies, while the parts below ∼200 m and above ∼3,500 m are dominated by braided river facies (Li et al., 2016) (Figure 2). Magnetostratigraphic study of the HTG section indicates that the strata between 1,200 m and 4,100 m can be confidently determined to be 20.7–11.6 Ma, while there are two alternative age correlations for the lower (0–1,200 m) section: either ∼28–20.7 Ma or ∼31–20.7 Ma (Chang et al., 2015).
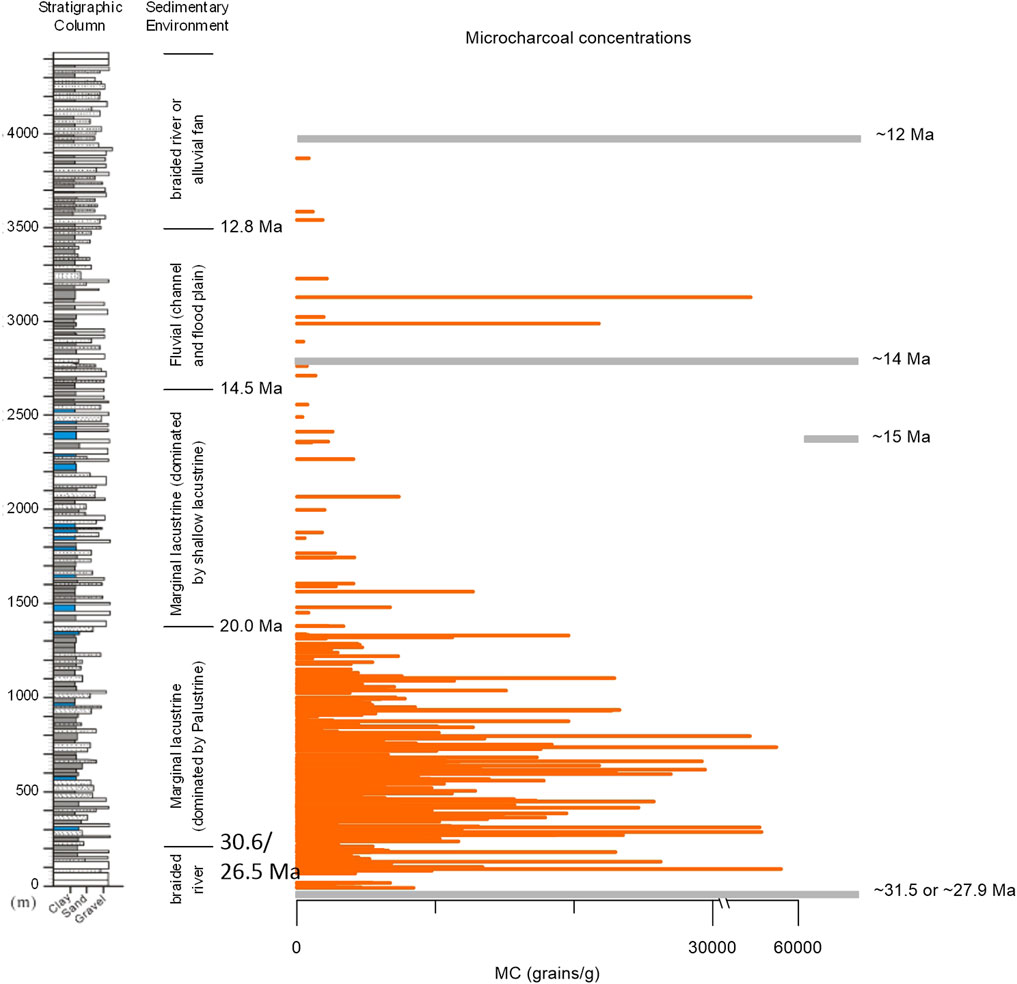
FIGURE 2. Lithology, sedimentary facies with age between boundary, and microcharcoal results from the HTG section. Age estimates (Chang et al., 2015) and sedimentary facies (Li et al., 2016). MC: total microcharcoal concentrations. Dashed arrows show the dominant MC trends.
Methods and Results
Microcharcoal Extraction and Identification
A total of 251 samples of mudstone, sandy mudstone, and siltstone were collected for microcharcoal analysis. Over two-thirds samples were from the lower part of the section (older than 25 Ma), while 28 samples are from the upper section (younger than 20 Ma) due to the lack of suitable sampling lays because of the coarse grain size (Figure 2). All samples were pretreated using the following procedures. After gentle grinding to fine powders, two different types of acid were added successively, 10% HCl to remove carbonates completely (usually 2–3 days) and then 40% HF to remove silicates; after which the remaining powders went through 10 μm sieve again to enrich microcharcoal grains. Then, the pretreated specimens were mounted in glycerol for identification (Miao et al., 2019). Samples were counted under a light microscope at 400 × magnification with regularly spaced traverses. Microcharcoal grains were counted and photographed under the microscope. All samples were analyzed at the Northwest Institute of Eco-Environment and Resources, Chinese Academy of Sciences. A known number of Lycopodium clavatum spores (batch # 27,600) were initially added to each sample to facilitate the calculation of microcharcoal concentrations (MC). The MC is calculated as:
N, identified number of microcharcoals (grains); L, number of Lycopodium clavatum (grains); W, sample dry weight (g).
Microcharcoal Results
The results show that in the HTG section the MC varied between 410 and 54,650 grains/g with an average of 9,720 grains/g. In general, the MC keeps at high values from the bottom of the section to 800 m (corresponding to ∼31.0–26.0 Ma or 27.0–23.5 Ma according to the two different age models), with the MC varies between 720 and 54,650 grains/g (average of 11,240 grains/g) in 146 samples. Then the MC experiences a continuous decreasing trend until ∼2,800 m (∼14 Ma), with values ranging between 410 and 45,060 grains/g (average 5,620 grains/g) in 98 samples. Except for two samples that have high MC values of 21,470 and 45,270 grains/g at 3,001 m and 3,141 m, respectively. The MC remains low in the upper section but slightly higher than that at ∼14 Ma, with values ranging between 480 and 2,170 grains/g (average 1,410 grains/g) in six samples.
Discussion
Data Evaluation and Wildfire Trends
The relationship between microcharcoal concentration and wildfire is intuitive: higher MC corresponds to wildfires with higher frequency or intensity, and vice-versa (Herring, 1985; Patterson et al., 1987; Ward and Hardy, 1991; Whitlock and Larsen, 2002; Miao et al., 2016a; Miao et al., 2019). This interpretation is based on the assumption that sediment provenance is stable and that sedimentary processes have little influence on microcharcoal transport and accumulation, or at least that any influence is constant (Patterson et al., 1987; Anne-Laure et al., 2013).
Three lines of evidence indicate that the MC variations in the HTG section are not controlled by provenance or sedimentary processes. Firstly, provenance studies of sandstones and mudstones collected in the western Qaidam Basin show negligible (Rieser et al., 2005) or a small variation (Cheng et al., 2019) during the Oligocene to the middle Miocene. Secondly, the sediment transport dynamics also have no influence on MC. As shown in Figure 3, no correlations were found between MC and lithofacies, e.g., mudstone, sandy mudstone, and siltstone, which reflect different transport dynamics. Thirdly, magnetostratigraphic analyses indicate that the accumulation rate increased abruptly around 15 Ma (from 180 m/Ma or 130 m/Ma to ∼500 m/Ma) (Chang et al., 2015), but no abnormal changes in MC occurred around 15 Ma (Figure 4). In contrast, the most significant MC change occurred within the fluvial environment at ∼13.5 Ma.
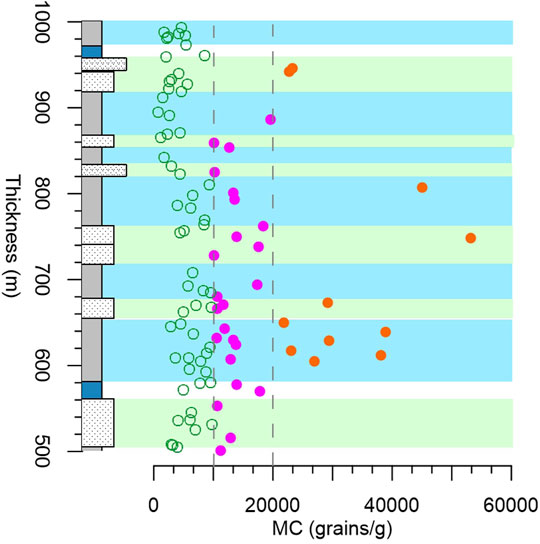
FIGURE 3. Correlations between lithological stratigraphy and MC at 500–1,000 m of the HTG section. No regular MC change with the lithology, the high values can exist in either mudstone or sandstone.
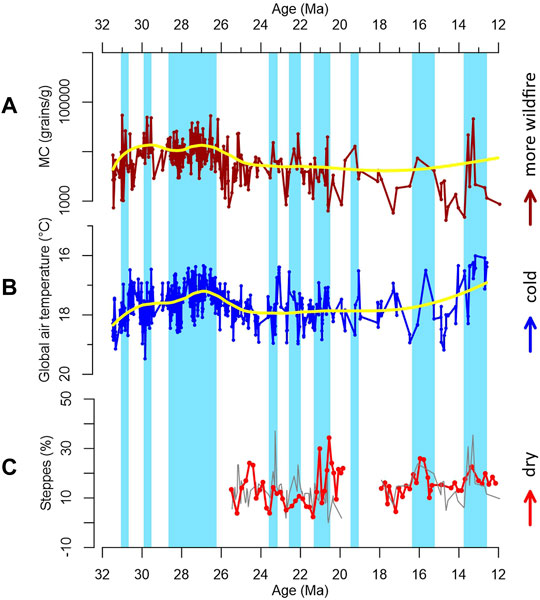
FIGURE 4. Correlations between wildfire to aridity and temperature. (A). MC records according to older age model. (B). global temperature curve (Westerhold et al., 2020) chosen according to the MC old model. (C). aridity records basing on steppes in pollen assemblages during ∼26–20 Ma (Sun et al., 2014) and 18–5 Ma (Miao et al., 2011). Gray curve is a copy of the (A) to couple to these two intervals and sample frequency in Lunpola is three. The vertical light blue rectangles showed the positive correlations. Yellowish solid line shows the LOESS Fit with span: 0.5.
Compared with the other depositional environments, e.g., shallow lacustrine and fluvial, the interval of palustrine environment shows a much higher MC (Figure 2). This is reasonable as palustrine is a nearly still water environment compared with the running water conditions in the other depositional environments, and thus would accumulate more microcharcoals for the same period. However, the MC variations within the palustrine environment (both the short-term large variations and the long-term general variations) and the very high MC in the braided river environments indicate that, excluding the influence of different depositional environments, the long-term general variations still reflect wildfire intensities and frequencies.
Based on the above arguments, we conclude that the MC in the HTG section can be used to reconstruct ancient wildfire activities in the northern Tibetan Plateau. The results indicate that the wildfire experienced a higher frequency and/or intensity during the Oligocene (e.g., before ∼26 or ∼23 Ma), then decreased gradually to stay at relatively lower levels since after and last until the middle Miocene (In this study, we prefer the later age model, see Supplementary Materials, Figure 4A).
Wildfire Mechanisms
Pollen records would have been ideal for reconstruction of the aridification history of the Qaidam Basin (Cai et al., 2012; Koutsodendris et al., 2019; Miao et al., 2012; Wang et al., 1999; Wu et al., 2011), which could then be compared to the MC records (Miao et al., 2019). However, no continuous pollen records have been successfully retrieved from the HTG section or any sections within or adjacent to the Qaidam Basin with reliable age constraints. Here we compare our MC data with global mean air temperature change reconstructed from benthic δ18O changes (Westerhold et al., 2020) (Figure 4B) and two intervals of xerophytic pollen records from the Lunpola Basin (25.5–19.8 Ma) (Sun et al., 2014) and Qaidam Basin (18–12 Ma) (Miao et al., 2011) (Figure 4C).
It is observed that during stages of high amounts of steppes (such as Ephedra, Nitraria and Chenopodiaceae, etc.), the MC is also high and vice versa. This correlation indicates potential links between strong wildfire and dry conditions (low precipitation). In fact, such a correlation has also been found in other parts of East Asia. For example, high-resolution soot records from the Loess Plateau to the east, have been correlated to glacial–interglacial cycles of monsoonal precipitation during the past 2.6 Ma (Han et al., 2020) (Figure 5B): stronger wildfires always correlate with less precipitation during glacial periods. Similarly, two separate Miocene (e.g., 18–5 Ma) microcharcoal–based wildfire records from the Qaidam Basin also agree with enhanced aridification under a global cooling trend (Miao et al., 2019) (Figures 5A,C). All these records support the hypothesis that dryness (less precipitation) plays a crucial role in driving wildfires, such that dryness (less precipitation) facilitates dehydration of vegetation, which becomes more flammable (Pechony and Shindell, 2010; Jolly et al., 2015).
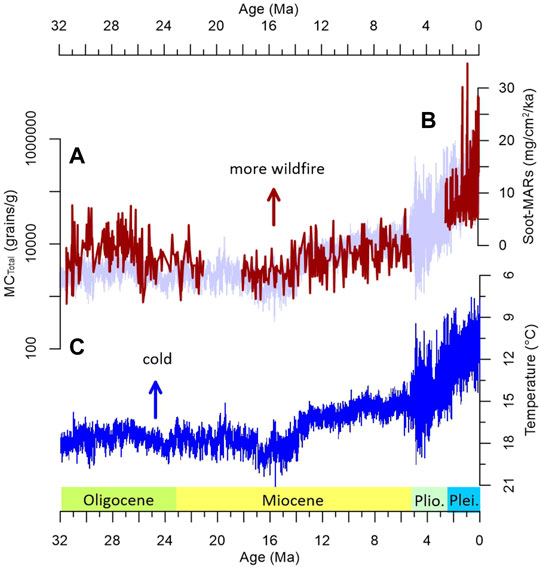
FIGURE 5. Correlations between wildfire in Inner Asia and global temperature. (A). the MC records from the HTG section (this study) and KC-1 core (18–5 Ma) from the Qaidam Basin (Miao et al., 2019). (B). Quaternary fires indicated by soot-MARs reconstructed from Chinese Loess Plateau (Han et al., 2020). (C). global temperature curve (Westerhold et al., 2020).
Furthermore, positive correlations are also observed between the MC records and global temperature changes, which further support the hypothesis that stronger wildfires always occur during periods of less precipitation (dry conditions). Lower global temperature helps to reduce the amount of vapors evaporated from oceans and other water bodies, which would then reduce the precipitation amounts in continental interiors that would lead to higher fire frequency. Further detailed explanations are as follows: according to Avogadro’s Law, temperature determines the maximum concentration of water vapor at sea level and standard air pressure. As temperature rises, the water vapor concentration increases significantly (Machine Applications Corporation, 1999). The higher the temperature is, the more water vapor is pumped into the atmosphere, leading to increased precipitation on land (Cai et al., 2012; Held and Soden, 2006; Miao et al., 2017; Ruddiman, 2008; Singh, 1988). For example, the annual precipitation data obtained from passive microwave radiometry are correlated well with sea surface temperatures (Stephens, 1990). Modeling studies have also shown that when annual temperatures increase by 2.0°C, the annual precipitation amount in the monsoonal Tibetan Plateau would increase by ∼3.9%; while in the westerlies dominated region of the Tibetan Plateau, precipitation only increases by 0.8% (Wang et al., 2021). Over large areas such as the Asian-Australian monsoon regions, the total precipitation is likely to increase significantly (by 4.5% per °C) under the representative concentration pathways (RCP) scenarios of 4.5 anthropogenic warming scenario (Wang et al., 2014).
The positive correlations between global temperatures and evaporation rates have been observed and supported by previous studies (Machine Applications Corporation, 1999). We, therefore, consider that global temperature might have played the primary role to influence precipitation amount changes, which further drives wildfire evolution in Inner Asia.
As in Introduction, the surface uplift the Tibetan Plateau has been regarded as a dominant agent in the local and regional paleoclimate, e.g., breeding the Asian summer monsoon (wetting the East Asia) and bifurcating the westerlies, which might have influenced the wildfire evolution. However, the onset of the surface uplift of the Tibetan Plateau has been hotly debated, such as early Cenozoic (e.g., Ding et al., 2017; Wang et al., 2014; Su et al., 2019; Wang et al., 2008) versus late Cenozoic (e.g., Coleman and Hodges, 1995; An et al., 2001; Spicer et al., 2003; Deng and Ding, 2015; Sun et al., 2014), which has directly affected our judgment of the uplift of the Tibetan Plateau on the wildfire.
Conclusion
In this study, we present a new microcharcoal dataset of 251 samples from the early Oligocene-late Miocene section in the western Qaidam Basin, northern Tibetan Plateau. We argue that the microcharcoal concentration is minimally controlled by sedimentation processes, and thus can be used to reconstruct wildfire activities in the northern Tibetan Plateau. The data indicate high frequency and/or intensity of wildfire during the Oligocene (before ∼26), then decreased gradually to stay at relatively low levels until ∼12 Ma. Comparisons between dryness records and global temperature change indicate that high levels of wildfire are well linked to colder and drier periods. We infer that wildfire is strongly influenced by temperature changes through influencing the atmospheric moisture content that are evaporated from ocean surface and other water bodies. Such correlations have also been observed in the middle to late Miocene microcharcoal records in the Qaidam Basin and soot-based Quaternary glacial-interglacial cycles in the Loess Plateau.
Data Availability Statement
The original contributions presented in the study are included in the article/Supplementary Material, further inquiries can be directed to the corresponding author.
Author Contributions
YM collected samples and designed the experiment. YM and HC analyzed the data. All authors wrote and reviewed the manuscript.
Funding
The project was supported by the Strategic Priority Research Program of CAS (Nos. XDA20070200 and XDB40010100), NSFC (Nos. 41888101, 42161144012, 41772181, and 41290252); Young Top Talents Project of the “Ten Thousand Youth Program” of the Organization Department of the Central Committee of the CPC; Youth Innovation Promotion Association, CAS (No. 2014383), and “Light of West China” Program, CAS.
Conflict of Interest
The authors declare that the research was conducted in the absence of any commercial or financial relationships that could be construed as a potential conflict of interest.
Publisher’s Note
All claims expressed in this article are solely those of the authors and do not necessarily represent those of their affiliated organizations, or those of the publisher, the editors and the reviewers. Any product that may be evaluated in this article, or claim that may be made by its manufacturer, is not guaranteed or endorsed by the publisher.
Acknowledgments
We are grateful to Yindi Duan, Xicai Zheng, Hongjie Yan and Fuzheng Zhang, who helped in the lab, and also to the reviewers for their constructive suggestions.
Supplementary Material
The Supplementary Material for this article can be found online at: https://www.frontiersin.org/articles/10.3389/feart.2022.850809/full#supplementary-material
Supplementary Figure S1 | Correlations between wildfire obtained in the HTG section and global air temperature at the bottom (<1750 m) of the section by two age models. (A,B), the MC curve by older age model and comparison with the global air temperature curve (Westerhold et al., 2020). (C,D), the MC curve by younger age model and comparison with the global air temperature curve (Westerhold et al., 2020). The temperature curves have been also shown as gray shading in A and C for comparison. Shallow blue rectangles show the unmatched correlations and thick solid blue lines show the LOESS Fit with span: 0.5.
References
An, Z., Kutzbach, J. E., Prell, W. L., and Porter, S. C. (2001). Evolution of Asian monsoons and Phased Uplift of the Himalaya-Tibetan Plateau Since Late Miocene Times. Nature 441, 62–66. doi:10.1038/35075035
Anne-Laure, D., Maria Fernanda, S. G. I., Philippe, M., Urrego, D. H., Viviane, B. R., Stéphanie, D., et al. (2013). Orbital-scale Climate Forcing of Grassland Burning in Southern Africa. Proc. Natl. Acad. Sci. 110, 5069–5073. doi:10.1073/pnas.1214292110
Bond, W. J. (2015). Fires in the Cenozoic: a Late Flowering of Flammable Ecosystems. Front. Plant Sci. 5, 749. doi:10.3389/fpls.2014.00749
Bowman, D. M. J. S., Balch, J. K., Artaxo, P., Bond, W. J., Carlson, J. M., Cochrane, M. A., et al. (2009). Fire in the Earth System. science 324, 481–484. doi:10.1126/science.1163886
Cai, M., Fang, X., Wu, F., Miao, Y., and Appel, E. (2012). Pliocene-Pleistocene Stepwise Drying of Central Asia: Evidence from Paleomagnetism and Sporopollen Record of the Deep Borehole SG-3 in the Western Qaidam Basin, NE Tibetan Plateau. Glob. Planet. Change 94-95, 72–81. doi:10.1016/j.gloplacha.2012.07.002
Chang, H., Li, L., Qiang, X., Garzione, C. N., Pullen, A., and An, Z. (2015). Magnetostratigraphy of Cenozoic Deposits in the Western Qaidam Basin and its Implication for the Surface Uplift of the Northeastern Margin of the Tibetan Plateau. Earth Planet. Sci. Lett. 430, 271–283. doi:10.1016/j.epsl.2015.08.029
Cheng, F., Jolivet, M., Fu, S., Zhang, Q., Guan, S., Yu, X., et al. (2014). Northward Growth of the Qimen Tagh Range: A New Model Accounting for the Late Neogene Strike-Slip Deformation of the SW Qaidam Basin. Tectonophysics 632, 32–47. doi:10.1016/j.tecto.2014.05.034
Cheng, F., Fu, S., Jolivet, M., Zhang, C., and Guo, Z. (2016). Source to Sink Relation between the Eastern Kunlun Range and the Qaidam Basin, Northern Tibetan Plateau, during the Cenozoic. Bulletin 128, 258–283. doi:10.1130/b31260.1
Cheng, F., Garzione, C. N., Mitra, G., Jolivet, M., Guo, Z., Lu, H., et al. (2019). The Interplay between Climate and Tectonics during the Upward and Outward Growth of the Qilian Shan Orogenic Wedge, Northern Tibetan Plateau. Earth-Science Rev. 198, 102945. doi:10.1016/j.earscirev.2019.102945
Cheng, F., Jolivet, M., Guo, Z., Wang, L., Zhang, C., and Li, X. (2021). Cenozoic Evolution of the Qaidam basin and Implications for the Growth of the Northern Tibetan Plateau: A Review. Earth-Science Rev. 220, 103730. doi:10.1016/j.earscirev.2021.103730
Coleman, M., and Hodges, K. (1995). Evidence for Tibetan Plateau Uplift Before 14 Myr Ago from a New Minimumage for East-west Extension. Nature 374, 49–52. doi:10.1038/374049a0
Dale, V. H., Joyce, L. A., McNulty, S., Neilson, R. P., Ayres, M. P., Flannigan, M. D., et al. (2001). Climate Change and Forest Disturbances. BioScience 51, 723–734. doi:10.1641/0006-3568(2001)051[0723:ccafd]2.0.co;2
Deng, T., and Ding, L. (2015). Paleoaltimetry Reconstructions of the Tibetan Plateau: Progress and Contradictions. Natl. Sci. Rev. 2, 417–437. doi:10.1093/nsr/nwv062
Ding, L., Spicer, R. A., Yang, J., Xu, Q., Cai, F., Li, S., et al. (2017). Quantifying the Rise of the Himalaya Orogen and Implications for the South Asian Monsoon. Geology 45, 215–218. doi:10.1130/G38583.1
Edwards, E. J., Osborne, C. P., Strömberg, C. A. E., Smith, S. A., Bond, W. J., Christin, P.-A., et al. (2010). The Origins of C 4 Grasslands: Integrating Evolutionary and Ecosystem Science. science 328, 587–591. doi:10.1126/science.1177216
Han, Y., An, Z., Marlon, J. R., Bradley, R. S., Zhan, C., Arimoto, R., et al. (2020). Asian Inland Wildfires Driven by Glacial–Interglacial Climate Change. Proc. Natl. Acad. Sci. 117, 5184–5189. doi:10.1073/pnas.1822035117
Held, I. M., and Soden, B. J. (2006). Robust Responses of the Hydrological Cycle to Global Warming. J. Clim. 19, 5686–5699. doi:10.1175/jcli3990.1
Herring, J. R. (1985). Charcoal Fluxes into Sediments of the North Pacific Ocean: the Cenozoic Record of Burning. The carbon Cycle Atmos. CO2: Nat. variations Archean present 32, 419–442. doi:10.1029/gm032p0419
Hoetzel, S., Dupont, L., Schefuß, E., Rommerskirchen, F., and Wefer, G. (2013). The Role of Fire in Miocene to Pliocene C4 Grassland and Ecosystem Evolution. Nat. Geosci 6, 1027–1030. doi:10.1038/ngeo1984
Hui, Z., Gowan, E. J., Hou, Z., Zhou, X., Ma, Y., Guo, Z., et al. (2021). Intensified Fire Activity Induced by Aridification Facilitated Late Miocene C4 Plant Expansion in the Northeastern Tibetan Plateau, China. Palaeogeogr. Palaeoclimatol. Palaeoecol. 573, 110437. doi:10.1016/j.palaeo.2021.110437
Jia, G., Peng, P. a., Zhao, Q., and Jian, Z. (2003). Changes in Terrestrial Ecosystem since 30 Ma in East Asia: Stable Isotope Evidence from Black Carbon in the South China Sea. Geol 31, 1093–1096. doi:10.1130/g19992.1
Jian, X., Guan, P., Zhang, D.-W., Zhang, W., Feng, F., Liu, R.-J., et al. (2013). Provenance of Tertiary sandstone in the Northern Qaidam basin, Northeastern Tibetan Plateau: Integration of Framework Petrography, Heavy mineral Analysis and mineral Chemistry. Sediment. Geology. 290, 109–125. doi:10.1016/j.sedgeo.2013.03.010
Jolly, W. M., Cochrane, M. A., Freeborn, P. H., Holden, Z. A., Brown, T. J., Williamson, G. J., et al. (2015). Climate-induced Variations in Global Wildfire Danger from 1979 to 2013. Nat. Commun. 6, 7537. doi:10.1038/ncomms8537
Kershaw, A. P., Clark, J. S., Gill, A. M., and D’Costa, D. (2002). “A History of Fire in Australia,” in Flammable Australia: The Fire Regimes and Biodiversity of a Continent. Editors R. A. Bradstock, J. E. Williams, and A. M. Gill (Cambridge: Cambridge University Press), 3–25.
Koutsodendris, A., Allstädt, F. J., Kern, O. A., Kousis, I., Schwarz, F., Vannacci, M., et al. (2019). Late Pliocene Vegetation Turnover on the NE Tibetan Plateau (Central Asia) Triggered by Early Northern Hemisphere Glaciation. Glob. Planet. Change 180, 117–125. doi:10.1016/j.gloplacha.2019.06.001
Li, L., Garzione, C. N., Pullen, A., and Chang, H. (2016). Early-middle Miocene Topographic Growth of the Northern Tibetan Plateau: Stable Isotope and Sedimentation Evidence from the Southwestern Qaidam basin. Palaeogeogr. Palaeoclimatol. Palaeoecol. 461, 201–213. doi:10.1016/j.palaeo.2016.08.025
Lu, H., Ye, J., Guo, L., Pan, J., Xiong, S., and Li, H. (2019). Towards a Clarification of the Provenance of Cenozoic Sediments in the Northern Qaidam Basin. Lithosphere 11, 252–272. doi:10.1130/l1037.1
Machine Applications Corporation (1999). The MAC Humidity/Moisture Handbook. Sandusky, OH: Machine Instruments Corporation, 1–20.
Métivier, F., Gaudemer, Y., Tapponnier, P., and Meyer, B. (1998). Northeastward Growth of the Tibet Plateau Deduced from Balanced Reconstruction of Two Depositional Areas: The Qaidam and Hexi Corridor Basins, China. Tectonics 17, 823–842.
Miao, Y., Fang, X., Herrmann, M., Wu, F., Zhang, Y., and Liu, D. (2011). Miocene Pollen Record of KC-1 Core in the Qaidam Basin, NE Tibetan Plateau and Implications for Evolution of the East Asian Monsoon. Palaeogeogr. Palaeoclimatol. Palaeoecol. 299, 30–38. doi:10.1016/j.palaeo.2010.10.026
Miao, Y., Herrmann, M., Wu, F., Yan, X., and Yang, S. (2012). What Controlled Mid-late Miocene Long-Term Aridification in Central Asia? - Global Cooling or Tibetan Plateau Uplift: A Review. Earth-Science Rev. 112, 155–172. doi:10.1016/j.earscirev.2012.02.003
Miao, Y., Fang, X., Song, C., Yan, X., Zhang, P., Meng, Q., et al. (2016a). Late Cenozoic Fire Enhancement Response to Aridification in Mid-latitude Asia: Evidence from Microcharcoal Records. Quat. Sci. Rev. 139, 53–66. doi:10.1016/j.quascirev.2016.02.030
Miao, Y., Wu, F., Chang, H., Fang, X., Deng, T., Sun, J., et al. (2016b). A Late-Eocene Palynological Record from the Hoh Xil Basin, Northern Tibetan Plateau, and its Implications for Stratigraphic Age, Paleoclimate and Paleoelevation. Gondwana Res. 31, 241–252. doi:10.1016/j.gr.2015.01.007
Miao, Y., Warny, S., Clift, P. D., Liu, C., and Gregory, M. (2017). Evidence of Continuous Asian Summer Monsoon Weakening as a Response to Global Cooling over the Last 8 Ma. Gondwana Res. 52, 48–58. doi:10.1016/j.gr.2017.09.003
Miao, Y., Wu, F., Warny, S., Fang, X., Lu, H., Fu, B., et al. (2019). Miocene Fire Intensification Linked to Continuous Aridification on the Tibetan Plateau. Geology 47, 303–307. doi:10.1130/g45720.1
Morley, R. J., and Richards, K. (1993). Gramineae Cuticle: a Key Indicator of Late Cenozoic Climatic Change in the Niger Delta. Rev. palaeobotany palynology 77, 119–127. doi:10.1016/0034-6667(93)90060-8
Nie, J., Ren, X., Saylor, J. E., Su, Q., Horton, B. K., Bush, M. A., et al. (2020). Magnetic Polarity Stratigraphy, Provenance, and Paleoclimate Analysis of Cenozoic Strata in the Qaidam Basin, NE Tibetan Plateau. GSA Bull. 132, 310–320. doi:10.1130/b35175.1
Patterson, W. A., Edwards, K. J., and Maguire, D. J. (1987). Microscopic Charcoal as a Fossil Indicator of Fire. Quat. Sci. Rev. 6, 3–23. doi:10.1016/0277-3791(87)90012-6
Pechony, O., and Shindell, D. T. (2010). Driving Forces of Global Wildfires over the Past Millennium and the Forthcoming century. Proc. Natl. Acad. Sci. 107, 19167–19170. doi:10.1073/pnas.1003669107
Rieser, A. B., Neubauer, F., Liu, Y., and Ge, X. (2005). Sandstone Provenance of north-western Sectors of the Intracontinental Cenozoic Qaidam basin, Western China: Tectonic vs. Climatic Control. Sediment. Geology. 177, 1–18. doi:10.1016/j.sedgeo.2005.01.012
Scott, A. C., and Glasspool, I. J. (2006). The Diversification of Paleozoic Fire Systems and Fluctuations in Atmospheric Oxygen Concentration. Proc. Natl. Acad. Sci. 103, 10861–10865. doi:10.1073/pnas.0604090103
Shen, X., Wan, S., Colin, C., Tada, R., Shi, X., Pei, W., et al. (2018). Increased Seasonality and Aridity Drove the C4 Plant Expansion in Central Asia since the Miocene-Pliocene Boundary. Earth Planet. Sci. Lett. 502, 74–83. doi:10.1016/j.epsl.2018.08.056
Singh, G. (1988). History of Aridland Vegetation and Climate: a Global Perspective. Biol. Rev. 63, 159–195. doi:10.1111/j.1469-185x.1988.tb00629.x
Spicer, R. A., Harris, N. B., Widdowson, M., Herman, A. B., Guo, S., Valdes, P. J., et al. (2003). Constant Elevation of Southern Tibet Over the Past 15 Million Years. Nature 421, 622–624. doi:10.1038/nature01356
Song, T., and Wang, X. (1993). Structural Styles and Stratigraphic Patterns of Syndepositional Faults in a Contractional Setting: Examples from Quaidam Basin, Northwestern China. AAPG Bull. 77, 102–117. doi:10.1306/bdff8b78-1718-11d7-8645000102c1865d
Stephens, G. L. (1990). On the Relationship between Water Vapor over the Oceans and Sea Surface Temperature. J. Clim. 3, 634–645. doi:10.1175/1520-0442(1990)003<0634:otrbwv>2.0.co;2
Sun, J., Xu, Q., Liu, W., Zhang, Z., Xue, L., and Zhao, P. (2014). Palynological Evidence for the Latest Oligocene−early Miocene Paleoelevation Estimate in the Lunpola Basin, central Tibet. Palaeogeogr. Palaeoclimatol. Palaeoecol. 399, 21–30. doi:10.1016/j.palaeo.2014.02.004
Su, T., Farnsworth, A., Spicer, R., Huang, J., Wu, F., Liu, J., et al. (2019). No High Tibetan Plateau Until the Neogene. Sci. Adv. 5, eaav2189. doi:10.1126/sciadv.aav2189
Wang, J., Wang, Y. J., Liu, Z. C., Li, J. Q., and Xi, P. (1999). Cenozoic Environmental Evolution of the Qaidam Basin and its Implications for the Uplift of the Tibetan Plateau and the Drying of central Asia. Palaeogeogr. Palaeoclimatol. Palaeoecol. 152, 37–47. doi:10.1016/s0031-0182(99)00038-3
Wang, E., Xu, F.-Y., Zhou, J.-X., Wan, J., and Burchfiel, B. C. (2006). Eastward Migration of the Qaidam basin and its Implications for Cenozoic Evolution of the Altyn Tagh Fault and Associated River Systems. Geol. Soc. America Bull. 118, 349–365. doi:10.1130/b25778.1
Wang, C., Zhao, X., Liu, Z., Lippert, P. C., Graham, S. A., Coe, R. S., et al. (2008). Constraints on the Early Uplift History of the Tibetan Plateau. Proc. Natl. Acad. Sci. 105, 4987–4992. doi:10.1073/pnas.0703595105
Wang, B., Yim, S.-Y., Lee, J.-Y., Liu, J., and Ha, K.-J. (2014). Future Change of Asian-Australian Monsoon under RCP 4.5 Anthropogenic Warming Scenario. Clim. Dyn. 42, 83–100. doi:10.1007/s00382-013-1769-x
Wang, T., Zheng, W., Zhang, P., Li, Q., Kirby, E., Yuan, D., et al. (2017). Expansion of the Tibetan Plateau during the Neogene. Nat. Commun. 8, 15887. doi:10.1038/ncomms15887
Wang, T., Zhao, Y., Xu, C., Ciais, P., Liu, D., Yang, H., et al. (2021). Atmospheric Dynamic Constraints on Tibetan Plateau Freshwater under Paris Climate Targets. Nat. Clim. Chang. 11, 219–225. doi:10.1038/s41558-020-00974-8
Ward, D. E., and Hardy, C. C. (1991). Smoke Emissions from Wildland Fires. Environ. Int. 17, 117–134. doi:10.1016/0160-4120(91)90095-8
Westerhold, T., Marwan, N., Drury, A. J., Liebrand, D., Agnini, C., Anagnostou, E., et al. (2020). An Astronomically Dated Record of Earth's Climate and its Predictability over the Last 66 Million Years. Science 369, 1383–1387. doi:10.1126/science.aba6853
Whitlock, C., and Larsen, C. (2002). “Charcoal as a Fire Proxy,” in Tracking Environmental Change Using lake Sediments (Dordrecht: Springer), 75–97. doi:10.1007/0-306-47668-1_5
Wu, F., Fang, X., Herrmann, M., Mosbrugger, V., and Miao, Y. (2011). Extended Drought in the interior of Central Asia since the Pliocene Reconstructed from Sporopollen Records. Glob. Planet. Change 76, 16–21. doi:10.1016/j.gloplacha.2010.11.007
Yin, A., Dang, Y., Zhang, M., McRivette, M. W., Burgess, W. P., and Chen, X. (2007). Cenozoic Tectonic Evolution of Qaidam basin and its Surrounding Regions (Part 2): Wedge Tectonics in Southern Qaidam basin and the Eastern Kunlun Range. Geol. Soc. America Spec. Pap. 433, 369–390. doi:10.1130/2007.2433(18)
Keywords: wildfire, global temperature, vegetation, moisture, Tibetan plateau
Citation: Miao Y, Chang H, Li L, Cheng F, Garzione C and Yang Y (2022) Early Oligocene—Late Miocene Wildfire History in the Northern Tibetan Plateau and Links to Temperature-Driven Precipitation Changes. Front. Earth Sci. 10:850809. doi: 10.3389/feart.2022.850809
Received: 08 January 2022; Accepted: 21 February 2022;
Published: 15 March 2022.
Edited by:
Dabang Jiang, Institute of Atmospheric Physics (CAS), ChinaReviewed by:
Ran Zhang, Institute of Atmospheric Physics (CAS), ChinaRui Zhang, Northwest University, China
Copyright © 2022 Miao, Chang, Li, Cheng, Garzione and Yang. This is an open-access article distributed under the terms of the Creative Commons Attribution License (CC BY). The use, distribution or reproduction in other forums is permitted, provided the original author(s) and the copyright owner(s) are credited and that the original publication in this journal is cited, in accordance with accepted academic practice. No use, distribution or reproduction is permitted which does not comply with these terms.
*Correspondence: Hong Chang, Y2hhbmdoQGxvZXNzLmxscWcuYWMuY24=